- 1Key Laboratory of Northern Urban Agriculture of Ministry of Agriculture and Rural Affairs, College of Bioscience and Resource Environment, Beijing University of Agriculture, Beijing, China
- 2Key Laboratory of Pollinating Insect Biology of Agriculture, Institute of Apicultural Research, Chinese Academy of Agricultural Sciences, Beijing, China
Background: Chlorothalonil and acetamiprid are chemical pesticides commonly used in agricultural production and have been shown to have negative effects on bee’s fitness. Despite many studies have revealed that honey bee (Apis mellifera L.) larvae are posting a high risk on exposure to pesticides, but the toxicology information of chlorothalonil and acetamiprid on bee larvae remain limited.
Results: The no observed adverse effect concentration (NOAEC) of chlorothalonil and acetamiprid for honey bee larvae were 4 μg/mL and 2 μg/mL, respectively. Except for CarE, the enzymic activities of GST and P450 were not influenced by chlorothalonil at NOAEC, while chronic exposure to acetamiprid slightly increased the activities of the three tested enzymes at NOAEC. Further, the exposed larvae showed significantly higher expression of genes involved in a series of different toxicologically relevant process following, including caste development (Tor (GB44905), InR-2 (GB55425), Hr4 (GB47037), Ac3 (GB11637) and ILP-2 (GB10174)), immune system response (abaecin (GB18323), defensin-1 (GB19392), toll-X4 (GB50418)), and oxidative stress response (P450, GSH, GST, CarE).
Conclusion: Our results suggest that the exposure to chlorothalonil and acetamiprid, even at concentrations below the NOAEC, showed potentially effects on bee larvae’s fitness, and more important synergistic and behavioral effects that can affect larvae fitness should be explored in the further.
Introduction
Over the last few decades, significant declines in insect populations and diversity in several regions of the globe have been alarming, especially for bee species (El-Seedi et al., 2022). Although multiple factors likely contribute to the observed bee declines, e.g., the parasitic mite, viruses, pollution and climate change (Human et al., 2014; Myers et al., 2017; Dolezal et al., 2019; Traynor et al., 2020), an essential reason is excessive pesticide use of agrochemicals, which has been proved to lead to impairment of essential functions, such as reproduction, foraging and homing, thereby affecting overall health and population of the bee colony (Wang et al., 2020; Wagner et al., 2021). Thus, much attention has been directed toward the safety of pesticides to bees.
Compared to adults, Apis mellifera (honey bee) larvae are more susceptible to long-term exposure to sublethal doses of pesticides (Desneux et al., 2007). During flowering period, pollen and nectar with pesticide residues collected by foragers will be processed by the nurse bees and fed to developing larvae, providing potential opportunities for bee larvae to be exposed to pesticides during whole pre-brood stage (Mullin et al., 2010; Dai et al., 2018; Quintana et al., 2019). Moreover, high levels of pesticides were detected in beeswax from the brood nest where larvae develop (Medici et al., 2012; McAfee et al., 2021). Despite indirectly exposed to pesticides, larvae are usually less tolerant to pesticides than adults (Tan et al., 2017; Dai et al., 2018; O’neal et al., 2019; Tavares et al., 2019), nevertheless, the toxicology information on honey bee larvae are rare at present.
Pesticides have a range of sublethal effects on larvae of honey bee. Previous studies have revealed that chronic exposure to pesticides during the larval period can disrupt the normal growth and development of honey bee larvae, leading to malformations, reduced body size, and delayed development (Wu et al., 2011; Tan et al., 2015; Rosa et al., 2016). Long-term exposure to pesticides at early life stages can damage the nervous system of honey bee larvae, leading to behavioral abnormalities and reduced cognitive function as adult individuals (Tan et al., 2017). In addition, pesticides can weaken the immune and defense system of honey bee larvae, making them more susceptible to diseases and parasites (Tarek et al., 2018). For instance, exposure to sublethal doses of thiacloprid significantly aggravated the proliferation of black queen cell virus (BQCV) on host larval and boost the harmful effects of the virus on honey bees (Doublet et al., 2015). Sublethal pesticide exposure in honey bees larvae can cause oxidative stress by increasing the levels of reactive oxygen species (ROS) in the bees’ bodies, which can cause damage to cellular components such as DNA, proteins, and lipids (Prezenska et al., 2019; Yu et al., 2021). The GST (glutathione S-transferase) gene family encodes enzymes that participates in the process of ROS removal by interaction with glutathione (Schultzhaus et al., 2017). Catalase (CAT) and superoxide dismutase (SOD) are important enzymes establishing the first line of antioxidant defense systems. Previous studies have demonstrated that when exposed to low doses of pesticides, honey bee larvae are able to neutralize harmful oxidative compounds produced by oxidative stress via upregulating the expression of these enzymes or elevating their activities (du Rand et al., 2017; Yu et al., 2021).
Fungicides and neonicotinoid insecticides are common xenobiotics detected in bee products (Pareja et al., 2011; Bridi et al., 2018). Chlorothalonil (2,4,5,6-tetrachloroisophthalonitrile) is one of the most popular broad-spectrum protective fungicide in the world (Wang et al., 2021). Chlorothalonil is commonly applied to flowering crops, thus providing a possible route of exposure for bees. Mullin et al. (2010) have reported a maximum residue value of 99 mg a.i./kg for chlorothalonil detected in pollen (Mullin et al., 2010). Although not acutely toxic to bees, several studies have identified potential sublethal effects, especially on larvae (Zhu et al., 2014; Dai et al., 2018; O’neal et al., 2019). As a fungicide, chlorothalonil may affect the fungal community in the honey bee gut. Our previous work showed that chlorothalonil significantly decreased the survival rate of immature bees and altered the gut microbiota of newly emerged honeybees when exposure concentrations were higher than 2 μg/mL (Wu et al., 2022). Acetamiprid is a representative of the first generation of neonicotinoids with broad-spectrum characteristic, widely used to control destructive agricultural pests (Shi et al., 2019). Given their low toxicity to mammalian and non-target pollinators, acetamiprid has become the key available neonicotinoid pesticides throughout the world (Wang et al., 2017; Grassl et al., 2018). It has been shown that sublethal acetamiprid doses had a negative effect on the learning and memory ability of adult bees (Wen et al., 2017; Chen et al., 2020). Chronic exposure to acetamiprid was found to have an effect on the expression of genes related to immune, detoxification, and memory in larvae and adults (Shi et al., 2020).
The goal of this study was to investigate the effects of chronic exposure to the chlorothalonil and acetamiprid on A. mellifera larvae. Through the in vitro larval rearing method, we measure the no observed adverse effect concentration (NOAEC) of larval bees. Furthermore, we evaluate the sublethal effects of chlorothalonil and acetamiprid on humoral immunity and biochemical markers of exogenous substance, as well as gene expression involved in honey bee caste development. This work will provide additional information on the risks of chlorothalonil and acetamiprid exposure to honey bee larvae, and ultimately help to determine the integrated pest management strategies that minimize the harm of pesticides to honey bees.
Materials and methods
Honey bees
Larvae source colonies were kept in the Institute of Apicultural Research, Chinese Academy of Agricultural Sciences (40°01′23′′N, 116°21′24′′E). The honey bee larvae were transferred to the laboratory and reared in incubator (Ningbo Haishu Saifu Experimental Instrument Factory) (rearing temperature 35°C ± 0.5°C, relative humidity 95% ± 5%).
Chronic toxicity
The experiment consisted of 13 treatments, including chlorothalonil (purity 99.7% purchased from Sigma-Aldrich, Shanghai, China) at concentrations of 1, 2, 4, 8 and 16 μg/mL; acetamiprid (purity 99.9% purchased from BePure, Shanghai, China) at concentrations of 0.5, 1, 2, 4 and 8 μg/mL; a negative control; a solvent control (0.1% acetone) and a positive control (dimethoate purity (99.3% purchased from Sigma-Aldrich, Shanghai, China) 45 μg/mL). Each treatment was repeated three times. Larvae were taken from three different colonies, a single colony per replicate. A. mellifera larvae were reared in vitro as described by Yang et al. (2020). We obtained excessive 1-day-old larvae and fed 20 μL normal diet on D1. On the third day, 12 healthy larvae were selected from each replicate and fed with a 20 μL diet that contained different dilutions of chlorothalonil and acetamiprid. Each larva was respectively fed with 30, 40, 50 μL treated diet on D4, D5 and D6.
Preparation of cDNA and qRT-PCR
On day 7, the A. mellifera larvae were collected and frozen in liquid nitrogen, then stored at −8 °C. RNA was extracted from larvae using TRIzol reagent. RNA quality was checked using the BioSpectrometer® kinetic (Eppendorf, America). An OD260/280 ratio of RNA between 1.8 and 2.0 is required to meet the criteria. Then, using PrimeScript™ RT reagent Kit with gDNA Eraser (Takara, Japan), cDNA was obtained from 1.0 μg RNA. The RT-qPCR was performed as follows: 30 s at 95°C, followed by 40 cycles of 5 s at 95°C, then 30 s at 60°C. RT-qPCR was carried in triplicate. Table 1 summarizes the primers information and uses the house keeping gene β-actin as the control. The relative expression of the test gene was calculated using the method reported by Qi et al. (2020).
Activity of detoxification enzymes
All of honey bee larvae were collected and quickly placed in liquid nitrogen and stored at −80°C on D7. Homogenize each larval sample independently on ice in pre-chilled pH 7.4 PBS, then Centrifuge at 3,500 rpm for 20 min at 4°C. The protein concentration in the supernatant was determined by using the BCA Protein Assay Kit (Thermo Fisher Scientific, America). The P450, GST and CarE enzyme activities of the supernatant were determined using the ELISA assay kit from Shanghai Mlbio, China.
Statistics
All data are expressed as the mean ± SE. The JMP 13 software produced the Kaplan-Meier curve. GraphPad Prism 9.0 were performed for One-way ANOVA on enzyme activity and gene expression levels. Tukey’s test (p = 0.05) was performed to determine the difference between every treatment and solvent control.
Results
Chronic toxicity of acetamiprid and chlorothalonil to honey bee larvae
The survival of larvae fed with 8 and 16 μg/mL chlorothalonil was significantly lower than that of larvae fed the negative and solvent control diets (Figure 1A). However, the survival of larvae fed with 1, 2 and 4 μg/mL chlorothalonil was not significantly different from that of larvae fed with the solvent control and the negative control diets. The NOAEC of chlorothalonil to honey bee larvae was 4 μg/mL. The survival of larvae fed with 4 and 8 μg/mL acetamiprid was significantly lower than that of larvae fed the negative and solvent control diets (Figure 1B). However, the survival of larvae fed with 0.5, 1 and 2 μg/mL acetamiprid was not significantly different from that of larvae fed with the solvent control and the negative control diets. The NOAEC of acetamiprid to honey bee larvae was 2 μg/mL.
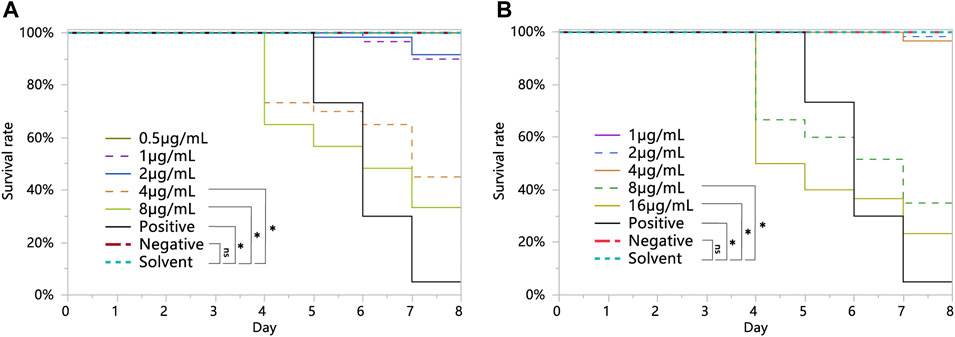
FIGURE 1. Overall survival of Apis mellifera larvae exposed to sublethal concentrations of chlorothalonil (A), acetamiprid (B), during larval development on D3 through D6 after grafting (n = 3 replicates of 12 larvae/replicate, or 36 larvae, per test substance). Larvae were fed a dimethoate-contaminated diet (45 mg/L) as a positive control, an acetone-contaminated diet as a solvent control. Significant differences were set at * for p < 0.05.
Influence of acetamiprid and chlorothalonil on the expression of division related genes
Through statistical analysis, we found that survival rate had no significant difference between negative controls and solvent controls in our research. Thus, only solvent control was used for the following analysis.
The relative expression of division related genes in honey bee larvae were quantified. As shown in Figure 2, the Tor and AmInR-2 transcripts were upregulated upon exposure to 1, 2 and 4 μg/mL chlorothalonil. The expression of Hr4 was upregulated at 4.1 folds and 3.5 folds at 1 μg/mL chlorothalonil and 2 μg/mL acetamiprid, but this change did not occur after treatment with 2 and 4 μg/mL chlorothalonil (Figure 2C). The transcript of Ac3 was strong upregulated at 2 and 4 μg/mL chlorothalonil (Figure 2D). Acetamiprid induced the expression of ILP-2 at 2 μg/mL, but did not alter the abundance of the other transcripts. And the ILP-2 transcript was upregulated after expose to 1, 2, 4 μg/mL chlorothalonil (Figure 2E). When the concentration of acetamiprid was less than 2 μg/mL, the expression of all division related genes we tested was not significantly affected.
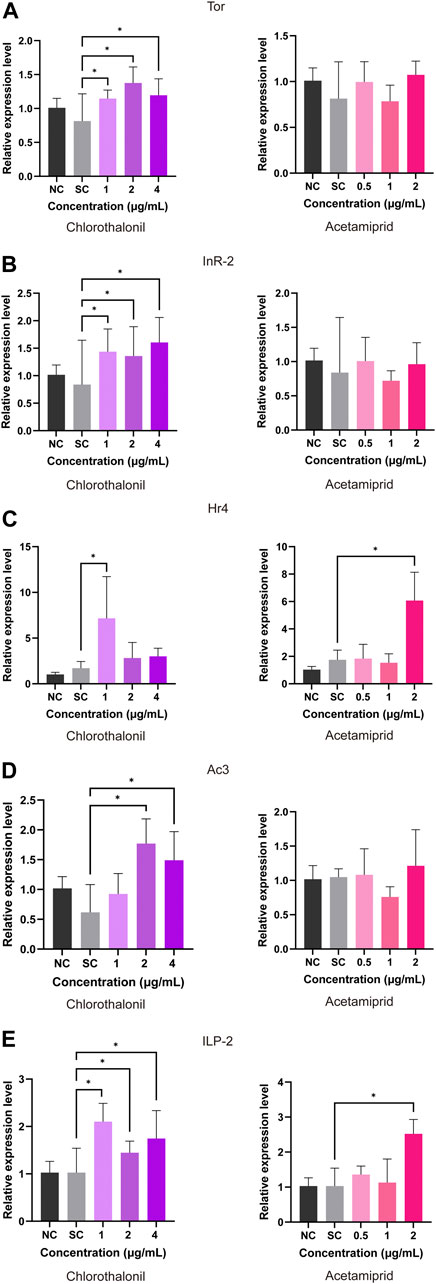
FIGURE 2. Effect of chlorothalonil and acetamiprid on the relative expression levels on Tor (A), AmInR-2 (B), Hr4 (C), Ac3 (D) and ILP-2 (E) genes in A. mellifera larvae. After exposed to 1, 2 and 4 μg/mL chlorothalonil or 0.5, 1 and 2 μg/mL acetamiprid for 4 days, larvae were collected and total RNA was extracted. Each sample was assayed 4 times. Expression levels were normalized to actin and then to the gene expression level of the solvent control (SC). Significant differences were set at * for p < 0.05.
Influence of acetamiprid and chlorothalonil on the expression of immune related genes
The transcript of abaecin was significantly upregulated at 2 μg/mL acetamiprid but did not remarkably affected by chlorothalonil (Figure 3A). In comparison to the solvent control, the expression of defensin was facilitated by 2 μg/mL acetamiprid or 4 μg/mL chlorothalonil (Figure 3B). Exposure to chlorothalonil resulted in upregulation of the toll-X4 transcript at 1 and 2 μg/mL chlorothalonil, while acetamiprid had no effects on the expression of these transcripts (Figure 3C). But when the concentration of chlorothalonil reached 4 μg/mL, the expression of toll-X4 was downregulated to the control value (Figure 3C).
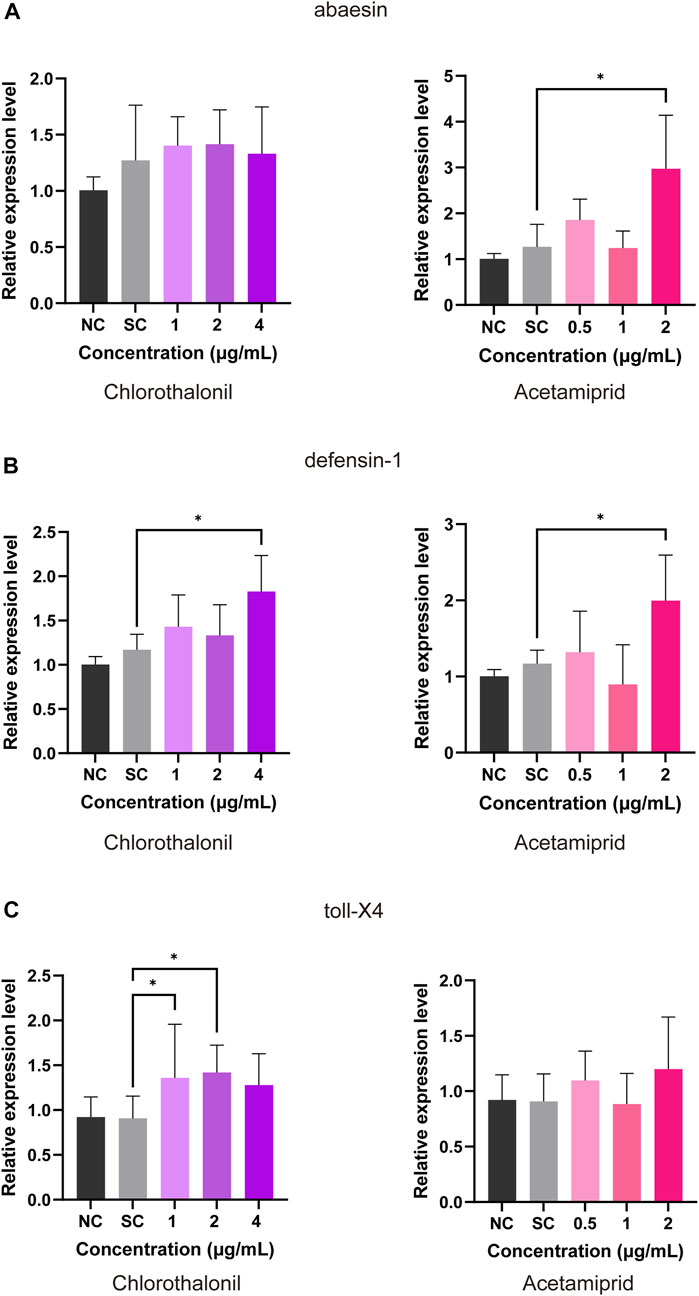
FIGURE 3. Effect of chlorothalonil and acetamiprid on the relative expression levels on abaecin (A), defensin (B) and toll-X4 (C) genes in A. mellifera larvae. After exposed to 1, 2 and 4 μg/mL chlorothalonil or 0.5, 1 and 2 μg/mL acetamiprid for 4 days, larvae were collected and total RNA was extracted. Each sample was assayed 4 times. Expression levels were normalized to actin and then to the gene expression level of the solvent control (SC). Significant differences were set at * for p < 0.05.
Influence of acetamiprid and chlorothalonil on the expression of detoxification related genes
In comparison to the solvent control, the expression of CYP9Q2 was upregulated after expose to 2 μg/mL acetamiprid (Figure 4A). No significant changes of the CYP9Q3 transcript occurred in the treatment of chlorothalonil and acetamiprid (Figure 4B). Exposure to both acetamiprid and chlorothalonil also had no significant effects on the expression of GtpX1 (Figure 4C). In addition, the transcript of GSH was significantly upregulated at 2 μg/mL chlorothalonil, but had no significant difference compared to the control at 4 μg/mL chlorothalonil (Figure 4D).
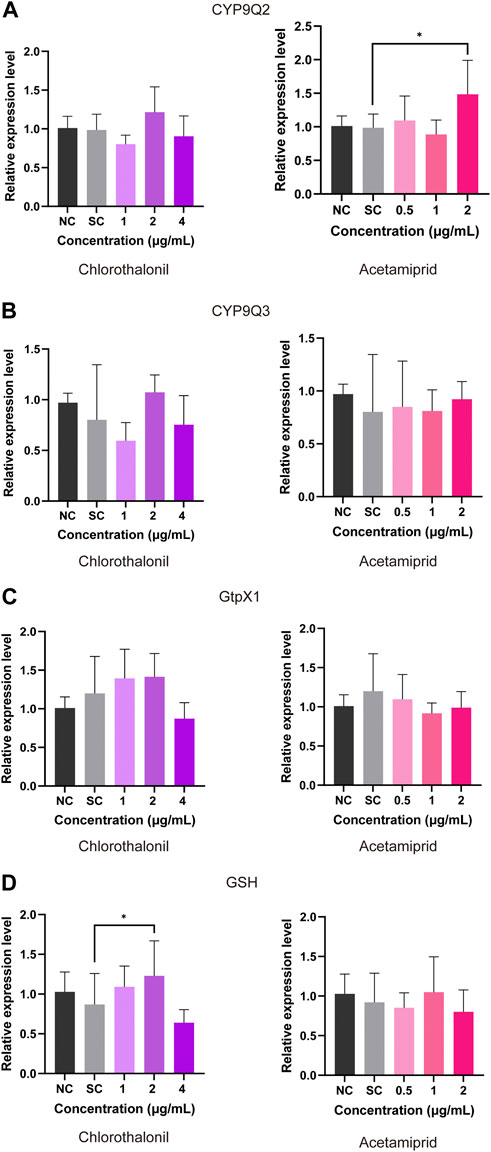
FIGURE 4. Effect of chlorothalonil and acetamiprid on the relative expression levels on CYP9Q2 (A), CYP9Q3 (B), GtpX1 (C) and GSH (D) genes in A. mellifera larvae. After exposed to 1, 2 and 4 μg/mL chlorothalonil or 0.5, 1 and 2 μg/mL acetamiprid for 4 days, larvae were collected and total RNA was extracted. Each sample was assayed 4 times. Expression levels were normalized to actin and then to the gene expression level of the Solvent Control (SC). Significant differences were set at * for p < 0.05.
The effect of chlorothalonil and acetamiprid on detoxification enzymes in larvae
After continuous intake of the diet contained chlorothalonil or acetamiprid, significant variation detoxification enzyme activity was identified in honey bee larvae. As seen in Figure 5A, the activity of P450 was significantly increased in the treatment of 2 μg/mL acetamiprid and 4 μg/mL chlorothalonil. The P450 activity of solvent control was 8.25 ± 0.67 nmol/min/mg pro and increased to 9.50 ± 0.94 nmol/min/mg pro, after exposed to 4 μg/mL chlorothalonil. But the difference of GSTs activity between solvent control and all treatments were not significant (Figure 5B). In addition, acetamiprid and chlorothalonil also had no significant effects on the activity of CarE in honey bee larvae (Figure 5C).
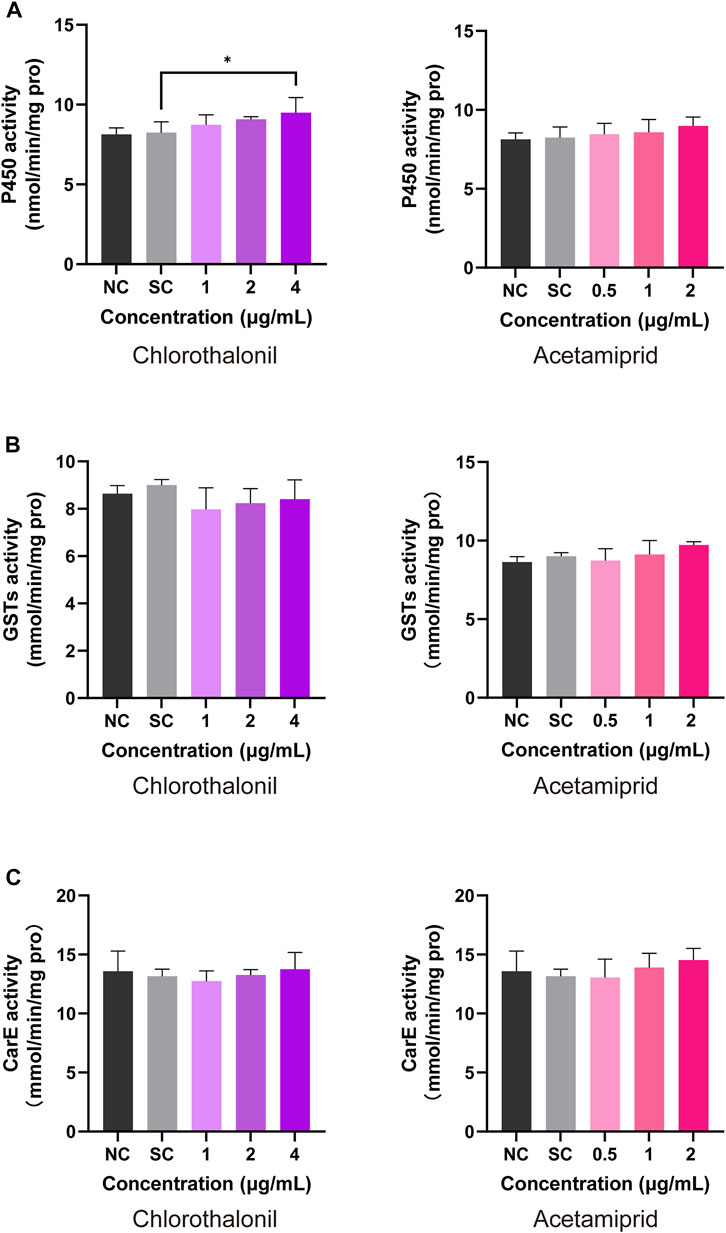
FIGURE 5. Response of honey bee larval P450 (A), GST (B) and CarE (C) activity to chlorothalonil and acetamiprid. After exposed to 1, 2 and 4 μg/mL chlorothalonil or 0.5, 1 and 2 μg/mL acetamiprid for 4 days, larvae were collected. Each sample was assayed 3 times. Significant differences were set at * for p < 0.05.
Discussion
Previous research shows that exposure of honey bees to pesticides during larval stages can cause significant hidden harm to colonies (Davis et al., 1988; Davis, 1989). Broad spectrum fungicides and neonicotinoids are commonly used in agriculture and have been found in bee bread, beeswax and foraged environment (McAfee et al., 2021). Although not acutely toxic to bees, several studies have reported potential sublethal effects of chlorothalonil and acetamiprid, particularly in larvae (Wagner et al., 2021; Wu et al., 2022). In this study, we investigated the chronic toxicity of chlorothalonil and acetamiprid on developing larvae. Further, we tested the gene expression and enzyme activity to further demonstrate whether honey bee larvae were adversely affected in terms of immune and detoxification responses when exposed to the two pesticides at the level where no adverse effects were observed.
The chronic toxicity results indicated that the NOAEC of chlorothalonil and acetamiprid were 4 μg/mL and 2 μg/mL (Figure 1), respectively, which were lower than those reported in previous studies (Dai et al., 2018; Yang et al., 2020). This is not surprising since colonies of the same bee species with different genetic resources may have different tolerances to chemical xenobiotics (Johnson, 2015). In addition, the NOAEC of chlorothalonil and acetamiprid were much lower than the median residual level in pollen and nectar, suggesting that developing bee larvae are posing high risks of exposure to these insecticides (Mullin et al., 2010).
Numerous studies have been suggested that exposure to agricultural products have negative effects on growth, development and worker division of labor in honey bee colonies in honey bee (Ma et al., 2016; Tesovnik et al., 2020; Li et al., 2022). In the present study, we determined the transcriptional levels of Tor (GB44905), AmInR-2 (GB55425), Hr4 (GB17681), Ac3 (GB11637) and ILP-2 (GB10174), which are parts of the Insulin/insulin-like (ILP) and target of rapamycin (TOR) nutrient signaling pathway involved in the regulation of queen/worker caste development in honeybees (Wheeler et al., 2006; de Azevedo and Hartfelder, 2008). We observed that the expressions of the test genes were all upregulated in larvae exposed to chlorothalonil, although the exposure dose was lower than NOAEC. However, these changes were not shown in the acetamiprid-exposed groups treated at concentrations lower than NOAEC in this study (Figure 2). The overexpression of insulin-like growth factor signaling pathway related genes implies the increased nutrient storage. As one of the key regulators in energy metabolism, the insulin/TOR pathway also related to xenobiotics metabolism and stress resistance (Siede et al., 2012; du Rand et al., 2017; Gao et al., 2022). Continuously exposure to organic insecticide could contribute to insulin resistance and metabolic disorders in mammal and insects (de Azevedo and Hartfelder, 2008; Zhang et al., 2021). It has been reported that dietary nicotine stimulated an increase in the synthesis of energy by upregulated two proteins involved in the Insulin/Insulin-like growth factor signaling pathway (IIS) in honey bee larvae (du Rand et al., 2017). The upregulation of genes in this pathway implies that honeybee larvae cope with long-term insecticide exposure by energetically compensating for the adaptive cost of maintaining a highly resistant phenotype. Whether the low doses of chlorothalonil could promote the growth of honey bee larvae need further evidence for validation.
Sublethal concentration of insecticides are known to induce transcriptional changes associated with honeybee immune (Evans et al., 2006; Randolt et al., 2008; Shi et al., 2020). Antimicrobial peptides and protein toll are essential components of the Toll-like receptor pathway, which have been identified in the researches for innate immunity in honey bee (Chan et al., 2009; Danihlík et al., 2015; Horak et al., 2020; Bartling et al., 2021). According to our data, antimicrobial peptides including abaecin (GB18323) and defensin-1 (GB19392) were significantly upregulated in larvae exposed to acetamiprid at NOAEC. Exposure of larvae to NOAEC of chlorothalonil increased the expression of defensin-1 (GB19392) and toll-X4 (GB50418), but not abaecin (GB18323) (Figure 3). Exposure to concentrations below the NOAED did not cause transcriptional changes, reflecting a dose-effect relationship (Figure 3). The antimicrobial peptides (AMPs) response to xenobiotic toxicity or pathogens disease via the rapid expression (Bulet et al., 1999). Defensin-1 (GB19392) is expressed in the salivary glands and is effective against both G+ and G-bacteria, whereas abaecin is less active against most G-bacteria (Klaudiny et al., 2005). The protein toll was originally shown to be a type 1 transmembrane receptor that controls dorsal-ventral patterning and later was identified to be involved in host resistance against pathogens (Jang et al., 2006). The upregulation of these genes can lead to immunity activation for improving the resistance to toxin.
We also assayed the mRNA expression levels and activities of enzymic biomarkers in larvae exposed to different concentrations of chlorothalonil and acetamiprid, respectively. As shown in Figure 4, the expression of three detoxification-related genes (CYP9Q3, GtpX1, GSH) had no significant difference between the acetamiprid-exposed and control groups. In addition, the enzymic activities of P450, GST and CarE was also not influenced by acetamiprid (Figure 5). Our findings were consistent with previous studies where exposure to low doses of acetamiprid had very limited effects on molecular disturbances in worker larvae (Wright et al., 2015; Gao et al., 2020). The most probable explanation is that low dose of acetamiprid can be quickly distributed and detoxified in larval, resulting in relatively low toxicity (Iwasa et al., 2004; Brunet et al., 2005; Yang et al., 2020). Cytochrome P450s is a major detoxification enzyme superfamily involved in metabolic detoxification and resistance of many insecticides (Johnson et al., 2012; Katsavou et al., 2022). CYP9Q2 (GB17793) and CYP9Q3 (GB19967), members in clade CYP9, were most frequently involved in xenobiotic metabolism and evolution of the hormonal and chemosensory processes in A. mellifera and Bombus terrestris (Claudianos et al., 2006; Manjon et al., 2018). In the present study, we found that only CYP9Q2 (GB17793) was upregulated in larvae after exposed to acetamiprid for 4 days, suggesting that honey bees activate the expression of specific isoforms to response to chemical toxicity. When pesticides induced oxidative stress in honey bee, reactive oxygen species (ROS) are generated with P450-mediated detoxification processes. Glutaredoxins act as immediate electron donor and its contribution to providing resistance to oxidative stress during honeybee detoxification processes has been well described (Xu et al., 2013; Yao et al., 2014; Orčić et al., 2022).
The relative expression of glutaredoxin-C4 (GSH) was significantly increased in bees exposed to 2 μg/mL chlorothalonil (Figure 4D). Moreover, the P450 activity was slightly induced by 4 μg/mL chlorothalonil (Figure 5A). Our findings are in line with previous statement that these antioxidant proteins can be temporarily overproduced under low-dose pesticide stress (du Rand et al., 2017; Pal et al., 2022). However, at 4 μg/mL chlorothalonil, the transcript of GSH was not significantly upregulated (Figure 4D). The result implied that the transcript levels of GSH are not different from control, but the GSH cycle has been broken by 4 μg/mL chlorothalonil (Qi et al., 2020).
Conclusion
With the wide use of synthetic organic pesticides, the fungicides and neonicotinoids are posing potential risks to honey bees larvae. By testing the transcriptional levels and activities of major enzymic biomarkers, the risk of chronic exposure of acetamiprid at NOAEC to bee larvae were acceptable. However, the exposure to chlorothalonil, even at concentrations below the NOAEC, had potential effects on honey bee larvae. Thus, the effects of chlorothalonil at no observed adverse effect concentration on colony fitness should be explored in the future, and should be given due consideration for its application in crops pollinated and visited by honey bees.
Data availability statement
The original contributions presented in the study are included in the article/Supplementary Material, further inquiries can be directed to the corresponding authors.
Author contributions
PD and CB conceived this research and designed experiments; YL, TW, BH, BQ, MS, and SY performed experiments and analysis; YL, JG, MS, and QD participated in the interpretation of the data; YL, JG, and PD wrote the paper; PD, CB, JG, and YL participated in the revisions of it. All authors read and approved the final manuscript.
Funding
This study was financially supported by the Beijing Natural Science Foundation (6212004, 6202032), the Agricultural Science and Technology Innovation Program of the Chinese Academy of Agricultural Sciences (CAAS-ASTIP-2021-IAR) and the National Natural Science Foundation of China (31670648).
Acknowledgments
We thank Yujuan Qiu and Pinhong Wang for beekeeping. We thank Lan Zhang (Institute of Plant Protection, Chinese Academy of Agricultural Sciences) for her guidance on the detoxification enzyme test.
Conflict of interest
The authors declare that the research was conducted in the absence of any commercial or financial relationships that could be construed as a potential conflict of interest.
Publisher’s note
All claims expressed in this article are solely those of the authors and do not necessarily represent those of their affiliated organizations, or those of the publisher, the editors and the reviewers. Any product that may be evaluated in this article, or claim that may be made by its manufacturer, is not guaranteed or endorsed by the publisher.
Abbreviations
Ac3, Adenylate cyclase 3; AmInR-2, Apis mellifera insulin-like receptor-like 2; CarE, Carboxylesterase; CYP, Cytochrome P450; CYP9Q2, Cytochrome P450 9Q2; CYP9Q3, Cytochrome P450 9Q3; GSH, Glutaredoxin-C4; GSTs, Glutathione S-transferases; GtpX1, Glutathione peroxidase-like 1; Hr4, Hormone receptor 4; ILP-2, Insulin-like peptide 2; NOAEC, No observed adverse effect concentration; Tor, Target of rapamycin.
References
Bartling M. T., Thümecke S., Russert J. H., Vilcinskas A., Lee K.-Z. (2021). Exposure to low doses of pesticides induces an immune response and the production of nitric oxide in honeybees. Sci. Rep. 11, 6819. doi:10.1038/s41598-021-86293-0
Bridi R., Larena A., Nunez Pizarro P., Giordano A., Montenegro G. (2018). LC-MS/MS analysis of neonicotinoid insecticides: Residue findings in chilean honeys. Cienc. E Agrotecnologia 42, 51–57. doi:10.1590/1413-70542018421021117
Brunet J. L., Badiou A., Belzunces L. P. (2005). In vivo metabolic fate of [14C]-acetamiprid in six biological compartments of the honeybee, Apis mellifera L. Pest. Manag. Sci. 61, 742–748. doi:10.1002/ps.1046
Bulet P., Hetru C., Dimarcq J.-L., Hoffmann D. (1999). Antimicrobial peptides in insects structure and function. Dev. Comp. Immunol. 23, 329–344. doi:10.1016/s0145-305x(99)00015-4
Chan Q. W. T., Melathopoulos A. P., Pernal S. F., Foster L. J. (2009). The innate immune and systemic response in honey bees to a bacterial pathogen, Paenibacillus larvae. BMC Genomics 10, 387. doi:10.1186/1471-2164-10-387
Chen D., Liu X., Luo L., Huang X., Lu S., Li G., et al. (2020). Plant secondary metabolites of four rangeland plant species and their effects on the activities of detoxification enzymes of Oedaleus asiaticus in a steppe grassland. Acta Prataculturae Sin. 29, 183–192. doi:10.11686/cyxb2019128
Claudianos C., Ranson H., Johnson R. M., Biswas S., Schuler M. A., Berenbaum M. R., et al. (2006). A deficit of detoxification enzymes: Pesticide sensitivity and environmental response in the honeybee. Insect Mol. Biol. 15, 615–636. doi:10.1111/j.1365-2583.2006.00672.x
Dai P., Jack C. J., Mortensen A. N., Bloomquist J. R., Ellis J. D. (2018). The impacts of chlorothalonil and diflubenzuron on Apis mellifera L. larvae reared in vitro. Ecotoxicol. Environ. Saf. 164, 283–288. doi:10.1016/j.ecoenv.2018.08.039
Danihlík J., Aronstein K., Petřivalský M. (2015). Antimicrobial peptides: A key component of honey bee innate immunity: Physiology, biochemistry, and chemical ecology. J. Apic. Res. 54, 123–136. doi:10.1080/00218839.2015.1109919
Davis A. R., Solomon K. R., Shuel R. W. (1988). Laboratory studies of honeybee larval growth and development as affected by systemic insecticides at adult-sublethal levels. J. Apic. Res. 27, 146–161. doi:10.1080/00218839.1988.11100795
Davis A. R. (1989). The study of insecticide poisoning of honeybee brood. Bee World 70, 163–174. doi:10.1080/0005772x.1989.11099013
De Azevedo S. V., Hartfelder K. (2008). The insulin signaling pathway in honey bee (Apis mellifera) caste development - differential expression of insulin-like peptides and insulin receptors in queen and worker larvae. J. Insect Physiol. 54, 1064–1071. doi:10.1016/j.jinsphys.2008.04.009
Desneux N., Decourtye A., Delpuech J. M. (2007). The sublethal effects of pesticides on beneficial arthropods. Annu. Rev. Entomol. 52, 81–106. doi:10.1146/annurev.ento.52.110405.091440
Dolezal A. G., Carrillo-Tripp J., Judd T. M., Allen Miller W., Bonning B. C., Toth A. L. (2019). Interacting stressors matter: Diet quality and virus infection in honeybee health. R. Soc. Open Sci. 6, 181803. doi:10.1098/rsos.181803
Doublet V., Labarussias M., De Miranda J. R., Moritz R. F. A., Paxton R. J. (2015). Bees under stress: Sublethal doses of a neonicotinoid pesticide and pathogens interact to elevate honey bee mortality across the life cycle. Environ. Microbiol. 17, 969–983. doi:10.1111/1462-2920.12426
Du Rand E. E., Human H., Smit S., Beukes M., Apostolides Z., Nicolson S. W., et al. (2017). Proteomic and metabolomic analysis reveals rapid and extensive nicotine detoxification ability in honey bee larvae. Insect Biochem. Mol. Biol. 82, 41–51. doi:10.1016/j.ibmb.2017.01.011
El-Seedi H. R., Ahmed H. R., El-Wahed A. a. A., Saeed A., Algethami A. F., Attia N. F., et al. (2022). Bee stressors from an immunological perspective and strategies to improve bee health. Veterinary Sci. 9, 199. doi:10.3390/vetsci9050199
Evans J. D., Aronstein K., Chen Y. P., Hetru C., Imler J. L., Jiang H., et al. (2006). Immune pathways and defence mechanisms in honey bees Apis mellifera. Insect Mol. Biol. 15, 645–656. doi:10.1111/j.1365-2583.2006.00682.x
Gao J., Jin S.-S., He Y., Luo J.-H., Xu C.-Q., Wu Y.-Y., et al. (2020). Physiological analysis and transcriptome analysis of asian honey bee (Apis cerana cerana) in response to sublethal neonicotinoid imidacloprid. Insects (Basel, Switz. 11, 753. doi:10.3390/insects11110753
Gao J., Yang Y., Ma S., Liu F., Wang Q., Wang X., et al. (2022). Combined transcriptome and metabolite profiling analyses provide insights into the chronic toxicity of carbaryl and acetamiprid to Apis mellifera larvae. Sci. Rep. 12, 16898. doi:10.1038/s41598-022-21403-0
Grassl J., Holt S., Cremen N., Peso M., Hahne D., Baer B. (2018). Synergistic effects of pathogen and pesticide exposure on honey bee (Apis mellifera) survival and immunity. J. Invertebr. Pathology 159, 78–86. doi:10.1016/j.jip.2018.10.005
Horak R. D., Leonard S. P., Moran N. A. (2020). Symbionts shape host innate immunity in honeybees. Proc. Biol. Sci. 287, 20201184. doi:10.1098/rspb.2020.1184
Human H., Archer C. R., Du Rand E. E., Pirk C. W. W., Nicolson S. W. (2014). Resistance of developing honeybee larvae during chronic exposure to dietary nicotine. J. Insect Physiology 69, 74–79. doi:10.1016/j.jinsphys.2014.03.012
Iwasa T., Motoyama N., Ambrose J. T., Roe R. M. (2004). Mechanism for the differential toxicity of neonicotinoid insecticides in the honey bee, Apis mellifera. Crop Prot. 23, 371–378. doi:10.1016/j.cropro.2003.08.018
Jang I. H., Chosa N., Kim S. H., Nam H. J., Lemaitre B., Ochiai M., et al. (2006). A Spatzle-processing enzyme required for toll signaling activation in Drosophila innate immunity. Dev. Cell. 10, 45–55. doi:10.1016/j.devcel.2005.11.013
Johnson R. M. (2015). Honey bee toxicology. Annu. Rev. Entomol. 60, 415–434. doi:10.1146/annurev-ento-011613-162005
Johnson R. M., Mao W., Pollock H. S., Niu G., Schuler M. A., Berenbaum M. R. (2012). Ecologically appropriate xenobiotics induce cytochrome P450s in Apis mellifera. PLoS One 7, e31051. doi:10.1371/journal.pone.0031051
Katsavou E., Riga M., Ioannidis P., King R., Zimmer C. T., Vontas J. (2022). Functionally characterized arthropod pest and pollinator cytochrome P450s associated with xenobiotic metabolism. Pestic. Biochem. Physiol. 181, 105005. doi:10.1016/j.pestbp.2021.105005
Klaudiny J., Albert Š., Bachanová K., Kopernický J., Šimúth J. (2005). Two structurally different defensin genes, one of them encoding a novel defensin isoform, are expressed in honeybee Apis mellifera. Insect Biochem. Mol. Biol. 35, 11–22. doi:10.1016/j.ibmb.2004.09.007
Li B., Ke L., Li A.-R., Diao Q.-Y., Wang Q., Liu Y.-J. (2022). Exposure of larvae to sublethal thiacloprid delays bee development and affects transcriptional responses of newly emerged honey bees. Front. Insect Sci. 2. doi:10.3389/finsc.2022.844957
Ma L., Wang Y., Zhang W., Wang H., Liu Z., Xu B. (2016). Alterations in protein and amino acid metabolism in honeybees (Apis mellifera) fed different l -leucine diets during the larval stage. J. Asia-Pacific Entomology 19, 769–774. doi:10.1016/j.aspen.2016.07.005
Manjon C., Troczka B. J., Zaworra M., Beadle K., Randall E., Hertlein G., et al. (2018). Unravelling the molecular determinants of bee sensitivity to neonicotinoid insecticides. Curr. Biol. 28, 1137–1143. doi:10.1016/j.cub.2018.02.045
Mcafee A., Milone J. P., Metz B., Mcdermott E., Foster L. J., Tarpy D. R. (2021). Honey bee queen health is unaffected by contact exposure to pesticides commonly found in beeswax. Sci. Rep. 11, 15151. doi:10.1038/s41598-021-94554-1
Medici S. K., Castro A., Sarlo E. G., Marioli J. M., Eguaras M. J. (2012). The concentration effect of selected acaricides present in beeswax foundation on the survival of Apis mellifera colonies. J. Apic. Res. 51, 164–168. doi:10.3896/ibra.1.51.2.03
Mullin C. A., Frazier M., Frazier J. L., Ashcraft S., Simonds R., Vanengelsdorp D., et al. (2010). High levels of miticides and agrochemicals in north American apiaries: Implications for honey bee health. Plos One 5, e9754. doi:10.1371/journal.pone.0009754
Myers S. S., Smith M. R., Guth S., Golden C. D., Vaitla B., Mueller N. D., et al. (2017). “Climate change and global food systems: Potential impacts on food security and undernutrition,”. Annual review of public health. Editor J. E. Fielding, Vol. 38, 259–277.
O'neal S. T., Reeves A. M., Fell R. D., Brewster C. C., Anderson T. D. (2019). Chlorothalonil exposure alters virus susceptibility and markers of immunity, nutrition, and development in honey bees. J. Insect Sci. 19, 14. doi:10.1093/jisesa/iez051
Orčić S. M., Čelić T. V., Purać J. S., Vukašinović E. L., Kojić D. K. (2022). Acute toxicity of sublethal concentrations of thiacloprid and clothianidin to immune response and oxidative status of honey bees. Apidologie 53, 50. doi:10.1007/s13592-022-00959-w
Pal E., Almasri H., Paris L., Diogon M., Pioz M., Cousin M., et al. (2022). Toxicity of the pesticides imidacloprid, difenoconazole and glyphosate alone and in binary and ternary mixtures to winter honey bees: Effects on survival and antioxidative defenses. Toxics 10, 104. doi:10.3390/toxics10030104
Pareja L., Colazzo M., Perez-Parada A., Niell S., Carrasco-Letelier L., Besil N., et al. (2011). Detection of pesticides in active and depopulated beehives in Uruguay. Int. J. Environ. Res. Public Health 8, 3844–3858. doi:10.3390/ijerph8103844
Prezenska M., Sobekova A., Sabova L. (2019). Antioxidant enzymes of honeybee larvae exposed to oxamyl. Folia Veterinaria 63, 9–14. doi:10.2478/fv-2019-0032
Qi S., Niu X., Wang D. H., Wang C., Zhu L., Xue X., et al. (2020). Flumethrin at sublethal concentrations induces stresses in adult honey bees (Apis mellifera L.). Sci. Total Environ. 700, 134500. doi:10.1016/j.scitotenv.2019.134500
Quintana S., Brasesco C., Negri P., Marin M., Pagnuco I., Szawarski N., et al. (2019). Up-regulated pathways in response to deformed wing virus infection in Apis mellifera (hymenoptera: Apidae). Rev. De. La Soc. Entomol. Argent. 78, 1–11. doi:10.25085/rsea.780101
Randolt K., Gimple O., Geissendörfer J., Reinders J., Prusko C., Mueller M. J., et al. (2008). Immune-related proteins induced in the hemolymph after aseptic and septic injury differ in honey bee worker larvae and adults. Arch. Insect Biochem. Physiol. 69, 155–167. doi:10.1002/arch.20269
Rosa A. D. S., Teixeira J. S. G., Vollet-Neto A., Queiroz E. P., Blochtein B., Pires C. S. S., et al. (2016). Consumption of the neonicotinoid thiamethoxam during the larval stage affects the survival and development of the stingless bee, Scaptotrigona aff. depilis. Apidologie 47, 729–738. doi:10.1007/s13592-015-0424-4
Schultzhaus J. N., Saleem S., Iftikhar H., Carney G. E. (2017). The role of the Drosophila lateral horn in olfactory information processing and behavioral response. J. Insect Physiology 98, 29–37. doi:10.1016/j.jinsphys.2016.11.007
Shi J., Liao C., Wang Z., Zeng Z., Wu X. (2019). Effects of sublethal acetamiprid doses on the lifespan and memory-related characteristics of honey bee (Apis mellifera) workers. Apidologie 50, 553–563. doi:10.1007/s13592-019-00669-w
Shi J., Zhang R., Pei Y., Liao C., Wu X. (2020). Exposure to acetamiprid influences the development and survival ability of worker bees (Apis mellifera L.) from larvae to adults. Environ. Pollut. 266, 115345. doi:10.1016/j.envpol.2020.115345
Siede R., Meixner M. D., Buechler R. (2012). Comparison of transcriptional changes of immune genes to experimental challenge in the honey bee (Apis mellifera). J. Apic. Res. 51, 320–328. doi:10.3896/ibra.1.51.4.05
Tan K., Chen W., Dong S., Liu X., Wang Y., Nieh J. C. (2015). A neonicotinoid impairs olfactory learning in Asian honey bees (Apis cerana) exposed as larvae or as adults. Sci. Rep. 5, 10989. doi:10.1038/srep10989
Tan K., Wang C., Dong S., Li X., Nieh J. C. (2017). The pesticide flupyradifurone impairs olfactory learning in Asian honey bees (Apis cerana) exposed as larvae or as adults. Sci. Rep. 7, 17772. doi:10.1038/s41598-017-18060-z
Tarek H., Hamiduzzaman M. M., Morfin N., Guzman-Novoa E. (2018). Research Article Sub-lethal doses of neonicotinoid and carbamate insecticides reduce the lifespan and alter the expression of immune health and detoxification related genes of honey bees (Apis mellifera). Genet. Mol. Res. 17. doi:10.4238/gmr16039908
Tavares D. A., Roat T. C., Silva-Zacarin E. C. M., Nocelli R. C. F., Malaspina O. (2019). Exposure to thiamethoxam during the larval phase affects synapsin levels in the brain of the honey bee. Ecotoxicol. Environ. Saf. 169, 523–528. doi:10.1016/j.ecoenv.2018.11.048
Tesovnik T., Zorc M., Ristanic M., Glavinic U., Stevanovic J., Narat M., et al. (2020). Exposure of honey bee larvae to thiamethoxam and its interaction with Nosema ceranae infection in adult honey bees. Environ. Pollut. 256, 113443. doi:10.1016/j.envpol.2019.113443
Traynor K. S., Mondet F., De Miranda J. R., Techer M., Kowallik V., Oddie M. a. Y., et al. (2020). Varroa destructor: A complex parasite, crippling honey bees worldwide. Trends Parasitol. 36, 592–606. doi:10.1016/j.pt.2020.04.004
Wagner D. L., Grames E. M., Forister M. L., Berenbaum M. R., Stopak D. (2021). Insect decline in the Anthropocene: Death by a thousand cuts. Proc. Natl. Acad. Sci. U. S. A. 118, e2023989118. doi:10.1073/pnas.2023989118
Wang K., Pang Q., Zhang W., Ji T. (2017). Effects of sublethal doses of carbendazim on the growth and detoxifying enzyme activities of honeybee (Apis mellifera ligustica) larvae. Acta Entomol. Sin. 60, 642–649. doi:10.16380/j.kcxb.2017.06.004
Wang X., Goulson D., Chen L., Zhang J., Zhao W., Jin Y., et al. (2020). Occurrence of neonicotinoids in Chinese apiculture and a corresponding risk exposure assessment. Environ. Sci. Technol. 54, 5021–5030. doi:10.1021/acs.est.9b07162
Wang Y., Jin C., Wang D., Zhou J., Yang G., Shao K., et al. (2021). Effects of chlorothalonil, prochloraz and the combination on intestinal barrier function and glucolipid metabolism in the liver of mice. J. Hazard. Mater. 410, 124639. doi:10.1016/j.jhazmat.2020.124639
Wen J., Zhi J., Zeng G. (2017). Variance in enzymes activities of frankliniella occidentalis(pergande) feeding on tomato plant damaged by tetranychus urticae koch. J. Henan Agric. Sci. 46, 79–82. 87. doi:10.15933/j.cnki.1004-3268.2017.09.014
Wheeler D. E., Buck N., Evans J. D. (2006). Expression of insulin pathway genes during the period of caste determination in the honey bee, Apis mellifera. Insect Mol. Biol. 15, 597–602. doi:10.1111/j.1365-2583.2006.00681.x
Wright G. A., Softley S., Earnshaw H. (2015). Low doses of neonicotinoid pesticides in food rewards impair short-term olfactory memory in foraging-age honeybees. Sci. Rep. 5, 15322. doi:10.1038/srep15322
Wu J. Y., Anelli C. M., Sheppard W. S. (2011). Sub-lethal effects of pesticide residues in brood comb on worker honey bee (Apis mellifera) development and longevity. PLoS One 6, e14720. doi:10.1371/journal.pone.0014720
Wu T., Han B., Wang X., Tong Y., Liu F., Diao Q., et al. (2022). Chlorothalonil alters the gut microbiota and reduces the survival of immature honey bees reared in vitro. Pest Manag. Sci. 78, 1976–1981. doi:10.1002/ps.6816
Xu J., Strange J. P., Welker D. L., James R. R. (2013). Detoxification and stress response genes expressed in a Western North American bumble bee, Bombus huntii (Hymenoptera: Apidae). BMC Genomics 14, 874. doi:10.1186/1471-2164-14-874
Yang Y., Ma S., Liu F., Wang Q., Wang X., Hou C., et al. (2020). Acute and chronic toxicity of acetamiprid, carbaryl, cypermethrin and deltamethrin to Apis mellifera larvae reared in vitro. Pest Manag. Sci. 76, 978–985. doi:10.1002/ps.5606
Yao P., Chen X., Yan Y., Liu F., Zhang Y., Guo X., et al. (2014). Glutaredoxin 1, glutaredoxin 2, thioredoxin 1, and thioredoxin peroxidase 3 play important roles in antioxidant defense in Apis cerana cerana. Free Radic. Biol. Med. 68, 335–346. doi:10.1016/j.freeradbiomed.2013.12.020
Yu L., Yang H., Cheng F., Wu Z., Huang Q., He X., et al. (2021). Honey bee Apis mellifera larvae gut microbial and immune, detoxication responses towards flumethrin stress. Environ. Pollut. 290, 118107. doi:10.1016/j.envpol.2021.118107
Zhang C., Seong K. M., Sun W., Mittapalli O., Qiu B., Clark J. M., et al. (2021). The insulin signaling pathway in Drosophila melanogaster: A nexus revealing an “achilles' heel” in ddt resistance. Pestic. Biochem. Physiol. 171, 104727. doi:10.1016/j.pestbp.2020.104727
Keywords: honey bees, chlorothalonil, acetamiprid, toxicity, oxidative stress
Citation: Lu Y, Gao J, Wu T, Han B, Qian B, Shi M, Yang S, Diao Q, Bu C and Dai P (2023) Exposure of chlorothalonil and acetamiprid reduce the survival and cause multiple internal disturbances in Apis mellifera larvae reared in vitro. Front. Physiol. 14:1114403. doi: 10.3389/fphys.2023.1114403
Received: 02 December 2022; Accepted: 02 February 2023;
Published: 13 February 2023.
Edited by:
Rui Guo, Fujian Agriculture and Forestry University, ChinaCopyright © 2023 Lu, Gao, Wu, Han, Qian, Shi, Yang, Diao, Bu and Dai. This is an open-access article distributed under the terms of the Creative Commons Attribution License (CC BY). The use, distribution or reproduction in other forums is permitted, provided the original author(s) and the copyright owner(s) are credited and that the original publication in this journal is cited, in accordance with accepted academic practice. No use, distribution or reproduction is permitted which does not comply with these terms.
*Correspondence: Chunya Bu, YnVjaHVueWFAMTYzLmNvbQ==; Pingli Dai, ZGFpcGluZ2xpQGNhYXMuY24=
†These authors have contributed equally to this work and share first authorship