- School of Sports Medicine and Rehabilitation, Beijing Sport University, Beijing, China
Sarcopenia is a chronic degenerative disease affecting primarily older adults. A growing aging population is gradually increasing the number of patients suffering from sarcopenia, placing increasing financial pressure on patients’ families and society in general. There is a strong link between mitochondrial dysfunction and sarcopenia pathogenesis. As a result, treating sarcopenia by improving mitochondrial dysfunction is an effective strategy. Numerous studies have demonstrated that exercise has a positive effect on mitochondrial dysfunction when treating sarcopenia. Exercise promotes mitochondrial biogenesis and mitochondrial fusion/division to add new mitochondria or improve dysfunctional mitochondria while maintaining mitochondrial calcium homeostasis, mitochondrial antioxidant defense system, and mitochondrial autophagy to promote normal mitochondrial function. Furthermore, exercise can reduce mitochondrial damage caused by aging by inhibiting mitochondrial oxidative stress, mitochondrial DNA damage, and mitochondrial apoptosis. Exercise effectiveness depends on several factors, including exercise duration, exercise intensity, and exercise form. Therefore, Moderate-intensity exercise over 4 weeks potentially mitigates sarcopenia in older adults by ameliorating mitochondrial dysfunction. HIIT has demonstrated potential as a viable approach to addressing sarcopenia in aged rats. However, further investigation is required to validate its efficacy in treating sarcopenia in older adults.
Introduction
Sarcopenia is characterized by a progressive decline in skeletal muscle strength, mass, and function. It is also a progressive and generalized degenerative disease of aging (Cruz-Jentoft and Sayer, 2019). Sarcopenia is characterized by symptoms such as weakness, loss of muscle strength, and loss of muscle mass, which are affected by several factors, including age, gender, physical activity, and chronic illness (Wu et al., 2022). Sarcopenia may worsen symptoms of chronic diseases such as chronic obstructive pulmonary disease, chronic heart failure, diabetes, and osteoporosis. In addition, it can cause motor dysfunction in these patients, increasing the risk of falls, fractures, and even the loss of independence. Global aging and sedentary lifestyles have led to an increased prevalence of sarcopenia (de Rezende et al., 2014; Chen Z. et al., 2021). A meta-analysis found that 10%–27% of people globally suffer from the condition (Petermann-Rocha et al., 2022). Therefore, sarcopenia has become a serious health concern. Moreover, Sarcopenia places a tremendous financial burden on the patient’s family and society as a whole. The current systematic review indicates that exercise can significantly improve muscle strength and physical performance in older adults with sarcopenia, but does not alter muscle mass (Escriche-Escuder et al., 2021; Wang H. et al., 2022). This may be due to the close connection between muscle strength and physical performance (Kim YH. et al., 2016). Resistance exercise, aerobic exercise, and mixed exercise are common exercise forms for treating sarcopenia (Chen N. et al., 2021; Talar et al., 2021; Huang et al., 2022; Shen et al., 2023). However, resistance and mixed exercise are generally recognized as the most effective intervention for increasing muscle mass (Lu et al., 2021; Shen et al., 2023). Additionally, HIIT, blood flow restriction training, and vibration exercise may be effective strategies for improving muscle strength and physical performance in older adults with sarcopenia (Wu et al., 2020; Hayes et al., 2021; Lu et al., 2021; Alizadeh Pahlavani, 2022; Liu et al., 2022). A large-scale clinical trial will be required in the future to verify the efficacy of HIIT, blood flow restriction training, and vibration training.
Besides improving muscle mass, strength, and physical performance, exercise for sarcopenia causes adaptive changes in mitochondrial function as well. Mitochondria are the most critical organelles for energy production, metabolism of free radicals, and programmed apoptosis of cells. The mitochondrial quality control system protects mitochondrial quantity and quality through the regulation of mitochondrial biosynthesis, mitochondrial dynamic homeostasis, and mitochondrial autophagy (Stotland and Gottlieb, 2015). In this way, mitochondrial dysfunction caused by aging can be alleviated. Therefore, previous studies provided preliminary evidence that dysfunctional mitochondrial quality control may contribute to muscle atrophy and physical disability associated with aging (Joseph et al., 2012). Moreover, increased reactive oxygen species (ROS) in older patients with sarcopenia can disrupt the balance between oxidative stress and antioxidant defenses in mitochondria. As a result, mitochondrial apoptosis and damage to mitochondrial DNA (mtDNA) are increased, mitochondrial calcium (Ca2+) homeostasis is disrupted, and mitochondrial fusion/fission and mitochondrial biogenesis are inhibited (Chabi et al., 2008; Andersson et al., 2011; Alway et al., 2017). Therefore, mitochondrial dysfunction not only results from aging but also plays a crucial role in the pathogenesis of Sarcopenia. However, no studies have confirmed that pharmacological therapies can restore mitochondrial function in aging skeletal muscles. Exercise improves mitochondrial dysfunction and prevents progressive loss of muscle function and mass with age (Carter et al., 2015). Therefore, exercise is an effective strategy for improving muscle function and quality of life in older adults by stimulating mitochondrial adaptation. Nevertheless, it is unclear what form, frequency, and duration of exercise can be prescribed for the treatment of sarcopenia. The purpose of this paper is to review the research literature on exercise for improving mitochondrial dysfunction in the treatment of sarcopenia in recent years and summarize the mechanisms by which exercise regulates sarcopenia through mitochondria, to provide a theoretical foundation and references for studies regarding optimal exercise recommendations for alleviating sarcopenia.
Diagnosis of sarcopenia
The concept of sarcopenia is constantly evolving (Dent et al., 2021). Sarcopenia was initially understood to be a decrease in muscle strength (Langer et al., 2019). As clinical medicine has developed, the European Working Group on Sarcopenia in Old People (EWGSOP) introduced a contemporary definition of sarcopenia in 2010, which includes low muscle mass, low muscle strength, and/or low physical performance (Cruz-Jentoft et al., 2010). Currently, there is no gold standard for diagnosing sarcopenia. However, sarcopenia can also be diagnosed by low muscle mass, low muscle strength, and low physical performance. Recently, EWGSOP (Cruz-Jentoft et al., 2019), the Asian Working Group for Sarcopenia (AWGS) (Chen et al., 2020), and the Sarcopenia Definition and Outputs Consortium (SDOC) (Bhasin et al., 2020) have updated the diagnostic consensus for sarcopenia (Table 1). These consensus guidelines differ in their diagnostic criteria for sarcopenia. EWGSOP and AWGS evaluation content are similar, but critical values differ. The two consensuses may target different ethnic and geographical groups (Woo et al., 2014). The SDOC excludes muscle mass from sarcopenia diagnostic criteria, perhaps due to its inability to accurately predict adverse health outcomes (Bhasin et al., 2020). Consequently, it will be necessary to develop a universally applicable diagnostic method for sarcopenia in the future.
Exercise ameliorates sarcopenia by increasing mitochondrial biogenesis
Mitochondrial biogenesis is the process of intracellular production of newly formed and functioning normal mitochondria (Johnson et al., 2021). Some proteins play a significant role in mitochondrial biogenesis, but their expression decreases with age (Carter et al., 2018). PGC-1α plays a major role in regulating mitochondrial biogenesis in muscle cells and is positively associated with mitochondrial biogenesis. Numerous studies have confirmed that PGC-1α is regulated by AMP-activated protein kinase (AMPK), silence information regulator 1 (SIRT1), and p53 (Zong et al., 2002; Saleem et al., 2009; Menzies et al., 2013). Under stress conditions such as exercise, PGC-1α controls the expression of multiple transcription factors (nuclear respiratory factors-1 (NRF-1) and nuclear respiratory factors-1 (NRF-2) and the expression of mitochondrial transcription factor A (TFAM) to produce newly formed mitochondria (Scarpulla et al., 2012; Granata et al., 2018). In addition, the expression of PGC-1α in older muscles is decreased as a result of a decrease in upstream signaling (Ljubicic and Hood, 2009; Carter et al., 2018). Therefore, PGC-1α expression cannot be ignored when researching the effects of exercise on mitochondrial biogenesis in sarcopenic patients. But there are also studies suggesting that PGC-1α、P53 is not necessary for adaptive response to exercise training (Rowe et al., 2012; Beyfuss et al., 2018). Even older skeletal muscles without PGC-1α and P53 can adapt to exercise to maintain normal metabolic functions (Table 2).
Exercise form plays a critical role in mitochondrial biogenesis (Table 2). Kitaoka found that a 4-week resistance exercise had little effect on mitochondrial biogenesis in a somatic animal model (Kitaoka et al., 2015). Further, Mesquita investigated the histopathology of the lateral femoral muscle in older adult subjects and concluded that 10-week resistance exercise did not affect mitochondrial biogenesis as well (Mesquita et al., 2020). Therefore, resistance exercise does not affect mitochondrial biogenesis. However, 6 weeks of endurance exercise can reverse the decline in SIRT1, AMPK, and PGC-1α in aged rats, and diminish the gap in mitochondrial biogenesis-related proteins between aged and young rats (Koltai et al., 2012). Aged rats undergoing endurance exercise for 12 weeks exhibited significantly higher levels of PGC-1α, TFAM, SIRT1, and AMPK than other sedentary rats (Kang et al., 2013). Therefore, upregulation of PGC-1α signaling may be responsible for promoting mitochondrial biogenesis in aged rat skeletal muscle after endurance training. This conclusion has also been confirmed by human experiments. Endurance exercise can increase mitochondrial biogenesis by increasing the expression of mitochondrial biogenesis-related proteins (e.g., PGC-1α, TFAM, NRF-1), as well as activating the AMPK/PGC-1Α signaling pathway (Moore et al., 2019; Gao et al., 2021). Therefore, endurance training promotes mitochondrial biogenesis and is correlated with the duration of endurance training. However, research has shown that HIIT promotes mitochondrial biogenesis more effectively than moderate-intensity continuous training (MICT) (Han et al., 2022; Pirani et al., 2023). Therefore, HIIT has demonstrated superior efficacy in augmenting mitochondrial biogenesis in aged rats with sarcopenia.
Exercise increases PGC-1α expression in older adults, pointing to the possibility that older muscles can respond to exercise efficiently to increase mitochondrial biogenesis (Carter et al., 2018). In addition, it was confirmed that PGC-1α and p53 protein levels increased with training volumes, and a short-term decrease in training volumes decreased the levels of both proteins, indicating that training volume remains a factor influencing training-induced mitochondrial biogenesis (Granata et al., 2018). Nonetheless, the upregulation of PGC-1α does not exhibit a linear correlation with escalating training volumes. Edgett’s experimental findings indicate that HIIT with 100% peak aerobic power is the most effective in promoting PGC-1α expression following a single exercise session of varying intensities (Edgett et al., 2013). While the 73% and 133% conditions have comparable effects on mitochondrial biogenesis, both fall slightly short of the biogenesis observed under 100% conditions (Edgett et al., 2013). Furthermore, the activation of PGC-1α transcription by AMPK may not be influenced by exercise intensity and may not be augmented by ultra-high-intensity exercise (Edgett et al., 2013). Moreover, it has been observed that as the level of physical exertion escalates, the manifestation of PGC-1α is inferior to that of Electromyography derived muscle activation, potentially leading to a dampening of PGC-1α signaling mediated activation. Consequently, it remains uncertain whether a single bout of supramaximal intensity training can confer exercise advantages that surpass those attained through submaximal and maximal intensity exercises. Conversely, Granada has demonstrated that the intensity-dependent regulation of PGC-1α protein may persist beyond the pinnacle of power after prolonged durations and intensities of exercise (Granata et al., 2016). The inconsistent timing of muscle biopsy, exercise duration, and exercise frequency in the two experiments may have contributed to the observed possibility. However, Granata confirmed this conclusion with guaranteed consistent muscle biopsy timing (Granata et al., 2020). These findings underscore the significance of exercise intensity in mitochondrial biogenesis. While optimal exercise intensity remains a topic of debate, both endurance training and HIIT have been shown to promote mitochondrial biogenesis. For older adults, endurance training is recommended based on their physical condition.
Exercise ameliorates sarcopenia by balancing mitochondrial dynamics
Mitochondrial dynamics include mitochondrial fusion and fission. Mitochondrial autophagy, aging, and decreased physical activity promote mitochondrial fragmentation, which leads to mitochondrial dysfunction (Wai and Langer, 2016). Mitochondrial fission isolates dysfunctional mitochondria, improving mitochondrial function (Wai and Langer, 2016). Additionally, mitochondrial fusion plays a significant role in maintaining mitochondrial function. For example, mitochondrial fusion regulates oxidative phosphorylation (OXPHOS) and mitochondrial DNA replication (Silva Ramos et al., 2019). Mitochondrial fusion processes include mitofusin 1 (Mfn1) and mitofusin 2 (Mfn2) mediated mitochondrial outer membrane fusion and optical atrophy protein 1 (Opa1) mediated mitochondrial inner membrane fusion (Bell et al., 2019). Mahmoodzadeh (Mahmoodzadeh et al., 2021) also confirmed that Ahnak1 is likely located in the outer mitochondrial membrane and plays an important role in mitochondrial fusion. The mitochondrial division is mediated by mitochondrial fusion protein 1 (FIS1), dynamin-related protein 1 (Drp1), and mitochondrial fission factor (MFF) (Liu et al., 2021). In contrast, to direct mitochondrial fission, there has been evidence that FIS1 inhibits mitochondrial fusion (Yu et al., 2019). The downregulation of Mfn2, FIS1, Drp1, and Opa1 in sarcopenia in old people can be associated with a significant decline in exercise performance (Liu et al., 2021). This suggests that aging and exercise can alter the dynamic balance of mitochondria. Therefore, the mitochondrial dynamic imbalance is associated with sarcopenia and skeletal muscle atrophy.
Observations of mitochondria in skeletal muscles after exercise indicate that they exist in a more fused state (Mishra et al., 2015). But acute exercise does not alter mitochondrial fusion proteins expression. For example, Picard found that after a voluntary round run of 3 hours on mice, mitochondrial morphology and Mfn2 and Opa1 abundance did not change (Picard et al., 2013). Acute resistance exercise in rats has also verified this conclusion (Kitaoka et al., 2015). Moreover, Marshall demonstrated in human experiments that acute resistance exercise may not alter mitochondrial function in an older adult with muscle disuse (Marshall et al., 2022). However, research has shown that prolonged exercise training may result in mitochondrial fusion. Resistance training for 4 weeks increased the levels of Mfn1, Mfn2, and Opa1 proteins in the gastrocnemius muscles of rats (Kitaoka et al., 2015). Nevertheless, this only increased mitochondrial bulk density, regardless of mitochondrial content. Furthermore, resistance training for 10 weeks can also increase the levels of Mfn1, Mfn2, and Opa1 proteins in older people (Mesquita et al., 2020). Aerobic exercise for 20 weeks can increase the levels of OPA1 and MFF in the gastrocnemius muscle of healthy adult males (Zoladz et al., 2022). The use of HIIT and MICT for 12 weeks has been shown to reduce Drp1 and FIS1 levels and to increase the levels of Mfn1, Mfn2, and Opa1 to promote mitochondrial fusion (Li B. et al., 2019). However, Wyckelsma believes that HIIT is more effective (Wyckelsma et al., 2017). As a result of the correlation between exercise intensity and Mfn2 expression (Fiorenza et al., 2018). While the majority of studies have been conducted on healthy adults, the number of mitochondria in the muscles of young and healthy older adults is similar (Wyckelsma et al., 2017). Therefore, chronic exercise for 4 weeks or more reduces sarcopenia by activating mitochondrial fusion.
FIS1 and Drp1 protein levels in the mitochondria of the gastrocnemius muscle did not change within 1 h following acute resistance exercise (Kitaoka et al., 2015). However, Moore developed a mouse model (mDrp1) which maintained high levels of FIS1 and Opa1 for 3 h following the cessation of endurance training (Moore et al., 2019). These results demonstrate that exercise form and Drp1 signal transduction play a significant role in regulating exercise performance and adapting to endurance training. In contrast, acute sprint interval training of different intensities can increase DRP1 levels in the lateral femoral muscle (Granata et al., 2017). DRP1 expression is influenced by the impact of high-intensity exercise on β-adrenergic stimulation of adrenaline (Fiorenza et al., 2018). Moreover, Ding found that the levels of FIS1 mRNA and protein increased significantly after 120–150 min of endurance training (Ding et al., 2010). The rapid expression of the FIS1 gene in skeletal muscle may be a result of increased metabolic demands during exercise. This may have a significant impact on the efficiency of oxidative phosphorylation. Therefore, exercise can improve mitochondrial dynamics in older adults with sarcopenia. Long-term, moderately intense, or highly intense exercise can reduce sarcopenia and muscle atrophy in older adults.
Exercise ameliorates sarcopenia by inducing mitochondrial autophagy
The mitochondria within the cell undergo depolarization due to external stimuli such as ROS, a lack of nutrients, and the aging of the cells. Mitochondrial autophagy mechanisms are usually divided into two categories: ubiquitin-dependent pathways and non-ubiquitin-dependent pathways (Lu et al., 2023). The ubiquitin-dependent pathway refers to the widespread ubiquitination of mitochondrial surface proteins that promote mitochondrial autophagy. The PTEN-induced oxidative kinase protein 1 (PINK1)/Parkin pathway is currently the most extensively studied pathway (Guan et al., 2018). When mitochondrial membrane potential (MMP, ΔΨ m) When damaged, the pathway of PINK1 entering the inner mitochondrial membrane is blocked, leading to the stable aggregation of PINK1 on the cytoplasmic surface of the outer membrane of mitochondria. In parallel, this will recruit and activate Parkin, and the spatial conformation of Parkin protease will change into activated E3 ubiquitin ligase, which will ubiquitinate proteins in mitochondria (Riley et al., 2013). In addition, PINK1 directs the recruitment of autophagic receptor proteins (such as Nip3-like protein X (NIX), BCL2-interacting protein 3(BNIP3), and FUN14 domain containing 1(FUNDC1)) to mitochondria through ubiquitin phosphorylation (Lu et al., 2023). The receptor proteins recruit LC3, which enables autophagosomes to engulf mitochondria. Moreover, the expression of LC3, BNIP3, beclin1, Atg7, p62, and Parkin decreases with age (Liang et al., 2020). Therefore, autophagy levels are low in the skeletal muscles of older animals. Under stress factors, such as exercise, AMPK promotes autophagy by promoting mitochondrial division (Zhang and Lin, 2016).
A cross-sectional study has shown that older adults who exercise have higher levels of mitochondrial phagocytosis proteins Parkin and BNIP3 (Dethlefsen et al., 2018; Balan et al., 2019). Therefore, an active lifestyle contributes to mitochondrial autophagy. Nevertheless, mitochondrial autophagy varies according to the age at which exercise is initiated. As compared with mice aged 18 months, mice aged 8 months had increased mitochondrial autophagy activity, improving their physical function (Gao et al., 2020). Therefore, older adults should begin training as early as possible and maintain their exercise habits to effectively prevent sarcopenia. Acute exercise such as downhill running acutely, swimming, or running on a treadmill can promote the expression of mitochondrial autophagy-related proteins (Kim et al., 2012; Lenhare et al., 2017; Shang et al., 2019). Moreover, chronic exercises such as endurance training, downhill/uphill training, and resistance training increased autophagic activity in the gastrocnemius muscle of aged rats (Kim et al., 2013; Kim JS. et al., 2016; Zeng et al., 2020). Therefore, exercise duration, exercise form, and exercise intensity are associated with differences in autophagy marker expression. Meta-analyses have shown that long-term aerobic and resistance exercise may increase the expression of autophagy-related proteins in aging skeletal muscles (Wang C. et al., 2022). However, a prolonged period of high-intensity exercise may lead to excessive autophagy, while a short period of low-intensity exercise (intervention duration< 12 weeks, frequency< 3 times/week) may not reach the level of exercise-induced autophagy that is required for metabolic adaptation. This conclusion was also supported by Yu’s comparative experiment (Yu et al., 2020). Furthermore, Yu found that exercise can increase the LC3-II/LC3-I ratio as well as decrease the expression of the p62 protein (Yu et al., 2020). But under the same exercise intensity, the autophagy activity of skeletal muscle mitochondria after 4 weeks of exercise is higher than that after 2 weeks of exercise (Yu et al., 2020). Under the same exercise time, the mitochondrial autophagy activity of high-intensity exercise was higher than that of moderate-intensity exercise (Yu et al., 2020). Therefore, different exercise durations and intensities have varying effects on autophagy-related protein expression. However, Yu’s experimental time was relatively short, and the results of the meta-analysis may be heterogeneous. Nevertheless, moderate-intensity exercise for more than 4 weeks increases mitochondrial autophagy.
Exercise ameliorates sarcopenia by improving mitochondrial Ca2+ homeostasis
The mitochondrial uptake of Ca2+ is crucial to the regulation of their intrinsic functions. Ca2+ in mitochondria plays a significant role in regulating metabolic activity (Gherardi et al., 2020). The mitochondrial calcium transporter (MCU) is one of the most important Ca2+ transport complexes within mitochondrial Ca2+ uptake channels. A disturbance in mitochondrial Ca2+ may lead to impaired function of the MCU and abnormalities in mitochondrial metabolism and kinetics (Wu et al., 2021). Furthermore, mitochondrial Ca2+ overload can lead to the opening of the mitochondrial membrane permeability transformation pore (mPTP), swelling of mitochondria, and the production of ROS (Strubbe-Rivera et al., 2021). Researchers have reported that fatigue patients and aging mice have reduced mitochondrial Ca2+ uptake and muscle function (Debattisti et al., 2019; Yang et al., 2021). Therefore, mitochondrial Ca2+ uptake imbalances impair muscle fiber contraction, and restoring mitochondrial Ca2+ uptake can improve skeletal muscle function in aging mice (Debattisti et al., 2019; Yang et al., 2021).
Research has demonstrated that regular exercise promotes the repair and maintenance of Ca2+ release units, Ca2+ entry units, and mitochondria during denervation and aging (Protasi et al., 2021). Li compared the effects of HIIT and MICT on Ca2+ conduction in an aged rat model (Li FH. et al., 2019). According to the study, HIIT can increase the expression of some genes, thereby improving Ca2+ signal transduction and systolic function by supporting these proteins. Both HIIT and MICT improved Ca2+ signaling and muscle contractile protein expression in older skeletal muscles, which offset age-related changes in excitatory contraction coupling. Additionally, Zampieri examined mitochondrial Ca2+ homeostasis in response to neuromuscular electrical stimulation and proprioceptive training (Zampieri et al., 2016). Based on 9 weeks of training, both types of training can increase MCU protein levels, thus improving muscle function and structure. Under electron microscopy, ultrastructural analysis has revealed that mitochondrial changes in neuromuscular electrical stimulation training muscles may be mediated by increases in the expression of mitochondrial fusion protein OPA1. Furthermore, mitochondria that lack Drp1 have a larger morphology and are more dysfunctional. Therefore, mitochondrial dynamic balance is essential for maintaining mitochondrial Ca2+ homeostasis (Favaro et al., 2019). Exercise has been shown to maintain mitochondrial Ca2+ homeostasis in older skeletal muscle based on current evidence. Nevertheless, more research is required in the future to determine the optimal exercise form, intensity, and duration.
Exercise ameliorates sarcopenia by inhibiting mitochondrial oxidative stress
Two fundamental biological processes determine the level of oxidative stress in the older adult with sarcopenia: the production of ROS and the decline in antioxidant defenses caused by aging (Ji, 2002). The imbalance between ROS production and antioxidant defense systems results in mitochondrial oxidative stress. Several pathways (e.g., electron transfer chain (ETC.) and aging can promote excessive ROS production, which can cause mitochondrial damage (Selivanov et al., 2011; Brand, 2016; Thoma et al., 2020). A lack of exercise also elevates ROS, which further reduces muscle function and mass (Waltz et al., 2018). It has been demonstrated that increased ROS is associated with fiber atrophy and necrosis in sarcopenia cases (Brioche and Lemoine-Morel, 2016). Therefore, high ROS levels in mitochondria contribute to the pathogenesis of sarcopenia in older adults. The mitochondrial antioxidant defense system includes catalase (CAT) and superoxide dismutase (SOD). A higher level of CAT is effective at reducing ROS production, prolonging lifespan, and reducing fatigue and atrophy in muscles (Selsby, 2011; Xu et al., 2021). Moreover, an imbalance between ROS and antioxidant defenses leads to high levels of oxidative stress that exacerbate ROS-induced sarcopenia (Sullivan-Gunn and Lewandowski, 2013). Therefore, inhibiting mitochondrial oxidative stress is crucial.
Exercise-induced ROS production improves mitochondrial efficiency, while chronic ROS production is detrimental to mitochondria. This may be because exercise can improve the coupling efficiency of, ETCs, reduce electron leakage and excessive mitochondrial ROS production, as well as restoring the balance of mitochondrial oxidative stress in the body to a healthy level (Corsetto et al., 2019). Furthermore, studies have shown that training time has a significant effect on CAT activity. An aerobic exercise can cause a transient peroxidation state in white adipose tissue, increasing the expression of antioxidant defenses (Matta et al., 2021). This may be due to the activation of the redox effector factor-1 (Ref1) and nuclear factor e2-related factor 2 (Nrf2) antioxidant defense pathways, which may prevent cellular oxidative stress resistance during acute exercise (Wang et al., 2016). Furthermore, chronic exercise can improve the antioxidant defense system. An 8-week aerobic and resistance exercise program increased SOD2 and CAT activity in aged rats (Vilela et al., 2018). Furthermore, 8-month HIIT and MICT can upregulate the expression of SOD2 and Acetaldehyde dehydrogenase (ALDH) (Li FH. et al., 2019). Nevertheless, the level of SOD2 in the MICT group did not reach statistical significance. Further, HIIT provides greater benefits to aged rats than MICT in terms of combating chronic low-level inflammation and oxidative stress (Li et al., 2018). A possible explanation for this may be related to the tissue-specific oxidative stress response of HIIT and MICT (Groussard et al., 2019). Therefore, acute aerobic exercise and chronic HIIT improve mitochondrial oxidative stress more effectively.
Exercise ameliorates sarcopenia by inhibiting MtDNA
Mitochondrial DNA replication, deletion, and mutation degrees increase with age, directly affecting cell metabolism and mitochondrial function (Cortopassi and Arnheim, 1990; Harper et al., 2021; Herbst et al., 2021). Additionally, mtDNA mutant mice showed a higher level of mitochondrial fission, autophagy, and mitochondrial oxidative stress (Joseph et al., 2013; Ma et al., 2020). As a result, in older adults with mitochondrial dysfunction, mutated mtDNA may result in reduced oxidative phosphorylation. The effect of this is to affect the assembly of functional, ETC., complexes. However, it does not increase oxidative stress, resulting in skeletal muscle apoptosis and sarcopenia in the long run (Hiona et al., 2010). In muscle fibers containing, ETC., defects, mtDNA deletion mutations increased by 1,200%, muscle fiber numbers decreased by 18%, and muscle mass loss worsened by 22% (Herbst et al., 2016). These results confirm the importance of mitochondrial DNA deletions and mutations in the development of muscle atrophy in older adults. This is in contrast to the reactions observed in normal aging muscles and may provide a means for detecting muscle atrophy at an early stage.
Exercise can regulate mtDNA levels in the skeletal muscles of the older adult. For example, exercise can promote mtDNA replication in older skeletal muscles. Upon reviewing the literature, Erlich found that exercise-induced mitochondrial biogenesis occurred simultaneously with an increase in mtDNA copy number (Erlich et al., 2016). Furthermore, exercise may stimulate the synthesis of mtDNA in human skeletal muscle (Robinson et al., 2011). Acute endurance exercise is capable of increasing P53 and mitochondrial DNA levels (Saleem and Hood, 2013). Therefore, exercise may be an effective mechanism for sarcopenia treatment by activating P53 to promote metabolic function. P53 can also interact with TFAM and DNA polymerase gamma to promote the binding of mtDNA and maintain mtDNA integrity (Achanta et al., 2005; Park et al., 2009; Park et al., 2016). Moreover, exercise can reduce mtDNA mutations in skeletal muscle and normalize protein levels (Maclaine et al., 2020). Safdar found that mtDNA in sedentary and exercised PolG mice contained similar numbers of mutations (Safdar et al., 2016). Nevertheless, sedentary mice suffer from more non-mutational damage, which can be mitigated through exercise. Additionally, the significant relief of mtDNA mutation phenotypes in muscle from exercise may not be due to a reduction in mutation load, but rather to a reduction in damage to mtDNA and/or oxidative stress. Therefore, endurance exercise promotes mtDNA replication and inhibits mtDNA mutations. Nevertheless, further investigation is required to identify the mechanisms of mitochondrial dysfunction and mtDNA dysfunction.
Exercise ameliorates sarcopenia by inhibiting mitochondrial apoptosis
Upon stimulation by internal apoptotic factors, such as oxidative stress, calcium overload, and DNA damage, an imbalance between anti-apoptotic proteins (e.g., B-cell lymphoma-2 (Bcl-2), Bcl-xL) and pro-apoptotic proteins (e.g., Bcl-2-associated X protein (Bax), Bak, Bid) occurs, increasing mitochondrial outer membrane permeability and opening of the mitochondrial permeability transition pore (mPTP) (Lindsay et al., 2011). This process leads to the release of mitochondrial pro-apoptotic factors, including cytochrome C (cyto C), apoptosis-inducing factor (AIF), the second mitochondria-derived activator of caspases/direct inhibitor of apoptosis-binding protein with low pI (SMAC/DIABLO), high-temperature requirement protein A2/omi (HTRA2/OMI), and endonuclease G (ENDOG), into the cytoplasm (Bano and Prehn, 2018). Upon being released into the cell, cyto C engages in an interaction with Apaf-1, resulting in the formation of an apoptotic complex that is facilitated by ATP and dATP (Yadav et al., 2020). This complex serves to recruit and activate Pro-Caspase9, ultimately giving rise to the Caspase9 holoenzyme. The entire Caspase9 enzyme then proceeds to activate Caspase3 and Caspase7, thereby initiating the caspase cascade reaction and ultimately culminating in cell apoptosis (Ola et al., 2011). The activation of Caspase3 and Caspase7 can be inhibited by Apoptosis inhibitory proteins (IAPs), which in turn can impede the process of cell apoptosis (Scott et al., 2005). The release of SMAC/DIABLO and HTRA2/OMI from mitochondria into the cell results in their binding to IAPs, thereby releasing the inhibitory effect of IAPs and indirectly promoting apoptosis (Scott et al., 2005; Vande Walle et al., 2008). Simultaneously, apoptosis can activate the E3 ubiquitin ligase MAFbx/atrogin-1 and Muscle RING finger 1 (MuRF1), leading to increased protein degradation and exacerbating aging-induced skeletal muscle loss (Cheema et al., 2015) (Figure 1). Consequently, the upregulation of pro-apoptotic processes may contribute to age-related muscle mass and cachexia loss.
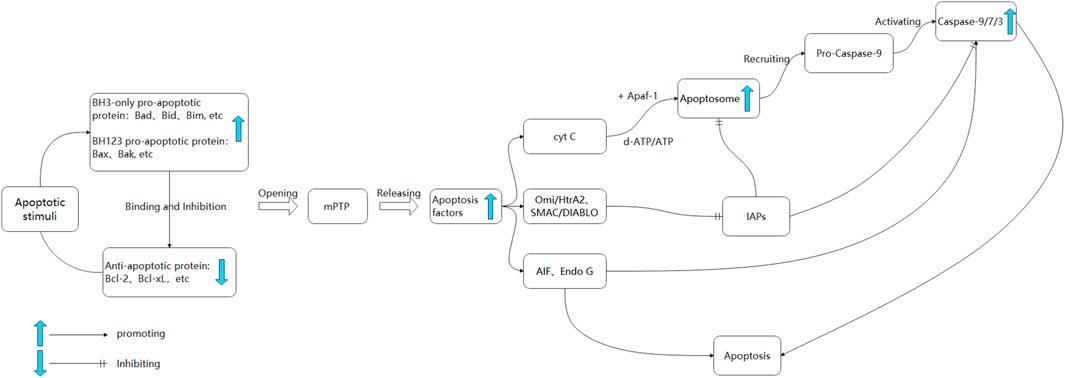
FIGURE 1. The mitochondrial apoptosis pathway. Upon exposure to apoptotic signals, the upregulation of pro-apoptotic proteins occurs, resulting in their binding to anti-apoptotic proteins. This interaction leads to an increase in mitochondrial permeability and the subsequent opening of the mPTP, ultimately resulting in the release of apoptotic factors (Cruz-Jentoft and Sayer, 2019). Cyt C binds to Apaf-1, forming a mitochondrial complex facilitated by ATP/dATP. This complex recruits Caspase 9 proenzyme, forming apoptosomes and initiating downstream Caspase cascade reactions (Wu et al., 2022). SMAC, Omi/HtrA2 can bind to IAPs, inhibiting Caspase 3 and 9 (Chen Z. et al., 2021). AIF and Endo G translocate to the nucleus, causing chromatin condensation and large-scale DNA fragmentation. In the cytoplasm, AIF activates Caspase 3 and subsequently activates Caspase 9.
Exercise training has been shown to reduce metabolic changes caused by mitochondrial apoptosis. As an example, exercise training at 60% VO2 max for 6 weeks can reverse age-related catabolism and apoptosis (Ziaaldini et al., 2015). Additionally, treadmill exercise training for 12 weeks decreased Caspase-3, Bax, and Bax/Bcl-2 ratios, as well as increased anti-apoptotic Bcl-2 in the white gastrocnemius and soleus muscles of aged rats (Song et al., 2006). The activity of nuclear factor kappa B (NF-B) increases with exercise training and decreases with aging (Song et al., 2006). Based on these results, aerobic exercise may be able to reduce fiber atrophy and proapoptotic signal transduction in aging skeletal muscle. Compared to the effects of not exercising during adulthood or starting regular exercise later in life, lifelong exercise in rats increases autophagic activity and prevents skeletal muscle apoptosis (Gao et al., 2021). Additionally, Zeng believes that both aerobic exercise and resistance exercise can inhibit excessive apoptosis caused by the upregulation of Bcl-2 and downregulation of Bax in the skeletal muscles of aged rats (Zeng et al., 2020). However, resistance exercise has a greater impact on anti-apoptosis (Zeng et al., 2020). It may be possible that resistance exercise increases autophagic activity and reduces apoptosis in older adult skeletal muscle by regulating insulin-like growth factor-1 (IGF-1) and its receptors, and downregulating Akt/mTOR and Akt/FOXO3a signaling pathways (Luo et al., 2013). Alternatively, resistance exercise stimulation of autophagy may prevent NOD-like receptor thermal protein domain associated protein 3 (NLRP3) activation and reduce apoptosis in peripheral blood mononuclear cells (PBMCs) of the older adult (Mejías-Peña et al., 2017). To compare the effects of HIIT and resistance exercise, Su conducted a 32-week intervention and found that both showed a decrease in mitochondrial apoptosis (Su et al., 2022). Resistance training has a greater effect on reversing age-related muscle loss than HIIT. In contrast, HIIT is more effective in suppressing proapoptotic factors over the long term (Su et al., 2022). However, Further research is required to validate the potential of HIIT in enhancing sarcopenia among older adults through the inhibition of mitochondrial apoptosis.
Conclusions and perspectives
With aging and inactivity, mitochondrial dysfunction, muscle reduction, or even muscle atrophy occur within the skeletal muscles of older adults and animals. This review analyzes the effects of exercise on mitochondrial biogenesis, mitochondrial dynamics, mitochondrial autophagy, mtDNA, mitochondrial Ca2+ homeostasis, mitochondrial oxidative stress, and mitochondrial apoptosis in aged rats and adults with sarcopenia. This review also sheds light on the interaction and potential mechanisms of mitochondrial dysfunction following exercise (Figure 2). Furthermore, this review summarizes the best exercise forms, intensities, and timings for regulating mitochondrial dysfunction. There is evidence that exercise improves the mitochondrial function of older adults with Sarcopenia. However, exercise form, intensity, and duration are significant factors that affect exercise effectiveness. HIIT has been shown to effectively stimulate mitochondrial biogenesis and dynamics in aged rats, thereby facilitating the generation of new mitochondria or the restoration of dysfunctional ones. Moreover, it has been observed to mitigate oxidative stress and mitochondrial apoptosis in aged rats. Moderate-intensity exercise maintains mitochondrial dynamic balance, promotes mitochondrial autophagy, reduces mitochondrial DNA damage and apoptosis, and eliminates dysfunctional mitochondria from the body. Acute exercise can promote mitochondrial fission and reduce mitochondrial oxidative stress. Chronic exercise is more suitable for improving mitochondrial dysfunction in older sarcopenia patients. Therefore, Moderate-intensity exercise over 4 weeks potentially mitigates sarcopenia in older adults by ameliorating mitochondrial dysfunction. HIIT has demonstrated potential as a viable approach to addressing sarcopenia in aged rats. However, further investigation is required to validate its efficacy in treating sarcopenia in older adults.
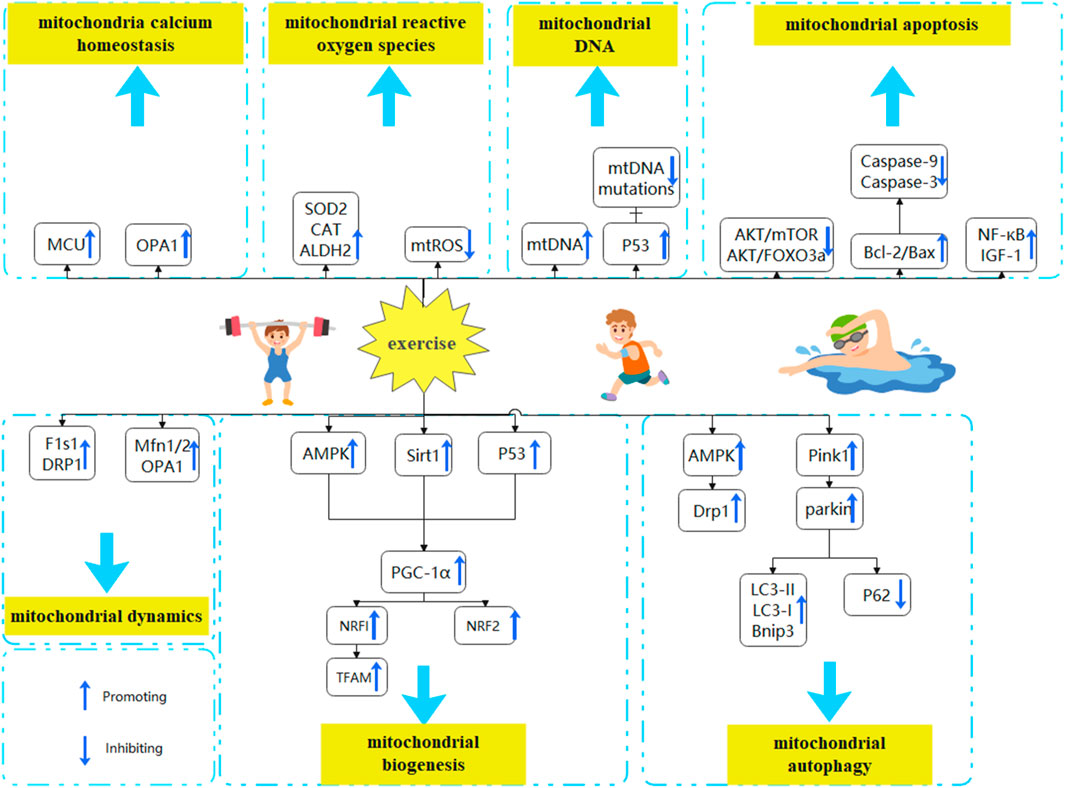
FIGURE 2. Exercise to improve mitochondrial dysfunction in the treatment of sarcopenia. Mitochondrial dysfunction is characterized by alterations in mitochondrial biogenesis, mitochondrial dynamics, mitochondrial autophagy, mtDNA, mitochondrial calcium homeostasis, mitochondrial oxidative stress, and mitochondrial apoptosis. Exercise may be able to reverse the aging process of skeletal muscle by modulating mitochondrial dysfunction (A) Exercise promotes mitochondrial biogenesis. By activating the AMPK, P53, and SIRT1 pathways and promoting PGC-1α expression, exercise reverses aging effects (B)Exercising balances mitochondrial dynamics. By increasing the expression of Mfn1/2, OPA1, DRP1, and FIS1, exercise reverses aging effects (C) Exercise enhances mitochondrial autophagy. By increasing the expression of AMPK and PINK1/Parkin pathways, promoting LC3-II/LC3-I ratio and BNIP3 expression, and downregulating p62 expression, exercise reverses aging effects (D) Exercise maintains mitochondrial Ca2+ homeostasis. By promoting MCU and OPA1 expression and restoring mitochondrial Ca2+ uptake, exercise reverses aging effects (E) Exercise reduces mitochondrial oxidative stress. By inhibiting mitochondrial ROS synthesis and promoting the production of antioxidant defense systems (CAT, SOD2, ALDH2), exercise reverses aging effects (F) Exercise reverses aging effects by promoting mitochondrial DNA synthesis and replication through P53 and inhibiting mtDNA mutations (H) Exercise decreases mitochondrial apoptosis. By downregulating Akt/mTOR and Akt/FOXO3a signaling pathways, and upregulating IGF-1, NF-κB, and Bcl-2/Bax ratios, exercise reverses aging effects.
Author contributions
Conceptualization, YZ and XZ; methodology, AZ; validation, YZ; investigation, SX and JX; resources, ZB; writing—original draft preparation, YZ; writing—review and editing, XZ; funding acquisition, ZB. All authors contributed to the article and approved the submitted version.
Funding
This work was supported by “the Fundamental Research Funds for the Central Universities” (20221021) and a grant from the Beijing High-Grade, Precision, and Advanced Disciplines Project.
Acknowledgments
We want to thank Mogo (http://www.mogoedit.com/) for English language editing.
Conflict of interest
The authors declare that the research was conducted in the absence of any commercial or financial relationships that could be construed as a potential conflict of interest.
Publisher’s note
All claims expressed in this article are solely those of the authors and do not necessarily represent those of their affiliated organizations, or those of the publisher, the editors and the reviewers. Any product that may be evaluated in this article, or claim that may be made by its manufacturer, is not guaranteed or endorsed by the publisher.
References
Achanta, G., Sasaki, R., Feng, L., Carew, J. S., Lu, W., Pelicano, H., et al. (2005). Novel role of p53 in maintaining mitochondrial genetic stability through interaction with DNA Pol gamma. Embo J. 24 (19), 3482–3492. eng. Epub 20050915. Cited in: Pubmed; PMID 16163384. doi:10.1038/sj.emboj.7600819
Alizadeh Pahlavani, H. (2022). Exercise therapy for people with sarcopenic obesity: Myokines and adipokines as effective actors. Front. Endocrinol. (Lausanne) 13, 811751. eng. The author declares that the research was conducted in the absence of any commercial or financial relationships that could be construed as a potential conflict of interest. Epub 20220217. Cited in: Pubmed; PMID 35250869. doi:10.3389/fendo.2022.811751
Alway, S. E., Mohamed, J. S., and Myers, M. J. (2017). Mitochondria initiate and regulate sarcopenia. Exerc Sport Sci. Rev. 45 (2), 58–69. eng. Cited in: Pubmed; PMID 28098577. doi:10.1249/jes.0000000000000101
Andersson, D. C., Betzenhauser, M. J., Reiken, S., Meli, A. C., Umanskaya, A., Xie, W., et al. (2011). Ryanodine receptor oxidation causes intracellular calcium leak and muscle weakness in aging. Cell Metab. 14 (2), 196–207. eng. Conflict of interest: A.R. Marks is a consultant for a start-up company, ARMGO Pharma Inc., that is targeting RyR1 to improve exercise capacity in muscle diseases. Cited in: Pubmed; PMID 21803290. doi:10.1016/j.cmet.2011.05.014
Balan, E., Schwalm, C., Naslain, D., Nielens, H., Francaux, M., and Deldicque, L. (2019). Regular endurance exercise promotes fission, mitophagy, and oxidative phosphorylation in human skeletal muscle independently of age. Front. Physiol. 10, 1088. eng. Epub 20190822. Cited in: Pubmed; PMID 31507451. doi:10.3389/fphys.2019.01088
Bano, D., and Prehn, J. H. M. (2018). Apoptosis-inducing factor (AIF) in Physiology and disease: The tale of a repented natural born killer. EBioMedicine 30, 29–37. eng. Epub 20180323. Cited in: Pubmed; PMID 29605508. doi:10.1016/j.ebiom.2018.03.016
Bell, M. B., Bush, Z., McGinnis, G. R., and Rowe, G. C. (2019). Adult skeletal muscle deletion of Mitofusin 1 and 2 impedes exercise performance and training capacity. J. Appl. Physiol. (1985) 126 (2), 341–353. eng. No conflicts of interest, financial or otherwise, are declared by the authors. Epub 20180927. Cited in: Pubmed; PMID 30260752. doi:10.1152/japplphysiol.00719.2018
Beyfuss, K., Erlich, A. T., Triolo, M., and Hood, D. A. (2018). The role of p53 in determining mitochondrial adaptations to endurance training in skeletal muscle. Sci. Rep. 8 (1), 14710. eng. The authors declare no competing interests. Epub 20181002. Cited in: Pubmed; PMID 30279494. doi:10.1038/s41598-018-32887-0
Bhasin, S., Travison, T. G., Manini, T. M., Patel, S., Pencina, K. M., Fielding, R. A., et al. (2020). Sarcopenia definition: The position statements of the sarcopenia definition and outcomes Consortium. J. Am. Geriatr. Soc. 68 (7), 1410–1418. eng. Epub 20200309. in: Pubmed; PMID 32150289. doi:10.1111/jgs.16372
Brand, M. D. (2016). Mitochondrial generation of superoxide and hydrogen peroxide as the source of mitochondrial redox signaling. Free Radic. Biol. Med. 100, 14–31. eng. Epub 20160413. Cited in: Pubmed; PMID 27085844. doi:10.1016/j.freeradbiomed.2016.04.001
Brioche, T., and Lemoine-Morel, S. (2016). Oxidative stress, sarcopenia, antioxidant strategies and exercise: Molecular aspects. Curr. Pharm. Des. 22 (18), 2664–2678. eng. Cited in: Pubmed; PMID 26891808. doi:10.2174/1381612822666160219120531
Carter, H. N., Chen, C. C., and Hood, D. A. (2015). Mitochondria, muscle health, and exercise with advancing age. Physiol. (Bethesda) 30 (3), 208–223. engin: Pubmed; PMID 25933821. doi:10.1152/physiol.00039.2014
Carter, H. N., Pauly, M., Tryon, L. D., and Hood, D. A. (2018). Effect of contractile activity on PGC-1α transcription in young and aged skeletal muscle. J. Appl. Physiol. (1985) 124 (6), 1605–1615. eng. Epub 20180315. Cited in: Pubmed; PMID 29543139. doi:10.1152/japplphysiol.01110.2017
Chabi, B., Ljubicic, V., Menzies, K. J., Huang, J. H., Saleem, A., and Hood, D. A. (2008). Mitochondrial function and apoptotic susceptibility in aging skeletal muscle. Aging Cell 7 (1), 2–12. eng. Epub 20071119 in: Pubmed; PMID 18028258. doi:10.1111/j.1474-9726.2007.00347.x
Cheema, N., Herbst, A., McKenzie, D., and Aiken, J. M. (2015). Apoptosis and necrosis mediate skeletal muscle fiber loss in age-induced mitochondrial enzymatic abnormalities. Aging Cell 14 (6), 1085–1093. eng. Epub 20150914. Cited in: Pubmed; PMID 26365892. doi:10.1111/acel.12399
Chen, L. K., Woo, J., Assantachai, P., Auyeung, T. W., Chou, M. Y., Iijima, K., et al. (2020). Asian working group for sarcopenia: 2019 consensus update on sarcopenia diagnosis and treatment. J. Am. Med. Dir. Assoc. 21 (3), 300–307.e2. e2. eng. Epub 20200204. Cited in: Pubmed; PMID 32033882. doi:10.1016/j.jamda.2019.12.012
Chen, N., He, X., Feng, Y., Ainsworth, B. E., and Liu, Y. (2021b). Effects of resistance training in healthy older people with sarcopenia: A systematic review and meta-analysis of randomized controlled trials. Eur. Rev. Aging Phys. Act. 18 (1), 23. eng. The author reports no conflicts of interest in this work. Epub 20211111 Cited in: Pubmed; PMID 34763651. doi:10.1186/s11556-021-00277-7
Chen, Z., Ho, M., and Chau, P. H. (2021a). Prevalence, incidence, and associated factors of possible sarcopenia in community-dwelling Chinese older adults: A population-based longitudinal study. Front. Med. (Lausanne) 8, 769708. The authors declare that the research was conducted in the absence of any commercial or financial relationships that could be construed as a potential conflict of interest. Epub 20220110. Cited in: Pubmed; PMID 35083235. doi:10.3389/fmed.2021.769708
Corsetto, P. A., Montorfano, G., Klersy, C., Massimino, L., Infantino, V., Iannello, G., et al. (2019). Fatty acid profile and antioxidant status fingerprint in sarcopenic elderly patients: Role of diet and exercise. Nutrients 11 (11), 2569. eng. The authors declare no conflict of interest. Epub 20191024 in: Pubmed; PMID 31653011. doi:10.3390/nu11112569
Cortopassi, G. A., and Arnheim, N. (1990). Detection of a specific mitochondrial DNA deletion in tissues of older humans. Nucleic Acids Res. 18 (23), 6927–6933. eng. Cited in: Pubmed; PMID 2263455. doi:10.1093/nar/18.23.6927
Cruz-Jentoft, A. J., Baeyens, J. P., Bauer, J. M., Boirie, Y., Cederholm, T., Landi, F., et al. (2010). Sarcopenia: European consensus on definition and diagnosis: Report of the European working group on sarcopenia in older people. Age Ageing 39 (4), 412–423. eng. Epub 20100413. Cited in: Pubmed; PMID 20392703. doi:10.1093/ageing/afq034
Cruz-Jentoft, A. J., Bahat, G., Bauer, J., Boirie, Y., Bruyère, O., Cederholm, T., et al. (2019). Sarcopenia: Revised European consensus on definition and diagnosis. Age Ageing 48 (1), 16–31. eng. Cited in: Pubmed; PMID 30312372. doi:10.1093/ageing/afy169
Cruz-Jentoft, A. J., and Sayer, A. A. (2019). Sarcopenia. Lancet. 393 (10191), 2636–2646. Cited in: Pubmed; PMID 31171417. eng. Epub 20190603. doi:10.1016/S0140-6736(19)31138-9
de Rezende, L. F., Rey-López, J. P., Matsudo, V. K. R., and do Carmo Luiz, O. (2014). Sedentary behavior and health outcomes among older adults: A systematic review. BMC Public Health 14, 333–2458. (Electronic)). eng. doi:10.1186/1471-2458-14-333
Debattisti, V., Horn, A., Singh, R., Seifert, E. L., Hogarth, M. W., Mazala, D. A., et al. (2019). Dysregulation of mitochondrial Ca(2+) uptake and sarcolemma repair underlie muscle weakness and wasting in patients and mice lacking MICU1. Cell Rep. 29 (5), 1274–1286.e6. e6. eng. DECLARATION OF INTERESTS The authors declare no competing interests. Cited in: Pubmed; PMID 31665639. doi:10.1016/j.celrep.2019.09.063
Dent, E., Woo, J., Scott, D., and Hoogendijk, E. O. (2021). Sarcopenia measurement in research and clinical practice. Eur. J. Intern Med. 90, 1–9. eng. Epub 20210706. Cited in: Pubmed; PMID 34238636. doi:10.1016/j.ejim.2021.06.003
Dethlefsen, M. M., Halling, J. F., Møller, H. D., Plomgaard, P., Regenberg, B., Ringholm, S., et al. (2018). Regulation of apoptosis and autophagy in mouse and human skeletal muscle with aging and lifelong exercise training. Exp. Gerontol. 111, 141–153. eng. Epub 20180717. Cited in: Pubmed; PMID 30030137. doi:10.1016/j.exger.2018.07.011
Ding, H., Jiang, N., Liu, H., Liu, X., Liu, D., Zhao, F., et al. (2010). Response of mitochondrial fusion and fission protein gene expression to exercise in rat skeletal muscle. Biochim. Biophys. Acta 1800 (3), 250–256. eng. Epub 20090828. Cited in: Pubmed; PMID 19716857. doi:10.1016/j.bbagen.2009.08.007
Edgett, B. A., Foster, W. S., Hankinson, P. B., Simpson, C. A., Little, J. P., Graham, R. B., et al. (2013). Dissociation of increases in PGC-1α and its regulators from exercise intensity and muscle activation following acute exercise. PLoS One 8 (8), e71623. eng. Competing Interests: The authors have declared that no competing interests exist. Epub 20130812. Cited in: Pubmed; PMID 23951207. doi:10.1371/journal.pone.0071623
Erlich, A. T., Tryon, L. D., Crilly, M. J., Memme, J. M., Moosavi, Z. S. M., Oliveira, A. N., et al. (2016). Function of specialized regulatory proteins and signaling pathways in exercise-induced muscle mitochondrial biogenesis. Integr. Med. Res. 5 (3), 187–197. eng. Epub 20160517. Cited in: Pubmed; PMID 28462117. doi:10.1016/j.imr.2016.05.003
Escriche-Escuder, A., Fuentes-Abolafio, I. J., Roldán-Jiménez, C., and Cuesta-Vargas, A. I. (2021). Effects of exercise on muscle mass, strength, and physical performance in older adults with sarcopenia: A systematic review and meta-analysis according to the EWGSOP criteria. Exp. Gerontol. 151, 111420. eng. Epub 20210523in: Pubmed; PMID 34029642. doi:10.1016/j.exger.2021.111420
Favaro, G., Romanello, V., Varanita, T., Andrea Desbats, M., Morbidoni, V., Tezze, C., et al. (2019). DRP1-mediated mitochondrial shape controls calcium homeostasis and muscle mass. Nat. Commun. 10 (1), 2576. eng. The authors declare no competing interests. Epub 20190612. Cited in: Pubmed; PMID 31189900. doi:10.1038/s41467-019-10226-9
Fiorenza, M., Gunnarsson, T. P., Hostrup, M., Iaia, F. M., Schena, F., Pilegaard, H., et al. (2018). Metabolic stress-dependent regulation of the mitochondrial biogenic molecular response to high-intensity exercise in human skeletal muscle. J. Physiol. 596 (14), 2823–2840. eng. Epub 20180626. Cited in: Pubmed; PMID 29727016. doi:10.1113/jp275972
Gao, H. E., Li, F. H., Xie, T., Ma, S., Qiao, Y. B., Wu, D. S., et al. (2021). Lifelong exercise in age rats improves skeletal muscle function and MicroRNA profile. Med. Sci. Sports Exerc 53 (9), 1873–1882. eng. Cited in: Pubmed; PMID 34398060. doi:10.1249/mss.0000000000002661
Gao, H. E., Wu, D. S., Sun, L., Yang, L. D., Qiao, Y. B., Ma, S., et al. (2020). Effects of lifelong exercise on age-related body composition, oxidative stress, inflammatory cytokines, and skeletal muscle proteome in rats. Mech. Ageing Dev. 189, 111262. eng. Epub 20200515 in: Pubmed; PMID 32422206. doi:10.1016/j.mad.2020.111262
Gherardi, G., Monticelli, H., Rizzuto, R., and Mammucari, C. (2020). The mitochondrial Ca(2+) uptake and the fine-tuning of aerobic metabolism. Front. Physiol. 11, 554904. eng. Epub 20201007. Cited in: Pubmed; PMID 33117189. doi:10.3389/fphys.2020.554904
Granata, C., Jamnick, N. A., and Bishop, D. J. (2018). Principles of exercise prescription, and how they influence exercise-induced changes of transcription factors and other regulators of mitochondrial biogenesis. Sports Med. 48 (7), 1541–1559. eng. Cited in: Pubmed; PMID 29675670. doi:10.1007/s40279-018-0894-4
Granata, C., Oliveira, R. S., Little, J. P., Renner, K., and Bishop, D. J. (2017). Sprint-interval but not continuous exercise increases PGC-1α protein content and p53 phosphorylation in nuclear fractions of human skeletal muscle. Sci. Rep. 7, 44227. eng. The authors declare no competing financial interests. Epub 20170310 in: Pubmed; PMID 28281651. doi:10.1038/srep44227
Granata, C., Oliveira, R. S., Little, J. P., Renner, K., and Bishop, D. J. (2016). Training intensity modulates changes in PGC-1α and p53 protein content and mitochondrial respiration, but not markers of mitochondrial content in human skeletal muscle. Faseb J. 30 (2), 959–970. eng. Epub 20151116. Cited in: Pubmed; PMID 26572168. doi:10.1096/fj.15-276907
Granata, C., Oliveira, R. S. F., Little, J. P., and Bishop, D. J. (2020). Forty high-intensity interval training sessions blunt exercise-induced changes in the nuclear protein content of PGC-1α and p53 in human skeletal muscle. Am. J. Physiol. Endocrinol. Metab. 318 (2), E224–e236. eng. No conflicts of interest, financial or otherwise, are declared by the authors. Epub 20191203. Cited in: Pubmed; PMID 31794264. doi:10.1152/ajpendo.00233.2019
Groussard, C., Maillard, F., Vazeille, E., Barnich, N., Sirvent, P., Otero, Y. F., et al. (2019). Tissue-specific oxidative stress modulation by exercise: A comparison between MICT and HIIT in an obese rat model. Oxid. Med. Cell Longev. 2019, 1965364. eng. No conflicts of interest, financial or otherwise, are declared by the authors. Epub 20190714 in: Pubmed; PMID 31396298. doi:10.1155/2019/1965364
Guan, R., Zou, W., Dai, X., Yu, X., Liu, H., Chen, Q., et al. (2018). Mitophagy, a potential therapeutic target for stroke. J. Biomed. Sci. 25 (1), 87. eng. AUTHORS’ INFORMATION: WZ is Vice chairman of China Institute of traditional Chinese medicine,encephalopathy branch; Vice chairman of World Federation of traditional Chinese Medicine, Mental disorder branch; Vice chairman of Heilongjiang province Association of Acupuncture and Moxibustion; Vice chairman of Association of the Integration of Traditional and Western Medicine and: Association of Traditional Chinese, neurology branch in Heilongjiang province; Expert reviewer of National Awards for science and technology . ETHICS APPROVAL AND CONSENT TO PARTICIPATE: Not applicable. CONSENT FOR PUBLICATION: Not applicable. COMPETING INTERESTS: The authors declare that they have no competing interests. PUBLISHER’S NOTE: Springer Nature remains neutral with regard to jurisdictional claims in published maps and institutional affiliations. Epub 20181130. Cited in: Pubmed; PMID 30501621. doi:10.1186/s12929-018-0487-4
Han, C., Lu, P., and Yan, S. Z. (2022). Effects of high-intensity interval training on mitochondrial supercomplex assembly and biogenesis, mitophagy, and the AMP-activated protein kinase pathway in the soleus muscle of aged female rats. Exp. Gerontol. 158, 111648. eng. Epub 20211130. in: Pubmed; PMID 34861356. doi:10.1016/j.exger.2021.111648
Harper, C., Gopalan, V., and Goh, J. (2021). Exercise rescues mitochondrial coupling in aged skeletal muscle: A comparison of different modalities in preventing sarcopenia. J. Transl. Med. 19 (1), 71. eng. The authors declare that they have no competing interests. Epub 20210216. Cited in: Pubmed; PMID 33593349. doi:10.1186/s12967-021-02737-1
Hayes, L. D., Elliott, B. T., Yasar, Z., Bampouras, T. M., Sculthorpe, N. F., Sanal-Hayes, N. E. M., et al. (2021). High intensity interval training (HIIT) as a potential countermeasure for phenotypic characteristics of sarcopenia: A scoping review. Front. Physiol. 12, 715044. eng. The authors declare that the research was conducted in the absence of any commercial or financial relationships that could be construed as a potential conflict of interest. Epub 20210824. Cited in: Pubmed; PMID 34504439. doi:10.3389/fphys.2021.715044
Herbst, A., Lee, C. C., Vandiver, A. R., Aiken, J. M., McKenzie, D., Hoang, A., et al. (2021). Mitochondrial DNA deletion mutations increase exponentially with age in human skeletal muscle. Aging Clin. Exp. Res. 33 (7), 1811–1820. eng. Conflicts of interest/Competing interests The authors declare no conflicts of interest/competing interests. Epub 20200923. Cited in: Pubmed; PMID 32965609. doi:10.1007/s40520-020-01698-7
Herbst, A., Wanagat, J., Cheema, N., Widjaja, K., McKenzie, D., and Aiken, J. M. (2016). Latent mitochondrial DNA deletion mutations drive muscle fiber loss at old age. Aging Cell 15 (6), 1132–1139. eng. Epub 20160825. Cited in: Pubmed; PMID 27561813. doi:10.1111/acel.12520
Hiona, A., Sanz, A., Kujoth, G. C., Pamplona, R., Seo, A. Y., Hofer, T., et al. (2010). Mitochondrial DNA mutations induce mitochondrial dysfunction, apoptosis and sarcopenia in skeletal muscle of mitochondrial DNA mutator mice. PLoS One 5 (7), e11468. eng. Competing Interests: GCK and TAP were awarded United States patent 7,126,040 for the PolgD257A mouse model. TAP is a co-founder and scientific consultant for LifeGen Technologies, specializing in the application of DNA microarray analysis to analyzing nutraceutical interventions in aging. Dr. Barger of LifeGen Technologies was involved with analysis of gene expression data from microarray experiments. This does not alter the authors' adherence to all of the PLoS ONE policies on sharing data and materials. Epub 20100707. Cited in: Pubmed; PMID 20628647. doi:10.1371/journal.pone.0011468
Huang, C. Y., Mayer, P. K., Wu, M. Y., Liu, D. H., Wu, P. C., and Yen, H. R. (2022). The effect of tai chi in elderly individuals with sarcopenia and frailty: A systematic review and meta-analysis of randomized controlled trials. Ageing Res. Rev. 82, 101747. eng. Conflict of interest The authors have no conflicts of interest relevant to this article to disclose. Epub 20221009. Cited in: Pubmed; PMID 36223875. doi:10.1016/j.arr.2022.101747
Ji, L. L. (2002). Exercise-induced modulation of antioxidant defense. Ann. N. Y. Acad. Sci. 959, 82–92. eng. Cited in: Pubmed; PMID 11976188. doi:10.1111/j.1749-6632.2002.tb02085.x
Johnson, J., Mercado-Ayon, E., Mercado-Ayon, Y., Dong, Y. N., Halawani, S., Ngaba, L., et al. (2021). Mitochondrial dysfunction in the development and progression of neurodegenerative diseases. Arch. Biochem. Biophys. 702, 108698. (Electronic)):108698. eng. Epub 20201128. Cited in: Pubmed; PMID 33259796. doi:10.1016/j.abb.2020.108698
Joseph, A. M., Adhihetty, P. J., Buford, T. W., Wohlgemuth, S. E., Lees, H. A., Nguyen, L. M., et al. (2012). The impact of aging on mitochondrial function and biogenesis pathways in skeletal muscle of sedentary high- and low-functioning elderly individuals. Aging Cell 11 (5), 801–809. eng. Epub 20120709 in: Pubmed; PMID 22681576. doi:10.1111/j.1474-9726.2012.00844.x
Joseph, A. M., Adhihetty, P. J., Wawrzyniak, N. R., Wohlgemuth, S. E., Picca, A., Kujoth, G. C., et al. (2013). Dysregulation of mitochondrial quality control processes contribute to sarcopenia in a mouse model of premature aging. PLoS One 8 (7), e69327. eng. Competing Interests: The authors have declared that no competing interests exist. Epub 20130723. Cited in: Pubmed; PMID 23935986. doi:10.1371/journal.pone.0069327
Kang, C., Chung, E., Diffee, G., and Ji, L. L. (2013). Exercise training attenuates aging-associated mitochondrial dysfunction in rat skeletal muscle: Role of PGC-1α. Exp. Gerontol. 48 (11), 1343–1350. eng. Epub 20130830. Cited in: Pubmed; PMID 23994518. doi:10.1016/j.exger.2013.08.004
Kim, J. S., Lee, Y. H., and Yi, H. K. (2016b). Gradual downhill running improves age-related skeletal muscle and bone weakness: Implication of autophagy and bone morphogenetic proteins. Exp. Physiol. 101 (12), 1528–1540. eng. Epub 20161113. Cited in: Pubmed; PMID 27641238. doi:10.1113/ep085852
Kim, Y. A., Kim, Y. S., Oh, S. L., Kim, H. J., and Song, W. (2013). Autophagic response to exercise training in skeletal muscle with age. J. Physiol. Biochem. 69 (4), 697–705. eng. Epub 20130308. Cited in: Pubmed; PMID 23471597. doi:10.1007/s13105-013-0246-7
Kim, Y. A., Kim, Y. S., and Song, W. (2012). Autophagic response to a single bout of moderate exercise in murine skeletal muscle. J. Physiol. Biochem. 68 (2), 229–235. eng. Epub 20111229. Cited in: Pubmed; PMID 22205581. doi:10.1007/s13105-011-0135-x
Kim, Y. H., Kim, K. I., Paik, N. J., Kim, K. W., Jang, H. C., and Lim, J. Y. (2016a). Muscle strength: A better index of low physical performance than muscle mass in older adults. Geriatr. Gerontol. Int. 16 (5), 577–585. eng. Epub 20150528. Cited in: Pubmed; PMID 26017097. doi:10.1111/ggi.12514
Kitaoka, Y., Ogasawara, R., Tamura, Y., Fujita, S., and Hatta, H. (2015). Effect of electrical stimulation-induced resistance exercise on mitochondrial fission and fusion proteins in rat skeletal muscle. Appl. Physiol. Nutr. Metab. 40 (11), 1137–1142. eng. Epub 20150714. Cited in: Pubmed; PMID 26513006. doi:10.1139/apnm-2015-0184
Koltai, E., Hart, N., Taylor, A. W., Goto, S., Ngo, J. K., Davies, K. J., et al. (2012). Age-associated declines in mitochondrial biogenesis and protein quality control factors are minimized by exercise training. Am. J. Physiol. Regul. Integr. Comp. Physiol. 303 (2), R127–R134. eng. Epub 20120509. Cited in: Pubmed; PMID 22573103. doi:10.1152/ajpregu.00337.2011
Langer, H. T., Mossakowski, A. A., Baar, K., Alcazar, J., Martin-Rincon, M., Alegre, L. M., et al. (2019). Commentaries on viewpoint: Rejuvenation of the term sarcopenia. J. Appl. Physiol. (1985) 126 (1), 257–262. eng. Cited in: Pubmed; PMID 30694711. doi:10.1152/japplphysiol.00816.2018
Lenhare, L., Crisol, B. M., Silva, V. R. R., Katashima, C. K., Cordeiro, A. V., Pereira, K. D., et al. (2017). Physical exercise increases Sestrin 2 protein levels and induces autophagy in the skeletal muscle of old mice. Exp. Gerontol. 97, 17–21. eng. Epub 20170717. Cited in: Pubmed; PMID 28729213. doi:10.1016/j.exger.2017.07.009
Li, B., Liang, F., Ding, X., Yan, Q., Zhao, Y., Zhang, X., et al. (2019a). Interval and continuous exercise overcome memory deficits related to β-Amyloid accumulation through modulating mitochondrial dynamics. Behav. Brain Res. 376, 112171. eng. Epub 20190822. in: Pubmed; PMID 31445975. doi:10.1016/j.bbr.2019.112171
Li, F. H., Sun, L., Wu, D. S., Gao, H. E., and Min, Z. (2019b). Proteomics-based identification of different training adaptations of aged skeletal muscle following long-term high-intensity interval and moderate-intensity continuous training in aged rats. Aging (Albany NY) 11 (12), 4159–4182. eng. Cited in: Pubmed; PMID 31241467. doi:10.18632/aging.102044
Li, F. H., Sun, L., Zhu, M., Li, T., Gao, H. E., Wu, D. S., et al. (2018). Beneficial alterations in body composition, physical performance, oxidative stress, inflammatory markers, and adipocytokines induced by long-term high-intensity interval training in an aged rat model. Exp. Gerontol. 113, 150–162. eng(Electronic)):. Epub 20181009. Cited in: Pubmed; PMID 30308288. doi:10.1016/j.exger.2018.10.006
Liang, J., Zeng, Z., Zhang, Y., and Chen, N. (2020). Regulatory role of exercise-induced autophagy for sarcopenia. Exp. Gerontol. 130, 110789. eng. Epub 20191122. Cited in: Pubmed; PMID 31765742. doi:10.1016/j.exger.2019.110789
Lindsay, J., Esposti, M. D., and Gilmore, A. P. (2011). Bcl-2 proteins and mitochondria--specificity in membrane targeting for death. Biochim. Biophys. Acta 1813 (4), 532–539. eng. Epub 20101105. Cited in: Pubmed; PMID 21056595. doi:10.1016/j.bbamcr.2010.10.017
Liu, D., Fan, Y. B., Tao, X. H., Pan, W. L., Wu, Y. X., Wang, X. H., et al. (2021). Mitochondrial quality control in sarcopenia: Updated overview of mechanisms and interventions. Aging Dis. 12 (8), 2016–2030. eng. Conflicts of interest The authors disclose no potential conflicts of interest. Epub 20211201. Cited in: Pubmed; PMID 34881083. doi:10.14336/ad.2021.0427
Liu, Q. Q., Xie, W. Q., Luo, Y. X., Li, Y. D., Huang, W. H., Wu, Y. X., et al. (2022). High intensity interval training: A potential method for treating sarcopenia. Clin. Interv. Aging 17, 857–872. eng. The authors declare that they have no competing interests. Epub 20220526. Cited in: Pubmed; PMID 35656091. doi:10.2147/cia.S366245
Ljubicic, V., and Hood, D. A. (2009). Diminished contraction-induced intracellular signaling towards mitochondrial biogenesis in aged skeletal muscle. Aging Cell 8 (4), 394–404. eng. Epub 20090422 in: Pubmed; PMID 19416128. doi:10.1111/j.1474-9726.2009.00483.x
Lu, L., Mao, L., Feng, Y., Ainsworth, B. E., Liu, Y., and Chen, N. (2021). Effects of different exercise training modes on muscle strength and physical performance in older people with sarcopenia: A systematic review and meta-analysis. BMC Geriatr. 21 (1), 708. eng. The authors declare that they have no competing interests. Epub 20211215. Cited in: Pubmed; PMID 34911483. doi:10.1186/s12877-021-02642-8
Lu, Y., Li, Z., Zhang, S., Zhang, T., Liu, Y., and Zhang, L. (2023). Cellular mitophagy: Mechanism, roles in diseases and small molecule pharmacological regulation. Theranostics 13 (2), 736–766. eng. Competing Interests: The authors have declared that no competing interest exists. Epub 20230101. Cited in: Pubmed; PMID 36632220. doi:10.7150/thno.79876
Luo, L., Lu, A. M., Wang, Y., Hong, A., Chen, Y., Hu, J., et al. (2013). Chronic resistance training activates autophagy and reduces apoptosis of muscle cells by modulating IGF-1 and its receptors, Akt/mTOR and Akt/FOXO3a signaling in aged rats. Exp. Gerontol. 48 (4), 427–436. eng. Epub 20130216. Cited in: Pubmed; PMID 23419688. doi:10.1016/j.exger.2013.02.009
Ma, K., Chen, G., Li, W., Kepp, O., Zhu, Y., and Chen, Q. (2020). Mitophagy, mitochondrial homeostasis, and cell fate. Front. Cell Dev. Biol. 8, 467. Epub 20200624. Cited in: Pubmed; PMID 32671064. doi:10.3389/fcell.2020.00467
Maclaine, K. D., Stebbings, K. A., Llano, D. A., and Rhodes, J. S. (2020). Voluntary wheel running has no impact on brain and liver mitochondrial DNA copy number or mutation measures in the PolG mouse model of aging. PLoS One 15 (3), e0226860. eng. The authors have declared that no competing interests exist. Epub 20200302. Cited in: Pubmed; PMID 32119683. doi:10.1371/journal.pone.0226860
Mahmoodzadeh, S., Koch, K., Schriever, C., Xu, J. M., Steinecker, M., Leber, J., et al. (2021). Age-related decline in murine heart and skeletal muscle performance is attenuated by reduced Ahnak1 expression. J. Cachexia Sarcopenia Muscle 12 (5), 1249–1265. English. Cited in: Pubmed; PMID WOS:000668827200001. doi:10.1002/jcsm.12749
Marshall, R. N., Smeuninx, B., Seabright, A. P., Morgan, P. T., Atherton, P. J., Philp, A., et al. (2022). No effect of five days of bed rest or short-term resistance exercise prehabilitation on markers of skeletal muscle mitochondrial content and dynamics in older adults. Physiol. Rep. 10 (13), e15345. eng. None of the authors have any conflicts of interest to disclose in: Pubmed; PMID 35785448. doi:10.14814/phy2.15345
Matta, L., Fonseca, T. S., Faria, C. C., Lima-Junior, N. C., De Oliveira, D. F., Maciel, L., et al. (2021). The effect of acute aerobic exercise on redox homeostasis and mitochondrial function of rat white adipose tissue. Oxid. Med. Cell Longev. 2021, 4593496. eng. The authors declare that they have no conflicts of interest. Epub 20210131 in: Pubmed; PMID 33603946. doi:10.1155/2021/4593496
Mejías-Peña, Y., Estébanez, B., Rodriguez-Miguelez, P., Fernandez-Gonzalo, R., Almar, M., de Paz, J. A., et al. (2017). Impact of resistance training on the autophagy-inflammation-apoptosis crosstalk in elderly subjects. Aging (Albany NY) 9 (2), 408–418. eng. CONFLICTS OF INTEREST The authors declare no potential conflicts of interest Cited in: Pubmed; PMID 28160545. doi:10.18632/aging.101167
Menzies, K. J., Singh, K., Saleem, A., and Hood, D. A. (2013). Sirtuin 1-mediated effects of exercise and resveratrol on mitochondrial biogenesis. J. Biol. Chem. 288 (10), 6968–6979. eng. Epub 20130117. in: Pubmed; PMID 23329826. doi:10.1074/jbc.m112.431155
Mesquita, P. H. C., Lamb, D. A., Parry, H. A., Moore, J. H., Smith, M. A., Vann, C. G., et al. (2020). Acute and chronic effects of resistance training on skeletal muscle markers of mitochondrial remodeling in older adults. Physiol. Rep. 8 (15), e14526. eng. MDR and KCY perform contracted studies for nutritional supplement companies, but they nor do any of the other authors have financial or other conflicts of interest to report with regard to these data. in: Pubmed; PMID 32748504. doi:10.14814/phy2.14526
Mishra, P., Varuzhanyan, G., Pham, A. H., and Chan, D. C. (2015). Mitochondrial dynamics is a distinguishing feature of skeletal muscle fiber types and regulates organellar compartmentalization. Cell Metab. 22 (6), 1033–1044. eng. Epub 20151022. Cited in: Pubmed; PMID 26603188. doi:10.1016/j.cmet.2015.09.027
Moore, T. M., Zhou, Z., Cohn, W., Norheim, F., Lin, A. J., Kalajian, N., et al. (2019). The impact of exercise on mitochondrial dynamics and the role of Drp1 in exercise performance and training adaptations in skeletal muscle. Mol. Metab. 21, 51–67. eng. Epub 20181204. Cited in: Pubmed; PMID 30591411. doi:10.1016/j.molmet.2018.11.012
Ola, M. S., Nawaz, M., and Ahsan, H. (2011). Role of Bcl-2 family proteins and caspases in the regulation of apoptosis. Mol. Cell Biochem. 351 (1-2), 41–58. eng. Epub 20110106. Cited in: Pubmed; PMID 21210296. doi:10.1007/s11010-010-0709-x
Park, J. H., Zhuang, J., Li, J., and Hwang, P. M. (2016). p53 as guardian of the mitochondrial genome. FEBS Lett. 590 (7), 924–934. eng. Epub 20160203 in: Pubmed; PMID 26780878. doi:10.1002/1873-3468.12061
Park, J. Y., Wang, P. Y., Matsumoto, T., Sung, H. J., Ma, W., Choi, J. W., et al. (2009). p53 improves aerobic exercise capacity and augments skeletal muscle mitochondrial DNA content. Circ. Res. 105 (7), 705–712. 11 p following 712, 11 p following 712. eng. Epub 20090820. Cited in: Pubmed; PMID 19696408. doi:10.1161/circresaha.109.205310
Petermann-Rocha, F., Balntzi, V., Gray, S. R., Lara, J., Ho, F. K., Pell, J. P., et al. (2022). Global prevalence of sarcopenia and severe sarcopenia: A systematic review and meta-analysis. J. Cachexia Sarcopenia Muscle 13 (1), 86–99. eng. None to declare. Epub 20211123. Cited in: Pubmed; PMID 34816624. doi:10.1002/jcsm.12783
Picard, M., Gentil, B. J., McManus, M. J., White, K., St Louis, K., Gartside, S. E., et al. (2013). Acute exercise remodels mitochondrial membrane interactions in mouse skeletal muscle. J. Appl. Physiol. (1985) 115 (10), 1562–1571. Epub 20130822. Cited in: Pubmed; PMID 23970537. doi:10.1152/japplphysiol.00819.2013
Pirani, H., Bakhtiari, A., Amiri, B., and Salehi, O. R. (2023). Beneficial mitochondrial biogenesis in gastrocnemius muscle promoted by high-intensity interval training in elderly female rats. Cell J. 25 (1), 11–16. eng. Epub 20230101. Cited in: Pubmed; PMID 36680479. doi:10.22074/cellj.2022.557565.1078
Protasi, F., Pietrangelo, L., and Boncompagni, S. (2021). Improper remodeling of organelles deputed to Ca(2+) handling and aerobic ATP production underlies muscle dysfunction in ageing. Int. J. Mol. Sci. 22 (12), 6195. eng. The authors declare no conflict of interest. Epub 20210608. Cited in: Pubmed; PMID 34201319. doi:10.3390/ijms22126195
Riley, B. E., Lougheed, J. C., Callaway, K., Velasquez, M., Brecht, E., Nguyen, L., et al. (2013). Structure and function of Parkin E3 ubiquitin ligase reveals aspects of RING and HECT ligases. Nat. Commun. 4, 1982. eng. Cited in: Pubmed; PMID 23770887. doi:10.1038/ncomms2982
Robinson, M. M., Turner, S. M., Hellerstein, M. K., Hamilton, K. L., and Miller, B. F. (2011). Long-term synthesis rates of skeletal muscle DNA and protein are higher during aerobic training in older humans than in sedentary young subjects but are not altered by protein supplementation. Faseb J. 25 (9), 3240–3249. eng. Epub 20110525 . Cited in: Pubmed; PMID 21613572. doi:10.1096/fj.11-186437
Rowe, G. C., El-Khoury, R., Patten, I. S., Rustin, P., and Arany, Z. (2012). PGC-1α is dispensable for exercise-induced mitochondrial biogenesis in skeletal muscle. PLoS One 7 (7), e41817. eng. Competing Interests: The authors have declared that no competing interests exist. Epub 20120724. Cited in: Pubmed; PMID 22848618. doi:10.1371/journal.pone.0041817
Safdar, A., Annis, S., Kraytsberg, Y., Laverack, C., Saleem, A., Popadin, K., et al. (2016). Amelioration of premature aging in mtDNA mutator mouse by exercise: The interplay of oxidative stress, PGC-1α, p53, and DNA damage. A hypothesis. Curr. Opin. Genet. Dev. 38, 127–132. eng. Epub 20160803. Cited in: Pubmed; PMID 27497229. doi:10.1016/j.gde.2016.06.011
Saleem, A., Adhihetty, P. J., and Hood, D. A. (2009). Role of p53 in mitochondrial biogenesis and apoptosis in skeletal muscle. Physiol. Genomics 37 (1), 58–66. eng. Epub 20081223. Cited in: Pubmed; PMID 19106183. doi:10.1152/physiolgenomics.90346.2008
Saleem, A., and Hood, D. A. (2013). Acute exercise induces tumour suppressor protein p53 translocation to the mitochondria and promotes a p53-Tfam-mitochondrial DNA complex in skeletal muscle. J. Physiol. 591 (14), 3625–3636. eng. Epub 20130520 in: Pubmed; PMID 23690562. doi:10.1113/jphysiol.2013.252791
Scarpulla, R. C., Vega, R. B., and Kelly, D. P. (2012). Transcriptional integration of mitochondrial biogenesis. Trends Endocrinol. Metab. 23 (9), 459–466. Epub 20120718. Cited in: Pubmed; PMID 22817841. doi:10.1016/j.tem.2012.06.006
Scott, F. L., Denault, J. B., Riedl, S. J., Shin, H., Renatus, M., and Salvesen, G. S. (2005). XIAP inhibits caspase-3 and -7 using two binding sites: Evolutionarily conserved mechanism of IAPs. Embo J. 24 (3), 645–655. eng. Epub 20050113. Cited in: Pubmed; PMID 15650747. doi:10.1038/sj.emboj.7600544
Selivanov, V. A., Votyakova, T. V., Pivtoraiko, V. N., Zeak, J., Sukhomlin, T., Trucco, M., et al. (2011). Reactive oxygen species production by forward and reverse electron fluxes in the mitochondrial respiratory chain. PLoS Comput. Biol. 7 (3), e1001115. eng. The authors have declared that no competing interests exist. Epub 20110331. Cited in: Pubmed; PMID 21483483. doi:10.1371/journal.pcbi.1001115
Selsby, J. T. (2011). Increased catalase expression improves muscle function in mdx mice. Exp. Physiol. 96 (2), 194–202. eng. Epub 20101101. Cited in: Pubmed; PMID 21041317. doi:10.1113/expphysiol.2010.054379
Shang, H., Xia, Z., Bai, S., Zhang, H. E., Gu, B., and Wang, R. (2019). Downhill running acutely elicits mitophagy in rat soleus muscle. Med. Sci. Sports Exerc 51 (7), 1396–1403. eng. Cited in: Pubmed; PMID 30649103. doi:10.1249/mss.0000000000001906
Shen, Y., Shi, Q., Nong, K., Li, S., Yue, J., Huang, J., et al. (2023). Exercise for sarcopenia in older people: A systematic review and network meta-analysis. J. Cachexia Sarcopenia Muscle 14, 1199–1211. eng. Epub 20230414. Cited in: Pubmed; PMID 37057640. doi:10.1002/jcsm.13225
Silva Ramos, E., Motori, E., Brüser, C., Kühl, I., Yeroslaviz, A., Ruzzenente, B., et al. (2019). Mitochondrial fusion is required for regulation of mitochondrial DNA replication. PLoS Genet. 15 (6), e1008085. eng. The authors have declared that no competing interests exist. Epub 20190606. Cited in: Pubmed; PMID 31170154. doi:10.1371/journal.pgen.1008085
Song, W., Kwak, H. B., and Lawler, J. M. (2006). Exercise training attenuates age-induced changes in apoptotic signaling in rat skeletal muscle. Antioxid. Redox Signal 8 (3-4), 517–528. eng. Cited in: Pubmed; PMID 16677096. doi:10.1089/ars.2006.8.517
Stotland, A., and Gottlieb, R. A. (2015). Mitochondrial quality control: Easy come, easy go. Biochim. Biophys. Acta 1853 (10), 2802–2811. eng. Epub 20150114. Cited in: Pubmed; PMID 25596427. doi:10.1016/j.bbamcr.2014.12.041
Strubbe-Rivera, J. O., Schrad, J. R., Pavlov, E. V., Conway, J. F., Parent, K. N., and Bazil, J. N. (2021). The mitochondrial permeability transition phenomenon elucidated by cryo-EM reveals the genuine impact of calcium overload on mitochondrial structure and function. Sci. Rep. 11 (1), 1037. eng. The authors declare no competing interests. Epub 20210113. Cited in: Pubmed; PMID 33441863. doi:10.1038/s41598-020-80398-8
Su, H., Wen, T., Liu, D., Shao, J., Zhao, L., and Gao, Q. (2022). Effect of 32-weeks high-intensity interval training and resistance training on delaying sarcopenia: Focus on endogenous apoptosis. Front. Physiol. 13, 811369. eng. The authors declare that the research was conducted in the absence of any commercial or financial relationships that could be construed as a potential conflict of interest. Epub 20220428. Cited in: Pubmed; PMID 35574455. doi:10.3389/fphys.2022.811369
Sullivan-Gunn, M. J., and Lewandowski, P. A. (2013). Elevated hydrogen peroxide and decreased catalase and glutathione peroxidase protection are associated with aging sarcopenia. BMC Geriatr. 13, 104. eng. Epub 20131007. Cited in: Pubmed; PMID 24093947. doi:10.1186/1471-2318-13-104
Talar, K., Hernández-Belmonte, A., Vetrovsky, T., Steffl, M., Kałamacka, E., and Courel-Ibáñez, J. (2021). Benefits of resistance training in early and late stages of frailty and sarcopenia: A systematic review and meta-analysis of randomized controlled studies. J. Clin. Med. 10 (8), 1630. eng. The authors declare no conflict of interest. Epub 20210412. Cited in: Pubmed; PMID 33921356. doi:10.3390/jcm10081630
Thoma, A., Akter-Miah, T., Reade, R. L., and Lightfoot, A. P. (2020). Targeting reactive oxygen species (ROS) to combat the age-related loss of muscle mass and function. Biogerontology 21 (4), 475–484. eng. Epub 20200523. Cited in: Pubmed; PMID 32447556. doi:10.1007/s10522-020-09883-x
Vande Walle, L., Lamkanfi, M., and Vandenabeele, P. (2008). The mitochondrial serine protease HtrA2/omi: An overview. Cell Death Differ. 15 (3), 453–460. eng. Epub 20080104. Cited in: Pubmed; PMID 18174901. doi:10.1038/sj.cdd.4402291
Vilela, T. C., Effting, P. S., Dos Santos Pedroso, G., Farias, H., Paganini, L., Rebelo Sorato, H., et al. (2018). Aerobic and strength training induce changes in oxidative stress parameters and elicit modifications of various cellular components in skeletal muscle of aged rats. Exp. Gerontol. 106, 21–27. eng. Epub 20180219. Cited in: Pubmed; PMID 29471131. doi:10.1016/j.exger.2018.02.014
Wai, T., and Langer, T. (2016). Mitochondrial dynamics and metabolic regulation. Trends Endocrinol. Metab. 27 (2), 105–117. eng. Epub 20160102. Cited in: Pubmed; PMID 26754340. doi:10.1016/j.tem.2015.12.001
Waltz, T. B., Fivenson, E. M., Morevati, M., Li, C., Becker, K. G., Bohr, V. A., et al. (2018). Sarcopenia, aging and prospective interventional strategies. Curr. Med. Chem. 25 (40), 5588–5596. eng. in: Pubmed; PMID 28762310. doi:10.2174/0929867324666170801095850
Wang, C., Liang, J., Ren, Y., Huang, J., Jin, B., Wang, G., et al. (2022b). A preclinical systematic review of the effects of chronic exercise on autophagy-related proteins in aging skeletal muscle. Front. Physiol. 13, 930185. eng. The authors declare that the research was conducted in the absence of any commercial or financial relationships that could be construed as a potential conflict of interest. Epub 20220714. Cited in: Pubmed; PMID 35910582. doi:10.3389/fphys.2022.930185
Wang, H., Huang, W. Y., and Zhao, Y. (2022a). Efficacy of exercise on muscle function and physical performance in older adults with sarcopenia: An updated systematic review and meta-analysis. Int. J. Environ. Res. Public Health 19 (13), 8212. eng. The authors declare no conflict of interest. Epub 20220705. Cited in: Pubmed; PMID 35805870. doi:10.3390/ijerph19138212
Wang, P., Li, C. G., Qi, Z., Cui, D., and Ding, S. (2016). Acute exercise stress promotes Ref1/Nrf2 signalling and increases mitochondrial antioxidant activity in skeletal muscle. Exp. Physiol. 101 (3), 410–420. eng. Epub 20160123. Cited in: Pubmed; PMID 26682532. doi:10.1113/ep085493
Woo, J., Arai, H., Ng, T. P., Sayer, A. A., Wong, M., Syddall, H., et al. (2014). Ethnic and geographic variations in muscle mass, muscle strength and physical performance measures. Eur. Geriatr. Med. 5 (3), 155–164. doi:10.1016/j.eurger.2014.04.003
Wu, D., Dasgupta, A., Read, A. D., Bentley, R. E. T., Motamed, M., Chen, K. H., et al. (2021). Oxygen sensing, mitochondrial biology and experimental therapeutics for pulmonary hypertension and cancer. Free Radic. Biol. Med. 170, 150–178. eng. Epub 20210112. Cited in: Pubmed; PMID 33450375. doi:10.1016/j.freeradbiomed.2020.12.452
Wu, S., Ning, H. T., Xiao, S. M., Hu, M. Y., Wu, X. Y., Deng, H. W., et al. (2020). Effects of vibration therapy on muscle mass, muscle strength and physical function in older adults with sarcopenia: A systematic review and meta-analysis. Eur. Rev. Aging Phys. Act. 17, 14. eng. Competing interestsThe authors declare that there is no conflict of interest. Epub 20200917. Cited in: Pubmed; PMID 32963629. doi:10.1186/s11556-020-00247-5
Wu, Y. Z., Loh, C. H., Hsieh, J. G., and Lin, S. Z. (2022). Physical inactivity and possible sarcopenia in rural community daycare stations of taiwan: A cross-sectional study. Int. J. Environ. Res. Public Health 19 (4), 2182. The authors declare no conflict of interest. Epub 20220215. Cited in: Pubmed; PMID 35206367. doi:10.3390/ijerph19042182
Wyckelsma, V. L., Levinger, I., McKenna, M. J., Formosa, L. E., Ryan, M. T., Petersen, A. C., et al. (2017). Preservation of skeletal muscle mitochondrial content in older adults: Relationship between mitochondria, fibre type and high-intensity exercise training. J. Physiol. 595 (11), 3345–3359. eng. Epub 20170423. Cited in: Pubmed; PMID 28251664. doi:10.1113/jp273950
Xu, H., Ranjit, R., Richardson, A., and Van Remmen, H. (2021). Muscle mitochondrial catalase expression prevents neuromuscular junction disruption, atrophy, and weakness in a mouse model of accelerated sarcopenia. J. Cachexia Sarcopenia Muscle 12 (6), 1582–1596. eng. None declared. Epub 20210924. Cited in: Pubmed; PMID 34559475. doi:10.1002/jcsm.12768
Yadav, N., Gogada, R., O'Malley, J., Gundampati, R. K., Jayanthi, S., Hashmi, S., et al. (2020). Molecular insights on cytochrome c and nucleotide regulation of apoptosome function and its implication in cancer. Biochim. Biophys. Acta Mol. Cell Res. 1867 (1), 118573. eng. CONFLICT OF INTEREST The authors declare that they have no conflicts of interest with the contents of this article. Epub 20191031. in: Pubmed; PMID 31678591. doi:10.1016/j.bbamcr.2019.118573
Yang, Y. F., Yang, W., Liao, Z. Y., Wu, Y. X., Fan, Z., Guo, A., et al. (2021). MICU3 regulates mitochondrial Ca(2+)-dependent antioxidant response in skeletal muscle aging. Cell Death Dis. 12 (12), 1115. eng. The authors declare no competing interests. Epub 20211129. Cited in: Pubmed; PMID 34845191. doi:10.1038/s41419-021-04400-5
Yu, L., Shi, X. Y., Liu, Z. M., Wang, Z., Li, L., Gao, J. X., et al. (2020). Effects of exercises with different durations and intensities on mitochondrial autophagy and FUNDC1 expression in rat skeletal muscles. Sheng Li Xue Bao 72 (5), 631–642. chi. Cited in: Pubmed; PMID 33106833.
Yu, R., Jin, S. B., Lendahl, U., Nistér, M., and Zhao, J. (2019). Human Fis1 regulates mitochondrial dynamics through inhibition of the fusion machinery. Embo J. 38 (8), e99748. eng. UL holds research grants from Merck AG and AstraZeneca, no personal remuneration. The other authors declare no competing financial interests. Epub 20190306. Cited in: Pubmed; PMID 30842096. doi:10.15252/embj.201899748
Zampieri, S., Mammucari, C., Romanello, V., Barberi, L., Pietrangelo, L., Fusella, A., et al. (2016). Physical exercise in aging human skeletal muscle increases mitochondrial calcium uniporter expression levels and affects mitochondria dynamics. Physiol. Rep. 4 (24), e13005. eng in: Pubmed; PMID 28039397. doi:10.14814/phy2.13005
Zeng, Z., Liang, J., Wu, L., Zhang, H., Lv, J., and Chen, N. (2020). Exercise-induced autophagy suppresses sarcopenia through at/mTOR and at/FoxO3a signal pathways and AMPK-mediated mitochondrial quality control. Front. Physiol. 11, 583478. eng. Epub 20201102. Cited in: Pubmed; PMID 33224037. doi:10.3389/fphys.2020.583478
Zhang, C. S., and Lin, S. C. (2016). AMPK promotes autophagy by facilitating mitochondrial fission. Cell Metab. 23 (3), 399–401. Cited in: Pubmed; PMID 26959181 eng. doi. doi:10.1016/j.cmet.2016.02.017
Ziaaldini, M. M., Koltai, E., Csende, Z., Goto, S., Boldogh, I., Taylor, A. W., et al. (2015). Exercise training increases anabolic and attenuates catabolic and apoptotic processes in aged skeletal muscle of male rats. Exp. Gerontol. 67, 9–14. eng. Epub 20150421. Cited in: Pubmed; PMID 25910622. doi:10.1016/j.exger.2015.04.008
Zoladz, J. A., Majerczak, J., Galganski, L., Grandys, M., Zapart-Bukowska, J., Kuczek, P., et al. (2022). Endurance training increases the running performance of untrained men without changing the mitochondrial volume density in the gastrocnemius muscle. Int. J. Mol. Sci. 23 (18), 10843. eng. The authors declare no conflicts of interest. Epub 20220916. Cited in: Pubmed; PMID 36142755. doi:10.3390/ijms231810843
Zong, H., Ren, J. M., Young, L. H., Young Lh Fau - Pypaert, M., Pypaert, M., Fau - Mu, J., et al. (2002). AMP kinase is required for mitochondrial biogenesis in skeletal muscle in response to chronic energy deprivation. Proc. Natl. Acad. Sci. U. S. A. 99, 15983–15987. (Print)). eng. doi:10.1073/pnas.252625599
Keywords: sarcopenia, exercise, mitochondrial dysfunction, mitochondrial biogenesis, mitochondrial apoptosis
Citation: Zhu Y, Zhou X, Zhu A, Xiong S, Xie J and Bai Z (2023) Advances in exercise to alleviate sarcopenia in older adults by improving mitochondrial dysfunction. Front. Physiol. 14:1196426. doi: 10.3389/fphys.2023.1196426
Received: 29 March 2023; Accepted: 23 June 2023;
Published: 05 July 2023.
Edited by:
Kai Chen, University of Western Australia, AustraliaReviewed by:
Raoof Negaresh, Tarbiat Modares University, IranHamed Alizadeh Pahlavani, Farhangian University, Iran
Copyright © 2023 Zhu, Zhou, Zhu, Xiong, Xie and Bai. This is an open-access article distributed under the terms of the Creative Commons Attribution License (CC BY). The use, distribution or reproduction in other forums is permitted, provided the original author(s) and the copyright owner(s) are credited and that the original publication in this journal is cited, in accordance with accepted academic practice. No use, distribution or reproduction is permitted which does not comply with these terms.
*Correspondence: Zhenmin Bai, tcmbai@bsu.edu.cn
†These authors share first authorship