- 1Key Laboratory of Land Surface Process and Climate Change in Cold and Arid Regions, Northwest Institute of Eco-Environment and Resources, Chinese Academy of Sciences, Lanzhou, China
- 2Key Laboratory of Ecohydrology of Inland River Basin, Northwest Institution of Eco-Environment and Resources, Chinese Academy of Sciences, Lanzhou, China
- 3Qilian Mountains Eco-environment Research Center in Gansu Province, Lanzhou, China
- 4Department of Bioresource Engineering, Faculty of Agricultural and Environmental Sciences, McGill University, Sainte-Anne-de-Bellevue, QC, Canada
- 5School of Environmental Sciences, University of Guelph, Guelph, ON, Canada
As an individual plant species can develop its own leaf stoichiometry to adapt to environmental changes, this stoichiometry can provide critical information about a plant species’ growth and its potential management in the ecosystem housing it. However, leaf stoichiometry is largely undocumented in regions with large environmental changes arising from differences in elevation. The leaf stoichiometry of Potentilla fruticosa L., a major alpine shrub playing an important role in supporting ecosystem functions and services in China’s Qilian Mountains (Northeast Qinghai–Tibetan Plateau), was investigated at different elevations (2,400, 2,600, 2,800, 3,000, 3,200, 3,500, and 3,800 m). At each elevation, leaf elemental (C, N, and P) concentrations were measured in P. fruticosa leaves sampled from three plots (10 × 10 m), and edaphic properties were assessed in nine quadrats (1 × 1 m, three quadrats per plot). Temperature and precipitation were calculated using an empirical formula. Maximum and minimum leaf carbon (C) concentrations ([C]leaf) of 524 ± 5.88 and 403 ± 3.01 g kg–1 were measured at 2,600 and 3,500 m, respectively. Leaf nitrogen (N) concentration ([N]leaf) showed a generally increasing trend with elevation and peaked at 3,500 m (27.33 ± 0.26 g kg–1). Leaf phosphorus (P) concentration ([P]leaf) varied slightly from 2,400 to 3,200 m and then dropped to a minimum (0.60 ± 0.10 g kg–1) at 3800 m. The [C]leaf:[N]leaf, [C]leaf:[P]leaf, and [N]leaf:[P]leaf varied little from 2,400 to 3,000 m but fluctuated somewhat at higher elevations. The main factors affecting P. fruticosa leaf stoichiometry were soil organic C, pH, and soil total P, and the main limiting element for the growth of P. fruticosa in the study area was P. In conclusion, changes in elevation affected leaf stoichiometry of P. fruticosa mainly due to altered soil properties, and addressing phosphorus limitation, especially at higher elevations mainly due to losses caused by high precipitation and sparse vegetation, is a key measure to promote P. fruticosa growth in this region.
Introduction
A vigorous, floriferous, deciduous shrub of high genetic diversity, the widely distributed rosaceous shrub Potentilla fruticosa L. (a.k.a. shrubby Potentilla or shrubby cinquefoil), supports many ecosystem functions in the world’s colder habitats, which makes it particularly sensitive to global warming (Elkington, 1969; Miliauskas et al., 2010; Shimono et al., 2010; Yuichiro et al., 2010). Its chemical composition (Ganenko et al., 1988; Zeng et al., 2019), edibility and cosmetic properties (Nkiliza, 1999; Liu et al., 2016), antioxidant content (Miliauskas et al., 2010; Luo et al., 2016), and effects on the expression of key enzymes and hormones of glucose and lipid metabolism in rats (Yan et al., 2019) have been documented. P. fruticosa’s nutritive value has been shown to be influenced by grazing (Yao et al., 2019). Its leaf morphology, physiological and biochemical characteristics have been found to be altered by atmospheric pollution and soil salinity (NaCl and Na2SO4) (Liu et al., 2013; Lugovskaya et al., 2018).
However, the leaf stoichiometry of P. fruticosa at high elevations on the Qinghai–Tibetan Plateau (QTP) remains relatively undocumented. Leaf stoichiometry can reflect the balance and limitations in the uptake of plant macronutrients (C, N, P) that influence plants’ growth rate and life history strategies (Baxter and Dilkes, 2012; Zhu et al., 2020) and global C, N, P biogeochemical cycles (Moe et al., 2005; Liu et al., 2018). Leaf stoichiometry information is critical developing an understanding of nutrient cycling processes, in developing biogeochemical models, and in predicting plant responses to global climate change (Zhao et al., 2014, 2018). Previous studies have shown that besides disturbances such as increased CO2, N, and P availability (Esmeijer-Liu et al., 2009; Scott et al., 2013) and grazing (Bai et al., 2012), topographic factors such as slope, aspect, and elevation (e.g., Qin et al., 2019; Cao et al., 2020) can also affect leaf stoichiometry. This occurs through their influence on soil formation (Jenny, 1941), water distribution, and microclimate. However, topographic factors are rarely considered in leaf stoichiometry, especially for individual species.
This study’s overall objective was to examine the effects of elevation (from 2,400 to 3,800 m) on leaf stoichiometry of P. fruticosa, a major alpine shrub. The study was conducted in the Qilian Mountains of the Qinghai–Tibetan Plateau (QTP), the world’s highest elevation plateau. Since mean annual precipitation (MAP), mean annual temperature (MAT), and soil properties vary with elevation (Table 1), our hypothesis was that leaf stoichiometry of P. fruticosa would vary with elevation, and, based on Cao et al. (2020), that P would be a limiting nutrient for P. fruticosa growth.
Materials and Methods
Study Area
With a mean elevation of 4,000 m (closer to 3,000 m in the northeast), mean annual precipitation (MAP) of 400 mm, and mean annual temperature (MAT) below −4°C, the Qinghai–Tibetan Plateau covers 2.5 × 106km2. Ranging in elevation from 2,200 to 5,500 m, and located in the northeastern portion of the QTP, the Qilian Mountains present two main slope aspects: south-facing and north-facing. On the south-facing slope aspects, grasslands growing on sandy-textured Kastanozem are the dominant vegetation type, whereas on the north-facing slope aspects, Qinghai spruce (Picea crassifolia Kom.), growing on silty-sand-textured Podzol, is the dominant species (Qin et al., 2016).
Field Sampling
In August and September 2018, when most plant species were at the late flowering or fruiting stages, leaves from top and middle of P. fruticosa plants were sampled from three random 10 × 10 m plots situated at each of seven elevations: 2,400, 2,600, 2,800, 3,000, 3,200, 3,500, and 3,800 m (Figure 1). In each plot, multiple soil samples were collected from three quadrats (1 × 1 m) along the diagonal transect. A 70-mm diameter soil drill was used to sample from 3 depth profiles (0–10, 10–20, and 20–40 cm).
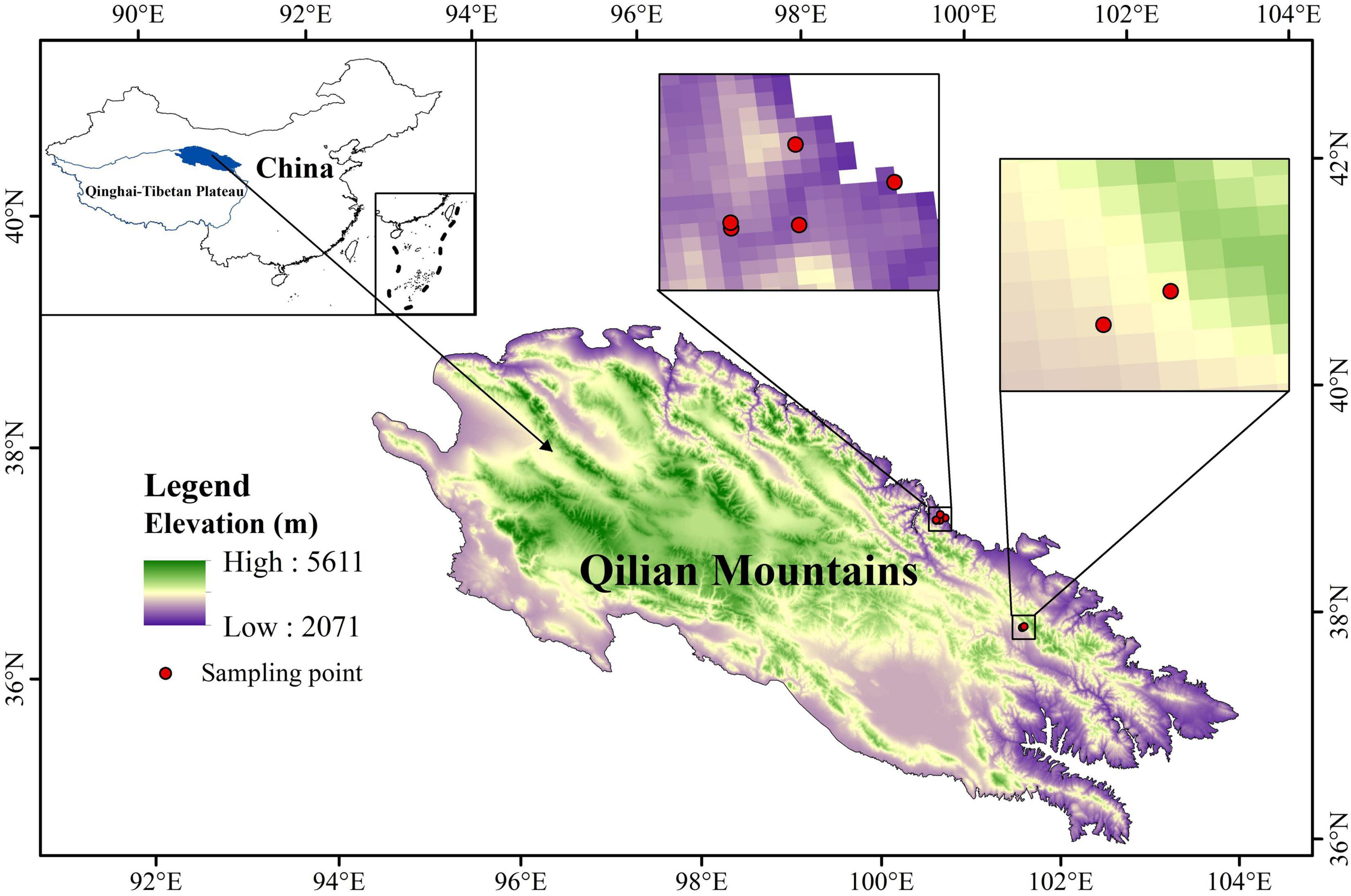
Figure 1. Sampling sites at different elevations (2,400, 2,600, 2,800, 3,000, and 3,200, 3,500, and 3,800 m) in the study area.
Laboratory Analyses
The leaves were dried and ground to determine leaf carbon (C) concentration ([C]leaf), leaf nitrogen (N) concentration ([N]leaf), and leaf phosphorus (P) concentration ([P]leaf). Soil samples were air-dried and ground to pass through a 100-mesh sieve prior to the analysis of soil properties. A volumetric potassium dichromate method was used to determine [C]leaf and soil organic carbon (SOC) (Nelson and Sommers, 1982), and a SmartChen 200 (AMS Rome, Italy) element analyzer was used to measure [N]leaf, [P]leaf, soil total nitrogen (STN), and soil total phosphorus (STP). A standard pH meter was used to determine soil pH in a slurry of 2.5:1 water to air-dried soil ratio (Cao et al., 2018).
Data Analysis
The MAT and MAP (Table 1) were based on Zhao et al. (2005, 2006). For the Qilian Mountain region, these were calculated as:
where MAT = mean annual temperature (°C), MAP = mean annual precipitation (mm), ELEV = elevation (m), LAT = latitude (°), and LONG = longitude (°). R2 indicates the coefficient of determination.
All data were expressed as mean and standard error (SE). The fixed effect (elevation) and random effect (plot) on soil properties and leaf ecological stoichiometry of P. fruticosa were tested by fitting generalized linear mixed models (GLMMs). The t-test was used to check the significant difference (p < 0.05) of each measured parameter between different elevations. Redundancy analysis (RDA) was performed to find the dominant environmental variables influencing leaf stoichiometry of P. fruticosa (Maccherini et al., 2011; Yang et al., 2018a). The significance of the eigenvalues of the canonical axes was tested by a reduced Monte Carlo model with 270 unrestricted permutations (Sun et al., 2017; Yuan, 2017). The Pearson’s correlation coefficient was used to determine the correlation between leaf stoichiometry and abiotic factors. SPSS 22.0 for Windows (SPSS, Inc., Chicago, IL, United States) was used to determine the Pearson’s correlation coefficient. Other analyses were performed in R4.1.1 (vegan and nlme packages). The figures were prepared using Origin 2021 (OriginLab Corp, Roundhouse Plaza, Northampton, MA, United States) and R4.1.1 (ggplot2 and ggrepel packages).
Results
Effects of Elevation on Soil Properties and Leaf Stoichiometry of Potentilla fruticosa
The results from GLMMs showed that except [P]leaf, [C]leaf:[P]leaf, and [N]leaf:[P]leaf, soil properties and other elemental stoichiometries of P. fruticosa were significantly affected by elevation (Table 1). Compared to elevations below 3,000 m, SOC and STN significantly increased (3- to 4-fold) at elevations of 3,200 m and above. However, STP did not show this trend, as it peaked at 2,600 m (0.58 ± 0.02 g kg–1). Variation in SOC:STN across elevations was minimal, with the largest value (11.22 ± 0.10) occurring at 3,500 m and the smallest (8.96 ± 0.46) at 2,600 m. For SOC:STP and STN:STP, the largest and the smallest values were at 3,500 m (487 ± 133 and 42.74 ± 11.46, respectively) and 2,600 m (20.90 ± 0.67 and 2.43 ± 0.12, respectively), respectively. Soil pH values shifted from 6.12 ± 0.03 at 3,500 m to 8.54 ± 0.02 at 2,600 m. The MAT showed a decreasing trend from 2,400 to 3,800 m, whereas MAP showed a converse trend (Table 2).
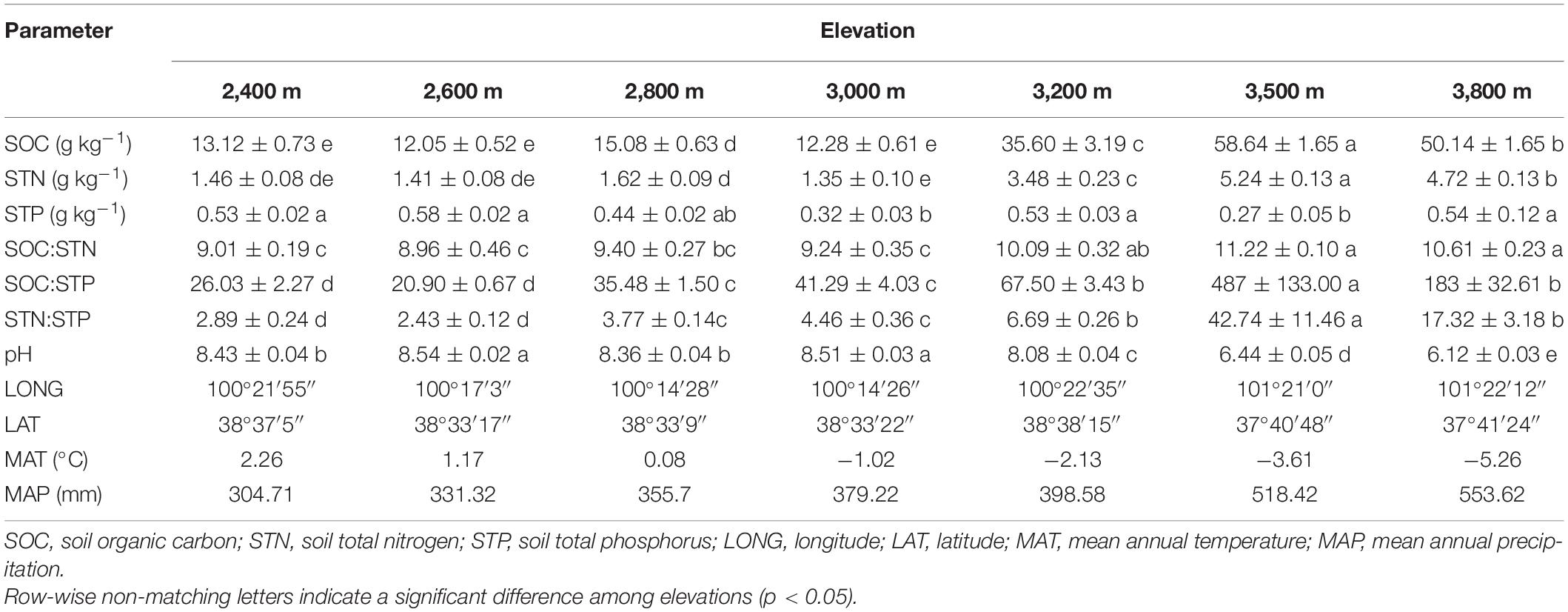
Table 2. Changes of temperature, precipitation, and soil properties at different elevations (mean ± standard error, n = 9).
At 3,500 m, [C]leaf (403 ± 3.01g kg–1) was significantly lower than that at any other elevation, whereas at 2,600 m [C]leaf (524 ± 5.88 g kg–1) was significantly greater than at any other elevations except 3,000 m (Figure 2A). The [N]leaf showed an increasing trend with increasing elevation. At 3,500 m, [N]leaf (27.33 ± 0.26 g kg–1) was significantly greater than at other elevations, whereas [N]leaf at 2,800 m (18.15 ± 0.10 g kg–1) was significantly lower than that at any other elevations (Figure 2B). The [P]leaf changed slightly at elevations between 2,400 and 3,200 m and had a decreasing trend at elevations from 3,500 to 3,800 m, with the lowest value (0.60 ± 0.10 g kg–1) recorded at 3,800 m, which was significantly lower than that at 2,600, 3,200, or 3,500 m (Figure 2C). From 2,400 to 3,000 m, [C]leaf:[N]leaf varied little, but increased significantly at or above 3,200 m. However, from 3,200 to 3,800 m, [C]leaf:[N]leaf showed a decreasing and then an increasing trend, with the value at 3,500 m (14.74 ± 0.19) being significantly lower than that at other elevations (Figure 2D). Changes in [C]leaf:[P]leaf and [N]leaf:[P]leaf along the elevation gradients were similar. Both of them varied slightly between 2,400 and 3,000 m; however, from 3,000 to 3,800 m, their values first decreased and then increased and reached minimums and maximums at 3,200 (392 ± 35.28 and 19.43 ± 1.50, respectively) and 3,800 m (1097 ± 349 and 62.79 ± 19.81, respectively), respectively (Figures 2E,F).
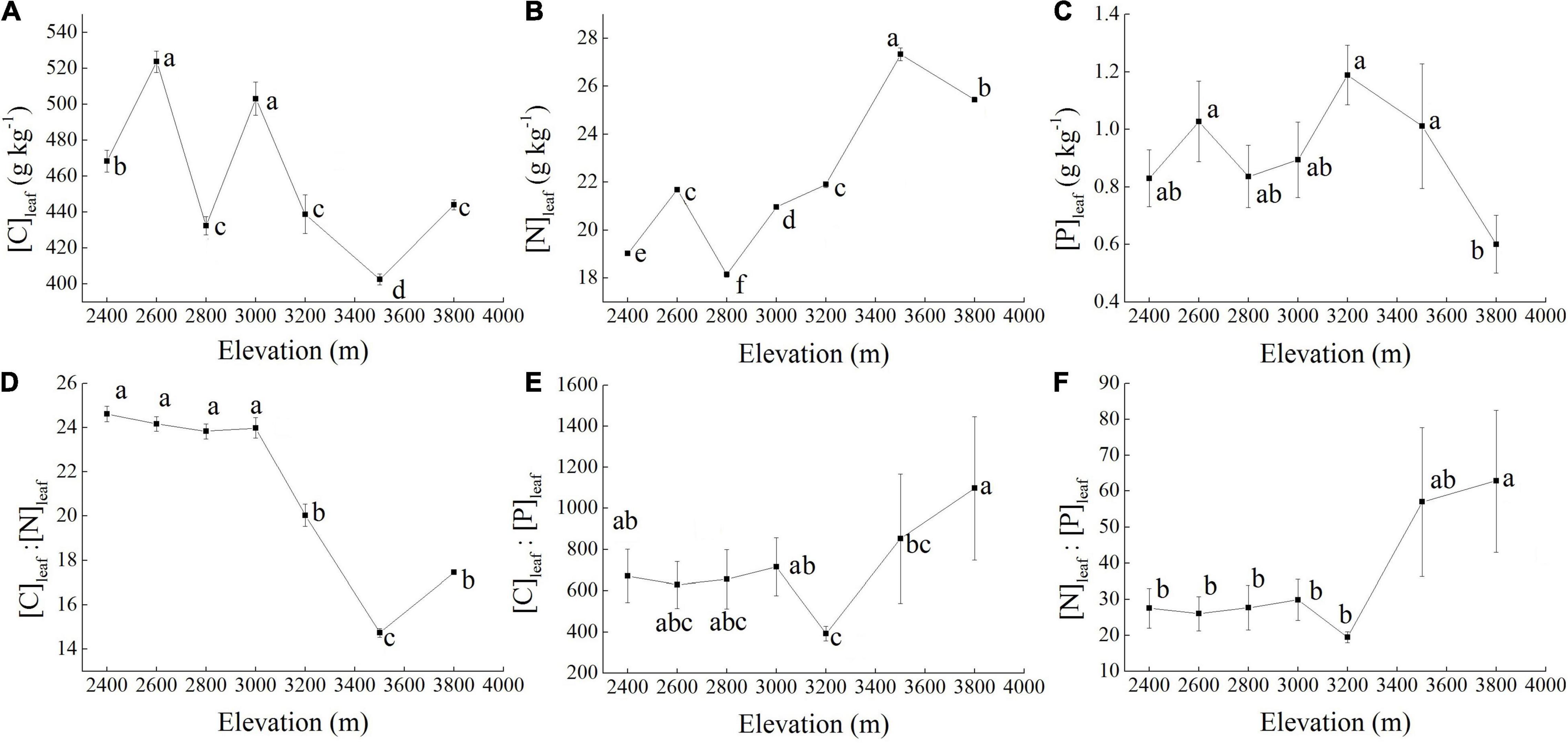
Figure 2. Potentilla fruticosa [C]leaf (A), [N]leaf (B), and [P]leaf (C) and [C]leaf:[N]leaf (D), [C]leaf:[P]leaf (E), and [N]leaf:[P]leaf (F) ratios from 2,400 to 3,800 m (n = 9). [C]leaf, leaf carbon (C) concentration; [N]leaf, leaf nitrogen (N) concentration; [P]leaf, leaf phosphorus (P) concentration. Data are described by their mean and standard error (SE). Different lowercase letters indicate a significant difference among elevations at p < 0.05.
Dominant Factors Influencing Leaf Stoichiometry of Potentilla fruticosa at Different Elevations
The RDA results showed that the eigenvalues of the first and second axes were 0.27 and 0.02, respectively (Figure 3), indicating that the two axes could explain about 29.00% of the total variation in leaf stoichiometry of P. fruticosa across elevations. Based on the RDA result, SOC, STP, and pH had significant effects on this variation, and they could explain 7.10, 6.80, and 6.00% of it, respectively (Table 3).
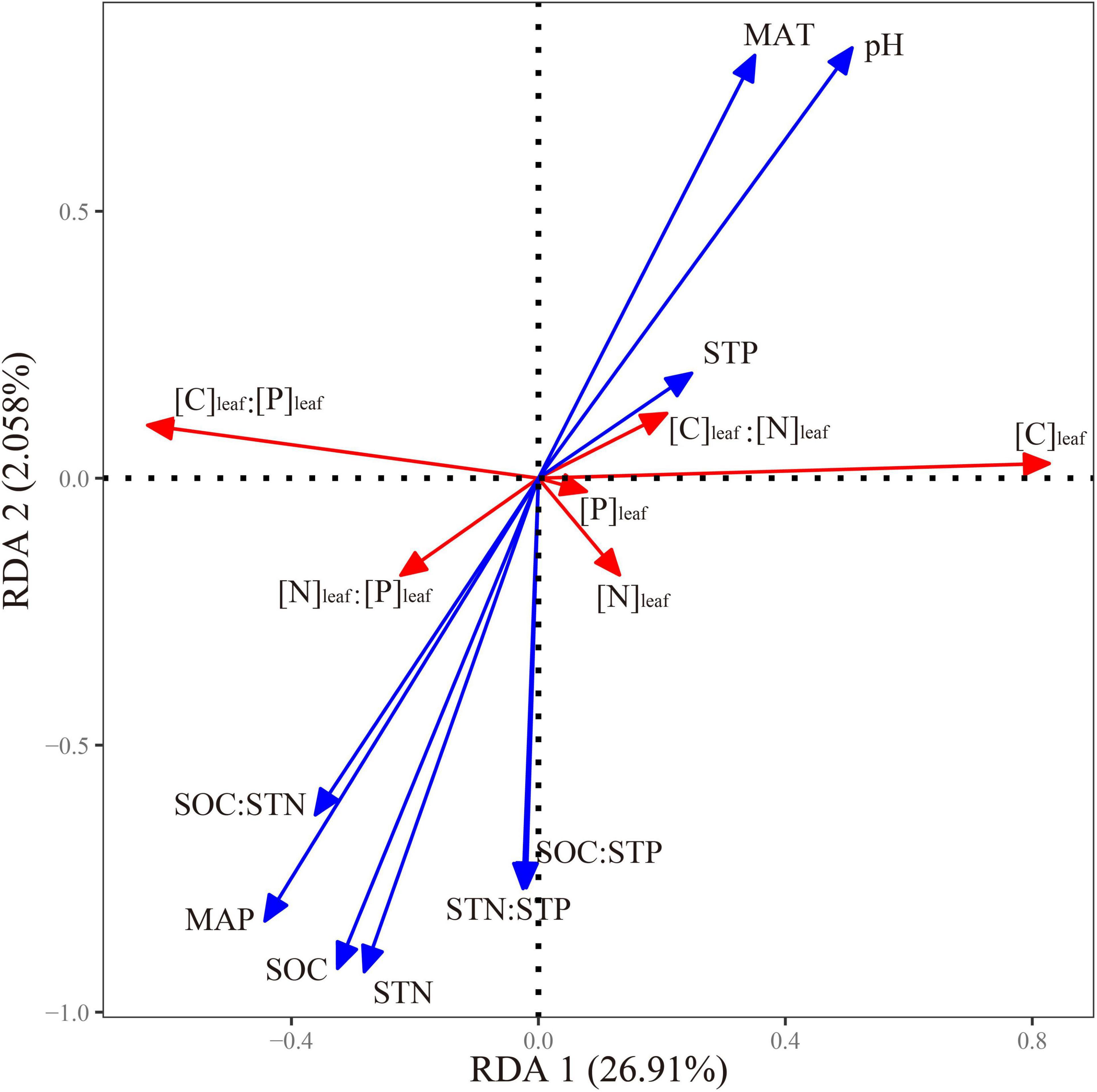
Figure 3. Redundancy analysis (RDA) ordination for the leaf stoichiometric indices of P. fruticosa and environmental characteristics. SOC, soil organic carbon; STN, soil total nitrogen; STP, soil total phosphorus; [C]leaf, leaf carbon (C) concentration; [N]leaf, leaf nitrogen (N) concentration; [P]leaf, leaf phosphorus (P) concentration; MAT, mean annual temperature; MAP, mean annual precipitation.
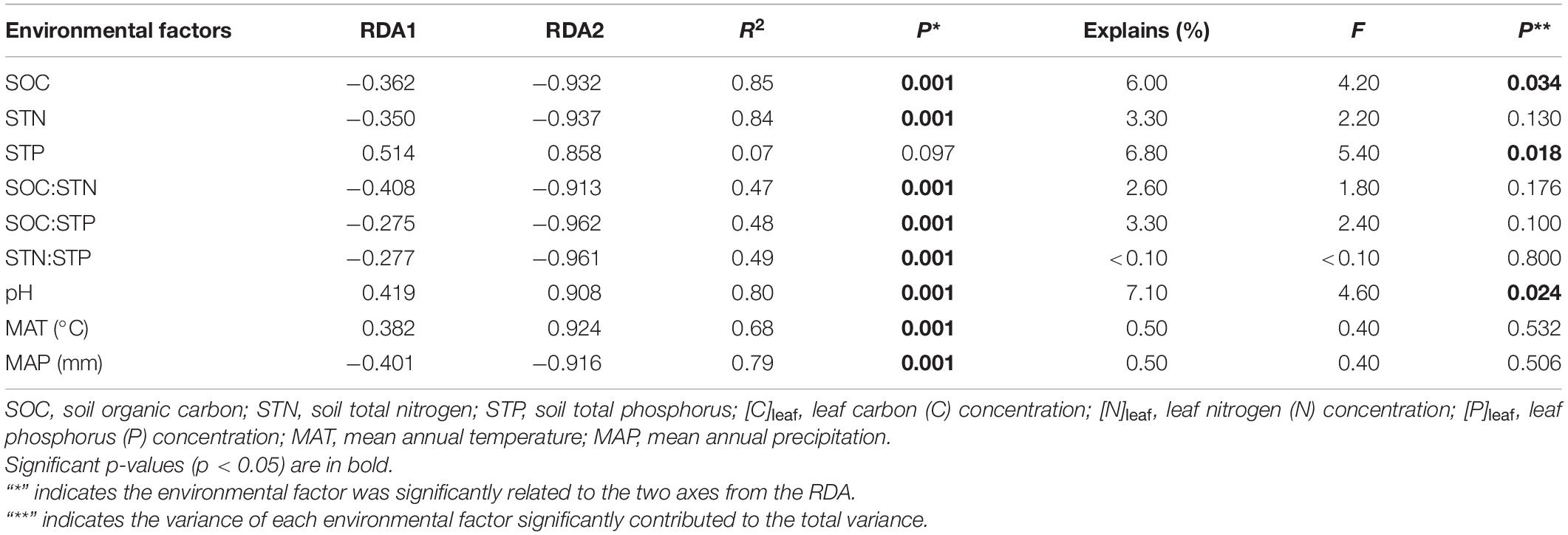
Table 3. Relationships between environmental factors and the two RDA axes, and also environmental factors that are significant contributors to leaf stoichiometry of P. fruticosa across elevations.
Soil pH was positively related to [C]leaf and [C]leaf:[N]leaf and negatively with [N]leaf, [C]leaf:[P]leaf, and [N]leaf:[P]leaf (Table 4). In contrast, the relationship between SOC and leaf stoichiometry was the opposite of those of soil pH, except that there was no significant relationship between SOC and [C]leaf:[P]leaf. The STP was not related to any index of leaf stoichiometry.
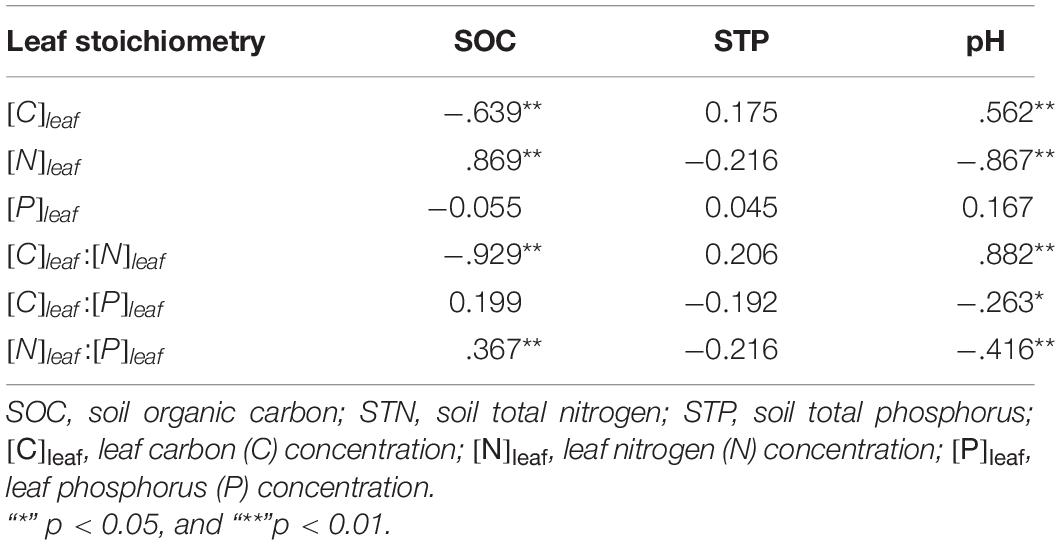
Table 4. Relationships between leaf stoichiometry of P. fruticosa and the dominant factors from the RDA.
Discussion
Reasons for Variation in Soil Properties and Leaf Stoichiometry of Potentilla fruticosa With Elevation
By mainly influencing solar radiation and condensation of water vapor (Sevruk, 1997; Ohmura, 2012), elevation regulates temperature and precipitation (Lozano-García et al., 2016; Zhu et al., 2019), which in turn exerts effects on the distribution of vegetation. For example, in the Qilian Mountains, temperature decreases and precipitation increases with increasing elevation (Chang et al., 2014), resulting in shifting vegetation types: <2,400 m, steppe desert; 2,400–3,300 m, forest steppe; 3,300–3,600 m, subalpine scrub and grassland; 3,600–3,900 m, alpine scrubs and meadow; >3,900 m, ice and snow (Zhu et al., 2019). Likewise, different elevations differ in vegetation types, biomass, quantity and quality of litter, roots, and soil microbial communities (Bargali et al., 2018; Yang et al., 2018b), which in turn affect soil physical and chemical properties (Tables 1, 2; Zhou et al., 2013; Qin et al., 2019). In addition, microlandforms such as slope aspect, slope position, and slope gradient can also influence soil properties by reshaping hydrothermal conditions and patterns in the movement of the material and energy (Måren et al., 2015; Nabiollahi et al., 2018; Zhang et al., 2020) as found in this study (data unpublished).
With changes of biotic and abiotic environments with elevation, leaf stoichiometry of P. fruticosa also varied with elevation (Figure 2), concurring with other studies (e.g., Badano et al., 2005; Zhang et al., 2019). However, only [C]leaf, [N]leaf, and [C]leaf:[N]leaf of P. fruticosa were significantly affected by elevation (Table 1), partly supporting our hypothesis that the leaf stoichiometry of P. fruticosa would vary with elevation. In contrast, Cao et al. (2020) found that except for [N]leaf:[P]leaf, leaf stoichiometries of Oxytropis ochrocephala Bunge in the Qilian Mountains were significantly affected by elevation. This suggests that each species may have its unique strategies to adapt to local environmental changes.
From 2,400 to 3,800 m, [C]leaf of P. fruticosa showed a decreasing trend (Figure 2A), which was in contrast to Zhao et al. (2014) and Rong et al. (2016), who found that [C]leaf increased with decreasing temperature to balance the osmotic pressure of cells and resist freezing. This result may reflect the fact that low temperatures inhibit photosynthesis in P. fruticosa. In contrast, [N]leaf of P. fruticosa showed an increasing trend with a decrease in temperature (Figure 2B), as reported by others (e.g., Oleksyn and Przybyl, 1987; Cao et al., 2020). This may be because [N]leaf can enhance metabolic activity and the growth rate of tissues in cold habitats and short growing seasons (Ågren, 2008; Zhang et al., 2017). With a [C]leaf decrease and [N]leaf increase, P. fruticosa [C]leaf:[N]leaf decreased with rising elevation (Figure 2D). Similar observations were reported by Sun et al. (2017). Generally, [C]leaf:[N]leaf reflects a plant’s ability to simultaneously absorb C and N, and a low value can benefit plant growth (He et al., 2008; Yan et al., 2015).
The Dominant Environmental Factors Influencing Leaf Stoichiometry of Potentilla fruticosa
Based on RDA (Figure 3 and Table 3), it is clear that SOC, STP, and pH had a greater effect on leaf stoichiometry of P. fruticosa than temperature or precipitation in the Qilian Mountains. This is in slight contradiction with other studies (Sardans et al., 2011; Zhang et al., 2012a; Cao et al., 2020). For example, Cao et al. (2020) found that, across various elevations in the Qilian Mountains, temperature significantly affected leaf stoichiometry of O. ochrocephala, as it could dictate or control nutrient availability in soils, root absorption, and the plant nutrient budget (Reich and Oleksyn, 2004; Isles et al., 2017; Liu et al., 2019). Likewise, Zhang et al. (2012a) found that temperature and precipitation directly affected the spatial patterns of leaf elemental stoichiometry across China, as precipitation regulates the mobilization of soil nutrients (Müller et al., 2017).
Although SOC, STP, and pH were the main contributors to differences in leaf stoichiometry of P. fruticosa, STP was not related to any index of leaf stoichiometry (Table 4), suggesting that it had a synthetic effect on leaf stoichiometry of P. fruticosa in the Qilian Mountains, but this needs further study. Except [P]leaf, leaf nutrient concentrations and their ratios were all significantly related to SOC or pH or both (Table 4). The SOC was negatively related to [C]leaf, which was not consistent with Niu et al. (2016) who found that these were positively correlated because the C in leaves can enter the soil through litter. In this study, elevations ≥3,200 m had greater SOC but lower temperatures (Table 1), which limited photosynthesis and thus resulted in lower [C]leaf (Figure 2A). This suggests that the relationship between [C]leaf of P. fruticosa and SOC in the Qilian Mountains may not represent a true causality. This may also suggest that the C in soil is the structural basis for plants (Schade et al., 2003; Liu et al., 2011) as less C is captured from the atmosphere by leaves subjected to low temperatures. The SOC was positively related to [N]leaf of P. fruticosa, because SOC from amino acid metabolism contains N and it can be transferred from soil to plants by the process of nutrient cycling (Delgado-Baquerizo et al., 2015; Zhang et al., 2019). Given the positive relationship between SOC and [N]leaf, there exists a negative or positive relationship with [C]leaf:[N]leaf, or [N]leaf:[P]leaf (Table 4). Generally, SOC and pH are negatively correlated, as acidic soil is beneficial to the adsorption of organic C (Zhang et al., 2012b; Hobara et al., 2016). Therefore, the relationships between pH and leaf stoichiometry of P. fruticosa were converse to relationships between SOC and leaf stoichiometry (Table 4).
In this study, the measured parameters can only explain about 30.00% of the total variation of leaf stoichiometry of P. fruticosa (Figure 3), indicating that other factors, such as plant community composition (Wang and Moore, 2014; Zhang et al., 2019), may also control the variations. As the plant community in the study area changed with elevation, effects of intra- and interspecies competitions on leaf stoichiometry of P. fruticosa should also be considered to achieve a comprehensive understanding.
Limiting Nutrients for Potentilla fruticosa Across Elevations
It is well known that [N]leaf:[P]leaf rather than [N]leaf or [P]leaf individually can provide a better assessment of a plant’s nutrient limitations (Li et al., 2018) although this assessment is still debated. According to Soudzilovskaia et al. (2005), the growth of alpine vascular plants is limited first by N, then by P, with a mean foliar N:P mass ratio of 29 in their study area. In this study, the [N]leaf:[P]leaf of P. fruticosa at 3,000, 3,500, and 3,800 m were all >29 (Figure 2F). Following the criteria provided by Soudzilovskaia et al. (2005), our results suggest that P limited the growth of P. fruticosa at higher elevations. Soil P deficiency is common across China (Han et al., 2005; Zhao et al., 2016), including across the entire QTP (Niu et al., 2016) and the Qilian Mountains (Xu et al., 2018, 2019; Zhang et al., 2019; Cao et al., 2020). Furthermore, at elevations ≥3,500 m, the [N]leaf:[P]leaf was >50 (Figure 2F), suggesting that P. fruticosa growth was greatly restricted by available P. It is well known that in the Qilian Mountains, soil surface coverage by vegetation decreases as elevation increases. In combination with P leaching through the soil profile (Chardon and Schoumans, 2007), the lack of vegetative cover at high elevations can easily increase P losses through erosion and surface run-off (Nest et al., 2014) and can make P scarcer. However, Reich and Oleksyn (2004) concluded that plant growth in high elevations was more limited by N. This suggests that limitation of nutrient elements for plants is dependent on region.
Conclusion
In the Qilian Mountains of the northeast QTP, soil properties were more sensitive to elevations (ranging from 2,400 to 3,800 m) than leaf stoichiometry of P. fruticosa. From low to high elevation, [C]leaf and [P]leaf decreased, whereas [N]leaf of P. fruticosa increased, as an adaptation for maintaining metabolic activity in cold habitats.
Elevation only affected P. fruticosa [C]leaf, [N]leaf, and [C]leaf:[N]leaf, mainly through its effects on SOC, STP, and pH. Although [N]leaf:[P]leaf of P. fruticosa was not influenced by elevation, its value across all elevations was relatively large. This suggests that in the study area, P. fruticosa growth was commonly limited by soil P, especially regarding its growth at higher elevations. As P. fruticosa is a major alpine shrub, reducing P losses and improving its growth conditions will play an important role in maintaining the ecologically integrated functions and services of the whole QTP.
Data Availability Statement
The raw data supporting the conclusions of this article will be made available by the authors.
Author Contributions
YQ: conception and design of the research. XZ and YQ: acquisition of data. WL: analysis and interpretation of data. XZ: statistical analysis. WL, XZ, and YQ: drafting the manuscript. JA and AB: revision of manuscript drafting and revision of manuscript. All authors read and approved the final manuscript.
Funding
This study was supported by the Second Tibetan Plateau Scientific Expedition and Research Program (2019QZKK0103), the Strategic Priority Research Program of the Chinese Academy of Sciences (XDA2006010101), the National Natural Science Fund of China (41771252), Forestry and Grassland Science and Technology Innovation Program of Gansu Province [GYCX(2020)01], and the Natural Science of Gansu Province (21JR7RA045).
Conflict of Interest
The authors declare that the research was conducted in the absence of any commercial or financial relationships that could be construed as a potential conflict of interest.
Publisher’s Note
All claims expressed in this article are solely those of the authors and do not necessarily represent those of their affiliated organizations, or those of the publisher, the editors and the reviewers. Any product that may be evaluated in this article, or claim that may be made by its manufacturer, is not guaranteed or endorsed by the publisher.
References
Ågren, G. I. (2008). Stoichiometry and nutrition of plant growth in natural communities. Annu. Rev. Ecol. Evol. Syst. 39, 153–170. doi: 10.1146/annurev.ecolsys.39.110707.173515
Badano, E. I., Cavieres, L. A., Molina-Montenegro, M. A., and Quiroz, C. L. (2005). Slope aspect influences plant association patterns in the mediterranean matorral of central Chile. J. Arid. Environ. 62, 93–108. doi: 10.1016/j.jaridenv.2004.10.012
Bai, Y., Wu, J., Clark, C. M., Pan, Q., Zhang, L., Chen, S., et al. (2012). Grazing alters ecosystem functioning and C: N: P stoichiometry of grasslands along a regional precipitation gradient. J. Appl. Ecol. 49, 1204–1215. doi: 10.1111/j.1365-2664.2012.02205.x
Bargali, K., Manral, V., Padalia, K., Bargali, S. S., and Upadhyay, P. U. (2018). Effect of vegetation type and season on microbial biomass carbon in Central Himalayan forest soils, India. Catena 171, 125–135. doi: 10.1016/j.catena.2018.07.001
Baxter, I., and Dilkes, B. P. (2012). Elemental profiles reflect plant adaptations to the environment. Science 336, 1661–1663. doi: 10.1126/science.1219992
Cao, J., Wang, X., Adamowski, J. F., Biswas, A., Liu, C., Chang, Z., et al. (2020). Response of leaf stoichiometry of Oxytropis ochrocephala to elevation and slope aspect. Catena 194:104772. doi: 10.1016/j.catena.2020.104772
Cao, J., Xu, X., Deo, R. C., Holden, N. M., Adamowski, J. F., Gong, Y., et al. (2018). Multi-household grazing management pattern maintains better soil fertility. Agron. Sustain. Dev. 38:6. doi: 10.1007/s13593-017-0482-2
Chang, X. F., Zhu, X. X., Wang, S. P., Cui, S. J., Luo, C. Y., Zhang, Z. H., et al. (2014). Impacts of management practices on soil organic carbon in degraded alpine meadows on the Tibetan Plateau. Biogeosciences 11, 3495–3503. doi: 10.5194/bg-11-3495-2014
Chardon, W. J., and Schoumans, O. F. (2007). Soil texture effects on the transport of phosphorus from agricultural land in river deltas of Northern Belgium, The Netherlands and North-West Germany. Soil Use Manage. 23, 16–24. doi: 10.1111/j.1475-2743.2007.00108.x
Delgado-Baquerizo, M., García-Palacios, P., Milla, R., Gallardo, A., and Maestre, F. T. (2015). Soil characteristics determine soil carbon and nitrogen availability during leaf litter decomposition regardless of litter quality. Soil Biol. Biochem. 81, 134–142. doi: 10.1016/j.soilbio.2014.11.009
Elkington, T. T. (1969). Cytonaxonomic variation in Potentilla fruticosal. New Phytol. 68, 151–160. doi: 10.1111/j.1469-8137.1969.tb06428.x
Esmeijer-Liu, A. J., Aerts, R., Kürschner, W. M., Bobbink, R., Lotter, A. F., and Verhoeven, J. T. A. (2009). Nitrogen enrichment lowers Betula pendula green and yellow leaf stoichiometry irrespective of effects of elevated carbon dioxide. Plant Soil 316, 311–322. doi: 10.1007/s11104-008-9783-1
Ganenko, T. V., Lutskii, B. I., Larin, M. F., Vereshchagin, A. L., and Semenov, A. A. (1988). Chemical composition of Potentilla fruticosa. I. Flavonoids. Chem. Nat. Comp. 3, 387–388. doi: 10.1007/BF00598597
Han, W., Fang, J., Guo, D., and Zhang, Y. (2005). Leaf nitrogen and phosphorus stoichiometry across 753 terrestrial plant species in China. New Phytol. 168, 377–385. doi: 10.1111/j.1469-8137.2005.01530.x
He, J., Wang, L., Flynn, D. F. B., Wang, X., Ma, W., and Fang, J. (2008). Leaf nitrogen: phosphorus stoichiometry across Chinese grassland biomes. Oecologia 155, 301–310. doi: 10.1007/s00442-007-0912-y
Hobara, S., Kushida, K., Kim, Y., Koba, K., Lee, B. Y., and Ae, N. (2016). Relationships among pH, minerals, and carbon in soils from tundra to boreal forest across Alaska. Ecosystems 19, 1092–1103. doi: 10.1007/s10021-016-9989-7
Isles, P. D. F., Xu, Y., Stockwell, J. D., and Schroth, A. W. (2017). Climate-driven changes in energy and mass inputs systematically alter nutrient concentration and stoichiometry in deep and shallow regions of Lake Champlain. Biogeochemistry 133, 201–217. doi: 10.1007/s10533-017-0327-8
Jenny, H. (1941). Factors of soil formation: a system of quantitative pedology. Soil Sci. 5, 415–426. doi: 10.1134/S1064229309120138
Li, F., Hu, J., Xie, Y., Yang, G., Hu, C., Chen, X., et al. (2018). Foliar stoichiometry of carbon, nitrogen, and phosphorus in wetland sedge Carex brevicuspis along a small-scale elevation gradient. Ecol. Indic. 92, 322–329. doi: 10.1016/j.ecolind.2017.04.059
Liu, G., Ye, X., Huang, Z., and Cornelissen, J. H. C. (2019). Leaf and root nutrient concentrations and stoichiometry along aridity and soil fertility gradients. J. Veg. Sci. 2, 291–300. doi: 10.1111/jvs.12717
Liu, R. T., Zhao, H. L., Zhao, X. Y., and Drake, S. (2011). Facilitative effects of shrubs in shifting sand on soil macro-faunal community in Horqin Sand Land of Inner Mongolia, Northern China. Eur. J. Soil Biol. 47, 316–321. doi: 10.1016/j.ejsobi.2011.07.006
Liu, X., Zhang, Y., Han, W., Tang, A., Shen, J., Cui, Z., et al. (2013). Enhanced nitrogen deposition over China. Nature 494, 459–462. doi: 10.1038/nature11917
Liu, Y., Liu, Q., Wang, T., and Fang, S. (2018). Leaf nitrogen and phosphorus stoichiometry of Cyclocarya paliurus across China. Forests 9:771. doi: 10.3390/f9120771
Liu, Z., Luo, Z., Jia, C., Wang, D., and Li, D. (2016). Synergistic effects of Potentilla fruticosa L. leaves combined with green tea polyphenols in a variety of oxidation systems. J. Food. Sci. 81, C1091–C1101. doi: 10.1111/1750-3841.13292
Lozano-García, B., Parras-Alcántara, L., and Brevik, E. C. (2016). Impact of topographic aspect and vegetation (native and reforested areas) on soil organic carbon and nitrogen budgets in Mediterranean natural areas. Sci. Total Environ. 544, 963–970. doi: 10.1016/j.scitotenv.2015.12.022
Lugovskaya, A. Y., Khramova, E., and Chankina, O. V. (2018). Effect of transport and industrial pollution on morphometric parameters and element composition of Potentilla fruticosa. Contemp. Probl. Ecol. 1, 89–98. doi: 10.1134/S1995425518010092
Luo, Z., Wang, S., and Wang, D. (2016). Phenolic profiles and antioxidant capacities of crude extracts and subsequent fractions from Potentilla fruticosa L. leaves. Nat. Prod. Res. 30, 1890–1895. doi: 10.1080/14786419.2015.1084303
Maccherini, S., Marignani, M., Gioria, M., Renzi, M., Rocchini, D., Santi, E., et al. (2011). Determinants of plant community composition of remnant biancane badlands: a hierarchical approach to quantify species-environment relationships. Appl. Veg. Sci. 14, 378–387. doi: 10.1111/j.1654-109x.2011.01131.x
Måren, I. E., Karki, S., Prajapati, C., Yadav, R. K., and Shrestha, B. B. (2015). Facing north or south: does slope aspect impact forest stand characteristics and soil properties in a semiarid trans-Himalayan valley? J. Arid Environ. 121, 112–123. doi: 10.1016/j.jaridenv.2015.06.004
Miliauskas, G., Beek, T. A. V., Venskutonis, P. R., Linssen, J. P. H., Waard, P., and Sudhölter, E. J. R. (2010). Antioxidant activity of Potentilla fruticosa. J. Sci. Food Agric. 84, 1997–2009. doi: 10.1002/jsfa.1914
Moe, S. J., Stelzer, R. S., Forman, M. R., Harpole, W. S., Daufresne, T., and Yoshida, T. (2005). Recent advances in ecological stoichiometry: insights for population and community ecology. Oikos 109, 29–39. doi: 10.1111/j.0030-1299.2005.14056.x
Müller, M., Oelmann, Y., Schickhof, U., Böhner, J., and Scholten, T. (2017). Himalayan treeline soil and foliar C: N: P stoichiometry indicate nutrient shortage with elevation. Geoderma 291, 21–32. doi: 10.1016/j.geoderma.2016.12.015
Nabiollahi, K., Golmohamadi, F., Taghizadeh-Mehrjardi, R., Kerry, R., and Davari, M. (2018). Assessing the effects of slope gradient and land use change on soil quality degradation through digital mapping of soil quality indices and soil loss rate. Geoderma 318, 16–28. doi: 10.1016/j.geoderma.2017.12.024
Nelson, D. W., and Sommers, L. E. (1982). “Total carbon, organic carbon, and organic matter,” in Methods Of Soil Analysis Part 2. Chemical And Microbiological Properties, ed. A. L. Page (Madison, WI: American Society of Agronomy), 539–579.
Nest, T. V., Vandecasteele, B., Ruysschaert, G., Cougnon, M., Merckx, R., and Reheul, D. (2014). Effect of organic and mineral fertilizers on soil P and C levels, crop yield and P leaching in a long term trial on a silt loam soil. Agric. Ecosyst. Environ. 197, 309–317. doi: 10.1016/j.agee.2014.07.019
Niu, R., Gao, X., Xu, F., Wang, W., Wang, L., Sun, P., et al. (2016). Carbon, nitrogen, and phosphorus stoichiometric characteristics of soil and leaves from young and middle aged Larix principis-rupprechtii growing in a Qinling Mountain plantation. Acta Ecol. Sin. 36, 7384–7392. doi: 10.5846/stxb201601080057
Nkiliza, J. (1999). Process for Extracting Catechin Polyphenols From Potentillas, Extract Obtained and Its Use. United States Berkem (Gardonne, FR) 5928646. Available online at: https://www.freepatentsonline.com/5928646.html (accessed July 27, 1999).
Ohmura, A. (2012). Enhanced temperature variability in high-altitude climate change. Theor. Appl. Climatol. 110, 499–508. doi: 10.1007/s00704-012-0687-x
Oleksyn, J., and Przybyl, K. (1987). Oak decline in the Soviet Union-scale and hypotheses. Eur. J. For. Pathol. 6, 321–336. doi: 10.1111/1439-0329.ep8118658
Qin, Y., Adamowski, J. F., Deo, R. C., Hu, Z., Cao, J., Zhu, M., et al. (2019). Controlling factors of plant community composition with respect to the slope aspect gradient in the Qilian Mountains. Ecosphere 10:e02851. doi: 10.1002/ecs2.2851
Qin, Y., Feng, Q., Holden, N. M., and Cao, J. (2016). Variation in soil organic carbon by slope aspect in the middle of the Qilian Mountains in the upper Heihe River Basin, China. Catena 147, 308–314. doi: 10.1016/j.catena.2016.07.025
Reich, P. B., and Oleksyn, J. (2004). Global patterns of plant leaf N and P in relation to temperature and latitude. Proc. Natl. Acad. Sci. U.S.A. 101, 11001–11006. doi: 10.1073/pnas.0403588101
Rong, Q., Liu, J., Cai, Y., Lu, Z., Zhao, Z., Yue, W., et al. (2016). “Fertile island” effects of Tamarix chinensis Lour. on soil N and P stoichiometry in the coastal wetland of Laizhou Bay, China. J. Soil Sediment. 16, 864–877. doi: 10.1007/s11368-015-1296-y
Sardans, J., Rivas-Ubach, A., and Peñuelas, J. (2011). Factors affecting nutrient concentration and stoichiometry of forest trees in Catalonia (NE Spain). For. Ecol. Manag. 262, 2024–2034. doi: 10.1016/j.foreco.2011.08.019
Schade, J. D., Kyle, M., Hobbie, S. E., Fagan, W. F., and Elser, J. J. (2003). Stoichiometric tracking of soil nutrients by a desert insect herbivore. Ecol. Lett. 6, 96–101. doi: 10.1046/j.1461-0248.2003.00409.x
Scott, E. E., Prater, C., Norman, E., Baker, B. C., Evans-White, M., and Scott, J. T. (2013). Leaf-litter stoichiometry is affected by streamwater phosphorus concentrations and litter type. Freshw. Sci. 32, 753–761. doi: 10.1899/12-215.1
Sevruk, B. (1997). Regional dependency of precipitation-altitude relationship in the swiss alps. Clim. Change High Elevation Sites 36, 355–369. doi: 10.1007/978-94-015-8905-5_7
Shimono, A., Ueno, S., Gu, S., Zhao, X., Tsumura, Y., and Tang, Y. (2010). Range shifts of Potentilla fruticosa on the Qinghai-Tibetan Plateau during glacial and interglacial periods revealed by chloroplast DNA sequence variation. Heredity 104:534. doi: 10.1038/hdy.2009.158
Soudzilovskaia, N. A., Onipchenko1, V. G., Cornelissen, J. H. C., and Aerts, R. (2005). Biomass production, N: P ratio and nutrient limitation in a Caucasian alpine tundra plant community. J Veg. Sci. 16, 399–406. doi: 10.1111/j.1654-1103.2005.tb02379.x
Sun, L., Zhang, B., Wang, B., Zhang, G., Zhang, W., Zhang, B., et al. (2017). Leaf elemental stoichiometry of Tamarix Lour. species in relation to geographic, climatic, soil, and genetic components in China. Ecol. Eng. 106, 448–457. doi: 10.1016/j.ecoleng.2017.06.018
Wang, M., and Moore, T. R. (2014). Carbon, nitrogen, phosphorus, and potassium stoichiometry in an ombrotrophic peatland reflects plant functional type. Ecosystems 17, 673–684. doi: 10.1007/s10021-014-9752-x
Xu, X., Qin, Y., and Cao, J. (2019). Variation characteristics of potentilla bifurca leaf stoichiometry along the elevation gradient on the northeastern Qinghai-Tibetan Plateau. Acta Ecol. Sin. 39, 9044–9051. doi: 10.5846/stxb201805231128
Xu, X., Qin, Y., Cao, J., Li, M., Gong, Y., and Zhang, X. (2018). Elevational variations of leaf stoichiometry in Leontopodium leontopodioides on the Qinghai-Tibetan Plateau, China. Ying Yong Sheng Tai Xue Bao 29, 3934–3940. doi: 10.13287/j.1001-9332.201812.021
Yan, P., Liu, L., Zeng, Y., Chen, H., Li, M., and Li, J. (2019). Effects of Fruticosa potentilla L. on expression of glucose and lipid metabolism key enzymes and hormones in T2DM rats. Chinese Pharmacol. Bull. 35, 293–294. doi: 10.3969/j.issn.1001-1978.2019.02.029
Yan, Z., Kim, N., Han, W., Guo, Y., Han, T., and Du, E. (2015). Effects of nitrogen and phosphorus supply on growth rate, leaf stoichiometry, and nutrient resorption of Arabidopsis thaliana. Plant Soil 2, 147–155. doi: 10.1007/s11104-014-2316-1
Yang, F., Niu, K., Collins, C. G., Yan, X., Ji, Y., Ling, N., et al. (2018a). Grazing practices affect the soil microbial community composition in a Tibetan alpine meadow. Land Deg. Dev. 30, 49–59. doi: 10.1002/ldr.3189
Yang, F., Wu, J., Zhang, D., Chen, Q., Zhang, Q., and Cheng, X. (2018b). Soil bacterial community composition and diversity in relation to edaphic properties and plant traits in grasslands of southern China. Appl. Soil Ecol. 128, 43–53. doi: 10.1016/j.apsoil.2018.04.001
Yao, X., Wu, J., and Gong, X. (2019). Precipitation and seasonality affect grazing impacts on herbage nutritive values in alpine meadows on the Qinghai-Tibet Plateau. J. Plant Ecol. 6, 993–1008. doi: 10.1093/jpe/rtz027
Yuan, Z. (2017). Factors affecting lucerne-rich vegetation under revegetation in a semi-arid environment. Ecol. Eng. 108, 249–254. doi: 10.1016/j.ecoleng.2017.08.030
Yuichiro, Y., Yoko, S., Mitsuru, H., Ayako, S., and Toshiyuki, O. (2010). The role of shrub (Potentilla fruticosa) on ecosystem CO2 fluxes in an alpine shrub meadow. J. Plant Ecol. 2, 89–97. doi: 10.1093/jpe/rtq011
Zeng, Y., Sun, Y., Meng, X., Yu, T., and Zhang, Y. (2019). A new methylene bisflavan-3-ol from the branches and leaves of Potentilla fruticosa. Nat. Prod. Res. 34, 1–8. doi: 10.1080/14786419.2018.1557169
Zhang, J., Zhao, N., Liu, C., Yang, H., Li, M., Yu, G., et al. (2017). C: N: P stoichiometry in China’s forests: from organs to ecosystems. Funct. Ecol. 32, 50–60. doi: 10.1111/1365-2435.12979
Zhang, S., Zhang, J., Slik, J., and Cao, K. (2012a). Leaf element concentrations of terrestrial plants across China are influenced by taxonomy and the environment. Glob. Ecol. Biogeogr. 8, 809–818. doi: 10.1111/j.1466-8238.2011.00729.x
Zhang, S., Chen, D., Sun, D., Wang, X., Smith, J., and Du, G. (2012b). Impacts of altitude and position on the rates of soil nitrogen mineralization and nitrification in alpine meadows on the eastern Qinghai-Tibetan Plateau, China. Biol. Fertil. Soils. 4, 393–400. doi: 10.1007/s00374-011-0634-5
Zhang, X., Adamowski, J. F., Liu, C., Zhou, J., Zhu, G., Dong, X., et al. (2020). Which slope aspect and gradient provides the best afforestation-driven soil carbon sequestration on the China’s Loess Plateau? Ecol. Eng. 147:105782. doi: 10.1016/j.ecoleng.2020.105782
Zhang, X., Liu, X., Jing, W., and Cao, J. (2019). Variation characteristics of Leontopodium leontopodioides leaf stoichiometry with altitude and their relationship with soil nutrients in Qilian Mountains, Northwest China. Chinese J. Appl. Ecol. 30, 4012–4020. doi: 10.13287/j.1001-9332.201912.009
Zhao, C., Nan, Z., and Cheng, G. (2005). Methods for modelling of temporal and spatial distribution of air temperature at landscape scale in the southern Qilian mountains, China. Ecol. Model. 189, 209–220. doi: 10.1016/j.ecolmodel.2005.03
Zhao, C., Nan, Z., Cheng, G., Zhang, J., and Feng, Z. (2006). GIS-assisted modelling of the spatial distribution of Qinghai spruce (Picea crassifolia) in the Qilian Mountains, northwestern China based on biophysical parameters. Ecol. Model. 191, 487–550. doi: 10.1016/j.ecolmodel.2005.05.018
Zhao, N., He, N., Wang, Q., Zhang, X., Wang, R., Xu, Z., et al. (2014). The altitudinal patterns of leaf C: N: P stoichiometry are regulated by plant growth form, climate and soil on Changbai Mountain. China. PLoS One 9:e95196. doi: 10.1371/journal.pone.0095196
Zhao, W. Q., Reich, P. B., Yu, Q., Zhao, N., Yin, C., Zhao, C., et al. (2018). Shrub type dominates the vertical distribution of leaf C : N : P stoichiometry across an extensive altitudinal gradient. Biogeosciences 15, 2033–2053.
Zhao, W., Liu, X., Jin, M., Zhang, X., Che, Z., Jing, W., et al. (2016). Ecological stoichiometric characteristics of carbon, nitrogen and phosphorus in leaf-litter-soil system of Picea crassifolia forest in the Qilian Mountains. Acta Pedol. Sin. 53, 477–489. doi: 10.11766/trxb201501080511
Zhou, Z., Guo, C., and Meng, H. (2013). Temperature sensitivity and basal rate of soil respiration and their determinants in temperate forests of North China. PLoS One 8:e81793. doi: 10.1371/journal.pone.0081793
Zhu, D., Hui, D., Wang, M., Yang, Q., and Yu, S. (2020). Light and competition alter leaf stoichiometry of introduced species and native mangrove species. Sci. Total Environ. 738:140301. doi: 10.1016/j.scitotenv.2020.140301
Keywords: Mountainous regions, plant growth, plant adaptation, plant traits, Potentilla
Citation: Qin Y, Liu W, Zhang X, Adamowski JF and Biswas A (2022) Leaf Stoichiometry of Potentilla fruticosa Across Elevations in China’s Qilian Mountains. Front. Plant Sci. 13:814059. doi: 10.3389/fpls.2022.814059
Received: 19 November 2021; Accepted: 10 January 2022;
Published: 24 February 2022.
Edited by:
Shiliang Liu, Beijing Normal University, ChinaReviewed by:
Hao Shen, Beijing Normal University, ChinaVladimir Onipchenko, Lomonosov Moscow State University, Russia
Zhen Ma, Northwest Institute of Plateau Biology (CAS), China
Gang Fu, Institute of Geographic Sciences and Natural Resources Research (CAS), China
Copyright © 2022 Qin, Liu, Zhang, Adamowski and Biswas. This is an open-access article distributed under the terms of the Creative Commons Attribution License (CC BY). The use, distribution or reproduction in other forums is permitted, provided the original author(s) and the copyright owner(s) are credited and that the original publication in this journal is cited, in accordance with accepted academic practice. No use, distribution or reproduction is permitted which does not comply with these terms.
*Correspondence: Wei Liu, d2VpbGl1QGx6Yi5hYy5jbg==