- 1Key Laboratory of Agro-Environment in the Tropics, Ministry of Agriculture and Rural Affairs, South China Agricultural University, Guangzhou, China
- 2Guangdong Provincial Key Laboratory of Eco-Circular Agriculture, South China Agricultural University, Guangzhou, China
- 3Guangdong Engineering Research Centre for Modern Eco-Agriculture, South China Agricultural University, Guangzhou, China
- 4College of Natural Resources and Environment, South China Agricultural University, Guangzhou, China
Low temperatures are among the most commonly encountered environmental conditions that adversely affect plant growth and development, leading to substantial reductions in crop productivity. Plants have accordingly evolved coordinated mechanisms that confer low-temperature adaptation and resistance. The plant metabolic network, including polyamines (PAs) and γ-aminobutyric acid (GABA) is reprogrammed to ensure that essential metabolic homeostasis is maintained in response to cold stress conditions. Additionally, GABA might serve as a central molecule in the defense system during low-temperature tolerance in plants. However, our understanding of how these metabolites function in conferring cold tolerance is still far from complete. Here, we summarized how PAs and GABA function in conferring cold tolerance, and describe the crucial role of GABA in the mitigation of ROS during cold stress in plants.
Introduction
Low-temperature stress has two distinct components: chilling, which is generally defined as lower-than-normal and higher than 0°C growth temperatures, and freezing, which indicates temperatures below 0°C (Raju et al., 2018). The molecular mechanisms underlying low-temperature tolerance have been extensively studied. Several signaling pathways and cold-responsive genes have been characterized and identified in different species, including those from the ICE-CBF-COR transcriptional cascade. Emerging evidence has also indicated that several metabolites, such as polyamines (PAs) and γ-aminobutyric acid (GABA), play pivotal roles in alleviating the damage caused by low temperatures in different plant species (Baier et al., 2019).
Reprogramming of plant metabolism in response to low temperature
Plants, as sessile organisms, have evolved processes that confer protection against low-temperature conditions. Adaptive processes termed cold acclimation and chilling tolerance, have been developed that enhance tolerance in response to low-temperature exposure, which involves changes in physiological, biochemical, molecular, and metabolic processes (Yadav, 2010). Previous studies have shown that a wide range of metabolites play various roles in low-temperature tolerance, among which particular interest has focused on metabolites such as proline, sugars, secondary metabolites, and polyamines, which can function as osmolytes and are extensively involved in abiotic stress tolerance. Given the importance of such osmolytes in protecting plants against abiotic and biotic stress, they are often collectively referred to as cytoprotectants (Khan et al., 2010).
Plant metabolism responds sensitively and dynamically to low-temperature conditions (Xu and Fu, 2022). With the exposure to temperature stress, plants have developed metabolic modifications that are essential features in response to cold stress (Yadav, 2010; Xu and Fu, 2022). Chilling and/or freezing modify the structure, metabolic properties, and functions of enzymes, as well as the properties of membrane metabolite transporters (Kubien et al., 2003; Yadav, 2010), thereby leading to a diversion of the metabolic flux toward the synthesis of osmoprotectants, including soluble sugars, proline, and polyamines. Therefore, the plant metabolic network, particularly osmoprotectants, must be reprogrammed to ensure that essential metabolic homeostasis is maintained in response to low-temperature conditions.
Polyamine pathways are interconnected with GABA metabolic processes
Polyamines are aliphatic amines with low molecular mass that play roles in diverse biological processes, and these are mainly present in the free form in higher plants, such as putrescine (Put), spermidine (Spd), and spermine (Spm). Additionally, cadaverine (Cad) and thermospermine (t-Spm), a Spm isomer, are also reported to exist in higher plants (Wang et al., 2019).
Polyamine homeostasis is regulated by a dynamic balance among metabolic processes, conjugation, chemical alteration, and transport (Moschou and Roubelakis-Angelakis, 2014; Yu et al., 2019). Given the importance of polyamines, the regulation of their synthesis and accumulation has been well characterized in plants. The polyamine biosynthetic pathway commences primarily with arginine (Arg), which is converted to putrescine via three sequential reactions catalyzed by arginine decarboxylase, agmatine iminohydrolase, and N-carbamoylputrescine amidohydrolase (Figure 1). Subsequently Spd synthase (SPDS) catalyzes Put conversion to Spd. Finally, Spd is further converted to Spm or T-Spm, two tetraamine isomers, by Spm synthase (SPMS) and T-Spmsynthase, respectively (Hanzawa et al., 2002; Yu et al., 2019). The PA catabolic process is mainly catalyzed by two classes of amine oxidases (AOs): one is a copper-dependent diamine oxidase (DAO) and the other is a flavin adenine dinucleotide (FAD)-dependentpolyamine oxidase (PAO). Notably, putrescine can subsequently be converted into GABA in a reaction catalyzed by DAO. Consequently, the levels of GABA are partly dependent on modifications in polyamine metabolism.
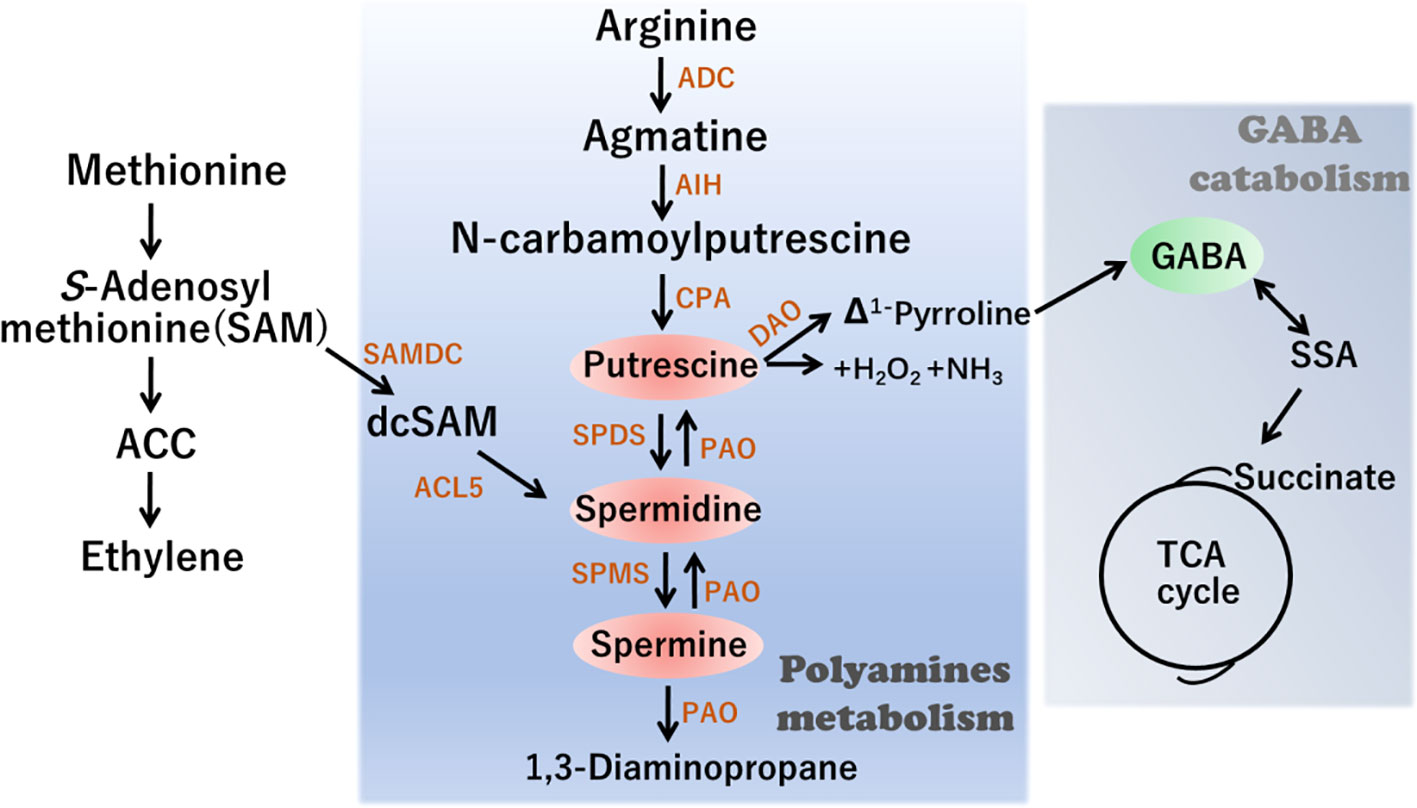
Figure 1 Schematic representation of Polyamines (PAs) metabolism and interconnection with γ-aminobutiric acid (GABA) synthesis. ADC, arginine decarboxylase; AIH, agmatine iminohydrolase; CPA, N-carbamoylputrescine amidohydrolase; DAOdiamine oxidase; PAO polyamine oxidase; SPDS, SPDS, spermidine synthase; SPMS, spermine synthase; ACL5, ACAULIS5; SAMDC, S-adenosylmethionine decarboxylase; ACC, 1-amino-cyclopropane-1-carboxylic-acid.
GABA is a four-carbon non-proteinogenic amino acid that acts as a signaling molecule playing multiple roles in a diverse range of organisms. For example, GABA plays a pivotal role in regulating C and N metabolic fluxes by linking amino acid metabolism to the tricarboxylic acid (TCA) cycle (Figure 1). Accumulating evidence indicates that GABA is involved in various aspects of plant growth and development, as well as in the biotic/abiotic stress responses in different plant species. Although GABA is generally metabolized via the GABA shunt pathway, under stress conditions, it can undergo synthesis via a non-enzymatic process involving certain polyamines, including spermidine and putrescine, together with proline (Signorelli et al., 2015; Ansari et al., 2021).
Role of polyamines in mediating low-temperature tolerance
Plants are sessile organisms that cannot physically escape from stressful environments, including low temperatures, also referred to as cold stress (Pareek et al., 2017). Generally, exposure to cold stress induces a range of physiological and biochemical disturbances, including metabolite imbalance and metabolic dysfunction (Kazemi-Shahandashti and Maali-Amiri, 2018). The production and accumulation of compatible solutes, including polyamines, is a common defense mechanism activated in response to cold stress (Alcázar et al., 2011; Pagter et al., 2017). An alteration in polyamines during the early stage of stress is considered a signal that promotes further signal transduction to activate transcription factors and stress-responsive pathways, such as the ICE-CBF-COR transcriptional cascade, reactive oxygen species (ROS) scavenging, and the antioxidant defense system (Wei et al., 2021; Hwarari et al., 2022).
In plants, polyamines are extensively involved in the responses to abiotic stresses, including low-temperature stress, and the association between polyamines and cold tolerance is well established. Accumulating evidence has revealed that exogenous polyamine treatment enhances tolerance to low temperatures in different plant species. For example, spermidine priming has been demonstrated to enhance polyamine metabolism and hence tolerance to chilling stress in rice (Sheteiwy et al., 2017). Furthermore, spray application of putrescine has been found to substantially reduce chilling injury in peach fruit during storage, regardless of the dose of putrescine applied or the time of application. In contrast, foliar spraying of seedlings with spermidine, spermine, and putrescine is believed to activate a defensive response to enhance cold resistance in winter oilseed rape (Jankovska-Bortkevič et al., 2020). SAM is a precursor not only in PA syntheses but also in ethylene biosynthesis (Figure 1), and ethylene was considered as a crucial hormone that plays essential roles in cold stress. For example, ethylene enhanced the cold tolerance via the MdERF1B–MdCIbHLH1 regulatory module in apple (Wang et al., 2021)
Genetic manipulation of polyamine biosynthetic genes enhances tolerance to cold stress. Arginine decarboxylase (ADC) is a rate-limiting enzyme that catalyzes the first step of polyamine biosynthesis (Urano et al., 2005). In Arabidopsis, exposure to cold stress has been observed to promote increased levels of AtADC1 and AtADC2 transcripts. Compared with wild-type plants, mutant plants with T-DNA insertional knockout (adc1 and adc2) of these enzymes were found to accumulate less free putrescine and were more sensitive to freezing. However, the damage caused by freezing conditions could be alleviated by the exogenous application of putrescine (Cuevas et al., 2008). Conversely, by modulating putrescine accumulation, the overexpression of ADC1 in potatoes has been found to confer a higher level of freezing tolerance (Kou et al., 2018).
GABA, a key player in mitigating ROS generation during cold stress in plants
In plants, GABA is a ubiquitous four-carbon metabolite and a vital signaling molecule that mediates the responses to biotic and abiotic stress conditions, including pathogen attack, low and high temperature, flooding, drought, soil salinity, and heavy metals (Li et al., 2021). Emerging evidence indicates that GABA participates in the low-temperature regulatory mechanisms of plants. Low temperatures are common unfavorable environmental conditions that limits plant development, leading to significantly reduced plant productivity. Generally, high level of GABA and shunt-related genes are induced in response to low-temperature conditions. For example, compared with non-stressed conditions, a 16-fold increase in GABA levels has been observed in barely seedlings directly exposed to -3°C, and amounts of GABA were also elevated in seedlings exposed to a temperature of -8°C (Mazzucotelli et al., 2006). Further studies in barley have revealed that the accumulation of GABA induces the expression of GABA-shunt genes (Mazzucotelli et al., 2006), thereby providing evidence to indicate the involvement of GABA metabolism in the cold tolerance in plants.
The exogenous application of GABA has been demonstrated to increase GABA levels and enhance cold tolerance in various plant species. In tomato seedlings, for example, accumulation of GABA has been observed in response to chilling treatment. The application of exogenous GABA induces substantially higher amounts of endogenous GABA in tomato seedlings compared with those in control plants. (Malekzadeh et al., 2014). Notably, the antioxidant enzyme activity, malondialdehyde (MDA) and proline displayed significantly decreased level after GABA treatment, whereas the sugar and proline level were significantly enhanced compared to un-treated seedlings (Malekzadeh et al., 2014). Exogenous GABA enhanced the endogenous GABA content by increasing the expression of the glutamate decarboxylase (GAD) gene and decreasing GABA transaminase (GABA-T) gene level (Shekari et al., 2021). In most cases, the application of GABA at low temperatures has been associated with the activation of the antioxidant defense system. For example, Wang et al. (2014) revealed that the application of GABA alleviated chilling injury in banana fruit by promoting proline accumulation and enhancing antioxidant capacity. Similarly, the application of GABA during the reproductive stage in tomato plants has been found to substantially alleviate chilling-induced oxidative damage by enhancing the activity of CAT, SOD and APX (Abd Elbar et al., 2021), thereby tending to indicate that GABA enhances the tolerance to low-temperature stress by modulating ROS content (Figure 2).
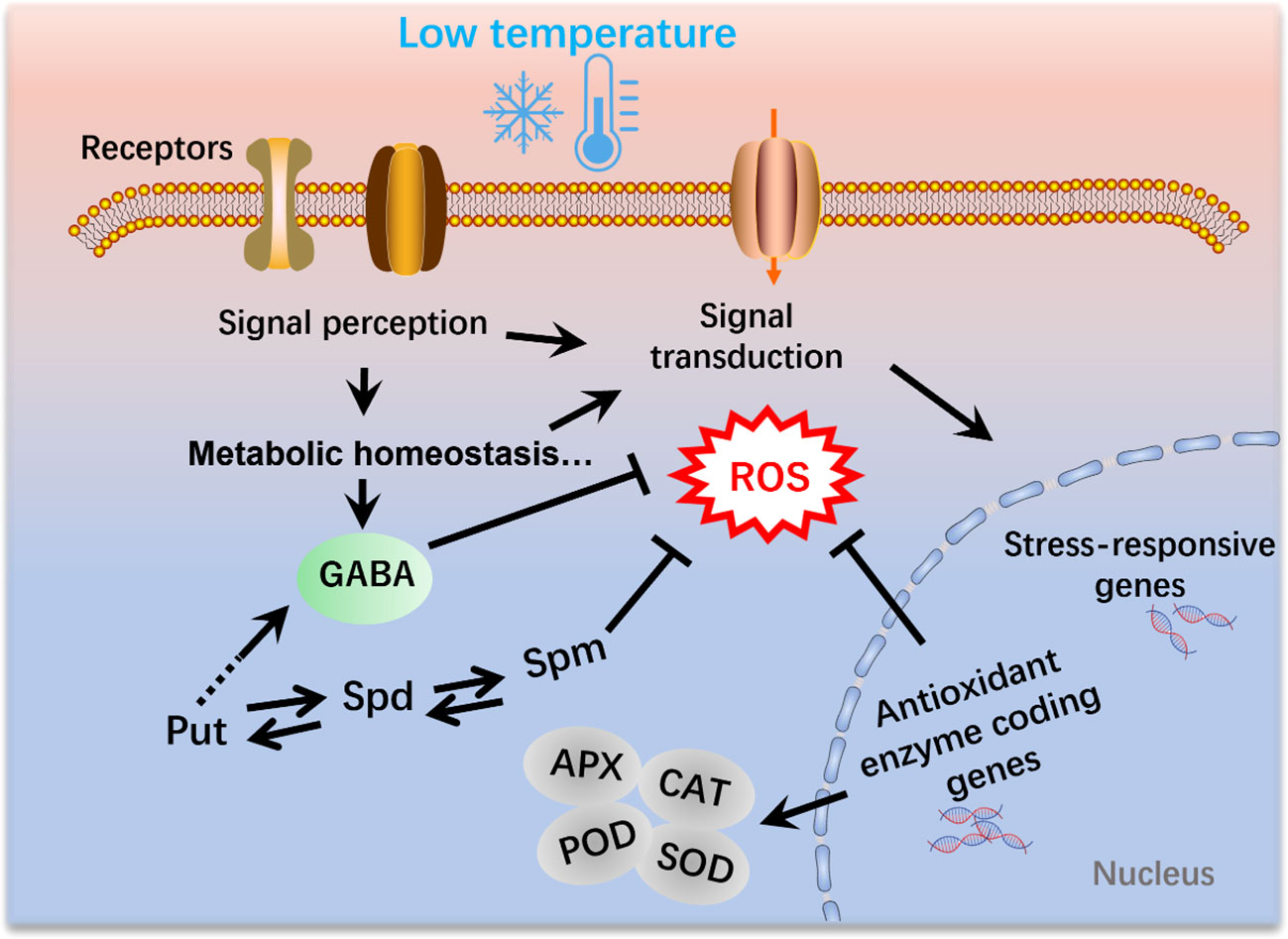
Figure 2 Polyamines and γ-aminobutyric acid (GABA) mediate the mitigation of reactive oxygen species (ROS) production under low-temperature conditions in plants. Cold signals are sensed by receptors and thereby induce a range of physiological, biochemical, and metabolic processes. Moreover, signal transduction leads to the activation of cold-responsive genes. SOD, superoxide dismutase; POD, Peroxidase activity; APX, ascorbate peroxidase; CAT, catalase.
The generation of ROS is an inevitable consequence of plant aerobic metabolism, which occurs in multiple cellular compartments, including the chloroplasts, mitochondria, apoplasts, and peroxisomes (Gill and Tuteja, 2010). Emerging evidence has indicated that the role of ROS in plants might be a double-edged sword, in that ROS also act as vital signal molecules involved in plant growth and development, particularly in response to abiotic and biotic stresses, including drought, salinity, metal toxicity, heat shock, and cold stress (Bailey-Serres and Mittler, 2006; Gapper and Dolan, 2006; Waszczak et al., 2018; Hasanuzzaman et al., 2020). GABA serves as a central molecule in the defense system of plants and is extensively involved in the mitigation of ROS in response to different stresses, including exposure to low temperatures (Ansari et al., 2021). The GABA shunt pathway effectively bypasses two enzymes in the TCA cycle that have been established to be sensitive to oxidative stress (Nicolas and Hillel, 2004; Janse van Rensburg and Van den Ende, 2020). Moreover, many GABA-related components have been demonstrated to play vital roles in ROS scavenging and detoxification under stress conditions (Jalil et al., 2017; Ansari et al., 2021). For example, succinic semialdehyde dehydrogenase (SSADH), a GABA shunt enzyme, catalyzes the conversion of succinic semialdehyde to succinate in the mitochondria. Previous studies have shown that SSADH is essential for ROS homeostasis in plants (Bao et al., 2015). Given that the application of GABA under low-temperature conditions generally modulates the activities of oxidative enzymes, suggests that GABA plays a crucial role in the mitigation of ROS during cold stress in plants (Figure 2).
Concluding remarks
Although the involvement of several key metabolites, including polyamines and GABA, has been demonstrated during the low-temperature stress response of plants, a detailed understanding of how metabolites participated in cold tolerance is imperative. Further studies are needed to gain a better understanding of the pathways and regulatory networks of key metabolites. Additional effort should also be devoted to elucidating the dynamics of these metabolites in the development of low-temperature tolerance. In addition, the rapid development of metabolomics technology in plants will provide new opportunities to identify novel and/or unknown metabolites associated with the response to low-temperature conditions. We believe that with further in-depth research on the mechanisms of key metabolites involved in low-temperature responses, the knowledge thus gained will make it possible to enhance the cold tolerance and productivity of plants.
Author contributions
MX, QY, and JY undertook the literature review and wrote the manuscript. GB and PL edited the manuscript. All authors contributed to the article and approved the submitted version.
Funding
This manuscript was funded by the National Natural Science Foundation of China (31970370, 32270417), jointly supported by the Science and Technology Project of Nujiang Prefecture, Yunnan Province, China (2020CY004) and the Science and Technology Planning Project of Guangdong Province, China (2019B030301007).
Conflict of interest
The authors declare that the research was conducted in the absence of any commercial or financial relationships that could be construed as a potential conflict of interest.
Publisher’s note
All claims expressed in this article are solely those of the authors and do not necessarily represent those of their affiliated organizations, or those of the publisher, the editors and the reviewers. Any product that may be evaluated in this article, or claim that may be made by its manufacturer, is not guaranteed or endorsed by the publisher.
References
Abd Elbar, O. H., Elkelish, A., Niedbała, G., Farag, R., Wojciechowski, T., Mukherjee, S., et al. (2021). Protective effect of γ-aminobutyric acid against chilling stress during reproductive stage in tomato plants through modulation of sugar metabolism, chloroplast integrity, and antioxidative defense systems. Front. Plant Sci. 12. doi: 10.3389/fpls.2021.663750
Alcázar, R., Cuevas, J. C., Planas, J., Zarza, X., Bortolotti, C., Carrasco, P., et al. (2011). Integration of polyamines in the cold acclimation response. Plant Sci. 180 (1), 31–38. doi: 10.1016/j.plantsci.2010.07.022
Ansari, M. I., Jalil, S. U., Ansari, S. A., Hasanuzzaman, M. (2021). GABA shunt: A key-player in mitigation of ROS during stress. Plant Growth Regul. 94 (2), 131–149. doi: 10.1007/s10725-021-00710-y
Baier, M., Bittner, A., Prescher, A., van Buer, J. (2019). Preparing plants for improved cold tolerance by priming. Plant Cell Environ. 42 (3), 782–800. doi: 10.1111/pce.13394
Bailey-Serres, J., Mittler, R. (2006). The roles of reactive oxygen species in plant cells. Plant Physiol. 141 (2), 311–311. doi: 10.1104/pp.104.900191
Bao, H., Chen, X., Lv, S., Jiang, P., Feng, J., Fan, P., et al. (2015). Virus-induced gene silencing reveals control of reactive oxygen species accumulation and salt tolerance in tomato by γ-aminobutyric acid metabolic pathway. Plant Cell Environ. 38 (3), 600–613. doi: 10.1111/pce.12419
Cuevas, J. C., López-Cobollo, R., Alcázar, R., Zarza, X., Koncz, C., Altabella, T., et al. (2008). Putrescine is involved in arabidopsis freezing tolerance and cold acclimation by regulating abscisic acid levels in response to low temperature. Plant Physiol. 148 (2), 1094–1105. doi: 10.1104/pp.108.122945
Gapper, C., Dolan, L. (2006). Control of plant development by reactive oxygen species. Plant Physiol. 141 (2), 341–345. doi: 10.1104/pp.106.079079
Gill, S. S., Tuteja, N. (2010). Reactive oxygen species and antioxidant machinery in abiotic stress tolerance in crop plants. Plant Physiol. Bioch 48 (12), 909–930. doi: 10.1016/j.plaphy.2010.08.016
Hanzawa, Y., Imai, A., Michael, A. J., Komeda, Y., Takahashi, T. (2002). Characterization of the spermidine synthase-related gene family in Arabidopsis thaliana. FEBS Lett. 527 (1-3), 176–180. doi: 10.1016/s0014-5793(02)03217-9
Hasanuzzaman, M., Bhuyan, M. H. M., Zulfiqar, F., Raza, A., Mohsin, S. M., Mahmud, J. A., et al. (2020). Reactive oxygen species and antioxidant defense in plants under abiotic stress: Revisiting the crucial role of a universal defense regulator. Antioxidants 9 (8), 681. doi: 10.3390/antiox9080681
Hwarari, D., Guan, Y., Ahmad, B., Movahedi, A., Min, T., Hao, Z., et al. (2022). ICE-CBF-COR signaling cascade and its regulation in plants responding to cold stress. Int. J. Mol. Sci. 23 (3), 1549. doi: 10.3390/ijms23031549
Jalil, S. U., Ahmad, I., Ansari, M. I. (2017). Functional loss of GABA transaminase (GABA-T) expressed early leaf senescence under various stress conditions in Arabidopsis thaliana. Curr. Plant Biol. 9, 11–22. doi: 10.1016/j.cpb.2017.02001
Jankovska-Bortkevič, E., Gavelienė, V., Šveikauskas, V., Mockevičiūtė, R., Jankauskienė, J., Todorova, D., et al. (2020). Foliar application of polyamines modulates winter oilseed rape responses to increasing cold. Plants 9 (2), 179. doi: 10.3390/plants9020179
Janse van Rensburg, H. C., Van den Ende, W. (2020). riming with γ-Aminobutyric acid against Botrytis cinerea reshuffles metabolism and reactive oxygen species: Dissecting signalling and metabolism. Antioxidants (Basel) 9(12), 1174. doi: 10.3390/antiox9121174
Kazemi-Shahandashti, S. S., Maali-Amiri, R. (2018). Global insights of protein responses to cold stress in plants: Signaling, defence, and degradation. J. Plant Physiol. 226, 123–135. doi: 10.1016/j.jplph.2018.03.022
Khan, S. H., Ahmad, N., Ahmad, F., Kumar, R. (2010). Naturally occurring organic osmolytes: from cell physiology to disease prevention. IUBMB Life 62 (12), 891–895. doi: 10.1002/iub.406
Kou, S., Chen, L., Tu, W., Scossa, F., Wang, Y., Liu, J., et al. (2018). The arginine decarboxylase gene ADC1, associated to the putrescine pathway, plays an important role in potato cold-acclimated freezing tolerance as revealed by transcriptome and metabolome analyses. Plant J. 96 (6), 1283–1298. doi: 10.1111/tpj.14126
Kubien, D. S., von Caemmerer, S., Furbank, R. T., Sage, R. F. (2003). C4 photosynthesis at low temperature. a study using transgenic plants with reduced amounts of rubisco. Plant Physiol. 132 (3), 1577–1585. doi: 10.1104/pp.103.021246
Li, L., Dou, N., Zhang, H., Wu, C. (2021). The versatile GABA in plants. Plant Signal Behav. 16 (3), 1862565. doi: 10.1080/15592324.2020.1862565
Malekzadeh, P., Khara, J., Heydari, R. (2014). Alleviating effects of exogenous gamma-aminobutiric acid on tomato seedling under chilling stress. Physiol. Mol. Biol. Pla 20 (1), 133–137. doi: 10.1007/s12298-013-0203-5
Mazzucotelli, E., Tartari, A., Cattivelli, L., Forlani, G. (2006). Metabolism of gamma-aminobutyric acid during cold acclimation and freezing and its relationship to frost tolerance in barley and wheat. J. Exp. Bot. 57 (14), 3755–3766. doi: 10.1093/jxb/erl141
Moschou, P. N., Roubelakis-Angelakis, K. A. (2014). Polyamines and programmed cell death. J. Exp. Bot. 65 (5), 1285–1296. doi: 10.1093/jxb/ert373
Nicolas, B., Hillel, F. (2004). GABA in plants: just a metabolite. Trends Plant Sci. 9 (3), 110–115. doi: 10.1016/j.tplants.2004.01.006
Pagter, M., Alpers, J., Erban, A., Kopka, J., Zuther, E., Hincha, D. K. (2017). Rapid transcriptional and metabolic regulation of the deacclimation process in cold acclimated Arabidopsis thaliana. BMC Genomics 18 (1), 731. doi: 10.1186/s12864-017-4126-3
Pareek, A., Khurana, A., Sharma, A. K., Kumar, R. (2017). An overview of signaling regulons during cold stress tolerance in plants. Curr. Genomics 18 (6), 498–511. doi: 10.2174/1389202918666170228141345
Raju, S. K. K., Barnes, A. C., Schnable, J. C., Roston, R. L. (2018). Low-temperature tolerance in land plants: Are transcript and membrane responses conserved? Plant Sci. 276, 73–86. doi: 10.1016/j.plantsci.2018.08.002
Shekari, A., Hassani, R. N., Aghdam, M. S. (2021). Exogenous application of GABA retards cap browning in agaricus bisporus and its possible mechanism. Postharvest Biol. Tec 174, 111434. doi: 10.1016/j.postharvbio.2020.111434
Sheteiwy, M., Shen, H., Xu, J., Guan, Y., Song, W., Hu, J. (2017). Seed polyamines metabolism induced by seed priming with spermidine and 5-aminolevulinic acid for chilling tolerance improvement in rice (Oryza sativa l.) seedlings. Environ. Exp. Bot. 37, 58–72. doi: 10.1016/j.envexpbot.2017.02.007
Signorelli, S., Dans, P. D., Coitiño, E. L., Borsani, O., Monza, J. (2015). Connecting proline and γ-aminobutyric acid in stressed plants through non-enzymatic reactions. PloS One 10 (3), e0115349. doi: 10.1371/journal.pone.0115349
Urano, K., Hobo, T., Shinozaki, K. (2005). Arabidopsis ADC genes involved in polyamine biosynthesis are essential for seed development. FEBS Lett. 579 (6), 1557–1564. doi: 10.1016/j.febslet.2005.01.048
Wang, Y., Jiang, H., Mao, Z., Liu, W., Jiang, S., Xu, H., et al. (2021). Ethylene increases the cold tolerance of apple via the MdERF1B–MdCIbHLH1 regulatory module. Plant J. 106 (2), 379–393. doi: 10.1111/tpj.15170
Wang, Y., Luo, Z., Huang, X., Yang, K., Gao, S., Du, R. (2014). Effect of exogenous γ-aminobutyric acid (GABA) treatment on chilling injury and antioxidant capacity in banana peel. Sci. Hortic. 168, 132–137. doi: 10.1016/j.scienta.2014.01.022
Wang, W., Paschalidis, K., Feng, J. C., Song, J., Liu, J. H. (2019). Polyamine catabolism in plants: auniversal process with diverse functions. Front. Plant Sci. 10. doi: 10.3389/fpls.2019.00561
Waszczak, C., Carmody, M., Kangasjärvi, J. (2018). Reactive oxygen species in plant signaling. Annu. Rev. Plant Biol. 69, 209–236. doi: 10.1146/annurev-arplant-042817-040322
Wei, X., Liu, S., Sun, C., Xie, G., Wang, L. (2021). Convergence and divergence: Signal perception and transduction mechanisms of cold stress in arabidopsis and rice. Plants (Basel) 9, 10(9):1864. doi: 10.3390/plants10091864
Xu, Y., Fu, X. (2022). Reprogramming of plant central metabolism in response to abiotic stresses: ametabolomics view. Int. J. Mol. Sci. 23 (10)5716. doi: 10.3390/ijms23105716
Yadav, S. K. (2010). Cold stress tolerance mechanisms in plants. a review. Agron. Sustain Dev. 30 (3), 515–527. doi: 10.1051/agro:2009050
Keywords: cold stress, polyamines, GABA, ROS, osmolytes
Citation: Xu M, Yang Q, Bai G, Li P and Yan J (2022) Polyamine pathways interconnect with GABA metabolic processes to mediate the low-temperature response in plants. Front. Plant Sci. 13:1035414. doi: 10.3389/fpls.2022.1035414
Received: 02 September 2022; Accepted: 05 October 2022;
Published: 20 October 2022.
Edited by:
Baohong Zou, Nanjing Agricultural University, ChinaReviewed by:
Lei Huang, Purdue University, United StatesMichael Gerard Muszynski, University of Hawaii at Manoa, United States
Hao Liu, Guangdong Academy of Agricultural Sciences (GDAAS), China
Copyright © 2022 Xu, Yang, Bai, Li and Yan. This is an open-access article distributed under the terms of the Creative Commons Attribution License (CC BY). The use, distribution or reproduction in other forums is permitted, provided the original author(s) and the copyright owner(s) are credited and that the original publication in this journal is cited, in accordance with accepted academic practice. No use, distribution or reproduction is permitted which does not comply with these terms.
*Correspondence: Jian Yan, eWFuamlhbjc4QHNjYXUuZWR1LmNu