- 1State Key Laboratory of Freshwater Ecology and Biotechnology, Institute of Hydrobiology, Chinese Academy of Sciences, Wuhan, China
- 2School of Life Sciences, Institute of Ecology and Biodiversity, Shandong University, Qingdao, China
- 3School of Ecology and Environmental Science, Institute for Ecological Research and Pollution Control of Plateau Lakes, Yunnan University, Kunming, China
- 4College of Fisheries and Life Science, Dalian Ocean University, Dalian, China
- 5School of Marine Sciences, Ningbo University, Ningbo, China
- 6Australian Rivers Institute, Griffith University, Nathan, QLD, Australia
- 7Department of Ecoscience, WATEC, Aarhus University, Aarhus, Denmark
- 8Sino-Danish Center for Education and Research, Beijing, China
- 9Limnology Laboratory, Department of Biological Sciences, Middle East Technical University, Ankara, Turkey
- 10Centre for Ecosystem Research and Implementation, Middle East Technical University, Ankara, Turkey
- 11Institute of Marine Sciences, Middle East Technical University, Erdemli, Turkey
Ammonium (NH4-N) produces a paradoxical effect on submersed macrophytes because it is not only the preferred nitrogen source for the growth of plants but also threatens the growth of plants at high concentration. Whether short-term and small-scale physiological toxicity experiments at an individual level can reflect the effects of high ammonium on populations of submersed macrophytes in natural conditions is still unclear. In this study, an 18-month experiment was conducted in six 600 m2 ponds subjected to different levels of ammonium loading. The effects of high ammonium on populations of canopy-forming Myriophyllum spicatum and rosette-forming Vallisneria natans were explored. The results showed that M. spicatum and V. natans populations can develop high cover and height at high ammonium concentration (7 mg/L) at short-term exposures, and V. natans may be tolerant to 18 mg/L ammonium concentration. However, the cover of M. spicatum and the height of both species were inhibited at 2.4 mg/L at long-term exposures. The height of M. spicatum was two to six times higher than that of V. natans across all treatments and control by the end of the experiment, and the cover of M. spicatum was 7–11 times higher than that of V. natans in most NH4-N loading treatments, except the cover of M. spicatum in the highest NH4-N loading treatment with 18 mg/L NH4-N. The rosette-forming V. natans resists ammonium stress by slow growth (shoot elongation) to reduce consumption, while canopy-forming species resist ammonium stress by shoot elongation and canopy development to capture light. Although increasing ammonium concentration may induce severe stress on M. spicatum, the morphological characteristics of this species may, to some extent, release the plants from this stress. Our present study indicates that the negative effects of ammonium stress on the development of populations increased with exposure duration, and the submersed macrophyte community with stronger ability for light capture and dispersal may resist high ammonium stress. Nevertheless, in strongly ammonium-enriched systems, competition and succession cannot be neglected.
Introduction
Due to their complex external morphology, submersed macrophytes create habitat heterogeneity in aquatic ecosystems, and therefore play an important role in the structure and function of shallow lakes (Jeppesen et al., 1998; Su et al., 2019). However, submersed macrophytes are experiencing a global decline in shallow lakes, resulting in eventually a shift from a macrophyte-dominated healthy ecosystem state to a phytoplankton-dominated state (Scheffer et al., 1993; Chao et al., 2022) and the deterioration of various functions of aquatic ecosystems (Sayer et al., 2010; Zhang et al., 2017). Therefore, to explore the responses of submersed macrophytes to the stressors is necessary for the macrophyte restoration in the management of lake ecosystems.
An array of stressors underlying the loss of submersed macrophytes have been identified, such as herbivore grazing (Mormul et al., 2018), extreme precipitation (Cobbaert et al., 2014), shading by phytoplankton in the process of eutrophication due to high loading of nutrients such as ammonia nitrogen and phosphorus (Søndergaard et al., 2017), their interactive effects under a background of climate change (Moss et al., 2011; Short et al., 2016), and heavy metal, such as cadmium and copper (Krayem et al., 2016; Bai et al., 2018). Among them, elevated levels of ammonium receive increasing attention (Shi et al., 2020; Xian et al., 2020), because the excessive input of ammonia nitrogen to aquatic ecosystems is caused by nitrogen deposition (Ti et al., 2018). Ammonium (NH4-N, including the ionic form NH4+ and aqueous NH3) is a paradoxical nutrient because it is not only the preferred nitrogen source for the growth of submersed macrophytes (Bornette and Puijalon, 2011) but also damages submersed macrophytes at a high concentration. The impacts associated with ammonium toxicity include (1) physiological changes, such as reactive oxygen species (ROS) accumulation (Nimptsch and Pflugmacher, 2007), imbalance of nitrogen/carbon metabolism (Zhang et al., 2011), and decline in photosynthesis (Shi et al., 2020); and (2) morphological changes, mainly decreased shoot height (or leaf length) (Saunkaew et al., 2011), biomass, and relative growth rate (Zhu Z. J. et al., 2018; Tan et al., 2019). It is evident from the abovementioned data that conclusions about the role of NH4-N in the decline of macrophytes in aquatic ecosystems have been almost exclusively based on physiological and morphological responses on individual plants, with exposures lasting from a few days up to 2 months, and conducted in aquaria of volume <1 m3. Great progress has been made in the previous studies of the mechanisms of ammonium on submersed macrophyte individuals. However, the stress from high NH4-N was most likely lower at higher shoot density because of direct uptake of NH4-N by the leaves and shoots (van der Heide et al., 2008), and a previous pond experiment found that high NH4-N had little or no effect on plant number (Yu et al., 2015). Therefore, based on the previous results, we argued that extrapolating short-term and small-scale experiments to large natural ecosystems may not give reliable or accurate results. It is necessary to explore the effects and mechanisms of high ammonium on the development of submersed macrophytes at a community level in natural conditions.
The effects and mechanisms of ammonium are closely related to the spatial and temporal scale of the experiments. For example, the pot experiments conducted in the ponds near natural conditions showed that high ammonium in winter and spring had a negative impact on the leaf length and leaf dry weight of V. natans, while it has a weak or even no impact on the number of plants and root dry weight; moreover, the tolerance of submersed macrophytes in summer and autumn is higher than that in winter and spring (Yu et al., 2015, 2017). The 11-month mesocosm experiment showed that long-term high nitrogen exposure had a negative impact on the growth of submersed macrophytes (Olsen et al., 2015). Thus, the effect of ammonium is related to exposure duration, and the sensitivity of submersed macrophytes to ammonium is also changeable in different growth periods (Ochieng et al., 2021). These results indicated that the previous small-scale toxicity experiments had focused on the responses of individual plants rather than the effects on the entire plant community and little was known about how high ammonium concentrations affect the development of submersed macrophytes at the community level. The previous experiments may have limited relevance to community succession in natural shallow lakes and have not extended over the lifetime of macrophyte development. Therefore, larger temporal-spatial scale studies are needed to explore the effects and mechanisms of high ammonium on the development of submersed macrophytes.
Growth form may also influence the responses of submersed macrophytes to the stress of high NH4-N loading. For example, canopy-forming and rosette-forming species are the two most common submersed macrophytes. Canopy-forming species form canopies on the water surface, whereas rosette-forming species spread under water through the formation of daughter ramet (He et al., 2019). The canopy-forming species have better access to light than rosette-forming species. Low light may aggravate NH4-related physiological stress, as suggested in previous short-term mesocosm experiments (Cao et al., 2011; Yuan et al., 2016; Zhu et al., 2018). This is because submersed macrophytes may synthesize free amino acids (FAAs) to avoid NH4-N toxicity when experiencing high NH4-N stress. On the one hand, this process is energetically expensive, and extra energy and carbohydrates are needed for the detoxication process (Bittsanszky et al., 2015), which may lead to the reduction of the macrophyte height and biomass growth. On the other hand, previous short-term experiments have found that high shoot density will alleviate the stress from high NH4-N (van der Heide et al., 2008), and plant number will not be inhibited by NH4-N stress (Yu et al., 2015). This suggests that rapid growth and strong alight capture ability at the community level may help submersed macrophyte populations to alleviate high NH4-N stress. Therefore, we hypothesized that the submersed macrophyte populations with stronger abilities for light capture and spread may resist high NH4-N stress.
To explore the responses of macrophytes to exposure to high NH4-N on a large temporal-spatial scale at the community level, two common and widely distributed submersed macrophytes were selected, namely, rosette-forming Vallisneria natans and canopy-forming Myriophyllum spicatum. In this study, the experiment was carried out in six experimental ponds (the average area was 600 m2, the water depth was 1.5 m), mimicking natural shallow lake ecosystems over 18 months, covering the entire life cycles of the two test macrophytes. To investigate the effects of NH4-N loading on the development of submersed macrophyte populations, six NH4-N loading treatments (N0: 0; N1: 5; N2: 11; N3: 22; N4: 36; N5: 50 kg/month, representing NH4-N concentrations of approximately 0, 2, 4, 8, 13, and 19 mg/L, respectively) were used without phosphorus addition. The objectives of the study were (1) to examine the effects of NH4-N on two coexisting populations of submersed macrophytes on the larger temporal-spatial scale and (2) to compare the responses of two growth forms of submersed macrophytes to the NH4-N additions at the community level.
Materials and Methods
Experimental Treatments
The experimental ponds were located in the north-eastern part of Lake Bao’an (N37°17′17″, E114°43′45″) (Figure 1). The ponds had an average area of 600 m2 with a 1.5 m mean water depth and were constructed after dredging sediments. Water and sediments from Lake Bao’an were added to mimic the natural lake ecosystem.
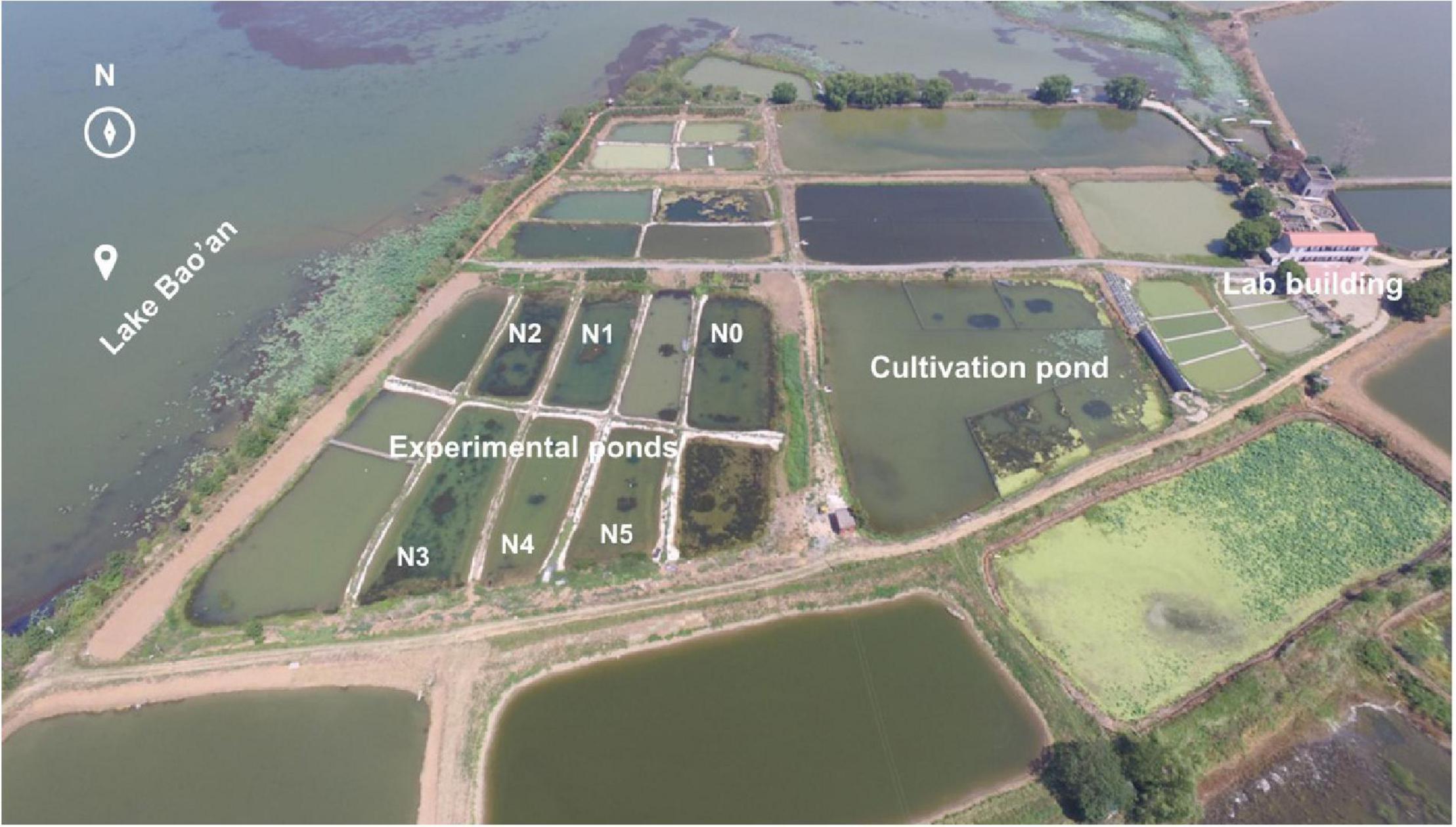
Figure 1. The experimental ponds and cultivation pond. NH4Cl fertilizer was added once per month from March 2017; the fertilization amount per month was 0 kg for N0, 5 kg for N1, 11 kg for N2, 22 kg for N3, 36 kg for N4, and 50 kg for N5. The photo was taken in June 2017.
On 15 September 2016, plants were collected from mesotrophic Lake Xi’liang (N29°58′30″, E114°6′0″, 72.1 km2 in area, 1.9 m in mean depth). Plants of similar size were chosen and cut to a leaf length of 15 cm before their introduction to a neighboring cultivation pond (Figure 1). On 25 December 2016, after 3 months of cultivation, the plants were cut again to a leaf length of 15 cm and introduced to the six experimental ponds (Figure 1), in an area of 2 m × 2 m, with bamboo poles marking the boundaries and water depth maintained at 30 cm to ensure sufficient light for macrophyte growth. The distance between individual plants was 10 cm, and the distance between M. spicatum areas and V. natans areas was 5 m. On 10 March 2017, the cover and height of submersed macrophytes were measured. Water levels were then increased to 1.6 m. Fertilization started on 20 March 2017, with additions of NH4Cl of 0 (N0), 5 (N1), 11 (N2), 22 (N3), 36 (N4), and 50 (N5) kg per month, which may ideally maintain a gradient of NH4-N concentration (i.e., 0, 2, 4, 8, 13, and 19 mg/L). The NH4Cl fertilizer (≥98.5% purity; Sinopharm Chemical Reagent Co., Ltd., Shanghai, China) was the only artificial nitrogen source and was dissolved in a polyethylene bucket with pond water for its application randomly across the surface of each fertilized pond. The experiment lasted for 18 months, from December 2016 to May 2018.
Sampling and Measurements
The height of M. spicatum and V. natans was measured each month at 10 randomly selected sites in each pond. The areal cover (S1) of M. spicatum and V. natans and the water surface area of the pond (S2) were measured simultaneously to calculate the percentage plant cover:
Environmental parameters were also measured every month. Water-dissolved oxygen (DO), pH, and conductivity (Cond) were measured in situ at 0.1 and 1.0 m depth with a YSI Pro Plus (Yellow Spring Inc., OH, Yellow Springs, United States). Water samples for chemical analysis were collected at five randomly chosen locations from each pond by integrating the water column with a tube sampler (1.5 m in height, 10 cm in diameter). Total nitrogen (TN) and total phosphorus (TP) were determined using spectrophotometry after digestion with K2S2O8 solution (Huang et al., 1999). Chlorophyll a of phytoplankton (Chl a) was determined by filtering a known volume of pond water through GF/C filters and extraction in 90% acetone at 4°C for 24 h (Whatman, GE Healthcare UK Limited, Buckinghamshire, United Kingdom). Absorbance values of the acetone extract were then read at two wavelengths (665 and 750 nm) by a spectrophotometer before and after acidification using 10% HCl, and calculations were used to obtain the Chl a concentration (Huang et al., 1999).
For analysis of ammonium (NH4-N), water was filtered using an acetate fiber microporous membrane (0.45 μm pore diameter; Shanghai Xingya Purification Material Factory, Shanghai, China), after which NH4-N was measured using Nessler’s reagent colorimetric method. The measured NH4-N concentration is total ammonia nitrogen (TAN, including both NH4+ and NH3). To estimate the relative concentrations of NH4+ and NH3 from TAN, the following equations were used (Emerson et al., 1975; Wood, 1993):
and
where, NH4-N, the concentration of ammonium (NH4-N, including both NH4+ and NH3) in mg/L; NH3, the concentration of un-ionized ammonia (NH3-N) in mg/L; pH, pH of the pond water; and Temp, temperature of the pond water in °C.
At the end of the experiment, about 0.5–1 g of fresh leaves from both species were ground in a 10% acetic acid solution to determine FAA. The filtered liquid was used to examine the FAA via the ninhydrin colorimetric method using leucine standards (Li et al., 2004).
Statistical Analysis
The variables that did not follow normal distributions (Shapiro–Wilk test, p < 0.05) were log10 (x)-transformed, including TN, NH4-N, NH3, and height of populations. Pearson’s correlation was used to analyze the relationships between environmental variables and the height and cover of submersed macrophytes. SPSS 25, Microsoft Excel 2019, and R 4.1.2 (Rcore Team, 2020) were used to analyze the data. We conducted Seasonal and Regional Kendall trend tests to analyze long-term trends among different treatments (Jassby and Cloern, 2016). A Sen’s slope was considered significant when the associated p-value was <0.05. We also carried out Pettitt’s test to detect abrupt changes in submersed macrophyte populations (Pohlert, 2020).
Results
Nutrient Conditions in the Experimental Ponds
Throughout the pre-fertilization period (December 2016 to March 2017), no statistical differences among treatments were observed for TN, NH4-N, NH3, TP, Chl a, and pH (Supplementary Table 1). For the pre-fertilization period (3 months), the mean concentration of TN, NH4-N, and NH3-N in the six experimental ponds was <0.5, 0.2, and 0.01 mg/L, respectively. The corresponding mean concentrations of TP and Chl a were <0.01 mg/L and 1 μg/L, respectively.
For the post-fertilization (N loading) period, the concentrations of the three nitrogen variables (TN, NH4-N, and NH3-N) varied with N treatment. TN and NH4-N were significantly higher in N1, N2, N3, N4, and N5 than in N0 (Figures 2A–C and Supplementary Table 1), with TN ranging between 0.8 and 27.0 mg/L, NH4-N between 0.2 and 18.0 mg/L, and NH3-N ranging between 0.01 and 6.9 mg/L. TN in N1, N4, and N5 showed a significant increasing trend over the 18-month study period (p < 0.05, Sen’s slope >0), and NH4-N showed a significant increasing trend in N1 and N5 (Supplementary Table 2). There was a clear increasing trend of NH3-N in N5 (Supplementary Table 2). The target gradient of NH4-N concentration based on loads was 0 (N0), 2 (N1), 4 (N2), 8 (N3), 13 (N4), and 19 (N5) mg/L; however, the actual concentration was 0.2, 0.8, 1.0, 2.4, 7.0, and 18.0 mg/L in the six respective treatments.
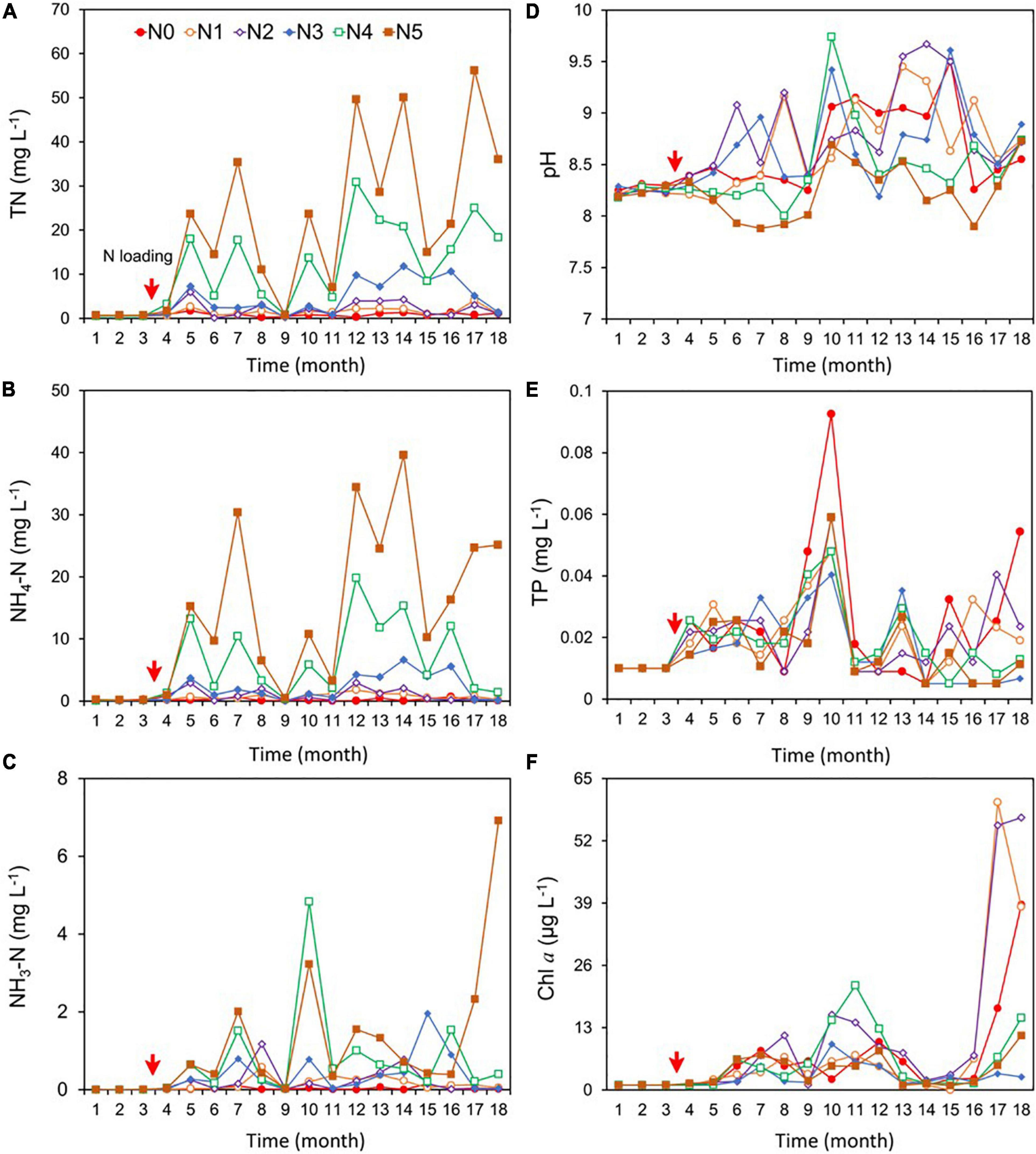
Figure 2. Changes in the concentrations of total nitrogen (TN) (A), ammonium (NH4-N) (including both NH3 and NH4+) (B), un-ionized ammonia (NH3-N) (C), pH (D), total phosphorus (TP) (E), and phytoplankton chlorophyll a (Chl a) (F) during the experiment (December 2016–May 2018). NH4Cl fertilizer was added once per month from March 2017; the fertilization amount per month was 0 kg for N0, 5 kg for N1, 11 kg for N2, 22 kg for N3, 36 kg for N4, and 50 kg for N5. The red arrows indicate the start of fertilization, after which nitrogen fertilizer is applied monthly.
pH showed no significant difference in the N0 control and the N1, N2, N3, and N4 treatments, while pH was significantly lower in N5 than in N0–N4, with the mean for the 18 months ranging between 7.9 and 9.7 in all treatments (Figure 2D and Supplementary Table 1). The mean concentration of TP was 0.02 mg/L in each treatment (Figure 2E), with no significant trends among treatments during the 18 months (Supplementary Table 2).
Chlorophyll a showed no significant difference between N1, N2, N4, N5, and N0, but N0 was significantly higher than N3. Chl a concentration ranged between 4.0 and 14.0 μg/L in the six treatments (Figure 2F). Significant increasing trends of Chl a occurred in N0, N3, and N4 during the 18 months (Supplementary Table 2).
Submersed Macrophyte Cover
The Seasonal and Regional Kendall trend test indicated that the cover of M. spicatum and V. natans increased significantly (p < 0.05, Sen’s slope >0) during the 18 months, except for a decreasing trend of M. spicatum in N5 (p < 0.05, Sen’s slope <0) (Figures 3A,B and Supplementary Table 2). Pettitt’s test can detect single change-point in cover of both species in time series in all treatments. Most of the change-points occurred after 4–6 months of NH4-N loading.
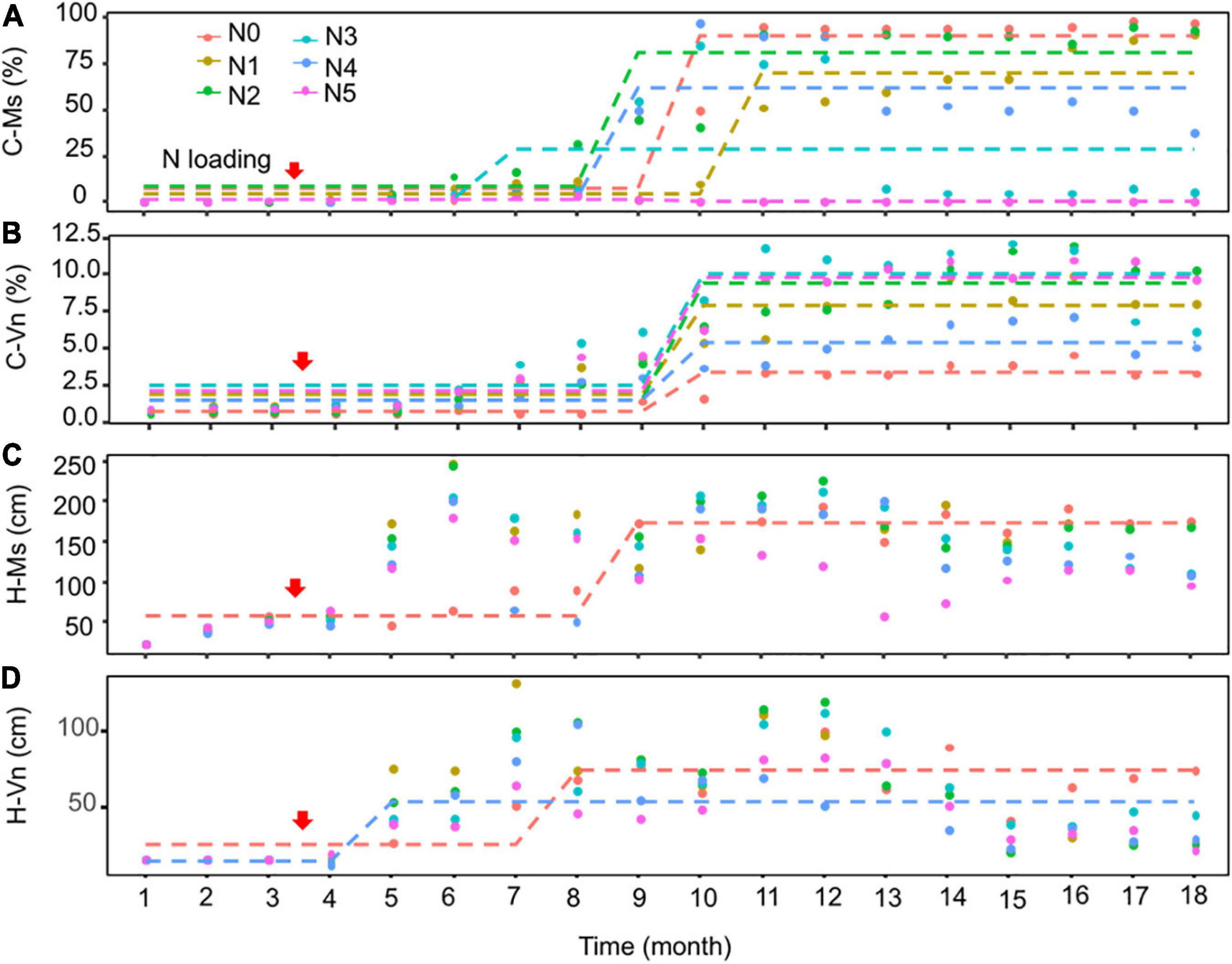
Figure 3. Pettitt’s test for single change-point detection for the cover (C-Ms, C-Vn, %) (A,B) and height (H-Ms, H-Vn, cm) (C,D) of Myriophyllum spicatum (Ms) and Vallisneria natans (Vn) for various treatments during the experiment. The dotted lines indicate that probable change point was detected (p < 0.05). The red arrows indicate the start of fertilization, after which nitrogen fertilizer is applied monthly. Refer to Figure 2 for the fertilization amounts of various treatment.
For the cover of M. spicatum, there were no significant differences between N2 (1.0 mg/L), N3 (2.4 mg/L), N4 (7 mg/L), and N0, and the cover of M. spicatum was significantly higher in N0 than in N5 (with concentrations of 27 mg/L TN and 18 mg/L NH4-N) (Supplementary Table 1 and Figures 4A–F). In the N0–N2 treatments, the M. spicatum cover started to increase after 6 months of N loading and was >90% at the end of the experiment (Figures 4A–C). In the N3 (2.4 mg/L) and N4 (7 mg/L) treatments, the cover of M. spicatum was ∼90% during autumn 2017 after which a major drop occurred (Figures 4D,E).
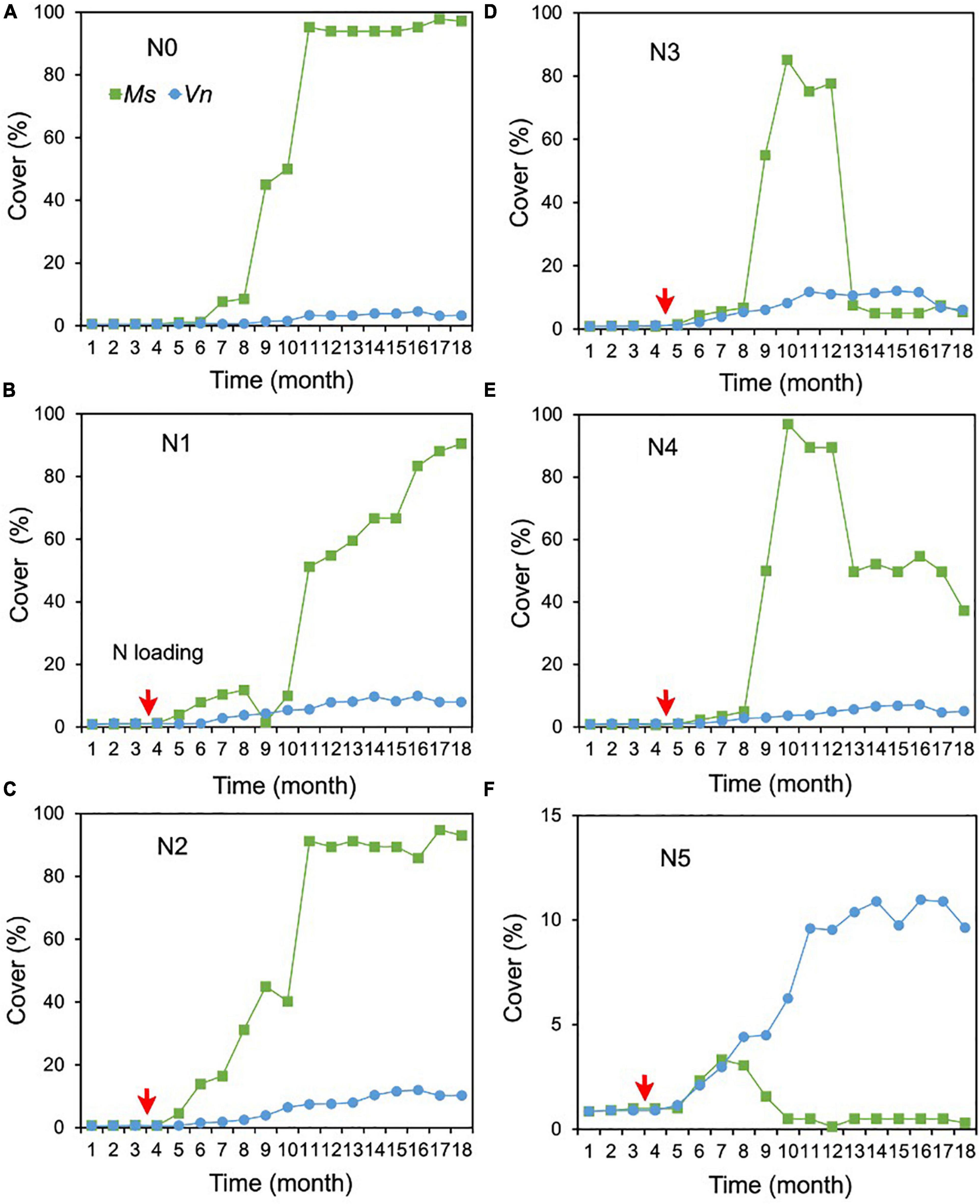
Figure 4. Changes in the cover of Myriophyllum spicatum (Ms) and Vallisneria natans (Vn) (A–F) for various treatments during the experiment. The red arrows indicate the start of fertilization, after which nitrogen fertilizer is applied monthly. Refer to Figure 2 for the fertilization amounts of various treatments.
As for the cover of V. natans, significant increasing trends were observed in all treatments (p < 0.05, Sen’s slope >0) during the 18 months (Figure 3B and Supplementary Table 2). The cover of V. natans in N1–N5 was significantly higher than in N0 (Supplementary Table 1). At the end of the experiment, the lowest cover (3.3%) was recorded in the N0 treatment (Figure 4A), and in the N1–N5 treatments, cover ranged between 5 and 10% (Figures 4B–F).
At the end of the experiment, the cover of M. spicatum in N1–N4 was 7–11 times higher than that of V. natans, while the cover of M. spicatum in N5 was lower than that of V. natans (Figure 4). The results of Pettitt’s test showed that the change points of M. spicatum cover were earlier than those of V. natans in N2–N5, while the change point was downward in N5 and upward in N2–N4 (Figures 3A,B).
Submersed Macrophyte Height
There were no change points identified for the height of the two species in most treatments using Pettitt’s test, except for the height of M. spicatum in N0 and the height of V. natans in N0 and N4 (Figures 3C,D). The height of M. spicatum and V. natans mostly leveled off or decreased in all treatments during the 18 months (Figures 5A–F).
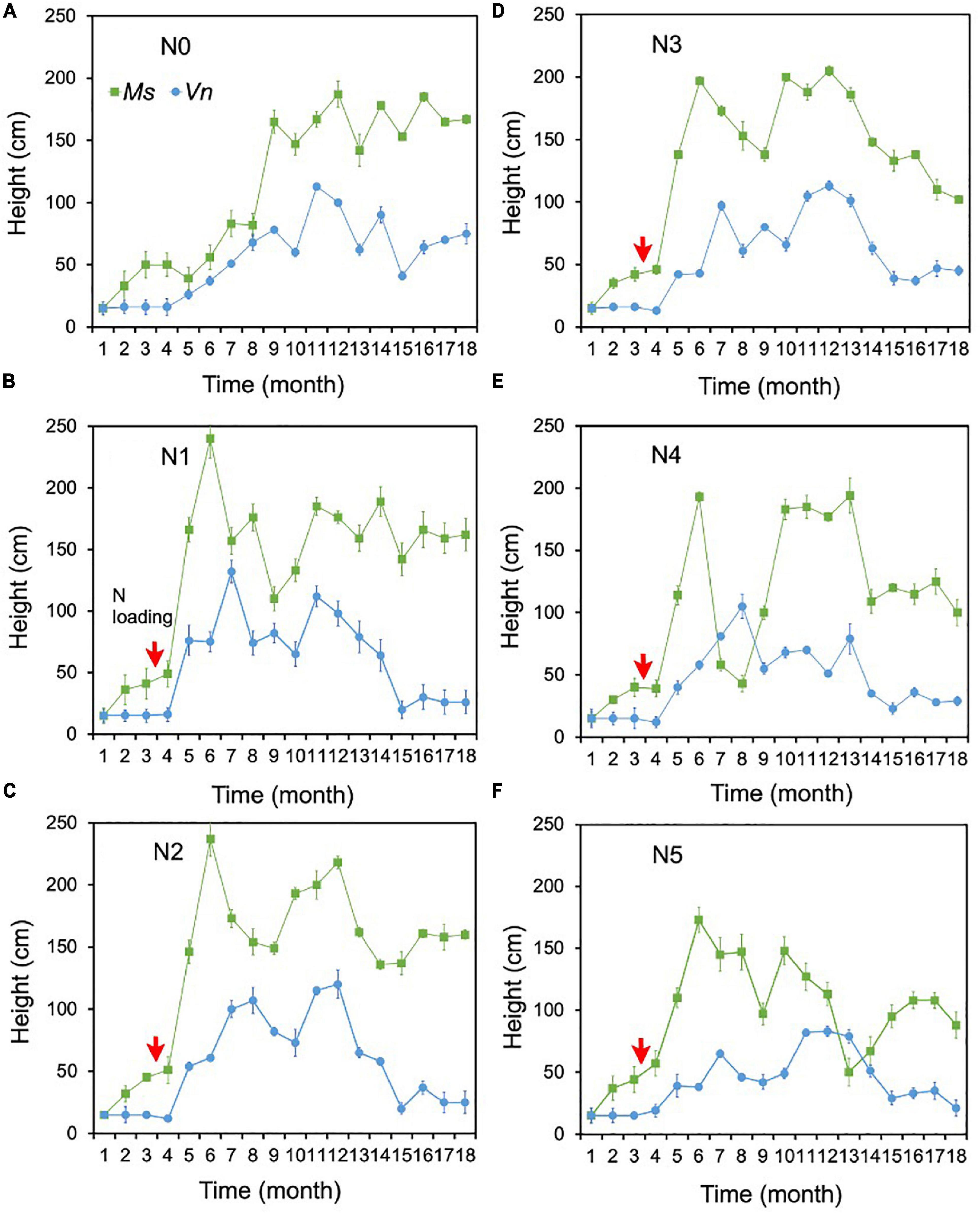
Figure 5. Changes in the heights of Myriophyllum spicatum (Ms) and Vallisneria natans (Vn) (A–F) for treatments during the experiment. The red arrows indicate the start of fertilization, after which nitrogen fertilizer is applied monthly. Refer to Figure 2 for the fertilization amounts of various treatment.
The height of M. spicatum showed no significant difference between N1–N5 (i.e., for concentrations up to 18 mg/L NH4-N) and N0, although they increased across all treatments over the duration of the experiment. The height in N1–N3 was significantly greater than in N4 and N5 (Supplementary Table 1). The height of M. spicatum showed a clear increasing trend (p < 0.05, Sen’s slope >0) from the initial value of 15–167 cm in N0 (Figure 5A and Supplementary Table 2). In N1–N5, despite no significant trends (p > 0.05), the height still increased to greater than the initial value of 15 cm (Supplementary Table 2 and Figures 5B–F). However, the height of M. spicatum showed declining trends in N3–N5 treatments in the last few months of the experiment.
For V. natans, there were no significant differences in height in N1–N4 (with concentrations <7 mg/L NH4-N) and N0, while the height in N5 was significantly greater than in N0 (Supplementary Table 1 and Figures 5A–F). A clear increasing trend (p < 0.05, Sen’s slope >0) of V. natans occurred in N0 compared with the initial values of 15 cm, while the height in other treatments generally declined from 13 months (Supplementary Table 2 and Figures 5B–F).
The height of M. spicatum was two to six times higher than that of V. natans across all treatments and control by the end of the experiment.
Relationships Between Submersed Macrophytes and Environmental Variables
Figure 6 illustrates the relationships among TN, NH4-N, NH3-N, pH, Chl a, FAA, and the height and cover of M. spicatum and V. natans at the end of the experiment. The cover of M. spicatum was positively related (p < 0.05) to Chl a, TP, and pH, and the height of M. spicatum was negatively related to NH4-N, while positively related to pH and Chl a (Figure 6). The relationship between the cover of V. natans and pH was positively related, and the height of V. natans was positively related to TN, NH4-N, NH3-N, Chl a, and pH.
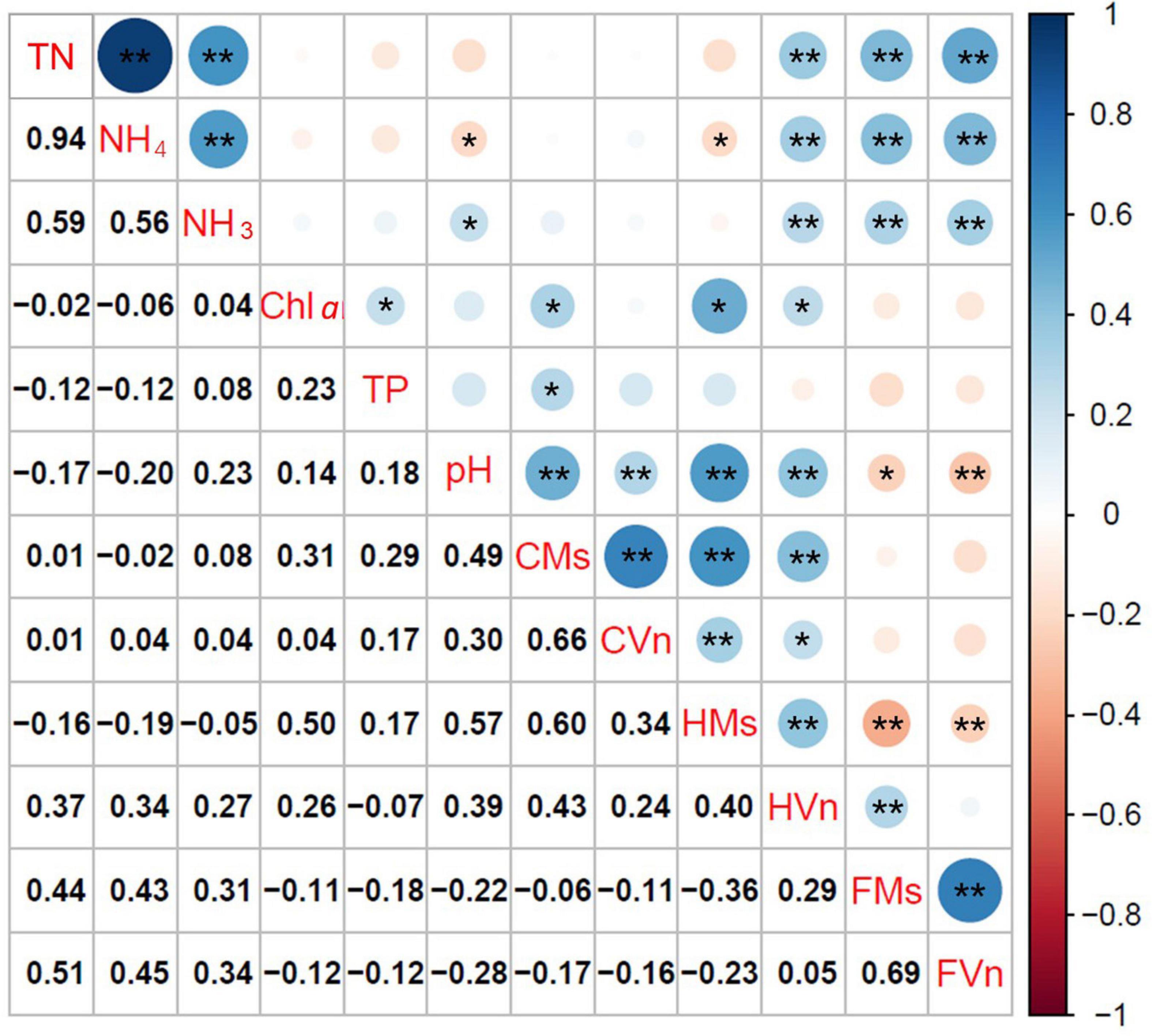
Figure 6. Coefficients of Pearson rank correlation of free amino acid content (FAA, mg/g), height (H, cm), and cover (C, %) of Myriophyllum spicatum (Ms) and Vallisneria natans (Vn), with the key environmental variables (*p < 0.05, **p < 0.01). TN, total nitrogen; NH4, ammonia nitrogen expressed as an ionized form; NH3, ammonia nitrogen expressed as an un-ionized form; Chl a, chlorophyll a of phytoplankton; TP, total phosphorus; CMs, cover of M. spicatum; CVn, cover of V. natans; HMs, height of M. spicatum; HVn, height of V. natans; FMs, FAA of M. spicatum; FVn, FAA of V. natans.
Free amino acids of both plants accumulated with increasing TN, NH4-N, and NH3-N (p < 0.05), but no significant relationships were established between cover and FAA. The relationships between FAA of M. spicatum and V. natans and pH were negatively correlated.
Discussion
Clear seasonal dynamics were observed for TP and phytoplankton Chl a in the ponds, being higher in the summer and autumn, likely reflecting higher summer growth of phytoplankton combined with the stronger release of phosphorus from the sediment during the warmer season (Søndergaard et al., 2003; Xie, 2006).
Our pond experiment is, to our knowledge, the first attempt to reveal the influence of nitrogen loading at the community level for submersed macrophytes. In contrast to the experiments that have focused on the physiology and morphology of individual plants (Wang et al., 2008; Apudo et al., 2016; Zhu et al., 2018; Gao et al., 2019), our 18-month study dealing with populations revealed that M. spicatum and V. natans tolerated short-term ammonium stress when NH4-N was <7 mg/L, while the development under long-term ammonium stress for both species occurred when NH4-N exceeded 2.4 mg/L. A decline in submersed macrophyte height has previously been recorded at lower NH4-N <2–6 mg/L, which has been attributed to the direct toxic effects of NH4-N and associated with the combined stress of high pH and low light (Cao et al., 2011; Netten et al., 2013; van Diggelen et al., 2016; Zhu et al., 2018). In the present study, at NH4-N of 7 mg/L (N4), the cover and height of both M. spicatum and V. natans increased after 4 months of NH4-N loading, and the cover and height of V. natans also increased at NH4-N of 18 mg/L (N5). Relationships between pH and cover of M. spicatum and V. natans were positively significant, and there were positive relationships between cover and height of M. spicatum and Chl a, suggesting that pH and phytoplankton biomass did not impede the growth of submersed macrophytes. In a short-term experiment (up to 5 weeks), the results showed that plants were tolerant to NH4-N at high shoot densities (van der Heide et al., 2008). We found almost no loss of cover or height in most of the ponds (N1–N4) with <7 mg/L NH4-N after 9 months, and only the cover of M. spicatum was suppressed in the N5 treatment with a concentration of 18 mg/L NH4-N. However, during the last 6 months of the experiment, the tolerance concentration of both species was reduced to 2.4 mg/L. Therefore, our larger temporal-spatial pond experiment results suggest that exposure duration is of key importance in determining the effect of ammonium stress, which increased with exposure duration.
Our findings differ from previous findings revealing that NH4-N has a negative effect at a concentration of 2–6 mg/L, which causes physiological toxicity in plants with exposure duration <10 months (Netten et al., 2013; Rao et al., 2018; Zhu et al., 2018). In N1–N4 of our experiment, pH was close to 9, which should enhance the physiological toxicity of NH3 (Caicedo et al., 2000; Körner et al., 2001; Gao et al., 2015), but the cover of both submersed macrophyte species showed increasing trends, and there was a significant positive correlation between pH and height and cover of both growth forms of submersed macrophytes. Moreover, we did not find significant relationships between FAA and the population cover and height of both submersed macrophytes through the marked accumulation of FAA in M. spicatum and V. natans at the end of the experiment. Our results are consistent with some other field studies that moderate to high concentrations of NH4-N do not inhibit the growth of submersed macrophytes by physiological changes related to toxicity (Olsen et al., 2015; Zhao et al., 2016; Yu et al., 2017). Furthermore, we did not find that accumulation of FAA is a useful indicator of macrophyte growth and the physiological stress of plants in our field study as otherwise seen in pot experiments (Cao et al., 2009a; Yuan et al., 2015; Rao et al., 2018).
Due to the marked inhibition in the cover of M. spicatum in N5 treatment, the higher height of M. spicatum than V. natans at the highest NH4-N concentration (18 mg/L) and higher height and cover of M. spicatum than that of V. natans in N1–N4 treatments tend to support our hypothesis that the submersed macrophyte community with stronger abilities of light capture and spread may resist high ammonium stress. Generally, the canopy-forming species M. spicatum might resist NH4-N stress by shoot elongation to enhance light capture, while V. natans may resist NH4-N stress by slow elongation growth of shoots to reduce resource consumption. During the experiment, the population cover and height of M. spicatum in N0–N4 increased to a much greater extent than that of V. natans. When exposed to 7 mg/L NH4-N, the cover of M. spicatum was around seven times higher than that of V. natans, and the height of M. spicatum was three times higher than that of V. natans, suggesting greater resilience of M. spicatum to high NH4-N. These results can be attributed to the physiological and morphological characteristics of the two species. First, to prevent NH4-N accumulation, the major physiological pathway (GS/GOGAT cycle) of NH4-N assimilation is activated, which synthesizes FAA from NH4-N (Xian et al., 2020). In many cases, this may result in disrupted metabolism and high carbon requirements for internal NH4-N detoxification (Netten et al., 2013; Gao et al., 2020). When the NH4-N supply is high, the GS/GOGAT cycle is modified and adapted to increase FAA, leading to increased energy requirements and high demand for carbohydrates. Thus, carbon depletion is a mechanism behind NH4-N inhibition of growth (Cao et al., 2007, 2011). However, M. spicatum has another pathway than V. natans, for ammonia detoxification, catalyzed by glutamate dehydrogenase (GDH) under high NH4-N conditions (Gao et al., 2020; Xian et al., 2020). Moreover, M. spicatum forms canopy vegetation with leaves on the water surface, where there is sufficient light for the production of carbohydrates to assist plants to overcome NH4-N stress (Fu et al., 2012; Yu et al., 2018; Angove et al., 2020). Finally, M. spicatum can reproduce asexually using vegetative fragments (produced naturally or broken mechanically) and disperse to other areas of our experimental ponds, e.g., via waves, invertebrates, and human disturbance (Li, 2014). However, the disturbance caused by wind wave may have led to a decline in height of M. spicatum in the last several months of the experiment, as the shoots of M. spicatum exposed to high N loading become fragile and easily breakable (Zhu et al., 2018), and canopy-forming species, like M. spicatum, are most vulnerable to wind disturbance. In contrast, V. natans roots in sediment disperse by plagiotropic stolon and spread horizontally above the ground (Xiao et al., 2006). Therefore, the cover of V. natans in N0–N4 treatments was much lower than that of M. spicatum. These results concurred with experiments demonstrating that the canopy-forming species (i.e., Potamogeton crispus) is tolerant to NH4-N concentration (<7 mg/L) (Cao et al., 2009b; Yu et al., 2018). Although the cover of V. natans keeps growing in all NH4-N addition treatments, the height began to decline after 13 months of N loading. This suggests that the rosette-forming species resist NH4-N stress by reducing the elongation growth of populations, i.e., reducing consumption to resist stress. The results of the community level coincide with the physiological imbalance of nitrogen/carbon metabolism (Zhang et al., 2011; Yuan et al., 2015; Rao et al., 2018).
Conclusion
We proposed a conceptual framework to describe the population development of submersed macrophytes responses to ammonium loading (Figure 7). The main conclusions are as follows: (1) populations of both growth forms can disperse in short-term exposure duration at 7 mg/L NH4-N, while the cover of M. spicatum and height of both species were inhibited at 2.4 mg/L in long-term exposures; (2) canopy-forming species can resist NH4-N stress through fast shoot elongation and canopy growth to capture light; (3) rosette-forming species can resist 18 mg/L NH4-N stress through reducing consumption; and (4) the negative effects of ammonium stress on the development of populations increased with exposure duration. Our findings have implications for decision-making on submersed macrophyte restoration in water with high N content. Both canopy-forming and rosette-forming species may potentially be used in the restoration of shallow lakes. However, canopy-forming species, if covering the water surface, may compete with rosette-forming submersed macrophyte populations for light and block their development and tolerance to high NH4-N concentration. These results point to the need to integrate interspecific competition with physiological stress indicators, morphological traits, and population characteristics, to better understand the mechanisms underlying macrophytes decline in shallow lakes. Our results also point to the importance of exposure duration to NH4-N loading, with lower thresholds of response at longer exposure time. The interactions between seasonal dynamics of submersed macrophyte populations and NH4-N or other environmental variables need to be further studied.
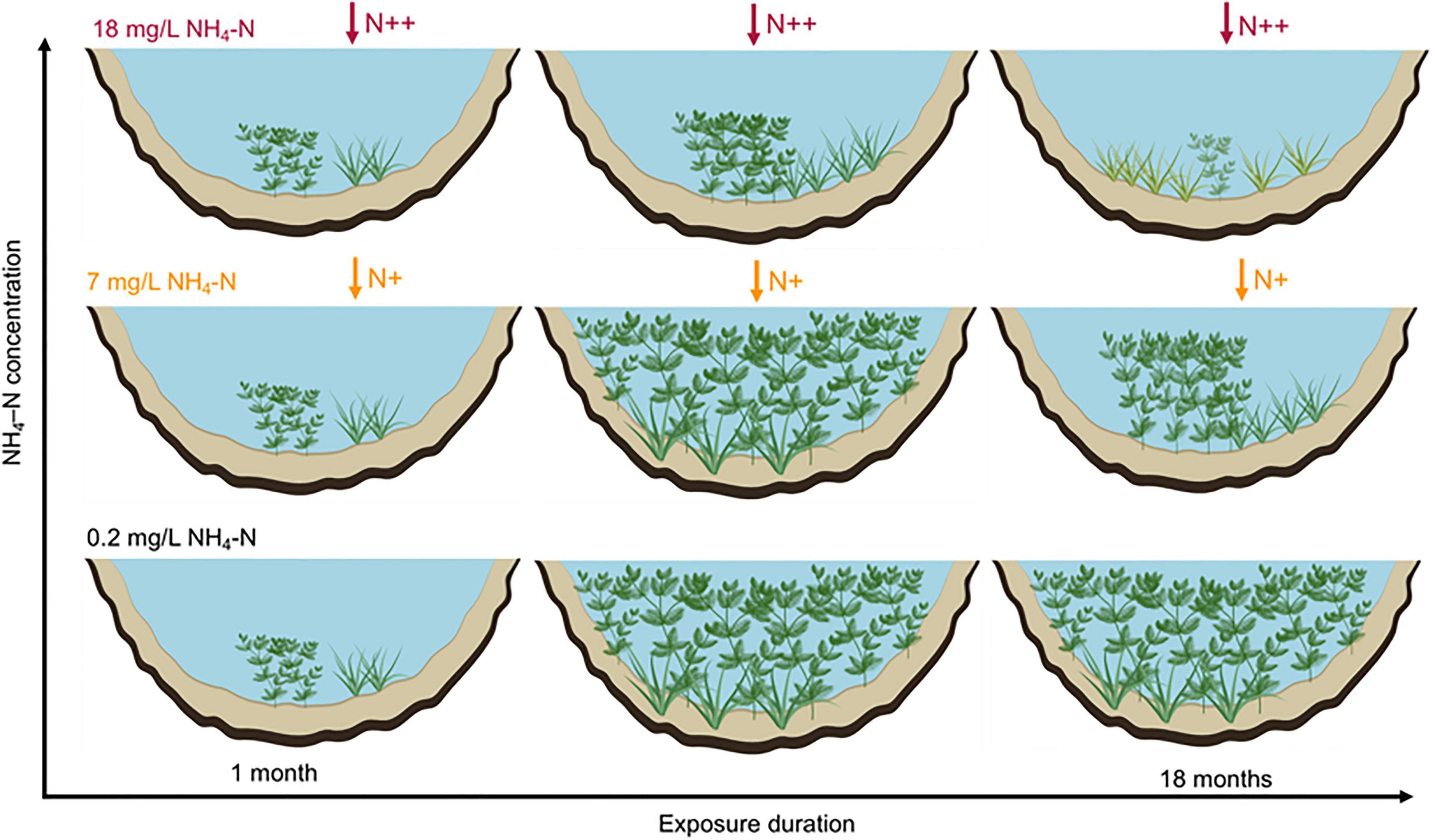
Figure 7. Schematic graphs showing the population development of canopy-forming Myriophyllum spicatum and rosette-forming Vallisneria natans in response to NH4-N concentrations at larger temporal-spatial scale.
Data Availability Statement
The original contributions presented in this study are included in the article/Supplementary Material, further inquiries can be directed to the corresponding authors.
Author Contributions
QY: validation, conceptualization, investigation, methodology, formal analysis, data curation, and writing – original draft and review and editing. HJW: conceptualization, methodology, writing – review, supervision, resources, project administration, and funding acquisition. HZW: conceptualization, methodology, supervision, and project administration. CX, YM, and SM: validation and investigation. ML: validation, investigation, and data curation. YL: validation and writing – review and editing. DH: methodology and writing – review and editing. EJ: conceptualization, methodology, and writing – review and editing. All authors contributed to the article and approved the submitted version.
Funding
This research was sponsored by the National Key Research and Development Program of China (2018YFD0900805), Major Science and Technology Program for Water Pollution Control and Treatment of China (2017ZX07302-002), State Key Laboratory of Freshwater Ecology and Biotechnology (2019FBZ01), and Yunnan Provincial Department of Science and Technology (202001BB050078 and 202103AC100001). HJW was supported by the Youth Innovation Association of Chinese Academy of Sciences as an excellent member (Y201859). QY was supported by the National Science Foundation of Shandong Province (ZR2021QC081).
Conflict of Interest
The authors declare that the research was conducted in the absence of any commercial or financial relationships that could be construed as a potential conflict of interest.
Publisher’s Note
All claims expressed in this article are solely those of the authors and do not necessarily represent those of their affiliated organizations, or those of the publisher, the editors and the reviewers. Any product that may be evaluated in this article, or claim that may be made by its manufacturer, is not guaranteed or endorsed by the publisher.
Acknowledgments
We thank Chi Xu for his valuable comments, Anne Mette Poulsen for editing the study, and Lele Liu for helping in using the R software.
Supplementary Material
The Supplementary Material for this article can be found online at: https://www.frontiersin.org/articles/10.3389/fpls.2022.939589/full#supplementary-material
References
Angove, C., Norkko, A., and Gustafsson, C. (2020). The fight to capture light: functional diversity is related to aquatic plant community productivity likely by enhancing light capture. Front. Mar. Sci. 7:140. doi: 10.3389/fmars.2020.00140
Apudo, A. A., Cao, Y., Wakibia, J., Li, W., and Liu, F. (2016). Physiological plastic responses to acute NH4+–N toxicity in Myriophyllum spicatum L. cultured in high and low nutrient conditions. Environ. Exp. Bot. 130, 79–85. doi: 10.1016/j.envexpbot.2016.05.009
Bai, L., Liu, X. L., Hu, J., Li, J., Wang, Z. L., Han, G. L., et al. (2018). Heavy metal accumulation in common aquatic plants in rivers and lakes in the Taihu Basin. Int. J. Environ. Res. Public Health 15:2857. doi: 10.3390/ijerph15122857
Bittsanszky, A., Pilinszky, K., Gyulai, G., and Komives, T. (2015). Overcoming ammonium toxicity. Plant Sci. 231, 184–190. doi: 10.1016/j.plantsci.2014.12.005
Bornette, G., and Puijalon, S. (2011). Response of aquatic plants to abiotic factors: a review. Aquat. Sci. 73, 1–14. doi: 10.1007/s00027-010-0162-7
Caicedo, J. R., Van der Steen, N. P., Arce, O., and Gijzen, H. J. (2000). Effect of total ammonia nitrogen concentration and pH on growth rates of duckweed (Spirodela polyrrhiza). Water Res. 34, 3829–3835. doi: 10.1016/S0043-1354(00)00128-7
Cao, T., Xie, P., Li, Z. Q., Ni, L. Y., Zhang, M., and Xu, J. (2009a). Physiological stress of high NH4+ concentration in water column on the submersed macrophyte Vallisneria natans L. Bull. Environ. Contaminat. Toxicol. 82, 296–299. doi: 10.1007/s00128-008-9531-5
Cao, T., Xie, P., Ni, L., Zhang, M., and Xu, J. (2009b). Carbon and nitrogen metabolism of an eutrophication tolerative macrophyte, Potamogeton crispus, under NH4+ stress and low light availability. Environ. Exp. Bot. 66, 74–78.
Cao, T., Xie, P., Ni, L. Y., Wu, A. P., Zhang, M., Wu, S. K., et al. (2007). The role of NH4+ toxicity in the decline of the submersed macrophyte Vallisneria natans in lakes of the Yangtze River basin, China. Mar. Freshw. Res. 58, 581–587. doi: 10.1071/MF06090
Cao, T. E., Ni, L., Xie, P., Xu, J., and Zhang, M. (2011). Effects of moderate ammonium enrichment on three submersed macrophytes under contrasting light availability. Freshw. Biol. 56, 1620–1629.
Chao, C. X., Lv, T., Wang, L. G., Li, Y., Han, C., Yu, W. C., et al. (2022). The spatiotemporal characteristics of water quality and phytoplankton community in a shallow eutrophic lake: implications for submerged vegetation restoration. Sci. Total Environ. 821:153460. doi: 10.1016/j.scitotenv.2022.153460
Cobbaert, D., Wong, A., and Bayley, S. E. (2014). Precipitation-induced alternative regime switches in shallow lakes of the Boreal Plains (Alberta, Canada). Ecosystems 17, 535–549. doi: 10.1007/s10021-013-9741-5
Emerson, K., Russo, R. C., Lund, R. E., and Thurston, R. V. (1975). Aqueous ammonia equilibrium caculations-effect of pH and temperature. J. Fish. Res. Board Can. 32, 2379–2383. doi: 10.1139/f75-274
Fu, H., Yuan, G., Cao, T., Ni, L., Zhang, M., and Wang, S. (2012). An alternative mechanism for shade adaptation: implication of allometric responses of three submersed macrophytes to water depth. Ecol. Res. 27, 1087–1094. doi: 10.1007/s11284-012-0991-z
Gao, J., Li, L., Hu, Z., Zhu, S., Zhang, R., and Xiong, Z. (2015). Ammonia stress on the carbon metabolism of Ceratophyllum demersum. Environ. Toxicol. Chem. 34, 843–849. doi: 10.1002/etc.2866
Gao, J., Liu, L., Ma, N., Yang, J., Dong, Z., Zhang, J., et al. (2020). Effect of ammonia stress on carbon metabolism in tolerant aquatic plant-Myriophyllum aquaticum. Environ. Pollut. 263:114412. doi: 10.1016/j.envpol.2020.114412
Gao, J., Ren, P., Zhou, Q., and Zhang, J. (2019). Comparative studies of the response of sensitive and tolerant submerged macrophytes to high ammonium concentration stress. Aquat. Toxicol. 211, 57–65. doi: 10.1016/j.aquatox.2019.03.020
He, L., Zhu, T. S., Wu, Y., Li, W., Zhang, H., Zhang, X. L., et al. (2019). Littoral slope, water depth and alternative response strategies to light attenuation shape the distribution of submerged macrophytes in a mesotrophic lake. Front. Plant Sci. 10:169. doi: 10.3389/fpls.2019.00169
Huang, X. F., Chen, W. M., and Cai, Q. M. (1999). Standard methods for observation and analysis in Chinese ecosystem research network-survey, Observation and Analysis of Lake Ecology. Beijing: Standards Press of China.
Jassby, A. D., and Cloern, J. E. (2016). Wq: Some tools for exploring water quality monitoring data, R package version 0. 4.9. (accessed May 18, 2016).
Jeppesen, E., Søndergaard, M., Søndergaard, M., and Christoffersen, K. (1998). The Structuring Role of Submerged Macrophytes in Lakes. New York, NY: Springer-Verlag. doi: 10.1007/978-1-4612-0695-8
Körner, S., Das, S. K., Veenstra, S., and Vermaat, J. E. (2001). The effect of pH variation at the ammonium/ammonia equilibrium in wastewater and its toxicity to Lemna gibba. Aquat. Bot. 71, 71–78. doi: 10.1016/S0304-3770(01)00158-9
Krayem, M., Baydoun, M., Deluchat, V., Lenain, J. F., Kazpard, V., and Labrousse, P. (2016). Absorption and translocation of copper and arsenic in an aquatic macrophyte Myriophyllum alterniflorum DC. in oligotrophic and eutrophic conditions. Environ. Sci. Pollut. Res. 23, 11129–11136. doi: 10.1007/s11356-016-6289-x
Li, H. S., Sun, Q., and Zhao, S. J. (2004). Plant Physiology Biochemistry Principle and Experimental Technique. Beijing: Higher Education Press.
Li, W. (2014). Environmental opportunities and constraints in the reproduction and dispersal of aquatic plants. Aquat. Bot. 118, 62–70. doi: 10.1111/1365-2656.12709
Mormul, R. P., Ahlgren, J., and Bronmark, C. (2018). Snails have stronger indirect positive effects on submerged macrophyte growth attributes than zooplankton. Hydrobiologia 807, 165–173. doi: 10.1007/s10750-017-3391-0
Moss, B., Kosten, S., Meerhoff, M., Battarbee, R. W., Jeppesen, E., Mazzeo, N., et al. (2011). Allied attack: climate change and eutrophication. Inland Waters 1, 101–105. doi: 10.5268/IW-1.2.359
Netten, J. J. C., van der Heide, T., and Smolders, A. J. P. (2013). Interactive effects of pH, temperature and light during ammonia toxicity events in Elodea canadensis. Chem. Ecol. 29, 448–458. doi: 10.1080/02757540.2013.769971
Nimptsch, J., and Pflugmacher, S. (2007). Ammonia triggers the promotion of oxidative stress in the aquatic macrophyte Myriophyllum mattogrossense. Chemosphere 66, 708–714. doi: 10.1016/j.chemosphere.2006.07.064
Ochieng, W. A., Xian, L., Nasimiyu, A. T., Muthui, S. W., Ndirangu, L. N., Otieno, D. O., et al. (2021). Exploring the ammonium detoxification mechanism of young and mature leaves of the macrophyte Potamogeton lucens. Aquat. Toxicol. 237:105879. doi: 10.1016/j.aquatox.2021.105879
Olsen, S., Chan, F., Li, W., Zhao, S., Sondergaard, M., and Jeppesen, E. (2015). Strong impact of nitrogen loading on submerged macrophytes and algae: a long-term mesocosm experiment in a shallow Chinese lake. Freshw. Biol. 60, 1525–1536. doi: 10.1111/fwb.12585
Pohlert, T. (2020). Trend: Non-Parametric Trend Tests and Change-point Detection. R Package Version 1.1.4. Available online at: https://CRAN.R-project.org/package=trend (accessed September 17, 2020).
Rao, Q., Deng, X., Su, H., Xia, W., Wu, Y., Zhang, X., et al. (2018). Effects of high ammonium enrichment in water column on the clonal growth of submerged macrophyte Vallisneria natans. Environ. Sci. Pollut. Res. 25, 32735–32746. doi: 10.1007/s11356-018-3146-0
Rcore Team. (2020). R: A Language and Environment for Statistical Computing. R version 4.1.2. Vienna: R Foundation for Statistical Computing.
Saunkaew, P., Wangpakapattanawong, P., and Jampeetong, A. (2011). Growth, morphology, ammonium uptake and nutrient allocation of Myriophyllum brasiliense Cambess. under high NH4+ concentrations. Ecotoxicology 20, 2011–2018. doi: 10.1007/s10646-011-0744-8
Sayer, C. D., Burgess, A. M. Y., Kari, K., Davidson, T. A., Peglar, S., Yang, H., et al. (2010). Long-term dynamics of submerged macrophytes and algae in a small and shallow, eutrophic lake: implications for the stability of macrophyte-dominance. Freshw. Biol. 55, 565–583. doi: 10.1111/j.1365-2427.2009.02353.x
Scheffer, M., Hosper, S. H., Meijer, M. L., Moss, B., and Jeppesen, E. (1993). Alternative Equilibria in Shallow Lakes. Trends Ecol. Evol. 8, 275–279. doi: 10.1016/0169-5347(93)90254-M
Shi, D., Zhuang, K., Chen, Y., Xu, F., Hu, Z., and Shen, Z. (2020). Effects of excess ammoniacal nitrogen (NH4+-N) on pigments, photosynthetic rates, chloroplast ultrastructure, proteomics, formation of reactive oxygen species and enzymatic activity in submerged plant Hydrilla verticillata (L.f.) Royle. Aquat. Toxicol. 226:105585. doi: 10.1016/j.aquatox.2020.105585
Short, F. T., Kosten, S., Morgan, P. A., Malone, S., and Moore, G. E. (2016). Impacts of climate change on submerged and emergent wetland plants. Aquat. Bot. 135, 3–17. doi: 10.1016/j.scitotenv.2018.06.080
Søndergaard, M., Jensen, J. P., and Jeppesen, E. (2003). Role of sediment and internal loading of phosphorus in shallow lakes. Hydrobiologia 506, 135–145. doi: 10.1023/B:HYDR.0000008611.12704.dd
Søndergaard, M., Lauridsen, T. L., Johansson, L. S., and Jeppesen, E. (2017). Nitrogen or phosphorus limitation in lakes and its impact on phytoplankton biomass and submerged macrophyte cover. Hydrobiologia 795, 35–48. doi: 10.1007/s10750-017-3110-x
Su, H., Chen, J., Wu, Y., Chen, J., Guo, X., Yan, Z., et al. (2019). Morphological traits of submerged macrophytes reveal specific positive feedbacks to water clarity in freshwater ecosystems. Sci. Total Environ. 684, 578–586. doi: 10.1016/j.scitotenv.2019.05.267
Tan, X., Yuan, G., Fu, H., Peng, H., Ge, D., Lou, Q., et al. (2019). Effects of ammonium pulse on the growth of three submerged macrophytes. PLoS One 14:e0219161. doi: 10.1371/journal.pone.0219161
Ti, C. P., Gao, B., Luo, Y. X., Wang, S. W., Chang, S. X., and Yan, X. Y. (2018). Dry deposition of N has a major impact on surface water quality in the Taihu Lake region in southeast China. Atmos. Environ. 190, 1–9. doi: 10.1016/j.atmosenv.2018.07.017
van der Heide, T., Smolders, A. J., Rijkens, B. G., van Nes, E. H., van Katwijk, M. M., and Roelofs, J. G. (2008). Toxicity of reduced nitrogen in eelgrass (Zostera marina) is highly dependent on shoot density and pH. Oecologia 158, 411–419. doi: 10.1007/s00442-008-1155-2
van Diggelen, J. M. H., Smolders, A. J. P., Visser, E. J. W., Hicks, S., Roelofs, J. G. M., and Lamers, L. P. M. (2016). Differential responses of two wetland graminoids to high ammonium at different pH values. Plant Biol. 18, 307–315. doi: 10.1111/plb.12398
Wang, C., Zhang, S. H., Wang, P. F., Hou, J., Li, W., and Zhang, W. J. (2008). Metabolic adaptations to ammonia-induced oxidative stress in leaves of the submerged macrophyte Vallisneria natans (Lour.) Hara. Aquat. Toxicol. 87, 88–98. doi: 10.1016/j.aquatox.2008.01.009
Xian, L., Zhang, Y., Cao, Y., Wan, T., Gong, Y., Dai, C., et al. (2020). Glutamate dehydrogenase plays an important role in ammonium detoxification by submerged macrophytes. Sci. Total Environ. 722:137859. doi: 10.1016/j.scitotenv.2020.137859
Xiao, K. Y., Yu, D., and Wang, J. W. (2006). Habitat selection in spatially heterogeneous environments: a test of foraging behaviour in the clonal submerged macrophyte Vallisneria spiralis. Freshw. Biol. 51, 1552–1559. doi: 10.1111/j.1365-2427.2006.01590.x
Xie, P. (2006). Biological mechanisms driving the seasonal changes in the internal loading of phosphorus in shallow lakes. Sci. China Ser. D Earth Sci. 49, 14–27. doi: 10.1007/s11430-006-8102-z
Yu, Q., Wang, H. J., Wang, H. Z., Li, Y., Liang, X. M., Xu, C., et al. (2017). Does the responses of Vallisneria natans (Lour.) Hara to high nitrogen loading differ between the summer high-growth season and the low-growth season? Sci. Total Environ. 60, 1513–1521. doi: 10.1016/j.scitotenv.2017.05.268
Yu, Q., Wang, H. Z., Li, Y., Shao, J. C., Liang, X. M., Jeppesen, E., et al. (2015). Effects of high nitrogen concentrations on the growth of submersed macrophytes at moderate phosphorus concentrations. Water Res. 83, 385–395. doi: 10.1016/j.watres.2015.06.053
Yu, Q., Wang, H. Z., Xu, C., Li, Y., Ma, S. N., Liang, X. M., et al. (2018). Higher tolerance of canopy-forming Potamogeton crispus than rosette-forming Vallisneria natans to high nitrogen concentration as evidenced from experiments in 10 ponds with contrasting nitrogen levels. Front. Plant Sci. 9:1845. doi: 10.3389/fpls.2018.01845
Yuan, G. X., Fu, H., Zhong, J. Y., Cao, T., Ni, L. Y., Zhu, T. S., et al. (2015). Nitrogen/carbon metabolism in response to NH4+ pulse for two submersed macrophytes. Aquat. Bot. 121, 76–82. doi: 10.1016/j.aquabot.2014.12.001
Yuan, G. X., Fu, H., Zhong, J. Y., Lou, Q., Ni, L. Y., and Cao, T. (2016). Growth and C/N metabolism of three submersed macrophytes in response to water depths. Environ. Exp. Bot. 122, 94–99. doi: 10.1016/j.envexpbot.2015.09.009
Zhang, M., Wang, Z., Xu, J., Liu, Y., Ni, L., Cao, T., et al. (2011). Ammonium, microcystins, and hypoxia of blooms in eutrophic water cause oxidative stress and C-N imbalance in submersed and floating-leaved aquatic plants in Lake Taihu, China. Chemosphere 82, 329–339. doi: 10.1016/j.chemosphere.2010.10.038
Zhang, Y. L., Jeppesen, E., Liu, X. H., Qin, B. Q., Shi, K., Zhou, Y. Q., et al. (2017). Global loss of aquatic vegetation in lakes. Earth Sci. Rev. 173, 259–265. doi: 10.1016/j.earscirev.2017.08.013
Zhao, S. T., Yin, L. Y., Chang, F. Y., Olsen, S., Sondergaard, M., Jeppesen, E., et al. (2016). Response of Vallisneria spinulosa (Hydrocharitaceae) to contrasting nitrogen loadings in controlled lake mesocosms. Hydrobiologia 766, 215–223. doi: 10.1007/s10750-015-2456-1
Zhu, G., Yuan, C., Di, G., Zhang, M., Ni, L., Cao, T., et al. (2018). Morphological and biomechanical response to eutrophication and hydrodynamic stresses. Sci. Total Environ. 62, 421–435. doi: 10.1016/j.scitotenv.2017.11.322
Keywords: pond experiment, growth form, submersed macrophytes, ammonium, population development
Citation: Yu Q, Wang H, Wang H, Xu C, Liu M, Ma Y, Li Y, Ma S, Hamilton DP and Jeppesen E (2022) Effects of High Ammonium Loading on Two Submersed Macrophytes of Different Growth Form Based on an 18-Month Pond Experiment. Front. Plant Sci. 13:939589. doi: 10.3389/fpls.2022.939589
Received: 09 May 2022; Accepted: 14 June 2022;
Published: 14 July 2022.
Edited by:
Helena Freitas, University of Coimbra, PortugalReviewed by:
Peter Pal’Ove-Balang, Pavol Jozef Šafárik University in Košice, SlovakiaFernanda Fidalgo, University of Porto, Portugal
Copyright © 2022 Yu, Wang, Wang, Xu, Liu, Ma, Li, Ma, Hamilton and Jeppesen. This is an open-access article distributed under the terms of the Creative Commons Attribution License (CC BY). The use, distribution or reproduction in other forums is permitted, provided the original author(s) and the copyright owner(s) are credited and that the original publication in this journal is cited, in accordance with accepted academic practice. No use, distribution or reproduction is permitted which does not comply with these terms.
*Correspondence: Haijun Wang, d2FuZ2hhaWp1bkB5bnUuZWR1LmNu; Hongzhu Wang, d2FuZ2h6QGloYi5hYy5jbg==