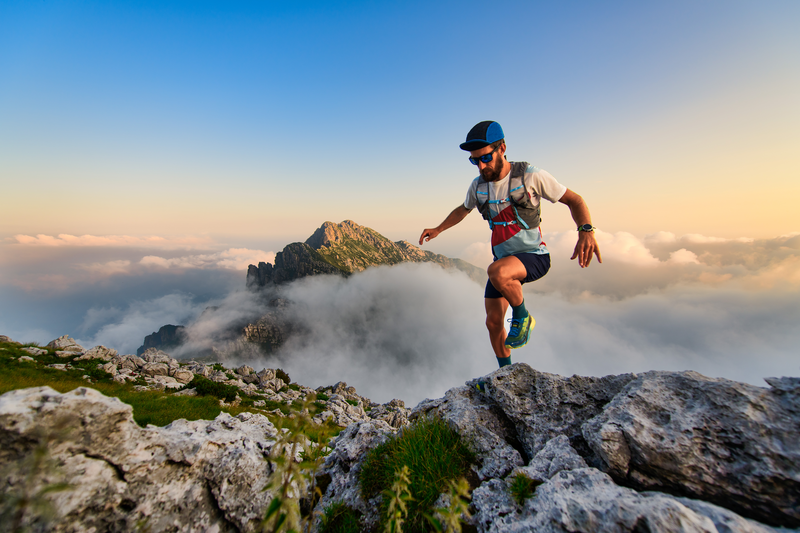
94% of researchers rate our articles as excellent or good
Learn more about the work of our research integrity team to safeguard the quality of each article we publish.
Find out more
ORIGINAL RESEARCH article
Front. Plant Sci. , 17 May 2022
Sec. Plant Pathogen Interactions
Volume 13 - 2022 | https://doi.org/10.3389/fpls.2022.867803
This article is part of the Research Topic Sustainable Strategies for the Management of Plant Parasitic Nematodes View all 5 articles
The scarce availability of efficient and eco-friendly nematicides to control root-knot nematodes (RKN), Meloidogyne spp., has encouraged research toward the development of bionematicides. Naphthoquinones, juglone (JUG) and 1,4-naphthoquinone (1,4-NTQ), are being explored as alternatives to synthetic nematicides to control RKN. This study expands the knowledge on the effects of these natural compounds toward M. luci life cycle (mortality, hatching, penetration, reproduction). M. luci second-stage juveniles (J2)/eggs were exposed to each compound (250, 150, 100, 50, and 20 ppm) to monitor nematode mortality and hatching during 72 h and 15 days, respectively. Tomato seedlings were then inoculated with 200 J2, which had been exposed to JUG/1,4-NTQ for 3 days. The number of nematodes inside the roots was determined at 3 days after inoculation, and the final population density was assessed at 45 days after inoculation. Moreover, the potential mode of action of JUG/1,4-NTQ was investigated for the first time on RKN, through the assessment of reactive oxygen species (ROS) generation, acetylcholinesterase (AChE) in vitro inhibitory activity and expression analysis of ache and glutathione-S-transferase (gst) genes. 1,4-NTQ was the most active compound, causing ≥50% J2 mortality at 250 ppm, within 24 h. At 20 and 50 ppm, hatching was reduced by ≈50% for both compounds. JUG showed a greater effect on M. luci penetration and reproduction, decreasing infection by ≈80% (50 ppm) on tomato plants. However, 1,4-NTQ-induced generation of ROS and nematode vacuolization was observed. Our study confirms that JUG/1,4-NTQ are promising nematicidal compounds, and new knowledge on their physiological impacts on Meloidogyne was provided to open new avenues for the development of innovative sustainable nematicides.
To date, with 105 valid species described, the genus Meloidogyne [root-knot nematodes (RKN)] are among the top 10 plant-parasitic nematodes (PPN) in plant pathology with major ecological and economic impact worldwide (Jones et al., 2013; Ghaderi and Karssen, 2020). RKN are able to parasitize almost any species of plants, and due to their wide distribution in most agricultural areas all over the world, it seems understandable to estimate higher economic losses due to Meloidogyne species. Despite the lack of actual and global data on their real economic impact, Hussey and Janssen (2002) reported that every year, RKN are responsible for 5% of the crop losses, but this value is probably underestimated because plant symptoms caused by RKN are non-specific and losses are often attributed to other causes.
M. arenaria, M. hapla, M. incognita, and M. javanica are regarded as the most common RKN species; however, other species with apparently restricted distribution are considered species of emerging importance. M. luci is included in the Alert List of Pests of the European and Mediterranean Plant Protection Organization since 2017, as it represents a threat to the production of several important crops in European countries, where this species has been detected (Greece, Italy, Portugal, Slovenia, Turkey), but also in other parts of the world (Brazil, Chile, Guatemala, and Iran) (EPPO, 2017, 2021). Although M. luci has already been detected associated with economically important crops, no data are available on its real impacts on the quality and quantity of crop production. However, host suitability studies carried out in pots have shown that significant economic losses can be predicted due to M. luci (Maleita et al., 2018, 2022; Aydinli et al., 2019; Sen and Aydinli, 2021). In Portugal, M. luci was found the parasitizing roots of potato (Solanum tuberosum), tomato (S. lycopersicum), the ornamental plant Cordyline australis, and the weed Oxalis corniculata (Maleita et al., 2018; Santos et al., 2019; Rusinque et al., 2021).
Many strategies have been applied in RKN management. For the last 50 years, control of RKN largely depend on the use of synthetic nematicides, readily available in the market, rapid-acting, and highly reliable (Zasada et al., 2010; Desaeger et al., 2017). Nevertheless, many nematicides are being banned from the market or their use restricted (Nyczepir and Thomas, 2009; Johnson et al., 2012; Ebone et al., 2019). Concerns about the impacts of chemical nematicides on human health and the environment led many regulatory agencies, such as European Food Safety Authority (EFSA) and US Environmental Protection Agency (EPA), to strengthen pesticide regulation (European Commission, 2009a,b; Kopits et al., 2014). Today, the need for searching environmental friendly nematicides, deriving from plant extracts, posing low risks to humans or animals, is widely consensual (Haydock et al., 2006; Chen et al., 2020).
Several plant secondary metabolites (alcohols, aldehydes, fatty acid derivatives, terpenoids, and phenolics) have been identified as nematicidal compounds and/or with effects on second-stage juveniles (J2) hatching, paralysis and nematode root attraction, penetration, and reproduction (Aoudia et al., 2012; Ntalli and Caboni, 2012; Caboni and Ntalli, 2014; Aissani et al., 2015; Lu et al., 2017; Sikder and Vestergård, 2020).
Naphthoquinones (NTQ) are naturally occurring compounds in several families of plants, such as Ancistrocladaceae, Avicenniaceae, Balsaminaceae, Bignoniaceae, Boraginaceae, Dioncophyllaceae, Droseraceae, Ebenaceae, Gentianaceae, Iridaceae, Juglandaceae, Plumbaginaceae, Scrophulariaceae, and Verbenaceae, algae, fungi, lichens, and animals, such as beetles and arachnids (Raspotnig et al., 2005; Pankewitz and Hilker, 2008; Babula et al., 2009). Among NTQ, juglone (5-hydroxynaphthalene-1,4-dione; JUG) and 1,4-naphthoquinone (naphthalene-1,4-dione; 1,4-NTQ) were shown to have nematicidal activity, among other properties (insecticide, herbicidal, anti-inflammatory, antibacterial, antifungal, anticancer, and cytotoxic properties) and thus are promising compounds to develop novel, natural, and effective nematicides, with lower environmental half-lives than traditional nematicides (Babula et al., 2009; Fischer et al., 2012; Esteves et al., 2017; Maleita et al., 2017; Wang et al., 2017; Cha et al., 2019; Laxmikant, 2019; Aminin and Polonik, 2020; Islam and Widhalm, 2020). However, knowledge on the effects of these compounds through RKN life cycle and their potential mode(s) of action are still unknown. A possible mode of action is the inhibition of the acetylcholinesterase (AChE) enzyme, which is responsible for the termination of impulse transmissions at cholinergic synapses within the nervous system by rapid hydrolysis of the neurotransmitter acetylcholine. When it is inhibited, there is an excessive accumulation of acetylcholine, leading to nematode hyperactivity, incoordination, and finally paralysis and death (Piotte et al., 1999). Considering this mechanism of action, AChE is the target of some pesticides, which inhibit the enzyme, such as carbamates and organophosphates (Al-Rehiayani, 2008). Therefore, assays should be conducted to analyze nematicidal effect of JUG and 1,4-NTQ and possible action on AChE enzyme. The in vitro inhibition potency of some widely used nematicides has been already evaluated to control PPN, such as M. javanica, Heterodera avenae, and Tylenchulus semipenetrans, pathogens that are damaging to a wide range of crops (Islam and Widhalm, 2020).
Another hypothesis is the generation of reactive oxygen species (ROS) as a consequence of the reaction of quinones with glutathione (GSH) (Inbaraj and Chignell, 2004). ROS are strong oxidizing agents that are associated with several toxic effects playing a significant role in cell death (Widhalm and Rhodes, 2016).
The objectives of the study were as follows: (1) to evaluate the effects of JUG and 1,4-NTQ on the in vitro mortality, hatching, penetration, and reproduction of M. luci on tomato plants and (2) to infer their mode of action through the assessment of ROS generation, AChE in vitro inhibitory activity and expression analysis of ache and glutathione-S-transferase (gst) genes.
Pure bioactive compounds, JUG and 1,4-NTQ (Sigma-Aldrich, purity >97 and ≥96.5% w/w, respectively), were solubilized in Tween® 80 (Sigma-Aldrich, suitable for cell culture) at 2,500 ppm aqueous solution to obtain final concentrations ranging from 20 to 2,000 ppm, depending on the bioassay. Each concentration of bioactive compounds was prepared individually. Water and Tween® 80 were used as the controls in mortality, hatching, penetration, and reproduction bioassays. All bioassays were repeated two times, except penetration and reproduction bioassays. Solutions were stirred for 3 days, at 37°C, at 100 rpm, away from light.
An isolate of M. luci obtained from potato roots in Sepins (Cantanhede), Coimbra, Portugal (Maleita et al., 2018) was maintained on tomato cv. Coração-de-Boi, in pots containing sterilized sandy loam soil and sand (1:1 v/v), at 25 ± 2°C in a growth chamber. The isolate identification was confirmed by esterase phenotype analysis (Maleita et al., 2018).
M. luci egg masses were handpicked from infected roots and placed in a hatching chamber. Hatched J2 from the first 24 h were discarded and subsequent J2, from the second 24 h, were collected. From these, 20 nematodes were handpicked individually into excavated glass blocks containing 1 ml of each JUG/1,4-NTQ concentration (20, 50, 100, 150, and 250 ppm). Glass blocks were maintained in a moist chamber, in the dark, at 22°C. J2 mortality was monitored at 6, 12, 24, 48, and 72 h after exposure. At each observation time, nematodes not showing movements when touched with a bristle were transferred to water and considered dead if they still failed to react. Each treatment consisted of four replicates.
M. luci egg masses were collected from infected tomato roots and eggs were extracted using 0.52% sodium hypochlorite (NaOCl; Acros Organics B.V.B.A.) solution (Hussey and Barker, 1973). A total of fifty eggs (≈100 μl) were transferred into a 10-μm sieve placed in a glass block, and 1.5 ml of each JUG/1,4-NTQ concentration (20, 50, 100, 150, and 250 ppm) was added. The concentration of JUG/1,4-NTQ 20 and 50 ppm solutions was confirmed by high-performance liquid chromatography (HPLC) (data not shown; Seabra et al., 2019). Nematode hatching was monitored daily for 3 days and afterwards at 2-day intervals for 15 days, and it was assumed that NTQ activity was preserved during the tested period. Each treatment consisted of four replicates.
J2 were obtained as referred above for the mortality bioassay: hatched J2 from the second 24 h and thereafter were collected and stored at 4°C, until a maximum of 3 days. Before inoculation, M. luci J2 were exposed for 3 days to JUG/1,4-NTQ solutions of 20 and 50 ppm; water and 2,500 ppm Tween® 80 were included as controls. A number of forty-eight tomato cv. Coração-de-Boi plants (3 weeks old) were transferred to pots (50 cm3) containing autoclaved sandy loam soil, sand, and substrate (1:1:1, v/v), and each was inoculated with 200 M. luci J2 (initial nematode population density, Pi). Only the mobile J2 were considered to define the volume of nematode suspension to be inoculated. Then, 3 days after inoculation, four roots/treatment were removed, roots were washed, stained with fuchsin acid (Sigma-Aldrich, purity ≥70%, w/w), and the number of nematodes inside the roots was recorded. The remaining five tomato plants/treatment were transferred into new pots (150 cm3). At 45 days after inoculation, the plants were harvested, and the root systems were washed carefully. The number of galls and egg masses per plant was recorded and categorized using a 0–5 scale (0 = no galls, 1 = 1–2, 2 = 3–10, 3 = 11–30, 4 = 31–100, 5 ≥ 100 galls) (Taylor and Sasser, 1978). Eggs were extracted from each root system using a 1% NaOCl solution (Hussey and Barker, 1973), the final nematode population (Pf) was determined, and the reproduction factor (Rf = Pf/Pi) was calculated.
Hydrochloric acid (HCl, 37%, p.a.) was purchased from Carlo Erba. AChE (Type VI-S, 500 U/mg protein), 5,5-dithiobis[2-nitrobenzoic acid] (DTNB, ≥98%), acetylthiocholine iodide (AChI, ≥98%), ethanol (≥99.8%, p.a.), and tris(hydroxymethyl)aminomethane (Tris Buffer) were obtained from Sigma-Aldrich.
AChE inhibitory activity in vitro assay was performed according to the modified procedure described by Ellman et al. (1961), and as previously carried out by Gaspar et al. (2020a,b).
Briefly, DTNB (3 mM, 500 μl), AChI (15 mM, 100 μl), Tris–HCl buffer at pH 8 (50 mM, 275 μl), and the JUG/1,4-NTQ (100 μl) were added to a 1-ml cuvette. Compounds JUG/1,4-NTQ were solubilized in ethanol and Tris-HCl buffer (50:50, v/v) and homogenized. The concentrations were based on preliminary assays and were 100, 250, 500, 1,000, and 2,000 ppm for JUG and 200, 250, 500, 1,000, and 2,000 ppm for 1,4-NTQ. The enzyme AChE (0.28 U/ml, 25 μl) was then added to start the reaction, which was monitored for 5 min, at 25°C and 405 nm (UV–vis spectrophotometer, Jasco, Model V650) for the determination of the reaction rate. The AChE activity was calculated as a percentage of this velocity compared to the control (ethanol:Tris-HCl buffer, 50:50 v/v), and the inhibitory activity was calculated by subtraction. The blank was performed for each sample and concentration, and using all reagents, except the enzyme to reduce the possible NTQ interference in the measured absorbance. For each sample, the assay was conducted in triplicate, with five concentrations.
Additional studies with several concentrations of the surfactant Tween® 80 (100, 2,500, and 5,000 ppm) were also conducted to understand about its possible action on AChE activity, since it is used to prepare the JUG/1,4-NTQ solutions used in nematode bioassays.
Production of ROS was evaluated by microscopic observation. M. luci J2 were incubated at 22°C for 3 days to JUG/1,4-NTQ at 20, 50, 100, 150, and 250 ppm. Water and Tween® 80 were also included as controls. After exposure, nematodes were washed two times with sterilized water, pelleted (336 g for 2 min), and incubated for 40 min in 2′,7′-dichlorofluorescein diacetate 20 μM (Sigma-Aldrich) at 20°C. Then, the nematodes were washed two times with sterile water, transferred to a 24-well plate, and paralyzed by the addition of 10 mM sodium azide (Sigma-Aldrich) (Sun et al., 2017; Rangsinth et al., 2019). Nematodes were randomly photographed using a Zeiss Observer Z.1 inverted microscope (Carl Zeiss) equipped with an AxioCam HRm camera and Zen Blue 2012 software, using a N-Achroplan 5 × /0.15 or a LD Plan-Neofluar 40 × /0.6. All conditions within an experiment were processed simultaneously and imaging settings (exposure time) were conserved.
The expression of ache and gst genes encoding an enzyme responsible for the primary termination of cholinergic nerve impulse transmission and an enzyme related to protection against the plant defense, respectively, were evaluated.
Approximately 20,000 J2 were incubated in JUG/1,4-NTQ 20 ppm, at 25°C also in the dark. Water and Tween® 80 at 2,500 ppm were used as controls. After 24 and 72 h, J2 were concentrated by centrifugation for 2 min at 336 g, washed three times with sterilized water and two times with RNAse-free water, and stored at −80°C, until RNA extraction.
Total RNA were extracted from J2 of M. luci and isolated with TRIzol reagent (Invitrogen). Nematodes were homogenized in TRIzol reagent (Sigma-Aldrich) through six freeze-thawing cycles in TyssueLyser (liquid nitrogen; 37°C; and 30 s at 50 Hz, respectively). Afterward, the RNA was purified using the Direct-zol RNA kit (Zymo research), and any remaining DNA was digested using the TURBO DNA-free kit (Ambion). The concentration and purity of the RNA were determined in a Nanodrop 2000c spectrophotometer (ThermoFisher), and the samples were stored at −80°C.
The RNA was converted into cDNA by reverse transcription (RT) using the iScript™ Reverse Transcription Supermix Kit (Bio-Rad Laboratories) in a volume of 20 μl, according to the instructions, and the samples were stored at −20°C, until polymerase chain reaction (PCR) analysis. Primer sets used are described in Table 1. The GST reverse primer (GST_R) was designed after amplification, cloning, and sequencing of gst M. luci fragment with MIHA-GSTS-1f/r primers (Table 1) (Duarte, 2014). PCR were performed in a 25 μl volume containing 1× Taq reaction buffer, 1.5 mM MgCl2, 0.2 mM dNTPs, 0.4 μM each primer, 2.5 U of Taq DNA polymerase (Bioline), and 25 ng of nematode cDNA as a template. An internal control—β-actin—was included. The amplifications were carried out in an MJ Mini Thermal Cycler (Bio-Rad) using the following conditions: an initial denaturation at 94°C for 3 min; followed by 30 cycles of denaturation at 94°C for 30 s, annealing at 52 and 53°C for 30 s for ache and gst, respectively, extension at 72°C for 30 s, and a final extension for 10 min at 72°C. PCR were analyzed on a 1% agarose gel electrophoresis in 1× Tris-borate EDTA buffer stained with GreenSafe (NZYTech).
Data on M. luci J2 mortality were converted to percentage cumulative mortality, corrected by Schneider Orelli's formula with reference to water, used as experimental control:
The effects of JUG and 1,4-NTQ on mortality, hatching, penetration, and reproduction were compared in one-way analysis of variance (ANOVA) followed by post-hoc Fisher's least significant difference (LSD) statistical test. Data on J2 mortality, during exposure in JUG, and J2 penetration were transformed (logarithmic and square root, respectively) to fulfill the assumptions of ANOVA (normality and variance homogeneity). Even after transformation of data on effect of 1,4-NTQ on J2 mortality, the assumptions of ANOVA were not fulfilled. Nonetheless, data were compared in ANOVA followed by post-hoc Fisher's LSD statistical test. A parametric test seemed to be more robust than a non-parametric test. Statistical analyses of the data were performed using Statsoft Statistica, version 7 for Windows.
Data on J2 mortality (48- and 72-h observations) and hatching inhibition (15-day observation) were subjected to Probit analysis (Finney, 1971), using PriProbit 1.63 software, and the lethal concentrations causing 50% mortality and 50% hatching inhibition (LC50) calculated. The concentration of bioactive compound able to inhibit 50% of the AChE activity (IC50) was also computed.
According to our experiments, M. luci mortality was significantly affected by the exposure to JUG and 1,4-NTQ (p > 0.05), for concentrations ≥100 ppm, and, in general, increases as compounds concentration increased. Nonetheless, compounds were not equally effective (Figure 1). 24 h after exposure to NTQ, JUG only induced 10% mortality, whereas 46% mortality was observed in 1,4-NTQ. JUG induced 32–70% mortality within 48 h, and 1,4-NTQ induced 61–96%, at 150 and 250 ppm, respectively (Figure 1). At 72 h after exposure, 1,4-NTQ induced 99% mortality and JUG 87%, at 250 ppm. A similar effect was detected for compounds at 100 ppm (38 and 40%, respectively) (Figure 1).
Figure 1. Corrected cumulative mortality (%) of Meloidogyne luci second-stage juveniles exposed to different concentrations of juglone (JUG, A) and 1,4-naphthoquinone (1,4-NTQ, B). Data are an average of four replicates, and bars represent standard errors. Average followed by the same lower case, at the same exposure time, do not differ significantly (p > 0.05) according to the Fisher's LSD test. *At 6 and 12 h after exposure, no significant differences were found. **IV and VI were significantly different from I, II, and III; V does not differ significantly from all treatments.
Estimated values of LC50, at 48 h after exposure, were 174.45 and 131.76 ppm to JUG and 1,4-NTQ, respectively, and 127.75 and 111.45 ppm at 72 h after exposure (Table 2). Therefore, 1,4-NTQ was more effective than JUG to induce M. luci mortality. At 24 h of exposure and for compound concentrations ≥100 ppm, most J2 remained alive but were immobile and only recovered the movement after being touched with a bristle. Dead nematodes showed, in general, a straight shape and vacuoles formation (Figures 5, 6).
Table 2. Estimated values of lethal concentration (ppm) necessary to result in 50% Meloidogyne luci second-stage juveniles' mortality and hatching inhibition (LC50), after exposure to juglone (JUG) and 1,4-naphthoquinone (1,4-NTQ).
M. luci mortality in Tween® 80 (2,500 ppm), used for JUG and 1,4-NTQ solubilization, was not significantly different from that observed in water (control) and in the two NTQ, at 20 and 50 ppm, within 72 h after exposure (Figure 1).
Both compounds have similar effects on hatching, inhibiting ≥49% J2 hatching within 15 days. At 20 and 50 ppm, J2 hatching was reduced by ~50% for both compounds reaching 96% at 250 ppm (Figure 2). In controls, water and Tween® 80 at 2,500 ppm (surfactant used to NTQ solubilization), 100% of M. luci J2, were hatched.
Figure 2. Meloidogyne luci second-stage juveniles (J2; %) hatched for 15 days. Eggs were soaked in water, 2,500 ppm Tween® 80 solution, and juglone and 1,4-naphthoquinone at different concentrations. Each bar represents the average ± standard deviation of four replicates and bars denoted by different letters differ significantly at p > 0.05, according to the Fisher's LSD test.
Estimated values of LC50, at 15 days after exposure, were 31.81 and 28.72 ppm to JUG and 1,4-NTQ (Table 2).
Juglone arose as most efficient than 1,4-NTQ restricting significantly nematode root penetration and reproduction at 50 ppm. At this concentration, the number of J2 found inside the roots decreased ~69% for 1,4-NTQ and 80% for JUG when compared to water control. Penetration in water control was not significantly different from that observed in the Tween® 80 solvent solution and in both bioactive compounds at 20 ppm (Figure 3).
Figure 3. Number of Meloidogyne luci second-stage juveniles (J2) found inside tomato cv. Coração-de-Boi roots, 3 days after inoculation. Before inoculation, J2 were soaked for 3 days in water, 2,500 ppm Tween® 80 solution, and juglone and 1,4-naphthoquinone at 20 and 50 ppm. Each bar represents the average ± standard deviation of four replicates and bars denoted by different letters differ significantly at p > 0.05, according to the Fisher's LSD test.
In terms of M. luci reproduction, 45 days after inoculation, in the water and Tween® 80 (2,500 ppm) controls, tomato cv. Coração-de-Boi root systems were highly infected with GI = 5 and Rf values of 268.5 and 344.8, respectively (Table 3). No significant differences were found between the controls. M. luci J2 developed and reproduced, after exposure to the bioactive compounds at 20 and 50 ppm, but the number of egg masses, Pf, and eggs/egg masses varied among treatments (Table 3). Although no significant effects were observed between the M. luci reproduction in water control and after exposure to 20 ppm of both compounds, the number of egg masses and Pf across treatments indicated significant differences between the bioactive compounds at 50 ppm and both controls (p > 0.05; Table 3). The number of eggs/egg masses after exposure to JUG 50 ppm decreased significantly, in ~32–33% when compared to water and Tween® 80 (2,500 ppm) controls (p > 0.05; Table 3).
Table 3. Number of galls (G) and egg masses (EM) and respective indices (GI, EMI), final population density (Pf), number of eggs/EM, and reproduction factor (Rf) of Meloidogyne luci, 45 days after inoculationa.
Considering the effect of JUG and 1,4-NTQ on AChE activity, both compounds had similar inhibition of this enzyme activity, for concentrations over 200 ppm, and no effects are expected at 20 ppm. Results are expressed as IC50 values and AChE inhibitory curves are presented in Figure 4. The ethanol:Tris-HCl (50:50, v/v) buffer solution did not inhibit the AChE activity, and therefore, it was used for the solubilization of compounds.
Figure 4. Acetylcholinesterase (AChE) inhibitory curves and respective R2 values obtained for juglone (A) and 1,4-naphthoquinone (B).
For JUG, the IC50 was 1,097 ± 50 ppm, while for 1,4-NTQ, it was 1,274 ± 42 ppm. Considering the AChE inhibitory curves and the IC50 values, JUG was slightly more active than 1,4-NTQ, and both compounds showed inhibition of this enzyme activity, between 200 and 2,000 ppm, with values close to 100% inhibition for JUG at the highest concentration (Figure 4).
Additional experiments were conducted to analyze the possible inhibitory effect of Tween® 80 on AChE activity, and about 4 and 10% inhibition were observed at 2,500 and 5,000 ppm, respectively. This means that the Tween® 80 (2,500 ppm) has very low inhibitory activity on AChE, and it may be used in the assays, with caution and always performing the control (Supplementary Material).
After nematode staining with 2′,7′-dichlorofluorescein diacetate (ROS indicator) and fluorescence monitoring, no J2 treated with water or Tween® 80 at 2,500 ppm (controls) generated ROS fluorescence or vacuoles development (Figures 5, 6). M. luci J2 treated with JUG did not exhibit ROS fluorescence but the formation of small and multiple vacuoles associated with mortality was observed whereas J2 treated with 1,4-NTQ exhibited ROS fluorescence for concentrations ≥100 ppm, and multiple giant vacuoles in the central and tail region were detected associated with nematode death. Several J2 exposed to 100 ppm 1,4-NTQ remained mobile and did not show vacuoles or ROS production (Figure 6,a).
Figure 5. In vitro production of reactive oxygen species in Meloidogyne luci second-stage juveniles, after exposure for 3 days to juglone (JUG) and 1,4-naphthoquinone (1,4-NTQ) at different concentrations. *Similar results were obtained for referred concentrations; images presented correspond to the higher concentration. Scale bars = 100 μm.
Figure 6. Effects of juglone (JUG) and 1,4-naphthoquinone (1,4-NTQ) on reactive oxygen species production in Meloidogyne luci second-stage juveniles' mortality. Nematodes were exposed to JUG and 1,4-NTQ at 100 and 250 ppm for 3 days. At 1,4-NTQ 100 ppm, some nematodes remained mobile (a) and others were dead (b). Scale bars = 50 μm.
Reverse transcription polymerase chain reaction, using the primers for ache and gst genes, displayed the expression of these genes in M. luci J2. Amplification of the β-actin gene was used as an internal control (Figure 7). The specific band for the ache gene has a molecular weight of ~150 bp and for the gst gene of 100 bp (Figure 7). The cDNA fragments of ache have a clear difference in band intensity after 24 h of J2 exposure to JUG and 1,4-NTQ and after 72 h of exposure to 1,4-NTQ when compared to those obtained in water. Tween® 80 (2,500 ppm) itself seems to slightly affect the ache gene expression. For gst and β-actin genes, the cDNA fragments were equally amplified in all treatments (Figure 7).
Figure 7. Expression of the genes acetylcholinesterase (ache), glutathione-S-transferase (gst) and β-actin by PCR amplification of cDNA from Meloidogyne luci second-stage juveniles after exposure for 24 h (1) and 72 h (2) to 1,4-naphthoquinone (N) and juglone (J) 20 ppm. Water (H) and Tween® 80 at 2,500 ppm (T) were used as controls. M - DNA Marker (HyperLadder II; Bioline).
JUG and 1,4-NTQ were shown to be active against M. luci, inducing J2 mortality and inhibited hatching, penetration, and reproduction on tomato. Previously, few studies have been conducted to evaluate the in vitro and semi-in vivo nematicidal activity of these compounds against PPN (Mahajan et al., 1992; Dama, 2002; Esteves et al., 2017; Maleita et al., 2017; Cha et al., 2019; Laxmikant, 2019). According to Mahajan et al. (1992), JUG revealed a high degree of nematicidal activity promoting 100% M. incognita mortality at 1,100 ppm, within 48 h. Later, it was demonstrated that a concentration of 20 ppm JUG was efficient against M. javanica causing 97.94% mortality within 24 h (Dama, 2002). More recently, the nematicidal activity of JUG and 1,4-NTQ against the RKN M. hispanica, the root lesion nematode Pratylenchus thornei, and the pinewood nematode Bursaphelenchus xylophilus was proven (Esteves et al., 2017; Maleita et al., 2017; Cha et al., 2019). JUG and 1,4-NTQ 250 ppm caused 100% mortality in M. hispanica J2, after 24 and 12 h of exposure, respectively. 1,4-NTQ was most effective than JUG at 50 ppm, causing 42% M. hispanica J2 mortality (Maleita et al., 2017). Both compounds also induced >90% B. xylophilus mortality at 250 ppm, within 6 h and 100% P. thornei mortality within 24 h at 500 ppm (Esteves et al., 2017; Cha et al., 2019). Only Maleita et al. (2017) and Cha et al. (2019) studied the effects of these NTQ through semi-in vivo assays demonstrating the potential applications of these compounds in agriculture and forest industry. Incubation of M. hispanica J2 in a Juglans nigra extract, enriched in JUG and 1,4-NTQ, affected significantly nematode root penetration, and a reduction on reproduction was perceived (Maleita et al., 2017). The population of B. xylophilus decreased significantly, in Pinus thunbergii blocks, after treatment with JUG (Cha et al., 2019).
According to our experiments, 1,4-NTQ was most active than JUG on M. luci J2 mortality, despite being less effective against M. hispanica in the previous experiments (Maleita et al., 2017). Differences in the results obtained may be due to the use of a different surfactant (Triton X-100 vs. Tween® 80) to solubilize the bioactive compounds (Maleita et al., 2017) or a different sensitivity of these RKN species to the compounds. M. incognita isolates were reported as more sensitive to fluensulfone than M. javanica, whereas M. javanica was more sensitive to fluopyram, with a significant difference in median lethal concentrations (Oka and Saroya, 2019).
Both compounds have similar effects on hatching, but JUG arose as most efficient than 1,4-NTQ restricting significantly nematode root penetration and reproduction at 50 ppm. The NTQ, JUG and 1,4-NTQ, can be obtained from different plant species, such as Pterocarya fraxinifolia, Carya spp., Lomatia spp., Caesalpinia sappan, Eleutherine palmifolia, among others, and are found at higher concentrations in Juglans sp. tissues, depending on processing measures and solvent used for extraction (Borazjani et al., 1985; Lee and Lee, 2006; Solar et al., 2006; Jakopic et al., 2007; Cosmulescu et al., 2010, 2011; Widhalm and Rhodes, 2016; Maleita et al., 2017; Deans et al., 2018; Mahdavi et al., 2019; Annisa et al., 2020; Medic et al., 2021a,b). For example, Maleita et al. (2017) reported concentrations of 49.4 and 36.8 mg/g of extract of JUG and 1,4-NTQ, respectively, from in natura J. nigra with effects on M. hispanica J2 mortality and penetration; and McKenry and Anwar (2003) stated a reduction of 75% on M. incognita population, compared to the phenamiphos, after treatment of planted grapevines with a Juglans spp. tea.
Nowadays, pesticide registration is a very complex and highly regulated process. The risk assessment of JUG and 1,4-NTQ on the soil environment, which is an important source of concern for soil-applied nematicides, has been assessed previously for 1,4-NTQ (Chelinho et al., 2017). Although a dose–response was observed in plants (Zea mays and Brassica napus), non-target nematodes, and other soil invertebrates (Eisenia andrei, Folsomia candida, Enchytraeus crypticus), results showed that a concentration of ≈20 mg/kg 1,4-NTQ is likely to be environmentally safe (Chelinho et al., 2017).
Several studies have also been developed and reported the effect of JUG on the growth and development of several economically important plants, including aubergine (Solanum melongena), cabbage (Brassica oleracea), corn (Zea mays), cucumber (Cucumis sativus), lettuce (Lactuca sativa), pepper (Capsicum annuum), and tomato, to concentrations between 10 μM and 1 mM (Kocaçaliskan et al., 2019; Islam and Widhalm, 2020). For tomato crop, with potential yield losses of 25–100% due to RKN (Seid et al., 2015), concentrations of 1 mM JUG (Kocaçaliskan and Terzi, 2001) and ≥192 mg/Kg of JUG/1,4-NTQ (Maleita et al., unpublished results) caused a decrease in seed germination and seedling growth. The available results lead to consider that the JUG and 1,4-NTQ concentration needed to reduce M. luci penetration and reproduction (50 mg/kg) may be lower than the concentrations that negatively impact tomato development, and no significant effects are expected on non-target plants and soil organisms, including non-target nematodes.
To further develop the potential use of JUG and 1,4-NTQ as natural product-based nematicides, it is essential to improve the knowledge about the bioactive compound mode(s) of action. According to Inbaraj and Chignell (2004), the cytotoxicity of quinones is due to a redox cycling and reaction with GSH, resulting in the generation of ROS and decreasing the GSH intracellular levels which leads to significant overexpression of gst gene, respectively (Sytykiewicz, 2011). Glutathione transferases are a large family of enzymes involved in detoxification metabolism limiting oxidative damage of cellular macromolecules. These enzymes, produced by several organisms, are secreted by RKN during parasitism, protecting the parasite against ROS, but transcripts of the gene are also found in RKN J2 (Dubreuil et al., 2007).
The 1,4-NTQ has a general biological tendency to accept electrons originating highly reactive and unstable species that can be then auto-(re)oxidized, by molecular oxygen or by other chemical species, leading to the formation of the original NTQ and of highly ROS (Widhalm and Rhodes, 2016). Nevertheless, JUG may have pro- or antioxidant characteristics depending on the concentration, and generation of ROS can be not reported at lower concentrations due to the antioxidant activities of the compound (Chobot and Hadacek, 2009; Jha et al., 2015; Ahmad and Suzuki, 2019).
The vacuolization of M. luci J2 after exposure to 1,4-NTQ and the lack of fluorescence of nematodes exposed to JUG observed in this study suggest that the mode of action of the two compounds is probably different at 20 ppm. The 1,4-NTQ induced the formation of ROS and the development of multiple fused giant vacuoles inside nematodes; however, the transcriptional activity of gst was similar to the control. Glutathione-S-transferases are a large family of enzymes; therefore, further gene expression studies on a broader range of gst are needed to clarify the role of these enzymes on the metabolization of reactive compounds. Treatment with JUG 20 ppm induced the formation of multiple and small vacuoles, but no ROS formation was detected. Results may be related to the pro-antioxidant activity of JUG, which impedes the generation of ROS at lower concentrations. Consecutively, JUG may not influence GSH in RKN, and thus not inducing an overexpression of the gst gene.
Considering the effect of JUG and 1,4-NTQ on AChE activity, both compounds had similar inhibition of this enzyme activity, for concentrations over 200 ppm, and no effects are expected at 20 ppm. At a molecular level, a decrease in intensity of ache cDNA fragments at 24 h was found in nematodes exposed to 20 ppm JUG and 1,4-NTQ, compared to respective water control, which may indicate that, although at low concentrations of these compounds it is not possible to observe biological changes, the expression of these nematode genes seems to be affected. AChE is responsible for nematode nerve impulse transmission, and it is the predominant target of chemical nematicides used in agriculture against PPN, reducing the parasitic ability of the nematodes and impairing the life cycle completion (Combes et al., 2001; Cui et al., 2017). The results reveal that the 1,4-NTQ and JUG compounds slightly affect direct or indirectly nematode nerve impulse transmission, which was verified by J2 immobility, reacting only after being touched with a bristle. Nevertheless, multiple molecular forms of AChE were found in Meloidogyne spp., which specific functions were not identified in mutation studies, suggesting an overlapping function of the different classes (Chang and Opperman, 1991).
Therefore, the mode of action of these compounds on M. luci should be further investigated. Future studies on the M. luci transcriptome analysis after exposure to these NTQ will help to better understand the mode of action of these compounds on RKN.
In conclusion, the bioactive compounds JUG and 1,4-NTQ are very promising and attractive alternatives to the use of synthetic nematicides to control PPN, such as the RKN M. luci. The negative impact observed on hatching, root penetration, and reproduction of M. luci after exposure to JUG/1,4-NTQ at 50 ppm suggests that these compounds may be helpful in reducing the M. luci population in soil, contributing to limit the crop damage caused by this nematode to a level economically acceptable. Although 1,4-NTQ was shown to be most active than JUG on M. luci J2 mortality, low concentrations of JUG were efficient in restricting significantly nematode root penetration and reproduction. Thus, research on the development of nematicide formulations containing mixtures of both NTQ should be considered in the future. The development of bionematicide product based on NTQ compounds also opens the opportunity for valorization of agro-industrial byproducts, through the extraction of these compounds from walnut husk residues.
The original contributions presented in the study are included in the article/Supplementary Material, further inquiries can be directed to the corresponding author.
CM, IE, MB, JF, and MG: methodology. CM and HS: funding acquisition. CM: writing—original draft. All authors: contributed to the conceptualization, analysis, investigation, reviewing, and editing and read and contributed to the article and approved the submitted version.
This research was carried out at the R&D Units Chemical Process Engineering and Forest Products Research Centre (CIEPQPF) and Centre for Functional Ecology—Science for People and the Planet (CFE), with references UIDB/00102/2020, UIDP/00102/2020 (CIEPQPF), and UID/BIA/04004/2020 (CFE), respectively, financed by Fundação para Ciência e a Tecnologia (FCT)/MCTES through national funds (PIDDAC) and at the European Regional Development Fund (FEDER) through the Programa Operacional Factores de Competitividade 2020 (COMPETE 2020) and national funds through FCT and FCT/MEC (PIDDAC), under contracts POCI-01-0145-FEDER-029392 and Centro-01-0145-FEDER-000007, CEECIND/02082/2017 (IE), CEECIND/000527/2017 (MG), and by Instituto do Ambiente, Tecnologia e Vida.
The authors declare that the research was conducted in the absence of any commercial or financial relationships that could be construed as a potential conflict of interest.
All claims expressed in this article are solely those of the authors and do not necessarily represent those of their affiliated organizations, or those of the publisher, the editors and the reviewers. Any product that may be evaluated in this article, or claim that may be made by its manufacturer, is not guaranteed or endorsed by the publisher.
The Supplementary Material for this article can be found online at: https://www.frontiersin.org/articles/10.3389/fpls.2022.867803/full#supplementary-material
Ahmad, T., and Suzuki, Y. J. (2019). Juglone in oxidative stress and cell signaling. Antioxidants 8, 91. doi: 10.3390/antiox8040091
Aissani, N., Urgeghe, P. P., Oplos, C., Saba, M., Tocco, G., Petretto, G. L., et al. (2015). Nematicidal activity of the volatilome of Eruca sativa on Meloidogyne incognita. J. Agric. Food Chem. 63, 6120–6125. doi: 10.1021/acs.jafc.5b02425
Al-Rehiayani, S. M. (2008). Acetylcholinesterase in selected plant-parasitic nematodes: inhibition, kinetic and comparative studies. Pestic. Biochem. Phys. 90, 19–25. doi: 10.1016/j.pestbp.2007.07.004
Aminin, D., and Polonik, S. (2020). 1,4-Naphthoquinones: some biological properties and application. Chem. Pharm. Bull. 68, 46–57. doi: 10.1248/cpb.c19-00911
Annisa, R., Hendradi, E., and Yuwono, M. (2020). Analysis of 1,4 naphthoquinone in the Indonesian medical plant from extract Eleutherine palmifolia (L.) Merr by UHPLC. IOP Conf. Ser. Earth Environ. Sci. 456, 012020. doi: 10.1088/1755-1315/456/1/012020
Aoudia, H., Ntalli, N., Aissani, N., Yahiaoui-Zaidi, R., and Caboni, P. (2012). Nematotoxic phenolic compounds from Melia azedarach against Meloidogyne incognita. J. Agric. Food Chem. 60, 11675–11680. doi: 10.1021/jf3038874
Aydinli, G., Kurtar, E. S., and Mennan, S. (2019). Screening of Cucurbita maxima and Cucurbita moschata genotypes for resistance against Meloidogyne arenaria, M. incognita, M. javanica, and M. luci. J. Nematol. 51, e2019–e2057. doi: 10.21307/jofnem-2019-057
Babula, P., Adam, V., Havel, L., and Kizek, R. (2009). Noteworthy secondary metabolites naphthoquinones – their occurrence, pharmacological properties and analysis. Curr. Pharm. Anal. 5, 47–68. doi: 10.2174/157341209787314936
Borazjani, A., Graves, C. H. Jr., and Hedin, P. A. (1985). Occurrence of juglone in various tissues of pecan and related species. Phytopathology 75, 1419–1421. doi: 10.1094/Phyto-75-1419
Caboni, P., and Ntalli, N. G. (2014). “Botanical nematicides, recent findings” in The Biopesticides: State of the Art and Future Opportunities, eds. A. D. Gross, J. R. Coats, S. O. Duke, and J. N. Seiber (Washington, DC: American Chemical Society; ACS Symposium Series) 1172, 145–157.
Cha, D. J., Kim, J., and Kim, D. S. (2019). Nematicidal activities of three naphthoquinones against the pine wood nematode, Bursaphelenchus xylophilus. Molecules 24, 3634. doi: 10.3390/molecules24203634
Chang, S., and Opperman, C. H. (1991). Characterization of acetylcholinesterase molecular forms of the root-knot nematode, Meloidogyne. Mol. Biochem. Parasitol. 49, 205–214. doi: 10.1016/0166-6851(91)90064-D
Chelinho, S., Maleita, C. M. N., Francisco, R., Braga, M. E. M., Cunha, M. J. M., da Abrantes, I., et al. (2017). Toxicity of the bionematicide 1,4-naphthoquinone on non-target soil organisms. Chemosphere 181, 579–588. doi: 10.1016/j.chemosphere.2017.04.092
Chen, J., Li, Q. X., and Song, B. (2020). Chemical nematicides: recent research progress and outlook. J. Agric. Food Chem. 68, 12175–12188. doi: 10.1021/acs.jafc.0c02871
Chobot, V., and Hadacek, F. (2009). Milieu-dependent pro- and antioxidant activity of juglone may explain linear and nonlinear effects on seedling development. J. Chem. Ecol. 35, 383–390. doi: 10.1007/s10886-009-9609-5
Combes, D., Fedon, Y., Toutant, J. P., and Arpagaus, M. (2001). Acetylcholinesterase genes in the nematode Caenorhabditis elegans. Int. Rev. Cytol. 209, 207–239. doi: 10.1016/S0074-7696(01)09013-1
Cosmulescu, S., Trandafir, I., Achim, G., Botu, M., Baciu, A., and Gruia, M. (2010). Phenolics of green husk in mature walnut fruits. Not. Bot. Horti. Agrobot. Cluj-Napoca 38, 53–56.
Cosmulescu, S. N., Trandafir, I., Achim, G., and Baciu, A. (2011). Juglone content in leaf and green husk of five walnut (Juglans regia L.) cultivars. Not Bot Horti Agrobot Cluj-Napoca 39, 237–240. doi: 10.15835/nbha3915728
Cui, R., Zhang, L., Chen, Y., Huang, W., Fan, C., Wu, Q., et al. (2017). Expression and evolutionary analyses of three acetylcholinesterase genes (Mi-ace-1, Mi-ace-2, Mi-ace-3) in the root-knot nematode Meloidogyne incognita. Exp. Parasitol. 176, 75–81. doi: 10.1016/j.exppara.2017.01.008
Dama, L. B. (2002). Effect of naturally occurring napthoquinones on root-knot nematode Meloidogyne javanica. Indian Phytopathol. 55, 67–69.
Deans, B. J., Tedone, L., Bissember, A. C., and Smith, J. A. (2018). Phytochemical profile of the rare, ancient clone Lomatia tasmanica and comparison to other endemic Tasmanian species L. tinctoria and L. polymorpha. Phytochemistry 153, 74–78. doi: 10.1016/j.phytochem.2018.05.019
Desaeger, J., Dickson, D. W., and Locascio, S. J. (2017). Methyl bromide alternatives for control of root-knot nematode (Meloidogyne spp.) in tomato production in Florida. J. Nematol. 49, 140. doi: 10.21307/jofnem-2017-058
Duarte, A. (2014). Meloidogyne hispanica Parasitism Genes: Molecular and Functional Characterization (dissertation/PhD thesis). Coimbra: University of Coimbra.
Dubreuil, G., Magliano, M., Deleury, E., Abad, P., and Rosso, M. N. (2007). Transcriptome analysis of root-knot nematode functions induced in the early stages of parasitism*. New Phytol. 176, 426–436. doi: 10.1111/j.1469-8137.2007.02181.x
Ebone, L. A., Kovaleski, M., and Deuner, C. C. (2019). Nematicides: history, mode, and mechanism action. Plant Sci. Today 6, 91–97. doi: 10.14719/pst.2019.6.2.468
Ellman, G. L., Courtney, K. D., Andres, V., and Featherstone, R. M. (1961). A new and rapid colorimetric determination of acetylcholinesterase activity. Biochem. Pharmacol. 7, 88–95. doi: 10.1016/0006-2952(61)90145-9
EPPO (2017). EPPO Alert List: Addition of Meloidogyne luci Together With M. ethiopica. EPPO Reporting Service, 2017/2018. Available online at: https://gd.eppo.int/reporting/article-6186 (accessed October 4, 2021).
EPPO (2021). Meloidogyne luci. Distribution. Available online at: https://gd.eppo.int/taxon/MELGLC/distribution (accessed October 4, 2021).
Esteves, I., Maleita, C., Fonseca, L., Braga, M. E. M., Abrantes, I., and de Sousa, H. C. (2017). In vitro nematicidal activity of naphthoquinones against the root-lesion nematode Pratylenchus thornei. Phytopathol. Mediterr. 56, 127–132. doi: 10.14601/Phytopathol_Mediterr-19314
European Commission (2009a). Regulation (EC) No 1107/2009 of the European Parliament and of the council of 21 october 2009 concerning the placing of plant protection products on the market and repealing council directives 79/117/EEC and 91/414/EEC. Off. J. Eur. Union 309, 1–50.
European Commission (2009b). Council Directive 2009 / 128 / EC of the European Parliament and of the Council of 21 October 2009, establishing a framework for Community action to achieve the sustainable use of pesticides. Off. J. Eur. Union 309, 71–86.
Fischer, T. C., Gosch, C., Mirbeth, B., Gselmann, M., Thallmair, V., and Stich, K. (2012). Potent and specific bactericidal effect of juglone (5-Hydroxy-1,4-naphthoquinone) on the fire blight pathogen Erwinia amylovora. J. Agric. Food Chem. 60, 12074–12081. doi: 10.1021/jf303584r
Gaspar, M. C., Agostinho, B., Fonseca, L., Abrantes, I., de Sousa, H. C., and Braga, M. E. M. (2020a). Impact of the pinewood nematode on naturally-emitted volatiles and scCO2 extracts from Pinus pinaster branches: a comparison with P. pinea. J. Supercrit. Fluids 159, 104784. doi: 10.1016/j.supflu.2020.104784
Gaspar, M. C., de Sousa, H. C., Seabra, I. J., and Braga, M. E. M. (2020b). Environmentally-safe scCO2 P. pinaster branches extracts: Composition and properties. J. CO2 Util. 37, 74–84. doi: 10.1016/j.jcou.2019.11.027
Ghaderi, R., and Karssen, G. (2020). An updated checklist of Meloidogyne Göldi, 1887 species, with a diagnostic compendium for second-stage juveniles and males. J. Crop Prot. 9, 183–193.
Haydock, P. P. J., Woods, S. R., Grove, I. G., and Hare, M. C. (2006). “Chemical control of nematodes” in Plant Nematology, eds. R. N. Perry, and M. Moens (Wallingford: CABI Publishing), 392–410.
Hussey, R. S., and Barker, K. R. (1973). A comparison of methods of collecting inocula of Meloidogyne spp., including a new technique. Plant Dis. Rep. 57, 1025–1028.
Hussey, R. S., and Janssen, G. J. W. (2002). “Root-knot nematodes: Meloidogyne species” in Plant Resistance to Parasitic Nematodes, eds J. L. Starr, R. Cook, and J. Bridge (New York, NY: CABI Publishing), 43–70.
Inbaraj, J. J., and Chignell, C. F. (2004). Cytotoxic action of juglone and plumbagin: a mechanistic study using HaCaT keratinocytes. Chem. Res. Toxicol. 17, 55–62. doi: 10.1021/tx034132s
Islam, A. K. M. M., and Widhalm, J. R. (2020). Agricultural uses of juglone: opportunities and challenges. Agronomy 10, 1500. doi: 10.3390/agronomy10101500
Jakopic, J., Colaric, M., Veberic, R., Hudina, M., Solar, A., and Štampar, F. (2007). How much do cultivar and preparation time influence on phenolics content in walnut liqueur? Food Chem. 104, 100–105. doi: 10.1016/j.foodchem.2006.11.008
Jha, B. K., Jung, H. J., Seo, I., Suh, S. I., Suh, M. H., and Baek, W. K. (2015). Juglone induces cell death of Acanthamoeba through increased production of reactive oxygen species. Exp. Parasitol. 159, 100–106. doi: 10.1016/j.exppara.2015.09.005
Johnson, J. A., Walse, S. S., and Gerik, J. S. (2012). Status of alternatives for methyl bromide in the United States. Outlooks on Pest. Manag. 23, 53–58. doi: 10.1564/23apr02
Jones, J. T., Haegeman, A., Danchin, E. G. J., Gaur, H. S., Helder, J., Jones, M. G. K., et al. (2013). Top 10 plant-parasitic nematodes in molecular plant pathology. Mol. Plant Pathol. 14, 946–961. doi: 10.1111/mpp.12057
Kocaçaliskan, I., Akgul, T., and Erisen, S. (2019). Effect of juglone on seed germination and seedling growth of four common vegetables. Int. J. Life Sci. Biotechnol. 2, 43–49. doi: 10.38001/ijlsb.546134
Kocaçaliskan, I., and Terzi, I. (2001). Allelopathic effects of walnut leaf extracts and juglone on seed germination and seedling growth. J. Hortic. Sci. Biotechnol. 76, 436–440. doi: 10.1080/14620316.2001.11511390
Kopits, E., McGartland, A., Morgan, C., Pasurka, C., Shadbegian, R., Simon, N. B., et al. (2014). Retrospective cost analyses of EPA regulations: a case study approach. J. Benefit Cost Anal. 5, 173–193. doi: 10.1515/jbca-2014-0024
Laxmikant, D. (2019). In vitro nematicidal activity of juglone against Meloidogyne incognita race 2 infesting pomegranate. J. Life Sci. Biomed. 9, 164–169. doi: 10.36380/scil.2019.jlsb26
Lee, C.-H., and Lee, H.-S. (2006). Color alteration and acaricidal activity of juglone isolated from Caesalpinia sappan heartwoods against Dermatophagoides spp. J. Microbiol. Biotechnol. 16, 1591–1596.
Lu, H., Xu, S., Zhang, W., Xu, C., Li, B., Zhang, D., et al. (2017). Nematicidal activity of trans-2-hexenal against southern root-knot nematode (Meloidogyne incognita) on tomato plants. J. Agric. Food Chem. 65, 544–550. doi: 10.1021/acs.jafc.6b04091
Mahajan, R., Kaur, D. J., and Bajaj, K. L. (1992). Nematicidal activity of phenolic compounds against Meloidogyne incognita. Nematol. Mediterr. 20, 217–219.
Mahdavi, M., Azadbakht, M., Vahdati, A., Shokrzadeh, M., and Farhadi, A. (2019). Cytotoxic effects of juglone and Pterocarya fraxinifolia on prostate cancer cells. J. Pharm. Bioallied Sci. 11, 195–204. doi: 10.4103/jpbs.JPBS_203_18
Maleita, C., Correia, A., Abrantes, I., and Esteves, I. (2022). Susceptibility of crop plants to the root-knot nematode Meloidogyne luci, a threat to agricultural productivity. Phytopathol. Mediterr. 61, 169–179.
Maleita, C., Esteves, I., Cardoso, J. M. S., Cunha, M. J., Carneiro, R. M. D. G., and Abrantes, I. (2018). Meloidogyne luci, a new root-knot nematode parasitizing potato in Portugal. Plant Pathol. 67, 366–376. doi: 10.1111/ppa.12755
Maleita, C., Esteves, I., Chim, R., Fonseca, L., Braga, M. E. M., Abrantes, I., and de Sousa, H. C. (2017). Naphthoquinones from walnut husk residues show strong nematicidal activities against the root-knot nematode Meloidogyne hispanica. ACS Sustain. Chem. Eng. 5, 3390–3398. doi: 10.1021/acssuschemeng.7b00039
McKenry, M. V., and Anwar, S. A. (2003). Nematicidal activity of walnut extracts against root-knot nematodes. J. Nematol. 35, 353.
Medic, A., Jakopic, J., Solar, A., Hudina, M., and Veberic, R. (2021a). Walnut (J. regia) agro-residues as a rich source of phenolic compounds. Biology 10, 535. doi: 10.3390/biology10060535
Medic, A., Zamljen, T., Hudina, M., and Veberic, R. (2021b). Identification and quantification of naphthoquinones and other phenolic compounds in leaves, petioles, bark, roots, and buds of Juglans regia L., using HPLC-MS/MS. Horticulturae 7, 326. doi: 10.3390/horticulturae7090326
Ntalli, N. G., and Caboni, P. (2012). Botanical nematicides: a review. J. Agric. Food Chem. 60, 9929–9940. doi: 10.1021/jf303107j
Nyczepir, A. P., and Thomas, S. H. (2009). “Current and future management strategies in intensive crop production systems” in Root-Knot Nematodes, eds R. N. Perry, M. Moens, and J. L. Starr (Oxfordshire: CABI Publishing), 412–443.
Oka, Y., and Saroya, Y. (2019). Effect of fluensulfone and fluopyram on the mobility and infection of second-stage juveniles of Meloidogyne incognita and M. javanica. Pest Manag. Sci. 75, 2095–2106. doi: 10.1002/ps.5399
Pankewitz, F., and Hilker, M. (2008). Polyketides in insects: ecological role of these widespread chemicals and evolutionary aspects of their biogenesis. Biol. Rev. 83, 209–226. doi: 10.1111/j.1469-185X.2008.00040.x
Piotte, C., Arthaud, L., Abad, P., and Rosso, M.-N. (1999). Molecular cloning of an acetylcholinesterase gene from the plant parasitic nematodes, Meloidogyne incognita and Meloidogyne javanica. Mol. Biochem. Parasitol. 99, 247–256. doi: 10.1016/S0166-6851(99)00033-X
Rangsinth, P., Prasansuklab, A., Duangjan, C., Gu, X., Meemon, K., Wink, M., et al. (2019). Leaf extract of Caesalpinia mimosoides enhances oxidative stress resistance and prolongs lifespan in Caenorhabditis elegans. BMC Comp. Med. Ther. 19, 164. doi: 10.1186/s12906-019-2578-5
Raspotnig, G., Fauler, G., Leis, M., and Leis, H. J. (2005). Chemical profiles of scent gland secretions in the cyphophthalmid opilionid harvestmen, Siro duricorius and S. exilis. J. Chem. Ecol. 31, 1353–1368. doi: 10.1007/s10886-005-5291-4
Rusinque, L., Nóbrega, F., Cordeiro, L., Serra, C., and Inácio, M. L. (2021). First detection of Meloidogyne luci (Nematoda: Meloidogynidae) parasitizing potato in the Azores, Portugal. Plants 10, 99. doi: 10.3390/plants10010099
Santos, D., Correia, A., Abrantes, I., and Maleita, C. (2019). New hosts and records in Portugal for the root-knot nematode Meloidogyne luci. J. Nematol. 51, e2019–e2003. doi: 10.21307/jofnem-2019-003
Seabra, I. J., Braga, M. E. M., Oliveira, R. A., and de Sousa, H. C. (2019). Two-step high pressure solvent extraction of walnut (Juglans regia L.) husks: scCO2 + CO2/ethanol/H2O. J. CO2 Util. 34, 375–385. doi: 10.1016/j.jcou.2019.07.028
Seid, A., Fininsa, C., Mekete, T., Decraemer, W., and Wesemael, W. M. (2015). Tomato (Solanum lycopersicum) and root-knot nematodes (Meloidogyne spp.) – a century-old battle. Nematology 17, 995–1009. doi: 10.1163/15685411-00002935
Sen, F., and Aydinli, G. (2021). Host status of cultivated crops to Meloidogyne luci. Eur. J. Plant Pathol. 161, 607–618. doi: 10.1007/s10658-021-02346-0
Sikder, M. M., and Vestergård, M. (2020). Impacts of root metabolites on soil nematodes. Front. Plant Sci. 10, 1792. doi: 10.3389/fpls.2019.01792
Solar, A., Colaric, M., Usenik, V., and Stampar, F. (2006). Seasonal variations of selected flavonoids, phenolic acids and quinones in annual shoots of common walnut (Juglans regia L.). Plant Sci. 170, 453–461. doi: 10.1016/j.plantsci.2005.09.012
Sun, L., Liao, K., Hang, C., and Wang, D. (2017). Honokiol induces reactive oxygen species-mediated apoptosis in Candida albicans through mitochondrial dysfunction. PLoS ONE 13 12, e0172228. doi: 10.1371/journal.pone.0172228
Sytykiewicz, H. (2011). Expression patterns of glutathione transferase gene (GstI) in maize seedlings under juglone-induced oxidative stress. Int. J. Mol. Sci. 12, 7982–7995. doi: 10.3390/ijms12117982
Taylor, A. L., and Sasser, J. N. (1978). Biology, Identification and Control of Root knot Nematodes (Meloidogyne spp.). Raleigh, NC: Department of Plant Pathology, North Carolina State University and the United States Agency for International Development, North Carolina State University Graphics.
Wang, J., Zeng, G., Huang, X., Wang, Z., and Tan, N. (2017). 1,4-Naphthoquinone triggers nematode lethality by inducing oxidative stress and activating insulin/IGF signaling pathway in Caenorhabditis elegans. Molecules 22, 798. doi: 10.3390/molecules22050798
Widhalm, J., and Rhodes, D. (2016). Biosynthesis and molecular actions of specialized 1,4-naphthoquinone natural products produced by horticultural plants. Hortic. Res. 3, 16046. doi: 10.1038/hortres.2016.46
Keywords: bionematicides, hatching, mortality, penetration, plant-parasitic nematodes, reproduction
Citation: Maleita C, Esteves I, Braga MEM, Figueiredo J, Gaspar MC, Abrantes I and Sousa HCd (2022) Juglone and 1,4-Naphthoquinone—Promising Nematicides for Sustainable Control of the Root Knot Nematode Meloidogyne luci. Front. Plant Sci. 13:867803. doi: 10.3389/fpls.2022.867803
Received: 01 February 2022; Accepted: 19 April 2022;
Published: 17 May 2022.
Edited by:
Holger Heuer, Julius Kühn-Institut, GermanyReviewed by:
Anil Kumar, Agricultural Research Organization (ARO), IsraelCopyright © 2022 Maleita, Esteves, Braga, Figueiredo, Gaspar, Abrantes and Sousa. This is an open-access article distributed under the terms of the Creative Commons Attribution License (CC BY). The use, distribution or reproduction in other forums is permitted, provided the original author(s) and the copyright owner(s) are credited and that the original publication in this journal is cited, in accordance with accepted academic practice. No use, distribution or reproduction is permitted which does not comply with these terms.
*Correspondence: Carla Maleita, Y2FybGEubWFsZWl0YUB1Yy5wdA==
Disclaimer: All claims expressed in this article are solely those of the authors and do not necessarily represent those of their affiliated organizations, or those of the publisher, the editors and the reviewers. Any product that may be evaluated in this article or claim that may be made by its manufacturer is not guaranteed or endorsed by the publisher.
Research integrity at Frontiers
Learn more about the work of our research integrity team to safeguard the quality of each article we publish.