- 1Yunnan Key Laboratory of Plant Reproductive Adaptation and Evolutionary Ecology, Institute of Biodiversity, School of Ecology and Environmental Science, Yunnan University, Kunming, China
- 2Laboratory of Ecology and Evolutionary Biology, State Key Laboratory for Conservation and Utilization of Bio-Resources in Yunnan, Yunnan University, Kunming, China
Most plants in nature establish symbiotic associations with endophytic fungi in soil. Beneficial endophytic fungi induce a systemic response in the aboveground parts of the host plant, thus promoting the growth and fitness of host plants. Meanwhile, temperature elevation from climate change widely affects global plant biodiversity as well as crop quality and yield. Over the past decades, great progresses have been made in the response of plants to high ambient temperature and to symbiosis with endophytic fungi. However, little is known about their synergistic effect on host plants. The endophytic fungus Serendipita indica colonizes the roots of a wide range of plants, including Arabidopsis. Based on the Arabidopsis-S. indica symbiosis experimental system, we analyzed the synergistic effect of high ambient temperature and endophytic fungal symbiosis on host plants. By transcriptome analysis, we found that DNA replication-related genes were significantly upregulated during the systemic response of Arabidopsis aboveground parts to S. indica colonization. Plant hormones, such as jasmonic acid (JA) and ethylene (ET), play important roles in plant growth and systemic responses. We found that high ambient temperature repressed the JA and ET signaling pathways of Arabidopsis aboveground parts during the systemic response to S. indica colonization in roots. Meanwhile, PIF4 is the central hub transcription factor controlling plant thermosensory growth under high ambient temperature in Arabidopsis. PIF4 is also involving JA and/or ET signaling pathway. We found that PIF4 target genes overlapped with many differentially expressed genes (DEGs) during the systemic response, and further showed that the growth promotion efficiency of S. indica on the pif4 mutant was higher than that on the wild-type plants. In short, our data showed that high ambient temperature strengthened the growth promotion effect of S. indica fungi on the aboveground parts of the host plant Arabidopsis, and the growth promotion effect of the systemic response under high ambient temperature was regulated by PIF4.
Introduction
Beneficial endophytic fungi in soil establish symbiotic interactions with most plants in natural ecosystems and induced a systemic response in the aboveground parts (stems, leaves, etc.) of host plants (Pieterse et al., 2014; Weiss et al., 2016). Beneficial endophytic fungi can improve host plants’ resistance to biotic and abiotic stresses, and promote the growth and fitness of host plants (Cameron et al., 2013; Pieterse et al., 2014; van der Heijden et al., 2015). Serendipita indica (syn. Piriformospora indica) is a Sebacinales fungus first isolated from the rhizosphere in deserts and symbiotically colonizes the roots of many plants, including Brassicaceae plants (such as the model plant Arabidopsis thaliana), which are known as non-host plants for ectomycorrhizae and arbuscular mycorrhizae (Verma et al., 1998; Peskan-Berghofer et al., 2004; Zuccaro et al., 2011; Qiang et al., 2012; Weiss et al., 2016). S. indica colonization in roots has a significant growth promotion effect on the biomass of the aboveground parts of host plants, including Arabidopsis (Peskan-Berghofer et al., 2004; Waller et al., 2005; Shahollari et al., 2007; Abdelaziz et al., 2019; Li et al., 2021). Previous studies in root endophytism and/or mycorrhizal association have mainly focused on the symbiosis signaling pathways that are necessary for the establishment of successful symbiotic relationships in plant roots (Lahrmann et al., 2015; Zipfel and Oldroyd, 2017; Rey and Jacquet, 2018), while the systemic responses of the aboveground parts of host plants are largely unknown.
For symbiotic interactions with Arabidopsis, S. indica endophytically colonizes root epidermal and cortex cells mainly in the meristematic zone and does not penetrate the central cylinder (Jacobs et al., 2011). The growth promotion effect of S. indica is due to the regulation of nutrients (phosphate, nitrate, etc.) uptake (Sherameti et al., 2005; Yadav et al., 2010; Bakshi et al., 2017; Prasad et al., 2019) and hormone [auxin, jasmonic acid (JA), salicylic acid (SA), etc.] homeostasis or signal transduction in host plants (Vadassery et al., 2008; Camehl et al., 2010; Jacobs et al., 2011; Hilbert et al., 2012; Lahrmann et al., 2015; Peskan-Berghofer et al., 2015). S. indica also enhances the resistance of host plants to biotic stress (Waller et al., 2005; Sun et al., 2014: Ye et al., 2019; Li et al., 2021) and abiotic stress conditions, such as drought (Sherameti et al., 2008), salt stress (Jogawat et al., 2013, 2016; Abdelaziz et al., 2017, 2019; Lanza et al., 2019; Luo et al., 2021) and cold stress (Jiang et al., 2020, 2021). Thus, Arabidopsis-S. indica symbiosis represents a model system in studying host plant–beneficial microbe interactions at the molecular level (Peskan-Berghofer et al., 2004). In the context of global warming, temperature elevation has become a major environmental factor affecting plant growth and development. However, it is unclear how high ambient temperature affects plant growth and development in the context of fungal symbiosis.
Exposure to high ambient temperature results in plant development changes termed thermomorphogenesis, which is characterized by hypocotyl elongation and petiole elongation in Arabidopsis (Koini et al., 2009; Quint et al., 2016). In Arabidopsis, high ambient temperature (greater than 22°C, approximately 28°C) is perceived by several thermosensors (Chen et al., 2021; Zhang et al., 2021). In response to high ambient temperature, phytochrome B (phyB) and/or EARLY FLOWERING3 (ELF3), which are major thermosensors in Arabidopsis, relieved their inhibitory effects on the temperature signal transduction component PHYTOCHROME INTERCTING FACTOR 4 (PIF4) (Jung et al., 2016, 2020; Legris et al., 2016; Zhang et al., 2021). PIF4 is the central hub transcription factor controlling thermosensory growth and development in Arabidopsis (Zhang et al., 2021). When Arabidopsis plants shift to high ambient temperature, PIF4 binds to the promoter regions of downstream thermoresponsive target genes and upregulates their expression. These genes are mainly involved in cell elongation, as well as plant hormone biosynthesis and response (Sun et al., 2012; Nieto et al., 2015; Gangappa and Kumar, 2017; Zhang et al., 2021). Meanwhile, PIF4 negatively regulates plant immunity and coordinates thermosensory growth and immunity (Gangappa et al., 2017). PIF4 also regulates JA and ethylene (ET) signaling pathways (Yamashino et al., 2013; Zhang et al., 2018; Xiang et al., 2020). Plant hormones (JA, ET, etc.) play an important role in plant systemic responses (Pieterse et al., 2014), but it is unclear whether PIF4 is involved.
In this study, we performed the cocultivation of S. indica with Arabidopsis under different temperatures (22, 25, and 28°C) and found that high ambient temperature enhanced the growth promotion effect of S. indica on Arabidopsis aboveground parts. By transcriptome analysis of the systemic response of the host plant Arabidopsis, we comprehensively analyzed the differentially expressed genes (DEGs) between fungal symbiotic and non-symbiotic plants at normal (22°C) and high ambient temperatures (25 and 28°C). We found that DNA replication-related genes were significantly upregulated during the systemic response of Arabidopsis aboveground parts to S. indica colonization. High ambient temperatures decreased the number of upregulated DEGs involving the JA and/or ET pathways, which implied repression of the JA and/or ET signaling pathways during the systemic response. Meanwhile, we also found that many DEGs overlapped with PIF4 target genes, and the growth promotion efficiency of S. indica on the pif4 mutant was higher than that on wild-type (WT) plants at warm temperatures (28°C).
Materials and Methods
Co-cultivation of Arabidopsis Plant and Serendipita indica Fungus
Wild-type plant is A. thaliana Col-0 ecotype, and Arabidopsis pif4 loss-of-function mutant is pif4-101 (Wang et al., 2018). Arabidopsis was grown on half-strength MS medium with 1.2% sucrose (1/2 MS). The seeds were stratified at 4°C for 3 days and then first grown at 22°C under short-day growth condition (12:12 h, day:night photoperiod, 7000 LX light) in growth chambers. Serendipita indica (syn. P. indica, DSM11827) fungus were grown and conserved in PDA medium (200 g L–1 potato, 20 g L–1 dextrose, and 20 g L–1 agar). For cocultivation with S. indica, 9-day-old Arabidopsis seedlings were inoculated with S. indica in PNM medium plates (Johnson et al., 2011). S. indica-incubated (Si) and control (CK) samples were grown at 22, 25, or 28°C under short-day growth condition. The statistics of fresh weight were performed on 7 and 14 days post inoculation (dpi). Fresh weights of each seedling were the average weight of at least ten seedlings, which grew in the same plate. The mean and standard error statistics of fresh weights included at least three biological replicates for each sample. Significance analysis of differences were performed by t-test.
Transcriptome and Reverse Transcription-Quantitative PCR Analysis
The aboveground parts of S. indica-inoculated Arabidopsis at 7 dpi were harvested for transcriptome and reverse transcription-quantitative PCR (RT-qPCR) analysis. Total RNA extracted from Si and CK samples grown at 22, 25, or 28°C was used for sequencing with the NovaSeq 6000 sequencer by Majorbio (Shanghai, China). The clean reads were mapped to the A. thaliana TAIR10 database. Bioinformatics analyses of the transcriptome were performed using the online platform of Majorbio Cloud Platform1 and jvenn Venn diagram viewer (Bardou et al., 2014). Briefly, to identify DEGs between different samples, the expression level of each transcript was calculated according to the transcripts per million reads (TPM) method. RSEM was used to quantify gene abundances. Differential expression analysis was performed using the DESeq2 with Q-value ≤ 0.05, DEGs with | log2FC| > 1 and Q-value ≤ 0.05 were considered to be significantly differential expression genes (DEGs). Clusters of orthologous groups (COG) functional annotation of DEGs was obtained from eggNOG database. Gene Ontology (GO) functional enrichment analysis of DEGs were carried out by Goatools. For RT-qPCR, the total RNA of Si or CK samples were extracted with TRNzol Universal Reagent (DP424, TIANGEN, China). First-stand cDNA synthesis and qPCR were performed by using One-Step gDNA Removal and cDNA Synthesis SuperMix kit (AT311, TransGen, China) and SuperReal PreMix Plus (SYBR Green) kit (FP205, TIANGEN, China), respectively. The expression of AtUBQ5 was used as an internal control (Nizam et al., 2019). All the primers are listed in Supplementary Table 4.
Results
The Growth Promotion Effect of Serendipita indica on Arabidopsis Are Enhanced by High Ambient Temperature
Serendipita indica colonization in roots has a significant growth promotion effect on Arabidopsis aboveground parts, but it is not known whether it is affected by high ambient temperature. We analyzed the growth promotion effect of S. indica on Arabidopsis plants at normal temperature (22°C) and high ambient temperatures (25 and 28°C). The fresh weights of aboveground parts of Arabidopsis inoculated with S. indica (Si) were significantly higher than that of control (CK) at different temperatures (Figure 1). Meanwhile, the fresh weight of the symbiotic plants grown under high ambient temperatures (25 and 28°C) were significantly higher than that under normal growth temperature (22°C) at 7 or 14 dpi (Figure 1). High ambient temperatures have significantly increased the fresh weight of the aboveground parts of Arabidopsis at 7 dpi (Figure 1A). Meanwhile, the fungal colonization rates at 7 dpi have no remarkable differences under different temperatures (Supplementary Figure 1A). In short, these results indicated that high ambient temperature enhanced the growth promotion effect of S. indica fungi on the aboveground parts of the host plant Arabidopsis.
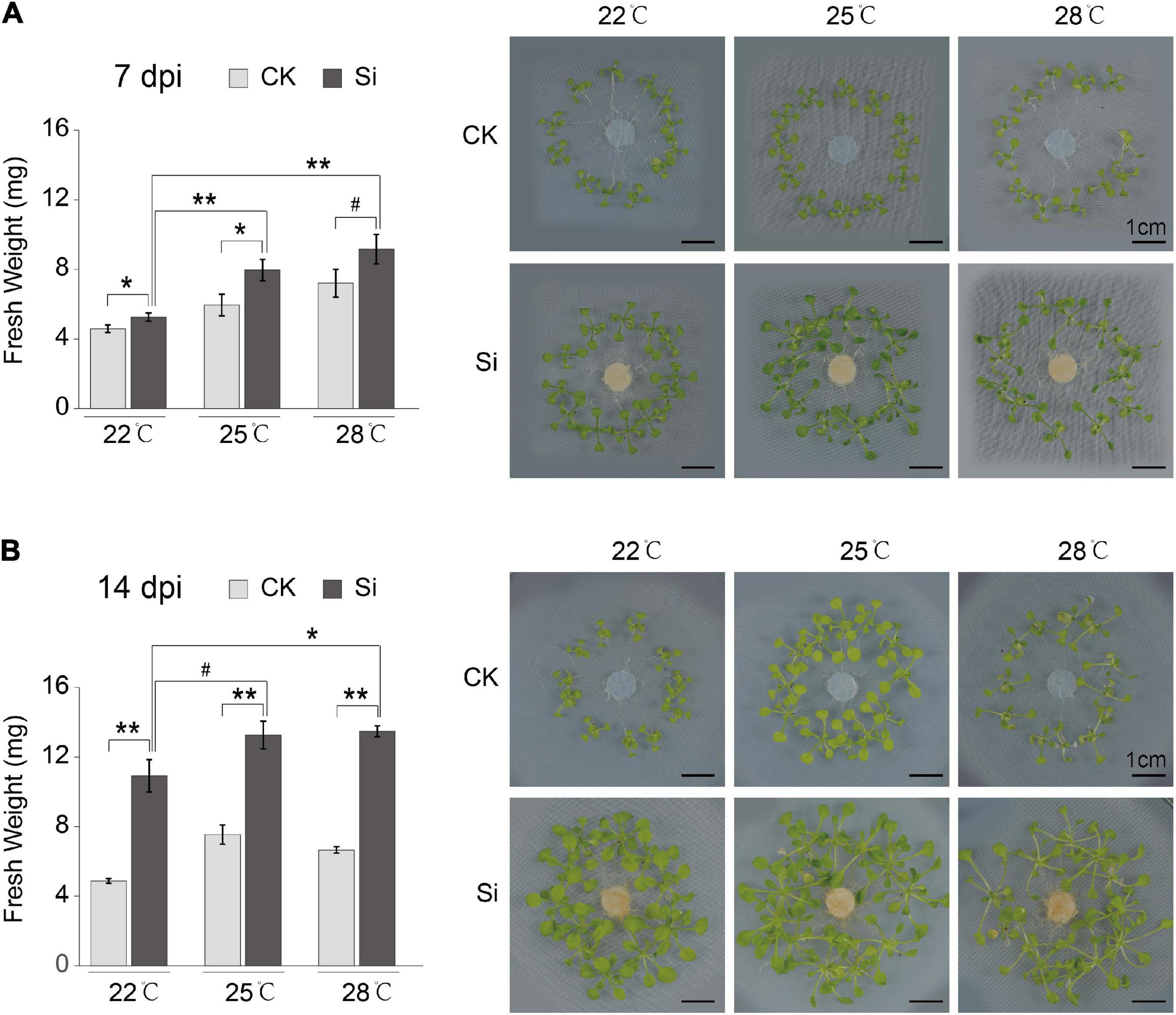
Figure 1. High ambient temperature strengthened the growth promotion effect of S. indica on Arabidopsis. Arabidopsis thaliana col-0 WT plants were cocultured with the endophytic fungus S. indica at different temperatures. S. indica significantly promoted the growth of Arabidopsis seedlings at 22, 25, and 28°C. The photos showed the control (CK) and S. indica cocultivated (Si) seedlings at the 7 days post inoculation (dpi) (A) and 14 dpi (B). Fresh weights of seedling aboveground parts at 25 and 28°C were heavier than that at 22°C, which suggested that the growth promotion effect of S. indica was enhanced by elevated ambient temperature. Error bars indicate SE (n ≥ 3). Significance analysis of differences between CK and Si samples were performed by t-test (**P < 0.01; *P < 0.05; #P < 0.1).
Systemic Response of Arabidopsis to Serendipita indica Colonization Under High Ambient Temperature
Serendipita indica colonization in Arabidopsis roots has several typical phases: the early biotrophic phase at about 3 dpi, the late biotrophic phase at about 7 dpi and the late saprotrophic phase at about 14 dpi (Zuccaro et al., 2011; Lahrmann et al., 2013, 2015). The intracellular colonization of S. indica were well established at 7 dpi (Lahrmann et al., 2015). To understand the mechanism underlying the S. indica-induced plant growth-promoting effect, we attempt to compare transcriptional changes in the aboveground parts of the host plant Arabidopsis. In our experimental system, S. indica also well colonized Arabidopsis roots and had significant growth promoting effects at 7 dpi (Figure 1A and Supplementary Figure 1A). Thus, we harvested the aboveground parts of Arabidopsis seedlings at 7 dpi under different temperatures for subsequent transcriptome analysis (Figure 2A). We compared the DEGs between S. indica incubated- and sterile cultured-samples at 22, 25, and 28°C separately, and found that a large part of the DEGs (that is, 981) were commonly regulated at different temperatures (Figures 2A,B). Under each temperature condition, the number of upregulated DEGs (1,601 approximately 72% at 22°C, 1,997 approximately 65.8% at 25°C, and 1,774 approximately 71% at 28°C) was greater than that of downregulated DEGs (624 approximately 28% at 22°C, 1,038 approximately 34.2% at 25°C, and 723 approximately 29% at 28°C) (Figure 2C and Supplementary Table 1). Then, we analyzed the upregulated and downregulated DEGs. GO analysis of upregulated DEGs showed that GO terms of “DNA replication origin binding,” “pre-replicative complex assembly involved in cell cycle DNA replication,” and “DNA replication initiation” were enriched (Figure 2D and Supplementary Figure 2), and upregulated DEGs in these DNA replication-related GO terms were closely related to promoting the cell cycle and growth. For the downregulated DEGs, photosynthesis-related GO terms, such as “photosynthesis, light harvesting in photosystem I,” etc. (Supplementary Figure 2), were enriched. Meanwhile, COG analysis also showed that there were many more upregulated DEGs than downregulated DEGs in the COG classifications of “Cell cycle control, cell division, chromosome partitioning” and “Replication, recombination and repair” (Figure 3 and Supplementary Table 2). In the COG classification of “Replication, recombination and repair,” the number of upregulated DEGs at high ambient temperature (44 at 25°C and 36 at 28°C) was greater than that at normal temperature (27 at 22°C) (Supplementary Table 2). Meanwhile, RT-qPCR analysis further confirmed that, compared with S. indica colonized seedlings grown under normal temperature (22°C), the gene expression of key DNA replication proteins, including MCM2, MCM4, and ORC2 (Collinge et al., 2004; Diaz-Trivino et al., 2005; Shultz et al., 2009), was more increased at high ambient temperature (28°C) (Figure 2E). Collectively, these data suggested that DNA replication-related pathways in the aboveground parts of host plants are activated by S. indica colonization, especially under high ambient temperature. These data supported the results in Figure 1 that high ambient temperature enhanced the growth-promoting effect of S. indica on the aboveground parts of Arabidopsis.
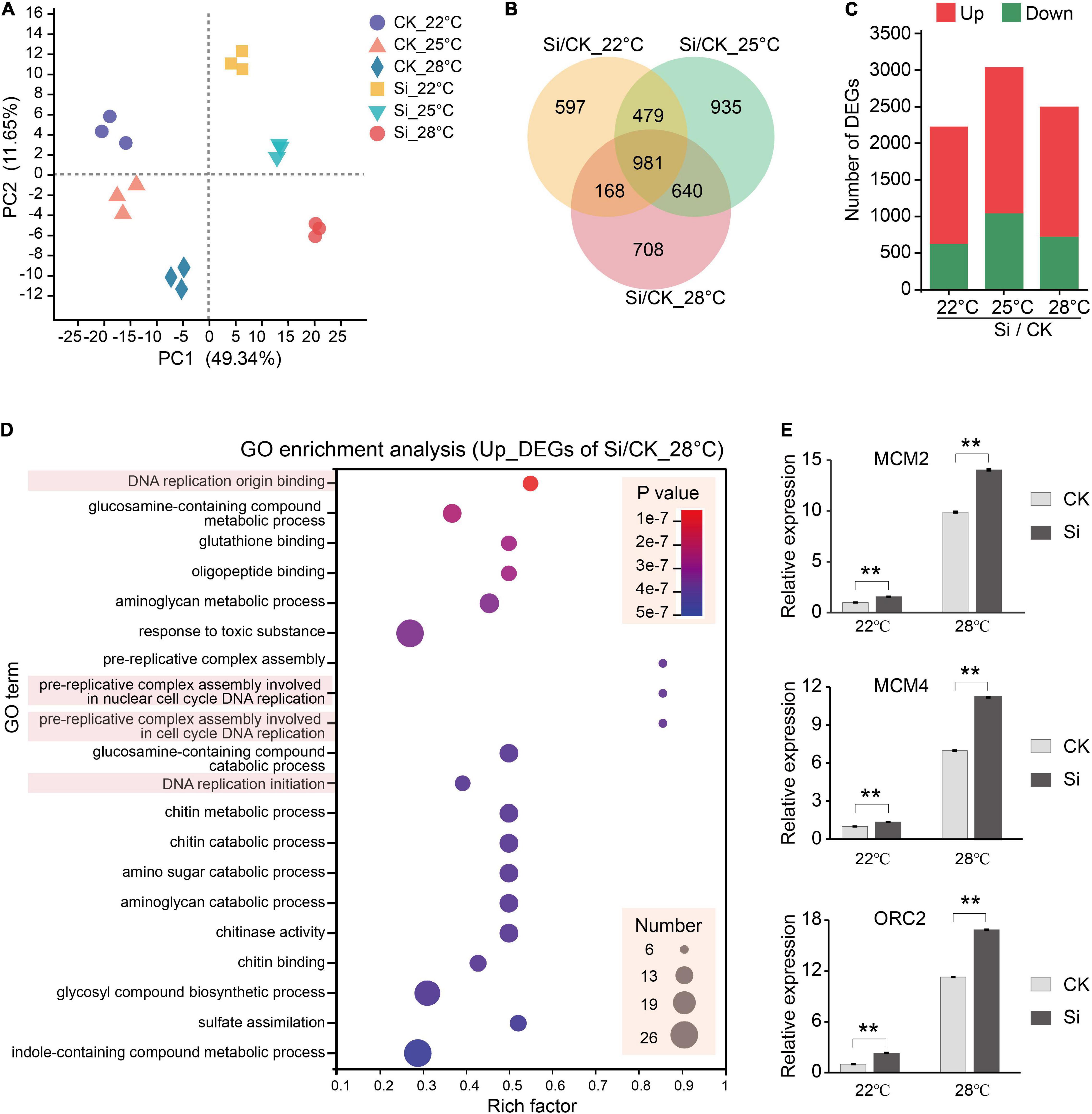
Figure 2. Transcriptome analysis of the symbiotic response of Arabidopsis at different temperatures. Arabidopsis seedlings incubated with S. indica were grown for 7 days at 22, 25, and 28°C, and the seedling aerial parts were harvested for subsequent RNA-seq and RT-qPCR analysis. (A) Principle component analysis (PCA) of Arabidopsis transcriptome samples. (B) Venn diagrams showing the number of common and specific differentially expressed genes (DEGs) between S. indica-incubated (Si) and control (CK) samples at 22, 25, and 28°C. (C) The number of DEGs upregulated or downregulated during S. indica colonization at different temperatures. (D) Gene Ontology (GO) enrichment analysis of the upregulated DEGs at 28°C, which showed that DNA replication-related genes were highly enriched. GO enrichment analysis of other DEGs is shown in Supplementary Figure 2. (E) The expression of the DNA replication-related genes MCM2, MCM4, and ORC2 was detected by RT-qPCR. Error bars indicate SE (n = 4). Significance analysis of differences were performed by t-test (**P < 0.01).
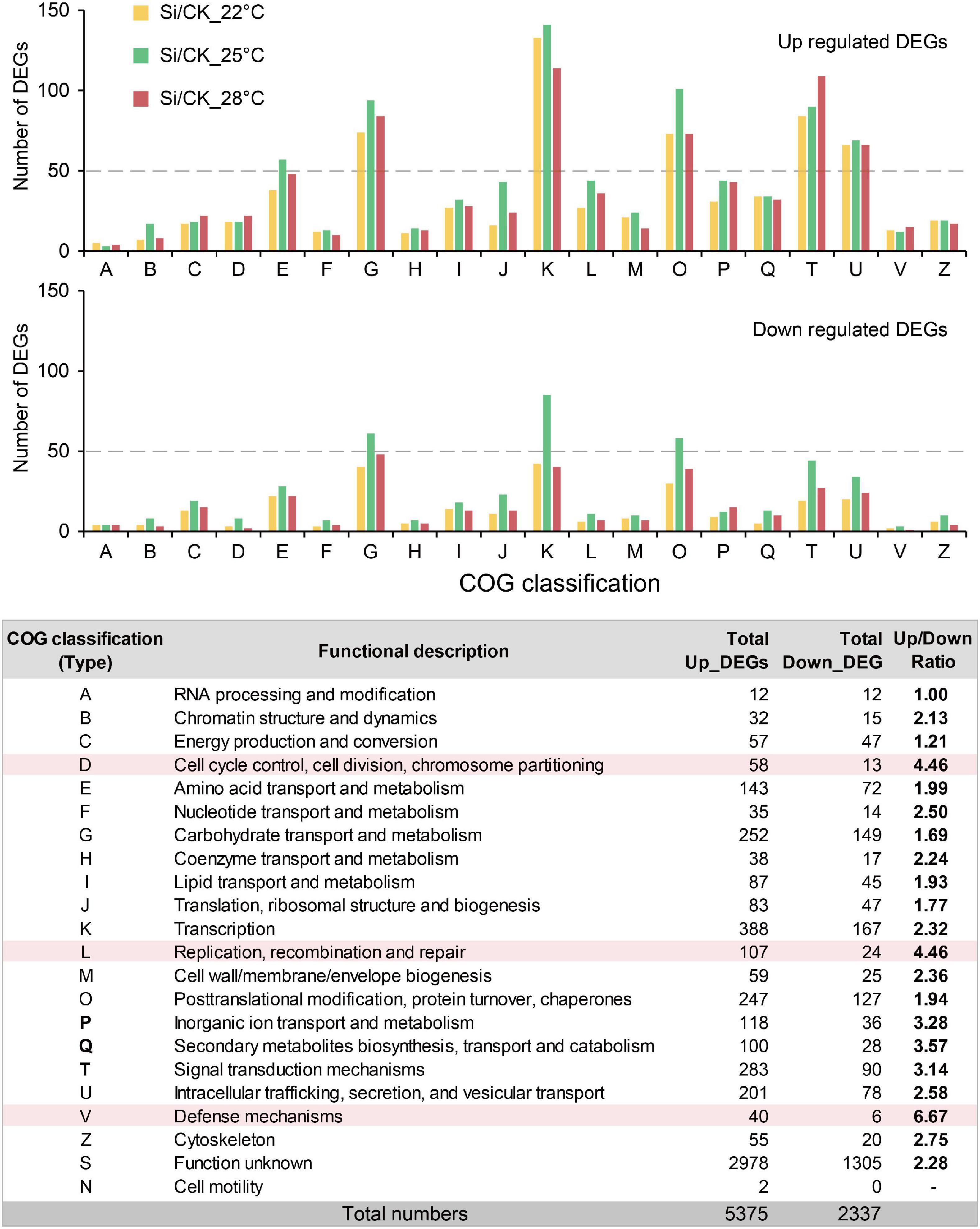
Figure 3. Clusters of orthologous groups (COG) analysis of DEGs during S. indica colonization at different temperatures. COG of proteins analysis showed that there were more upregulated DEGs than downregulated DEGs in nearly each COG classification. The ratio of upregulated DEGs to downregulated DEGs was highest in the D, L, and V types, which were related to the cell cycle and division, DNA replication and defense response. The number of DEGs in the COG classification at each temperature is shown in Supplementary Table 2.
High Ambient Temperatures Repressed the Jasmonic Acid and Ethylene Signaling Pathways During the Plant Systemic Response
In addition to regulating plant growth, high ambient temperature also strongly suppresses defense responses (Alcazar and Parker, 2011). Plant hormones, such as SA, JA, and ET, play important roles in the induced systemic defense responses of plants (Pieterse et al., 2014). JA and ET also involved in the Arabidopsis-S. indica interaction (Stein et al., 2008; Camehl et al., 2010; Lahrmann et al., 2015). Here, our transcriptome analysis showed that DEGs involved in the JA and ET pathways were all upregulated DEGs. High ambient temperatures greatly decreased the number of upregulated DEGs in the JA and/or ET signaling pathways but not in SA-related GO terms (Figure 4). These results suggested that the Arabidopsis JA and/or ET signaling pathways were activated and involved in the S. indica-induced systemic response, and were repressed by high ambient temperature.
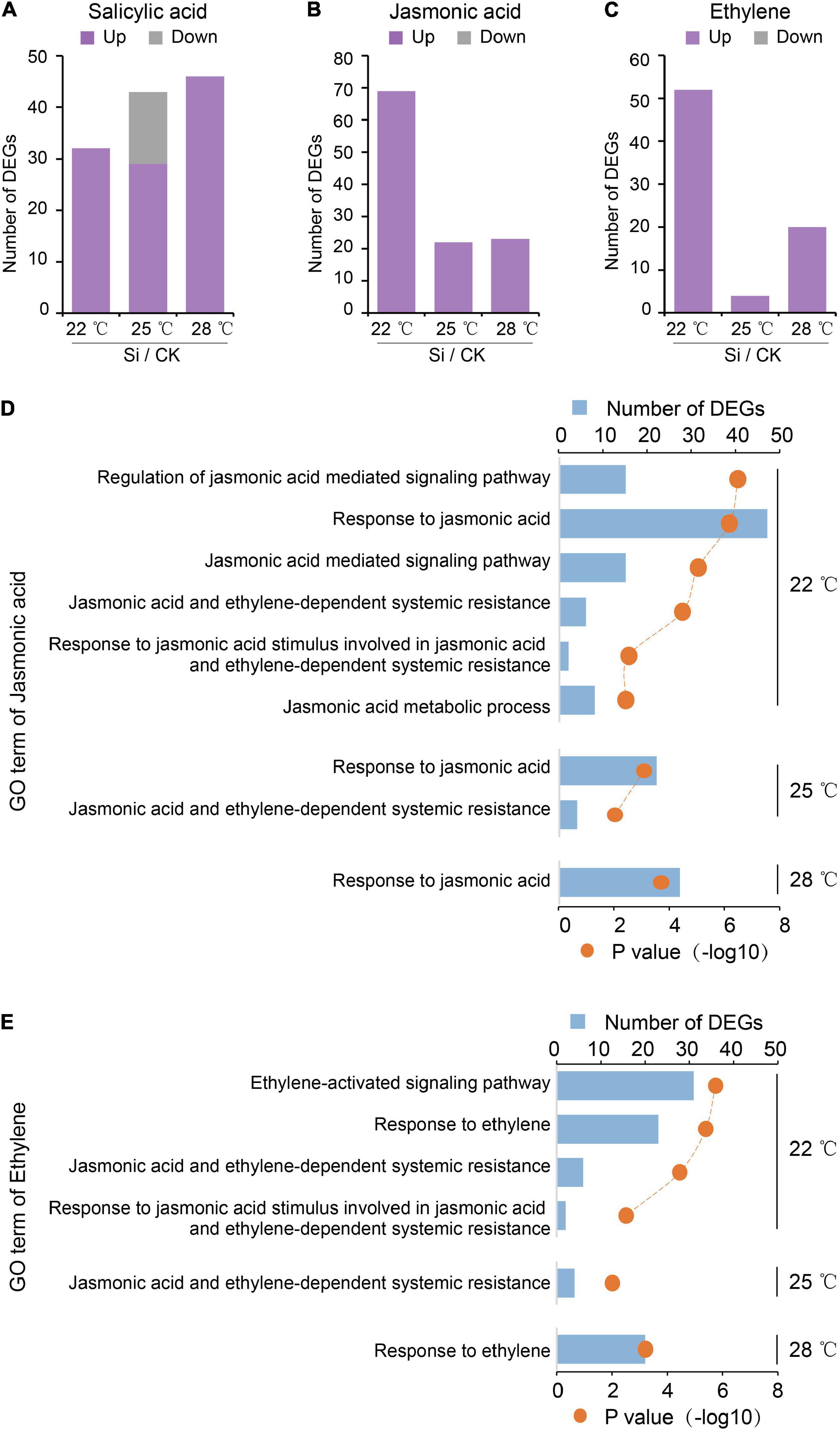
Figure 4. High ambient temperature repressed plant JA and ET pathways during the systemic response. High temperature affected the number of DEGs between S. indica-incubated (Si) and control (CK) samples. (A) The number of DEGs of the salicylic acid (SA) pathway in Arabidopsis aboveground parts. (B,C) High ambient temperatures decreased the number of DEGs in the jasmonic acid (JA) and ethylene (ET) pathways. These DEGs were all upregulated DEGs. These results suggested that Arabidopsis JA and ET pathways induced by S. indica colonization are downregulated by elevated temperature. (D,E) GO analysis to the DEGs of JA and ET pathways.
pif4 Mutant Enhanced the Growth Promotion Efficiency of Serendipita indica on Arabidopsis at High Ambient Temperatures
Arabidopsis JA and/or ET signaling pathways are also regulated by PIF4 (Yamashino et al., 2013; Zhang et al., 2018; Xiang et al., 2020). More importantly, PIF4 is the central hub transcription factor that regulates the aboveground tissue response to high ambient temperature in Arabidopsis (Lee et al., 2021; Zhang et al., 2021). Therefore, we wondered whether PIF4 is involved in the systemic response of Arabidopsis aboveground tissue to S. indica colonization under high ambient temperatures. Previous studies have reported 4,362 PIF4-binding target genes (Oh et al., 2012), so we first compared PIF4 targets with those DEGs during S. indica colonization. The Venn diagram shows significant overlap between PIF4-binding target genes and DEGs at high ambient temperature (28°C) (Figure 5A and Supplementary Table 3). Then, we incubated S. indica with a loss-of-function pif4 mutant (pif4-101) at 28°C and analyzed the fresh weight of their aboveground tissues. Interestingly, the growth promotion effect of S. indica on the pif4 mutant was significantly stronger than that on WT plants at 14 dpi under 28°C (Figures 5B–D). Meanwhile, the fungal colonization rate between pif4 mutant and WT plants have no significant difference at 14 dpi under 28°C (Supplementary Figure 1B). These data suggest that PIF4 was involved in the response of Arabidopsis aboveground tissues to high ambient temperature during beneficial endophytic fungal colonization.
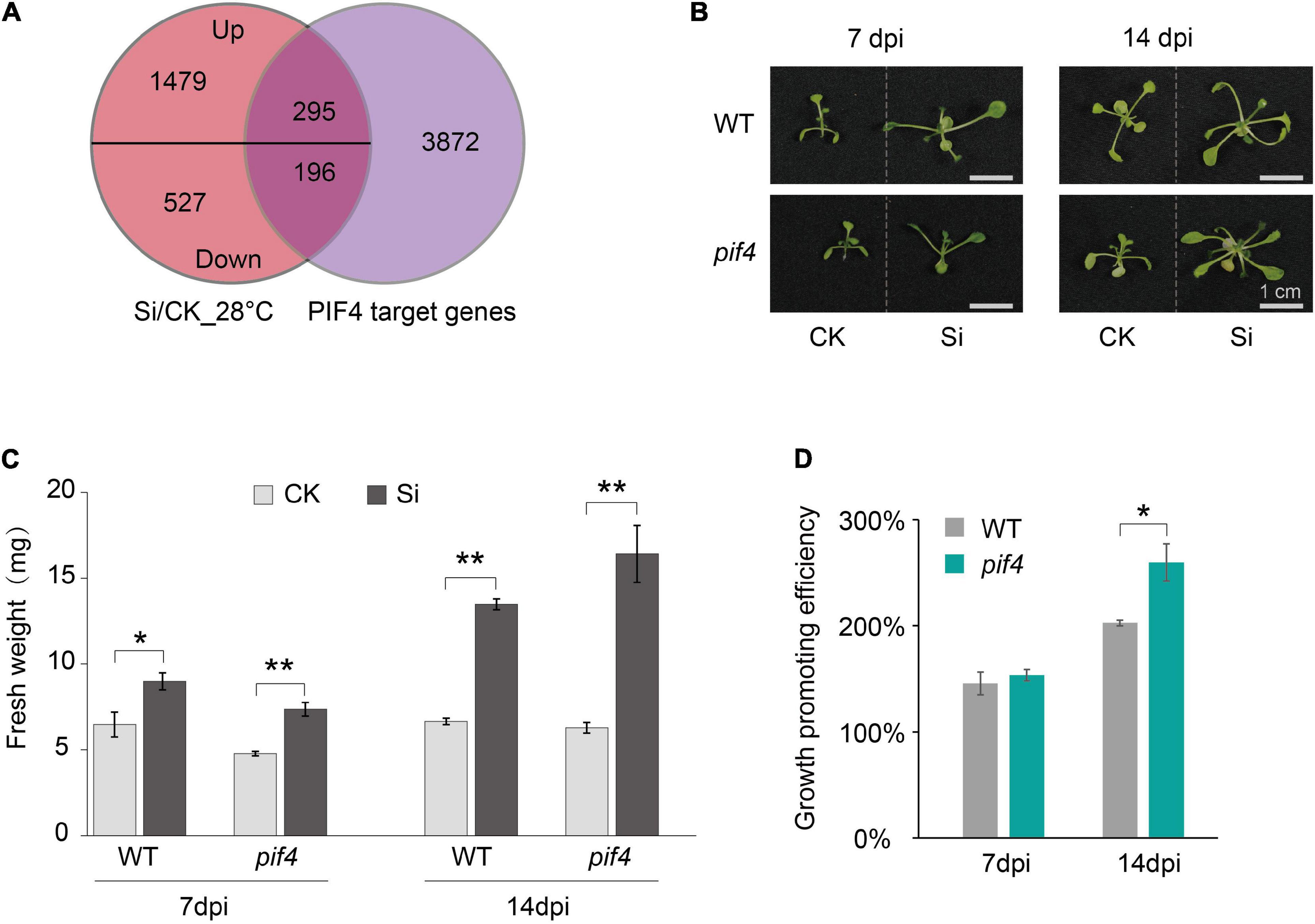
Figure 5. PIF4 regulated the growth promotion efficiency of S. indica in Arabidopsis under high ambient temperature. (A) Venn diagram showing that PIF4 target genes overlapped with many upregulated or downregulated DEGs during the systemic response of Arabidopsis aboveground parts to S. indica at high ambient temperature (28°C). (B) S. indica-incubated seedlings (Si) at 7 or 14 dpi and their control (CK) seedlings. (C) The fresh weight of wild-type (WT) and pif4 plants at 7 and 14 dpi when cocultured with S. indica at 28°C. (D) The growth promotion efficiency of S. indica on pif4 (pif4-101, a pif4 loss-of-function mutant) plants was higher than that on WT plants at 28°C. Growth promoting efficiency was obtained by dividing the fresh weight of Si samples by that of CK samples. Error bars indicate SE (n ≥ 3). Significance analysis of differences were performed by t-test (**P < 0.01; *P < 0.05).
Discussion
Soil fungi in nature establish beneficial symbiotic relationships with most vascular plants (Brundrett and Tedersoo, 2018; Genre et al., 2020). Beneficial endophytic fungi in roots promote the induced systemic resistance of plant aboveground parts to pathogenic microorganisms, herbivorous insects and abiotic stresses and promote the growth of plant aboveground parts (Franken, 2012; Cameron et al., 2013; Pieterse et al., 2014). Over the past decades, significant progress has been achieved in understanding the molecular mechanism of induced systemic resistance in plants, especially the systemic response induced by pathogenic microorganisms (Jung et al., 2012; Walters et al., 2013; Pieterse et al., 2014; Hilleary and Gilroy, 2018). However, the mechanisms by which it promotes plant growth are not well studied. S. indica colonization in roots has a significant growth promotion effect on many host plants (Qiang et al., 2012). Here, we examine the transcriptome response of aboveground parts of Arabidopsis plants to S. indica colonization under normal or high ambient temperatures. Compared with sterile seedlings, DEGs related to DNA replication were significantly enriched and upregulated in plants cocultured with S. indica (Figures 2, 3). These data are consistent with the result that higher ambient temperatures can enhance the growth-promoting effect of S. indica on the aboveground parts of Arabidopsis (Figure 1). By the way, photosynthesis-related GO terms were enriched in the downregulated DEGs (Supplementary Figure 2). Photosynthetic efficiency is vital for promoting plant biomass, and S. indica symbiosis promotes the photosynthesis of host plants (Li et al., 2021). Thus, the downregulation of photosynthesis-related genes may be feedback regulation from the increased photosynthesis efficiency of host plants.
Plant hormones (SA, JA, ET, etc.) play major roles in plant systemic responses, including defense and growth-promoting effects (Pieterse et al., 2014; Xu et al., 2018; Dai et al., 2019). S. indica colonization increased JA accumulation and decreased SA levels in Arabidopsis roots (Lahrmann et al., 2015). The systemic resistance response induced by S. indica colonization was independent of SA signaling but required an operative JA defense pathway (Stein et al., 2008). Overexpression of ETHYLENE RESPONSE FACTOR1 (ERF1), which directly activates many ET-inducible defense genes, strongly reduces S. indica colonization in roots and abolishes growth promotion in the aboveground part of Arabidopsis (Camehl et al., 2010). Here, we found that the number of DEGs related to the JA and ET pathways under high ambient temperatures was significantly decreased compared to that under normal temperature (Figure 4), suggesting that JA and/or ET signaling pathways were repressed under high ambient temperature. Activation of JA and ET signals in leaves inhibited plant growth by inhibiting cell division (Huang et al., 2017; Dubois et al., 2018). Thus, the repression of JA and/or ET signaling pathways (Figure 4) and the upregulation of DNA replication related genes (Figure 2) should all contribute to the higher growth promotion effects under high ambient temperature. Furtherly, the growth promotion and defense inhibition are antagonistically linked in plants (Huot et al., 2014; Guo et al., 2018). High ambient temperature promotes growth, whereas it suppresses defense responses in plants (Alcazar and Parker, 2011). Thus, the increased growth promotion efficiency under high ambient temperatures (Figures 1, 4) may be associated with inhibition of JA and/or ET-related defense responses. The role of JA and ET pathways in plant growth-defense trade-offs deserves attention in the future study of plant systemic responses under high ambient temperatures. Meanwhile, carbon availability has been suggested to modulate the plant growth-defense trade-offs, in which the activation of JA signaling pathway depletes sucrose and starch content (Smith and Stitt, 2007; Machado et al., 2017; Guo et al., 2018). The inhibition of JA signaling pathway by high ambient temperature may inhibit carbon consumption for defense response, thus allow more carbon metabolites to be used for plant growth.
The Arabidopsis PIF4 transcription factor plays a central role in the response to high ambient temperature (Koini et al., 2009; Zhang et al., 2021). PIF4 is also involved in the regulation of the JA and/or ET signaling pathways (Yamashino et al., 2013; Zhang et al., 2018; Xiang et al., 2020). Loss-of-function mutations of pif4 inhibit Arabidopsis petiole elongation and other phenotypes during thermosensory growth (Koini et al., 2009; Zhang et al., 2021). However, it should be noted that seedlings overexpressing PIF4 grew very thin and had significantly lower biomass than WT plants, especially at high ambient temperature (Kumar et al., 2012). Here, we found that the pif4 mutant promoted the increase in aboveground biomass induced by S. indica colonization under high ambient temperature (Figure 5). DEGs that regulate the systemic response to S. indica colonization and the target gene of PIF4 exhibited considerable overlap (Figure 5). In short, PIF4 is involved in the Arabidopsis systemic response and regulates the growth promotion effect of S. indica on aboveground parts of plants under high ambient temperature. The detailed mechanism of PIF4 regulating the growth promotion effect of S. indica on plants needs to be further studied, which may be through JA and/or ET signaling pathway.
A recent transcriptome study showed that only a small portion of systemic response DEGs overlapped with local response DEGs during abiotic stress in plants (Zandalinas et al., 2020). COG analysis to transcriptome data showed that most DEGs (2,978 upregulated DEGs and 1,305 downregulated DEGs) under different temperatures belonged to Function unknown (S) classification (Figure 3). Future studies on these DEGs with unknown functions will reveal the detailed molecular mechanisms by which beneficial endophytic symbiosis regulates the systemic response of aboveground tissues under high and/or normal ambient temperature conditions.
Data Availability Statement
The data presented in the study are deposited in the Gene Expression Omnibus (GEO) under accession number GSE197325.
Author Contributions
JJH and XJC designed the experiments, analyzed the data, and wrote the manuscript. XJC, YQY, XMZ, and XX performed the experiments. All authors contributed to the article and approved the submitted version.
Funding
This project was financially supported by grants from the National Natural Science Foundation of China (31970287) and the Innovative Research Foundation for Graduate Students of Yunnan University (2020238 and 2021Y409).
Conflict of Interest
The authors declare that the research was conducted in the absence of any commercial or financial relationships that could be construed as a potential conflict of interest.
Publisher’s Note
All claims expressed in this article are solely those of the authors and do not necessarily represent those of their affiliated organizations, or those of the publisher, the editors and the reviewers. Any product that may be evaluated in this article, or claim that may be made by its manufacturer, is not guaranteed or endorsed by the publisher.
Supplementary Material
The Supplementary Material for this article can be found online at: https://www.frontiersin.org/articles/10.3389/fpls.2022.844572/full#supplementary-material
Footnotes
References
Abdelaziz, M. E., Abdelsattar, M., Abdeldaym, E. A., Atia, M. A. M., Mahmoud, A. W. M., Saad, M. M., et al. (2019). Piriformospora indica alters Na+/K+ homeostasis, antioxidant enzymes and LeNHX1 expression of greenhouse tomato grown under salt stress. Sci. Hortic. 256:108532. doi: 10.1016/j.scienta.2019.05.059
Abdelaziz, M. E., Kim, D., Ali, S., Fedoroff, N. V., and Al-Babili, S. (2017). The endophytic fungus Piriformospora indica enhances Arabidopsis thaliana growth and modulates Na(+)/K(+) homeostasis under salt stress conditions. Plant Sci. 263, 107–115. doi: 10.1016/j.plantsci.2017.07.006
Alcazar, R., and Parker, J. E. (2011). The impact of temperature on balancing immune responsiveness and growth in Arabidopsis. Trends Plant Sci. 16, 666–675. doi: 10.1016/j.tplants.2011.09.001
Bakshi, M., Sherameti, I., Meichsner, D., Thurich, J., Varma, A., Johri, A. K., et al. (2017). Piriformospora indica Reprograms Gene Expression in Arabidopsis Phosphate Metabolism Mutants But Does Not Compensate for Phosphate Limitation. Front. Microbiol. 8:1262. doi: 10.3389/fmicb.2017.01262
Bardou, P., Mariette, J., Escudie, F., Djemiel, C., and Klopp, C. (2014). jvenn: an interactive Venn diagram viewer. BMC Bioinform. 15:293. doi: 10.1186/1471-2105-15-293
Brundrett, M. C., and Tedersoo, L. (2018). Evolutionary history of mycorrhizal symbioses and global host plant diversity. New Phytol. 220, 1108–1115. doi: 10.1111/nph.14976
Camehl, I., Sherameti, I., Venus, Y., Bethke, G., Varma, A., Lee, J., et al. (2010). Ethylene signalling and ethylene-targeted transcription factors are required to balance beneficial and nonbeneficial traits in the symbiosis between the endophytic fungus Piriformospora indica and Arabidopsis thaliana. New Phytol. 185, 1062–1073. doi: 10.1111/j.1469-8137.2009.03149.x
Cameron, D. D., Neal, A. L., van Wees, S. C., and Ton, J. (2013). Mycorrhiza-induced resistance: more than the sum of its parts? Trends Plant Sci. 18, 539–545. doi: 10.1016/j.tplants.2013.06.004
Chen, Z., Galli, M., and Gallavotti, A. (2021). Mechanisms of temperature-regulated growth and thermotolerance in crop species. Curr. Opin. Plant Biol. 65:102134. doi: 10.1016/j.pbi.2021.102134
Collinge, M. A., Spillane, C., Kohler, C., Gheyselinck, J., and Grossniklaus, U. (2004). Genetic interaction of an origin recognition complex subunit and the Polycomb group gene MEDEA during seed development. Plant Cell. 16, 1035–1046. doi: 10.1105/tpc.019059
Dai, Y., Luo, X., Zhou, W., Chen, F., Shuai, H., Yang, W., et al. (2019). Plant systemic signaling under biotic and abiotic stresses conditions. Chin. Bull. Bot. 54, 255–264. doi: 10.11983/CBB18152
Diaz-Trivino, S., del Mar Castellano, M., de la Paz Sanchez, M., Ramirez-Parra, E., Desvoyes, B., and Gutierrez, C. (2005). The genes encoding Arabidopsis ORC subunits are E2F targets and the two ORC1 genes are differently expressed in proliferating and endoreplicating cells. Nucleic Acids Res. 33, 5404–5414. doi: 10.1093/nar/gki854
Dubois, M., Van den Broeck, L., and Inze, D. (2018). The Pivotal Role of Ethylene in Plant Growth. Trends Plant Sci. 23, 311–323. doi: 10.1016/j.tplants.2018.01.003
Franken, P. (2012). The plant strengthening root endophyte Piriformospora indica: potential application and the biology behind. Appl. Microbiol. Biotechnol. 96, 1455–1464. doi: 10.1007/s00253-012-4506-1
Gangappa, S. N., Berriri, S., and Kumar, S. V. (2017). PIF4 Coordinates Thermosensory Growth and Immunity in Arabidopsis. Curr. Biol. 27, 243–249. doi: 10.1016/j.cub.2016.11.012
Gangappa, S. N., and Kumar, S. V. (2017). DET1 and HY5 Control PIF4-Mediated Thermosensory Elongation Growth through Distinct Mechanisms. Cell Rep. 18, 344–351. doi: 10.1016/j.celrep.2016.12.046
Genre, A., Lanfranco, L., Perotto, S., and Bonfante, P. (2020). Unique and common traits in mycorrhizal symbioses. Nat. Rev. Microbiol. 18, 649–660. doi: 10.1038/s41579-020-0402-3
Guo, Q., Major, I. T., and Howe, G. A. (2018). Resolution of growth-defense conflict: mechanistic insights from jasmonate signaling. Curr. Opin. Plant Biol. 44, 72–81. doi: 10.1016/j.pbi.2018.02.009
Hilbert, M., Voll, L. M., Ding, Y., Hofmann, J., Sharma, M., and Zuccaro, A. (2012). Indole derivative production by the root endophyte Piriformospora indica is not required for growth promotion but for biotrophic colonization of barley roots. New Phytol. 196, 520–534. doi: 10.1111/j.1469-8137.2012.04275.x
Hilleary, R., and Gilroy, S. (2018). Systemic signaling in response to wounding and pathogens. Curr. Opin. Plant. Biol. 43, 57–62. doi: 10.1016/j.pbi.2017.12.009
Huang, H., Liu, B., Liu, L., and Song, S. (2017). Jasmonate action in plant growth and development. J. Exp. Bot. 68, 1349–1359. doi: 10.1093/jxb/erw495
Huot, B., Yao, J., Montgomery, B. L., and He, S. Y. (2014). Growth-defense tradeoffs in plants: a balancing act to optimize fitness. Mol. Plant. 7, 1267–1287. doi: 10.1093/mp/ssu049
Jacobs, S., Zechmann, B., Molitor, A., Trujillo, M., Petutschnig, E., Lipka, V., et al. (2011). Broad-spectrum suppression of innate immunity is required for colonization of Arabidopsis roots by the fungus Piriformospora indica. Plant Physiol. 156, 726–740. doi: 10.1104/pp.111.176446
Jiang, W., Pan, R., Buitrago, S., Wu, C., Abdelaziz, M. E., Oelmüller, R., et al. (2021). Transcriptome analysis of Arabidopsis reveals freezing-tolerance related genes induced by root endophytic fungus Piriformospora indica. Physiol. Mol. Biol. Plants. 27, 189–201. doi: 10.1007/s12298-020-00922-y
Jiang, W., Pan, R., Wu, C., Xu, L., Abdelaziz, M. E., Oelmuller, R., et al. (2020). Piriformospora indica enhances freezing tolerance and post-thaw recovery in Arabidopsis by stimulating the expression of CBF genes. Plant Signal. Behav. 15:1745472. doi: 10.1080/15592324.2020.1745472
Jogawat, A., Saha, S., Bakshi, M., Dayaman, V., Kumar, M., Dua, M., et al. (2013). Piriformospora indica rescues growth diminution of rice seedlings during high salt stress. Plant Signal Behav. 8:e26891. doi: 10.4161/psb.26891
Jogawat, A., Vadassery, J., Verma, N., Oelmuller, R., Dua, M., Nevo, E., et al. (2016). PiHOG1, a stress regulator MAP kinase from the root endophyte fungus Piriformospora indica, confers salinity stress tolerance in rice plants. Sci. Rep. 6:36765. doi: 10.1038/srep36765
Johnson, M., Sherameti, I., Ludwig, A., Nongbri, P., Sun, C., Lou, B., et al. (2011). Protocols for Arabidopsis thaliana and Piriformospora indica co-cultivation – a model system to study plant benefcial traits. Endocytobios. Cell Res. 21, 101–113.
Jung, J. H., Barbosa, A. D., Hutin, S., Kumita, J. R., Gao, M., Derwort, D., et al. (2020). A prion-like domain in ELF3 functions as a thermosensor in Arabidopsis. Nature 585, 256–260. doi: 10.1038/s41586-020-2644-7
Jung, J. H., Domijan, M., Klose, C., Biswas, S., Ezer, D., Gao, M., et al. (2016). Phytochromes function as thermosensors in Arabidopsis. Science 354, 886–889. doi: 10.1126/science.aaf6005
Jung, S. C., Martinez-Medina, A., Lopez-Raez, J. A., and Pozo, M. J. (2012). Mycorrhiza-induced resistance and priming of plant defenses. J. Chem. Ecol. 38, 651–664. doi: 10.1007/s10886-012-0134-6
Koini, M. A., Alvey, L., Allen, T., Tilley, C. A., Harberd, N. P., Whitelam, G. C., et al. (2009). High temperature-mediated adaptations in plant architecture require the bHLH transcription factor PIF4. Curr. Biol. 19, 408–413. doi: 10.1016/j.cub.2009.01.046
Kumar, S. V., Lucyshyn, D., Jaeger, K. E., Alos, E., Alvey, E., Harberd, N. P., et al. (2012). Transcription factor PIF4 controls the thermosensory activation of flowering. Nature 484, 242–245. doi: 10.1038/nature10928
Lahrmann, U., Strehmel, N., Langen, G., Frerigmann, H., Leson, L., Ding, Y., et al. (2015). Mutualistic root endophytism is not associated with the reduction of saprotrophic traits and requires a noncompromised plant innate immunity. New Phytol. 207, 841–857. doi: 10.1111/nph.13411
Lahrmann, U., Ding, Y., Banhara, A., Rath, M., Hajirezaei, M. R. Döhlemann, S., et al. (2013). Host-related metabolic cues affect colonization strategies of a root endophyte. Proc. Natl. Acad. Sci. U. S. A. 110, 13965–13970. doi: 10.1073/pnas.1301653110
Lanza, M., Haro, R., Conchillo, L. B., and Benito, B. (2019). The endophyte Serendipita indica reduces the sodium content of Arabidopsis plants exposed to salt stress: fungal ENA ATPases are expressed and regulated at high pH and during plant co-cultivation in salinity. Environ. Microbiol. 21, 3364–3378. doi: 10.1111/1462-2920.14619
Lee, S., Wang, W., and Huq, E. (2021). Spatial regulation of thermomorphogenesis by HY5 and PIF4 in Arabidopsis. Nat. Commun. 12:3656. doi: 10.1038/s41467-021-24018-7
Legris, M., Klose, C., Burgie, E. S., Rojas, C. C., Neme, M., Hiltbrunner, A., et al. (2016). Phytochrome B integrates light and temperature signals in Arabidopsis. Science 354, 897–900. doi: 10.1126/science.aaf5656
Li, Q., Kuo, Y. W., Lin, K. H., Huang, W., Deng, C., Yeh, K. W., et al. (2021). Piriformospora indica colonization increases the growth, development, and herbivory resistance of sweet potato (Ipomoea batatas L.). Plant Cell Rep. 40, 339–350. doi: 10.1007/s00299-020-02636-7
Luo, X., Dai, Y., Zheng, C., Yang, Y., Chen, W., Wang, Q., et al. (2021). The ABI4-RbohD/VTC2 regulatory module promotes reactive oxygen species (ROS) accumulation to decrease seed germination under salinity stress. New Phytol. 229, 950–962. doi: 10.1111/nph.16921
Machado, R. A. R., Baldwin, I. T., and Erb, M. (2017). Herbivory-induced jasmonates constrain plant sugar accumulation and growth by antagonizing gibberellin signaling and not by promoting secondary metabolite production. New Phytol. 215, 803–812. doi: 10.1111/nph.14597
Nieto, C., Lopez-Salmeron, V., Daviere, J. M., and Prat, S. (2015). ELF3-PIF4 interaction regulates plant growth independently of the Evening Complex. Curr. Biol. 25, 187–193. doi: 10.1016/j.cub.2014.10.070
Nizam, S., Qiang, X., Wawra, S., Nostadt, R., Getzke, F., Schwanke, F., et al. (2019). Serendipita indica E5’NT modulates extracellular nucleotide levels in the plant apoplast and affects fungal colonization. EMBO Rep. 20:e47430. doi: 10.15252/embr.201847430
Oh, E., Zhu, J. Y., and Wang, Z. Y. (2012). Interaction between BZR1 and PIF4 integrates brassinosteroid and environmental responses. Nat. Cell. Biol. 14, 802–809. ncb2545 doi: 10.1038/ncb2545
Peskan-Berghofer, T., Shahollari, B., Giong, P. H., Hehl, S., Markert, C., Blanke, V., et al. (2004). Association of Piriformospora indica with Arabidopsis thaliana roots represents a novel system to study beneficial plant-microbe interactions and involves early plant protein modifications in the endoplasmic reticulum and at the plasma membrane. Physiol. Plantar. 122, 465–477. doi: 10.1111/j.1399-3054.2004.00424.x
Peskan-Berghofer, T., Vilches-Barro, A., Muller, T. M., Glawischnig, E., Reichelt, M., Gershenzon, J., et al. (2015). Sustained exposure to abscisic acid enhances the colonization potential of the mutualist fungus Piriformospora indica on Arabidopsis thaliana roots. New Phytol. 208, 873–886. doi: 10.1111/nph.13504
Pieterse, C. M., Zamioudis, C., Berendsen, R. L., Weller, D. M., Van Wees, S. C., and Bakker, P. A. (2014). Induced systemic resistance by beneficial microbes. Annu. Rev. Phytopathol. 52, 347–375. doi: 10.1146/annurev-phyto-082712-102340
Prasad, D., Verma, N., Bakshi, M., Narayan, O. P., Singh, A. K., Dua, M., et al. (2019). Functional Characterization of a Magnesium Transporter of Root Endophytic Fungus Piriformospora indica. Front. Microbiol. 9:3231. doi: 10.3389/fmicb.2018.03231
Qiang, X., Weiss, M., Kogel, K. H., and Schäfer, P. (2012). Piriformospora indica-a mutualistic basidiomycete with an exceptionally large plant host range. Mol. Plant Pathol. 13, 508–518. doi: 10.1111/j.1364-3703.2011.00764.x
Quint, M., Delker, C., Franklin, K. A., Wigge, P. A., Halliday, K. J., and van Zanten, M. (2016). Molecular and genetic control of plant thermomorphogenesis. Nat. Plants. 2:15190. doi: 10.1038/nplants.2015.190
Rey, T., and Jacquet, C. (2018). Symbiosis genes for immunity and vice versa. Curr. Opin. Plant Biol. 44, 64–71. doi: 10.1016/j.pbi.2018.02.010
Shahollari, B., Vadassery, J., Varma, A., and Oelmuller, R. (2007). A leucine-rich repeat protein is required for growth promotion and enhanced seed production mediated by the endophytic fungus Piriformospora indica in Arabidopsis thaliana. Plant J. 50, 1–13. doi: 10.1111/j.1365-313X.2007.03028.x
Sherameti, I., Shahollari, B., Venus, Y., Altschmied, L., Varma, A., and Oelmuller, R. (2005). The endophytic fungus Piriformospora indica stimulates the expression of nitrate reductase and the starch-degrading enzyme glucan-water dikinase in tobacco and Arabidopsis roots through a homeodomain transcription factor that binds to a conserved motif in their promoters. J. Biol. Chem. 280, 26241–26247. doi: 10.1074/jbc.M500447200
Sherameti, I., Tripathi, S., Varma, A., and Oelmuller, R. (2008). The root-colonizing endophyte Pirifomospora indica confers drought tolerance in Arabidopsis by stimulating the expression of drought stress-related genes in leaves. Mol. Plant Microbe Interact. 21, 799–807. doi: 10.1094/MPMI-21-6-0799
Shultz, R. W., Lee, T. J., Allen, G. C., Thompson, W. F., and Hanley-Bowdoin, L. (2009). Dynamic localization of the DNA replication proteins MCM5 and MCM7 in plants. Plant Physiol. 150, 658–669. doi: 10.1104/pp.109.136614
Smith, A. M., and Stitt, M. (2007). Coordination of carbon supply and plant growth. Plant Cell Environ. 30, 1126–1149. doi: 10.1111/j.1365-3040.2007.01708.x
Stein, E., Molitor, A., Kogel, K. H., and Waller, F. (2008). Systemic resistance in Arabidopsis conferred by the mycorrhizal fungus Piriformospora indica requires jasmonic acid signaling and the cytoplasmic function of NPR1. Plant Cell Physiol. 49, 1747–1751. doi: 10.1093/pcp/pcn147
Sun, C., Shao, Y., Vahabi, K., Lu, J., Bhattacharya, S., Dong, S., et al. (2014). The beneficial fungus Piriformospora indica protects Arabidopsis from Verticillium dahliae infection by downregulation plant defense responses. BMC Plant Biol. 14:268. doi: 10.1186/s12870-014-0268-5
Sun, J., Qi, L., Li, Y., Chu, J., and Li, C. (2012). PIF4-mediated activation of YUCCA8 expression integrates temperature into the auxin pathway in regulating arabidopsis hypocotyl growth. PLoS Genet. 8:e1002594. doi: 10.1371/journal.pgen.1002594
Vadassery, J., Ritter, C., Venus, Y., Camehl, I., Varma, A., Shahollari, B., et al. (2008). The role of auxins and cytokinins in the mutualistic interaction between Arabidopsis and Piriformospora indica. Mol. Plant Microbe Interact. 21, 1371–1383. doi: 10.1094/MPMI-21-10-1371
van der Heijden, M. G., Martin, F. M., Selosse, M. A., and Sanders, I. R. (2015). Mycorrhizal ecology and evolution: the past, the present, and the future. New Phytol. 205, 1406–1423. doi: 10.1111/nph.13288
Verma, S., Varma, A., Rexer, K.-H., Hassel, A., Kost, G., Sarbhoy, A., et al. (1998). Piriformospora indica, gen. et sp. nov., a New Root-Colonizing Fungus. Mycologia 90, 896–903.
Waller, F., Achatz, B., Baltruschat, H., Fodor, J., Becker, K., Fischer, M., et al. (2005). The endophytic fungus Piriformospora indica reprograms barley to salt-stress tolerance, disease resistance, and higher yield. Proc. Natl. Acad. Sci. U. S. A. 102, 13386–13391. doi: 10.1073/pnas.0504423102
Walters, D. R., Ratsep, J., and Havis, N. D. (2013). Controlling crop diseases using induced resistance: challenges for the future. J. Exp. Bot. 64, 1263–1280. doi: 10.1093/jxb/ert026
Wang, S., Ding, L., Liu, J. X., and Han, J. J. (2018). PIF4-Regulated Thermo-responsive Genes in Arabidopsis. Biotechnol. Bull. 34, 57–65. doi: 10.13560/j.cnki.biotech.bull.1985.2018-0211
Weiss, M., Waller, F., Zuccaro, A., and Selosse, M. A. (2016). Sebacinales - one thousand and one interactions with land plants. New Phytol. 211, 20–40. doi: 10.1111/nph.13977
Xiang, S., Wu, S., Zhang, H., Mou, M., Chen, Y., Li, D., et al. (2020). The PIFs Redundantly Control Plant Defense Response against Botrytis cinerea in Arabidopsis. Plants 9:1246. doi: 10.3390/plants9091246
Xu, L., Wu, C., Oelmuller, R., and Zhang, W. (2018). Role of Phytohormones in Piriformospora indica-Induced Growth Promotion and Stress Tolerance in Plants: More Questions Than Answers. Front. Microbiol. 9:1646. doi: 10.3389/fmicb.2018.01646
Yadav, V., Kumar, M., Deep, D. K., Kumar, H., Sharma, R., Tripathi, T., et al. (2010). A phosphate transporter from the root endophytic fungus Piriformospora indica plays a role in phosphate transport to the host plant. J. Biol. Chem. 285, 26532–26544. doi: 10.1074/jbc.M110.111021
Yamashino, T., Kitayama, M., and Mizuno, T. (2013). Transcription of ST2A encoding a sulfotransferase family protein that is involved in jasmonic acid metabolism is controlled according to the circadian clock- and PIF4/PIF5-mediated external coincidence mechanism in Arabidopsis thaliana. Biosci. Biotechnol. Biochem. 77, 2454–2460. doi: 10.1271/bbb.130559
Ye, W., Jiang, J., Lin, Y., Yeh, K. W., Lai, Z., Xu, X., et al. (2019). Colonisation of Oncidium orchid roots by the endophyte Piriformospora indica restricts Erwinia chrysanthemi infection, stimulates accumulation of NBS-LRR resistance gene transcripts and represses their targeting micro-RNAs in leaves. BMC Plant Biol. 19:601. doi: 10.1186/s12870-019-2105-3
Zandalinas, S. I., Fichman, Y., Devireddy, A. R., Sengupta, S., Azad, R. K., and Mittler, R. (2020). Systemic signaling during abiotic stress combination in plants. Proc Natl. Acad. Sci. U. S. A. 117, 13810–13820. doi: 10.1073/pnas.2005077117
Zhang, L. L., Luo, A., Davis, S. J., and Liu, J. X. (2021). Timing to grow: roles of clock in thermomorphogenesis. Trends Plant Sci. 26, 1248–1257. doi: 10.1016/j.tplants.2021.07.020
Zhang, X., Ji, Y., Xue, C., Ma, H., Xi, Y., Huang, P., et al. (2018). Integrated Regulation of Apical Hook Development by Transcriptional Coupling of EIN3/EIL1 and PIFs in Arabidopsis. Plant Cell. 30, 1971–1988. doi: 10.1105/tpc.18.00018
Zipfel, C., and Oldroyd, G. E. (2017). Plant signalling in symbiosis and immunity. Nature 543, 328–336. doi: 10.1038/nature22009
Keywords: plant systemic response, PIF4, Serendipita indica, high ambient temperature, endophytic fungus
Citation: Chen XJ, Yin YQ, Zhu XM, Xia X and Han JJ (2022) High Ambient Temperature Regulated the Plant Systemic Response to the Beneficial Endophytic Fungus Serendipita indica. Front. Plant Sci. 13:844572. doi: 10.3389/fpls.2022.844572
Received: 28 December 2021; Accepted: 15 February 2022;
Published: 16 March 2022.
Edited by:
Ziqiang Zhu, Nanjing Normal University, ChinaReviewed by:
Kai Shu, Northwestern Polytechnical University, ChinaXiaoyu Qiang, Northwest A&F University, China
Copyright © 2022 Chen, Yin, Zhu, Xia and Han. This is an open-access article distributed under the terms of the Creative Commons Attribution License (CC BY). The use, distribution or reproduction in other forums is permitted, provided the original author(s) and the copyright owner(s) are credited and that the original publication in this journal is cited, in accordance with accepted academic practice. No use, distribution or reproduction is permitted which does not comply with these terms.
*Correspondence: Jia-Jia Han, aGFuamlhamlhQHludS5lZHUuY24=