- 1Institute of Ecology, College of Urban and Environmental Sciences and Key Laboratory for Earth Surface Processes of Ministry of Education, Peking University, Beijing, China
- 2State Key Laboratory of Crop Genetics and Germplasm Enhancement, College of Resources and Environmental Sciences, Nanjing Agricultural University, Nanjing, China
Sulfur, widely present in the soil and atmosphere, is one of the essential elements for plants. Sulfate is a dominant form of sulfur in soils taken up by plant roots. In addition to the assimilation into sulfur compounds essential for plant growth and development, it has been reported recently that sulfate as well as other sulfur containing compounds can also induce stomatal movement. Here, we first summarized the uptake and transport of sulfate and atmospheric sulfur, including H2O and SO2, and then, focused on the effects of inorganic and organic sulfur on stomatal movement. We concluded all the transporters for different sulfur compounds, and compared the expression level of those transporters in guard cells and mesophyll cells. The relationship between abscisic acid and sulfur compounds in regulation of stomatal movement were also discussed.
Introduction
Sulfur is an essential macronutrient required for plant growth and development. Sulfur is a constituent of the amino acids cysteine (Cys) and methionine (Met) which are necessary for the synthesis of proteins and serve as precursors of important cofactors and sulfur containing secondary metabolites (Thomas et al., 2000; Mugford et al., 2011). The metabolites of sulfate assimilation and metabolism have important effects on plant growth, development, environmental response, resistance to biological and abiotic stress, crop quality and yield (Zhao et al., 1999; Hell et al., 2010; Gironde et al., 2014). Recent studies have suggested that sulfur containing compounds may also play a role in regulation of stomatal movement. Stomata are micro pores mainly found on leaf surface of terrestrial plants, controlled by two guard cells. CO2 and H2O exchanging through stomatal pores makes stomatal movement a key process for photosynthesis and drought resistance. In the present review, we focused on the transporters and summarized different sulfur compounds on regulating stomatal movement in details.
Sulfate Uptake in Roots and Long-Distance Transport
There are two sulfur uptake pathways in plants, including the sulfate uptake in roots and atmospheric sulfur entry pathway through stomata. Plants take up sulfur from soils by roots mainly in form of sulfate through sulfate transporters (SULTRs). In plants, genes encoding sulfate transporters are divided into four distinct subfamilies (SULTR1 to SULTR4) according to the similarity of the protein sequences (Vatansever et al., 2016). In the genome of Arabidopsis thaliana, there are eleven SULTR members and the functions of most of SULTRs have been comprehensively studied. In Arabidopsis, AtSULTR1;1 and AtSULTR1;2 are two high-affinity sulfate transporters responsible for sulfate uptake in roots (Figure 1A). AtSULTR1;2 is involved in the sulfate uptake in roots under sulfate sufficient condition, while AtSULTR1;1 is responsible for the absorption of sulfate under sulfate limitation condition (Hideki et al., 2000; Naoko et al., 2002; Rouached et al., 2008). AtSULTR1;2 is expressed in the root epidermal and cortical plasma membranes, and is co-localized with AtSULTR1;1 (Hideki et al., 2000; Naoko et al., 2002). Although sulfate deficiency induced the expressions of both AtSULTR1;1 and AtSULTR1;2, the induction of AtSULTR1;1 is much stronger than that of AtSULTR1;2 (Barberon et al., 2008; Rouached et al., 2008).
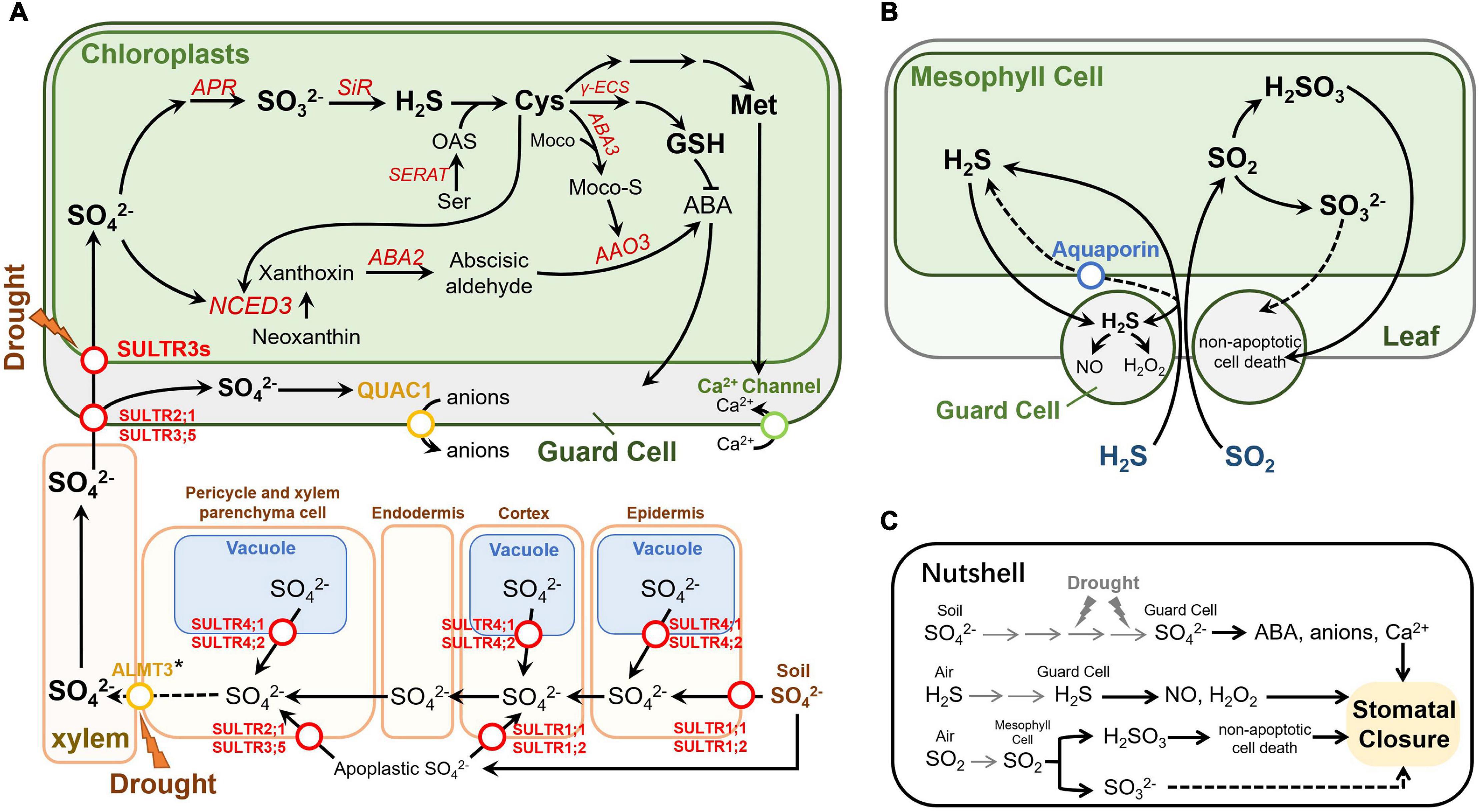
Figure 1. Effects of sulfur on stomatal movement in Arabidopsis thaliana. (A) Sulfate transport and metabolism in Arabidopsis under drought conditions. The sulfate transport function of ALMT3 marked with an asterisk was found in poplar trees (Malcheska et al., 2017). This figure partially refers to Takahashi et al. (2011) and Batool et al. (2018). (B) Transport and metabolism of exogenous H2S and SO2 in Arabidopsis. (C) A nutshell of the effect of sulfur on stomatal movement. Circles on the arrowed lines indicate transporters, and different transporter families are shown in different colors. Enzymes are indicated in red italics. Dashed arrows indicate disputable or putative pathways. Gray arrows indicate the transportation of the sulfur. Abbreviations not mentioned: ALMT3, putative aluminum-activated malate transporter 3.
After entering root cells, sulfate could be transported into the vacuole for storage or translocated to shoots for assimilation in plastids. The flux and allocation of sulfate in plants depends on the demand of plants organs and cells for sulfate, as well as sulfur status of the whole plant (Rennenberg and Herschbach, 2014; Spicer, 2014). The root-to-shoot or shoot-to-root long-distance transport of sulfur in high plants takes place in the vascular system which consists of xylem and phloem (Rennenberg et al., 1979). The existence of sulfate in phloem sap and phloem exudate has long been demonstrated (Toshiyuki et al., 1990). In rice, sulfate deprivation for 7 days results in a decrease in sulfate concentration in the phloem sap, which suggests that sulfate can be transferred between shoots and roots (Kuzuhara et al., 2000). In Arabidopsis, the loading sulfate into sieve tube of phloem is mediated by AtSULTR1;3 which is mainly expressed in the sieve element-companion cell complexes in the cotyledons and roots (Kuzuhara et al., 2000). In addition to phloem transport, xylem transport is also an important role of sulfate translocation (Yoshimoto et al., 2003). AtSULTR3;5 and AtSULTR2;1 are involved in loading sulfate to xylem parenchyma cell and facilitate the root-to-shoot translocation (Figure 1A; Kataoka et al., 2004a).
Remobilization of sulfate from vacuole is critical for maintenance of sulfur homeostasis in plants, particularly under sulfate limited condition. In Arabidopsis, it has been demonstrated that tonoplast-localized AtSULTR4;1 and AtSULTR4;2 facilitate the sulfate efflux from the vacuoles (Figure 1A) evidenced by the increase of vacuolar sulfate concentration in the atsultr4;1 atsultr4;2 double knockout mutant (Kataoka et al., 2004b). Interestingly, although both AtSULTR4;1 and AtSULTR4;2 are induced by sulfur deficiency, they display differential sulfur-dependent expression pattern in roots. AtSULTR4;2 can increase accumulation in respond to sulfate limitation while AtSULTR4;1 constitutively expresses under different sulfate conditions. Expression of AtSULTR4;1 in the sultr4;1 sultr4;2 double knockout mutant can rescue its phenotype indicating that AtSULTR4;1 plays a primary role in maintaining intracellular sulfate redistribution and homeostasis (Kataoka et al., 2004b; Martinoia et al., 2007). However, whether AtSULTR4;1 and AtSULTR 4;2 participate in the sulfate efflux from vacuole in guard cells is not clear.
Once translocation from roots to shoots, sulfate is transported into plastids for assimilation. In Arabidopsis, AtSULTR3 family proteins are involved in transport of sulfate into chloroplasts (Figure 1A). Simultaneous knockout of all five members of AtSULTR3 reduced sulfate accumulation in chloroplasts by more than 50% compared to the wild type (Chen et al., 2019). In plastids, sulfate is first converted to adenosine-5′-phosphosulfate (APS) by ATP sulfurylase (ATPS) (Logan et al., 1996; Thomas et al., 2000), and further reduced to sulfide in a two-step reduction reaction catalyzed by APS reductase (APR) and sulfite reductase (SiR) (Olivier et al., 2007; Khan et al., 2010a). In the last step of sulfate primary metabolism, cysteine (Cys) is produced by condensation of sulfide and O-acetylserine (OAS) catalyzed by OAS (thiol)lyase (OASTL) (Heeg et al., 2008). Cys serves as a precursor for the biosynthesis of Met, GSH and other sulfur containing compounds (Ravilious and Jez, 2012). In parallel, APS also can be phosphorylated to PAPS, which is involved into sulfation reaction in secondary metabolism as a precursor of active sulfate (Mugford et al., 2009, 2011).
Atmospheric Sulfur Uptake and Metabolism
In addition to absorb sulfate via roots, plants are also able to utilize foliary-absorbed sulfur gases as directly sulfur source, such as hydrogen sulfide (H2S) and sulfur dioxide (SO2) (Figure 1B; Herschbach et al., 1994; Sue et al., 2002; Aghajanzadeh et al., 2016). Atmospheric sulfur gases are derived from natural source and anthropogenic source. H2S and SO2 emitting by volcanic and geothermic activity are the main natural sulfur source while SO2 is the main anthropogenic sulfur source releasing from industrial processes and human life (Garrec, 1984; Stern, 2005). The kinetics of SO2 entry into plant leaves is correlated with stomatal conductance and SO2 level in the atmosphere (Noland and Kozlowski, 1979; By et al., 1996). SO2 is soluble in water phase in mesophyll cells, and reacts with water to release hydrogen ion (H+) and generate hydrogen sulfite (HSO3–). HSO3– can be directly reduced and assimilated into organic sulfur compounds in chloroplasts, or further oxidized into sulfate before entering the sulfur assimilation pathway (Noland and Kozlowski, 1979; De Bruyn et al., 1995). Different from SO2, the conductivity of mesophyll cells to H2S is largely determined by its metabolic rate in plants and the H2S level in atmosphere. Due to the poor solubility of H2S in water, H2S can be dissociated into H+ and hydrogen sulfide ion (HS–) in the atmosphere (By et al., 1996; Stuiver and De Kok, 2001; Lee et al., 2011).
The inorganic SO2 or H2S entry into plant leaves could be further assimilated into organic sulfur through sulfate assimilation pathway. Plants exposed to SO2 or H2S gases significantly increase the thiol content and change thiol composition in shoots (Aghajanzadeh et al., 2016; Ausma et al., 2021). In Arabidopsis, a short-term fumigation with 0.25 μl l–1 H2S strongly increase the concentrations of cysteine and glutathione by 20 and 4 times, respectively (Riemenschneider et al., 2005). However, the content and composition of glucosinolate in Brassica juncea and Brassica rapa were not affected by SO2 or H2S exposure regardless of sulfur sufficiency or deprivation (Aghajanzadeh et al., 2015). While SO2 and H2S exposure as sulfur compensation, can actually make foliar absorb more sulfur gas than sulfate-sufficient condition, but the absorption of sulfur nutrition in shoot does not affect the accumulation of transcript caused by sulfate limitation in roots, while their exposure can alleviated the up-regulated of APR, rather SULTR1;1, SULTR1;2 or OASTL (Stuiver and De Kok, 2001; Aleksandra et al., 2008; Birke et al., 2015). Although SO2 and H2S exposure may affect sulfate uptake in roots, the expression levels of SULTRs are independent on their exposure (Aleksandra et al., 2008). To be sure, SO2 and H2S is also a well-known toxic gas that can cause harm to plants at deleterious concentrations which may vary from plant species and environmental conditions (Thompson and Kats, 1978; Malhotra and Khan, 1984). It is controversial for determining what degree of foliar absorption contributes its toxification or helpfulness on account of variability of the growing environment and nutrient needs of different plants (Amaral et al., 2006; Yang et al., 2006; Lisjak et al., 2013).
Effects of Inorganic Sulfur on Stomatal Movement
Sulfate
Sulfate is the main inorganic sulfur form in plants, which has been found to induce stomatal closure under drought stress (Goodger et al., 2005; Ernst et al., 2010; Batool et al., 2018). Recent studies suggested that sulfate itself is not able to induce stomatal closure as knockout of key enzymes in sulfate assimilation pathway, such as SiR and Ser acetyltransferase (SERAT), abolishing the sulfate induced stomatal closure (Batool et al., 2018). Upon drought stress, plants increase the translocation of sulfate from root to shoot through xylem (Goodger et al., 2005). The accumulation of sulfate in shoots induces abscisic acid (ABA) synthesis through two paralleled pathways in Arabidopsis. In the first pathway, inorganic sulfate is reduced to organic sulfur compound Cys through sulfate assimilation pathway (Figure 1A). After that, using Cys and molybdenum cofactor (MoCo) as substrates, MoCo-S is synthesized by molybdenum cofactor sulfatase ABA3, thereby activating ABCISIC ALDEHYDE OXIDASE3 (AAO3) (Xiong et al., 2001; Wollers et al., 2008). Activated AAO3 could catalyze the final step in ABA biosynthesis (Seo et al., 2004). In the second parallel pathway, increased sulfate and synthesized Cys enhance the transcription of 9-cis-epoxycarotenoid dioxygenase 3 (NCED3), which is a drought-stress-induced isoform and provides a substrate precursor for AAO3, thus contributing to ABA biosynthesis (Nambara and Marion-Poll, 2005; Endo et al., 2008; Malcheska et al., 2017; Batool et al., 2018). The ABA induced by these two processes regulates the stomata closure through a series of signal transduction process (Blatt, 2000; Kim et al., 2010; Abhilasha and Choudhury, 2021). Moreover, the application of extracellular sulfate could directly regulate the R-Type anion channel QUICK ANION CHANNEL 1 (QUAC1), which was also known as aluminum-activated malate transporter 12 (ALMT12). The quac1 mutant fails to close stomata under the application of sulfate, indicating that QUAC1 is required for sulfate induced stomatal closure (Meyer et al., 2010; Malcheska et al., 2017).
The ion transport process after sulfate sensing in plants has not been studied in detail. Recently, it is speculated that sulfate may trigger a signal to close stomata in guard cells and vasculature. In guard cells, the completion of the above-mentioned processes requires to transport sulfate into plastids. This is because the reduction of sulfate and the beginning of ABA biosynthesis both occur in the plastid (Khan et al., 2010b). Members of the AtSULTR3 subfamily, preferentially expressed in the chloroplast membrane of leaves, are considered to be one of the most important sulfate transporters (Cao et al., 2013; Chen et al., 2019). Remarkably, transcription of four out of the five AtSULTR3 members (3;1, 3;2, 3;4, and 3;5) is enriched in guard cells (Bauer et al., 2013). The expression of AtSULTR 2;1, a AtSULTR3;5-activated sulfate transporter located on the plasma membrane, was also significantly higher than that of mesophyll cells (Leonhardt et al., 2004). Taken together, these results indicate that sulfate could be transported to guard cells even more efficiently than to mesophyll cells. The expression level of AtSULTR3;3 in mesophyll cells and guard cells is not significantly different, which may be due to the functionally redundant of SULTR3s, resulting in the total contribution of individual members higher than 100% (Cao et al., 2013). It does not seem necessary to increase the expression level of all SULTR3 members in guard cells. The unchanged expression levels of ATP-binding cassette (ABC) and triose-P/P-translocator (TPT), which are also plastid-localized backup sulfate transport systems, may also because the redundancy of SULTR3s (Hampp and Ziegler, 1977; Chen et al., 2003). Moreover, Chen et al. (2019) mutated all five members of the SULTR3 subfamily and found that in the quintuple mutants, in addition to the significant reduction in chloroplast sulfate absorption, the downstream Cys and ABA were also significantly reduced after the application of exogenous sulfate, and stomatal closure was also abolished. This defect could be compensated by adding sulfide or Cys to induce the stomatal closure (Cao et al., 2014). These results indicate that SULTR3s are also an important part of sulfate-induced stomatal signal transduction. Similarly, APR2, an enzyme that catalyzes the key step of sulfate reduction and tightly regulates the sulfate assimilation pathway, is also enriched in guard cells (Loudet et al., 2007; Bauer et al., 2013). The apr2 mutant accumulates less ABA than wild-type plants when external sulfate is applied (Cao et al., 2014), demonstrating that guard cells may be able to efficiently complete the sulfate assimilation process and produce ABA. This is supported by the fact that most of genes involved in sulfate assimilation are expressed in guard cells (Figure 2). Sulfate can also induce the transcription of NCED3 in guard cells, thereby accumulating ABA and promoting stomatal closure (Figure 1C; Malcheska et al., 2017).
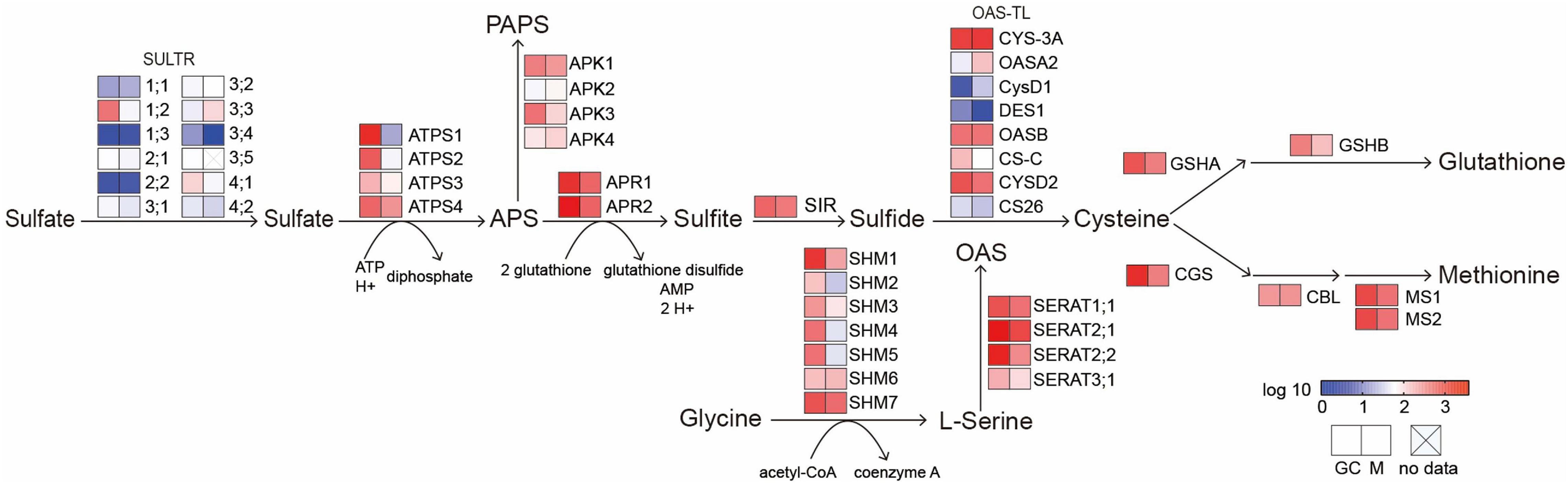
Figure 2. A diagram of sulfur metabolic pathways. Heat map indicates the expression levels of genes involved in sulfate transport and assimilation in Guard cells and mesophyll cells. SULTR, sulfate transporter; ATPS, ATP sulfurylase; APR, adenosine-5′-phosphosulfate reductase; APK, adenosine-5′-phosphosulfate (APS) kinase; SIR, sulfite reductase; OAS-TL, O-acetylserine (thiol) lyase; SERAT, serine acetyltransferase; SHM, serine hydroxymethyltransferase; GSHA, gamma-glutamylcysteine synthetase; GSHB, glutathione synthetase B; CGS, cystathionine gamma-synthase; CBL, cystathionine beta-lyase; MS, methionine synthase. GC, guard cells; M, mesophyll cells. Expression value derived from Arabidopsis eFP browser was normalized by log10 (http://bar.utoronto.ca/efp/cgi-bin/efpWeb.cgi).
In the vasculature, sulfate or Cys can also induce NCED3 transcription, suggesting that sulfate or Cys may also induce the synthesis of ABA in the vasculature. Consistent with this, the NCED3 transcription level and NCED3 protein level also increased significantly in the vasculature of drought-stressed plants (Endo et al., 2008). However, the detailed process remains to be determined.
Hydrogen Sulfide
The effect of H2S on stomatal movement has been well discussed in a recent review (Liu and Xue, 2021). Despite some controversy, most studies have shown that exogenous application of H2S can induce stomatal closure mainly in molecular form (Lisjak et al., 2010; Jin et al., 2013; Chen et al., 2020). Several phytohormones and signaling molecules, such as ABA, NO, hydrogen peroxide (H2O2), and 8-mercapto-cGMP, are also involved in H2S-induced stomatal movement (Figure 1C; Jin et al., 2013; Chen et al., 2020; Zhang et al., 2021). H2S also acts as a signal, interacts with various phytohormones (including ABA, ethylene, salicylic acid, and jasmonic acid) and other signaling molecules, such as NO and ROS, and regulates stomatal movement in response to biotic and abiotic stress (Garcia-Mata and Lamattina, 2001, 2010; Hou et al., 2013; Deng et al., 2020; Zhang et al., 2021). Furthermore, H2S mediates post-translation modification of protein through phosphorylation and S-persulfidation to control ABA-dependent stomatal closure. The persulfidation of DES1, a pivotal enzyme producing H2S, was induced by ABA accompanied by synthesis of ROS (Shen et al., 2020; Zhang et al., 2021). Persulfidation-base modification change the structure of the key kinase protein SNF1-RELATED PROTEIN KINASE 2.6 (SnKR2.6) in ABA signaling pathway, resulting in enhanced kinase activity, and the phosphorylation modification level at key sites of SnKR2.6 protein can positively regulate H2S-mediated sulfhydrylation modification (Chen et al., 2021).
Notably, H2S, as a highly lipophilic gaseous signaling molecule, can freely pass through the phospholipid membrane for signal transmission (Li and Moore, 2008). It is speculated that some channel proteins may contribute to H2S transport and increase the efficiency of H2S permeation (Figure 1B). Because the structure of H2S is as similar as of H2O, there is a hypothesis that aquaporins can promote the absorption of H2S (Lee et al., 2005). Aquaporins are membrane channels widely found in plants, animals, and microbe, which promote the passage of water and small neutral molecules through cell membranes (Maurel et al., 1993). By analyzing the crystal structure of the aquaporin-M (ApqM) in Methanothermobacter marburgensis, Lee et al. (2005) found that one of its pore geometries could easily accommodate H2S, therefore speculating that AqpM could promote the absorption of H2S. However, through the study of the aquaporin in a sulfide-reducing bacteria Archaeoglobus fulgidus (AfAQP), an evolutionarily close protein of AqpM, it was found that AfAQP cannot promote the absorption of H2S (Mathai et al., 2009). The homologous proteins of AfAQP also exist in plants (such as Aquaporin TIP 3;2 and NIP1;2 in Arabidopsis), but the H2S transport of these aquaporins has not been studied.
Sulfur Dioxide
Sulfur dioxide could be absorbed through stomata and dissolved in the cytoplasm, and is hydrolyzed to sulfurous acid (H2SO3), bisulfite ion (HSO3–) and sulfite ion (SO32–) according to the pH value (Figure 1B). However, the effect of SO2 on stomatal movement in plants is controversial. Different studies reported that SO2 could decrease (Winner and Mooney, 1980; Olszyk and Tibbitts, 1981; Rao et al., 1983), no change (Van der Kooij et al., 1997; Hu et al., 2014), or increase the stomatal aperture (Mansfield and Majernik, 1970; Black and Black, 1979; Biscoe et al., 2006). The different results may be due to different sulfur dioxide concentrations or different plant species. Recently, it was proposed that SO2 mainly induces stomatal closure in the form of H2SO3 (Figure 1C). The stomatal movement induced by SO2 is different from that induced by O3 and CO2, and is mainly caused by non-apoptotic cell death that does not depend on pH changes (Figure 1C; Ooi et al., 2019). This result is inconsistent with the previous study that SO2-induced stomatal movement depends on ABA accumulation (Taylor et al., 1981). Moreover, low concentration of SO2 could also induce stomatal opening under light and meanwhile stimulate cell death in guard cells in Arabidopsis (Ooi et al., 2019).
Sulfite
The sulfite in plants is mainly derived from the reduction of sulfate, the degradation of Cys and methionine, and the hydrolysis of atmospheric SO2 in the apoplastic mesophyll (Yi and Meng, 2003; Hansch and Mendel, 2005). Sulfite could be further reduced to sulfide by SiR or oxidized to sulfate by peroxisome-localized sulfite oxidase (SO). Long-term effects of sulfite on stomatal movement is usually associated with SO2. Therefore, similar to SO2, the effects of sulfite on stomatal movement, transpiration, and water loss in sulfite-applied plants is not conclusive (see SO2 section). Recently, overexpression of APR2 or knock down of SO by RNAi which both increase sulfite accumulation could induce stomatal opening and increase water loss, suggesting sulfite more like promote the stomatal opening rather closure (Bekturova et al., 2021).
Effects of Organic Sulfur Compounds on Stomatal Movement
Several organic sulfur compounds could also induce stomatal closure. Batool et al. (2018) found that the application of Cys could reduce stomatal aperture in Arabidopsis, and the sulfate-induced stomatal closure is dependent on the biosynthesis of Cys. GSH is involved in ABA-induced stomata closure (Okuma et al., 2011). Decreasing GSH level in guard cells in the GSH biosynthesis deficient mutant cad2-1 or inhibition of GSH biosynthesis enhanced ABA-induced stomatal closure (Figure 1A). Overexpression of gamma-glutamylcysteine synthetase (γ-ECS), a rate-limiting enzyme in GSH biosynthesis, significantly reduced the stomatal aperture and density (Lu et al., 2021). Moreover, exogenous application of L-methionine (L-Met) has been shown to enhance stomatal closure by activating Ca2+ channels and generation of reactive oxygen species (ROS) (Kong et al., 2016).
Conclusion and Future Perspectives
Whether from soil or atmosphere, sulfur compounds mainly cause stomatal closure, like stress signal, in an ABA dependent or independent pathway. Except for sulfate, the transporters of H2S and SO2 are still unrevealed. The underlying mechanisms of the relationship between drought stress and sulfate accumulation in guard cells need to be investigated in the future.
Author Contributions
ZR and R-YW wrote the manuscript. X-YH and YW reviewed and edited the manuscript. All authors have discussed and approved the submitted version of the manuscript.
Funding
This work was funded by the National Natural Science Foundation of China (31970271 to X-YH and 31972937 to YW).
Conflict of Interest
The authors declare that the research was conducted in the absence of any commercial or financial relationships that could be construed as a potential conflict of interest.
The reviewer YX declared a shared affiliation with several of the authors, R-YW and X-YH, to the handling editor at the time of the review.
Publisher’s Note
All claims expressed in this article are solely those of the authors and do not necessarily represent those of their affiliated organizations, or those of the publisher, the editors and the reviewers. Any product that may be evaluated in this article, or claim that may be made by its manufacturer, is not guaranteed or endorsed by the publisher.
References
Abhilasha, A., and Choudhury, R. S. (2021). Molecular and physiological perspectives of abscisic acid mediated drought adjustment strategies. Plants 10, 1–20. doi: 10.3390/plants10122769
Aghajanzadeh, T., Hawkesford, M. J., and De Kok, L. J. (2016). Atmospheric H2S and SO2 as sulfur sources for Brassica juncea and Brassica rapa: Regulation of sulfur uptake and assimilation. Environ. Exp. Bot. 124, 1–10. doi: 10.1016/j.envexpbot.2015.12.001
Aghajanzadeh, T., Kopriva, S., Hawkesford, M. J., Koprivova, A., and De Kok, L. J. (2015). Atmospheric H2S and SO2 as sulfur source for Brassica juncea and Brassica rapa: impact on the glucosinolate composition. Front. Plant Sci. 6:1–6. doi: 10.3389/fpls.2015.00924
Aleksandra, K., CElisabethE, S., FreekS, P., Stanislav, K., Hawkesford, M., and De Kok, L. J. (2008). Regulation of sulfate uptake, expression of the sulfate transporters Sultr1;1 and Sultr1;2, and APS reductase in Chinese cabbage (Brassica pekinensis) as affected by atmospheric H2S nutrition and sulfate deprivation. Funct. Plant Biol. 35, 318–327. doi: 10.1093/mp/ssq001
Amaral, A., Rodrigues, V., Oliveira, J., Pinto, C., Carneiro, V., et al. (2006). Chronic exposure to volcanic environments and cancer incidence in the Azores, Portugal. Sci. Total Environ. 367, 123–128. doi: 10.1016/j.scitotenv.2006.01.024
Ausma, T., Bansal, V., Kraaij, M., Verloop, A. C. M., Gasperl, A., et al. (2021). Floral displays suffer from sulphur deprivation. Environ. Exp. Bot. 192, 1–9. doi: 10.1016/j.envexpbot.2021.104656
Barberon, M., Berthomieu, P., Clairotte, M., Shibagaki, N., Davidian, J. C., et al. (2008). Unequal functional redundancy between the two Arabidopsis thaliana high-affinity sulphate transporters SULTR1;1 and SULTR1;2. New Phytol. 180, 608–619. doi: 10.1111/j.1469-8137.2008.02604.x
Batool, S., Uslu, V. V., Rajab, H., Ahmad, N., Waadt, R., Geiger, D., et al. (2018). Sulfate is Incorporated into Cysteine to Trigger ABA Production and Stomatal Closure. Plant Cell 30, 2973–2987. doi: 10.1105/tpc.18.00612
Bauer, H., Ache, P., Lautner, S., Fromm, J., Hartung, W., Al-Rasheid, K. A., et al. (2013). The stomatal response to reduced relative humidity requires guard cell-autonomous ABA synthesis. Curr. Biol. 23, 53–57. doi: 10.1016/j.cub.2012.11.022
Bekturova, A., Oshanova, D., Tiwari, P., Nurbekova, Z., Kurmanbayeva, A., Soltabayeva, A., et al. (2021). Adenosine 5’-phosphosulfate reductase and sulfite oxidase regulate sulfite-induced water loss in Arabidopsis. J. Exp. Bot. 72, 6447–6466. doi: 10.1093/jxb/erab249
Birke, H., De Kok, L. J., Wirtz, M., and Hell, R. (2015). The role of compartment-specific cysteine synthesis for sulfur homeostasis during H2S exposure in Arabidopsis. Plant Cell Physiol. 56, 358–367. doi: 10.1093/pcp/pcu166
Biscoe, P. V., Unsworth, M. H., and Pinckney, H. R. (2006). The effects of low concentrations of sulfur dioxide on stomatal behavior in Vicia faba. New Phytol. 72, 1299–1306. doi: 10.1111/j.1469-8137.1973.tb02106.x
Black, C. R., and Black, V. J. (1979). The effects of low concentrations of sulphur dioxide on stomatal conductance and epidermal cell survival in field bean (Vicia faba L.). J. Exp. Bot. 30, 291–298. doi: 10.1093/jxb/30.2.291
Blatt, R. M. (2000). Cellular signaling and volume control in stomatal movements in plants. Annu. Rev. Plant Biol. 16, 221–241. doi: 10.1146/annurev.cellbio.16.1.221
By, S. S., Siegmund, A., Fuhrer, H. W., and Heber, U. (1996). Stomatal uptake of SO2, NOx and O3 by spruce crowns (Picea abies) and canopy damage in Central Europe. New Phytol. 132, 661–676. doi: 10.1111/j.1469-8137.1996.tb01884.x
Cao, M. J., Wang, Z., Wirtz, M., Hell, R., Oliver, D. J., and Xiang, C. B. (2013). SULTR3;1 is a chloroplast-localized sulfate transporter in Arabidopsis thaliana. Plant J. 73, 607–616. doi: 10.1111/tpj.12059
Cao, M. J., Wang, Z., Zhao, Q., Mao, J. L., Speiser, A., Wirtz, M., et al. (2014). Sulfate availability affects ABA levels and germination response to ABA and salt stress in Arabidopsis thaliana. Plant J. 77, 604–615. doi: 10.1111/tpj.12407
Chen, H. C., Yokthongwattana, K., Newton, A. J., and Melis, A. (2003). SulP, a nuclear gene encoding a putative chloroplast-targeted sulfate permease in Chlamydomonas reinhardtii. Planta 218, 98–106. doi: 10.1007/s00425-003-1076-6
Chen, S. S., Wang, X. F., Jia, H. L., Li, F. l, Ma, Y., et al. (2021). Persulfidation-induced structural change in SnRK2.6 establishes intramolecular interaction between phosphorylation and persulfidation. Mol. Plant 14, 1814–1830. doi: 10.1016/j.molp.2021.07.002
Chen, S., Jia, H., Wang, X., Shi, C., Wang, X., Ma, P., et al. (2020). Hydrogen Sulfide Positively Regulates Abscisic Acid Signaling through Persulfidation of SnRK2.6 in Guard Cells. Mol. Plant 13, 732–744. doi: 10.1016/j.molp.2020.01.004
Chen, Z., Zhao, P. X., Miao, Z. Q., Qi, G. F., Wang, Z., Yuan, Y., et al. (2019). SULTR3s function in chloroplast sulfate uptake and affect aba biosynthesis and the stress response. Plant Physiol. 180, 593–604. doi: 10.1104/pp.18.01439
De Bruyn, W. J., Swartz, E., Hu, J. H., Shorter, J. A., Davidovits, P., Worsnop, D. R., et al. (1995). Henry’s law solubilities and etchenow coefficients for biogenic reduced sulfur species obtained from gas-liquid uptake measurements. J. Geophys. Res. 100, 7245–7251. doi: 10.1029/95JD00217
Deng, G., Zhou, L., Wang, Y., Zhang, G., and Chen, X. (2020). Hydrogen sulfide acts downstream of jasmonic acid to inhibit stomatal development in Arabidopsis. Planta 251:42. doi: 10.1007/s00425-019-03334-9
Endo, A., Sawada, Y., Takahashi, H., Okamoto, M., Ikegami, K., Koiwai, H., et al. (2008). Drought induction of Arabidopsis 9-cis-epoxycarotenoid dioxygenase occurs in vascular parenchyma cells. Plant Physiol. 147, 1984–1993. doi: 10.1104/pp.108.116632
Ernst, L., Goodger, J. Q. D., Alvarez, S., Marsh, E. L., Berla, B., Lockhart, E., et al. (2010). Sulphate as a xylem-borne chemical signal precedes the expression of ABA biosynthetic genes in maize roots. J. Exp. Bot. 61, 3395–3405. doi: 10.1093/jxb/erq160
Garcia-Mata, C., and Lamattina, L. (2001). Nitric oxide induces stomatal closure and enhances the adaptive plant responses against drought stress. Plant Physiol. 126, 1196–1204. doi: 10.1104/pp.126.3.1196
Garcia-Mata, C., and Lamattina, L. (2010). Hydrogen sulphide, a novel gasotransmitter involved in guard cell signalling. New Phytol. 188, 977–984. doi: 10.1111/j.1469-8137.2010.03465.x
Garrec, J. P. (1984). Impact of Volcanic Fluoride and SO2 Emissions from moderated activity volcanoes on the surrounding vegetation. Bulletin Volcanol. 47, 491–496. doi: 10.1007/BF01961221
Gironde, A., Dubousset, L., Trouverie, J., Etienne, P., and Avice, J. C. (2014). The impact of sulfate restriction on seed yield and quality of winter oilseed rape depends on the ability to remobilize sulfate from vegetative tissues to reproductive organs. Front. Plant Sci. 5:1–13. doi: 10.3389/fpls.2014.00695
Goodger, J. Q., Sharp, R. E., Marsh, E. L., and Schachtman, D. P. (2005). Relationships between xylem sap constituents and leaf conductance of well-watered and water-stressed maize across three xylem sap sampling techniques. J. Exp. Bot. 56, 2389–2400. doi: 10.1093/jxb/eri231
Hampp, R., and Ziegler, I. (1977). Sulfate and sulfite translocation via the phosphate translocator of the inner envelope membrane of chloroplasts. Planta 137, 309–312. doi: 10.1007/BF00388168
Hansch, R., and Mendel, R. R. (2005). Sulfite oxidation in plant peroxisomes. Photosynth. Res. 86, 337–343. doi: 10.1007/s11120-005-5221-x
Heeg, C., Kruse, C., Jost, R., Gutensohn, M., Ruppert, T., et al. (2008). Analysis of the Arabidopsis O-acetylserine(thiol)lyase gene family demonstrates compartment-specific differences in the regulation of cysteine synthesis. Plant Cell 20, 168–185. doi: 10.1105/tpc.107.056747
Hell, R., Khan, M. S., and Wirtz, M. (2010). Cell biology of metals and nutrients. Switzerland: Springer Verlag press, 243–279.
Herschbach, C., De Kok, L. J., and Rennenberg, H. (1994). Net uptake of sulfate and its transport to the shoot in spinach plants fumigated with H2S or SO2: does atmospheric sulfur affect the “inter-organ” regulation of sulfur nutrition? Acta Botanica 108, 41–46. doi: 10.1111/j.1438-8677.1995.tb00829.x
Hideki, T., Frank, W. S., Mechteld, B.-K., Malcolm, J. H., and Kazuki, S. (2000). The roles of three functional sulphate transporters involved in uptake and translocation of sulphate in Arabidopsis thaliana. Plant J. 23, 171–182. doi: 10.1046/j.1365-313x.2000.00768.x
Hou, Z., Wang, L., Liu, J., Hou, L., and Liu, X. (2013). Hydrogen sulfide regulates ethylene-induced stomatal closure in Arabidopsis thaliana. J. Integr. Plant Biol. 55, 277–289. doi: 10.1111/jipb.12004
Hu, K. D., Tang, J., Zhao, D. L., Hu, L. Y., Li, Y. H., Liu, Y. S., et al. (2014). Stomatal closure in sweet potato leaves induced by sulfur dioxide involves H2S and NO signaling pathways. Biol. Plant 58, 676–680. doi: 10.1007/s10535-014-0440-7
Jin, Z., Xue, S., Luo, Y., Tian, B., Fang, H., Li, H., et al. (2013). Hydrogen sulfide interacting with abscisic acid in stomatal regulation responses to drought stress in Arabidopsis. Plant Physiol. Biochem. 62, 41–46. doi: 10.1016/j.plaphy.2012.10.017
Kataoka, T., Hayashi, N., Yamaya, T., and Takahashi, H. (2004a). Root-to-shoot transport of sulfate in Arabidopsis evidence for the role of SULTR3;5 as a component of low-affinity sulfate transport system in the root vasculature. Plant Physiol. 136, 4198–4204. doi: 10.1104/pp.104.045625
Kataoka, T., Watanabe-Takahashi, A., Hayashi, N., Ohnishi, M., Mimura, T., et al. (2004b). Vacuolar sulfate transporters are essential determinants controlling internal distribution of sulfate in Arabidopsis. Plant Cell 16, 2693–2704. doi: 10.1105/tpc.104.023960
Khan, M. S., Haas, F. H., Samami, A. A., Gholami, A. M., Bauer, A., et al. (2010a). Sulfite reductase defines a newly discovered bottleneck for assimilatory sulfate reduction and is essential for growth and development in Arabidopsis thaliana. Plant Cell 22, 1216–1231.
Khan, M. S., Haas, F. H., Samami, A. A., Gholami, A. M., Bauer, A., Fellenberg, K., et al. (2010b). Sulfite reductase defines a newly discovered bottleneck for assimilatory sulfate reduction and is essential for growth and development in Arabidopsis thaliana. Plant Cell 22, 1216–1231. doi: 10.1105/tpc.110.074088
Kim, T., Böhmer, M., Hu, H.-H., Nishimura, N., Julian, I., and Schroeder, I. J. (2010). Guard cell signal transduction network: advances in understanding abscisic acid, CO2, and Ca2+ signaling. Annu. Rev. Plant Biol. 61, 561–591. doi: 10.1146/annurev-arplant-042809-112226
Kong, D., Hu, H. C., Okuma, E., Lee, Y., Lee, H. S., Munemasa, S., et al. (2016). L-Met activates Arabidopsis GLR Ca2+ Channels Upstream of ROS Production and Regulates Stomatal Movement. Cell. Rep. 17, 2553–2561. doi: 10.1016/j.celrep.2016.11.015
Kuzuhara, Y., Isobe, A., Awazuhara, M., Fujiwara, T., and Hayashi, H. (2000). Glutathione levels in phloem sap of rice plants under sulfur deficient conditions. Soil. Sci. Plant. Nutr. 46, 265–270. doi: 10.1080/00380768.2000.10408782
Lee, J. K., Kozono, D., Remis, J., Kitagawa, Y., Agre, P., and Stroud, R. M. (2005). Structural basis for conductance by the archaeal aquaporin AqpM at 1.68 angstrom. Proc. Natl. Acad. Sci. U S A. 102, 18932–18937. doi: 10.1073/pnas.0509469102
Lee, Z. W., Zhou, J., Chen, C. S., Zhao, Y., Tan, C. H., et al. (2011). The slow-releasing hydrogen sulfide donor, GYY4137, exhibits novel anti-cancer effects in vitro and in vivo. PLoS One 6:1–7. doi: 10.1371/journal.pone.0021077
Leonhardt, N., Kwak, J. M., Robert, N., Waner, D., Leonhardt, G., and Schroeder, J. I. (2004). Microarray expression analyses of Arabidopsis guard cells and isolation of a recessive abscisic acid hypersensitive protein phosphatase 2C mutant. Plant Cell 16, 596–615. doi: 10.1105/tpc.019000
Li, L., and Moore, P. K. (2008). Putative biological roles of hydrogen sulfide in health and disease: a breath of not so fresh air? Trends Pharmacol. Sci. 29, 84–90. doi: 10.1016/j.tips.2007.11.003
Lisjak, M., Srivastava, N., Teklic, T., Civale, L., Lewandowski, K., Wilson, I., et al. (2010). A novel hydrogen sulfide donor causes stomatal opening and reduces nitric oxide accumulation. Plant Physiol. Biochem. 48, 931–935. doi: 10.1016/j.plaphy.2010.09.016
Lisjak, M., Teklic, T., Wilson, I. D., Whiteman, M., and Hancock, J. T. (2013). Hydrogen sulfide: environmental factor or signalling molecule? Plant Cell Environ. 36, 1607–1616. doi: 10.1111/pce.12073
Liu, H., and Xue, S. (2021). Interplay between hydrogen sulfide and other signaling molecules in the regulation of guard cell signaling and abiotic/biotic stress response. Plant Commun. 2:100179. doi: 10.1016/j.xplc.2021.100179
Logan, H. M., Cathala, N., Grignon, C., and Davidian, J. C. (1996). Cloning of a cDNA encoded by a member of the Arabidopsis thaliana ATP sulfurylase multigene family. Expression studies in yeast and in relation to plant sulfur nutrition. J. Biol. Chem. 271, 12227–12233. doi: 10.1074/jbc.271.21.12227
Loudet, O., Saliba-Colombani, V., Camilleri, C., Calenge, F., Gaudon, V., Koprivova, A., et al. (2007). Natural variation for sulfate content in Arabidopsis thaliana is highly controlled by APR2. Nat. Genet. 39, 896–900. doi: 10.1038/ng2050
Lu, B., Luo, X., Gong, C., and Bai, J. (2021). Overexpression of gamma-glutamylcysteine synthetase gene from Caragana korshinskii decreases stomatal density and enhances drought tolerance. BMC Plant Biol. 21:1–13. doi: 10.1186/s12870-021-03226-9
Malcheska, F., Ahmad, A., Batool, S., Muller, H. M., Ludwig-Muller, J., Kreuzwieser, J., et al. (2017). Drought-enhanced xylem sap sulfate closes stomata by affecting ALMT12 and guard cell ABA synthesis. Plant Physiol. 174, 798–814. doi: 10.1104/pp.16.01784
Malhotra, S. S., and Khan, A. A. (1984). “Biochemical and physiological impacts of major pollutants,” in the Air Pollution and Plant Life, ed. M. Treshovv (New York, NY: John Wiley & Sons Ltd), 113–157.
Mansfield, T. A., and Majernik, O. (1970). Can stomata play a part in protecting plants against air pollutants? Environ. Pollut. 1, 149–154. doi: 10.1016/0013-9327(70)90015-7
Martinoia, E., Maeshima, M., and Neuhaus, H. E. (2007). Vacuolar transporters and their essential role in plant metabolism. J. Exp. Bot. 58, 83–102. doi: 10.1093/jxb/erl183
Mathai, J. C., Missner, A., Kugler, P., Saparov, S. M., Zeidel, M. L., Lee, J. K., et al. (2009). No facilitator required for membrane transport of hydrogen sulfide. Proc. Natl. Acad. Sci. 106, 16633–16638. doi: 10.1073/pnas.0902952106
Maurel, C., Reizer, J., Schroeder, J. I., and Chrispeels, M. J. (1993). The Vacuolar Membrane-Protein Gamma-Tip Creates Water Specific Channels in Xenopus-Oocytes. EMBO J. 12, 2241–2247. doi: 10.1002/j.1460-2075.1993.tb05877.x
Meyer, S., Mumm, P., Imes, D., Endler, A., Weder, B., Al-Rasheid, K. A., et al. (2010). AtALMT12 represents an R-type anion channel required for stomatal movement in Arabidopsis guard cells. Plant J. 63, 1054–1062. doi: 10.1111/j.1365-313X.2010.04302.x
Mugford, S. G., Lee, B.-R., Koprivova, A., Matthewman, C., and Kopriva, S. (2011). Control of sulfur partitioning between primary and secondary metabolism. Plant J. 65, 96–105. doi: 10.1111/j.1365-313X.2010.04410.x
Mugford, S. G., Yoshimoto, N., Reichelt, M., Wirtz, M., Hill, L., et al. (2009). Disruption of adenosine-5’-phosphosulfate kinase in Arabidopsis reduces levels of sulfated secondary metabolites. Plant Cell 21, 910–927. doi: 10.1105/tpc.109.065581
Nambara, E., and Marion-Poll, A. (2005). Abscisic acid biosynthesis and catabolism. Annu. Rev. Plant Biol. 56, 165–185. doi: 10.1146/annurev.arplant.56.032604.144046
Naoko, Y., Frank, W. S., Tomoyuki, Y., and Kazuki, S. (2002). Two distinct high-affinity sulfate transporters with different inducibilities mediate uptake of sulfate in Arabidopsis roots. Plant J. 29, 465–473. doi: 10.1046/j.0960-7412.2001.01231.x
Noland, T. L., and Kozlowski, T. T. (1979). Effect of SO2 on stomatal aperture and sulfur uptake of woody angiosperm seedlings. Can. J. Forest Res. 9, 57–62. doi: 10.1139/x79-010
Okuma, E., Jahan, M. S., Munemasa, S., Hossain, M. A., Muroyama, D., Islam, M. M., et al. (2011). Negative regulation of abscisic acid-induced stomatal closure by glutathione in Arabidopsis. J. Plant Physiol. 168, 2048–2055. doi: 10.1016/j.jplph.2011.06.002
Olivier, L., Vera, S. C., Christine, C., Fanny, C., Virginie, G., et al. (2007). Natural variation for sulfate content in Arabidopsis thaliana is highly controlled by APR2. Nat. Genet. 39, 896–900.
Olszyk, D. M., and Tibbitts, T. W. (1981). Stomatal Response and Leaf Injury of Pisum sativum L. with SO2 and O3 Exposures. Plant Physiol. 67, 539–544. doi: 10.1104/pp.67.3.539
Ooi, L., Matsuura, T., Munemasa, S., Murata, Y., Katsuhara, M., Hirayama, T., et al. (2019). The mechanism of SO2 -induced stomatal closure differs from O3 and CO2 responses and is mediated by nonapoptotic cell death in guard cells. Plant Cell Environ. 42, 437–447. doi: 10.1111/pce.13406
Rao, I. M., Amundson, R. G., Alscher-Herman, R., and Anderson, L. E. (1983). Effects of SO2 on Stomatal Metabolism in Pisum sativum L. Plant Physiol. 72, 573–577. doi: 10.1104/pp.72.2.573
Ravilious, G. E., and Jez, J. M. (2012). Structural biology of plant sulfur metabolism: from assimilation to biosynthesis. Nat. Prod. Rep. 29, 1138–1152. doi: 10.1039/c2np20009k
Rennenberg, H., and Herschbach, C. (2014). A detailed view on sulphur metabolism at the cellular and whole-plant level illustrates challenges in metabolite flux analyses. J. Exp. Bot. 65, 5711–5724. doi: 10.1093/jxb/eru315
Rennenberg, H., Schmitz, K., and Bergmann, L. (1979). Long-Distance Transport of Sulfur in Nicotiana tabacum. Planta 147, 57–62. doi: 10.1007/bf00384591
Riemenschneider, A., Nikiforova, V., Hoefgen, R., De Kok, L. J., and Papenbrock, J. (2005). Impact of elevated H2S on metabolite levels, activity of enzymes and expubion of genes involved in cysteine metabolism. Plant Physiol. Biochem. 43, 473–483. doi: 10.1016/j.plaphy.2005.04.001
Rouached, H., Wirtz, M., Alary, R., Hell, R., Arpat, A. B., et al. (2008). Differential regulation of the expression of two high-affinity sulfate transporters, SULTR1.1 and SULTR1.2, in Arabidopsis. Plant Physiol. 147, 897–911. doi: 10.1104/pp.108.118612
Seo, M., Aoki, H., Koiwai, H., Kamiya, Y., Nambara, E., and Koshiba, T. (2004). Comparative studies on the Arabidopsis aldehyde oxidase (AAO) gene family revealed a major role of AAO3 in ABA biosynthesis in seeds. Plant Cell Physiol. 45, 1694–1703. doi: 10.1093/pcp/pch198
Shen, J., Zhang, J., Zhou, M. J., Zhou, H., Cui, B. M., et al. (2020). Persulfidation-based Modification of Cysteine Desulfhydrase and the NADPH Oxidase RBOHD Controls Guard Cell Abscisic Acid Signaling. Plant Cell 32, 1000–1017. doi: 10.1105/tpc.19.00826
Spicer, R. (2014). Symplasmic networks in secondary vascular tissues: parenchyma distribution and activity supporting long-distance transport. J. Exp. Bot. 65, 1829–1848. doi: 10.1093/jxb/ert459
Stern, D. I. (2005). Global sulfur emissions from 1850 to 2000. Chemosphere 58, 163–175. doi: 10.1016/j.chemosphere.2004.08.022
Stuiver, C., and De Kok, L. (2001). Atmospheric H2S as sulfur source for Brassica oleracea: kinetics of H2S uptake and activity of O-acetylserine (thiol)lyase as affected by sulfur nutrition. Environ. Exp. Bot. 46, 29–36. doi: 10.1016/S0098-8472(01)00080-6
Sue, W., Ineke, S., Marianne, S., Christian, B., and De Kok, L. J. (2002). Atmospheric H2S as sulphur source for Brassica oleracea: Consequences for the activity of the enzymes of the assimilatory sulphate reduction pathway. Plant Physiol. Bioch. 39, 425–432. doi: 10.1016/S0981-9428(01)01258-X
Takahashi, H., Kopriva, S., Giordano, M., Saito, K., and Hell, R. (2011). Sulfur assimilation in photosynthetic organisms: molecular functions and regulations of transporters and assimilatory enzymes. Annu. Rev. Plant Biol. 62, 157–184. doi: 10.1146/annurev-arplant-042110-103921
Taylor, J. S., Reid, D. M., and Pharis, R. P. (1981). Mutual Antagonism of Sulfur Dioxide and Abscisic Acid in Their Effect on Stomatal Aperture in Broad Bean (Vicia faba L.) Epidermal Strips. Plant Physiol. 68, 1504–1507. doi: 10.1104/pp.68.6.1504
Thomas, L., Melinda, N. M., Julie-Ann, B., and John, P. D. (2000). Pathways and regulation of sulfur metabolism revealed through molecular and genetic studies. Annu. Rev. 51, 141–165. doi: 10.1146/annurev.arplant.51.1.141
Thompson, C. R., and Kats, G. (1978). Effects of continuous hydrogen sulphide fumigation on crop and forest plants. Environ. Sci. Technol. 12, 550–553. doi: 10.1021/es60141a001
Toshiyuki, O., Hiroaki, H., and Mitsuo, C. (1990). Collection and chemical composition of pure phloem sap from Zea mays L. Plant Cell Physiol. 31, 735–737. doi: 10.1093/oxfordjournals.pcp.a077972
Van der Kooij, T., de Kok, L. J., Haneklaus, S., and Schnug, E. (1997). Uptake and metabolism of sulphur dioxide by Arabidopsis thaliana. New Phytol. 135, 101–107. doi: 10.1046/j.1469-8137.1997.00619.x
Vatansever, R., Koc, I., Ozyigit, I. I., Sen, U., Uras, M. E., et al. (2016). Genome-wide identification and expression analysis of sulfate transporter (SULTR) genes in potato (Solanum tuberosum L.). Planta 244, 1167–1183. doi: 10.1007/s00425-016-2575-6
Winner, W. E., and Mooney, H. A. (1980). Responses of hawaiian plants to volcanic sulfur dioxide: stomatal behavior and foliar injury. Science 210, 789–791. doi: 10.1126/science.210.4471.789
Wollers, S., Heidenreich, T., Zarepour, M., Zachmann, D., Kraft, C., Zhao, Y., et al. (2008). Binding of sulfurated molybdenum cofactor to the C-terminal domain of ABA3 from Arabidopsis thaliana provides insight into the mechanism of molybdenum cofactor sulfuration. J. Biol. Chem. 283, 9642–9650. doi: 10.1074/jbc.M708549200
Xiong, L., Ishitani, M., Lee, H., and Zhu, J. K. (2001). The Arabidopsis LOS5/ABA3 locus encodes a molybdenum cofactor sulfurase and modulates cold stress- and osmotic stress-responsive gene expression. Plant Cell 13, 2063–2083. doi: 10.1105/tpc.010101
Yang, L., Stulen, I., and De Kok, L. J. (2006). Impact of sulfate nutrition on the utilization of atmospheric SO2 as sulfur source for Chinese cabbage. J. Plant Nutr. Soil. Sci. 169, 529–534. doi: 10.1002/jpln.200520574
Yi, H., and Meng, Z. (2003). Genotoxicity of hydrated sulfur dioxide on root tips of Allium sativum and Vicia faba. Mutat. Res. 537, 109–114. doi: 10.1016/s1383-5718(03)00054-8
Yoshimoto, N., Inoue, E., Saito, K., Yamaya, T., and Takahashi, H. (2003). Phloem-localizing sulfate transporter, Sultr1;3, mediates re-distribution of sulfur from source to sink organs in Arabidopsis. Plant Physiol. 131, 1511–1517. doi: 10.1104/pp.014712
Zhang, J., Zhou, M. J., Zhou, H., Zhao, D. D., Gotor, C., et al. (2021). Hydrogen sulfide, a signaling molecule in plant stress responses. J. Integr. Plant Biol. 2021, 146–160. doi: 10.1111/jipb.13022
Keywords: abscisic acid, guard cell, hydrogen sulfide, stomatal movement, sulfur compounds, sulfur dioxide
Citation: Ren Z, Wang R-Y, Huang X-Y and Wang Y (2022) Sulfur Compounds in Regulation of Stomatal Movement. Front. Plant Sci. 13:846518. doi: 10.3389/fpls.2022.846518
Received: 31 December 2021; Accepted: 09 February 2022;
Published: 11 March 2022.
Edited by:
Yizhou Wang, Zhejiang University, ChinaReviewed by:
Yanjie Xie, Nanjing Agricultural University, ChinaBo Xu, University of Adelaide, Australia
Copyright © 2022 Ren, Wang, Huang and Wang. This is an open-access article distributed under the terms of the Creative Commons Attribution License (CC BY). The use, distribution or reproduction in other forums is permitted, provided the original author(s) and the copyright owner(s) are credited and that the original publication in this journal is cited, in accordance with accepted academic practice. No use, distribution or reproduction is permitted which does not comply with these terms.
*Correspondence: Xin-Yuan Huang, eGlueXVhbi5odWFuZ0BuamF1LmVkdS5jbg==; Yin Wang, d2FuZ3lpbnBrdUBwa3UuZWR1LmNu
†These authors have contributed equally to this work