- 1Instituto Tecnológico Vale, Belém, Brazil
- 2Programa de Pós Graduação em Zoologia, Universidade Federal do Pará, Belém, Brazil
- 3Faculdade de Computação e Informática, Universidade Presbiteriana Mackenzie, São Paulo, Brazil
- 4Museu Paraense Emílio Goeldi, Belém, Brazil
- 5Departamento de Química Fundamental, Centro de Ciências Exatas e da Natureza, Universidade Federal de Pernambuco, Recife, Brazil
- 6Programa de Pós-Graduação em Botânica, Instituto de Ciências Biológicas, Universidade de Brasília, Brasília, Brazil
- 7Programa de Pós-Graduação em Botânica, Universidade Estadual de Feira de Santana, Feira de Santana, Brazil
- 8Departamento de Ecologia, Instituto de Biociências, Universidade de São Paulo, São Paulo, Brazil
Climate change has impacted biodiversity, affecting species and altering their geographical distribution. Besides understanding the impact in the species, it has been advocated that answering if different traits will be differently impacted could allow refined predictions of how climate change will jeopardize biodiversity. Our aim was to evaluate if climate change will potentially impact plant species differently, considering their traits. We evaluated 608 plant species that occur in the naturally open areas of ferruginous outcrops (namely, cangas) in the National Forest of Carajás (Eastern Amazon). Firstly, we estimated the effects of climate change on each species using species distribution modeling, and analyzed this impact in the set containing all species. Secondly, we classified plant species considering the following traits: (i) pollination syndromes (melittophily, phalaenophily, psychophily, cantharophily, entomophily, ornithophily, chiropterophily, anemophily); (ii) habit (tree, shrub, herb, liana, parasite); and (iii) the main habitat of occurrence (open areas and forests). Thirdly, we investigated if the effects of climate change could be significantly more intense considering all the different traits quoted. Our results showed that most plant species will potentially face reduction of suitable habitats under future climate and the scenarios showed that 42% of them may not find suitable areas in the cangas of Carajás. We found no significant difference within each analyzed trait, considering the potential impact of climate change. The most climatically suitable areas (i.e., areas with high probability of species occurrence in the future) are those in the southwest of the study area. These areas can be considered as priority areas for species protection against climate change.
Introduction
Climate change due to anthropogenic activities has had negative effects on biodiversity, especially due to its unprecedented speed (Pacifici et al., 2015; Venter et al., 2016). The flora may be affected by several factors, including physiological and adaptive incompatibility with the new climatic conditions (Ahuja et al., 2010), reduced capacity or inability to disperse to new habitats (Corlett and Westcott, 2013), or negative morphological and phenological changes (Cleland et al., 2007). Moreover, climate change can alter the original geographic distribution of species. For example, changes in the average altitude of occurrence of plants have been observed (Kelly and Goulden, 2008; Lenoir et al., 2008; Steinbauer et al., 2018), as have changes toward new latitudes (Chen et al., 2011). Thus, anticipating the potential impact of climate change on plant species, especially considering different traits, is urgently need to effective conservation strategies.
Species traits are any characteristic of an individual that can be assigned to a species, which could be phenological, morphological, physiological, reproductive, or behavioral (Kissling et al., 2018). They were also defined as a measurable property of organisms; more specifically, a functional trait is one that strongly influences organismal performance, and it can provide important information about the species performance, especially considering global changes (McGill et al., 2006).
One example of a set of traits related to reproductive success is pollination syndrome. It relies on a specific set of traits (Dellinger, 2020), such as the shape, color, odor, nectar amount, and pollen location (Faegri and Van Der Pijl, 1979; Fenster et al., 2004). The concept of pollination syndrome is based on the assumption that flowers are adapted to their most efficient pollinator group (Dellinger, 2020), with floral morphology being an important factor that restricts the number of possible interactions with pollinators (Stang et al., 2007). Despite the common criticism to pollination syndrome, based mainly on the fact that many plants can be pollinated by different pollinators (Ollerton et al., 2009), high accuracy on pollinator’s prediction was found even when based on only a few traits, showing that it can accurately circumscribe plant-pollinator interactions (Dellinger, 2020). However, as far as we know, there is no study that addressed the impact of climate change considering different pollination syndromes.
Habit is an important morphological trait (Panchen et al., 2014). There is also no study comparing the impact of climate change on this trait in a large number of species. However, it was showed that global warming altered vegetation functional structure, considering fast-growing species and two different growth-habits (erect and prostate) (Debouk et al., 2015). The influence of harsh condition in the plant size was also demonstrated to a Pyrenean saxifrage (Saxifraga longifolia Lapeyr.), where larger plants showed higher resistance to stress (Cotado and Munné-Bosch, 2020). When comparing different growth habits of Nothofagus pumilio (Poepp. & Endl.) Krasser under an altitudinal gradient, a study showed that at high altitude, juvenile shrubby growth habits are favored, probably due to cold stress effects (Soliani and Aparicio, 2020).
Additionally, a third characteristic that was still scarcely addressed, when comparing the climate change impact on large set of plant species, is their preferential habitat. For example, the richness of trees occurring in the tropical Amazon forest are expected to reduce by 31–37% (year 2050) under scenarios of climate change, and tree species can potentially lose 65% of their environmentally suitable area (Gomes et al., 2019). Considering the woody plant assemblage occurring in the Atlantic Forest, it was projected an overall reduction of its beta diversity under future climatic scenario (Zwiener et al., 2018). As for the Brazilian cerrado vegetation, an analysis of 1,553 plant species showed that species will lose an average of 34–40% (under the optimistic scenario) and 43–60% (under the pessimistic scenario) of their suitable occurrence area (Velazco et al., 2019).
National Forest of Carajás is one of the few remaining natural habitats located in the eastern Amazon, an area highly affected by deforestation (Souza-Filho et al., 2016). Rock outcrops presenting a high iron concentration and shallow soil occur within the Amazon forest and are locally known as canga (Mota et al., 2018). The canga vegetation is composed of annual and perennial grasses and shrubs that contrast visibly with the surrounded tropical forest. Inside cangas, there are also small patches of forest (called capão florestal) where the soil is deeper. Cangas are found in the uplands of Serra Sul (the southernmost mountain) and Serra Norte (the northernmost mountain) in the National Forest of Carajás, as well in the bordering of the National Park of Campos Ferruginosos, which included two other uplands named Serra da Bocaina and Serra do Tarzan (median altitude of 607 m; Souza-Filho et al., 2019). It was already noted that at higher altitudes, the impact of climate change on biodiversity is likely more severe (Klanderud and Totland, 2005; Grabherr et al., 2010). Different species that occur along elevation gradients have changed their distributions in recent years by increasing their altitude in search of suitable climate zones (Kelly and Goulden, 2008; Lenoir et al., 2008; Steinbauer et al., 2018). However, such species may not always be able to timely adjust their distributions to climatically milder areas due to limitations in their dispersal capacity, which makes them particularly vulnerable (Colwell et al., 2008; Bertrand et al., 2011). Specifically to Amazon biome, high projected temperature change and drought intensification are expected to occur due to climate change (Intergovernmental Panel on Climate Change-IPCC [IPCC], 2014), with direct deleterious effects on biodiversity.
The main objective of this study is to evaluate if climate change will potentially impact plant species differently, considering their traits. We evaluated 608 plant species that occur in the naturally open areas of ferruginous outcrops in the National Forest of Carajás. We analyzed the following traits: pollination syndromes, habit and their main habitat. More specifically, the present study aims to determine: (i) the overall impact in the canga plant species; (ii) if different traits can be differently impacted by climate change; and (iii) the priority areas that could safeguard canga plant species and traits in the future against climate change.
Materials and Methods
Study Area
The canga sites analyzed here (Figure 1A) belong to the National Forest of Carajás (hereafter referred to as Carajás) and are located in the southeast of the state of Pará (Eastern Amazon Biome) (Figure 1B). Canga sites are located on discontinuous island-like habitats (Andrino et al., 2020), surrounded by Amazon forest. These areas of iron-rich substrate receive high solar irradiance, have more than 2,000 mm of rainfall annually, and undergo a pronounced dry season (Souza-Filho et al., 2016).
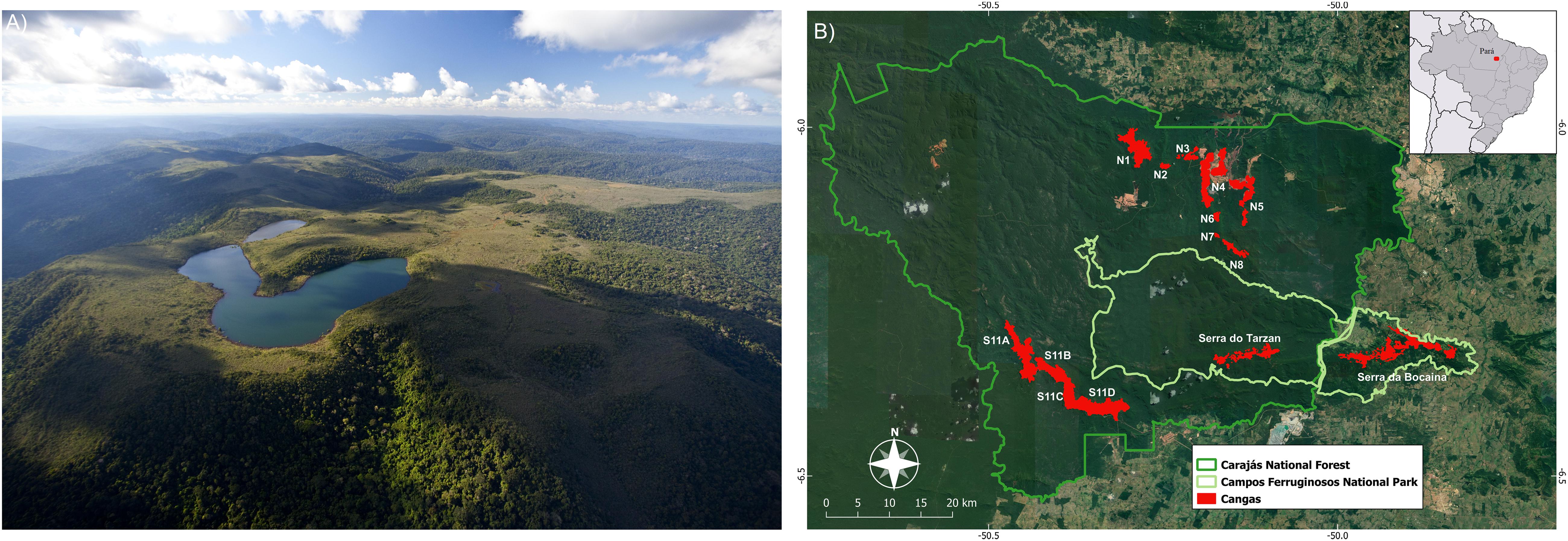
Figure 1. (A) Open vegetation occurring in upland outcrops (canga) (photo: João Marcos Rosa) (B) located in the National Forest of Carajás.
List of Plant Species
Recently, the flora of cangas of Carajás was surveyed and reviewed by experts (Mota et al., 2018), which enables great accuracy for the analysis proposed here. The list of species surveyed is significant because it corresponds to almost 13% of the flora recorded for the state of Pará (Mota et al., 2018), the second largest state of Brazil and entirely within the Amazon biome. Some species of cangas are widely distributed, and few species with restricted distributions have been reported for Carajás (36 angiosperm species; Giulietti et al., 2019). The plant species of cangas totalize 855 angiosperm species in 110 families (Mota et al., 2018).
Plant Species Habits and Pollination Syndromes
Habits of canga plant species were classified on five types (herbs, shrubs, trees, lianas, and parasites) and were defined in the study above mentioned (Mota et al., 2018). The pollination syndrome of those species was previously determined (Pinto et al., 2020) based on a group of plant characteristics (Supplementary Material A), following Faegri and Van Der Pijl (1979) and Rosas-Guerrero et al. (2014). Pollination syndrome for each plant species was determined based on available images, virtual herbaria sources, articles on the reproductive and flowering biology. It was possible to determine the syndrome of 771 species. Eight syndromes were analyzed: melittophily (pollinated by bees), phalaenophily (moths), psychophily (butterflies), cantharophily (beetles), entomophily (unspecified insects), ornithophily (birds), chiropterophily (bats), and anemophily (wind).
Occurrences of Plant Species and Main Habitat
A database of the occurrences of each plant species of the cangas was organized by retrieving data from: (1) internal databases on species distribution; (2) online biodiversity databases, specifically the speciesLink and the Global Biodiversity Information Facility (GBIF)1; (3) consulting the specialized literature; and (4) through new fieldwork. We excluded repeated, dubious and incorrect occurrence points from online database. The total known geographical distribution of each species was used to run the models (see below) (the database of species occurrence can be found in Supplementary Material B).
As already said, some species are widely distributed, and although few species with restricted distributions have been reported for Carajás (36 species; Giulietti et al., 2019), they have not been addressed in detail here. As we aimed to determine if species occurring only (or preferentially) in the cangas will be significantly more affected than those occurring mainly in forest, the occurrences used for distribution modeling were overlaid on a land use map (ESA Climate Change Initiative—Land Cover led by Université Catholique de Louvain for 2017). The percentage of occurrences was calculated for the two main types of land cover analyzed here, namely, forest and open-area vegetation.
Modeling of Species Distribution
The Biomod2 package (v.3.3-71; Thuiller, 2003) for R (R Development Core Team 2005) was used for species distribution modeling (SDM). Two algorithms were used: maximum entropy (MAXENT) (Phillips et al., 2006) and generalized linear model (GLM) (McCullagh and Nelder, 1989). Both were chosen due to their robustness and broad application (Li and Wang, 2013).
The environmental variables used in SDM were selected from the 20 less correlated bioclimatic layers (Aguirre-Gutiérrez et al., 2013) of the set available in WorldClim (Hijmans et al., 2005), which defines the mean temperature and precipitation data for 1970–2000 period, and altitude (5 arc min resolution). After selection, the following nine layers remained: Altitude, Mean Diurnal Range, Isothermality, Mean Temperature of Driest Quarter, Annual Precipitation, Precipitation of Driest Month, Precipitation Seasonality, Precipitation of Warmest Quarter, and Precipitation of Coldest Quarter. The predictive quality of the models was evaluated using the true skill statistic approach (TSS) (Allouche et al., 2006) with a minimum quality threshold ≥ 0.7, calculated by randomly partitioning the input data series into a quality testing set (25%) and a training set (75%), in successive rounds of cross-validation.
The climate change scenarios used for model projection refer to the estimates made for two future decades of short-medium term: 2050 and 2070. For both decades, we selected two IPCC scenarios of Representative Concentration Pathways (RCPs) (Intergovernmental Panel on Climate Change-IPCC [IPCC], 2014), which represent two different levels of radiative forcing (the difference between the insolation absorbed by the earth and the energy radiated back to space): 4.5 and 8.5 W/m2. The first scenario, 4.5, is more moderate (compared to extremes, RCP 2.6, and 8.5), whereas the second scenario, 8.5, represents more intensive climate change (Intergovernmental Panel on Climate Change-IPCC [IPCC], 2014). The scenarios were generated by two different institutes: Met Office Hadley Center (HadGEM2-ES, Hadley Global Environment Model 2—Earth System) and the National Center for Atmospheric Research (CCSM4, The Complete Coupled System).
The models obtained by the two institutes (HadGEM2-ES and CCSM4), the two algorithms (Maxent and GLM), and the different rounds were used to construct an ensemble forecast model to generate a single-scenario final model for each species. This model is even more robust because it groups the most important trends from the set of generated models based on the committee averaging method (Thuiller et al., 2009), producing normalized and comparable results among species.
In addition to the R package mentioned above, PostgreSQLs/PostGIS (The PostgreSQL Global Development Group) was used for the databases, together with the raster package (Hijmans and Etten, 2012) of R (R Development Core Team 2005), and the QGIS (Open Source Geospatial Foundation Project).
Analysis of the Results
To answer our main questions, three analyses were conducted:
(i) To determine the overall impact in the canga plant species, we grouped the models of all plant species. The committee averaging method (Thuiller et al., 2009) was used. Species that potentially would not find suitable habitats in Carajás in the future under the analyzed climate change scenarios were identified. For this identification, species with no pixels with high-suitability (defined as ≥ 75% of suitability in a 0–100% range of occurrence probability) in any of the future scenarios analyzed in the study area were accounted.
(ii) To answer if different traits can be differently impacted by climate change, i.e., if the climate change impact will be potentially more accentuated in one or more pollination syndromes, habits or habitats of plant species, we used Generalized Linear Mixed Effects models with beta error distribution and logit link function using the glmmTMB package in R (Brooks et al., 2017). Our model uses the proportional loss of area with high suitability as the response variable, the different categories of syndrome, habit or habitat as predictors, and the different scenarios as random effects. The significance of the terms was determined by analysis of variance (package car, Fox and Weisberg, 2019). Finally, the estimated marginal means function was used to compare the proportional loss of area between the different categories (package emmeans; Lenth, 2019).
(iii) To determine the priority areas that could safeguard canga plant species and traits in the future against climate change, we forecasted the same models obtained in the item (i) for the surroundings of Carajás (following Giulietti et al., 2019; considering only Pará state).
Results
Due to the scarcity of records of occurrence, not all species could be analyzed. Thus, models were obtained for 608 species (91 families; Supplementary Material C), belonging to eight syndromes, with a predominance of melittophily (bees; 53.5%) and, in the second place, anemophily (wind; 18.4%). Most of them are herbs (40%), followed by shrubs (27%), trees (18%), lianas (12%), and parasites (3%). A total of 483,632 occurrences were analyzed (Supplementary Material B). Almost half of the species (48%), considering the occurrence points obtained, is predominantly distributed in forest areas (>75% of the occurrence points) (Supplementary Material C). In regard specifically to Carajás, 257 plant species (42%) potentially will not find suitable habitats inside this protected area, as no pixel was detected there in any of the future scenarios analyzed (cells with zero value in Supplementary Material C).
Overall Species and Most Affected Traits
Potential loss of climatically suitable areas (i.e., areas with high climate resemblance considering current distribution and presenting high potential probability of species occurrence in the modeling processes) for most species was detected in all scenarios (Figure 2).
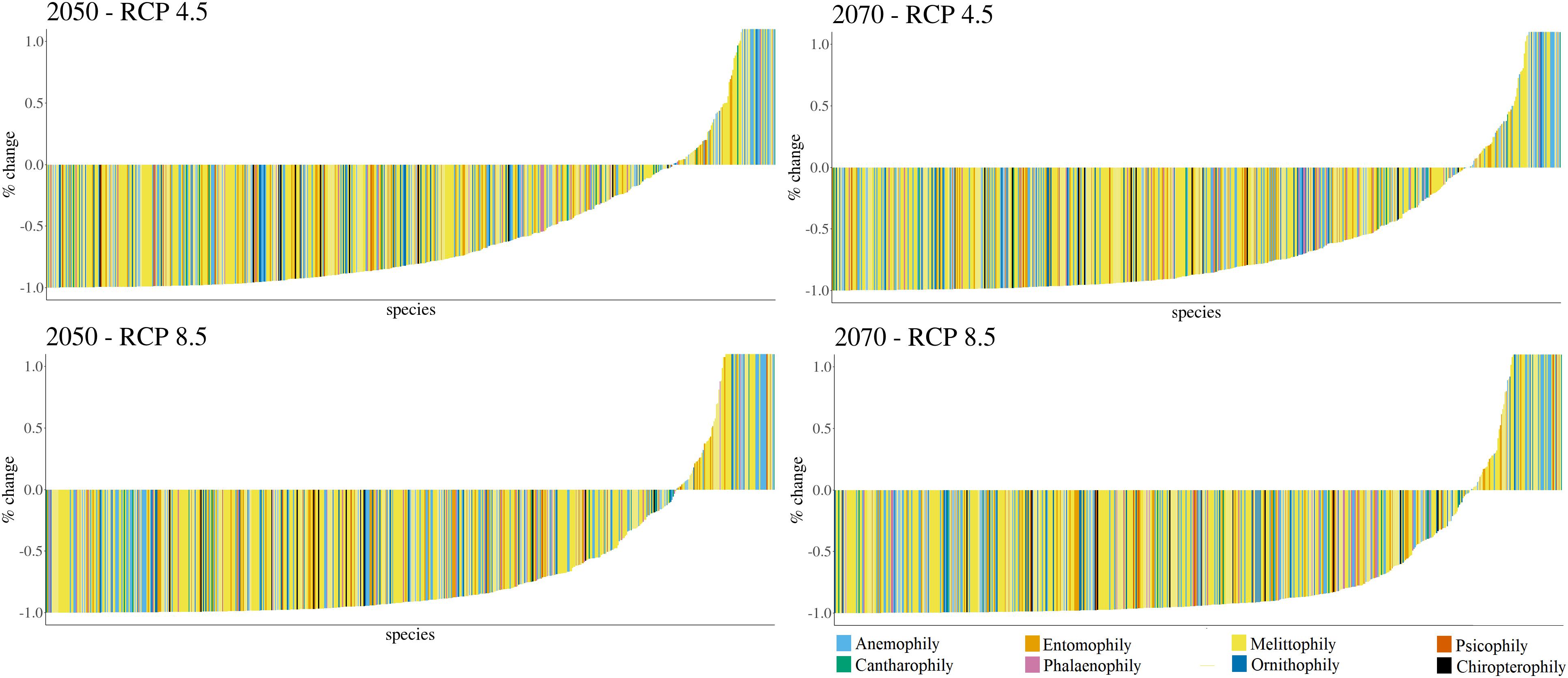
Figure 2. Area dynamics (in terms of future increase or decrease of suitable areas) for canga plant species due to climate change.
However, no significant difference was observed within the different syndromes, habits or habitats (syndrome: X2 = 10.766, df = 7, P = 0.1492; habit: X2 = 6.2308, df = 5, P = 0.2844; habitat: X2 = 1.102, df = 1, P = 0.2938) (Figure 3).
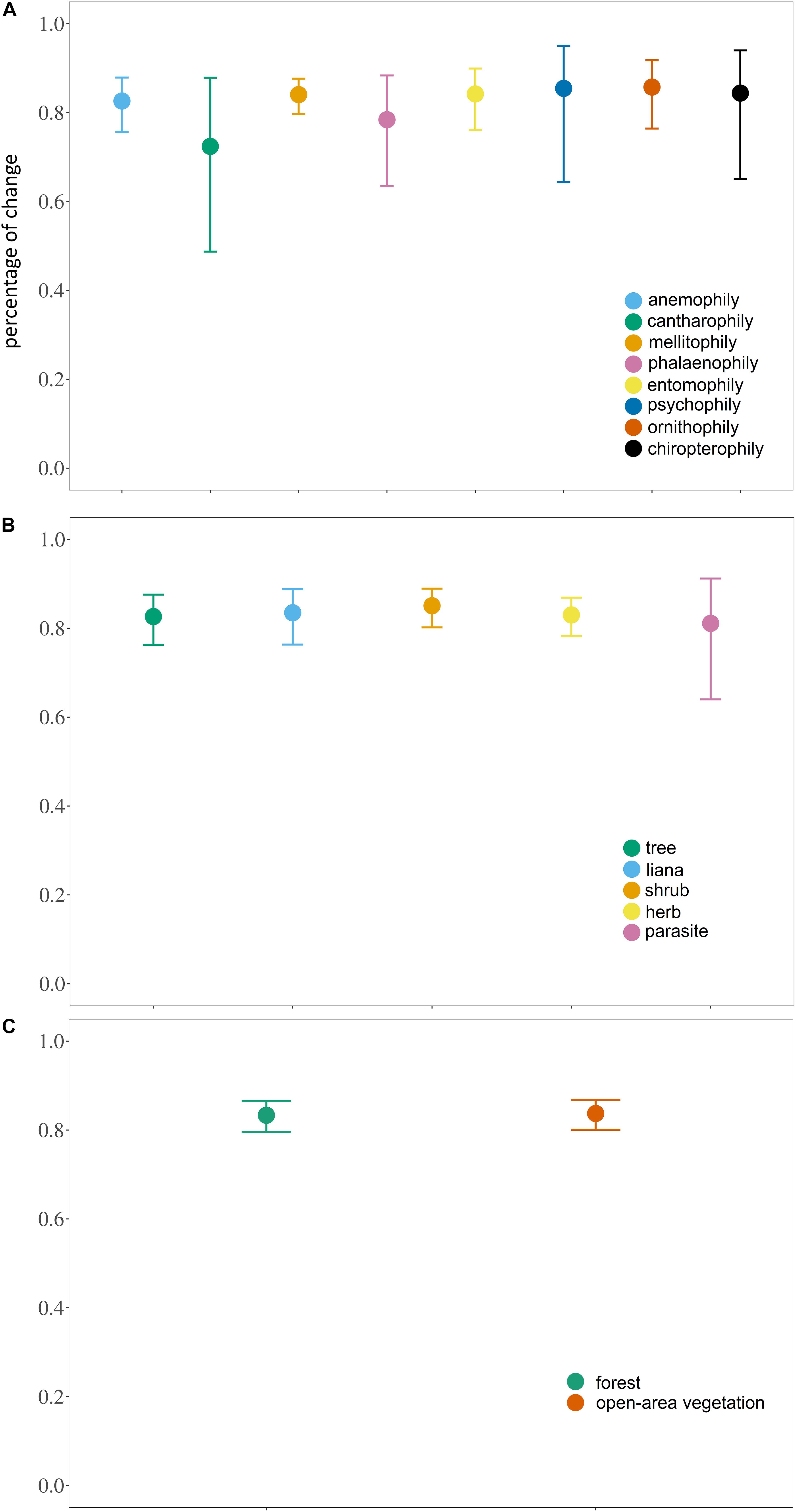
Figure 3. Estimated marginal mean (± 95% CI) of the proportional loss of areas with high habitat suitability (≥ 75%) for canga plant species with different (A) pollination syndromes; (B) habits and; (C) habitats.
Priority Areas
Proximal areas western of Carajás were already deforested, but most of the areas to the southwest and northwest are still protected (Figure 4A). Considering the model’s projection (Figures 4B–F), we found that the areas to the southwest of study area (warmer colors in Figure 4) will be potentially suitable for the distribution of flora species in 2050 (Figure 4C) and 2070 (Figure 4E) under RCP4.5. Regarding year 2050 and RCP 8.5 (Figure 4D), most of the southwest region of the study area was also highlighted as a suitable area for the plant species analyzed. As for the scenario for 2070 and RCP8.5 (Figure 4F) few scattered areas will be suitable for the analyzed species, especially those from southwest and southeast of study area. Carajás presents some areas with high climatic suitability in the RCP4.5 scenario but, under RCP8.5, there is basically no area with high suitability.
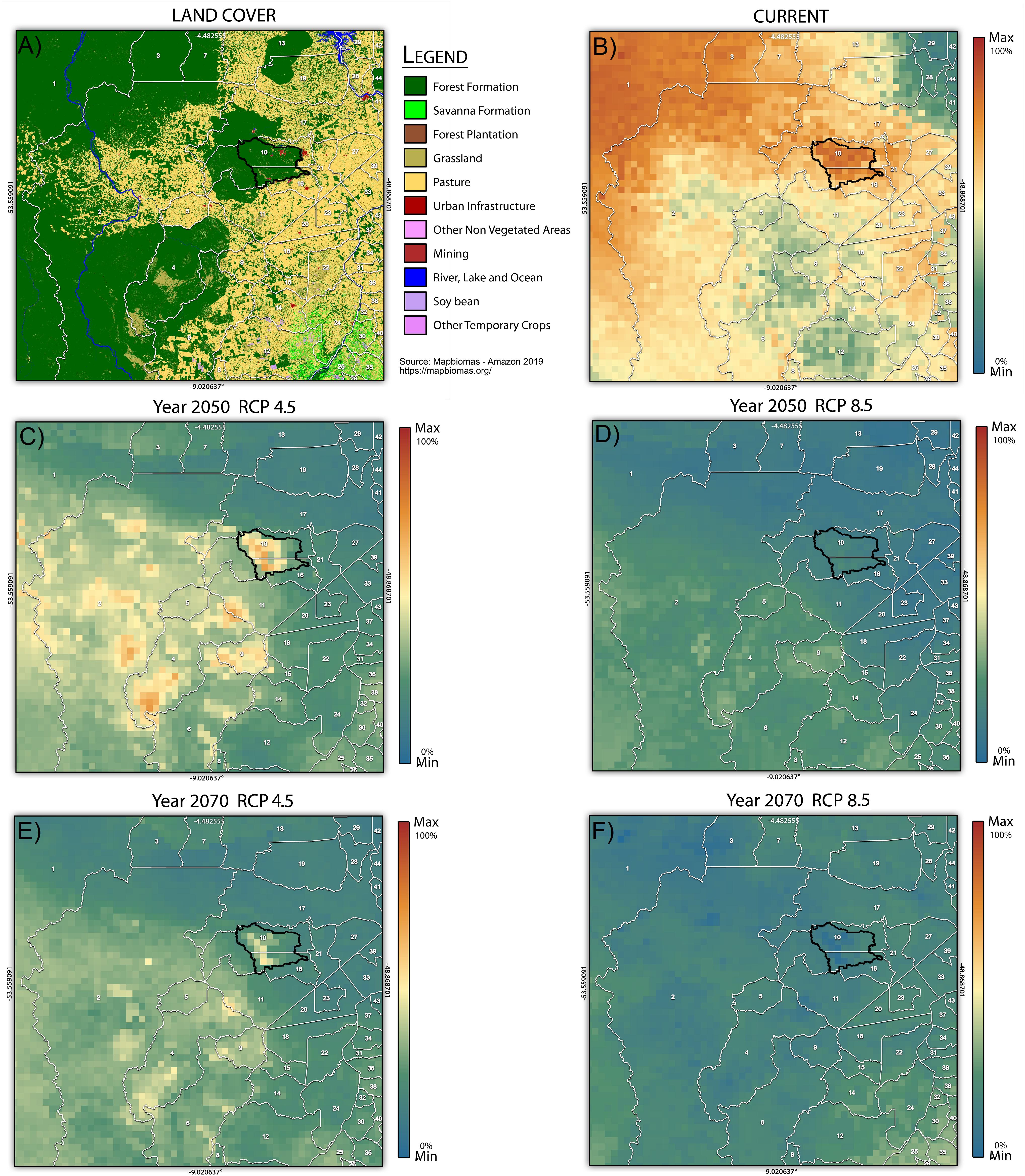
Figure 4. (A) The land cover of the projected area. (B) Current potential distribution and (C–F) future potential distribution considering the impact of climate change for all of the plant species of the cangas of the National Forest of Carajás (polygon in black). The scale variation corresponds to the percentage of species present per pixel (Max = 100%). White numbers refer to municipalities whose names can be found in Supplementary Material C.
Discussion
According to the scenarios used, most of the 608 analyzed plant species potentially will face a severe reduction in their geographic distribution in the future decades due to climate change. From the total, 257 species (42%) may not occur in Carajás in future scenarios since there will be practically no area considered to be climatically suitable for them there. We found no significant difference in the impact of climate change on each pollination syndromes, habit or main habitat, i.e., the traits analyzed will be potentially equally affected. When all plant species are analyzed, final models showed that the areas with the highest potential suitability in the future for the analyzed species are located in the southwest of Carajás.
Future climate projections indicate a drastic reduction in plant species regardless the trait analyzed. As already noted, we were unable to find other studies that considered the same set of plant species traits used here under climate change. However, it was shown that other traits related to leaf and root (leaf mass per area, thicker leaves, root carbon content) were good predictors to species abundance shifts under temperature increase (Soudzilovskaia et al., 2013). Considering land cover change, morphological traits showed an important response on a long-term study (Kahmen et al., 2002). However, it is likely that multiple trait sets and other aspects of plant behavior have to be included when looking for unambiguous results (Díaz and Cabido, 1997). Analyzing complex responses, mediated by traits, to environmental change is not a trivial task, reinforcing the need for more robust theory (Kimball et al., 2016). However, this is an important effort since identifying such traits could allow generalized predictions for how species with similar traits will likely respond to climate change (Gornish and Prather, 2014).
The future projections of geographical distributions indicated that the areas to the southwest of the study area (with small variation, depending on the scenario) are probably more climatically stable, and could act as important areas for protecting the set of analyzed plant species. A previous study considering only the current potential distribution of canga plant species showed the western region as climatically suitable for these species in the current climate (Giulietti et al., 2019). In fact, the same region has been identified previously as important areas to protect other species such as bats (Costa et al., 2018; Ribeiro et al., 2018), birds (Miranda et al., 2019), and bees (Giannini et al., 2020) against climate change, since they potentially will provide suitable habitats for these animal species. It is noteworthy that some of these areas are relatively well-preserved, especially in the southernmost area. Previous studies have analyzed other open areas near Carajás and their importance for canga plant species conservation, such as Serra Arqueada (Devecchi et al., 2020) and Serra de Campos, both in the São Félix do Xingu municipality (Andrino et al., 2020), located in the southwestern region of our projected area. Other protected areas with high probability of future occurrence in the same southwestern region, which could be the target of future studies aiming conservation of canga’s plant species, are the Serra da Fortaleza (São Félix do Xingu), Serra do Bacajá (São Félix do Xingu), and Serra do Cubencranquém (Ourilândia do Norte). All of them were also emphasized in the previous study related to the potential current distribution of narrow-endemic canga plant species of Carajás (Giulietti et al., 2019).
Our results are restricted to habitats presenting a potential future high suitability for canga plant species, relying on the set of selected climatic variables, which participates in the fundamental niche of the species. However, according to ecological niche theories, the geographic distribution of a species is also influenced by other environmental, biological, and evolutionary factors (Soberón and Peterson, 2005). One important aspect is ecological interactions, and earlier studies considering the impact of climate change on interacting-animal species occurring in Carajás indicated the potential future loss of nectarivorous bat species (pollinators) in the order of 66% (Costa et al., 2018); nectarivorous birds in the order of 60% (Miranda et al., 2019); and bees in the order of 85% (Giannini et al., 2020). This last finding makes the results presented here even more noteworthy, since we found a high number of melittophilous plants in our study area. It is important to note that species-specific interaction data are still scarce, which hinders a detailed analysis. For example, plant-bee interactions in the cangas of Carajás have previously been evaluated (Pinto et al., 2020), but there is still no information about the plant species interactions with other animal groups. The global deficit of information on interactions has been previously emphasized (Hortal et al., 2015) and considering the Brazilian Amazon, few studies have evaluated interactions. Some studies analyzed plant species of economic interest pollinated by bees (Cavalcante et al., 2018; Krug et al., 2018; Beyerlein et al., 2019) or by complex systems involving multiple insects (Dattilo et al., 2012; Campbell et al., 2018). A small additional amount of data is available on interactions between plants and pollinators in the region, including bees (Moura et al., 2011; Novais and Absy, 2013; Ferreira and Absy, 2017; Oliveira et al., 2017; Milet-Pinheiro et al., 2018), beetles (Seymour and Matthews, 2006; Gottsberger and Webber, 2018), moths (Cruz-Neto et al., 2011), wasps (Nazareno et al., 2007), birds (Vicentini and Fischer, 1999), and bats (Gribel et al., 1999). None of the quoted studies analyzed the potential impact of climate change on those interactions. Other important feature considering the niche theory above mentioned (Soberón and Peterson, 2005) is related to the species’ ability to reach a site (i.e., mobility and dispersal). For example, enhancing the connectivity of natural habitats where these species occur now and in the future is an important feature that can mitigate the effects of climate change, as they improve the movement of species through suitable habitats (Miranda et al., 2021). Therefore, it is likely that our predictions may underestimate climate change impacts because interacting partners and species dispersion were not explicitly included in the models.
Some previous studies suggest a process of radical alteration of the Amazon biome, especially in the southern-southeastern portion, if no policy is implemented to control the additional loss of forest habitats due to deforestation (Nobre and Borma, 2009; Gomes et al., 2019; Brando et al., 2020). Deforestation intensifies global patterns of climate change effects, which may generate additional and localized variation in temperature and precipitation, creating an even greater impact on biodiversity (Barkhordarian et al., 2019) and further forest dieback (Staal et al., 2020). In fact, a local temperature increase of 1°C and a reduction of 0.8 mm rainfall/day was cited as a consequence of deforestation in the Amazon (Pitman and Lorenz, 2016). Carajás is surrounded by a region that presents high rates of deforestation (Souza-Filho et al., 2016). Our models showed that the proximal areas to the west of Carajás will likely be important areas considering climate change, but most of them are already deforested. We hope that our study can foster restoration strategies on these areas. Our results can also help on providing a useful list of species that are potentially more resistant to climate change, and that can be prioritized aiming to potentially increase the medium- and long-term success of restoration strategies (Harris et al., 2006). This list can also help in the selection of species that can provide resources for different animal groups (Martins and Antonini, 2016; Giannini et al., 2017), which could assist in the restoration of interactions and ecosystem functions in the eastern Amazon. Such initiatives can help in the conservation and protection of biodiversity against the impacts of climate change.
Data Availability Statement
The original contributions presented in the study are included in the article/Supplementary Material, further inquiries can be directed to the corresponding author/s.
Author Contributions
TG and WC: conceptualization. WC, CP, MW, DZ, and AG: data curation. WC, AA, and LM: formal analysis. TG: funding acquisition. TG, AA, and LM: writing—original draft. All authors: review and editing.
Funding
This work was supported by the Conselho Nacional de Desenvolvimento Científico e Tecnológico (National Council for Scientific and Technological Development) (CNPq 446167/2015-0 and 303518/2017-0). LM also acknowledges the CNPq-MPEG “Programa de Capacitação Institucional” (#302202/2020-0 and #300541/2021-0). CP received grants from CNPq (300464/2016-9) and FAPESPA (ICAAF 019/2016).
Conflict of Interest
The authors declare that the research was conducted in the absence of any commercial or financial relationships that could be construed as a potential conflict of interest.
Publisher’s Note
All claims expressed in this article are solely those of the authors and do not necessarily represent those of their affiliated organizations, or those of the publisher, the editors and the reviewers. Any product that may be evaluated in this article, or claim that may be made by its manufacturer, is not guaranteed or endorsed by the publisher.
Supplementary Material
The Supplementary Material for this article can be found online at: https://www.frontiersin.org/articles/10.3389/fpls.2021.699034/full#supplementary-material
Footnotes
References
Aguirre-Gutiérrez, J., Carvalheiro, L. G., Polce, C., van Loon, E. E., Raes, N., Reemer, M., et al. (2013). Fit-for-purpose: species distribution model performance depends on evaluation criteria-Dutch hoverflies as a case study. PLoS One 8:e63708. doi: 10.1371/journal.pone.0063708
Ahuja, I., Vos, R. C. H., Bones, A. M., and Hall, R. D. (2010). Plant molecular stress responses face climate change. Trends Plant Sci. 15, 664–674. doi: 10.1016/j.tplants.2010.08.002
Allouche, O., Tsoar, A., and Kadmon, R. (2006). Assessing the accuracy of species distribution models: prevalence kappa and the true skill statistic (TSS). J. Appl. Ecol. 43, 1223–1232. doi: 10.1111/j.1365-2664.2006.01214.x
Andrino, C. O., Barbosa-Silva, R. G., Lovo, J., Viana, P. L., Moro, M. F., and Zappi, D. C. (2020). Iron islands in the Amazon: investigating plant beta diversity of canga outcrops. PhytoKeys 165, 1–25. doi: 10.3897/phytokeys.165.54819
Barkhordarian, A., Saatchi, S. S., Behrangi, A., Loikith, P. C., and Mechoso, C. R. (2019). A recent systematic increase in vapor pressure deficit over tropical South America. Sci. Rep. 9:15331.
Bertrand, R., Lenoir, J., Piedallu, C., Riofrío-Dillon, G., Ruffray, P., Vidal, C., et al. (2011). Changes in plant community composition lag behind climate warming in lowland forests. Nature 479, 517–520. doi: 10.1038/nature10548
Beyerlein, P., Mendes, A. M. S., and Pereira, H. S. (2019). Floral phenology seed germination and hybrid plants of the Amerindian yam (Dioscorea trifida). Acta Amazon. 49, 167–172. doi: 10.1590/1809-4392201802992
Brando, P. M., Soares-Filho, B., Rodrigues, L., Assunção, A., Morton, D., Tuchschneider, D., et al. (2020). The gathering firestorm in southern Amazonia. Sci. Adv. 6:eaay1632. doi: 10.1126/sciadv.aay1632
Brooks, M. E., Kristensen, K., van Benthem, K. J., Magnusson, A., Berg, C. W., Nielsen, A., et al. (2017). glmmTMB balances speed and flexibility among packages for zero-inflated generalized linear mixed modeling. R J. 9, 378–400. doi: 10.32614/rj-2017-066
Campbell, A. J., Carvalheiro, L. G., Maués, M. M., Jaffé, R., Giannini, T. C., Freitas, M. A. B., et al. (2018). Anthropogenic disturbance of tropical forests threatens pollination services to açaí palm in the Amazon river delta. J. Appl. Ecol. 55, 1725–1736. doi: 10.1111/1365-2664.13086
Cavalcante, M. C., Galetto, L., Maues, M. M., Pacheco, A. J. S., Bomfim, I. G. A., and Freitas, B. M. (2018). Nectar production dynamics and daily pattern of pollinator visits in Brazil nut (Bertholletia excelsa Bonpl.) plantations in central Amazon: implications for fruit production. Apidologie 49, 505–516. doi: 10.1007/s13592-018-0578-y
Chen, I., Hill, J. K., Ohlemüller, R., Roy, D. B., and Thomas, C. D. (2011). Rapid range shifts of species associated with high levels of climate warming. Science 333, 1024–1026. doi: 10.1126/science.1206432
Cleland, E. E., Chuine, I., Menzel, A., Mooney, H. A., and Schwartz, M. D. (2007). Shifting plant phenology in response to global change. Trends Ecol. Evol. 22, 357–365. doi: 10.1016/j.tree.2007.04.003
Colwell, R. K., Brehm, G., Cardelús, C. L., Gilman, A. C., and Longino, J. T. (2008). Global warming elevational range shifts and lowland biotic attrition in the wet tropics. Science 322, 258–261. doi: 10.1126/science.1162547
Corlett, R. T., and Westcott, D. A. (2013). Will plant movements keep up with climate change? Trends Ecol. Evol. 28, 482–488. doi: 10.1016/j.tree.2013.04.003
Costa, W. F., Ribeiro, M., Saraiva, A. M., Imperatriz-Fonseca, V. L., and Giannini, T. C. (2018). Bat diversity in Carajás National Forest (Eastern Amazon) and potential impacts on ecosystem services under climate change. Biol. Conserv. 218, 200–210. doi: 10.1016/j.biocon.2017.12.034
Cotado, A., and Munné-Bosch, S. (2020). Plasticity in the growth habit prolongs survival at no physiological cost in a monocarpic perennial at high altitudes. Ann. Bot. 125, 413–421. doi: 10.1093/aob/mcz202
Cruz-Neto, O., Machado, I. C., Duarte, J., and Lopes, A. V. (2011). Synchronous phenology of hawkmoths (Sphingidae) and Inga species (Fabaceae-Mimosoideae): implications for the restoration of the Atlantic forest of northeastern Brazil. Biodivers. Conserv. 20, 751–765. doi: 10.1007/s10531-010-9975-x
Dattilo, W., Martins, R. L., Uhde, V., Noronha, J. C., Florencio, F. P., and Izzo, T. J. (2012). Floral resource partitioning by ants and bees in a jambolan Syzygium jambolanum (Myrtaceae) agroforestry system in Brazilian Meridional Amazon. Agrofor. Syst. 85, 105–111. doi: 10.1007/s10457-012-9489-5
Debouk, H., de Bello, F., and Sebastià, M. T. (2015). Functional trait changes, productivity shifts and vegetation stability in mountain grasslands during a short-term warming. PLoS One 10:e0141899. doi: 10.1371/journal.pone.0141899
Dellinger, A. S. (2020). Pollination syndromes in the 21st century: where do we stand and where may we go? New Phytol. 228, 1193–1213. doi: 10.1111/nph.16793
Devecchi, M. F., Lovo, J., Moro, M. F., Andrino, C. O., Barbosa-Silva, R. G., Viana, P. L., et al. (2020). Beyond forests in the Amazon: biogeography and floristic relationships of the Amazonian savannas. Bot. J. Linn. Soc. 193, 478–503. doi: 10.1093/botlinnean/boaa025
Díaz, S., and Cabido, S. (1997). Plant functional types and ecosystem function in relation to global change. J. Veg. Sci. 8, 463–474. doi: 10.1111/j.1654-1103.1997.tb00842.x
Fenster, C. B., Armbruster, W. S., Wilson, P., Dudash, M. R., and Thomson, J. D. (2004). Pollination syndromes and floral specialization. Annu. Rev. Ecol. Evol. Syst. 35, 375–403.
Ferreira, M. G., and Absy, M. L. (2017). Pollen analysis of honeys of Melipona (Michmelia) seminigra merrillae and Melipona (Melikerria) interrupta (Hymenoptera: Apidae) bred in central Amazon Brazil. Grana 56, 436–449. doi: 10.1080/00173134.2016.1277259
Fox, J., and Weisberg, S. (2019). car Companion to Applied Regression. CRAN Repository R Project for Statistical Computing.
Giannini, T. C., Costa, W. F., Borges, R. C., Miranda, L., Costa, C. P. W., Saraiva, A. M., et al. (2020). Climate change in the Eastern Amazon: crop-pollinator and occurrence-restricted bees are potentially more affected. Reg. Environ. Change 20:9.
Giannini, T. C., Giulietti, A. M., Harley, R. M., Viana, P. L., Jaffe, R., Alves, R., et al. (2017). Selecting plant species for practical restoration of degraded lands using a multiple-trait approach. Austral Ecol. 42, 510–521. doi: 10.1111/aec.12470
Giulietti, A. M., Giannini, T. C., Mota, N. F. O., Watanabe, M. T. C., Viana, P. L., Pastore, M., et al. (2019). Edaphic endemism in the Amazon: vascular plants of the canga of Carajás Brazil. Bot. Rev. 85, 357–383. doi: 10.1007/s12229-019-09214-x
Gomes, V. H. F., Vieira, I. C. G., Salomão, R. P., and Steege, H. (2019). Amazonian tree species threatened by deforestation and climate change. Nat. Clim. Chang. 9, 547–553. doi: 10.1038/s41558-019-0500-2
Gornish, E. S., and Prather, C. M. (2014). Foliar functional traits that predict plant biomass response to warming. J. Veg. Sci. 25, 919–927. doi: 10.1111/jvs.12150
Gottsberger, G., and Webber, A. C. (2018). Nutritious tissue in petals of Annonaceae and its function in pollination by scarab beetles. Acta Bot. Bras. 32, 279–286. doi: 10.1590/0102-33062017abb0356
Grabherr, G., Gottfried, M., and Pauli, H. (2010). Climate change impacts in alpine environments. Geogr. Compass 4, 1133–1153. doi: 10.1111/j.1749-8198.2010.00356.x
Gribel, R., Gibbs, P. E., and Queiroz, A. L. (1999). Flowering phenology and pollination biology of Ceiba pentandra (Bombacaceae) in Central Amazonia. J. Trop. Ecol. 15, 247–263. doi: 10.1017/s0266467499000796
Harris, J. A., Hobbs, R. J., Higgs, E., and Aronson, J. (2006). Ecological restoration and global climate change. Restor. Ecol. 14, 170–176.
Hijmans, R. J., and Etten, J. (2012). raster: Geographic Analysis and Modeling With Raster Data. R Package Version 2.0-12. Available online at: http://CRAN.R-project.org/package=raster
Hijmans, R. J., Cameron, S. E., Parra, J. L., Jones, P. G., and Jarvis, A. (2005). Very high resolution interpolated climate surfaces for global land areas. Int. J. Climatol. 25, 1965–1978. doi: 10.1002/joc.1276
Hortal, J., Bello, F., Diniz-Filho, J. A. F., Lewinsohn, T. M., Lobo, J. M., and Ladle, R. J. (2015). Seven shortfalls that beset large-scale knowledge of biodiversity. Ann. Rev. Ecol. Evol. Syst. 46, 523–549. doi: 10.1146/annurev-ecolsys-112414-054400
Intergovernmental Panel on Climate Change-IPCC [IPCC] (2014). Climate Change 2014: Synthesis Report. Contribution of Working Groups I II and III to the Fifth Assessment Report of the Intergovernmental Panel on Climate Change. Geneva: IPCC.
Kahmen, S., Poschlod, O., and Schreiber, K. F. (2002). Conservation management of calcareous grasslands. Changes in plant species composition and response of functional traits during 25 years. Biol. Conserv. 104, 319–328. doi: 10.1016/s0006-3207(01)00197-5
Kelly, A. E., and Goulden, M. L. (2008). Rapid shifts in plant distribution with recent climate change. Proc. Natl. Acad. Sci. U.S.A. 105, 11823–11826. doi: 10.1073/pnas.0802891105
Kimball, S., Funk, J. L., Spasojevic, M. J., Suding, K. N., Parker, S., and Goulden, M. L. (2016). Can functional traits predict plant community response to global change? Ecosphere 7:e01602.
Kissling, W. D., Ahumada, J. A., Bowser, A., Fernandez, M., Fernández, N., García, E. A., et al. (2018). Building essential biodiversity variables (EBVs) of species distribution and abundance at a global scale. Biol. Rev. 93, 600–625.
Klanderud, K., and Totland, O. (2005). Simulated climate change altered dominance hierarchies and diversity of an alpine biodiversity hotspot. Ecology 86, 2047–2054. doi: 10.1890/04-1563
Krug, C., Cordeiro, G. D., Schaffler, I., Silva, C. I., Oliveira, R., Schlindwein, C., et al. (2018). Nocturnal bee pollinators are attracted to guarana flowers by their scents. Front. Plant Sci. 9:1072. doi: 10.3389/fpls.2018.01072
Lenoir, J., Gégout, J. C., Marquet, P. A., Ruffray, P., and Brisse, H. A. (2008). Significant upward shift in plant species optimum elevation during the 20th century. Science 320, 1768–1771. doi: 10.1126/science.1156831
Lenth, R. (2019). emmeans: Estimated Marginal Means aka Least-Squares Means. CRAN Repository R Project for Statistical Computing.
Li, X., and Wang, Y. (2013). Applying various algorithms for species distribution modelling. Integr. Zool. 8, 124–135. doi: 10.1111/1749-4877.12000
Martins, R., and Antonini, Y. (2016). Can pollination syndromes indicate ecological restoration success in tropical forests? Restor. Ecol. 24, 373–380. doi: 10.1111/rec.12324
McGill, B. J., Enquist, B. J., Weiher, E., and Westoby, M. (2006). Rebuilding community ecology from functional traits. Trends Ecol. Evol. 21, 178–185. doi: 10.1016/j.tree.2006.02.002
Milet-Pinheiro, P., Silva, J. B. F., Navarro, D. M. A. F., Machado, I. C. S., and Gerlach, G. (2018). Notes on pollination ecology and floral scent chemistry of the rare neotropical orchid Catasetum galeritum Rchb.f. Plant Species Biol. 33, 158–163. doi: 10.1111/1442-1984.12202
Miranda, L. S., Awade, M., Jaffé, R., Costa, W. F., Trevelin, L. C., Borges, R. C., et al. (2021). Combining connectivity and species distribution modeling to define conservation and restoration priorities for multiple species: a case study in the Eastern Amazon. Biol. Conserv. 257, 109148. doi: 10.1016/j.biocon.2021.109148
Miranda, L. S., Imperatriz-Fonseca, V. L., and Giannini, T. C. (2019). Climate change impact on ecosystem functions provided by birds in southeastern Amazonia. PLoS One 14:e0215229. doi: 10.1371/journal.pone.0215229
Mota, N. F. O., Watanabe, M. T. C., Zappi, D. C., Hiura, A. L., Pallos, J., Viveros, R. S., et al. (2018). Amazon canga: the unique vegetation of Carajás revealead by the list of seed plants. Rodriguesia 69, 1435–1488.
Moura, T. N., Webber, A. C., and Melo-Torres, L. N. (2011). Floral biology and a pollinator effectiveness test of the diurnal floral visitors of Tabernaemontana undulata Vahl. (Apocynaceae) in the understory of Amazon Rainforest Brazil. Acta Bot. Brasilica 25, 380–386. doi: 10.1590/s0102-33062011000200014
Nazareno, A. G., Silva, R. B. Q., and Pereira, R. A. S. (2007). Fauna of hymenoptera in Ficus spp. (Moraceae) in the central Amazon Brazil. Iheringia 97, 441–446. doi: 10.1590/s0073-47212007000400013
Nobre, C. A., and Borma, L. D. S. (2009). “Tipping points” for the Amazon forest. Curr. Opin. Environ. Sustain. 1, 28–36. doi: 10.1016/j.cosust.2009.07.003
Novais, J. S., and Absy, M. L. (2013). Palynological examination of the pollen pots of native stingless bees from the Lower Amazon region in Para Brazil. Palynology 37, 218–230. doi: 10.1080/01916122.2013.787127
Oliveira, P. E., Tome, C. E. R., Torezan-Silingardi, H. M., Dotterl, S., Silberbauer-Gottsberger, I., and Gottsberger, G. (2017). Differential pollination modes between distant populations of Unonopsis guatterioides (Annonaceae) in Minas Gerais and Amazonas Brazil. Flora 232, 39–46.
Ollerton, J., Alarcon, R., Waser, N. M., Price, M. V., Watts, S., Cranmer, L., et al. (2009). A global test of the pollination syndrome hypothesis. Ann. Bot. 103, 1471–1480. doi: 10.1093/aob/mcp031
Pacifici, M., Foden, W. B., Visconti, P., Watson, J. E. M., Butchart, S. H. M., Kovacs, K. M., et al. (2015). Assessing species vulnerability to climate change. Nat. Clim. Chang. 5, 215–225.
Panchen, Z. A., Primack, R. B., Nordt, B., Ellwood, E. R., Stevens, A. D., Renner, S. S., et al. (2014). Leaf out times of temperate woody plants are related to phylogeny, deciduousness, growth habit and wood anatomy. New Phytol. 203, 1208–1219. doi: 10.1111/nph.12892
Phillips, S. B., Aneja, V. P., Kang, D., and Arya, S. P. (2006). Modelling and analysis of the atmospheric nitrogen deposition in North Carolina. Int. J. Global Environ. Issues 6, 231–252. doi: 10.1504/ijgenvi.2006.010156
Pinto, C. E., Awade, M., Watanabe, M., Costa, W. F., Brito, R. M., Maia, U. M., et al. (2020). Size and isolation of naturally isolated habitats do not affect plant-pollinator interactions: a case study of the ferruginous outcrops inside the eastern Amazon forest. PLoS One 15:e0238685. doi: 10.1371/journal.pone.0238685
Pitman, A. J., and Lorenz, R. (2016). Scale dependence of the simulated impact of Amazonian deforestation on regional climate. Environ. Res. Lett. 11:094025. doi: 10.1088/1748-9326/11/9/094025
Ribeiro, B. R., Sales, L. P., and Loyola, R. (2018). Strategies for mammal conservation under climate change in the Amazon. Biodivers. Conserv. 27, 1943–1959. doi: 10.1007/s10531-018-1518-x
Rosas-Guerrero, V., Aguilar, R., Martén-Rodríguez, S., Ashworth, L., Lopezaraiza-Mikel, M., Bastida, J. M., et al. (2014). A quantitative review of pollination syndromes: do floral traits predict effective pollinators? Ecol. Lett. 17, 388–400. doi: 10.1111/ele.12224
Seymour, R. S., and Matthews, P. G. D. (2006). The role of thermogenesis in the pollination biology of the Amazon waterlily Victoria amazonica. Ann. Bot. 98, 1129–1135. doi: 10.1093/aob/mcl201
Soberón, J., and Peterson, A. T. (2005). Interpretation of models of fundamental ecological niches and species’ distributional areas. Biodivers. Inform. 2, 1–10.
Soliani, C., and Aparicio, A. G. (2020). Evidence of genetic determination in the growth habit of Nothofagus pumilio (Poepp. & Endl.) Krasser at the extremes of an elevation gradient. Scand. J. Forest Res. 35, 211–220. doi: 10.1080/02827581.2020.1789208
Soudzilovskaia, N. A., Elumeeva, T. G., Onipchenko, V. G., Shidakov, I. I., Salpagarova, F. S., Khubiev, A. B., et al. (2013). Functional traits predict relationship between plant abundance dynamic and long-term climate warming. Proc. Natl. Acad. Sci. U.S.A. 110, 18180–18184. doi: 10.1073/pnas.1310700110
Souza-Filho, P. W. M., Giannini, T. C., Jaffé, R., Giulietti, A. M., Santos, D. C., and Nascimento, W. R. Jr., et al. (2019). Mapping and quantification of ferruginous outcrop savannas in the Brazilian Amazon: a challenge for biodiversity conservation. PLoS One 14:e0211095. doi: 10.1371/journal.pone.0211095
Souza-Filho, P. W. M., Souza, E. B., Silva, R. O. Jr., Nascimento, W. R., Mendonça, B. R. V., Guimarães, J. T. F., et al. (2016). Four decades of land-cover land-use and hydroclimatology changes in the Itacaiúnas River watershed southeastern Amazon. J. Environ. Manag. 167, 175–184. doi: 10.1016/j.jenvman.2015.11.039
Staal, A., Flores, B. M., Aguiar, A. P. D., Bosmans, J. H. C., Fetzer, I., and Tuinenburg, O. A. (2020). Feedback between drought and deforestation in the Amazon. Environ. Res. Lett. 15:044024. doi: 10.1088/1748-9326/ab738e
Stang, M., Klinkhamer, P. G. L., and Van Der Meijden, E. (2007). Asymmetric specialization and extinction risk in plant-flower visitor webs: a matter of morphology or abundance? Oecologia 151, 442–453. doi: 10.1007/s00442-006-0585-y
Steinbauer, M. J., Grytnes, J. A., Jurasinski, G., Kulonen, A., Lenoir, J., Pauli, H., et al. (2018). Accelerated increase in plant species richness on mountain summits is linked to warming. Nature 256, 231–234.
Thuiller, W. (2003). BIOMOD-optimizing predictions of species distributions and projecting potential future shifts under global change. Global Change Biol. 9, 1353–1362. doi: 10.1046/j.1365-2486.2003.00666.x
Thuiller, W., Lafourcade, B., Engler, R., and Araújo, M. B. (2009). BIOMOD–a platform for ensemble forecasting of species distributions. Ecography 32, 369–373. doi: 10.1111/j.1600-0587.2008.05742.x
Velazco, S. J. E., Villalobos, F., Galvão, F., and De Marco Júnior, P. (2019). A dark scenario for Cerrado plant species: effects of future climate, land use and protected areas ineffectiveness. Divers. Distrib. 25, 660–673. doi: 10.1111/ddi.12886
Venter, O., Sanderson, E. W., Magrach, A., Allan, J. R., Beher, J., Jones, K. R., et al. (2016). Sixteen years of change in the global terrestrial human footprint and implications for biodiversity conservation. Nat. Commun. 7, 1–11.
Vicentini, A., and Fischer, E. A. (1999). Pollination of Moronobea coccinea (Clusiaceae) by the golden-winged parakeet in the central Amazon. Biotropica 31, 692–696. doi: 10.1111/j.1744-7429.1999.tb00419.x
Keywords: Amazon biome, biodiversity, canga, conservation, mutualism, plant-pollinator interaction
Citation: Giannini TC, Acosta AL, Costa WF, Miranda L, Pinto CE, Watanabe MTC, Zappi DC, Giulietti AM and Imperatriz-Fonseca VL (2021) Flora of Ferruginous Outcrops Under Climate Change: A Study in the Cangas of Carajás (Eastern Amazon). Front. Plant Sci. 12:699034. doi: 10.3389/fpls.2021.699034
Received: 22 April 2021; Accepted: 18 August 2021;
Published: 07 September 2021.
Edited by:
Péter Török, University of Debrecen, HungaryReviewed by:
Ping Ren, Anhui Normal University, ChinaSara Reverté, University of Mons, Belgium
Patricia Morellato, São Paulo State University, Brazil
Copyright © 2021 Giannini, Acosta, Costa, Miranda, Pinto, Watanabe, Zappi, Giulietti and Imperatriz-Fonseca. This is an open-access article distributed under the terms of the Creative Commons Attribution License (CC BY). The use, distribution or reproduction in other forums is permitted, provided the original author(s) and the copyright owner(s) are credited and that the original publication in this journal is cited, in accordance with accepted academic practice. No use, distribution or reproduction is permitted which does not comply with these terms.
*Correspondence: Tereza Cristina Giannini, tereza.giannini@itv.org