- State Key Laboratory of Crop Genetics & Germplasm Enhancement, Key Laboratory of Biology and Genetic Improvement of Horticultural Crops (East China), Ministry of Agriculture and Rural Affairs of the P. R. China, Engineering Research Center of Germplasm Enhancement and Utilization of Horticultural Crops, Ministry of Education of the P. R. China, Nanjing Suman Plasma Engineering Research Institute, Nanjing Agricultural University, Nanjing, China
The TEOSINTE BRANCHED1/CYCLOIDEA/PROLIFERATING CELL FACTOR (TCP) gene family, a kind of plant specific transcription factor, is essential for stress response, cell growth, and cell proliferation. However, the characterization of TCP family is still not clear in Pak-choi [Brassica campestris (syn. Brassica rapa) ssp. chinensis var. communis]. In this study, genome-wide analysis of TCP gene family was performed and 26 TCP genes were identified in Pak-choi. Phylogenetic analysis demonstrated that the 26 BcTCPs were divided into two classes: Class I and Class II. Class II was further classified into two subclasses, CIN and CYC/TB1. The qPCR results suggested that most BcTCPs respond to abiotic stresses. The expressions of BcTCP3, BcTCP12, BcTCP21, and BcTCP22 were significantly changed under ABA and cold treatment. BcTCP3 and BcTCP12 were also up-regulated under osmotic treatment. Subcellular localization showed that BcTCP3 and BcTCP21 were located in the nucleus. Our results will facilitate revealing the functions and regulatory mechanisms of BcTCPs.
Introduction
The TCP gene family is a class of plant-specific transcription factors and plays important roles in plant growth and development. TCP was named from four genes members: TEOSINTE BRANCHED 1 (TB1) from maize (Zea mays) (Doebley et al., 1995), CYCLOIDEA (CYC) from snapdragon (Antirrhinum majus) (Luo et al., 1996), and the PROLIFERATING CELL FACTORS 1 (PCF1) and PCF2 from rice (Oryza sativa) (Kosugi and Ohashi, 1997). TB1 is a key factor to control apical dominance in maize (Doebley et al., 1997). CYC involves in the regulation of floral asymmetry in snapdragons. PCF1 and PCF2 bind to the promoter of PROLIFERATING CELL NUCLEAR ANTIGEN (PCNA) gene in rice, which involves in DNA replication and repair, maintenance of chromatin structure, chromosome segregation, and cell-cycle progression (Luo et al., 1996; Kosugi and Ohashi, 1997). TCP proteins contain a 59-amino-acid domain with a bHLH motif that involved in DNA binding and protein-protein interaction (Martin-Trillo and Cubas, 2010). According to the conserved domain, TCP proteins can be divided into two classes: Class I (TCP-P Class) and Class II (TCP-C Class) (Kosugi and Ohashi, 2002; Navaud et al., 2007). Class II can be further divided into two subclades: CIN and CYC/TB1 (Martin-Trillo and Cubas, 2010). Class I includes PCF1 and PCF2 in rice, TCP8, TCP9, TCP14, TCP20, etc. in Arabidopsis. Class II contains TB1 in maize, TCP1, TCP2, TCP4, TCP10, BCR1, BCR2, etc. in Arabidopsis.
Abiotic stress has an important effect on plant growth and productivity. Cold stress slows down the metabolic processes of plants, inhibits auxin transport and root elongation (Shibasaki et al., 2009). Salt stress leads to the accumulation of toxic ions in cells, which influences plant absorption of nutrients and water (Boudsocq and Laurière, 2005). Plants are subjected to dehydration under low temperature, high salinity, and drought environmental conditions, of which cause osmotic stress, inducing Abscisic acid (ABA) biosynthesis. ABA functions in multiple stresses, regulating genes related to dehydration and cold stress and controlling osmotic stress tolerance (Yamaguchi-Shinozaki and Shinozaki, 2006; Fujita et al., 2011). Moreover, ABA can reduce water loss by governing stomatal closure (Kim et al., 2010). The growth and development of plants will be severely affected when plants suffer from abiotic stresses. Hence, the study of response mechanisms during abiotic stresses is vital for species. At present, it is reported that many genes of the TCP family are related to various stresses. TCP20 responds to nitrate availability by interacting with NIN-like protein 6 (NLP6) and NLP9, controlling plants root growth (Guan et al., 2017). OsTCP19 is up-regulated under drought, salt, and cold stress, indicating OsTCP19 may involve in the stress tolerance (Mukhopadhyay and Tyagi, 2015). In Cicer arietinum, five TCP genes (CaTCP3/13/15/20/21), which contained the MYB cis-elements, were strongly induced under drought conditions, and similar results were found in other legumes (Ling et al., 2020). Oryza sativa miR319 (Osa-miR319), an upstream gene of OsPCF5, OsPCF6, OsPCF8, and OsTCP21, is decreased in the cold condition in rice. Meanwhile, overexpressing Osa-miR319 results in down-regulation of its downstream target genes and enhances cold resistance, impling that TCP21 could involve in cold stress (Yang et al., 2013; Wang et al., 2014). In creeping bentgrass (Agrostis stolonifera), overexpressing Osa-miR319 also reduces the expression of its target genes (AsPCF5, AsPCF6, AsPCF8, and AsTCP14), enhancing salt and drought tolerance (Zhou et al., 2013). GmTCP8 interacts with GmPYL10 and involves in the ABA signal pathway in soybean (Feng et al., 2018). ZmTCP42 is associated with ABA and drought stress, which plays an active role in drought tolerance (Ding et al., 2019). AtTCP14 represses ABA1 (ABA DEFICIENT 1) and other ABA-related stress genes in Arabidopsis seeds (Rueda-Romero et al., 2012).
Until now, the study of the TCP family has been more and more comprehensive, which have been identified in many species including Arabidopsis thaliana (Li, 2015), rice (Oryza sativa) (Yao et al., 2007), turnips (Brassica rapa ssp. rapa) (Du et al., 2017), poplar (Populus trichocarpa) (Ma et al., 2016), tomato (Solanum lycopersicum) (Parapunova et al., 2014), Switchgrass (Panicum virgatum L.) (Huo et al., 2019), Brassica rapa and Brassica oleracea (Liu et al., 2019). Pak-choi [Brassica campestris (syn. Brassica rapa) ssp. chinensis var. communis] has gradually become worldwide vegetable crops. However, Pak-choi TCP genes and their regulatory mechanisms are still not clear.
In this study, genome-wide analysis of TCP family genes in Pak-choi was performed. 26 BcTCP genes were identified in Pak-choi. Phylogenetic relationship, subcellular localization, and conserved motifs were analyzed. QPCR was employed to illustrate the expression patterns of BcTCPs under salt, osmotic, cold, and ABA treatment. The results showed that BcTCPs may involve in multiple abiotic stresses. Our findings provide a theoretical basis for further research on the potential functions and regulatory mechanisms of BcTCP genes.
Materials and Methods
Cloning and Identification of BcTCP Gene Family Members
Total RNA was extracted from Pak-choi using the RNAeasy Mini Kit (Tiangen, Beijing, China). The CDSs of the BcTCPs were amplified with gene specific primers based on the sequences of AtTCPs by homology cloning (Huang et al., 2019 and Supplementary Table 1). The PCR products were cloned into the pMD18-T vector before sequencing. Then, the obtained plasmids were sequenced by TSINGKE (Nanjing, China). All putative BcTCP proteins sequences were analyzed using the Pfam database1. Additionally, protein molecular weight, isoelectric point, and amino acid length of BcTCPs were computed by the ExPASy ProtParam tool2.
Phylogenetic Tree and Cis-Acting Elements Analysis
24 AtTCP genes were retrieved from TAIR3. Multiple sequence alignments of putative BcTCPs and AtTCPs were performed using Clustal X 2.1. Phylogenetic tree was completed by the Maximum Likelihood (ML) method using MEGA 7.0 software. The bootstrap values were performed with 1,000 replications. PlantCARE4 was used to analyze cis-acting elements in promoters of each BcTCP gene. After classifying and counting the elements on each promoter (Supplementary Table 2), the heat map was made by TBtools.
Conserved Domain Analysis
The conserved motifs were analyzed using MEME program5 with the default settings except the maximum width was set to 200, and the minimum and maximum numbers of motifs were defifined as 2 and 10, respectively.
Plant Materials and Growth Conditions
The Pak-choi cultivar ‘49caixin’ was selected in our experiment, which has a short life cycle and may flower within two months after sowing under long-day conditions (Tian et al., 2004). Plants were cultivated in pots with medium (Soil matrix and vermiculite, 1:1). All seedlings were grown in a controlled artificial climatic chamber under the same conditions (16 h light at 22°C/8 h dark at 18°C, 60-70% relative humidity). One-month-old seedlings were treated with 100 μM ABA, 250 mM NaCl and 20% PEG6000, respectively. For cold treatment, one-month-old seedlings were transferred to 4°C. The leaves were collected under each stress treatment in a continuous time course (0, 10, 20, 30, 40, 50 min, 1, 2, 3, 4, 8 h) and stored at −70°C immediately for RNA extraction.
Expression Analysis of BcTCP Genes by qPCR
Following the manufacturer’s instructions, total RNA was isolated from leaves using the RNA easy mini kit (Tiangen, Beijing, China). Then first-strand cDNA was synthesized with a RevertAid First Strand cDNA Synthesis Kit (Thermo, Shanghai, China). Primers for qPCR were designed using Primer 5.0 (Supplementary Table 1). QPCR was performed using the SYBR® Premix Ex Taq kit (Takara, Dalian, China). BcActin was used as an internal reference gene. Results were calculated by the 2–ΔΔCT method (Livak and Schmittgen, 2001).
Subcellular Localization Analysis
WOLF PSORT6 was used to predict the subcellular localization of the putative BcTCP proteins. To further confirm their subcellular localization, the protein-coding regions of BcTCP3 and 21 without the termination codon were amplified and then cloned into pCambia 1,302 vector in fusion with GFP at C-terminal end (35S: GFP), generating the fusion constructs. The 35S: BcTCP3-GFP and 35S: BcTCP21-GFP were generated. Each plasmid was injected into the tobacco leaves by Agrobacterium tumefaciens-mediated transient transformation (strain GV3101). The tobacco was incubated for 12 h under darkness. 2-3 days after injection, the fluorescence of GFP was observed and photographed by confocal microscopy (Leica, TCS SP2, Wetzlar, Germany). DAPI (nucleus specific dye) was used as nuclei dye in the experiment.
Results
Identification of TCPs in Pak-Choi
A total of 26 TCP genes in Pak-choi were obtained by homology cloning based on the Arabidopsis thaliana TCP genes. These 26 genes were named BcTCP1∼BcTCP26. The CDS length of the 26 BcTCPs was ranged from 651 to 1,395 bp. The physical and chemical properties were further analyzed using the ExPASy ProtParam tool. The molecular weight of 26 putative BcTCP proteins varied from 23.12 to 56.74 kDa. The isoelectric point was ranged from 5.49 to 9.99, and the amino acid length was ranged from 216 to 520 aa (Supplementary Table 3).
Phylogenetic Analysis
An unrooted phylogenetic tree was constructed with the Maximum Likelihood (ML) method (Figure 1). 26 BcTCPs were divided into two classes, Class I and Class II. The Class II TCPs were further categorized into two subgroups, CIN and CYC/TB1. PCF Class (Class I) contained 13 genes, CYC/TB1 Class contained 7 genes, CIN only contained 6 genes. Sequence analysis showed that AtTCP3, 6, 11, 13, 16, 17, 20, 23 had no ortholog in Pak-choi. AtTCP1, 7, 15, 18, 21, and 24 had more than one ortholog in the Pak-choi.
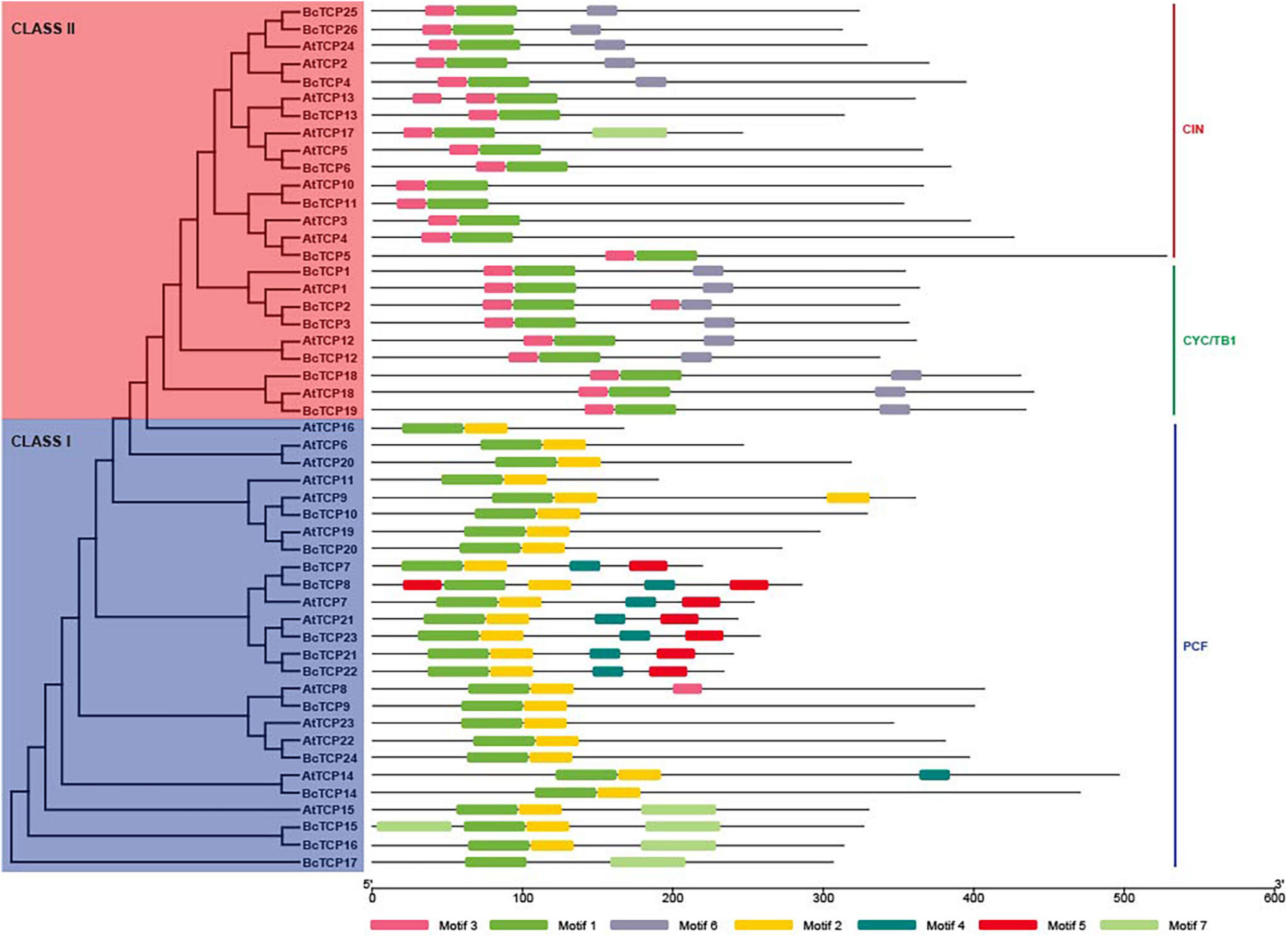
Figure 1. The phylogenetic tree and conserved motifs of BcTCP and AtTCP proteins. Each motif represented with a specific color was shown.
Conserved Domain Analysis
The conserved motifs of BcTCP proteins were analyzed using MEME. A total of 7 motifs were found in BcTCPs. The motifs were more similar in the same class. Furthermore, similar conserved motifs arrangements can be observed in the same clade. Motif 1 was conserved in all BcTCPs. Motif 3 and motif 6 only existed in Class II except for BcTCP5, 6, 11, and 13 (Figure 1), suggesting that these BcTCPs may have similar functions. However, proteins among different groups shared diverse motifs. Motif 2 is presented in Class I (PCF) except for BcTCP20. Motif 7 was only existed in BcTCP15, 16, and 17. Motif 4 and motif 5 both existed in BcTCP7, BcTCP8, BcTCP21, BcTCP22, and BcTCP23. These results indicated that TCP genes may perform different functions in Pak-choi.
Analysis of Cis-Acting Elements in BcTCPs Promoters
Cis-acting elements existed in gene promoters can affect gene expressions and functions. In this study, we found that BcTCPs promoters contained not only basic core elements, like TATA-box and CAAT-box, but also a variety of cis-acting elements, such as light response elements, hormone response elements, environment response elements, development response elements, and other functional elements (Figure 2). All Class II BcTCPs promoters contained G-box elements and ABRE elements except for BcTCP20 and BcTCP21. In Class I BcTCPs, the promoters of BcTCP2, 3, 11, 12, 13, 25, and 26 all contained the low temperature response element (LTR). AT∼TATA box1 was identified in most all BcTCP promoters except BcTCP6 and BcTCP23. The promoters of BcTCP2, 3, 4, 10, 11, 12, 14, 15, 20, 21, 22, 26 contained MYB, MYC, and MYB-like sequence elements at the same time. As a result, we hypothesized that these BcTCP genes may be involved in stress (Abe et al., 2003; Xu et al., 2021).
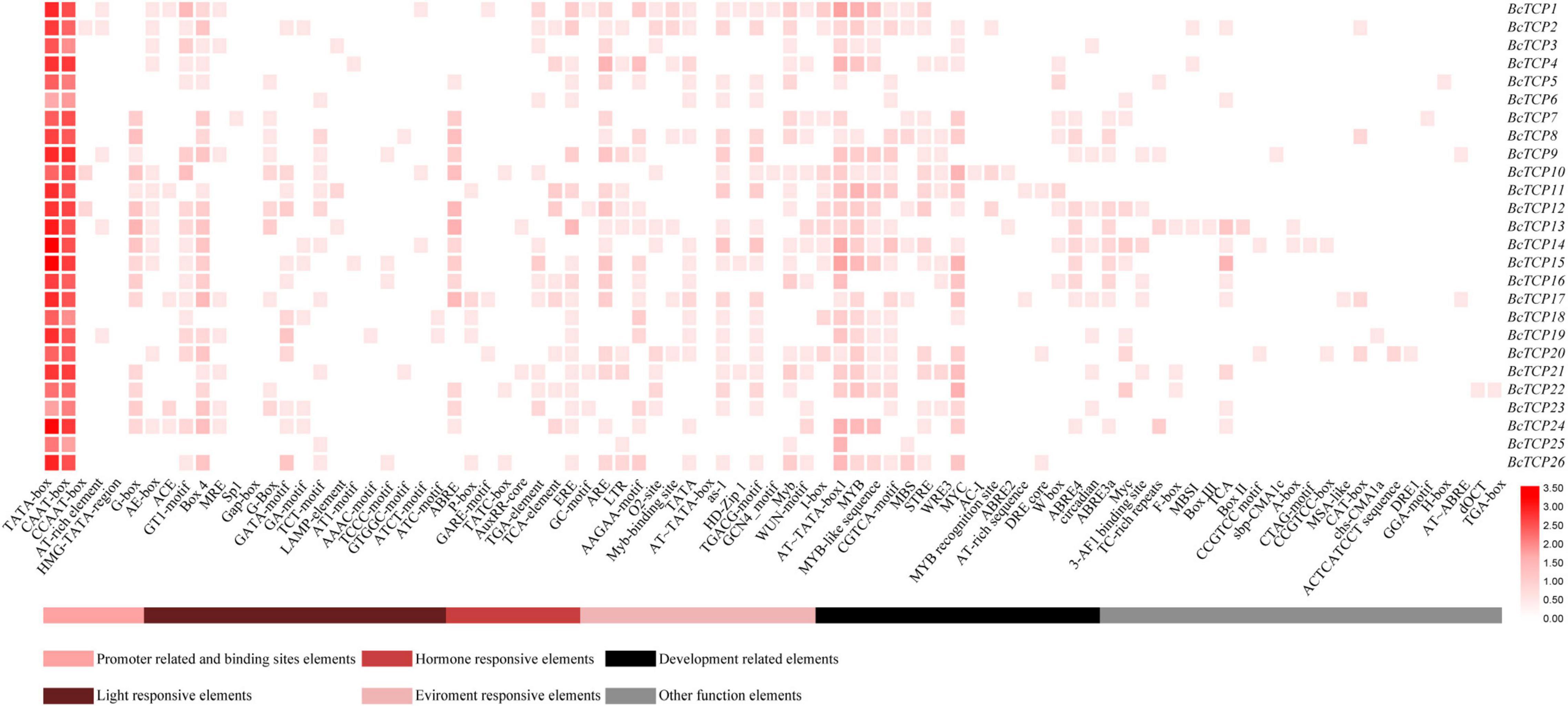
Figure 2. Cis-acting elements on promoters of BcTCP genes. The color bar showed the number of cis-acting elements.
Expression Analysis of BcTCP Genes Under Multiple Abiotic Stresses
To investigate the functions of BcTCPs, qPCR was performed to detect the expressions of 26 BcTCPs under four treatments (salt, osmotic, cold and ABA) (Figure 3). The results showed that the expressions of most BcTCPs, except for BcTCP12, were not significantly changed under salt treatment. BcTCP2, 3, 5, 6, 7, 8, 10, 12, 15, 16, 17, 20, 21, 22, 23, and 24 all up-regulated after 1 h of the ABA treatment. The expression levels of BcTCP2, 5, 6, 7, 8, 10, 17, 20, 21, 22, 23, and 24 reached the maximum at 2 h, BcTCP15 and 16 had the highest expression at 3h, the largest amount of BcTCP12 expressed in 4 h, and the largest amount of BcTCP3 expression quantity in 4 h. Under cold treatment, BcTCP3, 6, 7, 10, 12, 21, 22, and 24 showed a significant increase, indicating that these genes, especially BcTCP21, may involve in cold stress. Meanwhile, BcTCP2, 3, and 12 were notably up-regulated under osmotic treatment. BcTCP2 reached its peak at 4 h, and BcTCP3 and 12 reached their peak at 2 h. These suggested that most BcTCP genes were involved in abiotic stresses.
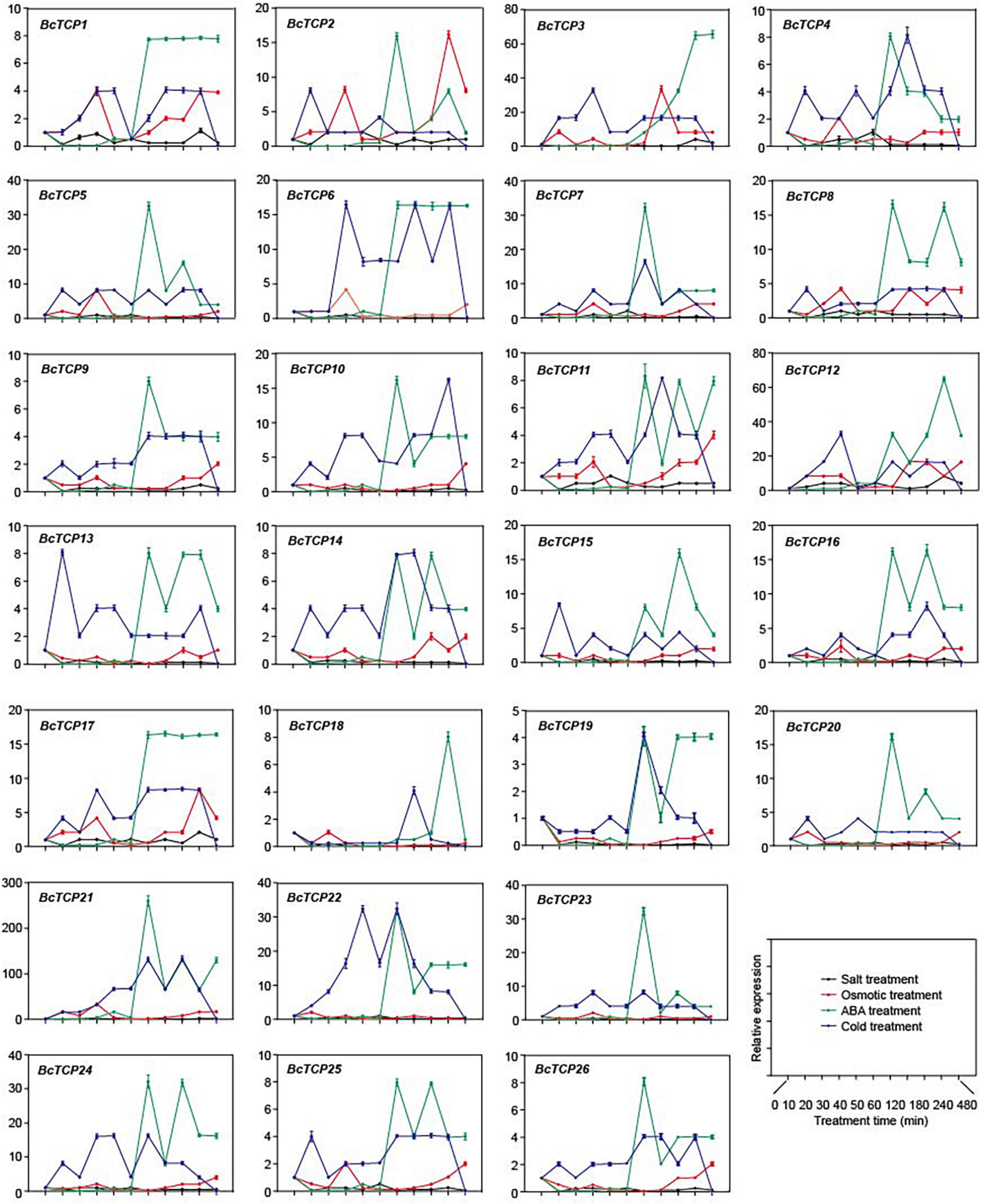
Figure 3. Expression of BcTCP genes under different stress treatments. Data shown were means ± SE of three independent experiments.
Subcellular Localization
WoLF PSORT predicted that BcTCP proteins were localized in the nucleus (Supplementary Table 3). To test this, 35S: GFP, 35S: BcTCP3-GFP, and 35S: BcTCP21-GFP constructs were transiently overexpressed in tobacco leaves (Figure 4A). As seen in Figure 4B, 35S: GFP was localized in the nucleus and cytoplasm. The GFP signals emitted by BcTCP3 -GFP and BcTCP21 -GFP fusion protein were detected in the nucleus and overlapped with the DAPI staining. This results indicated that BcTCP3 and BcTCP21 were nuclear-localized proteins and may function as transcription factors.
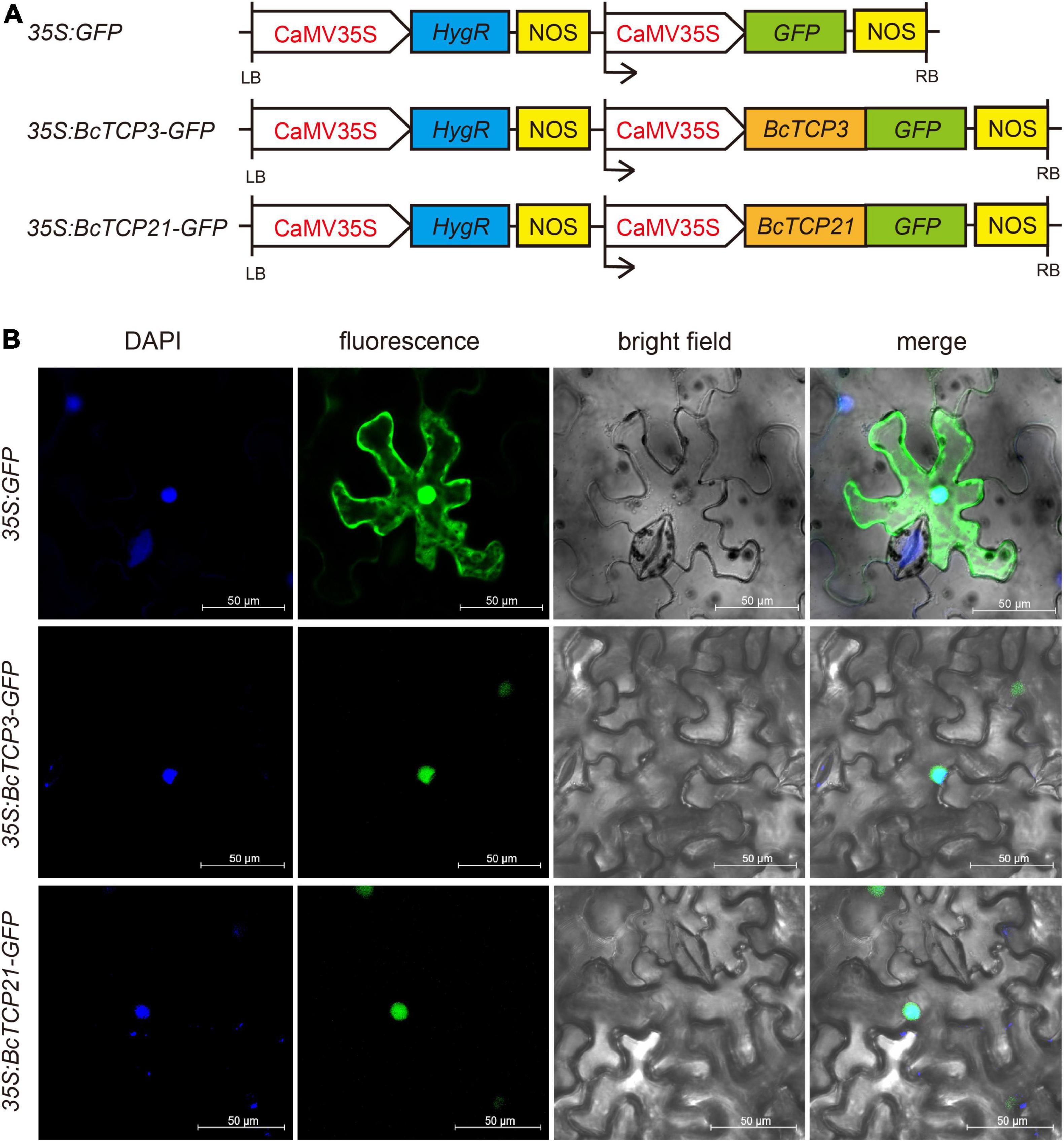
Figure 4. Subcellular localization of BcTCP3 protein and BcTCP21 protein: (A) 35S: GFP, 35S: BcTCP3, and 35S: BcTCP21 construct. (B) Transient expression of 35S:GFP, 35S: 35S: BcTCP3-GFP, and 35S: BcTCP21-GFP fusion protein in tobacco leaves (Scale bars = 50 μm).
Discussion
TCP gene family involves in multiple biological processes, including the circadian clock, abiotic stresses, leaf sizes and shapes, flower development and flowering (Takeda et al., 2006; Pruneda-Paz et al., 2009; Mimida et al., 2011; Niwa et al., 2013; Liu et al., 2017; Feng et al., 2018). The roles of TCP genes under abiotic stresses have been reported in some model plants, such as rice, Zea mays, Arabidopsis thaliana, and soybean (Rueda-Romero et al., 2012; Mukhopadhyay and Tyagi, 2015; Feng et al., 2018; Ding et al., 2019). However, virtually less systematic and comprehensive information of the TCP gene family in Pak-choi was reported, which is a nutritious and economically important vegetable crop and widely cultivated in Asia. Unlike A. thaliana, Brassicas crops not only underwent this complex evolutionary history, but also the whole-genome triplication (WGT) event between 13 and 17 million years ago (MYA). These events were essential for evolution and resulted in differences among species (Wang et al., 2011). A. thaliana has been identified 24 TCP genes (Yao et al., 2007). In this study, 26 BcTCP genes were isolated and studied in Pak-choi. These BcTCPs were divided into Class I and Class II, Class II was then divided into CIN and CYC/TB1, which was consistent with the previously described in Arabidopsis, rice, tomato, and strawberry (Yao et al., 2007; Parapunova et al., 2014; Wei et al., 2016). The phylogenetic analysis found that 16 AtTCPs had orthologs in Pak-choi. Among them, AtTCP1, 7, 15, 18, 21, and 24 had more than one ortholog. This indicated that BcTCP family genes and AtTCP family genes may have some similar functions. According to conserved domain, we found that the similarity of BcTCPs in the same class is higher than that of different classes of BcTCPs. For example, CYB/TB1 members all contained motif 1, motif 3, and motif 6. Analysis of promoter regions showed that some BcTCPs promoters contained MBS cis-regulatory elements, MYB cis-regulatory elements, ABRE elements, and G-box, suggesting that the BcTCP genes might play significant roles in stress responses (Abe et al., 2003; Xu et al., 2021).
QPCR analysis demonstrated that some BcTCPs were involved in multiple abiotic stress. For instance, BcTCP3, 12, 21, 22, and 24 all responded to cold and ABA treatment. BcTCP3 had no response to salt stress but had relatively obvious responses under cold and ABA treatments. BcTCP12 responded to salt, osmotic, ABA, and cold treatments. Under salt treatment, the expression of BcTCP12 was significantly activated, indicating that BcTCP12 may enhance salt tolerance, which is same as previous studies in Oryza sativa (Xiao et al., 2009; Almeida et al., 2017). The expressions of BcTCP3, 5, 7, 12, 21, 22, 23, and 24 displayed more than 30 fold up-regulation under ABA treatment. During drought treatment, BcTCP2, 3, and 12 were up-regulated by more than 15 fold. BcTCP3, 12, 21, and 22 were also up-regulated by more than 30 fold under cold treatment. These results were consistent with previous research results in rice (Yang et al., 2013; Wang et al., 2014). Subcellular localization analysis demonstrated BcTCP3 and BcTCP21 were located in the nucleus, indicating they may function as transcription factors.
These results revealed that BcTCPs were associated with multiple abiotic stresses. Our study may be helpful for improving plant stress tolerance. This will provide a piece of vital evidence for detecting the molecular mechanisms of TCPs under abiotic stresses. The systematic characterization of BcTCPs in Pak-choi will provide a better foundation for further functional studies of this gene family in plant growth and development.
Data Availability Statement
The original contributions presented in the study are included in the article/Supplementary Material, further inquiries can be directed to the corresponding author/s.
Author Contributions
FH, CS, and YZ completed the experiments and wrote the manuscript. XH revised and approved the manuscript. All authors have read and agreed to the published version of the manuscript.
Funding
This work was supported by grants from the National Natural Science Foundation of China (32102410), the Fundamental Research Funds for the Central Universities (KYQN2022054), the Natural Science Foundation of Jiangsu province (BK20190513), the Key Projects of National Key Research and Development Plan (2017YFD0101803) and the China Agriculture Research System (CARS-23-A-06).
Conflict of Interest
The authors declare that the research was conducted in the absence of any commercial or financial relationships that could be construed as a potential conflict of interest.
Publisher’s Note
All claims expressed in this article are solely those of the authors and do not necessarily represent those of their affiliated organizations, or those of the publisher, the editors and the reviewers. Any product that may be evaluated in this article, or claim that may be made by its manufacturer, is not guaranteed or endorsed by the publisher.
Supplementary Material
The Supplementary Material for this article can be found online at: https://www.frontiersin.org/articles/10.3389/fpls.2022.854171/full#supplementary-material
Footnotes
- ^ http://pfam.xfam.org/
- ^ https://web.expasy.org/protparam/
- ^ https://www.arabidopsis.org/
- ^ http://bioinformatics.psb.ugent.be/webtools/plantcare/html/
- ^ http://meme-suite.org/
- ^ http://wolfpsort.org/
References
Abe, H., Urao, T., Ito, T., Seki, M., Shinozaki, K., and Yamaguchi-Shinozaki, K. (2003). Arabidopsis AtMYC2 (bHLH) and AtMYB2 (MYB) Function as Transcriptional Activators in Abscisic Acid Signaling. Plant Cell 155, 63–78. doi: 10.1105/tpc.006130
Almeida, D. M., Gregorio, G. B., Oliveira, M. M., and Saibo, N. J. M. (2017). Five noveln transcription factors as potential regulators of OsNHX1 gene expression in a salt tolerant rice genotype. Plant Mol. Biol. 93, 61–77. doi: 10.1007/s11103-016-0547-7
Boudsocq, M., and Laurière, C. (2005). Osmotic Signaling in Plants. Multiple Pathways Mediated by Emerging Kinase Families. Plant Physiol. 138, 1185–1194. doi: 10.1104/pp.105.061275
Ding, S., Cai, Z., Du, H., and Wang, H. (2019). Genome-Wide Analysis of TCP Family Genes in Zea mays L. Identified a Role for ZmTCP42 in Drought Tolerance. Int. J. Mol. Sci. 20:2762. doi: 10.3390/ijms20112762
Doebley, J., Stec, A., and Gustus, C. (1995). teosinte branched1 and the Origin of Maize: evidence for Epistasis and the Evolution of Dominance. Genetics 141, 333–346. doi: 10.1093/genetics/141.1.333
Doebley, J., Stec, A., and Hubbard, L. (1997). The evolution of apical dominance in maize. Nature 386, 485–488. doi: 10.1038/386485a0
Du, J., Hu, S., Yu, Q., Wang, C., Yang, Y., Sun, H., et al. (2017). Genome-Wide Identification and Characterization of BrrTCP Transcription Factors in Brassica rapa ssp. rapa. Front. Plant Sci. 8:1588. doi: 10.3389/fpls.2017.01588
Feng, Z. J., Xu, S. C., Liu, N., Zhang, G. W., Hu, Q. Z., and Gong, Y. M. (2018). Soybean TCP transcription factors: evolution, classification, protein interaction and stress and hormone responsiveness. Plant Physiol. Biochem. 127, 129–142. doi: 10.1016/j.plaphy.2018.03.020
Fujita, Y., Fujita, M., Shinozaki, K., and Yamaguchi-Shinozaki, K. (2011). ABA-mediated transcriptional regulation in response to osmotic stress in plants. J. Plant Res. 124, 509–525. doi: 10.1007/s10265-011-0412-3
Guan, P., Ripoll, J. J., Wang, R., Vuong, L., Bailey-Steinitz, L. J., Ye, D., et al. (2017). Interacting TCP and NLP transcription factors control plant responses to nitrate availability. Proc. Natl. Acad. Sci. U. S. A. 114, 2419–2424. doi: 10.1073/pnas.1615676114
Huang, F. Y., Liu, T. K., Tang, J., Duan, W. K., and Hou, X. L. (2019). BcMAF2 activates BcTEM1 and represses flowering in Pak-choi (Brassica rapa ssp. chinensis). Plant Mol. Biol. 100, 19–32. doi: 10.1007/s11103-019-00867-1
Huo, Y., Xiong, W., Su, K., Li, Y., Yang, Y., Fu, C., et al. (2019). Genome-Wide Analysis of the TCP Gene Family in Switchgrass (Panicum virgatum L.). Int. J. Genomics 2019, 1–13. doi: 10.1155/2019/8514928
Kim, T. H., Böhmer, M., Hu, H., Nishimura, N., and Schroeder, J. I. (2010). Guard Cell Signal Transduction Network: advances in Understanding Abscisic Acid, CO2, and Ca2+ Signaling. Annu. Rev. Plant Biol. 61, 561–591. doi: 10.1146/annurev-arplant-042809-112226
Kosugi, S., and Ohashi, Y. (1997). PCF1 and PCF2 Specifically Bind to cis Elements in the Rice Proliferating Cell Nuclear Antigen Gene. Plant Cell 9, 1607–1619. doi: 10.2307/3870447
Kosugi, S., and Ohashi, Y. (2002). DNA binding and dimerization specificity and potential targets for the TCP protein family. Plant J. 30, 337–348. doi: 10.1046/j.1365-313X.2002.01294.x
Li, S. (2015). The Arabidopsis thaliana TCP transcription factors: a broadening horizon beyond development. Plant Signal. Behav. 10:e1044192. doi: 10.1080/15592324.2015.1044192
Ling, L., Zhang, W., An, Y., Du, B., Wang, D., and Guo, C. (2020). Genome-wide analysis of the TCP transcription factor genes in five legume genomes and their response to salt and drought stresses. Funct. Integr. Genomics 20, 537–550. doi: 10.1007/s10142-020-00733-0
Liu, J., Cheng, X., Liu, P., Li, D., Chen, T., Gu, X., et al. (2017). MicroRNA319-regulated TCPs interact with FBHs and PFT1 to activate CO transcription and control flowering time in Arabidopsis. PLoS Genet. 13:e1006833. doi: 10.1371/journal.pgen.1006833
Liu, M. M., Wang, M. M., Yang, J., Wen, J., Guo, P. C., Wu, Y. W., et al. (2019). Evolutionary and Comparative Expression Analyses of TCP Transcription Factor Gene Family in Land Plants. Int. J. Mol. Sci. 20:3591. doi: 10.3390/ijms20143591
Livak, K. J., and Schmittgen, T. D. (2001). Analysis of Relative Gene Expression Data Using Realtime Quantitative PCR and the 2–ΔΔCT Method. Methods 25, 402–408. doi: 10.1006/meth.2001
Luo, D., Carpenter, R., Vincent, C., Copsey, L., and Coen, E. (1996). Origin of floral asymmetry in Antirrhinum. Nature 383, 794–799. doi: 10.1038/383794a0
Ma, X., Ma, J., Fan, D., Li, C., Jiang, Y., and Luo, K. (2016). Genome-wide Identification of TCP Family Transcription Factors from Populus euphratica and their Involvement in Leaf Shape Regulation. Sci. Rep. 6:32795. doi: 10.1038/srep32795
Martin-Trillo, M., and Cubas, P. (2010). TCP genes: a family snapshot ten years later. Trends Plant Sci. 15, 31–39. doi: 10.1016/j.tplants.2009.11.003
Mimida, N., Kidou, S., Iwanami, H., Moriya, S., Abe, K., Voogd, C., et al. (2011). Apple FLOWERING LOCUS T proteins interact with transcription factors implicated in cell growth and organ development. Tree Physiol. 31, 555–566. doi: 10.1093/treephys/tpr028
Mukhopadhyay, P., and Tyagi, A. K. (2015). OsTCP19 influences developmental and abiotic stress signaling by modulating ABI4-mediated pathways. Sci. Rep. 5:9998. doi: 10.1038/srep09998
Navaud, O., Dabos, P., Carnus, E., Tremousaygue, D., and Hervé, C. (2007). TCP Transcription Factors Predate the Emergence of Land Plants. J. Mol. Evol. 65, 23–33. doi: 10.1007/s00239-006-0174-z
Niwa, M., Daimon, Y., Kurotani, K., Higo, A., Pruneda-Paz, J. L., Breton, G., et al. (2013). BRANCHED1 Interacts with FLOWERING LOCUS T to Repress the Floral Transition of the Axillary Meristems in Arabidopsis. Plant Cell 25, 1228–1242. doi: 10.1105/tpc.112.109090
Parapunova, V., Busscher, M., Busscher-Lange, J., Lammers, M., Karlova, R., Bovy, A. G., et al. (2014). Identification, cloning and characterization of the tomato TCP transcription factor family. BMC Plant Biol. 14:157. doi: 10.1186/1471-2229-14-157
Pruneda-Paz, J. L., Breton, G., Para, A., and Kay, S. A. (2009). A functional genomics approach reveals CHE as a component of the Arabidopsis circadian clock. Science 323, 1481–1485. doi: 10.1126/science.1167206
Rueda-Romero, P., Barrero-Sicilia, C., Gómez-Cadenas, A., Carbonero, P., and Oñate-Sánchez, L. (2012). Arabidopsis thaliana DOF6 negatively affects germination in non-after-ripened seeds and interacts with TCP14. J. Exp. Bot. 63, 1937–1949. doi: 10.1093/jxb/err388
Shibasaki, K., Uemura, M., Tsurumi, S., and Rahman, A. (2009). Auxin Response in Arabidopsis under Cold Stress: underlying Molecular Mechanisms. Plant Cell 21, 3823–3838. doi: 10.1105/tpc.109.069906
Takeda, T., Amano, K., Ohto, M. A., Nakamura, K., Sato, S., Kato, T., et al. (2006). RNA interference of the Arabidopsis putative transcription factor TCP16 gene results in abortion of early pollen development. Plant Mol. Biol. 61, 165–177. doi: 10.1007/s11103-006-6265-9
Tian, C., Wan, P., Sun, S., Li, J., and Chen, M. (2004). Genome-wide analysis of the GRAS gene family in rice and Arabidopsis. Plant Mol. Biol. 54, 519–532. doi: 10.1023/B:PLAN.0000038256.89809.57
Wang, S. T., Sun, X. L., Hoshino, Y., Yu, Y., Jia, B., Sun, Z. W., et al. (2014). MicroRNA319 Positively Regulates Cold Tolerance by Targeting OsPCF6 and OsTCP21 in Rice (Oryza sativa L.). PLoS One 9:e91357. doi: 10.1371/journal.pone.0091357
Wang, X. W., Wang, H. Z., Wang, J., Sun, R., Wu, J., Liu, S. Y., et al. (2011). The genome of the mesopolyploid crop species Brassica rapa. Nat. Genet. 43, 1035–1039. doi: 10.1038/ng.919
Wei, W., Hu, Y., Cui, M. Y., Han, Y. T., Gao, K., and Feng, J. Y. (2016). Identification and Transcript Analysis of the TCP Transcription Factors in the Diploid Woodland Strawberry Fragaria vesca. Front. Plant Sci. 7:1937. doi: 10.3389/fpls.2016.01937
Xiao, B. Z., Chen, X., Xiang, C. B., Tang, N., Zhang, Q. F., and Xiong, L. Z. (2009). Evaluation of Seven Function-Known Candidate Genes for their Effects on Improving Drought Resistance of Transgenic Rice under Field Conditions. Mol. Plant 2, 73–83. doi: 10.1093/mp/ssn068
Xu, J. L., Zhang, W. J., and Xiang, F. N. (2021). Advances in stress inducible promoter and cis-acting elements in higher plants. J. Plant Physiol. 57, 759–766. doi: 10.13592/j.cnki.ppj.2020.0221
Yamaguchi-Shinozaki, K., and Shinozaki, K. (2006). Transcriptional Regulatory Networks in Cellular Responses and Tolerance to Dehydration and Cold Stresses. Annu. Rev. Plant Biol. 57, 781–803. doi: 10.1146/annurev.arplant.57.032905.105444
Yang, C., Li, D., Mao, D., Liu, X., Ji, C., Li, X., et al. (2013). Overexpression of microRNA319 impacts leaf morphogenesis and leads to enhanced cold tolerance in rice (Oryza sativa L.). Plant Cell Environ. 36, 2207–2218. doi: 10.1111/pce.12130
Yao, X., Ma, H., Wang, J., and Zhang, D. B. (2007). Genome-Wide Comparative Analysis and Expression Pattern of TCP Gene Families in Arabidopsis thaliana and Oryza sativa. J. Integr. Plant Biol. 49, 885–897. doi: 10.1111/j.1744-7909.2007.00509.x
Keywords: abiotic stress, expression analysis, subcellular localization, TCP, Pak-choi
Citation: Huang F, Shi C, Zhang Y and Hou X (2022) Genome-Wide Identification and Characterization of TCP Family Genes in Pak-Choi [Brassica campestris (syn. Brassica rapa) ssp. chinensis var. communis]. Front. Plant Sci. 13:854171. doi: 10.3389/fpls.2022.854171
Received: 13 January 2022; Accepted: 17 March 2022;
Published: 09 May 2022.
Edited by:
Mukesh Jain, Jawaharlal Nehru University, IndiaReviewed by:
Xiao-Li Tan, Jiangsu University, ChinaJianjun Zhao, Agricultural University of Hebei, China
Copyright © 2022 Huang, Shi, Zhang and Hou. This is an open-access article distributed under the terms of the Creative Commons Attribution License (CC BY). The use, distribution or reproduction in other forums is permitted, provided the original author(s) and the copyright owner(s) are credited and that the original publication in this journal is cited, in accordance with accepted academic practice. No use, distribution or reproduction is permitted which does not comply with these terms.
*Correspondence: Xilin Hou, aHhsQG5qYXUuZWR1LmNu
†These authors have contributed equally to this work