- 1Poyang Lake Eco-economy Research Center, Jiujiang University, Jiujiang, China
- 2School of Water Resources and Hydropower Engineering, Wuhan University, Wuhan, China
- 3School of Water Conservancy and Environment, China Three Gorges University, Yichang, China
- 4Qingdao Institute of Bioenergy and Bioprocess Technology, Chinese Academy of Sciences, Qingdao, China
- 5School of Architectural and Environment, Hubei University of Technology, Wuhan, China
This study showed the new potential of using soluble contents and heavy metals in swine wastewater as nutrient supplements for the algae Chlorella pyrenoidosa with biofilm attached method. Algae with biofilm attached cultivation grew well in unpasteurized wastewater reaching a biomass productivity of 5.03 g m−2 d−1, lipid content of 35.9% and lipid productivity of 1.80 g m−2 d−1. Chlorella grew in BG11 medium delivered lower values for each of the aforementioned parameters. The FAMEs compositions in the algae paste were mainly consisted of C16:0, C18:2, and C18:3. Algae removed NH4+–N, total phosphorus (TP), and COD by 75.9, 68.4, and 74.8%, respectively. Notably, Zn2+, Cu+, and Fe2+ were removed from wastewater with a ratio of 65.71, 53.64, and 58.89%, respectively. Biofilm attached cultivation of C. pyrenoidosa in swine wastewater containing heavy metals could accumulate considerable biomass and lipid, and the removal ratio of NH4+–N, TP, COD, and as well as heavy metal were high. Treatment of wastewater with biofilm attached cultivation showed an increasingly popular for the concentration of microalgae and environmental sustainability.
Introduction
Large quantities of waste continuously produced from the intensive livestock industries worldwide represent an increasingly concerning threat to the environment, especially when they were not treated properly. Excessive nutrients in the piggery wastewater, such as, nitrogen and phosphorus, will cause eutrophication in natural water bodies (Cai et al., 2013; Ayre et al., 2017). Compared with municipal domestic sewage-based wastewater, swine wastewater, which is often derived from manure, may contain very high amounts of N and P and may also contain toxic metals (Sturm and Lamer, 2011; Zhou et al., 2012). The principle in wastewater treatment is the reduction of nutrient and toxic metal to acceptable limits prior to discharge and reuse (Miranda et al., 2017). The majority of examples for wastewater treatment technologies were based on chemical or physical methods that were not economical for livestock wastewater treatment (Xu et al., 2015; Li et al., 2016).
As we known, microalgae are considered promising for production of biofuels and higher value products, but require a lot of nutrients in their growth, resulting in high operation costs and possibly adverse environmental effects due to nutrient leakage into the environment (Mata et al., 2010; Borowitzka and Moheimani, 2013; Shah et al., 2016). Therefore, application of wastewater rich in nutrients, such as, nitrogen and phosphorus, for fertilizer-driven microalgal cultivation is a promising approach to enhance economic and environmental sustainability (Mulbry et al., 2008). In particular, the genus Chlorella is commonly used in the wastewater treatment system due to their high tolerance to soluble organic compounds.
Nitrogen and phosphorus are removed from wastewater in two separate processes in conventional wastewater treatment. Nitrogen is usually converted into N2 gas through coupled nitrification–denitrification, whereas phosphorus is precipitated with metal salts. By contrast, nitrogen and phosphorus could be removed from wastewater in a single process with the use of microalgae (Christenson and Sims, 2011; Abdelaziz et al., 2013; Beuckels et al., 2015). Microalgae absorb nitrogen and phosphorus from swine wastewater and convert these nutrients into biomass. Kothari et al. (2012) found that Chlorella pyrenoidosa could remove about 80–85% total phosphorus (TP) and 60–80% of total nitrogen (TN) from dairy wastewater. Markou et al. (2012) also found that the maximum removal of chemical oxygen demand (COD) was 73.18%, while phenols, phosphorus, and nitrates in some runs were completely removed when cultivating Arthrospira platensis in olive-oil mill wastewater.
However, studies of microalgae-based wastewater treatment were generally based on commercial microalgae cultivation systems, such as, open ponds or closed photobioreactors (PBRs), which exhibited relatively low biomass productivity, high water requirements and high liquid transportation and cumbersome and higher costs in harvesting (Ozkan et al., 2012; Berner et al., 2015). This research, we introduced a novel cultivation system, which was called “biofilm attached cultivation” by Liu et al. (2013). Unlike traditional cultivation methods based on suspended cultures of cells in aqua-medium, the biofilm attached cultivation is a different technology in which the algal cells are immobilized and settled on artificial supporting materials in high density. In brief, microalgal cells are attached to a supporting structure to form an artificial “leaf” and multiple of these leaves are vertically inserted into a glass chamber in this system (Cheng et al., 2013; Liu et al., 2013). Biofilm attached cultivation of microalgae in wastewater separated algae cells from wastewater instead of concentrating or filtering, and the treated wastewater can be recycled immediately. However, there were rarely reports that investigated the feasibility of using wastewater as nutrient supplements for biofilm attached cultivation of microalgae.
In this study, an integrated approach which combined freshwater microalgae C. pyrenoidosa biofilm attached cultivation with heavy metals rich swine wastewater treatment was investigated. The objectives of our study were: (1) to determine a feasibility study with undiluted piggery wastewater for algal cultivation, (2) to specify the productivities of biomass, lipids, and FAMEs, and (3) to reveal relevant nutrient and heavy metals removal abilities.
Materials and Methods
Algal Strain and Inoculum Preparation
The microalgae strain C. pyrenoidosa which was acclimated in poultry wastewater, was obtained from Hubei University of Technology. As we mentioned before, in preparing the inoculum for biofilm attached bioreactors, the alga was first cultivated in glass bubbling columns (diameter = 0.05 m) until the exponential growth phase was reached (approximately 5 days). The glass columns contained 0.6 L of algal broth and were continuously illuminated by cold-white fluorescent lamps (NFL28-T5, NVC, China) with a light intensity of 100 ± 10 μmol m−2s−1. The broth temperature was 20 ± 2°C. CO2 enriched air (1% v/v) was continuously injected into the bottom of the columns with a speed of 1 vvm (0.7 L/min for each column) to agitate the algal broth as well as supply carbon (Cheng et al., 2013).
Culture Medium and Photobioreactor
In this research, the algae cultivated in glass bubbling columns and biofilm attached cultivation system (control) was grown in a BG11 medium (Chiu et al., 2015), each liter of which contains 1.5 g NaNO3, 0.075 g MgSO4·7H2O, 0.036 g CaCl2·2H2O, 0.04 g KH2PO4·H2O, 0.02 g Na2CO3, 6.0 × 10−3 g citric acid, 1.0 × 10−3 g Na2EDTA, 6.0 × 10−3 g ferric ammonium citrate, 2.22 × 10−4 g ZnSO4·7H2O, 6.9 × 10−5 g CuSO4·5H2O, 1.81 × 10−3 g MnCl2·4H2O, 3.9 × 10−4 g Na2MoO4·2H2O, 4.94 × 10−5 g Co(NO3)2·6H2O, and 2.86 × 10−3 g H3BO3.
The biofilm attached cultivation system applied in this research was similar to the type 1 reactor used by Liu et al. (2013) and to that described by Cheng et al. (2014) (Figure 1). In brief, a glass chamber comprising a glass plate and attached algal biofilm disks was placed on an iron rack with a certain tilt angle against the horizontal plane. The medium was propelled (1.2 L in total, approximately 10 ml min−1) by a peristaltic pump (TP12DC 12V, Guangzhou JU PlasFitting Technology Co., Ltd., China) to facilitate circulation inside the system. Cold-white fluorescent lamps provided illumination at 100 ± 10 μmol m−2s−1 as measured inside the chamber at the position of the cultivated algae cells. Continuous air was injected into the glass chamber with a speed of 0.1 vvm and the temperature inside the glass chamber was 20 ± 2°C during the experiments. The culture time of 8 days for C. pyrenoidosa with biofilm attached culture was proved well in our trial test.
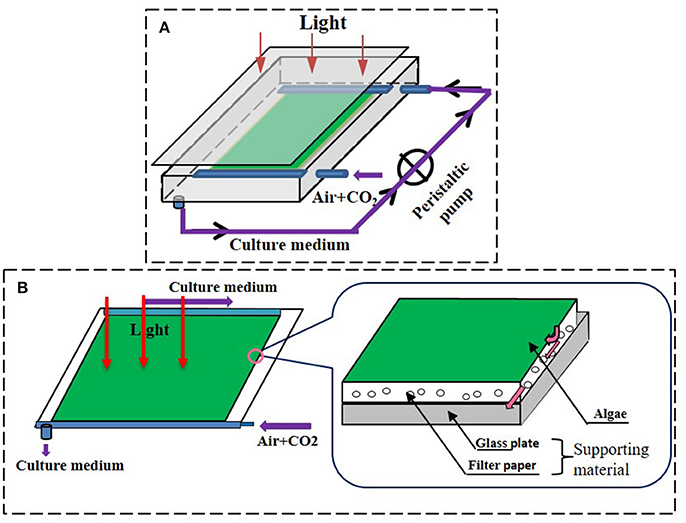
Figure 1. The schematic diagrams of attached cultivation devices. (A) Attached cultivation module of the photobioreactor, the residual medium was recycling. The medium was propelled to the system by a peristaltic pump when it flowed through the chamber during the cultivation. (B) The detailed structure of the cultivation surface of the attached photobioreactor. Permission to use and adapted from Cheng et al. (2014).
Culture of Chlorella pyrenoidosa with Swine Wastewater
Swine wastewater from a private farm in Wuhan of Hubei province, China, was used as a medium to cultivate C. pyrenoidosa. Pre-treatment was carried out by sedimentation and filtration with a filter cloth to remove large and non-soluble particulates. After filtration, the wastewater was not autoclaved and undiluted but used directly as a medium for algal cultivation in the biofilm attached PBR. The culture conditions for control group (BG11 medium) and the raw swine wastewater were identical to those described the above section of biofilm attached cultivation system. The characteristics of the raw swine wastewater are summarized in Table 1. Remarkably, the wastewater contained heavy metals of Zn2+, Cu+, and Fe2+.
Analytical Procedures
Biomass Estimation
The biomass was determined with the gravimetric method (Liu et al., 2013). During the experiments, two algae disks would be collected every 2 days; cells from the filter membrane were flushed with distilled water and then filtered to a pre-weighed 0.45 μm GF/C filter membrane (Whatman, England; DW0). The membrane was oven dried at 80°C for about 24 h and then cooled down to room temperature to measure dry weight (DW1). Finally, their average was used. The DW was calculated as follows:
where 0.001 represented the footprint area of the “algal disk” (m2).
Nutrients Analysis in Wastewater
A volume of 5 mL of recirculating medium was collected every 2 days from the attached PBRs for nutrient removal analysis. The samples were first centrifuged at 1,500 g for 10 min, and then the supernatants were filtered using a 0.45 mm nylon membrane filter. The filtrates were appropriately diluted and analyzed for COD, NH4+–N, and TP, parallel sample was made. The COD was measured using the standard potassium dichromate method based on the Chinese National Standard GB11914-1989 with a Speed Digester (Yao et al., 2015). Potentiometric analysis using a selective electrode method was used to measure ammonia (N–NH3) (APHA, 2012). TP were measured according to Standard Methods (Eaton et al., 2005; Hach, 2008).
Heavy Metal Elements Alterations
A volume of 50 mL of culture medium was gathered, respectively, at the second day, the fourth day, the sixth day, and the last day according to the limiting of the broth, and was examined the concentration of heavy metal of copper, zinc, iron with atom absorption spectrographic methods (PinAAcle 900T, USA).
Lipid Extraction
The attached algal cells were harvested by washing down with de-ionized water and centrifugation at 3,800 g for 10 min (Allegra X-22R, Beckman coulter, USA). The algal pellets were washed three times with de-ionized water to remove any attached salt. Then the total lipid was measured according to Bligh and Dyer's method (Bligh and Dyer, 1959) and Cheng et al. (2013).
FAMEs Content Analysis of Algae Biodiesel
FAMEs content analysis was according to Chen et al. (2012) and Wang et al. (2016). Briefly, algae powder was suspended in 2 mL 0.4 M KOH methanol solution, and was then heated at 70°C for 30 min in a water bath. After cooling, 2 mL 0.6 M H2SO4-methanol solution and 1 mL 14% BF3-methanol solution (Sigma-Aldrich, USA) were added, the mixture was heated in water bath at 70°C for 30 min again. After cooling, the fatty acid methyl esters (FAMEs) were extracted with 2 mL n-hexane, then centrifuged at 4,000 rpm for 5 min. The n-hexane layer was transferred to a vial. The prepared sample was (0.5 mg) was dissolved in heptane (1 mL) containing heptadecanoic acid methyl ester (C18H37COOCH3, 50 μg) as internal standard for FAMEs analysis on a Varian 450GC (Varian Inc., USA) equipped with a flame ionization detector (FID) and Agilent HP-5 GC Capillary Column (30 m × 0.25 mm × 0.25 μm). Nitrogen was used as carrier gas. The injector temperature was set at 280°C with an injection volume of 2 μL under split mode (10:1). The detector temperature was set at 280°C. The individual FAMEs were identified by chromatographic comparison with authentic standards (Sigma).
All experiments were performed in duplicate, and average values were reported. Results were analyzed with EXCEL (Microsoft Office Enterprise, 2010) and SPSS 11.5 for Windows (SPSS Inc., 2007); ANOVA was performed when applicable.
Results
Accumulation of Biomass of Chlorella pyrenoidosa with Biofilm Attached Cultivation
The growth curves of C. pyrenoidosa in swine wastewater for 8 days were showed in Figure 2. For the first 2 days, the growth was almost identical with that in BG11. After that, C. pyrenoidosa grew faster in swine wastewater. By the end of experiment, biomass and biomass productivity were 48.02 g m−2 and 5.03 g m−2 d−1, respectively, for the wastewater treatment. They were higher than that of control treatment (BG11). A smaller yet still statistically significant increase was also found under the swine wastewater for biomass productivity (repeated measures one-way ANOVA) corresponding to a mean increase of 7.3% of control.
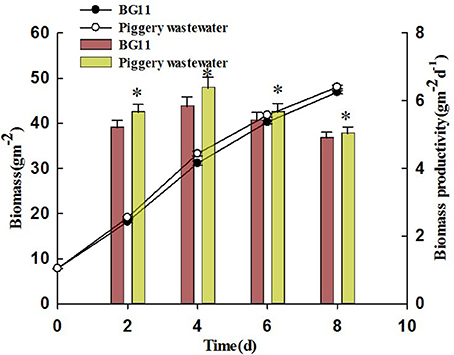
Figure 2. The accumulation of biomass of Chiarella pyrenoidosa in BG 11 (control), piggery wastewater with biofilm attached cultivation. The algal cells were cultivated in attached culture for 8 days under continuous illumination of 1 00 ± 10 μmol m−2 s−1. *Indicates significant differences among treatments (P < 0.05), Error bars are standard deviations.
Conventionally, in the lag state, algal cells need to adapt to the new environment since the nutrients contained in the wastewater are different from those found inside the BG11 medium. In this study, the microalgae cultivated in the biofilm attached PBR with the COD concentration of 601 mg L−1 had a short lag time and could quickly adapt to the environment.
Lipid Productivity and Analysis of the Fatty Acid Profile
The lipid content of C. pyrenoidosa in the two treatments was shown in Figure 3. The lipid content of the algae in the treatment with BG11 and swine wastewater was similar, reaching 34.6 and 35.9% and corresponded to a lipid productivity of 1.69 and 1.80 g m−2 d−1, respectively. So, there were no statistically different between the two treatments.
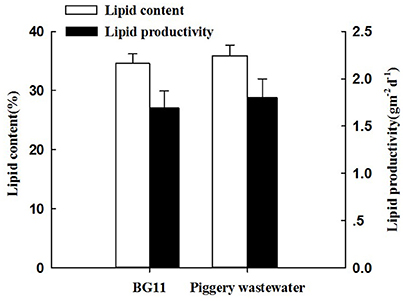
Figure 3. The lipid content and lipid productivity of Chlorella pyrenoidosa in the treatment of BG 11 (control) and piggery wastewater with biofilm attached cultivation. The algal cells were cultivated in biofihn attached culture for 8 days under continuous illumination of 100 ± 10 μmol m−2 s−1. Error bars are means ± standard deviations of three replicates.
The compositional distribution of fatty acids extracted from the microalgae is shown in Table 2. In the present study, the FAMEs compositions of C. pyrenoidosa mainly consisted of C16:0 (palmitic acid methyl ester), C18:2 (octadecadienoic acid methyl ester), and C18:3 (octadecatrienoic acid methyl ester). The highest overall FAMEs yield was obtained in piggery wastewater with C18:3 (43.39% of the total fatty acids) as the most abundant fatty acid, which was higher than in BG11 medium (32.14% of the total fatty acids). Linoleic acid (C18:2) content reached 37.38% of the total fatty acids in wastewater, only 25.47% in BG11 medium. Nevertheless, the fraction of oleic acid was higher with BG11 (27.22% of the total fatty acids) than when using swine wastewater as a source of nutrients (4.43% of the total fatty acids). The fraction of palmitic acid methyl ester (C16:0) in the two treatments was similar, corresponding to 8.63% in wastewater and 9.30% in BG11. When the algae cells cultivated in BG11 media were transferred to the swine wastewater, polyunsaturated fatty acid content increased from 57.61 to 81.17%, however, the amount of monounsaturated fatty acids decreased from 27.82 to 5.08%. The unsaturated FAMEs for C16:1, C18:2, and C18:3 in biofilm attached cultures with piggery wastewater were predominant in the FAMEs profile.
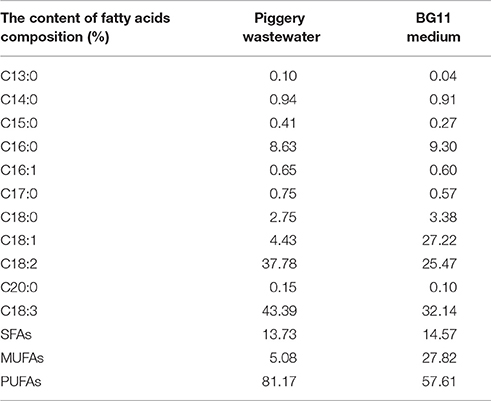
Table 2. Effect of piggery wastewater and BG11 medium on the fatty acids composition as % of total fatty acids of Chlorella Pyrenoidosa with biofilm attached cultivation for 8 days.
Nitrogen, Phosphorus, and COD Removal Capability
Figures 4, 5 describe the removals of NH4+–N, TP, and COD achieved with treatment of piggery wastewater by microalgae cultivation. In this study, the cultivation of C. pyrenoidosa in the form of a biofilm receiving wastewater as a source of nutrients decreased the initial NH4+–N concentration from 409 to 98 mg L−1, and TP concentration from 35 to 10 mg L−1, and the initial COD concentration from 601 to 152 mg L−1. This corresponded to a removal ratio of NH4+–N, TP, and COD of 75.9, 68.4, and 74.8%, respectively.
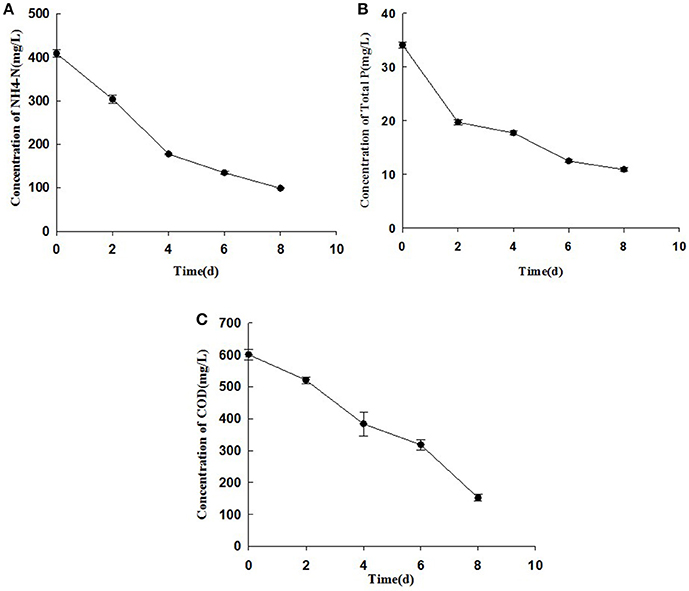
Figure 4. The changes of concentrations of NH4+–N (A), TP (B), and COD (C) of cultivation broth by Chiarella pyrenoidosa with biofilm attached cultivation. The algal cells were cultivated in biofilm attached culture for 8 days under continuous illumination of 100 ± 10 μmol m−2 s−1. Error bars are means ± standard deviations of three replicates.
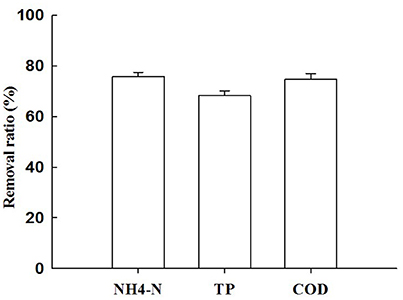
Figure 5. The removal ratio ofNH4+–N, TP, and COD with treatment ofpiggery wastewater by Chiarella pyrenoidosa biofilm attached cultivation. The algal cells were cultivated in biofilm attached culture for 8 days under continuous illumination of 100 ± 10 μmol m−2 s−1. Error bars are means ± standard deviations of three replicates.
The typical N/P ratio for optimal conditions for microalgae biomass production was 8:1 (US DOE, 2008); however, the N/P ratios in our swine wastewater were higher than that of values (Table 1). Current literature has reported that algae can assimilate NH4+–N, nitrate, and simple organic nitrogen such as, urea, acetic acid, and amino acids in wastewater; however, the concentration of NH4+–N of <300 mg L−1 in the accessible paper (Beuckels et al., 2015).
Heavy Metal Elements Disposal Ratio
The swine wastewater in this study was detected some of high concentration of heavy metals ions. Table 1 showed that the concentration of Zn2+ in swine wastewater reached 2.8 mg L−1, followed by Cu+ and Fe2+ (2.2 and 1.8 mg L−1, respectively). Heavy metals removal from swine wastewater by microalgae with attached culture has been tested (Figure 6). As shown in Figure 6A, the metal concentrations (Zn2+, Cu+, and Fe2+) decreased sharply after only 2 days of cultivation. The reduction rate greatly decreased in the following days. By the end of experiment, residual quantity of Zn2+, Cu+ and Fe2+ were still detected reaching concentrations of 0.96, 1.02, and 0.74 mg L−1, respectively (Figure 6A).
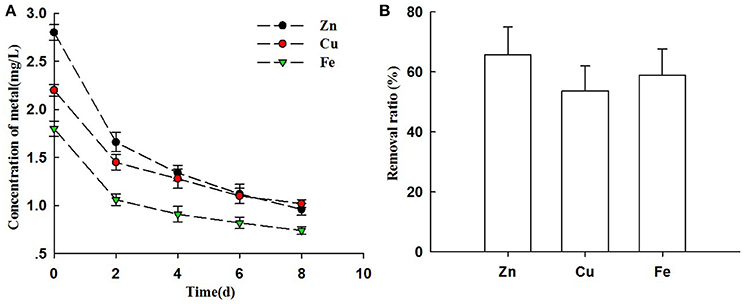
Figure 6. Heavy metals ions removal from piggery wastewater by biofihn attached culture (A) The changes of concentrations of Zn2+, Cu+, and Fe2+ of cultivation broth by Chlorella pyrenoidosa with biofilm attached cultivation. (B) The removal ratio of Zn2+, Cu+, and Fe2+ with treatment of piggery wastewater by Chlorella pyrenoidosa biofilm attached cultivation. The algal cells were cultivated in biofilm attached culture for 8 days under continuous illumination of 100 ± 10 μmol m−2 s−1. Error bars are means ± standard deviations of three replicates.
This result indicated the high removal ratio of Zn2+ (65.71%), Cu+ (53.64%), and Fe2+ (58.89%) with the attached cultivation of Chlorella (Figure 6B). Our results are supported from Carrilho and Gilbert (2000) who revealed that biosorption of algae was rapid and accumulation of metals happened within short time (Figure 6A).
Discussion
Ayre et al. (2017) reported that many microalgae species can grow in diluted digested piggery wastewater, and they utilize the abundant N, P, and other organic carbon in the water. Chlorella sp. was the dominant algae species in a wastewater treatment study. However, nearly all experiments were performed on piggery wastewater diluted with either synthetic media or water to reduce the toxicity of organic components (Min et al., 2011; Zhu et al., 2011). Min et al. (2011) and Zhu et al. (2011) also cultivated Chlorella sp. in swine wastewater with relatively high COD in a pilot-scale PBR, however, in their experiment the biomass productivity and initial NH4+–N concentration of wastewater were lower than our experiment (Figure 2). Flynn (1991) reported that ammonium assimilation causes a rapid and reversible inactivation of nitrate transport which influences growth of microalgae.
The dilution of swine wastewater for microalgae growth would be limited by the high cost of clean water consumption, sewage volume, need for artificial nutrients, and other inconvenient operations. Therefore, developing an efficient process that allow algae to grow well under undiluted piggery slurry is very important, rendering the application of microalgae-based technology feasible and economical for full-scale wastewater treatment and biodiesel production. This study aimed at investigating the feasibility of tested microalgae in undiluted slurry of piggery wastewater, and the microalgae treated with the biofilm attached culture method grew well in undiluted slurry.
Feng et al. (2012) and Rodolfi et al. (2009) proved that lipid accumulation can be raised through increasing light intensity. The wastewater had a darker color than the BG11; however, lipid accumulation for the swine wastewater treatment was not inhibited in biofilm attached cultivation. This might be because the algal cells cultivated with attached PBR are generally immobilized and fixed onto supporting materials in high density and separated with swine wastewater. Chlorella sp. are known to have high content of lipids, which have the potential to be converted to biofuels that can be used mixed to—or in substitution to traditional fossil fuels. Beal et al. (2012) reported the cost of algal biofuel production was mainly related to algal culture (77%), harvesting (12%), and lipid extraction phases (7.9%). It was obvious that the utilization of wastewater as a substitute for algae nutrients would significantly reduce the operational cost of algae cultivation (Cai et al., 2013).
The environmental and cultural conditions have been reported by previous research to have an effect on lipid content and fatty acid (Petkov and Garcia, 2007; Li et al., 2011). So, it was not surprising to observe the different FAMEs profiles in our study (Table 2). Not all lipids can be converted to FAMEs, such as, glycolipids and phospholipids and long-chain carbon (Li et al., 2011). However, C. pyrenoidosa cultivated in swine wastewater was found to have shorter carbon chains for fatty acids in our study. The degree of unsaturation of fatty acids is due to the metals toxicity more than to favoritism of piggery wastewater. They mainly contained 16–18 carbons (Table 2), which were ideal for biodiesel conversion (Huang et al., 2010). Integration of microalgal cultivation with wastewater for biodiesel production is a promising choice and could enhance the economic and environmental sustainability (Lee and Lee, 2002).
The high NH4+–N content in wastewater represents a growth inhibitory factor for microalgae because it may contribute to changes in pH value of the culture medium. Tam and Wong (1996) showed that the growth of Chlorella sp. was reduced at NH4+–N concentrations higher than 700 mg L−1 with a pH level below 7. Chiu et al. (2015) reported that the TP concentration for biomass productivity was lower than 100 mg L−1. The consumed phosphorus of swine wastewater was mainly assimilated by algal cells. The effects of NH4+–N and TP concentrations in wastewater on the Chlorella biomass production and productivity are similar (Chiu et al., 2015). The consumed COD in this study with attached cultivation of Chlorella were higher than the reported that of 73.18% COD removal ratio with Arthrospira (Spirulina) platensis cultivated in 25% olive oil mill wastewater (Markou et al., 2012).
The main physicochemical approaches to remove heavy metal ions from wastewaters include ion exchange, chemical precipitation, electrokinetic, membrane processing, and adsorption (Lee and Pandey, 2012; Goharshadi and Moghaddam, 2015). The incomplete removal of heavy metal ions and high costs of chemicals are the main limiting factors in the development of physicochemical approaches. However, biosorption of heavy metal ions in wastewater with proper microalgae species could offer an ecologically safer, cheaper, and more efficient means. Indeed, algae could be used to absorb toxic and radioactive metal ions and to recover precious metals (Pohl and Schimmack, 2006). And polysaccharides and proteins present in algae cell walls contain the most number of metal binding sites (Schiewer and Volesky, 2000). However, factors including concentration of metal ions, algae biomass, pH, temperature, and presence of competing ions affect the biosorption of heavy metals ions by algae. Algal cell walls are the first barrier against biosorption of heavy metal ions. Given the different distribution and abundance of cell wall compositions in different algal strains, the biosorption capacity for metal ions would vary depend on the considered algal strain.
Chlorella can effectively grow and utilize nutrients in swine wastewater, reducing the content of metal ions in this research. Table 3 compared of the growth, lipid production, and cultivation parameters of microalgae in wastewater for different cultivation methods. It could be observed that most of study concerned of algae-based treatment diluted wastewater (Ji et al., 2013), which would increase cost in practice. In addition, few papers focused on the question of heavy metal pollution of piggery wastewater, which recently has attracted growing attention to agricultural pollution (Zeraatkar et al., 2016). The cultivation scale of most recent studies was limited to the laboratory bench—or pilot plant scale. The significant question to consider is what kind of cultivation system could be more economically viable and environmental-friendly for large scale production of microalgal biomass. As a result of the difficulty of construction and the condition of vast land area, most of the PBRs and ponds used in recent studies are not economically convenient for large-scale microalgal biomass production. Therefore, the efforts on novel cultivation system are very important in the future research (Wang et al., 2015).
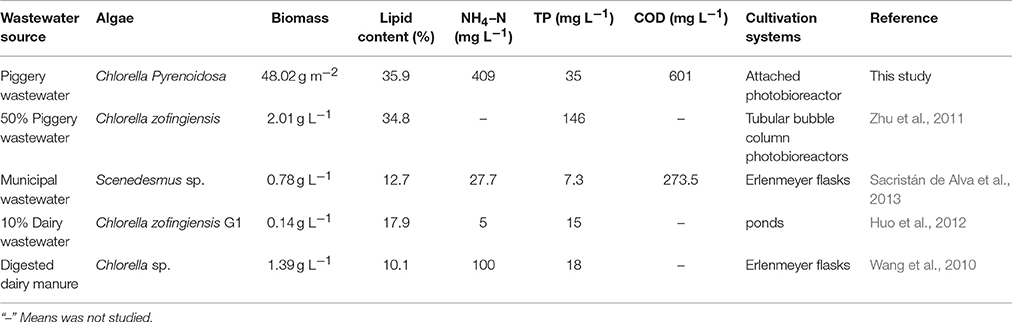
Table 3. Comparison of growth, lipid production and cultivation parameters of microalgae in wastewater for different cultivation methods.
In summary, C. pyrenoidosa could accumulate considerable biomass and lipid in swine wastewater with biofilm attached culture. A major portion of FAMEs compositions were ideal for biodiesel conversion. The removal of NH4+–N, TP, COD and, as well as heavy metal pollution of wastewater with C. pyrenoidosa by biofilm attached culture can be a remarkable solution for swine wastewater resource utilization.
Author Contributions
PC and DL proposed the idea and hypothesis. PC drafted the manuscript. YW carried out the experiment design and carried out the biofilm attached cultivation of C. pyrenoidosa in undiluted swine wastewater. TL performed the statistical analysis and helped to draft and revise the manuscript. All authors read and approved the final manuscript for publication.
Conflict of Interest Statement
The authors declare that the research was conducted in the absence of any commercial or financial relationships that could be construed as a potential conflict of interest.
Acknowledgments
This work was supported by the National Natural Science Foundation of China (31560724), China Postdoctoral Science Foundation (2017T100583, 2016M600616), Natural Science and Technology Major Special Program of China (2014ZX07104-005-02), and Collaborative Innovation Center for Major Ecological Security Issues of Jiangxi Province and Monitoring Implementation (JXS-EW-00).
References
Abdelaziz, A. E. M., Leite, G. B., and Hallenbeck, P. C. (2013). Addressing the challenges for sustainable production of algal biofuels: I. Algal strains and nutrient supply. Environ. Technol. 34, 1783–1805. doi: 10.1080/09593330.2013.827748
Ayre, J. M., Moheimani, N. R., and Borowitzka, M. A. (2017). Growth of microalgae on undiluted anaerobic digestate of piggery effluent with high ammonium concentrations. Algal Res. 24, 218–226. doi: 10.1016/j.algal.2017.03.023
Beal, C. M., Hebner, R. E., Webber, M. E., Ruoff, R. S., Seibert, A. F., and King, C. W. (2012). Comprehensive evaluation of algae production: experimental and target results. Energies 5, 1943–1981. doi: 10.3390/en5061943
Berner, F., Heimann, K., and Sheehan, M. (2015). Microalgal biofilms for biomass production. J. Appl. Phycol. 27, 1793–1804. doi: 10.1007/s10811-014-0489-x
Beuckels, A., Smolders, E., and Muylaert, K. (2015). Nitrogen availability influences phosphorus removal in microalgae-based wastewater treatment. Water Res. 77, 98–106. doi: 10.1016/j.watres.2015.03.018
Bligh, E. G., and Dyer, W. J. (1959). A rapid method of total lipid extraction and purification. Can. J. Biochem. Physiol. 37, 911–917. doi: 10.1139/o59-099
Borowitzka, M. A., and Moheimani, N. R. (2013). Sustainable biofuels from algae. Mitig. Adapt. Strateg. Glob. Change 18, 13–25. doi: 10.1007/s11027-010-9271-9
Cai, T., Park, S. Y., and Li, Y. (2013). Nutrient recovery from wastewater streams by microalgae: status and prospects. Renew. Sust. Energy Rev. 19, 360–369. doi: 10.1016/j.rser.2012.11.030
Carrilho, E. N. V. M., and Gilbert, T. R. (2000). Assessing metal sorption on the marine alga Pilayellalittoralis. J. Environ. Monitor. 2, 410–415. doi: 10.1039/B004128I
Chen, L., Liu, T. Z., Zhang, W., Chen, X. L., and Wang, J. F. (2012). Biodiesel production from algae oil high in free fatty acids by two-step catalytic conversion. Bioresour. Technol. 111, 208–214. doi: 10.1016/j.biortech.2012.02.033
Cheng, P., Ji, B., Gao, L., Zhang, W., Wang, J., and Liu, T. (2013). The growth, lipid and hydrocarbon production of Botryococcus braunii with attached cultivation. Bioresour. Technol. 138, 95–100. doi: 10.1016/j.biortech.2013.03.150
Cheng, P., Wang, J., and Liu, T. (2014). Effects of nitrogen source and nitrogen supply model on the growth and hydrocarbon accumulation of immobilized biofilm cultivation of B. braunii. Bioresour. Technol. 166, 527–533. doi: 10.1016/j.biortech.2014.05.045
Chiu, S. Y., Kao, C. Y., Chen, T. Y., Chang, Y. B., Kuo, C. M., and Lin, C. S. (2015). Cultivation of microalgal Chlorella for biomass and lipid production using wastewater as nutrient resource wastewater as nutrient resource. Bioresour. Technol. 184, 179–189. doi: 10.1016/j.biortech.2014.11.080
Christenson, L., and Sims, R. (2011). Production and harvesting of microalgae for wastewater treatment, biofuels, and bioproducts. Biotechnol. Adv. 29, 686–702. doi: 10.1016/j.biotechadv.2011.05.015
Eaton, A. D., Clesceri, L. S., and Greenberg, A. E. (2005). Standard Methods for the Examination of Water and Wastewater. Washington, DC: American Public Health Association/American Water Works Association/Water Environment Federation.
Feng, P., Deng, Z., Hu, Z., and Fan, L. (2012). Lipid accumulation and growth of Chlorella zofingiensis in flat plate photobioreactors outdoors. Bioresour. Technol. 102, 10577–10584. doi: 10.1016/j.biortech.2011.08.109
Flynn, J. (1991). Algal carbon–nitrogen metabolism: a biochemical basis for modelling the interactions between nitrate and ammonium uptake. J. Plankton Res. 13, 373–387. doi: 10.1093/plankt/13.2.373
Goharshadi, E. K., and Moghaddam, M. B. (2015). Adsorption of hexavalent chromium ions from aqueous solution by graphene nanosheets: kinetic and thermodynamic studies. Int. J. Environ. Sci. Technol. 12, 2153–2160. doi: 10.1007/s13762-014-0748-z
Huang, G. H., Chen, F., Wei, D., Zhang, X. W., and Chen, G. (2010). Biodiesel production by microalgal biotechnology. Appl. Energy 87, 38–46. doi: 10.1016/j.apenergy.2009.06.016
Huo, S., Wang, Z., Zhu, S., Zhou, W., Dong, R., and Yuan, Z. (2012). Cultivation of Chlorella zofingiensis in bench-scale outdoor ponds by regulation of pH using dairy wastewater in winter, South China. Bioresour. Technol. 121, 76–82. doi: 10.1016/j.biortech.2012.07.012
Ji, M. K., Kim, H. C., Sapireddy, V. R., Yun, H. S., Abou-Shanab, R. A. I., Choi, J., et al. (2013). Simultaneous nutrient removal and lipid production from pretreated piggery wastewater by Chlorella vulgaris YSW-04. Appl. Microbiol. Biotechnol. 97, 2701–2710. doi: 10.1007/s00253-012-4097-x
Kothari, R., Pathak, V. V., Kumar, V., and Singh, D. P. (2012). Experimental study for growth potential of unicellular alga Chlorella pyrenoidosa on dairy waste water: an integrated approach for treatment and biofuel production. Bioresour. Technol. 116, 466–470. doi: 10.1016/j.biortech.2012.03.121
Lee, K., and Lee, C. G. (2002). Nitrogen removal from wastewaters by microalgae without consuming organic carbon sources. J. Microbiol. Biotechnol. 12, 979–985.
Lee, J. C., and Pandey, B. D. (2012). Bio-processing of solid wastes and secondary resources for metal extraction-a review. Waste Manag. 32, 3–18. doi: 10.1016/j.wasman.2011.08.010
Li, X., Guo, J., Dong, R., Ahring, B. K., and Zhang, W. (2016). Properties of plant nutrient: comparison of two nutrient recovery techniques using liquid fraction of digestate from anaerobic digester treating pig manure. Sci. Total Environ. 544, 774–781. doi: 10.1016/j.scitotenv.2015.11.172
Li, Y., Chen, Y., Chen, P., Min, M., Zhou, W., Martinez, B., et al. (2011). Characterization of a microalga Chlorella sp. well adapted to highly concentrated municipal wastewater for nutrient removal and biodiesel production. Bioresour. Technol. 102, 5138–5144. doi: 10.1016/j.biortech.2011.01.091
Liu, T., Wang, J., Hu, Q., Cheng, P., Ji, B., Liu, J., et al. (2013). Attached cultivation technology of microalgae for cost-affordable biomass feedstock production. Bioresour. Technol. 127, 216–222. doi: 10.1016/j.biortech.2012.09.100
Markou, G., Chatzipavlidis, I., and Georgakakis, D. (2012). Cultivation of Arthrospira (Spirulina) platensis in olive-oil mill wastewater treated with sodium hypochlorite. Bioresour. Technol. 112, 234–241. doi: 10.1016/j.biortech.2012.02.098
Mata, T. M., Martins, A. A., and Caetano, N. S. (2010). Microalgae for biodiesel production and other applications: a review. Renew. Sust. Energy Rev. 14, 217–232. doi: 10.1016/j.rser.2009.07.020
Min, M., Wang, L., Li, Y., Mohr, M. J., Hu, B., Zhou, W., et al. (2011). Cultivating Chlorella sp. in a pilot-scale photobioreactor using centrate wastewater for microalgae biomass production and wastewater nutrient removal. Appl. Biochem. Biotechnol. 165, 123–137. doi: 10.1007/s12010-011-9238-7
Miranda, A. F., Ramkumar, N., Andriotis, C., Holtkemeier, T., Yasmin, A., Rochfort, S., et al. (2017). Applications of microalgal biofilms for wastewater treatment and bioenergy production. Biotechnol. Biofuels 10, 120–143. doi: 10.1186/s13068-017-0798-9
Mulbry, W., Kondrad, S., Pizarro, C., and Kebede-Westhead, E. (2008). Treatment of dairy manure effluent using freshwater algae: algal productivity and recovery of manure nutrients using pilot-scale algal turf scrubbers. Bioresour. Technol. 99, 8137–8142. doi: 10.1016/j.biortech.2008.03.073
Ozkan, A., Kinney, K., Katz, L., and Berberoglu, H. (2012). Reduction of water and energy requirement of algae cultivation using an algae biofilm photobioreactor. Bioresour. Technol. 114, 542–548. doi: 10.1016/j.biortech.2012.03.055
Petkov, G., and Garcia, G. (2007). Which are fatty acids of the green alga Chlorella. Biochem. Syst. Ecol. 35, 281–285. doi: 10.1016/j.bse.2006.10.017
Pohl, P., and Schimmack, W. (2006). Adsorption of radionuclides (134Cs, 85Sr, 226Ra, 241Am) by extracted biomasses of cyanobacteria (Nostoc Carneum, N. Insulare, Oscillatoria Geminata and Spirulina Laxis-sima) and phaeophyceae (Laminaria digitata and L. Japonica; waste products from alginate production) at different pH. J. Appl. Phycol. 18, 135–143. doi: 10.1007/s10811-006-9084-0
Rodolfi, L., Zittelli, G., Bassi, N., Padovani, G., Biondi, N., Bonini, G., et al. (2009). Microalgae for oil: strain selection, induction of lipid synthesis and outdoor mass cultivation in a low-cost photobioreactor. Biotechnol. Bioeng. 102, 100–112. doi: 10.1002/bit.22033
Sacristán de Alva, M., Luna-Pabello, V. M., Cadena, E., and Ortíz, E. (2013). Green microalga Scenedesmus acutus grown on municipal wastewater to couple nutrient removal with lipid accumulation for biodiesel production. Bioresour. Technol. 146, 744–748. doi: 10.1016/j.biortech.2013.07.061
Schiewer, S., and Volesky, B. (2000). “Biosorption by marine algae” in Bioremediation, ed J. Valdes (Berlin: Springer Netherlands), 139–169.
Shah, M. M. R., Liang, Y. M., Cheng, J. J., and Daroch, M. (2016). Astaxanthin-producing green microalga Haematococcus pluvialis: from single cell to high value commercial products. Front. Plant Sci. 7:531. doi: 10.3389/fpls.2016.00531
Sturm, B. S. M., and Lamer, S. L. (2011). An energy evaluation of coupling nutrient removal from wastewater with algal biomass production. Appl. Energy 88, 3499–3506. doi: 10.1016/j.apenergy.2010.12.056
Tam, N. F. Y., and Wong, Y. S. (1996). Effect of ammonia concentrations on growth of Chlorella vulgaris and nitrogen removal from media. Bioresour. Technol. 57, 45–50. doi: 10.1016/0960-8524(96)00045-4
Wang, J. F., Liu, J. L., and Liu, T. Z. (2015). The difference in effective light penetration may explain the superiority in photosynthetic efficiency of attached cultivation over the conventional open pond for microalgae. Biotechnol. Biofuels 8, 1–17. doi: 10.1186/s13068-015-0240-0
Wang, L., Li, Y., Chen, P., Min, M., Chen, Y., Zhu, J., et al. (2010). Anaerobic digested dairy manure as a nutrient supplement for cultivation of oil-rich green microalgae Chlorella sp. Bioresour. Technol. 101, 2623–2628. doi: 10.1016/j.biortech.2009.10.062
Wang, T., Ge, H. Y., Liu, T. T., Tian, X. W., Wang, Z. J., Guo, M. J., et al. (2016). Salt stress induced lipid accumulation in heterotrophic culture cells of Chlorella protothecoides: mechanisms based on the multi-level analysis of oxidative response, key enzyme activity and biochemical alteration. J. Biotechnol. 228, 18–27. doi: 10.1016/j.jbiotec.2016.04.025
Xu, J., Zhao, Y. J., Zhao, G. H., and Zhang, H. (2015). Nutrient removal and biogas upgrading by integrating freshwater algae cultivation with piggery anaerobic digestate liquid treatment. Appl. Microbiol. Biotechnol. 99, 6493–6501. doi: 10.1007/s00253-015-6537-x
Yao, N., Liu, Z., Chen, Y., Zhou, Y., and Xie, B. (2015). A novel thermal sensor for the sensitive measurement of chemical oxygen demand. Sensors 15, 20501–20510. doi: 10.3390/s150820501
Zeraatkar, A. K., Ahmadzadeh, H., Talebi, A. F., Moheimani, N. R., and McHenry, M. K. (2016). Potential use of algae for heavy metal bioremediation, a critical review. J. Environ. Manage. 181, 817–831. doi: 10.1016/j.jenvman.2016.06.059
Zhou, W., Min, M., Li, Y., Hu, B., Ma, X., Cheng, Y., et al. (2012). A hetero-photoautotrophic two-stage cultivation process to improve wastewater nutrient removal and enhance algal lipid accumulation. Bioresour. Technol. 110, 448–455. doi: 10.1016/j.biortech.2012.01.063
Keywords: Chlorella pyrenoidosa, swine wastewater, biofilm attached cultivation, heavy metals, lipid
Citation: Cheng P, Wang Y, Liu T and Liu D (2017) Biofilm Attached Cultivation of Chlorella pyrenoidosa Is a Developed System for Swine Wastewater Treatment and Lipid Production. Front. Plant Sci. 8:1594. doi: 10.3389/fpls.2017.01594
Received: 16 June 2017; Accepted: 30 August 2017;
Published: 21 September 2017.
Edited by:
Yuval Kaye, Carnegie Institution for Science (CIS), United StatesReviewed by:
Abdulsamie Hanano, Atomic Energy Commission of Syria, SyriaJian Li Yang, Zhejiang University, China
Copyright © 2017 Cheng, Wang, Liu and Liu. This is an open-access article distributed under the terms of the Creative Commons Attribution License (CC BY). The use, distribution or reproduction in other forums is permitted, provided the original author(s) or licensor are credited and that the original publication in this journal is cited, in accordance with accepted academic practice. No use, distribution or reproduction is permitted which does not comply with these terms.
*Correspondence: Defu Liu, ZGZsaXVAMTg5LmNu
†These authors have contributed equally to this work.