- 1State Key Laboratory Breeding Base of Green Pesticide and Agricultural Bioengineering, Key Laboratory of Green Pesticide and Agricultural Bioengineering, Ministry of Education, Guizhou University, Guiyang, China
- 2State Key Laboratory of Natural and Biomimetic Drugs, Peking University, Beijing, China
- 3College of Pharmacy, Guizhou Medical University, Guiyang, China
Botanical pesticides are one of the sources of third-generation pesticides, which have received much attention at home and abroad in recent years due to their degradable and pollution-free advantages in nature. This article explored a concise approach toward synthesizing a series of novel L-pyroglutamic acid analogues from L-hydroxyproline. Furthermore, bioassay studies of these sulfonyl ester derivatives against Pyricularia oryzae, Fusarium graminearum, Alternaria brassicae, Valsa mali, and Alternaria alternariae showed moderate antifungal activity. For instance, C08a and C08l provide potential lead agents for controlling Fusarium graminearum because of their inhibitory activity.
Introduction
Nature is a massive library of compounds. Natural plants have given the reputation of molecular manufacturing factories and organic chemists, providing constant inspiration for human drug design. The plant secondary metabolites mainly include organic acids, terpenoids, and alkaloids with specific structures. The effects of these natural products from plants on the target are shown as insecticidal (Zhao et al., 2016; Wang et al., 2018), antibacterial, (Lin et al., 2014), antitumor (Moutevelis-Minakakis et al., 2011), antimalarial (Amoa Onguéné et al., 2013), and other activities. They are considered ideal lead compounds for developing medical or agricultural chemicals and play an increasingly important role in discovering medicine and green pesticides.
Plant-derived drugs and their derivatives are the primary sources of many essential drugs in medicine and pesticides (Harvey, 2008). They are characterized by high selectivity, low toxicity, easy degradation, and less resistance to natural enemies. These outstanding advantages have led pesticide scientists to pay more attention to traditional herbs, which is significant for discovering novel, environmentally friendly, and sustainable plant-derived pesticides to control agricultural diseases (Newman and Cragg, 2020; Atanasov et al., 2021).
A typical Chinese herbal medicine, Disporopsis aspersa (Hua) Engl. ex Dells from the Disporopsis HANCE of Liliaceae perennial herb, has attracted considerable interest owing to its significant biological activities (Wang et al., 2015). For instance, the decoction of rhizomes in D. aspersa is primarily prescribed as a tonic for asthenia, night sweats, spermatorrhea, and polyuria. Moreover, it has other effects for treating persistent fever, dry cough, and cancer (Nguyen et al., 2006). Nonetheless, there are a few studies about the antifungal activities of this plant until 2018. As shown in Figure 1, Zhang and his colleagues investigated the antifungal activity of the crude extracts from D. aspersa. In the biological activity screening, L-pyroglutamic acid showed excellent antifungal activity against P. infestans and P. cubensis with EC50 values of 9.48 and 10.82 μg/ml, respectively, especially the inhibition rate of therapeutic effect, with a prevention rate of 87.1%, which is far more potent than the positive control drug and could be used as a candidate compound of antifungal lead compounds (Zhu et al., 2018).
In 2018, Zhang and his colleagues designed and synthesized a series of derivatives, such as L-pyroglutamate and amide, and systematically evaluated their biological activities. The bioassay and structure–activity relationship (SAR) study showed that most L-pyroglutamate esters had vigorous antibacterial activity (Gang et al., 2018). During the screening process of compound activity, the reaction of L-pyroglutamic acid with 4-chlorophenol to produce ester could effectively improve the antibacterial activity of the compounds. To further study the bioactivity and structure–activity relationship of L-pyroglutamate, the lead compound of botanical drugs was explored.
This study explored a concise approach to synthesize a series of novel L-pyroglutamic acid analogues from L-hydroxyproline, evaluated the antifungal activity of these compounds, and analyzed the structure–activity relationship of L-pyroglutamate.
Results and discussion
Design strategies for the skeleton of 4-chiral hydroxyl L-pyroglutamate compounds
Chiral hydroxyl can enhance the biological activity of chiral pesticides, such as uniconazole, diniconazole, and tebuconazole. If a chiral hydroxyl group is assembled in the precursor skeleton molecule of L-pyroglutamate, the bactericidal activity of the molecule can be enhanced without affecting other functional groups. As shown in Scheme 1, two methods exist to construct the 4S-hydroxy-L-pyroglutamate ester framework. In pathway A, the chiral hydroxyl was induced to the alpha position of the carbonyl group in L-pyroglutamic acid, as starting material via oxidation hydroxylation (Chen et al., 2022; Negi et al., 2022). However, chiral hydroxyl groups have yet to be successfully constructed after many asymmetric oxidation methods have been tried. On the other side of the strategy, the original idea may be realized by preassembling chiral hydroxyl groups onto pyrrole rings of L-hydroxyproline and then oxidative carbonylation of active methylene.
Based on the design idea, L-hydroxyproline was used as the starting material to oxidize carbonyl at the active methylene position. First, the three active functional groups of the reaction starting material must be protected; otherwise, side reactions are easy to occur. As shown in Scheme 2, the three functional groups are successively protected by N-Boc amide, OTBS silicon ether, and p-chlorophenyl formate to obtain the critical intermediate C04. Notably, 4-chlorophenol was introduced to increase the antibacterial activity of our derivatives, inspired by Zhang’s work.
With compound C04 in hand, the key carbonyl assembly will be investigated. As shown in Table 1, we tried to perform oxidative carbonylation via TBHP (Hossain and Shyu, 2016), DIB/TBHP (Zhao et al., 2013), and KMnO4 (Lai and Lee, 2002). However, we did not get the desired products with the three oxidants (entries 1~3). The subject fell into the darkest, and we searched everywhere for appropriate oxidation methods. Fortunately, Yoshifuji reported a two-phase oxidation reaction using RuO2 to convert cyclic A-amino acids to A-amino dicarboxylic acids in 1995 (Yoshifuji and Kaname, 1995). In 2001, Zhang successfully oxidized 4-hydroxyproline methyl ester into 4-hydroxypyroglutamate methyl ester using this method (Zhang et al., 2001). This method is intended to be used for oxidative carbonylation. Encouragingly, compound C04 was oxidized by RuO2·H2O in the solvent of EtOAc/H2O at room temperature to generate amide C05 with excellent reactivity in 90% yield (entry 4).
Synthesis of L-pyroglutamic acid 4-chiral hydroxyl sulfonyl ester derivatives
Sulfonyl esters are widely used in medicine and pesticides due to their remarkable biological activities. For example, the main active substance thiothiesulfate obtained from garlic extraction and separation not only has the effect of reducing blood lipid (Ackermann et al., 2001; Rahman, 2001) but also has antitumor (Bianchini and Vainio, 2001), antiviral, and a variety of medical activities such as antibacterial, bactericidal, and viricidal (Harris et al., 2001). In the study of pesticide activity, sulfonate compounds were shown to have insecticidal, acaricidal, and bactericidal activities. In addition, sulfonate compounds also have herbicidal and plant growth-regulating effects.
Sulfonyl and sulfonamide groups are important pharmacophore groups in many drugs, and introducing these groups can effectively improve the activity of compounds. In 2011, Zhao reported curcumin benzoyl sulfonate compounds’ synthesis and acaricidal activity (Zhao, 2011). Taking curcumin as the lead, the authors produced a reaction with benzene sulfonyl chloride compounds to synthesize a series of curcumin benzoyl sulfonate derivatives and tested the acaricidal activity. The results showed that compared with curcumin itself, its acaricidal and ovicidal activities were significantly improved.
As shown in Scheme 3, sulfonyl esterification was carried out on the chiral hydroxyl group, and different sulfonyl functional groups were introduced to enhance the efficacy. Most sulfonyl groups have a good reaction effect when introduced. Unfortunately, the synthesis of C07d showed a poor reaction effect and low yield. The analysis might be due to the presence of the N atom in the sulfonyl group, which increased the density of the electron cloud on the S atom and reduced the overall reactivity, resulting in poor reactivity.
Deprotection of N-Boc
The amide group, as an essential group in natural products, often appears in a state without other substitutions, which is conducive to the compound’s participation and thus enhances bioactivity. However, the amide group in the C07 series compounds obtained in this study is connected with the electron-pulling group, which is quite different from the natural products, and may impact the activity. In order to further explore whether the existence of electron-pulling groups on the amide N structure affects the antibacterial activity, as shown in Scheme 4, a C07 series of compounds was successfully removed from N-Boc to obtain C08a~n under a solution of trifluoroacetic acid (TFA) in dichloromethane.
Evaluation of antifungal activity of intermediates and derivatives
In this study, the antibacterial activity of 31 compounds against five species of fungi (Pyricularia oryzae, Fusarium graminearum, Alternaria brassicae, Valsa mali, and Alternaria alternariae) at 100 μg/ml was evaluated by an approach of inhibiting mycelium growth rate. The preliminary bioactivity test results displayed that most of the 31 target compounds had inhibitory effects on these five fungi. Among them, compounds C07l~m and C08a~n were more potent than any other against Fusarium graminearum and Valsa mali, they were superior to the commercial control drug hymexazol and equal to chlorothalonil. It is shown in Table 2 that the antibacterial activities of C07l and C07m were equal to those of C08l and C08m, but the antibacterial activities of other compounds were lower than those of C08 series compounds, the results indicated that the amide group attached to the Boc group was detrimental to the inhibitory activity. The activities of L-pyroglutamic acid derivatives with alkane chains were slightly higher than those of aromatic compounds. In addition, the introduction of naphthalene (C08l) and alkane (C08a) on the hydroxyl group could effectively enhance the inhibitory activity of Fusarium graminearum. As for the effects of substituents on aromatic compounds, available data exhibited that an electron-withdrawing group played an important role to the enhancement of antibacterial activity.
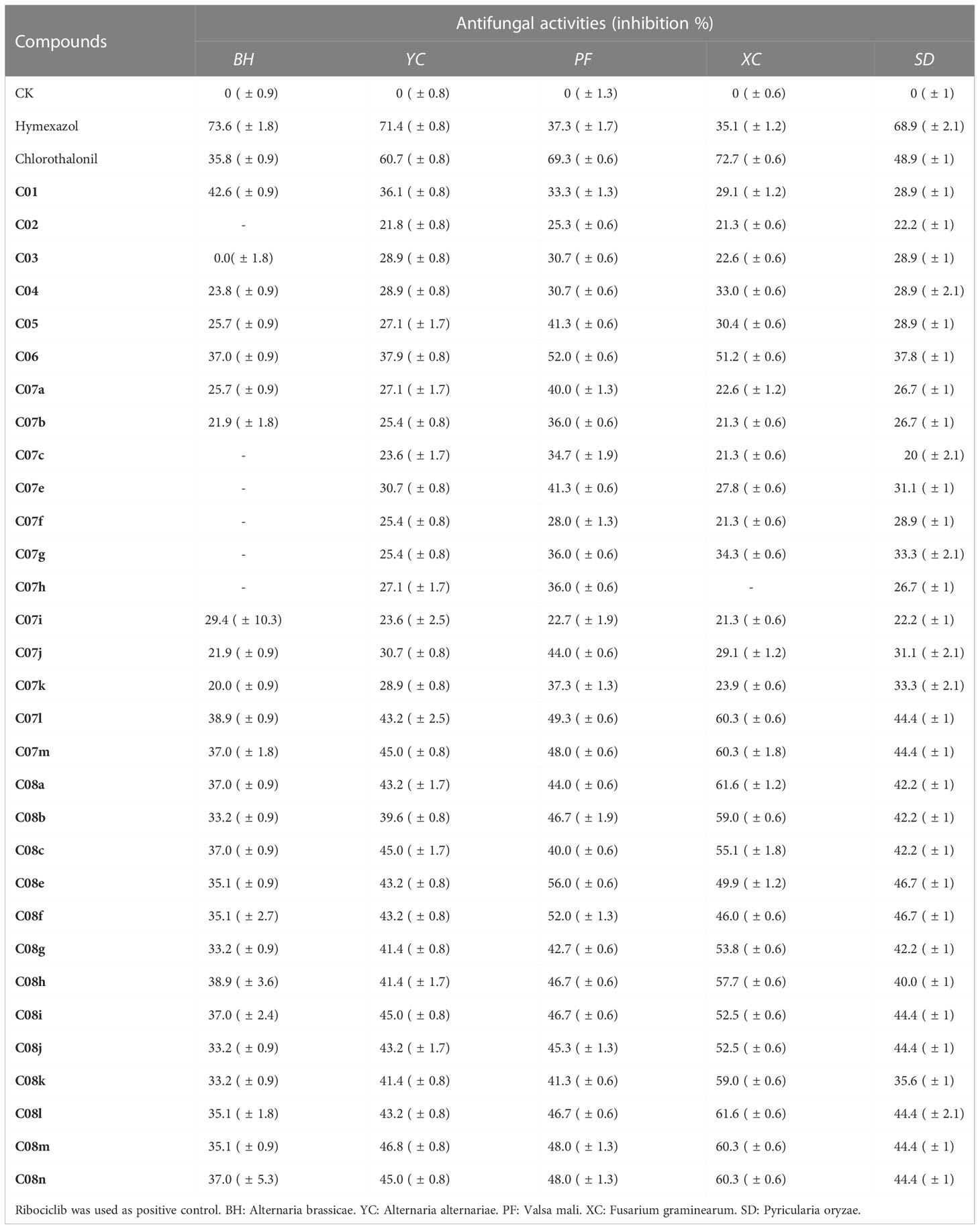
Table 2 The inhibitory rates of the L-pyroglutamic acid derivatives on phytopathogenic fungi (100 μmol/l).
According to the above results, the structure–activity relationship (SAR) could be done. All C08 series compounds showed significant antifungal activities against Fusarium graminearum (Table 2). In particular, the activity of the compounds with naphthalene was more potent than those with phenyls, and the electron-withdrawing groups on the aromatic ring facilitated the inhibitory effects of C08e~k. In addition, the introduction of 4-chlorophenol into compound C04 was beneficial to the antibacterial activity against the five fungi.
Materials and methods
Equipment and materials
All reactions were performed in flame-dried glassware under a nitrogen atmosphere. Solvents were distilled prior to use. Reagents were used as purchased from Aladdin, Macklin, Innochem, or TLC unless otherwise noted. Chromatographic separations were performed using a silica gel, AR, 200–300 mesh. 1H and 13C NMR spectra were obtained on Bruker 400 MHz NMR and JNM-ECZR 500 MHz NMR instruments using CDCl3 and DMSO as the solvent, which were provided by the School of State Key Laboratory Breeding Base of Green Pesticide and Agricultural Bioengineering, Key Laboratory of Green Pesticide and Agricultural Bioengineering, Ministry of Education, Guizhou University. Optical rotations were obtained on an InsMark digital polarimeter using a sodium (589 nm, D line) lamp and are reported as follows: (c = g/100 ml, solvent). TLC analysis was visualized using UV and phosphomolybdic acid stains. High-resolution mass spectra were obtained using Q Exactive. All spectral data obtained for new compounds are reported here.
The biological reagents used were glucose, AGAR, and streptomycin. In addition, potatoes were bought from supermarkets.
Synthetic procedures for the key intermediate C06
The synthetic procedures for the key intermediate C06 from L-hydroxyproline are shown in Scheme 5.
To a solution of L-hydroxyproline C01 [20.0 g, 152 mmol, in H2O (76 ml) was added a solution of 10% NaOH (aqueous, 60 ml, 167 mmol). The resulting solution was stirred for 2 h followed by adding a solution of Boc2O (36.6 g, 167 mmol) in THF (152 ml) in one portion via a syringe. The mixture was stirred for 24 h at room temperature (rt), and the reaction was quenched by addition of 10% KHSO4 solution in water to adjust pH = 4~5, and the organic layer was separated. The aqueous layer was further extracted with EtOAc (100 ml × 3). The combined organic extracts were washed with the saturated solution of NaCl (50 ml × 1), dried over anhydrous Na2SO4, filtered, and concentrated in vacuo to afford C02 as white solid (30.0 g, 85%) and was used directly in the next step without further purification. Mp = 117.8~118.6 °C. Rf = 0.3 (100% MeOH/CH2Cl2). 1H NMR (400 MHz, DMSO-d6, 25°C, mixture of rotamers) δ 12.49 (br s, 1H), 5.04 (br s, 1H), 4.25~4.22 (m, 1H), 4.11 (td, J = 7.9, 2.4 Hz, 1H), 3.33~3.41 (m, 1H), 3.28~3.20 (m, 1H), 2.05~2.14 (m, 1H), 1.84~1.92 (m, 1H), 1.36 (d, J = 18.8 Hz, 9H). 13C NMR (100 MHz, DMSO-d6, 25°C, mixture of rotamers) δ 174.5, 174.0, 153.9, 153.3, 78.9, 78.9, 68.6, 67.9, 57.8, 57.5, 54.7, 54.4, 28.2, 28.0. HRMS (ESI+): m/z calculated for C10H17NO5 [M+Na]+ 254.1106, found 254.0995.
To a solution of C02 (5.0 g, 22 mmol) in CH2Cl2 (45 ml) were added t-butylchlorodimethylsilane (7.2 g, 48 mmol) and imidazole (6.5 g, 95 mmol). The mixture was stirred for 10 h at room temperature, and the reaction was quenched by addition of 1 M HCl solution to adjust pH = 4~5. The organic layers were separated, and the aqueous layer was extracted with CH2Cl2 (50 ml × 3). The combined organic extracts were washed with the saturated solution of NaCl (50 ml × 1), dried over anhydrous NaSO4, filtered, and concentrated in vacuo. The residue was subjected to silica gel chromatography (petroleum ether: EtOAc = 3:1) to afford C03 as colorless oil (6.7 g, 90%). Rf = 0.3 (30% EtOAc/petroleum ether). 1H NMR (500 MHz, CDCl3, 25°C, mixture of rotamers) δ 6.42 (br s, 1H), 4.46~4.30 (m, 2H), 3.61~3.45 (m, 1H), 3.41~3.32 (m, 1H), 2.28~2.02 (m, 2H), 1.45 (d, J = 31.4 Hz, 9H), 0.86 (s, 9H), 0.06 (d, J = 3.5 Hz, 6H). 13C NMR (100 MHz, CDCl3, 25°C, mixture of rotamers) δ 178.3, 175.3, 156.6, 154.1, 81.5, 80.7, 70.1, 69.8, 58.1, 55.0, 54.7, 39.9, 38.0, 31.2, 28.5, 28.3, 25.8, 25.77, 25.7, 18.1. HRMS (ESI+): m/z calculated for C16H31NO5Si [M+Na]+ 368.2971, found 368. 3153.
To a solution of C03 (4.2 g, 12 mmol) in CH2Cl2 (40 ml) were added DCC (3.0 g, 15 mmol) and 4-chlorophenol (1.9 g, 15 mmol). The mixture was stirred for 3 h at room temperature. CH2Cl2 was added to dilute and filter solids. The organic layer was concentrated in vacuo. The residue was subjected to silica gel chromatography (petroleum ether: EtOAc = 10:1) to afford C04 as colorless solid (4.1 g, 74%). Mp = 56~59.8 °C. Rf = 0.3 (10% EtOAc/petroleum ether). 1H NMR (500 MHz, CDCl3, 25°C, mixture of rotamers) δ 7.35~7.29 (m, 2H), 7.07~7.02 (m, 2H), 4.61~4.48 (m, 1H), 4.47~4.45 (m, 1H), 3.65~3.58 (m, 1H), 3.51~3.35 (m, 1H), 2.37~2.26 (m, 1H), 2.21~2.11 (m, 1H), 1.45 (d, J = 12.7 Hz, 9H), 0.87 (s, 9H), 0.07 (s, 6H). 13C NMR (100 MHz, CDCl3, 25°C, mixture of rotamers) δ 171.6, 171.5, 154.7, 153.9, 149.3, 149.1, 131.4, 131.3, 129.6, 129.5, 123.0, 122.5, 80.6, 80.4, 70.6, 69.8, 58.3, 58.0, 55.1, 54.8, 40.1, 39.0, 35.0, 28.5, 28.4, 25.8, 25.8, 25.5, 24.8, 18.1, 18.0. HRMS (ESI+): m/z calculated for C22H34ClNO5Si [M+Na]+ 478.1894, found 478.1779.
To a solution of NaIO4 (3.8 g, 17 mmol) in H2O (30 ml) was added RuO2·H2O (0.13 g, 1 mmol) at room temperature. The resulting green yellow solution was stirred for 2 h followed by addition of C04 (2.0 g, 4 mmol) in EtOAc (15 ml) in one portion via a syringe. Additional aliquots of NaIO4 were added to maintain a yellow-colored solution during the reaction. The mixture was stirred for 9 h at room temperature, and EtOAc (100 ml) to dilution reaction. The reaction was quenched by addition of sat. aq. Na2S2O3, which immediately resulted in the precipitation of Ru black. The organic layers were separated, and the organic extract was filtered through a pad of Celite. The filtrate was then washed with the saturated solution of NaCl (50 ml × 1), dried over anhydrous Na2SO4, and evaporated under reduced pressure. The resulting residue was subjected to silica gel chromatography (petroleum ether: EtOAc = 10:1) to afford C05 as a white solid (1.85 g, 90%). Mp = 73.7~76.9°C. Rf = 0.33 (10% EtOAc/petroleum ether). 1H NMR (400 MHz, CDCl3, 25°C, mixture of rotamers) δ 7.20~7.16 (m, 2H), 6.89~6.85 (m, 2H), 4.60 (dd, J = 9.8, 1.7 Hz, 1H), 4.31 (dd, J = 10.0, 8.2 Hz, 1H), 2.36~2.30 (m, 1H), 2.19~2.11 (m, 1H), 1.34 (s, 9H), 0.72 (s, 9H), 0.05 (d, 6H). 13C NMR (100 MHz, CDCl3, 25°C, mixture of rotamers) δ 171.6, 169.7, 149.7, 148.7, 131.9, 129.7, 122.4, 84.4, 69.7, 55.2, 31.8, 28.0, 25.7, 18.2. HRMS (ESI+): m/z calculated for C22H32ClNO6Si [M+Na]+ 482.1687, found 492. 1571.
To a solution of C05 (1.8 g, 4 mmol) in THF (40 ml) at room temperature was added hydrogen fluoride (70% in HF, 3.1 ml, 24 mmol). The mixture was stirred for 4 h at room temperature, and the reaction was quenched by addition of solid NaHCO3 filtered and concentrated in vacuo. The residue was subjected to silica gel chromatography (petroleum ether: EtOAc = 3:1) to afford C06 as white solid (1.2 g, 85%). Mp = 120~121 °C. . Rf = 0.4 (100% EtOAc/petroleum ether). 1H NMR (400 MHz, CDCl3, 25°C, mixture of rotamers) δ 7.38~7.35 (m, 2H), 7.09~7.05 (m, 2H), 4.84 (dd, J = 9.9, 1.2 Hz, 1H), 4.55 (dd, J = 10.8, 8.4 Hz, 1H), 3.05~2.97 (m, 1H), 2.60~2.55 (m, 1H), 1.54 (s, 9H). 13C NMR (100 MHz, CDCl3, 25°C, mixture of rotamers) δ 200.9, 173.4, 169.2, 149.0, 148.6, 132.0, 129.8, 122.3 84.82, 68.7, 55.5, 30.6, 27.9. HRMS (ESI+): m/z calculated for C16H18ClNO6 [M+Na]+ 378.0822, found 378.3245.
Synthetic procedures for L-pyroglutamic acid 4-chiral hydroxyl sulfonyl ester derivatives
To a solution of C06 (100 mg, 0.28 mmol) in CH2Cl2 (1.0 ml) were added DMAP (0.03 mmol) and Et3N (45 mg, 0.42 mmol). The resulting solution was stirred for 10 min followed by addition of sulfonyl chloride (0.42 mmol) in CH2Cl2 (0.5 ml) in one portion via a syringe. The reaction mixture was stirred for 1~4 h at room temperature, and the reaction was quenched by addition of sat. aq. NH4Cl. The organic layers were separated and the aqueous layer was extracted with CH2Cl2 (2 ml × 3), and the combined organic extracts were washed with the saturated solution of NaCl (10 ml × 1) and dried over anhydrous Na2SO4. The residue was subjected to silica gel chromatography (petroleum ether: EtOAc = 10:1) to afford C07a~m.
Synthetic procedures for deprotection of N-Boc
TFA (2.2 equiv) was added to sulfonyl esters C07a~m (1.0 equiv) in CH2Cl2 (0.25 M), and the mixture was stirred at room temperature for 0.5~2 h. The reaction was quenched with saturated NaHCO3 aqueous solution, and the mixture was separated through a separating funnel. The aqueous phase was extracted by CH2Cl2 (×3) and dried with anhydrous Na2SO4. After removal solvent by reduce pressure, the crude residue was purified using silica gel flash column chromatography [eluent: EtOAc/petroleum ether] to give compound C08a~n.
Biological assays: the antifungal activity assay
In vitro antifungal activity: L-pyroglutamic acid analogues were screened in vitro for their antifungal activities against five phytopathogenic fungi by poisoned food technique. Five phytopathogenic fungi, namely, Pyricularia oryzae (SD), Fusarium graminearum (XC), Alternaria brassicae (BH), Valsa mali (PF), and Alternaria alternariae (YC), were used for the assays. Potato dextrose agar (PDA) medium was prepared in the flasks and sterilized. The target compounds were dissolved in acetone before mixing with PDA, and the concentration of the test compounds in the medium was fixed at 100 μg/ml. The medium was then poured into sterilized Petri dishes. All types of fungi were incubated in PDA at 27 ± 1°C for 4 days to get new mycelia for the antifungal assays, and a mycelium disk of approximately 4 mm in diameter cut from the culture medium was picked up with a sterilized inoculation needle and inoculated in the center of the PDA Petri dishes. The inoculated Petri dishes were incubated at 27 ± 1°C for 4 days. Acetone without any compounds mixed with PDA was served as a negative control, whereas hymexazol and chlorothalonil, two commercial agricultural fungicides, were used as positive controls. For each treatment, three replicates were conducted. The radial growths of the fungal colonies were measured, and data were statistically analyzed. The inhibitory effects of the test compounds on these fungi in vitro were calculated by the following formula: Inhibition rate (%) = (C-T) × 100/(C-4 mm), where C represents the diameter of fungi growth on untreated PDA and T represents the diameter of fungi on treated PDA. Statistical analysis was processed by the SPSS 21.0 (SPSS Inc., Chicago, USA) software.
Conclusion
In summary, a novel method of oxycarbonylation was used to construct the target skeleton successfully. At the same time, the introduction of the chiral hydroxyl group increased the reaction site, and a series of L-pyroglutamic acid derivatives were synthesized through diversification and their antifungal activities were evaluated. According to the bioassay results, most L-pyroglutamic acid derivatives showed good antibacterial activity against Fusarium graminearum, which are superior to commercially available hymexazol compounds C08a and C08l showing the best activity and being similar to commercially available chlorothalonil. To the best of our knowledge, this is the first report on antifungal properties of chiral 4-hydroxyl L-pyroglutamic acid derivatives.
Data availability statement
The original contributions presented in the study are included in the article/Supplementary Material. Further inquiries can be directed to the corresponding author.
Author contributions
YC designed the experiment. YC and LA wrote the manuscript. SF, MZ, and WH prepared all the derivatives and determined the structure via spectra. YL and JH performed antifungal activity experiment and analyzed the data. YC and ZJ supervised the entire project. All authors have read and approved the manuscript.
Funding
This work was supported by Guizhou Provincial Science and Technology Projects [2020]1Y108, the State Key Laboratory of Natural and Biomimetic Drugs (K202223), the Science and Technology Foundation of Guizhou Province (No. Qian Ke He platform talents [2018]5781-30), the PhD Foundation of Guizhou University (Gui Da Ren Ji He [2017]32), the Plant Protection and Inspection Station of Guizhou Province Project (K19-0201-007), and the State Key Laboratory Breeding Base of Green Pesticide and Agricultural Bioengineering/Key Laboratory of Ministry of Education, Guizhou University (QJHKYZ[2022]362).
Conflict of interest
The authors declare that the research was conducted in the absence of any commercial or financial relationships that could be construed as a potential conflict of interest.
Publisher’s note
All claims expressed in this article are solely those of the authors and do not necessarily represent those of their affiliated organizations, or those of the publisher, the editors and the reviewers. Any product that may be evaluated in this article, or claim that may be made by its manufacturer, is not guaranteed or endorsed by the publisher.
Supplementary material
The Supplementary Material for this article can be found online at: https://www.frontiersin.org/articles/10.3389/fpls.2022.1102411/full#supplementary-material
References
Ackermann, R. T., Mulrow, C. D., Ramirez, G., Gardner, C. D., Morbidoni, L., Lawrence, V. A. (2001). Garlic shows promise for improving some cardiovascular risk factors. Arch. Intern. Med. 161 (6), 813–824. doi: 10.1001/archinte.161.6.813
Amoa Onguéné, P., Ntie-Kang, F., Lifongo, L. L., Ndom, J. C., Sippl, W., Mbaze, L. M. A. (2013). The potential of anti-malarial compounds derived from African medicinal plants. part I: A pharmacological evaluation of alkaloids and terpenoids. Malar. J. 12 (1), 449. doi: 10.1186/1475-2875-12-449
Atanasov, A. G., Zotchev, S. B., Dirsch, V. M., International Natural Product Sciences, T, Supuran, C. T. (2021). Natural products in drug discovery: advances and opportunities. Nat. Rev. Drug Discov. 20 (3), 200–216. doi: 10.1038/s41573-020-00114-z
Bianchini, F., Vainio, H. (2001). Allium vegetables and organosulfur compounds: do they help prevent cancer? Environ. Health Perspect. 109 (9), 893–902. doi: 10.1289/ehp.01109893
Chen, B., Wu, Q., Xu, D., Zhang, X., Ding, Y., Bao, S., et al. (2022). A two-phase approach to fusicoccane synthesis to uncover a compound that reduces tumourigenesis in pancreatic cancer cells. Angew. Chem. Int. Ed. 61 (19), e202117476. doi: 10.1002/anie.202117476
Gang, F.-l., Zhu, F., Li, X.-T., Wei, J.-L., Wu, W.-J., Zhang, J.-W. (2018). Synthesis and bioactivities evaluation of l-pyroglutamic acid analogues from natural product lead. Bioorg. Med. Chem. 26 (16), 4644–4649. doi: 10.1016/j.bmc.2018.07.041
Harris, J. C., Cottrell, S. L., Plummer, S., Lloyd, D. (2001). Antimicrobial properties of allium sativum (garlic). Appl. Microbiol. Biotechnol. 57 (3), 282–286. doi: 10.1007/s002530100722
Harvey, A. L. (2008). Natural products in drug discovery. Drug Discov. Today 13 (19-20), 894–901. doi: 10.1016/j.drudis.2008.07.004
Hossain, M. M., Shyu, S.-G. (2016). Biphasic copper-catalyzed c-h bond activation of arylalkanes to ketones with tert-butyl hydroperoxide in water at room temperature. Tetrahedron 72 (29), 4252–4257. doi: 10.1016/j.tet.2016.05.066
Lai, S., Lee, D. G. (2002). Lewis Acid assisted permanganate oxidations. Tetrahedron 58 (49), 9879–9887. doi: 10.1016/S0040-4020(02)01227-9
Lin, Q., Zhang, L., Yang, D., Chunjie, Z. (2014). Contribution of phenolics and essential oils to the antioxidant and antimicrobial properties of disporopsis pernyi (Hua) diels. J. Med. Food 17 (6), 714–722. doi: 10.1089/jmf.2013.2905
Moutevelis-Minakakis, P., Papavassilopoulou, E., Michas, G., Georgikopoulou, K., Ragoussi, M.-E., Neophytou, N., et al. (2011). Synthesis, in silico docking experiments of new 2-pyrrolidinone derivatives and study of their anti-inflammatory activity. Bioorg. Med. Chem. 19 (9), 2888–2902. doi: 10.1016/j.bmc.2011.03.044
Negi, R., Jena, T. K., Jyoti, Tuti, N. K., Anindya, R., Khan, F. A. (2022). Solvent controlled synthesis of 2,3-diarylepoxy indenones and α-hydroxy diarylindanones and their evaluation as inhibitors of DNA alkylation repair. Org. Biomol. Chem. 20 (29), 5820–5835. doi: 10.1039/d2ob00595f
Newman, D. J., Cragg, G. M. (2020). Natural products as sources of new drugs over the nearly four decades from 01/1981 to 09/2019. J. Nat. Prod. 83 (3), 770–803. doi: 10.1021/acs.jnatprod.9b01285
Nguyen, A.-T., Fontaine, J., Malonne, H., Duez, P. (2006). Homoisoflavanones from disporopsis aspera. Phytochemistry 67 (19), 2159–2163. doi: 10.1016/j.phytochem.2006.06.021
Rahman, K. (2001). Historical perspective on garlic and cardiovascular disease. J. Nutr. 131 (3S), 977S–979S. doi: 10.1093/jn/131.3.977S
Wang, Y., Li, S., Han, D., Meng, K., Wang, M., Zhao, C. (2015). Simultaneous determination of rutin, luteolin, quercetin, and betulinic acid in the extract of Disporopsis pernyi (Hua) diels by UPLC. J. Anal. Methods Chem. 2015 (130873), 1–5. doi: 10.1155/2015/130873
Wang, D., Xie, N., Yi, S., Liu, C., Jiang, H., Ma, Z., et al. (2018). Bioassay-guided isolation of potent aphicidal erythrina alkaloids against aphis gossypii from the seed of erythrina crista-galli l. Pest Manage. Sci. 74 (1), 210–218. doi: 10.1002/ps.4698
Yoshifuji, S., Kaname, M. (1995). Asymmetric synthesis of lycoperdic acid. Chem. Pharm. Bull. 43 (10), 1617–1620. doi: 10.1248/cpb.43.1617
Zhang, X., Schmitt, A. C., Jiang, W. (2001). A convenient and high yield method to prepare 4-hydroxypyroglutamic acids. Tetrahedron Lett. 42 (32), 5335–5338. doi: 10.1016/S0040-4039(01)01030-9
Zhao, Y. (2011). Synthesis of curcumin benzenesulfonate analogues and their acaricidal activity against tetranychus cinnabarinus (Acari: Tetranychidae) (Southwest University). Available at: https://kns.cnki.net/KCMS/detail/detail.aspx?dbname=CMFD2011&filename=1011114044.nh.
Zhao, Y., Ang, J. Q. L., Ng, A. W. T., Yeung, Y.-Y. (2013). Oxidative transformation of cyclic ethers/amines to lactones/lactams using a DIB/TBHP protocol. RSC Adv. 3 (43), 19765–19768. doi: 10.1039/c3ra44184a
Zhao, X., Xi, X., Hu, Z., Wu, W., Zhang, J. (2016). Exploration of novel botanical insecticide leads: Synthesis and insecticidal activity of β-dihydroagarofuran derivatives. J. Agric. Food Chem. 64 (7), 1503–1508. doi: 10.1021/acs.jafc.5b05782
Keywords: L-pyroglutamic acid, chiral hydroxyl, benzenesulfonyl derivatives, antifungal activity, botanical Pesticides
Citation: Ai L, Fu S, Li Y, Zuo M, Huang W, Huang J, Jin Z and Chen Y (2022) Natural products-based: Synthesis and antifungal activity evaluation of novel L-pyroglutamic acid analogues. Front. Plant Sci. 13:1102411. doi: 10.3389/fpls.2022.1102411
Received: 18 November 2022; Accepted: 05 December 2022;
Published: 22 December 2022.
Edited by:
Zhiping Che, Henan University of Science and Technology, ChinaReviewed by:
Yanni Ma, Henan Academy of Sciences, ChinaPeng Xu, Chongqing University of Science and Technology, China
Panpan Wang, Huanghuai University, China
Copyright © 2022 Ai, Fu, Li, Zuo, Huang, Huang, Jin and Chen. This is an open-access article distributed under the terms of the Creative Commons Attribution License (CC BY). The use, distribution or reproduction in other forums is permitted, provided the original author(s) and the copyright owner(s) are credited and that the original publication in this journal is cited, in accordance with accepted academic practice. No use, distribution or reproduction is permitted which does not comply with these terms.
*Correspondence: Yang Chen, ychen1@gzu.edu.cn