- 1College of Plant Science and Technology, Huazhong Agricultural University, Wuhan, China
- 2Hubei Hongshan Laboratory, Wuhan, China
- 3Department of Biology, Saint Mary’s University, Halifax, NS, Canada
- 4College of Science, Health, Engineering and Education, Murdoch University, Murdoch, WA, Australia
Lodging reduces grain yield in cereal crops. The height, diameter and strength of stem are crucial for lodging resistance, grain yield, and photosynthate transport in barley. Understanding the genetic basis of stem benefits barley breeding. Here, we evaluated 13 stem related traits after 28 days of heading in a barley DH population in two consecutive years. Significant phenotypic correlations between lodging index (LI) and other stem traits were observed. Three mapping methods using the experimental data and the BLUP data, detected 27 stable and major QTLs, and 22 QTL clustered regions. Many QTLs were consistent with previously reported traits for grain filling rate, internodes, panicle and lodging resistance. Further, candidate genes were predicted for stable and major QTLs and were associated with plant development and adverse stress in the transition from vegetative stage to reproductive stage. This study provided potential genetic basis and new information for exploring barley stem morphology, and laid a foundation for map-based cloning and further fine mapping of these QTLs.
Introduction
Lodging largely impairs grain yield and quality, especially for high-yielding cultivars (Islam et al., 2007), which is divided into stem lodging or root lodging (Kashiwagi et al., 2005). In cereal crops, lodging is governed by genetic, field management and environmental factors (Wu and Ma, 2016). In many agricultural systems, this is a tough challenge due to the complexity of stem lodging (Berry et al., 2004). Especially the large panicles of modern varieties are more prone to lodging in the presence of wind, rain and diseases (Martinez-Vazquez, 2016). Lodging affects photosynthetic capacity of cereal crops and has an adverse effect on grain development (Shah et al., 2017; Shah et al., 2019). Besides, lodged barley is likely to be infected with diseases and pests (Berry et al., 2004; Caier̄ao, 2006).
Crop yield is affected by the source, sink and flow. Stems are the most important storage organs during assimilation translocation after anthesis (Matthias et al., 2016), and plants particularly rely on the dry matter and nitrogen fixation of stems under stress (Housley and Peterson, 1982). As a regular conduit for transporting water and nutrients from root or leaves to panicle, stem structure is the key to improve grain filling and high yield (Chen et al., 2004; Huang et al., 2016; Zhai et al., 2018). Additionally, stem is the supporting organ for proper distribution of leaves, which is beneficial to improve the efficiency of sunlight use. Changes at the horizontal location of plants in photosynthesis can lead to 27−31% reduction in the yield (Pinthus, 1974; Berry et al., 2004). After heading of barley, the flag leaf is the main photosynthetic organ (Yap and Harvey, 1972), therefore, as a bridge from flag leaf to panicle, the uppermost internode is especially crucial in the later stage for filling of grain. Selection of lodging resistant cultivars is one of several very important targets in cereal breeding program. The lodging resistance correlated with stem strength, plant height, panicle type and cell wall components (Shah et al., 2017; Khobra et al., 2019). The dwarf and semi-dwarf genes were preferentially used to lower lodging risk in barley during the Green Revolution (Hedden, 2003), but several studies have shown that plant over short affected crop yield (Islam et al., 2007), so enhancing the stem strength of plants is another viable option to avoid lodging.
Many studies have been done on barley lodging (Cui and Shen, 2011; Baker et al., 2014; Leblicq et al., 2016). Based on stalk characteristics and lodging factors, a lodging index was proposed to measure the lodging trait (Islam et al., 2007; Li et al., 2017), thus, the lodging index becomes a comprehensive trait composed of many single traits. Moreover, some studies have indicated that leaf sheaths and vascular bundles are also crucial contributing factors to lodging (Wu and Ma, 2020; Cornwall et al., 2021). Lin et al. (2005) indicated that a lower pith diameter/stem diameter ratio can improve stem strength. The lignin and cellulose content of secondary cell wall (CW) also affects the mechanical properties of stems (Jones et al., 2001; McFarlane et al., 2014).
In recent years, many studies on stem related traits in barley were reported. Such as Bellucci et al. (2017) performed genome-wide association mapping of grain yield and cell wall polymer content in winter barley. QTLs for the length of each internode (Schmalenbach and Pillen, 2009), chromosome regions containing significant associations with cellulose concentration (Houston et al., 2015), characterization of plant height (Wendt et al., 2016; Bélanger et al., 2018; Pu et al., 2021) have been reported. Kristensen et al. (2016) identified some barley lodging resistance loci. The focus of lodging in barley has been on root, plant height and spike traits, limited research was performed on the effect of stem strength, stem internode and node diameter on lodging.
In the study, we performed QTL mapping of 13 stem related traits after 28 days of heading using a barley DH population, including uppermost node diameter (UND), second node diameter (SND), third node diameter (TND), uppermost internode length (UIL), second internode diameter (SID), third internode diameter (TID), second internode length (SIL), third internode length (TIL), uppermost internode diameter (UID), main stem length of fracture (MSL), stem fresh weight (SW), third internode breaking force (TIBF) and lodging index (LI). The aims were to explore the relationship between stem internode traits and lodging traits, and to predict and screen out candidate genes related to stem development. The study will deepen our understanding of the genetic basis of stem, and provide new insights to boost lodging resistance breeding in barley.
Materials and methods
Plant material
A doubled haploid (DH) population with 122 lines derived from six-rowed barley Huaai11 and two-rowed barley Huadamai 6 was employed to identify QTLs (Ren et al., 2010). These accessions were evaluated over two crop seasons (2020-2021, 2021-2022) in the experimental farm of Huazhong Agricultural University, Wuhan, China (30°C 48’N, 114°C 36’E), with three replicates in a completely randomized block design. In each replicate, lines were planted in double rows with 15 cm plant spacing and 20 cm row spacing. Field cultivation management followed standard agricultural practices for barley production.
Phenotyping
The heading date of each line was recorded, after 28 days of heading, three plants with uniform growth were randomly selected from each replicate and their main stems were cut at the root to measure phenotypic data of stem related traits, and the mean value for each phenotype was used for the analysis. The main stem length (from the fracture of the stem to the apex of the panicle, MSL, cm), uppermost internode length (UIL, cm), second internode length (SIL, cm) and third internode length (TIL, cm) were measured using a straight edge, the uppermost node diameter (UND, mm), second node diameter (SND, mm), third node diameter (TND, mm), uppermost internode diameter (UID, mm), second internode diameter (SID, mm) and third internode diameter (TID, mm) were measured at the middle of them with a slide caliper. The breaking force of third internode (TIBF, N) was measured using a prostrate tester (DIK7400, Japan), and the fracture site was arranged in the center of the third internode (Supplementary Figure S1). Main stem fresh weight (SW, g) is the weight from the fracture to the apex of the panicle. Lodging index (LI) was calculated according to the equation: (Li et al., 2017)
Data analysis
The data was analyzed using SPSS 25 (USA). The best linear unbiased predictor (BLUP) of stem traits was forecast using the R package lme4. The broad-sense heritability (H2) was computed using: H2 =VG/(VE/N + VG), N was the number of environments (Liu et al., 2020). In this study, the BLUP was used to analyze the Pearson correlation between stem traits. The plots were drawn using R package ggplot2.
QTL analysis
A high-density genetic linkage map of this double haploid population was constructed by Ren et al. (2016), which included 1962 markers on all seven chromosomes. It spanned 1375.80 cM of the whole-genome with an average marker distance of 0.7 cM. Stem related traits QTLs detection were analyzed in QTL IciMapping v4.1 software. The mapping method ICIM-ADD in “MET” module and “BIP” module was used to perform Multi Environment and Single environment Trials analyses, respectively. The PIN was 0.001 and the step was 1.0 cM. Furthermore, QTL analysis for BLUP was performed in BIP module. In addition, to overcome the interference of row type (Rt), we used Rt as covariates. QTL analysis of covariates was performed using QTL.gCIMapping software of R (Feng et al., 2018). The LOD was set to 3.0, and the step was 1 cM.
Gene annotation for major QTLs
For the same trait, a QTL with an explained phenotypic variance (PVE) ≥10% and positioned in at least two years (including BLUP) as a stable QTL. To determine whether the QTL is novel, we compared the physical locations of loci detected here with those reported. The sequences of flanking markers were searched at the National Center for Biotechnology Information (NCBI). By searching the coding sequences, the predicted candidate genes of the main QTL in the physical interval were obtained from Barley genome assembly Morex_v2.0. The orthologs of other plants genes in the barley reference genome were identified using the Ensembl Plant Database (http://plants.ensembl.org/Hordeum_vulgare/Tools/Blast) (Mascher et al., 2017). We reviewed annotated information of the markers and identified potential candidate genes (Cantalapiedra et al., 2015).
Results
Phenotype analyses
We phenotyped 13 stem related traits 28 days after the heading in 2021 and 2022 (Table 1). Large variation of the stem traits was also found, the coefficient of variation (CV) was 8.09 -34.11%. Broad-sense heritability of these traits was 67.00-97.29%. The phenotypic differences in the stem of parents were shown in Table 1. T-tests showed significant differences (P< 0.05) in all stem related traits except TIBF and UND between parents. Huadamai 6 owned higher values for UND, SND, TND, SID, TID, UIL, SIL, TIL, MSL, SW, TIBF and LI in two years than Huaai11, while Huaai 11 had more UID than Huadamai 6. All 13 stem related traits showed normal distribution (Figure 1).
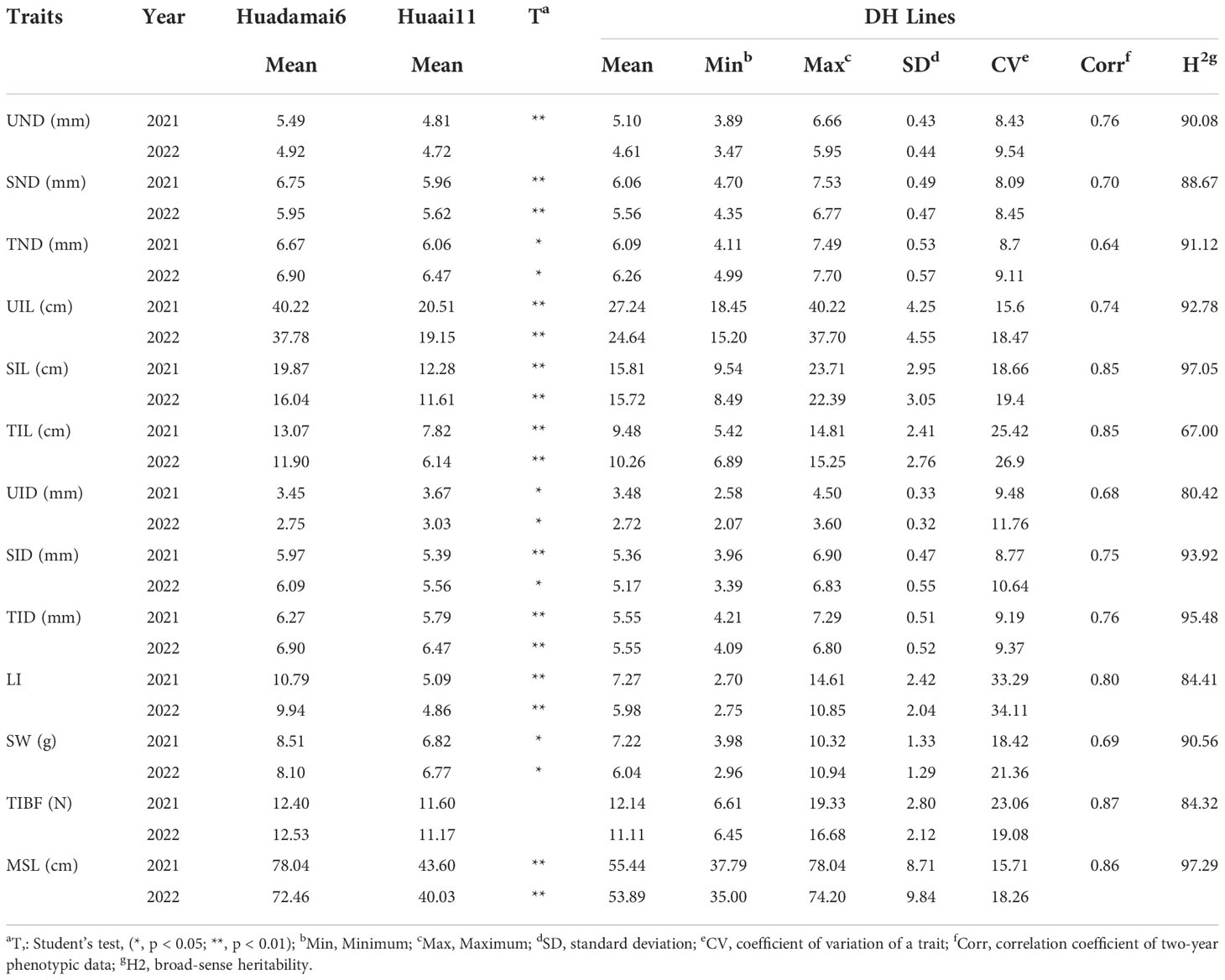
Table 1 Phenotypic performance for the thirteen stem related traits in the DH population and their parents.
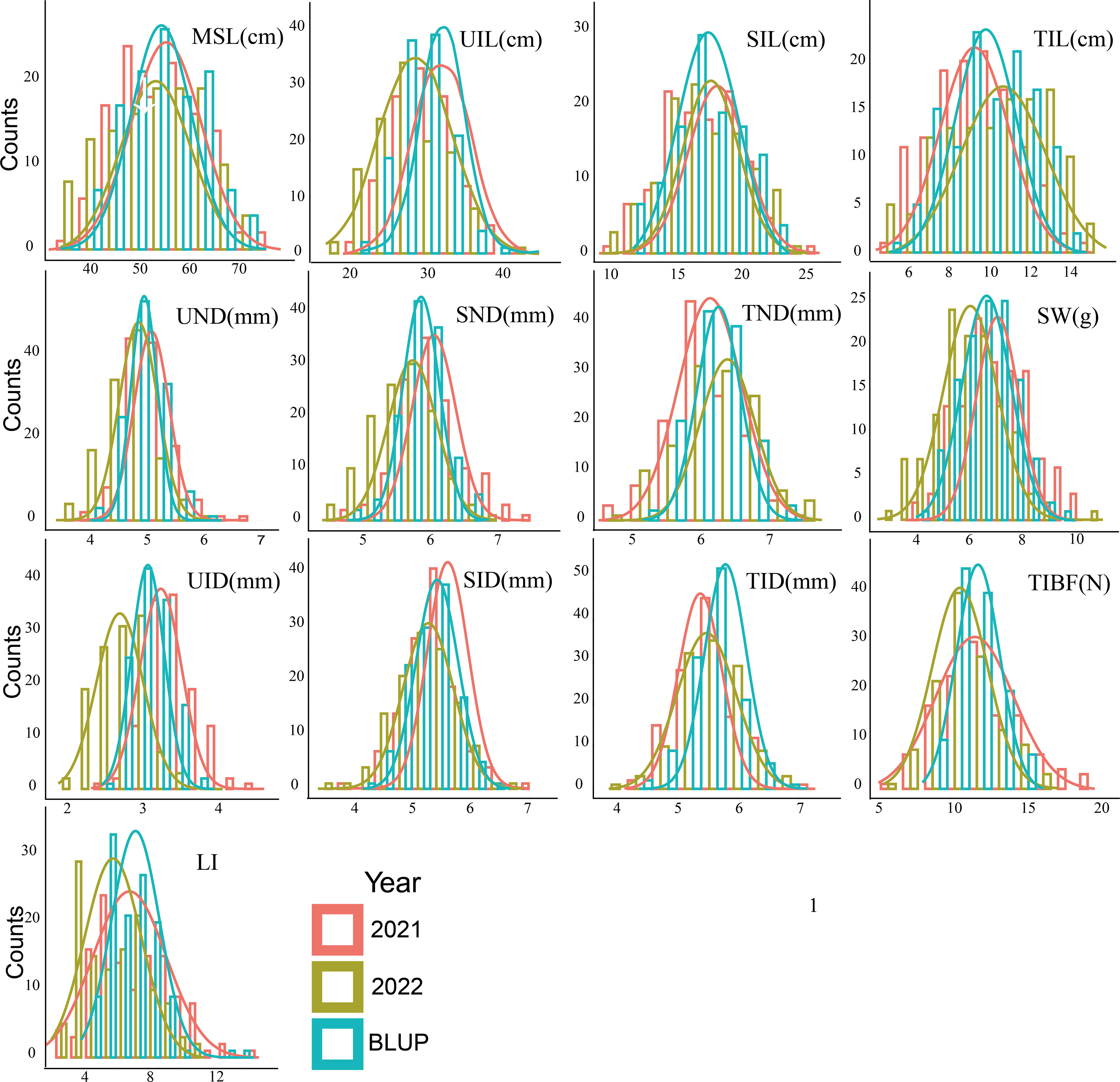
Figure 1 Phenotype distribution of 13 stem traits in each season (2021 and 2022) and BLUP value (best linear unbiased prediction environments).
Correlations between traits
The best linear unbiased predictor (BLUP) of stem lodging traits was used for Pearson correlation analysis (Figure 2). The results showed that TIBF was negatively correlated with LI and TIL (P<0.01). UIL, SIL and MSL showed a significant correlation with UND, SND and TND (P<0.01), and the length of each internode had no correlation with TID. SID was positively correlated (P<0.5) with UIL. The diameter of each stem node was positively correlated with the stem diameter of each internode (P<0.01). SW was positively correlated with UIL, SIL, MSL, TIL, LI and TIBF. TIL and MSL had the highest correlation with lodging index (LI).
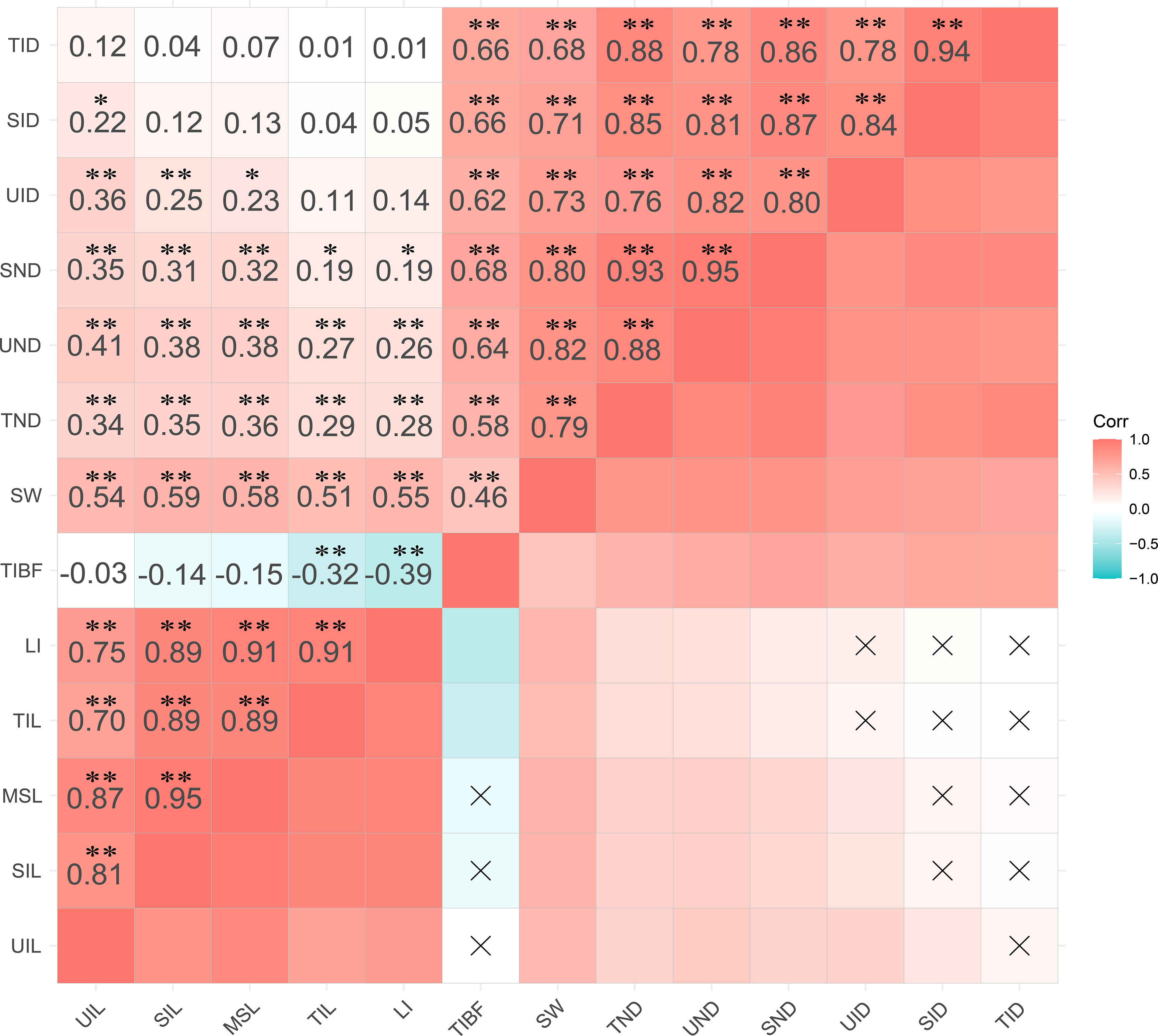
Figure 2 Pearson correlation coefficients among 13 stem traits of BLUP. The two-tailed t-test was applied to test the significance of correlation coefficients (*p < 0.05; **p < 0.01).
QTL analysis
In total 103 QTLs were mapped on all chromosomes of barley except 1H for stem related traits using the ICIM BIP module in 2021 and 2022, including UND (10 QTLS), SND (5), TND (7), UID (9), SID (13), TID (12), UIL (6), SIL (7), TIL (4), MSL (9), SW (6), TIBF (8) and LI (7) (Supplementary Table S1 and Figure 3A). Of them, the phenotypic variance of a single QTL was between 2.23% and 80.92%, with LOD values ranging from 3.01 to 32.68, 55(53.40%) major QTLs with PVE values greater than 10% were identified for UND (2 QTLS), SND (2), TND (3), UID (5), SID (6), TID (7), UIL (3), SIL (3), TIL (4), MSL (5), SW (4), TIBF (5) and LI (6) (Supplementary Table S1). 23 stable QTLs were detected for two consecutive years (Table 2), and 14 stable QTLs had PVE of more than 10% (two years average). Moreover, we identified 17 tightly linked or pleiotropic QTLs that influenced two or more traits, such as the QTL at 662.45-671.63 Mb on chromosome 2H simultaneously affected UIL, UND, UID, SID, TID and TIBF (Supplementary Table S2). To avoid the influence of row type (Rt), we used Rt as covariates for QTL mapping, a total of 91 QTLs were mapped, including 43 novel QTLs and 4 novel stable QTLs (Supplementary Table S3 and Table 2).
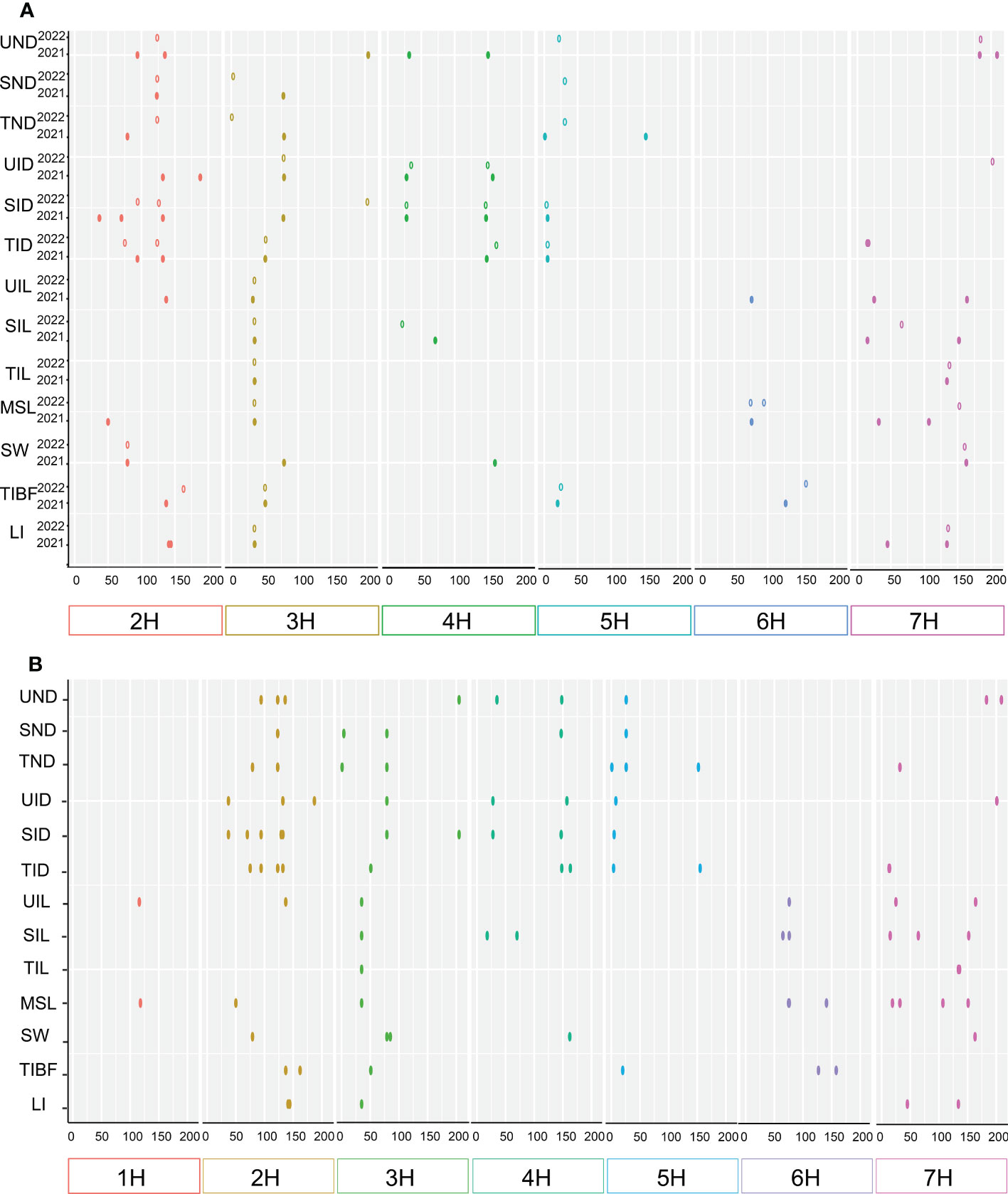
Figure 3 Chromosome distribution of QTLs associated with 13 stem related traits identified. (A) single-environment QTL analysis and (B) multi-environment trials (MET) analysis.
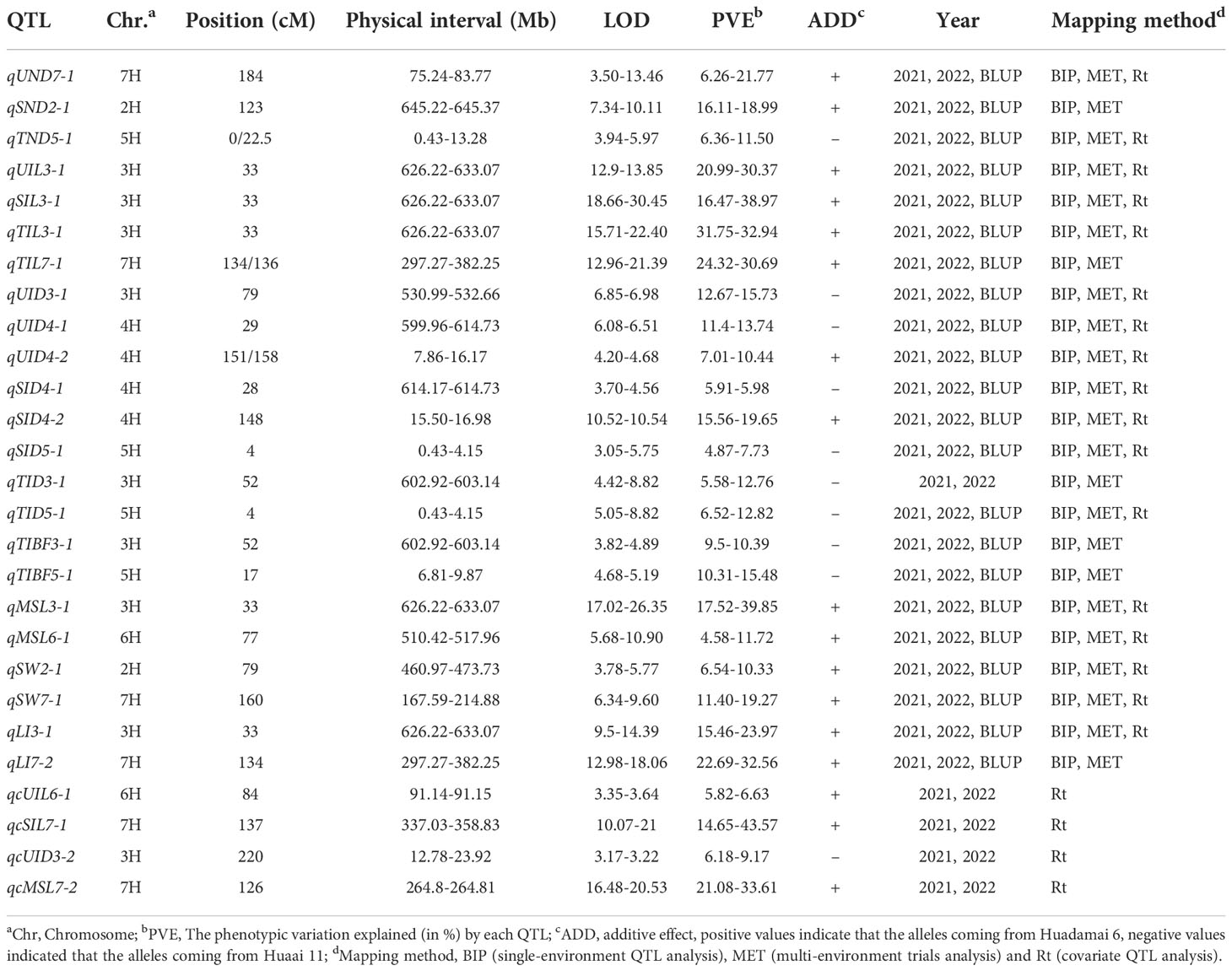
Table 2 Major and stable QTLs identified for thirteen stem related traits in two years using multiple mapping method.
In a multi-environment QTL analysis, 93 MET QTLs were identified for UND (9 QTLS), SND (5), TND (8), UID (8), SID (10), TID (10), UIL (6), SIL (8), TIL (3), MSL (10), SW (5), TIBF (6) and LI (5) (Figure 3B and Supplementary Table S4). Of them, 22 (23.66%) had PVE more than 10%. Besides, we found 21 QTL loci that influenced two or more traits synchronously detected in the ICIM MET module (Supplementary Table S5).
Further, to suppress the potential effect of the environment on stem lodging traits, we used the BLUP of stem traits for QTL analysis in the ICIM BIP module. 73QTLs were identified for UND (8 QTLS), SND (6), TND (8), UID (7), SID (7), TID (7), UIL (2), SIL (7), TIL (2), MSL (8), SW (5), TIBF (4) and LI (2) (Supplementary Table S6). Of them, the LOD values ranged from 3.03 to 32.23, and PVE of 26 (35.62%) QTLs was greater than 10%.
Most QTLs detected by the three mapping methods were located on chromosomes 2H, 3H, and 7H (Figure 4A). Figure 4B is the Venn diagram of the QTL mapped in three mapping methods. 45 QTLs were mapped by all three mapping methods, and 36 QTLs were identified in the MET module and the BIP module. The number of QTL for a single trait in each year was shown in Figure 4C. Importantly, we identified 27 major and stable QTLs (QTLs of one trait repeatedly mapped in multiple mapping methods and in at least two years) or 49 stable QTLs (QTLs of one trait repeatedly mapped in at least one year and BLUP) (Table 2 and Figure 5). The PVE of 49 stably QTLs was 3.79- 43.57%, with a LOD value from 3.01 to 32.68 (Supplementary Table S7). In addition, we integrated a large number of interlocking intervals for different traits. 22 regions were found on all chromosomes involving 288 QTLs, including 1, 6, 6, 3, 2, 1 and 3 intervals for chromosomes 1H, 2H, 3H, 4H, 5H, 6H and 7H, respectively (Table 3).
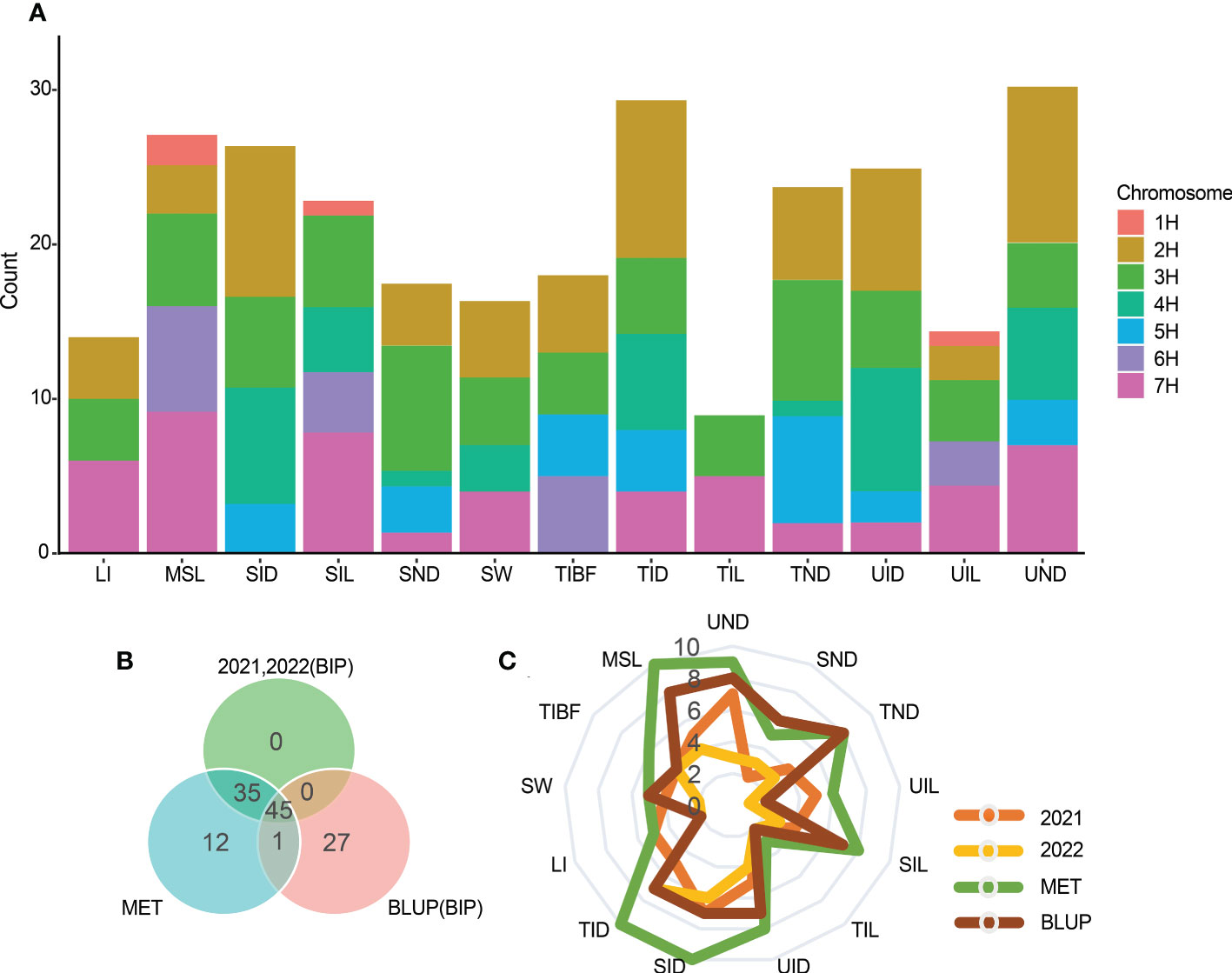
Figure 4 (A) Stacked map of QTL number of QTLs with 13 stem related traits identified on each chromosome. (B) Venn diagram of QTL detected by the three modes. (C) The number of QTL for a single trait in each year.
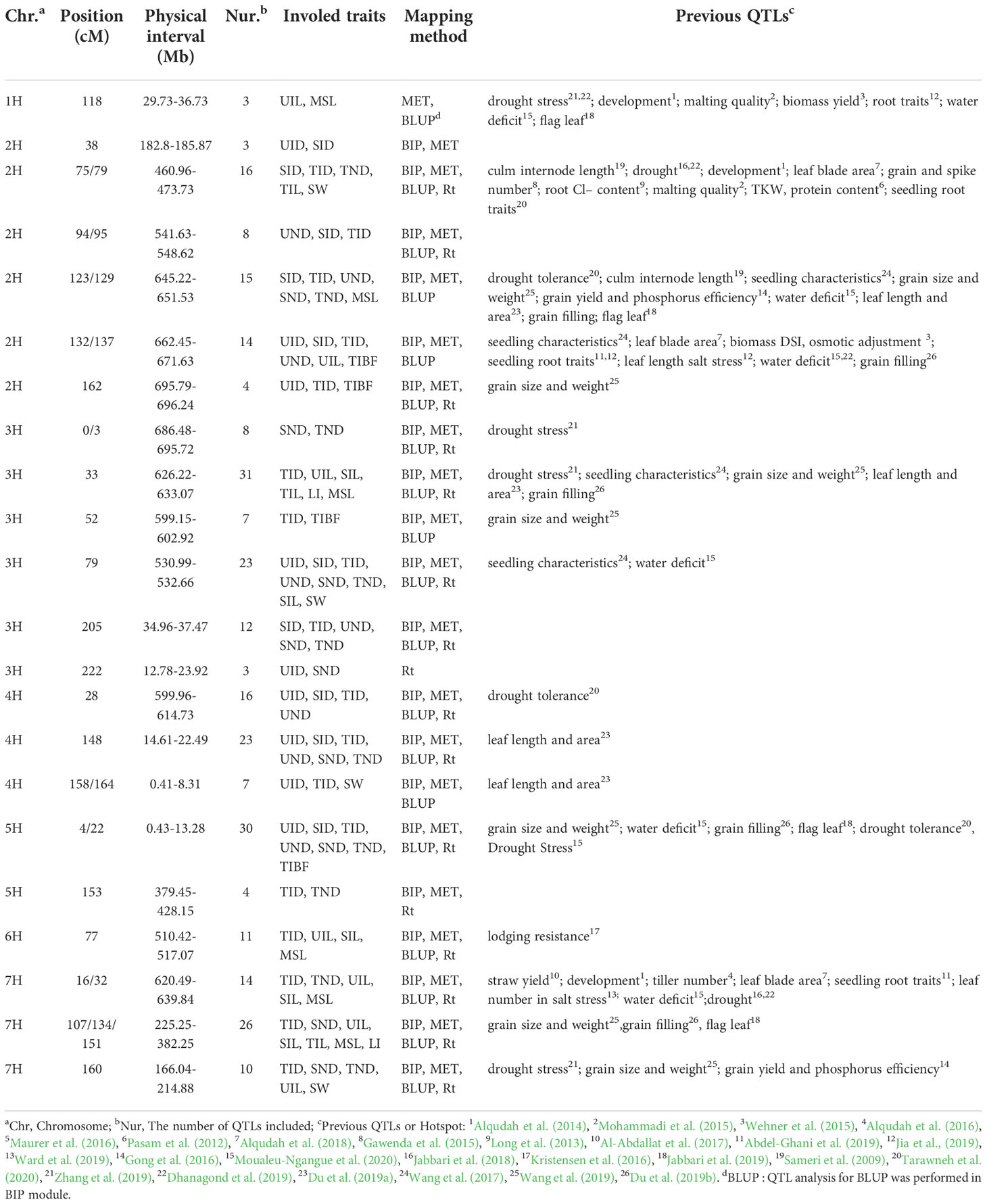
Table 3 Putative pleiotropy or linkage of QTLs on linkage groups in barley and other traits reported to be associated with these regions from the literatures.
Genes located within major QTLs intervals
In total, 49 stably QTLs were detected, and 26 stable QTLs had PVE of more than 10% (Supplementary Table S7). We explored a large number of interlocking intervals for different traits (Table 3), such as the QTL at 662.45-671.63 Mb on chromosomal 2H simultaneously affected UIL, UND, UID, SID, TID and TIBF. We reviewed annotated information of the markers and identified potential candidate genes (Supplementary Table S8), based on the barley physical map (Cantalapiedra et al., 2015). 44 genes were located at 460.97-473.73 Mb interval on chromosome 2H. A total of 147 genes were located at 626.22-633.07Mb and 530.99-532.66Mb on chromosome 3H. And 244 genes were found in the interval (599.96-614.73 Mb) on chromosome 4H. QTLs located at approximately 6.81-9.87 Mb on chromosome 5H contained 101 genes. The important intervals on chromosome 7H were 81.89-83.77Mb and 297.27-382.25 Mb, containing 271 genes. We also looked for genes in several other interlocking loci, which included 360, 273, 383, 203, 160, and 794 genes selected for chromosomes 2H, 3H, 4H, 5H, 6H and 7H, respectively.
Discussion
Major and linkage QTLs for stem traits
Most of the studies on lodging focused on plant height and stem chemical composition (JSameri et al., 2009; Ren et al., 2013; Ren et al., 2014; Wehner et al., 2015; Gong et al., 2016; Wendt et al., 2016; Al-Abdallat et al., 2017; Bélanger et al., 2018; Hu et al., 2018; Pu et al., 2021), on heading date focused on the change of agronomic traits under drought stress and panicle traits in barley (Pasam et al., 2012; Long et al., 2013; Alqudah et al., 2014; Gawenda et al., 2015; Mohammadi et al., 2015; Alqudah et al., 2016; Kristensen et al., 2016; Maurer et al., 2016; Alqudah et al., 2018; Jabbari et al., 2018; Abdel-Ghani et al., 2019; Jia et al., 2019; Ward et al., 2019; Jabbari et al., 2019; Moualeu-Ngangue et al., 2020), but few studies on QTL mapping of stem diameter of internode or node were reported.
In our study, 27 stable and major QTLs were identified for stem related traits (Table 2). To notarize whether the QTLs here are new loci, we compared the physical positions with those stem related loci reported previously, and found some QTLs for stem traits were consistent with QTLs for seedling traits or grain traits mapped in previous reports (Table 3). For instance, different traits were mapped previously in the interval of 122-129 cM and 131-137 cM on chromosome 2H (Wang et al., 2017; Du et al., 2019a; Wang et al., 2019; Du et al., 2019b). Our research also showed evidence to support the possible pleiotropy of the Vrs1 gene (Wang et al., 2016). Similarly, a large number of QTLs related to plant height related traits were also detected in the 626.22-633.07 Mb of chromosome 3H. In the same DH population, QTL for three internode length (Qith3-13) and heading date (Qhd3-13), were also near this region (Ren et al., 2013; Ren et al., 2014; Hu et al., 2018). In addition, in another hotspot on chromosome 5H at 0.43-13.28 Mb, the QTL of stem related traits we mapped was consistent with previously reported traits of grain filling rate or panicle related traits (Du et al., 2019b). These results showed certain correlation between barley stem and grain yield.
As expected, TIBF loci were mostly consistent with stem diameter loci, while LI loci were mostly consistent with internode length loci. In barley breeding, the relationship between traits should be considered, dwarf barley has lower grain yield due to its smaller biomass. We found that several interlocking intervals were simultaneously located by TID, TND, SND, MSL and LI (Table 3), which may be helpful to further explore the relationship between stem strength and plant height. QTL clusters of barley was iteratively reported (Qu et al., 2008; Schmalenbach and Pillen, 2009; Liu et al., 2015; Wang et al., 2016). The genetic mechanism of this general phenomenon might be gene linkage and pleiotropic effects in the same genomic region (Peng et al., 2003; Wang et al., 2015). Nevertheless, both linkage and pleiotropy need to be verified by further studies using cloning and fine mapping of gene or QTL.
Possible genes associated with barley stem development
Many genes have been shown to be pleiotropic in barley. Such as sdw1/denso controls grain size, grain yield, the number of tillers, and plant height (Kuczyńska et al., 2014). Annotating these genes in each stable QTL interval by KEGG database, we found some of them were related to cell division and plant development (Table 4). The three genes detected in the chromosome 6H interval at 510.42-517.07 Mb might involve in the stem length of barley. GS1a gene (HORVU6Hr1G074030) plays a role in nitrogen sensing or signaling and the efficiency of photosynthetic or water use (Molina-Rueda et al., 2013). RDRP1 gene (HORVU6Hr1G074220) and RDRP2 gene (HORVU6Hr1G074180) regulate organic acid and amino acid metabolites, biogenesis and spikelet development by small RNA (Song et al., 2012; Jha et al., 2021). Another significant QTL area underlying traits of UIL, SIL, TIL, MSL, LI on chromosome 3H at 626.22-633.07 Mb was physically close to HvGA20ox3 (HORVU3Hr1G089980) that encodes enzyme involved in gibberellin (GA) biosynthesis (Wendt et al., 2016; Bélanger et al., 2018). In addition, a region related to plant height traits was also detected on chromosome 7H at 297.27-382.25 Mb, the 14-3-3D gene (HORVU7Hr1G061920) and BRI1 gene (HORVU7Hr1G068990) located in the region plays an important intermediate in GA signal transduction and involves in the signaling of BRs (Pu et al., 2021), respectively. A stable QTL was detected near HvVRT-2 (a flowering repressor regulated by vernalization and photoperiod) on chromosome 7H (81.89-83.77 Mb), the gene maintains the transition from vegetative stage to reproductive stage, thereby influencing spikelet morphology and the internode development (Kane et al., 2005; Szűcs et al., 2006).
Comparative genomics has shown that the functions of homologs are generally conserved. In addition to the partially functional identification of genes in barley, we found several potential candidate genes within the markers (Table 4), that might play a key role in stem development. HORVU2Hr1G066890, HORVU4Hr1G006310, HORVU7Hr1G108580 and HORVU7Hr1G049340 annotate pyruvate dehydrogenase E2 component (Bohne et al., 2013), might coordinate the synthesis of lipids and proteins for the biogenesis of photosynthetic membranes. The gene SRP45 plays a role in chloroplast development in rice which is orthologs of the HORVU3Hr1G089450 (Zhang et al., 2013). HORVU2Hr1G093020 and HORVU4Hr1G006070 annotate beta-glucosidase, which is an important part of cellulase involved in various physiological processes in plants (Kawasaki et al., 2001). The GBE, a key enzyme in the catalytic regulation of α (1-6) glycosidic bond branch synthesis (Guan et al., 1995), annotated by HORVU7Hr1G111190. The gene (HORVU5Hr1G006350) that annotated as RHM (Saffer and Irish, 2018; Jiang et al., 2021), might be related to cell wall components. In addition, we identified several homologous genes associated with drought stress that might play a role in tiller and stem development. HORVU7Hr1G062120 annotates RBM25, an RNA binding protein in Arabidopsis thaliana that plays an important role in ABA-mediated alternative splicing and stress response (Cheng et al., 2017). YUCCA encodes a key auxin synthesis enzyme during drought stress of cotton (Wang et al., 2021), which is annotated by the HORVU7Hr1G067200. Another drought stress gene KCS encoded by HORVU2Hr1G094090 enhanced drought tolerance, increased the amount of chloroplast matrix, and increased stem diameter, stem coat thickness, growth rate, and lignin content in jute (Zhang et al., 2019; Tong et al., 2021). Moreover, two potential candidate genes (HORVU4Hr1G076460 and HORVU4Hr1G076250) were found next to each other on 4H, HORVU4Hr1G076460 encodes RBOH, DNA methylation of genes related to seed development affected by heat stress during grain filling (Mahalingam et al., 2021; Sakai et al., 2022). Brassinosteroid signaling kinases (BSK) encoded by HORVU4Hr1G076250 is a key family of receptor-like cytoplasmic kinases for BR signaling (Li et al., 2022), which is crucial for the development of plants, immunity and abiotic stress response, but these genes still are needed further verification in barley. Our studies showed that candidate genes may be involved in stem development and provide clues for further fine positioning.
Stem traits for barley breeding programs
In this study, we measured the breaking force of the third internode and calculated the lodging index to observe the relationship between the traits after 28 days of heading (Figure 2). The results showed that TIBF was negatively correlated with LI and TIL. Compared with the strength trait, the correlation between LI and plant height trait was highly significant. All the traits were significant and positively correlated with SW. And the diameter of each stem node was positively correlated with the stem diameter of each internode. SIL and TIL had no correlation with SID and TID, a similar phenomenon occurs in rice (Guo et al., 2021), suggesting that improving stem strength might not change plant height.
Stem strength can be affected by various factors. The leaf sheath could provide great physical support (Cornwall et al., 2021). Lodging resistance is usually related to stem diameter. Simonneau et al. (1993) showed that during drought stress stem diameters contract in response to changes in internal water status. Therefore, it can be used as a selection criterion for stress tolerance (Sallam et al., 2015). In our study, the finding is reflected in the consistency of QTL loci. Then, the study of the genetic basis of stem development may help us to fight global climate change.
Collectively, because of the importance of plant height and stem diameter in lodging resistance, stress resistance, grain yield, and photosynthate transport in barley, a stem with a suitable height and strength is usually preferred in barley breeding.
Conclusions
In this study, 27 stable and major QTLs, and 22 QTL clustered regions were identified for 13 stem related traits. We also methodically compared the genetic correspondence with other different traits at the same locus. In total, 22 genes were identified as promising candidates associated with plant development and adverse stress, which were closely related to lodging resistance, stress resistance, photosynthate transport and grain development in barley. The identification of QTL conferring stem related traits can help us to know the genetic basis of stem and ameliorate lodging resistance potential of barley, and is useful in future marker-assisted barley breeding programs.
Data availability statement
The original contributions presented in the study are included in the article/Supplementary Material. Further inquiries can be directed to the corresponding author.
Author contributions
XR and AZ conceived the study and worked on the approval of the manuscript. AZ, XH, TZ, HP and YA performed the experiments. AZ wrote the first draft. DS, GS, CL and XR revised the manuscript. AZ and YZ contributed to data analysis and managed reagents. All authors contributed to the article and approved the submitted version.
Funding
This research was supported by the earmarked fund for China Agriculture Research System (CARS-5).
Conflict of interest
The authors declare that the research was conducted in the absence of any commercial or financial relationships that could be construed as a potential conflict of interest.
Publisher’s note
All claims expressed in this article are solely those of the authors and do not necessarily represent those of their affiliated organizations, or those of the publisher, the editors and the reviewers. Any product that may be evaluated in this article, or claim that may be made by its manufacturer, is not guaranteed or endorsed by the publisher.
Supplementary material
The Supplementary Material for this article can be found online at: https://www.frontiersin.org/articles/10.3389/fpls.2022.1063988/full#supplementary-material
References
Abdel-Ghani, A. H., Sharma, R., Wabila, C., Dhanagond, S., Owais, S. J., Duwayri, M. A., et al. (2019). Genome-wide association mapping in a diverse spring barley collection reveals the presence of QTL hotspots and candidate genes for root and shoot architecture traits at seedling stage. BMC Plant Biol. 19, 216. doi: 10.1186/s12870-019-1828-5
Al-Abdallat, A. M., Karadsheh, A., Hadadd, N. I., Akash, M. W., Ceccarelli, S., Baum, M., et al. (2017). Assessment of genetic diversity and yield performance in Jordanian barley (Hordeum vulgare l.) landraces grown under rainfed conditions. BMC Plant Biol. 17, 191. doi: 10.1186/s12870-017-1140-1
Alqudah, A. M., Koppolu, R., Wolde, G. M., Graner, A., Schnurbusch, T. (2016). The genetic architecture of barley plant stature. Front. Gene. 7. doi: 10.3389/fgene.2016.00117
Alqudah, A. M., Sharma, R., Pasam, R. K., Graner, A., Kilian, B., Schnurbusch, T. (2014). Genetic dissection of photoperiod response based on GWAS of pre-anthesis phase duration in spring barley. PloS One 9, 11. doi: 10.1371/journal.pone.0113120
Alqudah, A. M., Youssef, H. M., Graner, A., Schnurbusch, T. (2018). Natural variation and genetic make-up of leaf blade area in spring barley. Theor. Appl. Genet. 131, 873–886. doi: 10.1007/s00122-018-3053-2
Baker, C. J., Sterling, M., Berry, P. (2014). A generalised model of crop lodging. J. Theor. Biol. 363, 1–12. doi: 10.1016/j.jtbi.2014.07.032
Bélanger, S., Paquet-Marceau, S., Díaz Lago, J. E., Belzile, F. (2018). QTL mapping uncovers a semi-dwarf 1 (sdw1) allele in the barley (Hordeum vulgare) ND23049 line. Genome 61, 429–436. doi: 10.1139/gen-2017-0211
Bellucci, A., Tondelli, A., Fangel, J. U., Torp, A. M., Xu, X., Willats, W. G. T., et al. (2017). Genome-wide association mapping in winter barley for grain yield and culm cell wall polymer content using the high-throughput CoMPP technique. PloS One 12, e0173313. doi: 10.1371/journal.pone.0173313
Berry, P. M., Sterling, M., Spink, J. H., Baker, C. J., Sylvester-Bradley, R., Mooney, S. J., et al. (2004). Understanding and reducing lodging in cereals. Adv. Agron. 84, 217–271. doi: 10.1016/S0065-2113(04)84005-7
Bohne, A. V., Schwarz, C., Schottkowski, M., Lidschreiber, M., Piotrowski, M., Zerges, W., et al. (2013). Reciprocal regulation of protein synthesis and carbon metabolism for thylakoid membrane biogenesis. PloS Biol. 11, e1001482. doi: 10.1371/journal.pbio.1001482
Caier̄ao, E. (2006). Effect of induced lodging on grain yield and quality of brewing barley. Crop Breed. Appl. Biot. 6, 215–221. doi: 10.12702/1984-7033.v06n03a05
Cantalapiedra, C. P., Boudiar, R., Casas, A. M., Igartua, E., Contreras-Moreira, B. (2015). BARLEYMAP: physical and genetic mapping of nucleotide sequences and annotation of surrounding loci in barley. Mol. Breed. 35, 13. doi: 10.1007/s11032-015-0253-1
Cheng, C. H., Wang, Z. J., Yuan, B. J., Li, X. (2017). RBM25 mediates abiotic responses in plants. Front. Plant Sci. 8. doi: 10.3389/fpls.2017.00292
Chen, S. Q., Wang, Y., Zhang, Z. H., Wang, L. X., Hu, Z. L., Li, P., et al. (2004). Genetic dissection of vascular bundle systems in peduncle and panicle characters in rice (Oryza sativa l.) by means of RFLP markers. J. Wuhan Botanical Res. 22, 15–21.
Cornwall, J., Stubbs, C. J., McMahan, C. S., Robertson, D. J. (2021). The overlooked biomechanical role of the clasping leaf sheath in wheat stalk lodging. Front. Plant Sci. 12, 617880. doi: 10.3389/fpls.2021.617880
Cui, H. L., Shen, H. S. (2011). Modelling and simulation of buckling and post buckling of plant stems under combined loading conditions. Int. J. Appl. Mech. 3, 119–130. doi: 10.1142/S1758825111000907
Dhanagond, S., Liu, G., Zhao, Y., Chen, D., Grieco, M., Reif, J., et al (2019). Non-invasive phenotyping reveals genomic regions involved in preanthesis drought tolerance and recovery in spring barley. Front. Plant Sci. 10, 1307. doi: 10.3389/fpls.2019.01307
Du, B. B., Liu, L. P., Wang, Q. F., Sun, G. L., Ren, X. F., Li, C. D., et al. (2019a). Identification of QTL underlying the leaf length and area of different leaves in barley. Sci. Rep. 9, 4431. doi: 10.1038/s41598-019-40703-6
Du, B. B., Wang, Q. F., Sun, G. L., Ren, X. F., Cheng, Y., Wang, Y. X., et al. (2019b). Mapping dynamic QTL dissects the genetic architecture of grain size and grain filling rate at different grain-filling stages in barley. Sci. Rep. 9, 18823. doi: 10.1038/s41598-019-53620-5
Feng, J. Y., Zhang, J., Wen, Y. J., Zhang, Y. M., Zhang, Y. W., Dunwell, J. M. (2018). An efficient multi-locus mixed model framework for the detection of small and linked QTLs in F2. Brief. Bioinform. 20, 1913–1924. doi: 10.1093/bib/bby058
Gawenda, I., Thorwarth, P., Günther, T., Ordon, F., Schmid, K. J. (2015). Genome-wide association studies in elite varieties of German winter barley using single-marker and haplotype-based methods. Plant Breed. 134, 28–39. doi: 10.1111/pbr.12237
Gong, X., Wheeler, R., Bovill, W. D., Mcdonald, G. K. (2016). QTL mapping of grain yield and phosphorus efficiency in barley in a Mediterranean-like environment. Theor. Appl. Genet. 129, 1657–1672. doi: 10.1007/s00122-016-2729-8
Guan, H., Kuriki, T., Sivak, M., Preiss, J. (1995). Maize branching enzyme catalyzes synthesis of glycogen-like polysaccharide in glgB-deficient escherichia coli. P. Natl. Acad. Sci. U.S.A. 92, 964–967. doi: 10.1073/pnas.92.4.964
Guo, Z. L., Liu, X., Zhang, B., Yuan, X. J., Xing, Y. Z., Liu, H. Y., et al. (2021). Genetic analyses of lodging resistance and yield provide insights into post-Green-Revolution breeding in rice. Plant Biotechnol. J. 19, 814–829. doi: 10.1111/pbi.13509
Hedden, P. (2003). The genes of the green revolution. Trends Genet. 19, 5–9. doi: 10.1016/S0168-9525(02)00009-4
Housley, T. L., Peterson, D. M. (1982). Oat stem vascular size in relation to kernel number and weight. I controlled environment I. Crop Sci. 22, 259–263. doi: 10.2135/cropsci1982.0011183X002200020014x
Houston, K., Burton, R. A., Sznajder, B., Rafalski, A. J., Dhugga, K. S., Mather, D. E., et al. (2015). A GenomeWide association study for culm cellulose content in barley reveals candidate genes Co-expressed with members of the CELLULOSE SYNTHASE a gene family. PloS One 10, e0130. doi: 10.1371/journal.pone.0130890
Huang, C., Chen, Q. Y., Xu, G. H., Xu, D. Y., Tian, J. G., Tian, F. (2016). Identification and fine mapping of quantitative trait loci for the number of vascular bundle in maize stem. J. Integr. Plant Biol. 58, 81–90. doi: 10.1111/jipb.12358
Hu, X., Zuo, J. F., Wang, J. B., Liu, L. P., Sun, G. L., Li, C. D., et al. (2018). Multi-locus genome-wide association studies for 14 main agronomic traits in barley. Front. Plant Sci. 9. doi: 10.3389/fpls.2018.01683
Islam, M. S., Peng, S. B., Visperas, R. M., Ereful, N., Bhuiya, M. S. U., Julfiquar, A. (2007). Lodging-related morphological traits of hybrid rice in a tropical irrigated ecosystem. Field Crop Res. 101, 240–248. doi: 10.1016/j.fcr.2006.12.002
Jabbari, M., Fakheri, B. A., Aghnoum, R., Nezhad, N. M., Ataei, R. (2018). GWAS analysis in spring barley (Hordeum vulgare l.) for morphological traits exposed to drought. PloS One 13, e020. doi: 10.1371/journal.pone.0204952
Jabbari, M., Fakheri, B. A., Aghnoum, R., Nezhad, N. M., Ataei, R., Koochakpour, Z. (2019). Association mapping of morphological and physiological traits of flag leaf related to drought tolerance in barley. Rev. Agricultura Neotropical. 67, 7–18. doi: 10.32404/rean.v6i2.3323
Jha, V., Narjala, A., Basu, D., Sujith, T. N., Pachamuthu, K., Chenna, S., et al. (2021). Essential role of γ-clade RNA-dependent RNA polymerases in rice development and yield-related traits is linked to their atypical polymerase activities regulating specific genomic regions. New Phytol. 232, 1674–1691. doi: 10.1111/nph.17700
Jia, Z. T., Liu, Y., Gruber, B. D., Neumann, K., Kilian, B., Graner, A., et al. (2019). Genetic dissection of root system architectural traits in spring barley. Front. Plant Sci. 10, 400. doi: 10.3389/fpls.2019.00400
Jiang, N., Dillon, F. M., Silva, A., Gomez-Cano, L., Grotewold, E. (2021). Rhamnose in plants - from biosynthesis to diverse functions. Plant Sci. 302, 110687. doi: 10.1016/j.plantsci.2020.110687
Jones, L., Ennos, A. R., Turner, S. R. (2001). Cloning and characterization of irregular xylem4 (irx4): a severely lignin-deficient mutant of arabidopsis. Plant J. 26, 205–216. doi: 10.1046/j.1365-313x.2001.01021.x
Kane, N. A., Danyluk, J., Tardif, G., Ouellet, F., Laliberte, J. F., Limin, A. E., et al. (2005). TaVRT-2, a member of the StMADS-11 clade of flowering repressors, is regulated by vernalization and photoperiod in wheat. Plant Physiol. 138, 2354–2363. doi: 10.1104/pp.105.061762
Kashiwagi, T., Sasaki, H., Ishimaru, K. (2005). Factors responsible for decreasing sturdiness of the lower part in lodging of rice. Plant Prod. Sci. 8, 166–172. doi: 10.1626/pps.8.166
Kawasaki, S., Borchert, C., Deyholos, M., Wang, H., Brazille, S., Kawai, K., et al. (2001). Gene expression profiles during the initial phase of salt stress in rice. Plant Cell. 13, 889–905. doi: 10.1105/tpc.13.4.889
Khobra, R., Sareen, S., Meena, B. K., Kumar, A., Tiwari, V., Singh, G. P. (2019). Exploring the traits for lodging tolerance in wheat genotypes: a review. Physiol. Mol. Biol. Pla. 25, 589–600. doi: 10.1007/s12298-018-0629-x
Kristensen, P. S., Dockter, C., Lundqvist, U., Lu, Q., Gregersen, P., Thordal-Christensen, H., et al. (2016). Genetic mapping of the barley lodging resistance locus Erectoides-k. Plant Breed. 135, 420–428. doi: 10.1111/pbr.12377
Kuczyńska, A., Mikołajczak, K., Ćwiek, H. (2014). Pleiotropic effects of the sdw1 locus in barley populations representing different rounds of recombination. Electron. J. Biotechn. 17, 217–223. doi: 10.1016/j.ejbt.2014.07.005
Leblicq, T., Smeets, B., Vanmaercke, S., Ramon, H., Saeys, W. (2016). A discrete element approach for modelling bendable crop stems. Comput. Electron. Agr. 124, 141–149. doi: 10.1016/j.compag.2016.03.022
Lin, H., Guo, H. J., Xiao, S. H., Jiang, G. L., Zhang, X. Y., Yan, C. X., et al. (2005). Quantitative trait loci (QTL) of stem strength and related traits in a doubled-haploid population of wheat (Triticum aestivum l.). Euphytica 141, 1–9. doi: 10.1007/s10681-005-4713-2
Liu, L. P., Sun, G. L., Ren, X. F., Li, C. D., Sun, D. F. (2015). Identification of QTL underlying physiological and morphological traits of flag leaf in barley. BMC Genet. 16, 29. doi: 10.1186/s12863-015-0187-y
Liu, H. J., Wang, X. Q., Xiao, Y. J., Luo, J. Y., Qiao, F., Yang, W. Y., et al. (2020). CUBIC: an atlas of genetic architecture promises directed maize improvement. Genome Biol. 21, 20. doi: 10.1186/s13059-020-1930-x
Li, F. C., Xie, G. S., Huang, J. F., Zhang, R., Li, Y., Zhang, M. M., et al. (2017). OsCESA9 conserved-site mutation leads to largely enhanced plant lodging resistance and biomass enzymatic saccharification by reducing cellulose DP and crystallinity in rice. Plant Biotechnol. J. 15, 1093–1104. doi: 10.1111/pbi.12700
Li, Y., Zhang, H., Zhang, Y. X., Liu, Y. S., Li, Y. Y., Tian, H. D., et al. (2022). Genome-wide identifcation and expression analysis reveals spinach brassinosteroid-signaling kinase (BSK) gene family functions in temperature stress response. BMC Genomics 23, 453. doi: 10.1186/s12864-022-08684-5
Long, N. V., Dolstra, O., Malosetti, M., Kilian, B., Graner, A., Visser, R. G. F., et al. (2013). Association mapping of salt tolerance in barley (Hordeum vulgare l.). Theor. Appl. Genet. 126, 2335–2351. doi: 10.1007/s00122-013-2139-0
Mahalingam, R., Graham, D., Walling, J. G. (2021). The barley (Hordeum vulgare ssp. vulgare) respiratory burst oxidase homolog (HvRBOH) gene family and their plausible role on malting quality. Front. Plant Sci. 12. doi: 10.3389/fpls.2021.608541
Martinez-Vazquez, P. (2016). Crop lodging induced by wind and rain. Agr. For. Meteorol. 228, 266–275. doi: 10.1016/j.agrformet.2016.07.003
Mascher, M., Gundlach, H., Himmelbach, A., Beier, S., Twardziok, S. O., Wicker, T., et al. (2017). A chromosome conformation capture ordered sequence of the barley genome. Nature 544, 427–433. doi: 10.1038/nature22043
Matthias, J., Shin, T., Martin, M., Axel, H., Takahisa, Y., Fahimeh, S., et al. (2016). A homolog of Blade-On-Petiole 1 and 2 (BOP1/2) controls internode length and homeotic changes of the barley inflorescence. Plant Physiol. 171, 1113–1127. doi: 10.1104/pp.16.00124
Maurer, A., Draba, V., Pillen, K. (2016). Genomic dissection of plant development and its impact on thousand grain weight in barley through nested association mapping. J. Exp. Bot. 67, 2507–2518. doi: 10.1093/jxb/erw070
McFarlane, H. E., Döring, A., Persson, S. (2014). The cell biology of cellulose synthesis. Annu. Rev. Plant Biol. 65, 69–94. doi: 10.1146/annurev-arplant-050213-040240
Mohammadi, M., Blake, T. K., Budde, A. D., Chao, S. M., Hayes, P. M., Horsley, R. D., et al. (2015). A genome-wide association study of malting quality across eight US barley breeding programs. Theor. Appl. Genet. 128, 705–721. doi: 10.1007/s00122-015-2465-5
Molina-Rueda, J. J., Tsai, C. J., Kirby, E. G. (2013). The Populus superoxide dismutase gene family and its responses to drought stress in transgenic poplar overexpressing a pine cytosolic glutamine synthetase (GS1a). PloS One 8, e56421. doi: 10.1371/journal.pone.0056421
Moualeu-Ngangue, D., Dolch, C., Schneider, M., Leon, J., Uptmoor, R., Stutzel, H., et al (2020). Physiological and morphological responses of different spring barley genotypes to water deficit and associated QTLs. PloS One 15, e0237834. doi: 10.1371/journal.pone.0237834
Pasam, R. K., Sharma, R., Malosetti, M., van Eeuwijk, F. A., Haseneyer, G., Kilian, B., et al. (2012). Genome-wide association studies for agronomical traits in a world wide spring barley collection. BMC Plant Biol. 12, 16. doi: 10.1186/1471-2229-12-16
Peng, J., Ronin, Y., Fahima, T., Röder, M. S., Li, Y., Nevo, E., et al. (2003). Domestication quantitative trait loci in Triticum dicoccoides, the progenitor of wheat. P. Natl. Acad. Sci. U.S.A. 100, 2489–2494. doi: 10.1073/pnas.252763199
Pinthus, M. J. (1974). Lodging in wheat, barley, and oats: The phenomenon, its causes, and preventive measures. Adv. Agron. 25, 209–263. doi: 10.1016/S0065-2113(08)60782-8
Pu, X., Tang, Y. Y., Zhang, M. H., Li, T., Qiu, X. B., Zhang, J. Y., et al. (2021). Identification and candidate gene mining of HvSS1, a novel qualitative locus on chromosome 6H, regulating the uppermost internode elongation in barley (Hordeum vulgare l.). Theor. Appl. Genet. 134, 2481–2494. doi: 10.1007/s00122-021-03837-8
Qu, Y. Y., Mu, P., Zhang, H. J., Chen, C. Y., Gao, Y. M., Tian, Y. X., et al. (2008). Mapping QTLs of root morphological traits at different growth stages in rice. Genetica 133, 187–200. doi: 10.1007/s10709-007-9199-5
Ren, X. F., Sun, D. F., Dong, W. B., Sun, G. L., Li, C. D. (2014). Molecular detection of QTL controlling plant height components in a doubled haploid barley population. Genet. Mol. Res. 13, 3089–3099. doi: 10.4238/2014.April.17.5
Ren, X. F., Sun, D. F., Guan, W. W., Sun, G. L., Li, C. D. (2010). Inheritance and identification of molecular markers associated with a novel dwarfing gene in barley. BMC Genet. 11, 89. doi: 10.1186/1471-2156-11-89
Ren, X. F., Sun, D. F., Sun, G. L., Li, C. D., Dong, W. B. (2013). Molecular detection of QTL for agronomic and quality traits in a doubled haploid barley population. Aust. J. Crop Sci. 7, 878–886. doi: 10.3316/informit.365096994848047
Ren, X. F., Wang, J. B., Liu, L. P., Sun, G. L., Li, C. D., Luo, H., et al. (2016). SNP-based high density genetic map and mapping of btwd1 dwarfing gene in barley. Sci. Rep. 6, 31741. doi: 10.1038/srep31741
Saffer, A. M., Irish, V. F. (2018). Flavonol rhamnosylation indirectly modifies the cell wall defects of RHAMNOSE BIOSYNTHESIS1 mutants by altering rhamnose flux. Plant J. 94, 649–660. doi: 10.1111/tpj.13885
Sakai, Y., Suriyasak, C., Inoue, M., Hamaoka, N., Ishibashi, Y. (2022). Heat stress during grain filling regulates seed germination through alterations of DNA methylation in barley (Hordeum vulgare l.). Plant Mol. Biol 110, 325–332. doi: 10.1007/s11103-022-01278-5
Sallam, A., Hashad, M., Hamed, E. S., Omara, M. (2015). Genetic variation of stem characters in wheat and their relation to kernel weight under drought and heat stresses. J. Crop Sci. Biotech. 18, 137–146. doi: 10.1007/s12892-015-0014-z
Sameri, M., Nakamura, S., Nair, S. K., Takeda, K., Komatsuda, T. (2009). A quantitative trait locus for reduced culm internode length in barley segregates as a Mendelian gene. Theor. Appl. Genet. 118, 643–652. doi: 10.1007/s00122-008-0926-9
Schmalenbach, I., Pillen, K. (2009). Detection and verification of malting quality QTLs using wild barley introgression lines. Theor. Appl. Genet. 118, 1411–1427. doi: 10.1007/s00122-009-0991-8
Shah, A. N., Tanveer, M., Rehman, A. U., Anjum, S. A., Iqbal, J., Ahmad, R. (2017). Lodging stress in cereal effects and management: an overview. Environ. Sci. pollut. R. Int. 24, 5222–5237. doi: 10.1007/s11356-016-8237-1
Shah, L., Yahya, M., Shah, S., Nadeem, M., Ali, A., Wang, J., et al. (2019). Improving lodging resistance: using wheat and rice as classical examples. Int. J. Mol. Sci. 20, 4211. doi: 10.3390/ijms20174211
Simonneau, T., Habib, R., Goutouly, J. P., Huguet, J. G. (1993). Diurnal changes in stem diameter depend upon variations in water content: Direct evidence in peach trees. J. Exp. Bot. 44, 615–621. doi: 10.1093/jxb/44.3.615
Song, X. W., Wang, D. K., Ma, L. J., Chen, Z. Y., Li, P. C., Cui, X., et al. (2012). Rice RNA-pendent RNA polymerase 6 acts in small RNA biogenesis and spikelet development. Plant J. 71, 378–389. doi: 10.1111/j.1365-313X.2012.05001.x
Szuűcs, P., Karsai, I., von Zitzewitz, J., Mészáros, K., Cooper, L. L. D., Gu, Y. Q., et al. (2006). Skinner Positional relationships between photoperiod response QTL and photoreceptor and vernalization genes in barley. Theor. Appl. Genet. 112, 1277–1285. doi: 10.1007/s00122-006-0229-y
Tarawneh, R. A., Alqudah, A. M., Nagel, M., Börner Andreas, Andreas. (2020). Genome-wide association mapping reveals putative candidate genes for drought tolerance in barley. Environ. Exp. Bot. 180, 104237. doi: 10.1016/j.envexpbot.2020.104237
Tong, T., Fang, Y. X., Zhang, Z. L., Zheng, J. J., Zhang, X., Li, J., et al. (2021). Genome-wide identifcation and expression pattern analysis of the KCS gene family in barley. Plant Growth Regul. 93, 89–103. doi: 10.1007/s10725-020-00668-3
Wang, X. Y., Chen, B. Z., Ma, C. K., Qiao, K. K., Li, Z. S., Wang, J. S., et al. (2021). Systematical characterization of YUCCA gene family in five cotton species, and potential functions of YUCCA22 gene in drought resistance of cotton. Ind. Crop Prod. 162, 113290. doi: 10.1016/j.indcrop.2021.113290
Wang, H. T., Huang, C., Guo, H. L., Li, X. M., Zhao, W. X., Dai, B. S., et al. (2015). QTL mapping for fiber and yield traits in upland cotton under multiple environments. PloS One 10, e0130742. doi: 10.1371/journal.pone.0130742
Wang, Q. F., Sun, G. L., Ren, X. F., Du, B. B., Cheng, Y., Wang, Y. X., et al. (2019). Dissecting the genetic basis of grain size and weight in barley (Hordeum vulgare l.) by QTL and comparative genetic analyses. Front. Plant Sci. 10. doi: 10.3389/fpls.2019.00469
Wang, J. B., Sun, G. L., Ren, X. F., Li, C. D., Liu, L. P., Wang, Q. F., et al. (2016). QTL underlying some agronomic traits in barley detected by SNP markers. BMC Genet. 17, 103. doi: 10.1186/s12863-016-0409-y
Wang, Q. F., Sun, G. L., Ren, X. F., Wang, J. B., Du, B. B., Li, C. D., et al. (2017). Detection of QTLs for seedling characteristics in barley (Hordeum vulgare l.) grown under hydroponic culture condition. BMC Genet. 18, 94. doi: 10.1186/s12863-017-0562-y
Ward, B., Brien, C., Oakey, H., Pearson, A., Negrão, S., Schilling, R. K., et al. (2019). High-throughput 3D modelling to dissect the genetic control of leaf elongation in barley (Hordeum vulgare). Plant J. 98, 555–570. doi: 10.1111/tpj.14225
Wehner, G., Balko, C., Enders, M., Humbeck, K., Ordon, F. (2015). Identification of genomic regions involved in tolerance to drought stress and drought stress induced leaf senescence in juvenile barley. BMC Plant Biol. 15, 125. doi: 10.1186/s12870-015-0524-3
Wendt, T., Holme, I., Dockter, C., Preuß, A., Thomas, W., Druka, A., et al. (2016). HvDep1 is a positive regulator of culm elongation and grain size in barley and impacts yield in an environment-dependent manner. PloS One 11, 12. doi: 10.1371/journal.pone.0168924
Wu, W., Ma, B. L. (2016). A new method for assessing plant lodging and the impact of management options on lodging in canola crop production. Sci. Rep. 6, 31890. doi: 10.1038/srep31890
Wu, W., Ma, B. L. (2020). The mechanical roles of the clasping leaf sheath in cereals: two case studies from oat and wheat plants. J. Agron. Crop Sci. 206, 118–129. doi: 10.1111/jac.12362
Yap, T. C., Harvey, B. L. (1972). Relations between grain yield and photosynthetic parts above the flag leaf node in barley. Can. J. Plant Sci. 52, 241–246. doi: 10.4141/cjps72-037
Zhai, L. Y., Zheng, T. Q., Wang, X. Y., Wang, Y., Chen, K., Wang, S., et al. (2018). QTL mapping and candidate gene analysis of peduncle vascular bundle related traits in rice by genome-wide association study. Rice 11, 13. doi: 10.1186/s12284-018-0204-7
Zhang, F. T., Luo, X. D., Hu, B. L., Wan, Y., Xie, J. K. (2013). YGL138(t), encoding a putative signal recognition particle 54 kDa protein, is involved in chloroplast development of rice. Rice 6, 7. doi: 10.1186/1939-8433-6-7
Keywords: barley (Hordeum vulgare L.), internode length, stem diameter, lodging resistance, quantitative trait loci (QTL)
Citation: Zhang A, Zhao T, Hu X, Zhou Y, An Y, Pei H, Sun D, Sun G, Li C and Ren X (2022) Identification of QTL underlying the main stem related traits in a doubled haploid barley population. Front. Plant Sci. 13:1063988. doi: 10.3389/fpls.2022.1063988
Received: 07 October 2022; Accepted: 14 November 2022;
Published: 01 December 2022.
Edited by:
Hongwei Wang, Shandong Agricultural University, ChinaReviewed by:
Meixue Zhou, University of Tasmania, AustraliaHong Luo, Clemson University, United States
Copyright © 2022 Zhang, Zhao, Hu, Zhou, An, Pei, Sun, Sun, Li and Ren. This is an open-access article distributed under the terms of the Creative Commons Attribution License (CC BY). The use, distribution or reproduction in other forums is permitted, provided the original author(s) and the copyright owner(s) are credited and that the original publication in this journal is cited, in accordance with accepted academic practice. No use, distribution or reproduction is permitted which does not comply with these terms.
*Correspondence: Xifeng Ren, cmVueGlmZW5nQG1haWwuaHphdS5lZHUuY24=