- 1State Key Lab of Desert and Oasis Ecology, Xinjiang Institute of Ecology and Geography, Chinese Academy of Sciences, Urumqi, China
- 2Fukang National Station of Observation and Research for Desert Ecosystem, Xinjiang, China
- 3University of Chinese Academy of Sciences, Beijing, China
- 4Xinjiang Institute of Ecology and Geography, Chinese Academy of Sciences, Urumqi, China
Water availability could alter multiple ecophysiological processes such as water use strategy, photosynthesis, and respiration, thereby modifying plant water use and carbon gain. However, a lack of field observations hinders our understanding of how water availability affects stem photosynthesis at both organ and plant levels of desert shrubs. In this study, we measured gas exchange and oxygen stable isotopes to quantify water sources, stem recycling photosynthesis, and whole-plant carbon balance in two coexisting Haloxylon species (Haloxylon ammodendron and Haloxylon persicum) at different groundwater depths in the Gurbantonggut Desert. The overall aim of the study was to analyze and quantify the important role of stem recycling photosynthesis for desert shrubs (Haloxylon species) under different groundwater depths. The results showed that (1) regardless of changes in groundwater depth, H. ammodendron consistently used groundwater and H. persicum used deep soil water as their main water source, with greater than 75% of xylem water being derived from groundwater and deep soil water for the two species, respectively; (2) stem recycling photosynthesis refixed 72–81% of the stem dark respiration, and its contribution to whole-plant carbon assimilation was 10–21% for the two species; and (3) deepened groundwater increased stem water use efficiency and its contribution to whole-plant carbon assimilation in H. persicum but not in H. ammodendron. Our study provided observational evidence that deepened groundwater depth induced H. persicum to increase stem recycling photosynthetic capacity and a greater contribution to whole-plant carbon assimilation, but this did not occur on H. ammodendron. Our study indicates that stem recycling photosynthesis may play an important role in the survival of desert shrubs in drought conditions.
Introduction
Leaves are widely accepted to be the primary organ where photosynthetic carbon assimilation occurs in plants (White et al., 2016). However, there is mounting evidence challenging this paradigm because stems can also assimilate carbon, providing an alternative and significant carbon source in plants (Saveyn et al., 2010; Bloemen et al., 2013a; Cernusak and Cheesman, 2015; Henry et al., 2020). Stem photosynthesis has been reported in a wide variety of tree species, with instantaneous rates ranging from 0.5 to 9 μmol m–2 s–1, and although mostly this is refixation of respiratory CO2, stems rarely achieve net positive assimilation (Nilsen, 1995; Pfanz et al., 2002; Ávila et al., 2014; Vandegehuchte et al., 2015). The CO2 fixed by stem photosynthesis is derived from either stems themselves through stem respiration in living cells or belowground root respiration, which subsequently transports upward via the transpiration stream (Bloemen et al., 2013b). Accumulating evidence has suggested that stem photosynthesis is an additional mechanism of carbon sequestration across biomes (Ávila et al., 2014; Vandegehuchte et al., 2015; De Roo et al., 2020).
With episodes of drought events increasing across most of the world (IPCC, 2019), photosynthetic stems may become an advantage for maintaining positive carbon balance at the whole-plant level, such as high water and carbon use efficiency. For example, up to 97% of the CO2 released from young branches of birch (Betula pendula Roth.) was refixed via stem photosynthesis (Wittmann et al., 2006). Similarly, young Eucalyptus globules seedlings have an increased capacity to refix internally respired CO2 (up to 96%) (Eyles et al., 2009). Cernusak and Marshall (2000) demonstrated that the water use efficiency of gross photosynthesis in Western white pine was 50 times higher in the bark than in the leaves. Additionally, stem photosynthesis may also help in the maintenance of hydraulic functioning, too (Sevanto, 2014; Ávila and Tezara, 2018). A recent study showed that stem photosynthesis can also drive bark water uptake to refill embolized vessels in excised Salix matsudana branches that are submerged in deionized water (Liu et al., 2019). Thus, it can be seen that stem photosynthesis plays an important role during drought stress and may become important for plant survival when leaf photosynthesis and phloem transport are limited (Cernusak and Cheesman, 2015; Wittmann and Pfanz, 2018; Hubeau et al., 2019; De Roo et al., 2020; Henry et al., 2020).
The Gurbantonggut Desert is the second largest desert in northwest China. The woody layer is dominated by Haloxylon ammodendron and Haloxylon persicum Bunge ex Boiss, which are phreatophytes and respond non-linearly to growing season precipitation (Dai et al., 2015). Both species of Haloxylon have a lower photosynthetic active area, as twigs replace leaves to perform photosynthetic functions (Xu et al., 2007; Zou et al., 2010). Most stems are lignified from twigs and have switched to twig-stem CO2 assimilation in drought stress after twigs senesce or abscission (Smith et al., 1996). As a result, stem photosynthesis may be an important, though often overlooked, component of plant carbon balance (Steppe et al., 2015; Vandegehuchte et al., 2015; Bloemen et al., 2016; Tarvainen et al., 2018; De Roo et al., 2020). Despite recent interest in stem photosynthesis and its effect on hydraulic integrity (Schmitz et al., 2012; Cernusak and Cheesman, 2015; Tarvainen et al., 2018; Liu et al., 2019; De Roo et al., 2020), less is known about how photosynthetic stems respond to drought in desert shrubby species.
This study aims to fill this knowledge gap by characterizing the stem carbon dynamics in Haloxylon species growing under two different groundwater depths. We measured two Haloxylon species native to the Gurbantonggut Desert in situ. Across two groundwater depths, stem gas exchange, environmental factors, and plant water sources were monitored with the goal of (1) assessing plant water use strategy; (2) comparing the differences in carbon exchange dynamics and water use efficiency of stems; (3) quantifying refixation of carbon released from dark respiration and the contribution of stem recycling photosynthesis to whole-plant carbon assimilation. We hypothesized that deeper groundwater depth will increase the contribution of stem assimilation at the whole plant level, as well as increase the ratio of stem area to leaf area or decrease leaf photosynthetic capacity. We hope to achieve a deep understanding of the impact of groundwater depth changes on stem photosynthesis and whole-tree carbon balance in desert ecosystems, resulting in better predictions under projected climatic change scenarios.
Materials and Methods
Study Site
This study was carried out near the Fukang Station of Desert Ecology and the southern periphery of the Gurbantonggut Desert in Central Asia. This region has a continental arid temperate climate, with a hot, dry summer and a cold winter. The air temperature ranges from a minimum of −42.2°C in winter to a maximum of 44.2°C in summer, and the annual mean temperature is 6.6°C. Annual mean precipitation is 163 mm, around 75% of which falls during the growing season (May–October). Precipitation prior to the growing season (November–April) is mostly in the form of snow, which covers the soil for most of this period and melts in April (Zhou et al., 2012). The soil type is desert solonetz at 0–100 cm depth, with eolian sandy soil on top. The groundwater depth ranges from 3 m in the Fukang Station of Desert Ecology to 16 m in the southern periphery of the Gurbantonggut Desert.
Two similar shrub-dominated desert communities were selected. Both sites are dominated by deep-rooted phreatophyte shrubs that depend primarily on groundwater for survival (Xu et al., 2007; Dai et al., 2015). The two sites were 13.5 km apart and located at the southern periphery of the Gurbantonggut desert, <7.5 km and <21.0 km away from Fukang Desert Station, and are dominated by H. ammodendron and H. persicum. The groundwater depth is 3.43 ± 0.02 m and 12.29 ± 0.19 m at these two sites. Throughout this study, we refer to the site with a groundwater depth of 3.43 m as a shallow groundwater site, and the site with a groundwater depth of 12.29 m as a deeper groundwater site (Figure 1).
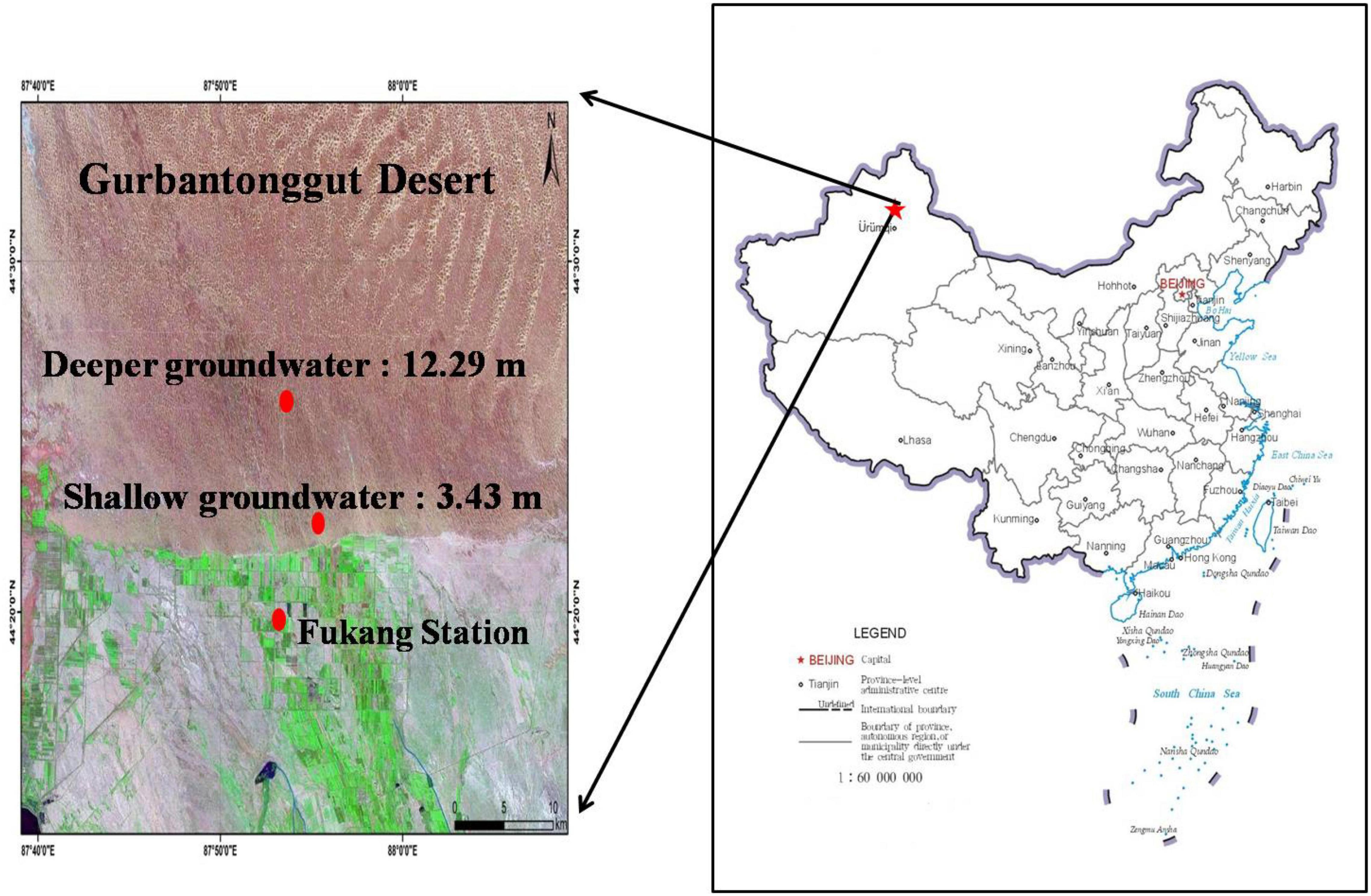
Figure 1. Location of the two experimental study sites. The shallow groundwater site has a groundwater depth of 3.43 m, and the deeper groundwater site has a groundwater depth of 12.29 m.
Leaf and Stem Recycling Photosynthetic Measurements
All photosynthetic measurements were carried out on clear days during July–August 2020. The photosynthetic light-response curve (09:00–14:00) and diurnal variation in instantaneous gas exchange rates (08:00–18:00) of stems were measured using one portable open gas exchange system (Li-6400XT, Li-Cor Inc., Lincoln, NE, United States) on consecutive campaigns of 2–3 days. For stem photosynthetic light-response curves, photosynthetic photon flux density (PPFD) was supplied by a 15 × 7 cm stem chamber with a red-blue light source (P-Chamber, MilletHill Biotech Co. Ltd., Shanghai, China) (Chang et al., 2020) and was reduced in a stepwise manner at 10 PPFD levels (2,000, 1,600, 1,200, 800, 400, 200, 150, 100, 50, and 0 μmol m–2 s–1), and the other conditions in the chamber were set to 400 ppm and 1.5 kPa for CO2 and atmospheric vapor pressure deficit (VPD), respectively. At each site, three stems with a diameter of 8–10 mm were selected for measurement in light and dark conditions. Each stem was allowed 6–8 min to equilibrate at each PPFD, and before measurements were recorded. Stem recycling photosynthetic light-response curve parameters were estimated using a modified rectangular hyperbola model (Equation 1):
where Pg is the gross photosynthetic rate of the stem (including dark respiration), I is the PPFD, Rd is the daytime dark respiration rate, α is the initial slope of the photosynthetic light-response curve where PPFD ≈ 0 μmol m–2 s–1, and β and γ are coefficients that are independent of I and were obtained by curve fitting (Ye and Yu, 2008).
The diurnal photosynthetic patterns of leaves and stems were measured simultaneously for each species and at each groundwater depth. Each measurement period of gas exchange lasted for approximately 10 h (between 08:00 and 18:00 h), and measurements were taken approximately every 2 h. A smart quantum sensor (MQ-500, Apogee, United States) was used to measure PPFDi at each measurement time point, and this value was manually entered into the Li-6400XT and P-Chamber to generate the corresponding irradiance. For the stem recycling photosynthetic rate (Pstem), positive net assimilation is, however, rarely reached, in most studies. Stem recycling photosynthesis was therefore assumed to be equal to the difference between the gas exchange rate under light (Rl) and dark (Rd) (Equation 2; Wittmann et al., 2006):
Additionally, as stem recycling photosynthesis refixes respiratory losses from the stem, refixation was estimated using Rd and Rl as shown in the following equation (Cernusak and Marshall, 2000):
To ensure the accuracy of stem recycling photosynthetic rate measurements, we used another method for cross-validation. A custom-made transparent polycarbonate chamber (15 cm × 7 cm × 7 cm) was attached to the stem, and CO2 and H2O fluxes inside the chamber were measured using a portable, open gas exchange system (Li-840A, Li-Cor Inc., Lincoln, NE, United States). Measurements using the transparent chamber directly obtained the net gas exchange rate. To estimate the dark respiration rate, we covered the chamber with an opaque canvas cover, allowing stem recycling photosynthetic rate to be calculated as the difference between measurements in the light and the dark (Supplementary Figure 1). The detailed procedures are described by Huang and Li (2015).
Determining Water Use Strategies of Two Species
Oxygen stable isotope (δ18O) measurements were used to determine the water use strategy of both Haloxylon species at different groundwater depths. For each species at each site, four healthy plants with average canopy size were randomly selected. Plant xylem and soil samples were collected simultaneously on three consecutive clear days. Only sunlit twigs from each plant were sampled. Excised leaves were quickly decorticated and placed in screw-cap glass vials that were sealed using Parafilm and stored in a freezer until subsequent extraction of the xylem water for δ18O analysis. Three soil cores were collected next to the sampled plants. Soil samples were collected at 20 cm intervals from the topsoil to the groundwater table. Four replicate soil samples at each layer were sampled and sealed in glass vials and frozen for soil water extraction and δ18O analysis.
Xylem water and soil water were extracted using cryogenic vacuum distillation, and the extracted water was stored in sealed glass vials at 2°C. The oxygen isotopic composition of the water was determined using a liquid water isotope analyzer (LWIA, DLT-100, Los Gatos Research Inc., Mountain View, CA, United States). Two methods were used to determine the water use of the two species. One was the direct inference approach, which compares δ18O composition between groundwater, the soil water profile, and xylem water by plotting them together. The other method was the IsoSource model, which determines the possible range of different water sources used by plants (Phillips and Gregg, 2003). Based on the similarities in the δ18O values of soil water, the soil profile was divided into two sections, and the following two potential water sources were finally identified using a post-integrated method: (1) deep soil layer, which was the intermediated section between the upper soil layer (0–1 m) and the near groundwater layer; and (2) groundwater layer (0–1.5 m above the groundwater depth), which could be identified by a significant increase in soil water content (SWC) and has a similar isotopic composition to that of groundwater [For details on the application of the IsoSource model, refer to Liu et al. (2016) and Wu et al. (2019)].
Stem Water Use Efficiency and Environmental Measurements
Stem water use efficiency was calculated using the ratio of stem recycling photosynthesis rate to transpiration rate (μmol CO2 m–2 s–1/mmol H2O m–2 s–1). Air temperature (Tair) (oC) and relative humidity (RH) (%) were measured at two sites every 10 s and averaged at 30 min intervals using a portable weather station (S-THB-M008, Onset, Bourne, MA, United States). Values of Tair and RH were used to calculate the atmospheric VPD. A TDR 150 probe was used to measure soil temperature and volumetric water content at 5 and 10 cm soil depths (FieldScout TDR 150, spectrum Technologies, Aurora, IL, United States). To quantify the contribution of stem recycling photosynthesis to the entire plant, plants were destructively harvested at the end of all measurements and measured for total stem surface area and total leaf area using Vernier calipers and a leaf area meter (Li-3100, Li-Cor Inc., Lincoln, NE, United States), respectively. In this study, we used the following equation to calculate the contribution of stem photosynthesis to the entire plant:
where Pstem is stem recycling photosynthesis rate, Sstem is total stem surface area, Pleaf is net leaf photosynthesis rate, and Sleaf is total leaf surface area.
Statistical Analyses
All descriptive statistics and statistical analyses (including linear regression, one-way ANOVA, paired t-test, independent samples t-test) were conducted using the R software (version 3.3.1) (R Core Team, 2020). Given the small sample size (n = 3–36), significance levels of 0.05 and 0.10 were used to represent strong and moderate effects, respectively. All data visualization was performed using the Origin 2017 software package (OriginLab Corporation Northampton, MA, United States).
Results
Meteorological and Groundwater Conditions in the Two Communities
The variation in the major meteorological conditions and groundwater depths in two sites during the growing season of 2020 are presented in Figure 2. Monthly average daily Tair and PPFD followed similar patterns at the two sites (P > 0.05) (Figures 2A,B), with maximum daily temperatures ranging from 27.5 to 29.0° Cand PPFD ranging from 567 to 645 μmol m–2 s–1. Deeper groundwater depth resulted in lower soil water content, especially in 1.8–3.0 m soil layer, with values of 12.47 and 3.94% at two sites (Figure 2C). There was no significant difference in VPD between the two sites, with an average value of 1.67 kPa. In contrast, groundwater depths varied significantly between the two sites (P < 0.01) (Figure 2D), with an average groundwater depth of 3.43 ± 0.02 and 12.29 ± 0.02 for the shallow and deeper groundwater sites, respectively.
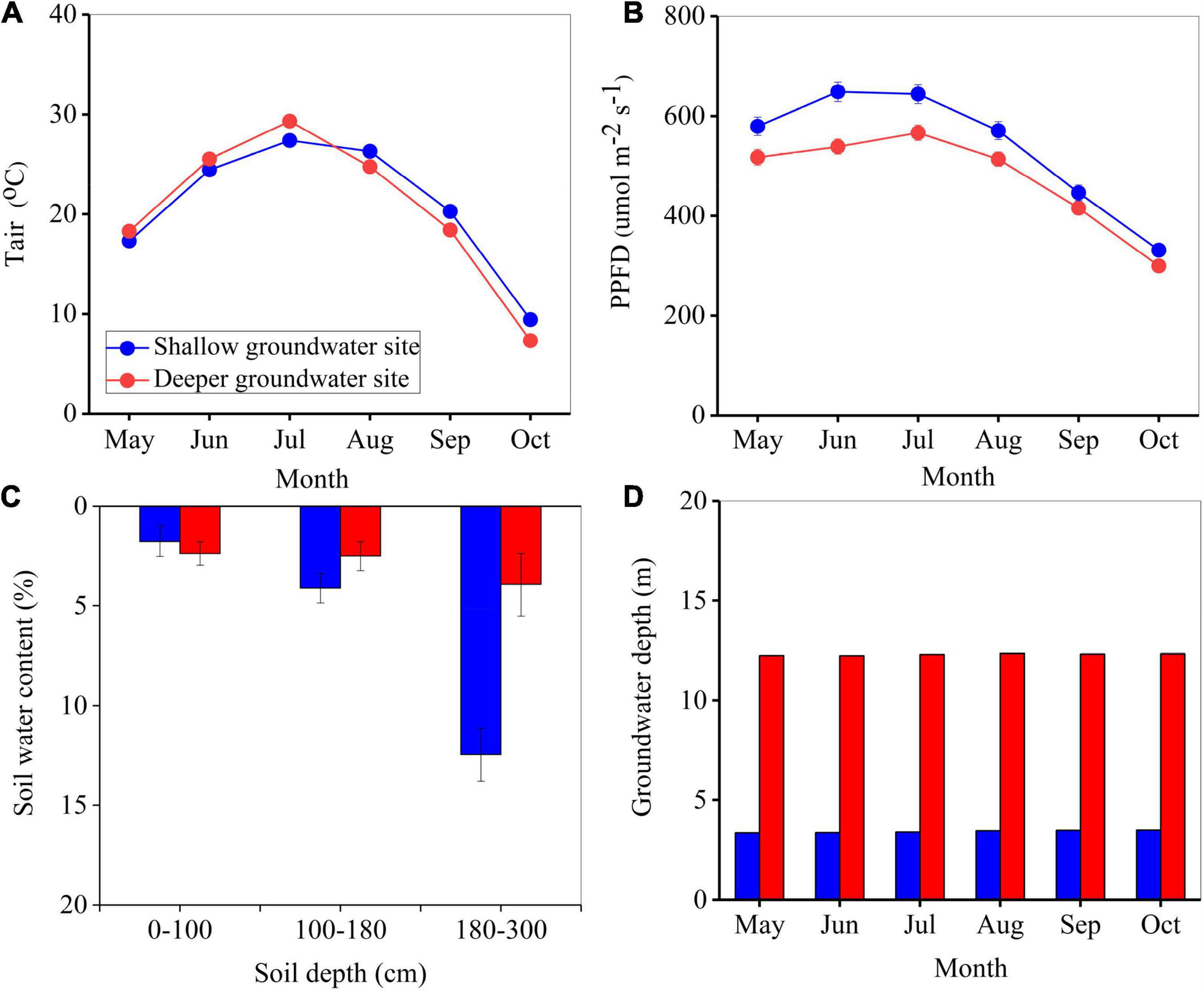
Figure 2. Variation in air temperature (Tair) (A), photosynthetic photon flux density (PPFD) (B), soil water content (C), and groundwater depth (D) during the growing season at the two study sites during 2020.
Water Use Strategy of Two Haloxylon Species
Variation in the water use strategies of the two Haloxylon species in two sites was used to determine if they may explain variability in stem photosynthesis. The values of δ18O of xylem, each soil layer, and groundwater are shown in Figure 3 and Supplementary Figure 2. Regardless of changes in groundwater depth, H. ammodendron used similar water sources; the value of δ18O of xylem water was much closer to the value of δ18O of groundwater (Figures 3A,B): greater than 75% of the xylem water was derived from groundwater (Figures 3C,D). Similarly, H. persicum used deep soil water as its main water source; greater than 78% of the xylem water was derived from deep soil layers (Figure 3).
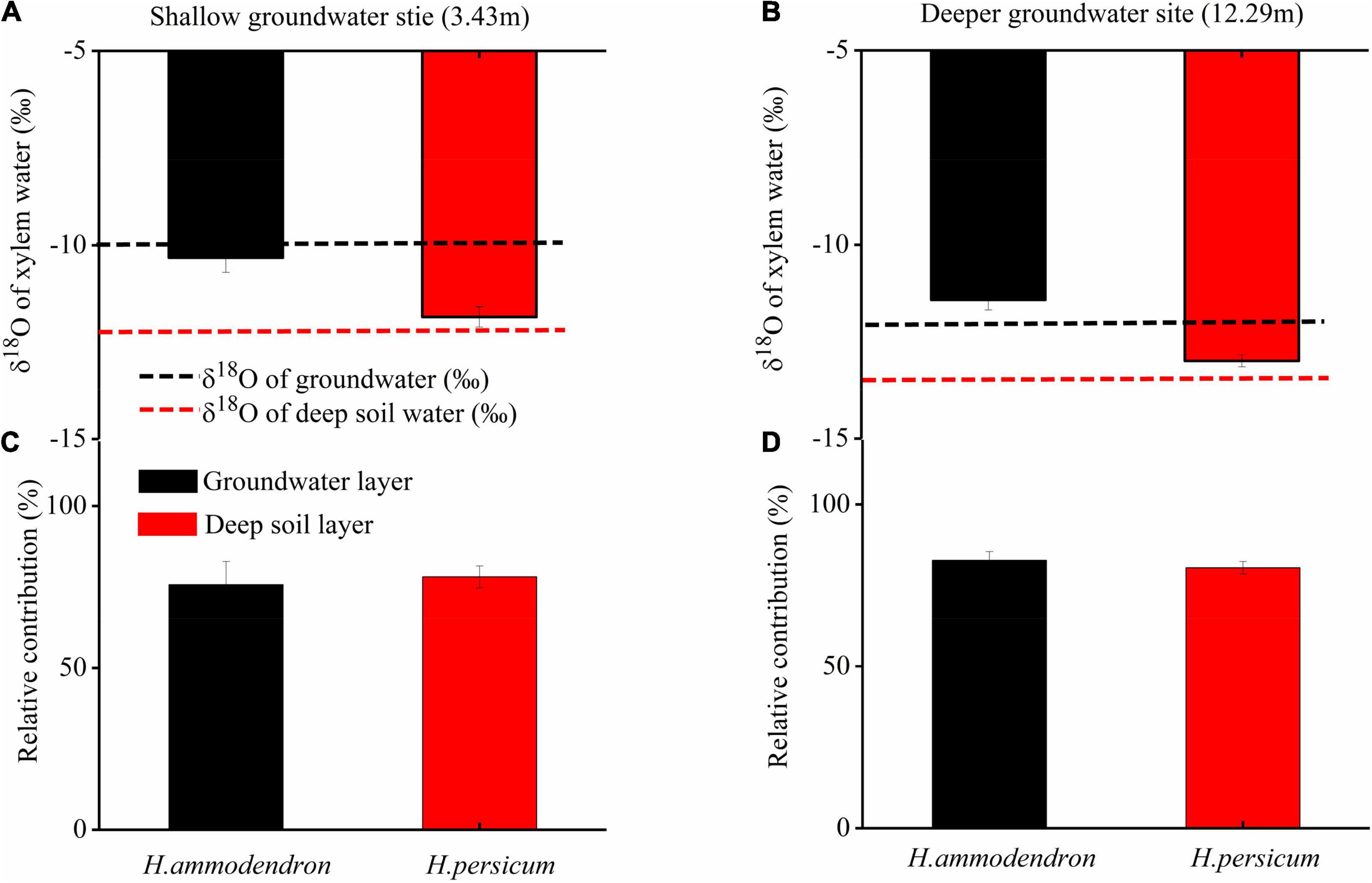
Figure 3. Comparison of the oxygen stable isotope ratio (δ18O) of xylem water and deep soil water and groundwater (A,B), and the relative contribution of different water sources (C,D) for the two Haloxylon species at two sites.
Photosynthetic Response to Light of Stems
Stems of both Haloxylon species became light-saturated at approximately 1,000 μmol m–2 s–1 PPFD (Figures 4A,B and Table 1). Both species had a lower Rd and maximum Pmax at deeper groundwater sites, with the values of −1.80 and −0.31 μmol m–2 s–1 for H. ammodendron and −1.29 and −0.32 μmol m–2 s–1 for H. persicum. In contrast, the two species had a higher initial slope (α) at a deeper groundwater site (Figures 4A,B and Table 1).
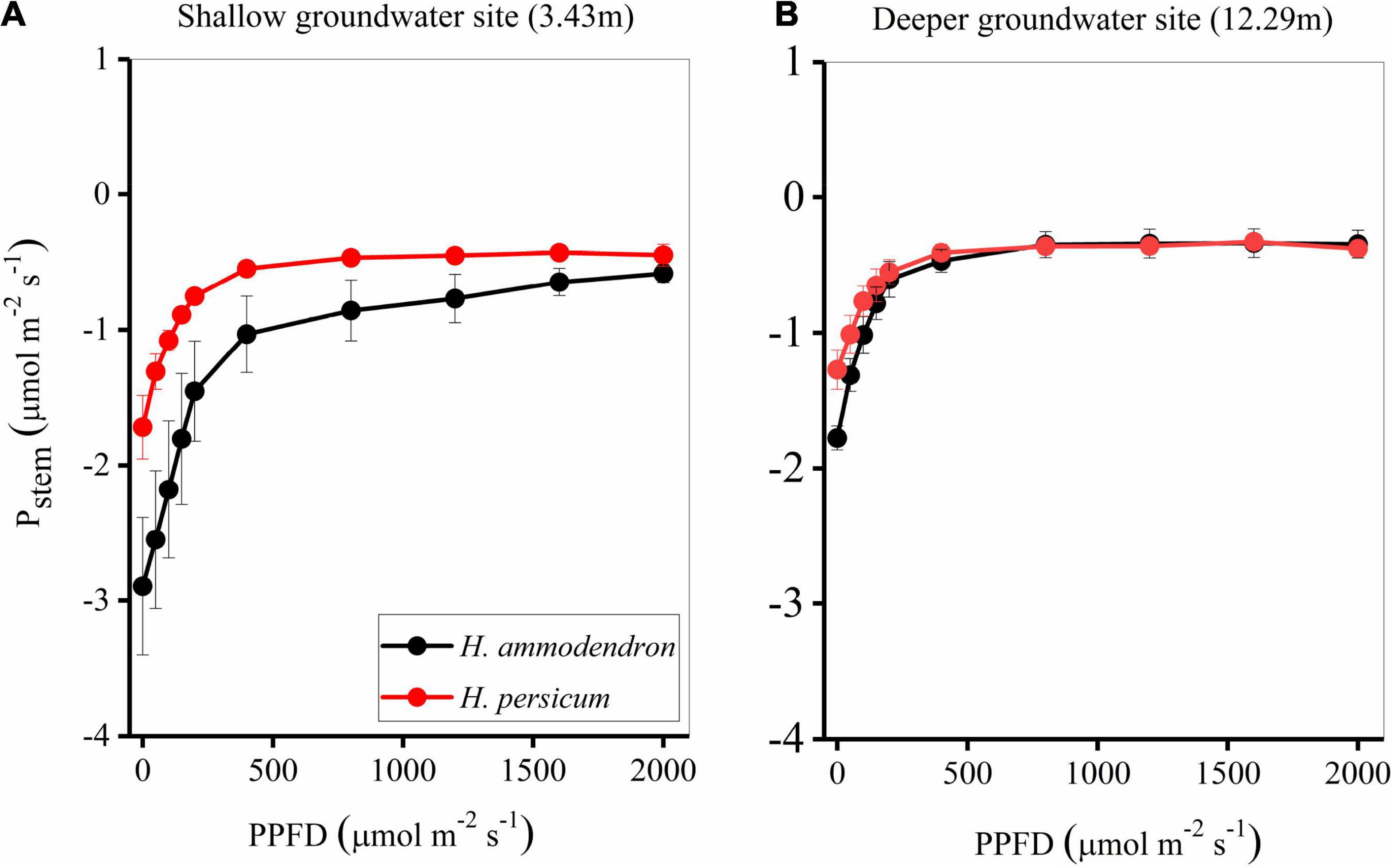
Figure 4. Photosynthetic light responses as measured in situ for stems (A,B) of the two Haloxylon species at two sites. Values are means of three replicates ± SE of the mean.
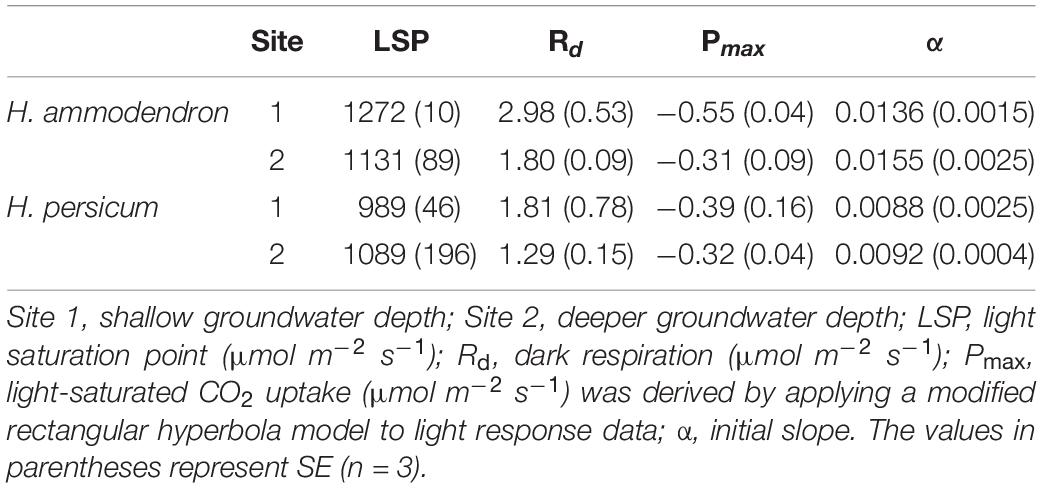
Table 1. Stem light response parameters for two Haloxylon species growing under different groundwater depths.
Diurnal Variations in Stem Recycling Photosynthesis and Water Use Efficiency
Throughout a diurnal time course, at a deeper groundwater site, the stem recycling photosynthetic rate of H. persicum was increased, with the maximum Pstem recorded being 1.84 vs. 2.35 μmol m–2 s–1 at shallow and deeper groundwater sites, respectively (Figures 5A,B). However, the opposite pattern was observed in H. ammodendron, with the maximum Pstem recorded being 2.47 vs. 1.55 μmol m–2 s–1 at each of the sites (Figures 5A,B).
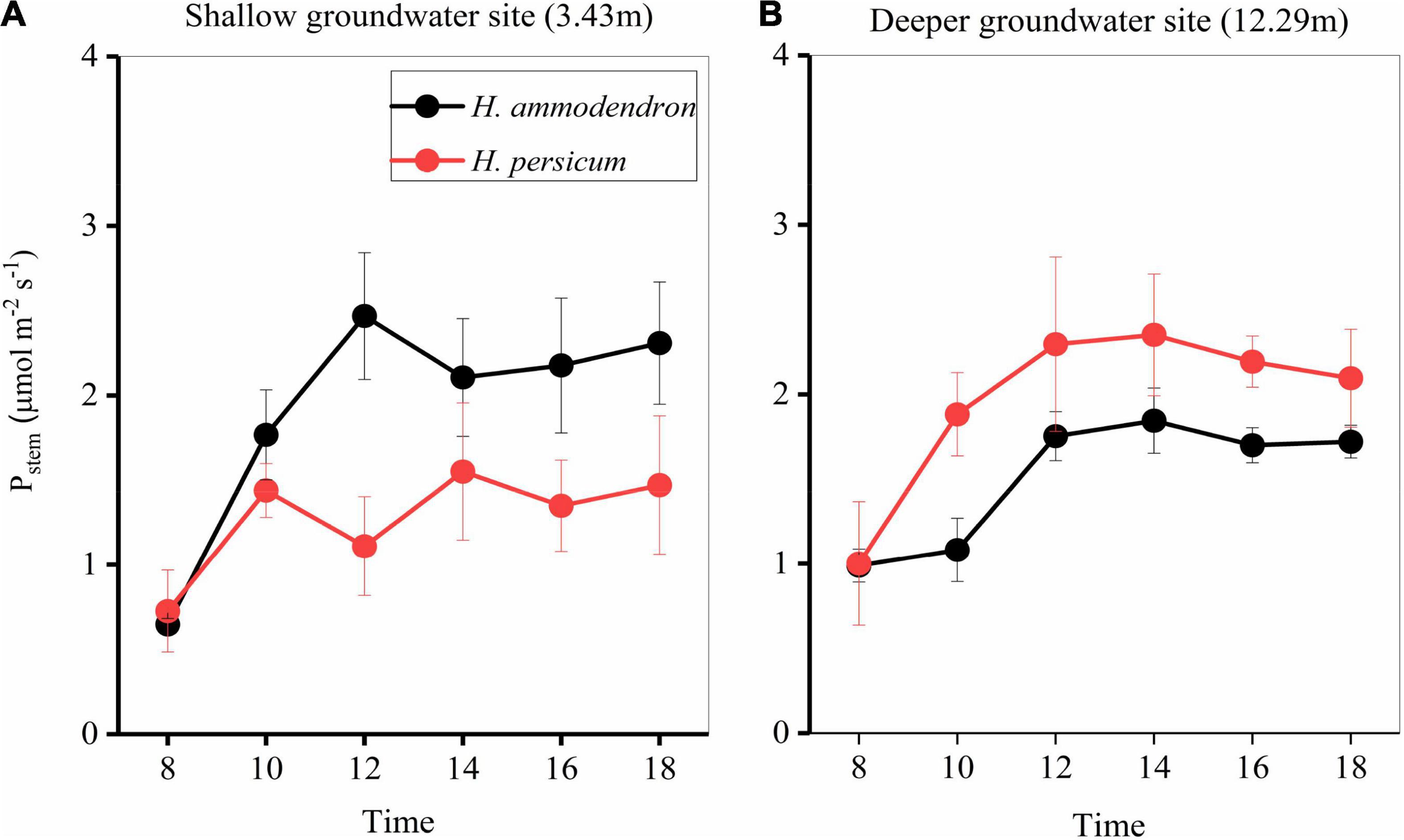
Figure 5. Average daily patterns of the stem (Pstem) (A,B) photosynthesis for two Haloxylon species at two sites. Error bars represent SE (n = 3).
On average, H. ammodendron had significantly lower values of Pstem (1.91 vs. 1.51 μmol m–2 s–1) and Rstem (2.67 vs. 2.04 μmol m–2 s–1) at the two sites (Figures 6A,B). The values of Pstem and Rstem of H. persicum increased up to 57% at the deeper groundwater site (P < 0.05) (Figures 6A,B). Furthermore, the amount of respired CO2 refixed by stem photosynthesis ranged from 72 to 81% in the two Haloxylon species at two sites (Figure 6C). Deeper groundwater depth resulted in higher stem water use efficiency of H. persicum, with the value of 7.90 vs. 22.61 μmol CO2 mmol H2O–1, but H. ammodendron remained unchanged 14.90 vs. 13.01 μmol CO2 mmol H2O–1 (Figure 6D).
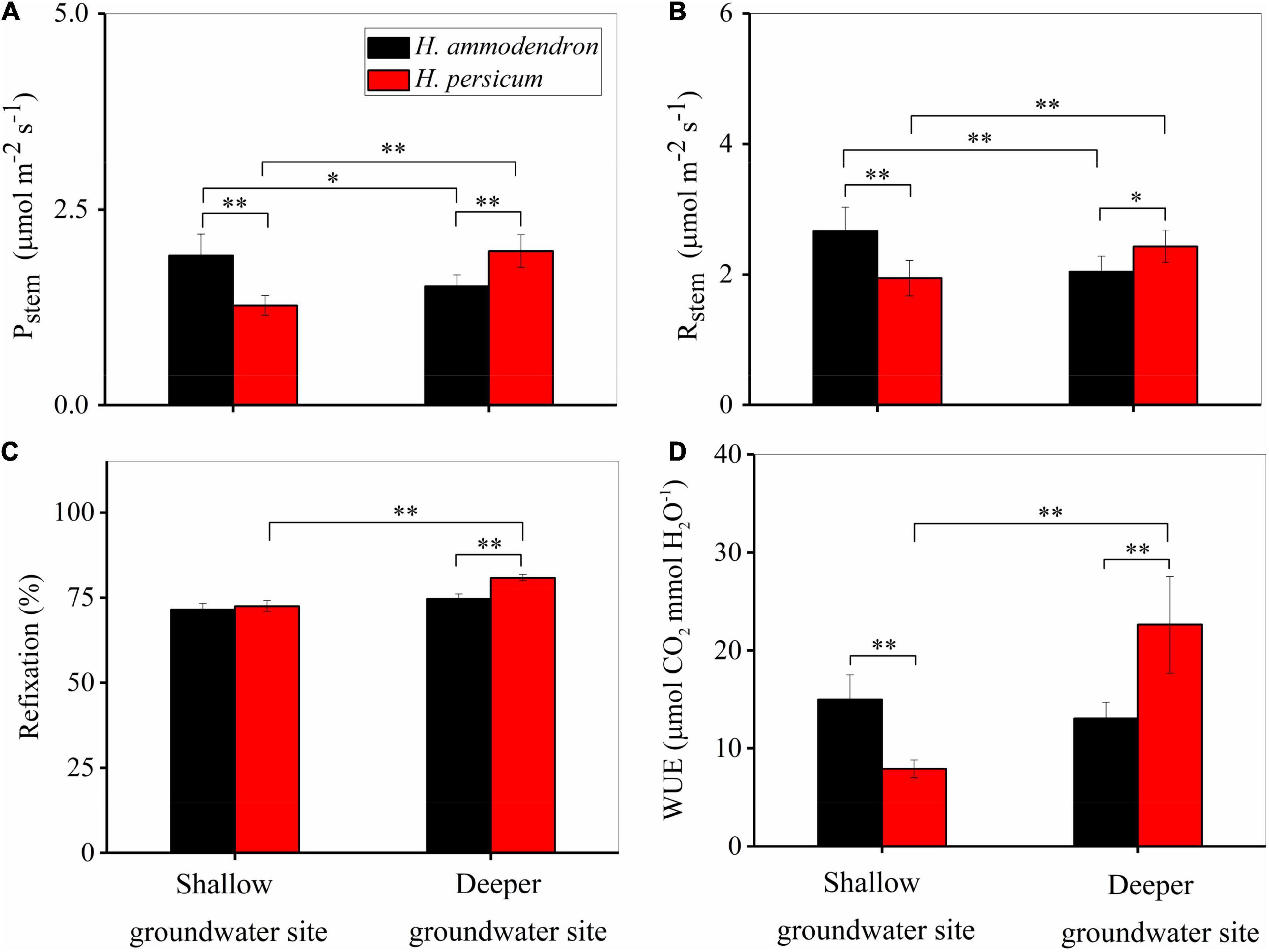
Figure 6. Comparison of daily average stem (Pstem) photosynthesis (A), stem dark respiration (Rstem) (B), stem refixation (C) (expressed as a percentage of Pstem and Rstem), and stem water use efficiency (D) for two Haloxylon species at two sites. Error bars represent SE (n = 18). *P < 0.1, **P < 0.05.
The Contribution of Stem Recycling Photosynthesis to Whole-Plant Carbon Assimilation
The ratio of stem area to leaf area (stem area/leaf area) of the two Haloxylon species at two sites was determined using destructive sampling (Figure 7A). The proportion of stem area in H. persicum was significantly higher than in H. ammodendron, but there was no difference within species between sites (Figure 7A). We estimated the contribution of stem recycling photosynthesis to whole-plant carbon assimilation to be at least 10% for H. ammodendron and to be as high as 21% for H. persicum at a deeper groundwater site (Figure 7B).
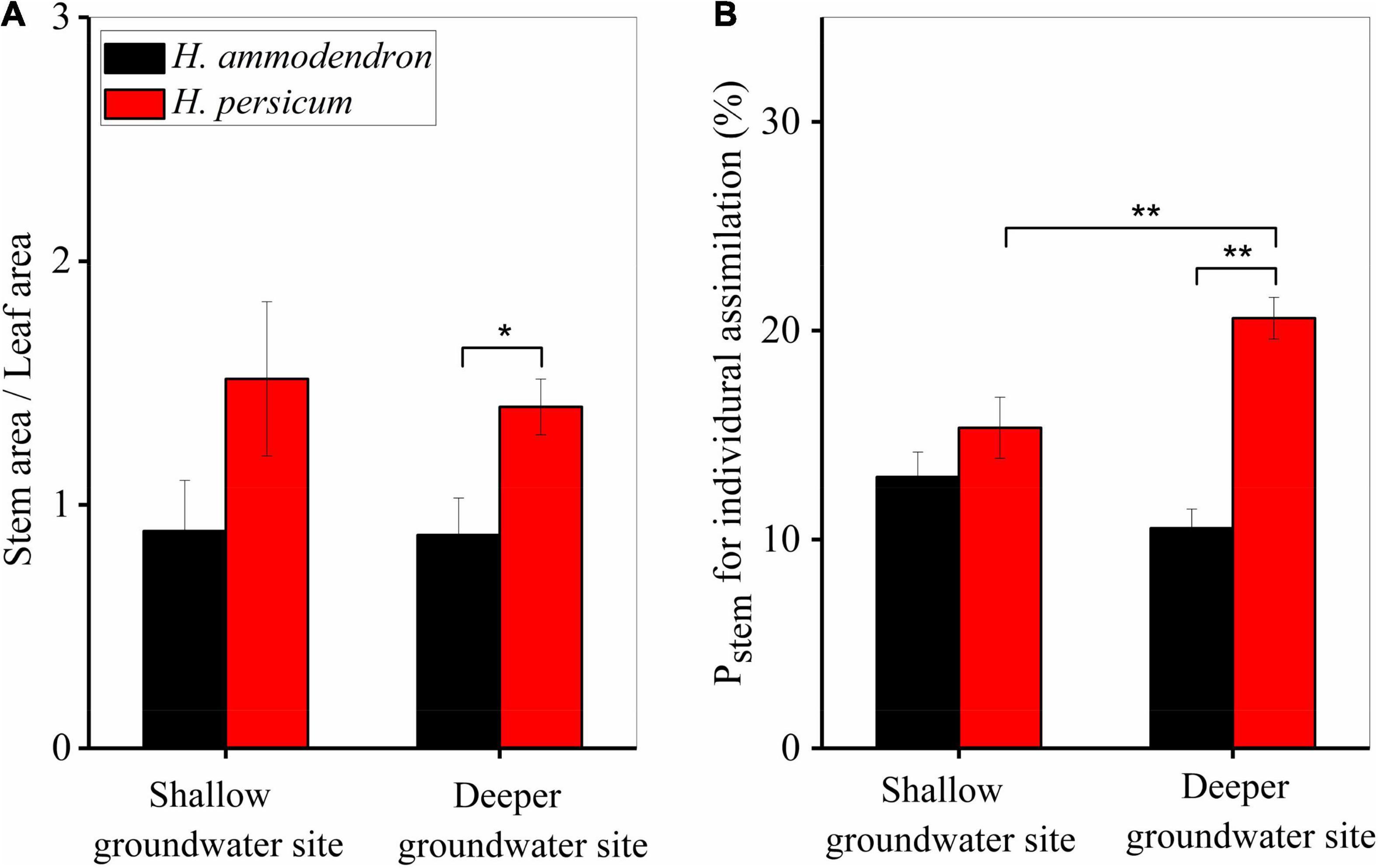
Figure 7. Variations in stem area per leaf area (A) and the contribution of stem photosynthesis to whole-plant carbon assimilation (B) for two Haloxylon species growing at two sites. Error bars represent SE (n = 3). *P < 0.1, **P < 0.05.
Discussion
Understanding the role of stem recycling photosynthesis in two Haloxylon species growing in habitats differing in groundwater depth is an important step toward improving predictions of carbon cycle dynamics under drought stress in arid and semiarid ecosystems (Ávila et al., 2014; Cernusak and Cheesman, 2015; Tarvainen et al., 2018; De Roo et al., 2020). In this study, we monitored the responses of stem recycling photosynthesis under an exceptionally wide range of groundwater depths from 3.43 to 12.29 m. We found that the deep soil water use strategy can induce the increase in stem recycling photosynthesis capacity of H. persicum when exposed to a deeper groundwater depth. We also found that stem recycling photosynthesis of H. ammodendron was shaped by Tair and SWC, and stem recycling photosynthesis of H. persicum showed a positive correlation with Pleaf (Figure 8).
Plant water use strategy is particularly important when there is a change in plant community structure that might influence the accessibility of shallower vs. deeper soil water pools or groundwater (Campos et al., 2013; Scott et al., 2014). In this study, the isotopic analysis showed that variation in groundwater depth did not affect the water use strategies of either Haloxylon species (Figure 3). H. ammodendron consistently used a greater fraction of groundwater as its main water source, while the largest contributor for H. persicum was the deeper soil water pool. Previous root-system investigation experiments characterized both of these species as being deep-rooted plants (Zou et al., 2010). The different water use strategies of Haloxylon species may be related to the microtopography where they are distributed. H. ammodendron mainly grows at the bottom of sand dunes, and its roots could penetrate up to 10 m below the surface (Xu et al., 2007). This deep rooting depth enables it to access groundwater (Lv et al., 2013; Dai et al., 2015; Wu et al., 2019). Unlike H. ammodendron, H. persicum usually grows on dune crests, placing it farther from the groundwater table. Excessive energy costs might prevent its roots from accessing groundwater (Tiemuerbieke et al., 2018). Therefore, H. persicum depends on deep soil water, which is derived from infiltration of precipitation and groundwater fluctuations (Figure 3).
Even at places where the groundwater was deeper, neither Haloxylon species changed its water use strategy, though photosynthesis of stems did respond to groundwater depth (Figures 4, 5). Stems had significantly lower light-saturated PPFD and maximum stem photosynthetic rates. The observed value of light-saturated stem photosynthesis corresponds to measurements reported for stems of most other woody species, which range from 200 to 400 μmol m–2 s–1 (Wittmann and Pfanz, 2008; Eyles et al., 2009). The maximum stem recycling photosynthetic rate was 1.58 ± 0.3 μmol m–2 s–1, which is consistent with reports for other species [0.1–9 μmol m–2 s–1, reviewed by Ávila et al. (2014)]. Surprisingly, deeper groundwater sites had a strong positive effect on stem recycling photosynthesis of H. persicum, but this did not occur on H. ammodendron (Figures 5–7). This could be attributed to the fact that the response of H. ammodendron to water changes mainly relies on morphological regulation to maintain the stability of physiological activities (Xu et al., 2007; Zou et al., 2010). The results partially support our initial hypothesis that stem photosynthesis would contribute more to the organ and whole-plant carbon balance of two species under deeper groundwater depths.
Stem recycling photosynthesis does not depend on the uptake of atmospheric CO2 but can instead rely on internal dark respiration (Cernusak and Marshall, 2000). Our results also suggested that stem recycling photosynthesis refixes CO2 released during stem dark respiration, resulting in 72–81% of respired CO2 being refixed (Figure 6). The observed refixation value corresponds to observations reported for stems recycling photosynthesis of most other woody species, which suggest that this recycling mechanism can offset between 7 and 98% of stem dark respiration (Wittmann et al., 2006; Ávila et al., 2014; De Roo et al., 2020). Refixation has two advantages over leaf photosynthesis: there is very less associated water loss and refixing respired carbon increases carbon use efficiency, which increases stand-level productivity (Vandegehuchte et al., 2015). Stems always have higher water use efficiency (Cernusak and Marshall, 2000); we found that two Haloxylon species’ water use efficiency of stems was 3–5 times higher than that of the leaves (Supplementary Table 1). This may be due to the fact that bark lenticels are far less than leaf stomata. Additionally, stem recycling photosynthesis can be accomplished with stomata closed as it can refix internally respired CO2 (Wittmann et al., 2006; Cernusak and Hutley, 2011).
Although stem recycling photosynthesis has been widely studied for its effects on tree carbon cycling (Nilsen, 1995; Pfanz et al., 2002; Ávila et al., 2014; De Roo et al., 2020), it has surprisingly been neglected on its contribution to the whole-plant carbon assimilation. We estimated that stem recycling photosynthesis contributed 10–21% of the total whole-plant carbon assimilation for the two Haloxylon species (Figure 7). Previous studies have shown that stem photosynthesis accounted for 10–15% of whole-tree carbon input in Populus tremula during the summer (Kharouk et al., 1995; Pfanz et al., 2002). In mature Eucalyptus miniata trees, 10% of branch diameter growth was fueled by stem photosynthesis (Cernusak and Hutley, 2011). Altogether, the results indicated that two Haloxylon species, especially H. persicum, exhibited higher stem recycling photosynthesis, which plays an important role in desert plant carbon balance (Cernusak and Marshall, 2000; Eyles et al., 2009; Liu et al., 2019).
Conclusion
Our study quantified the relative importance of changes in stem recycling photosynthetic capacity across different groundwater depths. Different water use strategies employed by the two Haloxylon species drove H. persicum, but not H. ammodendron, to increase its photosynthetic capacity when groundwater was deeper. Furthermore, deeper groundwater also resulted in a higher contribution of stem recycling photosynthesis to whole-plant carbon assimilation and stem water use efficiency, indicating that water use and carbon gain become more efficient under drought conditions. Ecophysiological studies on stem photosynthesis of woody plants are still very much under-explored. Future studies on different desert woody plants should also include characterizing how stem photosynthesis may impact the resistance and resilience of those species to drought. Accurate quantification of the capacity for refixation of respired carbon by stem photosynthesis and its contribution to the whole plant may reveal that the contribution of stem photosynthesis to the total carbon budget of desert ecosystems is significant.
Data Availability Statement
The raw data supporting the conclusions of this article will be made available by the authors, without undue reservation.
Author Contributions
RL, YW, CL, and YL planned and designed the research. RL, XF, and JM collected the data. RL and XF analyzed the data. RL, XF, and CL interpreted the results and wrote the manuscript. All authors contributed critically to the drafts.
Funding
This financial support was provided by the Western Light Program of the Chinese Academy of Sciences (Nos. 2020-XBQNXZ-013 and 2021-XBQNXZ-002), the National Natural Science Foundation of China (Nos. U1903301, 41771121, 41730638, and 31931731), and the Shanghai Cooperation and the Organization Science and Technology Partnership (No. 2020E01046).
Conflict of Interest
The authors declare that the research was conducted in the absence of any commercial or financial relationships that could be construed as a potential conflict of interest.
Publisher’s Note
All claims expressed in this article are solely those of the authors and do not necessarily represent those of their affiliated organizations, or those of the publisher, the editors and the reviewers. Any product that may be evaluated in this article, or claim that may be made by its manufacturer, is not guaranteed or endorsed by the publisher.
Acknowledgments
We thank all staff of the Fukang National Station of Observation and Research for Desert Ecosystem for their excellent field and laboratory assistance.
Supplementary Material
The Supplementary Material for this article can be found online at: https://www.frontiersin.org/articles/10.3389/fpls.2022.804786/full#supplementary-material
References
Ávila, E., Herrera, A., and Tezara, W. (2014). Contribution of stem CO2 fixation to whole-plant carbon balance in nonsucculent species. Photosynthetica 52, 3–15. doi: 10.1007/s11099-014-0004-2
Ávila, E., and Tezara, W. (2018). Water-use efficiency is higher in green stems than in leaves of a tropical tree species. Trees 32, 1547–1558. doi: 10.1007/s00468-018-1732-x
Bloemen, J., McGuire, M. A., Aubrey, D. P., Teskey, R. O., and Steppe, K. (2013a). Internal recycling of respired CO2 may be important for plant functioning under changing climate regimes. Plant Sign. Behav. 8:e27530. doi: 10.4161/psb.27530
Bloemen, J., Mcguire, M. A., Aubrey, D. P., Teskey, R. O., and Steppe, K. (2013b). Transport of root-respired CO2 via the transpiration stream affects aboveground carbon assimilation and CO2 efflux in trees. New Phytol. 197, 555–565. doi: 10.1111/j.1469-8137.2012.04366.x
Bloemen, J., Vergeynst, L. L., Overlaet-Michiels, L., and Steppe, K. (2016). How important is woody tissue photosynthesis in poplar during drought stress? Trees 30, 63–72.
Campos, G. E. P., Moran, M. S., Huete, A., Zhang, Y. G., Bresloff, C., Huxman, T. E., et al. (2013). Ecosystem resilience despite large-scale altered hydroclimatic conditions. Nature 494, 349–352.
Cernusak, L. A., and Cheesman, A. W. (2015). The benefits of recycling: How photosynthetic bark can increase drought tolerance. New Phytol. 208, 995–997.
Cernusak, L. A., and Hutley, L. B. (2011). Stable isotopes reveal the contribution of corticular photosynthesis to growth in branches of Eucalyptus miniata. Plant Physiol. 155, 515–523.
Cernusak, L. A., and Marshall, J. D. (2000). Photosynthetic refixation in branches of Western White Pine. Funct. Ecol. 14, 300–311.
Chang, T. G., Song, Q. F., Zhao, H. L., Chang, S., Xin, C., Qu, M., et al. (2020). An in situ approach to characterizing photosynthetic gas exchange of rice panicle. Plant Methods 16:92.
Dai, Y., Zheng, X. J., Tang, L. S., and Li, Y. (2015). Stable oxygen isotopes reveal distinct water use patterns of two Haloxylon species in the Gurbantunggut Desert. Plant Soil 389, 73–87.
De Roo, L., Salomon, R. L., and Steppe, K. (2020). Woody tissue photosynthesis reduces stem CO2 efflux by half and remains unaffected by drought stress in young Populus tremula trees. Plant Cell Environ. 43, 981–991.
Eyles, A., Pinkard, E. A., O’Grady, A. P., Worledge, D., and Warren, C. R. (2009). Role of corticular photosynthesis following defoliation in Eucalyptus globulus. Plant Cell Environ. 32, 1004–1014.
Henry, R. J., Furtado, A., and Rangan, P. (2020). Pathways of Photosynthesis in Non-leaf Tissues. Biology 9:438. doi: 10.3390/biology9120438
Huang, G., and Li, Y. (2015). Phenological transition dictates the seasonal dynamics of ecosystem carbon exchange in a desert steppe. J. Veg. Sci. 26, 337–347.
Hubeau, M., Mincke, J., Vanhove, C., Courtyn, J., Vandenberghe, S., and Steppe, K. (2019). Plant-PET to investigate phloem vulnerability to drought in Populus tremula under changing climate regimes. Tree Physiol. 39, 211–221. doi: 10.1093/treephys/tpy131
IPCC (2019). Climate Change and Land: An IPCC special report on climate change, desertification, land degradation, sustainable land management, food security, and greenhouse gas fluxes in terrestrial ecosystems. Geneva: IPCC
Kharouk, V. L., Middleton, E. M., Spencer, S. L., Rock, B. N., and Williams, D. L. (1995). Aspen bark photosynthesis and its significance to remote sensing and carbon budget estimates in the boreal ecosystem. Water Air Soil Pollut. 82, 483–497.
Liu, J., Gu, L., Yu, Y., Huang, P., Wu, Z., Zhang, Q., et al. (2019). Corticular photosynthesis drives bark water uptake to refill embolized vessels in dehydrated branches of Salix matsudana. Plant Cell Environ. 42, 2584–2596. doi: 10.1111/pce.13578
Liu, R., Cieraad, E., Li, Y., and Ma, J. (2016). Precipitation pattern determines the inter-annual variation of herbaceous layer and carbon fluxes in a phreatophyte-dominated desert ecosystem. Ecosystems 19, 601–614.
Lv, J. L., Zhang, X. M., Lv, C. Y., and Liu, G. J. (2013). A preliminary study on the water sources of Haloxylon ammodendron at the southern edge desert of Junggar Basin, China. J. Desert Res. 33, 110–117.
Nilsen, E. T. (1995). “Stem photosynthesis: extent, patterns, and role in plant carbon economy,” in Plant Stems: Physiology and Functional Morphology, ed. B. Gartner (San Diego: Academic Press), 223–240. doi: 10.1016/b978-012276460-8/50012-6
Pfanz, H., Aschan, G., Langenfeld-Heyser, R., Wittmann, C., and Loose, M. (2002). Ecology and ecophysiology of tree stems: corticular and wood photosynthesis. Naturwissenschaften 89, 147–162. doi: 10.1007/s00114-002-0309-z
Phillips, D. L., and Gregg, J. W. (2003). Source partitioning using stable isotopes: coping with too many sources. Oecologia 136, 261–269. doi: 10.1007/s00442-003-1218-3
R Core Team (2020). R: A Language and Environment for Statistical Computing. Vienna, Austria: R Foundation for Statistical Computing. Available online at: https://www.R-project.org/
Saveyn, A., Steppe, K., Ubierna, N., and Dawson, T. E. (2010). Woody tissue photosynthesis and its contribution to trunk growth and bud development in young plants. Plant Cell Environ. 33, 1949–1958. doi: 10.1111/j.1365-3040.2010.02197.x
Schmitz, N., Egerton, J. J. G., Lovelock, C. E., and Ball, M. C. (2012). Lightdependent maintenance of hydraulic function in mangrove branches: do xylary cholroplasts play a role in embolism repair? New Phytol. 195, 40–46.
Scott, R. L., Huxman, T. E., Barron-Gafford, G. A., Jenerette, G. D., Young, J. M., and Hamerlynck, E. P. (2014). When vegetation change alters ecosystem water availability. Glob. Chang. Biol. 20, 2198–2210. doi: 10.1111/gcb.12511
Smith, S. D., Monson, R. K., and Anderson, L. E. (1996). “Physiological Ecology of North American Desert Plants,” in Adaptations of Desert Organisms, ed. J. L. CloudsleyThompson (New York, NY: Springer).
Steppe, K., Sterck, F., and Deslauriers, A. (2015). Diel growth dynamics in tree stems: Linking anatomy and ecophysiology. Trends Plant Sci. 20, 1–9. doi: 10.1016/j.tplants.2015.03.015
Tarvainen, L., Wallin, G., Lim, H., Linder, S., Oren, R., Löfvenius, M. O., et al. (2018). Photosynthetic refixation varies along the stem and reduces CO2 efflux in mature boreal Pinus sylvestris trees. Tree Physiol. 38, 558–569. doi: 10.1093/treephys/tpx130
Tiemuerbieke, B., Min, X. J., Zang, Y. X., Xing, P., Ma, J. Y., and Sun, W. (2018). Water use patterns of co-occurring C3 and C4 shrubs in the Gurbantunggut desert in northwestern China. Sci. Total Environ. 634:341. doi: 10.1016/j.scitotenv.2018.03.307
Vandegehuchte, M. W., Bloemen, J., Vergeynst, L. L., and Steppe, K. (2015). Woody tissue photosynthesis in trees: Salve on the wounds of drought? New Phytol. 208, 998–1002. doi: 10.1111/nph.13599
White, A. C., Rogers, A., Rees, M., and Osborne, C. P. (2016). How can we make plants grow faster? A source–sink perspective on growth rate. J. Exp. Bot. 67, 31–45. doi: 10.1093/jxb/erv447
Wittmann, C., and Pfanz, H. (2008). Antitranspirant functions of stem periderms and their influence on corticular photosynthesis under drought stress. Trees 22, 187–196.
Wittmann, C., and Pfanz, H. (2018). More than just CO2-recycling: Corticular photosynthesis as a mechanism to reduce the risk of an energy crisis induced by low oxygen. New Phytol. 219, 551–564. doi: 10.1111/nph.15198
Wittmann, C., Pfanz, H., Loreto, F., Centritto, M., Pietrini, F., and Alessio, G. (2006). Stem CO2 release under illumination, corticular photosynthesis, photorespiration or inhibition of mitochondrial respiration? Plant Cell Environ. 29, 1149–1158.
Wu, X., Zheng, X. J., Li, Y., and Xu, G. Q. (2019). Varying responses of two Haloxylon species to extreme drought and groundwater depth. Environ. Exp. Bot. 158, 63–72.
Xu, H., Li, Y., Xu, G. Q., and Zou, T. (2007). Ecophysiological response and morphological adjustment of two Central Asian desert shrubs towards variation in summer precipitation. Plant Cell Environ. 30, 399–409. doi: 10.1111/j.1365-3040.2006.001626.x
Ye, Z. P., and Yu, Q. (2008). A coupled model of stomatal conductance and photosynthesis for winter wheat. Photosynthetica 46, 637–640. doi: 10.1007/s11099-008-0110-0
Zhou, H. F., Zheng, X. J., Zhou, B. J., Dai, Q., and Li, Y. (2012). Sublimation over seasonal snowpack at the southeastern edge of a desert in central Eurasia. Hydrol. Process. 26, 3911–3920.
Keywords: desert ecosystem, refixation, carbon balance, water use strategy, stem recycling photosynthesis
Citation: Liu R, Feng X, Li C, Ma J, Wang Y and Li Y (2022) The Importance of Stem Photosynthesis for Two Desert Shrubs Across Different Groundwater Depths. Front. Plant Sci. 13:804786. doi: 10.3389/fpls.2022.804786
Received: 29 October 2021; Accepted: 10 February 2022;
Published: 10 March 2022.
Edited by:
Marta Silva Lopes, Institute of Agrifood Research and Technology (IRTA), SpainReviewed by:
Junxiang Liu, Chinese Academy of Forestry, ChinaEleinis Ávila-Lovera, Smithsonian Tropical Research Institute, Panama
Wilmer Tezara, Central University of Venezuela, Venezuela
Copyright © 2022 Liu, Feng, Li, Ma, Wang and Li. This is an open-access article distributed under the terms of the Creative Commons Attribution License (CC BY). The use, distribution or reproduction in other forums is permitted, provided the original author(s) and the copyright owner(s) are credited and that the original publication in this journal is cited, in accordance with accepted academic practice. No use, distribution or reproduction is permitted which does not comply with these terms.
*Correspondence: Congjuan Li, bGlfY29uZ2p1YW5AMTYzLmNvbQ==