- 1Department of Acupuncture-Moxibustion, LongHua Hospital Shanghai University of Traditional Chinese Medicine, Shanghai University of Traditional Chinese Medicine, Shanghai, China
- 2Key Laboratory of Acupuncture and Immunological Effects, Shanghai University of Traditional Chinese Medicine, Shanghai, China
- 3Shanghai Research Institute of Acupuncture and Meridian, Shanghai, China
Fibrosis can occur in many organs, and severe cases leading to organ failure and death. No specific treatment for fibrosis so far. In recent years, microRNA-34a (miR-34a) has been found to play a role in fibrotic diseases. MiR-34a is involved in the apoptosis, autophagy and cellular senescence, also regulates TGF-β1/Smad signal pathway, and negatively regulates the expression of multiple target genes to affect the deposition of extracellular matrix and regulate the process of fibrosis. Some studies have explored the efficacy of miR-34a-targeted therapies for fibrotic diseases. Therefore, miR-34a has specific potential for the treatment of fibrosis. This article reviews the important roles of miR-34a in fibrosis and provides the possibility for miR-34a as a novel therapeutic target in fibrosis.
Introduction
Fibrosis (FB) is an excessive repair reaction of the body to external injury, resulting in structural damage and dysfunction of normal tissues and organs, which affects the patients’ physical and mental health and quality of life seriously (Jun and Lau, 2018; Henderson et al., 2020). It is a high-burden diseases, and the annualized incidence of major fibrosis-related conditions is nearly 1/20 (Tsou et al., 2014; Zhao et al., 2020). At present, the treatment methods are limited. In the early stage, drug therapy merely alleviate inflammation and symptoms; in the late stage, only surgery or organ transplantation can be selected. However, the cure rate is still low and the recurrence rate is high (Rieder et al., 2012; Villac Adde et al., 2018; Ramos et al., 2019; Cai et al., 2020). Some researches has investigated a variety of regulator (such as microRNA, TGF-β, interleukins, IFN-γ) for the treatment of FB, which only a certain efficacy (Ghosh et al., 2013; Richeldi et al., 2017; Gieseck et al., 2018; Weiskirchen et al., 2019). As the signal transduction network of FB is complex, the current researches on therapeutic targets is not sufficient to support the clinical practice of FB. We need to further clarify the specific function of various signal molecules in fibrosis to guide the clinical therapy.
Recently, many studies have found that microRNA-34a (miR-34a) plays a role in a variety of fibrotic diseases by regulating cell proliferation, differentiation, apoptosis and other processes (Chen and Hu, 2012; Alivernini et al., 2014; Zhou et al., 2017; Li et al., 2018) (Table 1). It has been found that miR-34a can regulate the extracellular matrix (ECM) deposition by acting on the processes of apoptosis, senescence and autophagy in epithelial/endothelial cells and fibroblasts (Tian et al., 2016; Cui et al., 2017a; Zhu et al., 2019), and also promote transforming growth factor-β1 (TGF-β1)-induced fibroblasts activation by targeting Smad4 (Huang et al., 2014; Qi et al., 2020); while the miR-34a inhibitor can improve collagen deposition and attenuate fibrosis by regulating cell apoptosis and differentiation through Bcl-2, TGF-β1, and PPAR—γ(Zhou et al., 2014; Li et al., 2015; Song et al., 2019).
According to current researches, miR-34a may be exploited as a potential target for anti-fibrosis therapy in the future. In this paper, we review studies on the involvement of miR-34a in fibrotic diseases in order to reveal the possible mechanism of miR-34a as a therapeutic target for FB.
Role of MicroRNA-34a in Various Molecular Pathways of Fibrosis
MicroRNA are a class of small non-coding RNA containing about 18–22 nucleotides that regulate gene expression at the post-transcriptional level through completely or partially complementary base binding to their target mRNAs (Tang et al., 2015). MiR-34a is a member of miRNA family, which is widely expressed in mammals (Hermeking, 2010). It has been found that miR-34a affects the occurrence and development of fibrotic diseases by regulating cell activities, including apoptosis, autophagy, cellular senescence, the expression of related target genes and TGF-β1/Smad signaling pathway (Figure 1).
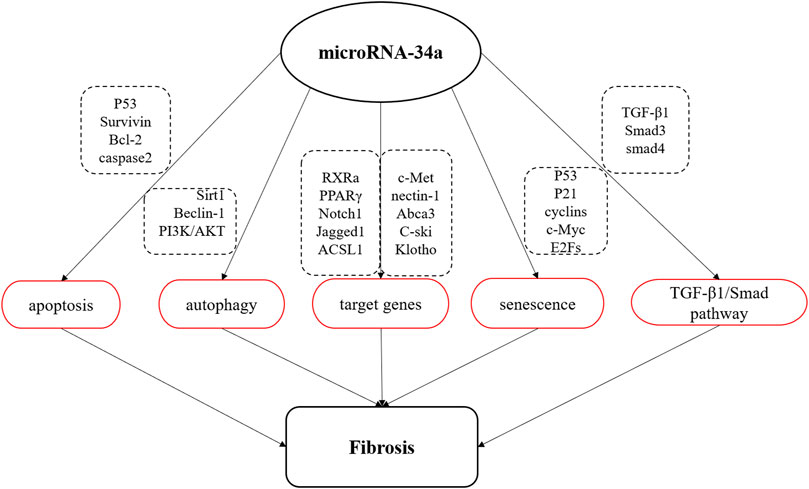
FIGURE 1. Schematic diagram of miR-34a involved in fibrosis process. MiR-34a is involved in the apoptosis, autophagy and senescence, regulates TGF-β1/Smad signal pathway, and negatively regulates the expression of multiple target genes to affect the process of organ fibrosis.
Role of MicroRNA-34a in Apoptosis
Apoptosis is a process of programmed cell death in multicellular organisms, which plays an important role in the development of fibrosis (Zhang et al., 2001; Docherty et al., 2006). After injury, cell apoptosis induce the recruitment of immune cells with amplification of inflammatory response and profibrogenic factors, enhance fibroblast proliferation, and then promotes the regeneration of granulation tissue, which eventually leads to the development of fibrotic lesions (Uhal, 2002; Bhandary et al., 2012; Jun and Lau, 2018). MiR-34a has been found to play an important role in the process of fibrosis by regulating apoptosis.
MiR-34a is the direct transcription target of p53, and can negatively regulate sirtuin 1(SIRT1), resulting in increased p53 acetylation. P53 and SIRT1 are typical genes involved in apoptosis regulation (Yamakuchi et al., 2008; Li et al., 2011), and p53 is a major contributor to the onset and progression of fibrotic diseases (Yang et al., 2010; Sutton et al., 2013; Overstreet et al., 2014; Valentijn et al., 2021; Fu et al., 2022; Li et al., 2022). Therefore, the miR-34a SIRT1/p53 signaling pathway forms a positive feedback loop that has a vital role in cell proliferation and apoptosis (Tarasov et al., 2007; Kumamoto et al., 2008; Kim et al., 2015). It was found that the expression level of miR-34a was positively correlated with the severity of liver injury (Castro et al., 2013). In liver tissue of rats with hepatic fibrosis, it has been observed that miR-34a and acetyl-p53 were up-regulated and SIRT1 was down-regulated; nevertheless, SIRT1 activator significantly reduced the levels of miR-34a and acetyl-p53, and inhibited fibrosis, which suggested that miR-34a/SIRT1/p53 signaling pathway was activated in fibrosis; in vitro, it was further confirmed that miR-34a/SIRT1/p53 signaling pathway was activated in epithelial cells to induce apoptosis, which activate hepatic stellate cells (HSCs) and accelerate the process of liver fibrosis (Tian et al., 2016). In addition, in the lung tissues of patients and mice with pulmonary fibrosis, the apoptosis levels of alveolar epithelial cells (AECs) were increased, the expression of acetyl-p53, PAI-1, and miR-34a was increased, and the expression of SIRT1 was decreased; however, the above process could be reversed by knockout of the miR-34a gene (Shetty et al., 2017). It can be seen that miR-34a/SIRT1/p53 is also involved in the apoptosis of pulmonary epithelial cells and the induction of pulmonary fibrosis.
Bcl-2 is an important antiapoptosis gene and one of the target genes of miR-34a. MiR-34a can promote apoptosis by inhibiting bcl-2 expression (Bommer et al., 2007). Tubular epithelial cells apoptosis is one of the mechanisms of tubular atrophy and tubulointerstitial fibrosis (Docherty et al., 2006). In the study of rats and mice with renal interstitial fibrosis, miR-34a was released from mesenchymal fibroblasts and transferred to proximal tubular epithelial cells, where it promoted apoptosis of renal tubular epithelial cells by inhibiting the transcription and translation of Bcl-2, further aggravating renal interstitial fibrosis (Zhou et al., 2014; Li et al., 2019).
Furthermore, caspase-2 is also the target gene of miR-34a, which helps to enhance apoptosis and plays a role in cell remodeling and tissue repair (Madesh et al., 2009). In the study of alcoholic liver disease, miR-34a was found to regulate apoptosis of hepatocytes and intrahepatic biliary epithelial cells by targeting caspase 2, affecting cell survival and migration, and regulating the release of matrix metalloproteinases (MMPs). Therefore, miR-34a plays a role in the repair of liver injury and liver fibrosis (Meng et al., 2012). The above results indicate that miR-34a participates in organ fibrosis by regulating apoptosis-related signal molecules.
Role of MicroRNA-34a in Autophagy
Autophagy is a conserved lysosomal degradation process in eukaryotic cells that plays an important role in maintaining homeostasis in cells and tissues. Autophagy disorders participate in the development of organ fibrosis. It has been confirmed that autophagy promote the clearance of damaged proteins and organelles, and accelerate the degradation of extracellular matrix proteins (Ding and Choi, 2014; Lv et al., 2017; Jesus et al., 2019); in addition, intracellular autophagy flux can increases the energy needed for extracellular matrix protein formation (Kota et al., 2017). Some studies have found that autophagy mediates fibrotic diseases regulated by miR-34a.
A study of epidural scar hyperplasia after laminectomy has found that the expression of miR-34a and autophagy-related molecules (beclin-1, ATG5, LC3B-2/1, p53) were changed, which suggests that the disorder of miR-34a and autophagy level may be involved in the formation of fibrosis (Wang B. B. et al., 2017). The PI3K/Akt signaling pathway is a classical autophagy regulatory pathway involved in the regulation of cell proliferation, migration and differentiation (Zundler et al., 2016; Aoki and Fujishita, 2017; Shi et al., 2017). This signaling pathway is concerned in the study of myocardial fibrosis. In the rat model of myocardial fibrosis induced by thyroid hormone, miR-34a expression and PI3K and Akt proteins were found to be upregulated, while autophagy related proteins (ATG5, Atg7, Atg16L1, Beclin1, LC3A) were significantly downregulated, and MMPs/TIMPs ratios appeared imbalance. This study suggested that myocardial fibrosis might be related to miR-34a-mediated regulation of the PI3K/Akt signaling pathway and inhibition of autophagy (Liu et al., 2018).
In addition, miR-34a indirectly interferes with the extension of autolysosomes by inhibiting SIRT1(Yang et al., 2013). SIRT1 is not only a molecule involved in autophagy activation, but also an important component of the EMT, which plays an important role in the process of organ fibrosis (Salminen and Kaarniranta, 2009; Simic et al., 2013). It has been found that miR-34a-5p is up-regulated accompanied by the corresponding down-regulation of SIRT1 in the renal tissue of mice with diabetic nephropathy. MiR-34a-5p was positively correlated with the expression of fibronectin (FN), type I collagen (COL 1), and TGF-β1; then the cell experiments further identified that miR-34a-5p directly suppressed SIRT1 to increase the profibrogenic effects of TGF-β1 by targeting the 3′-UTR of SIRT1; it has also been found that miR-34a-5p inhibitor increases the expression of SIRT1 and decreases the level of TGF-β1, FN, and COL 1, then a small interfering RNA (siRNA) targeting SIRT1 enhanced the expression of TGF-β1 and FB-related genes, indicating that miR-34a-5p could promote renal fibrosis by inhibiting SIRT1 (Xue et al., 2018). In diabetic cardiomyopathy, miR-34a was also found to aggravate myocardial injury related to inhibition of SIRT1 transcription (Zhu et al., 2019). According to the current research, we found that miR-34a is involved in the fibrosis process by inhibiting autophagy-related molecules. Unfortunately, there is insufficient evidence to explore the role of miR-34a in fibrosis by regulating autophagy at present, further research is needed to fill in this theory in the future.
Role of MicroRNA-34a in Cellular Senescence
Cellular senescence is a process in which cells undergo irreversible cell cycle arrest and is considered to play a key role in damage repair. Fibroblast senescence is one of the important factors of fibrosis pathology (Waters et al., 2018). It has been found that fibroblasts derived from fibrotic tissue have a variety of senescence-related characteristics. Myofibroblasts senescence stop synthesizing collagen and other ECM proteins, and secrete ECM protein-degrading enzymes to improve matrix deposition and limit the accumulation of fibrotic tissue (Harding et al., 2005; Krizhanovsky et al., 2008; Jun and Lau, 2010; Álvarez et al., 2017). Besides, epithelial cells senescence indirectly promotes the differentiation of fibroblasts into myofibroblasts, resulting in the excessive deposition of collage (Lehmann et al., 2017).
As a downstream transcription target of p53, a cell cycle regulator, miR-34a is closely related to cell senescence (Kyle et al., 2009; Harries, 2014). It has been proved that miR-34a can regulate cell cycle and senescence by targeting multiple genes, such as SIRT1, cyclin E2, cyclin D1, and E2F3 (Cui et al., 2017a). AECs are the main senescent cells of pulmonary fibrosis. In the lung tissues and purified AECs of patients with idiopathic pulmonary FB (IPF), the relative levels of miR-34a, miR-34b and miR-34c were significantly increased, the activity of p16, p21, p53, and SA-β-gal was increased, and the expression of miR-34 targets (E2F1, c-myc, and CCNE2) was downregulated, these changes stimulated the senescence of AECs, promoted myofibroblast transdifferentiation and induced IPF (Disayabutr et al., 2016; Cui et al., 2017b). In the study of hepatic fibrosis, the same results were obtained. MiR-34a was up-regulated in the patients with hepatic fibrosis, which promoting the senescence of hepatocytes and inducing hepatic fibrosis by reducing the senescence of HSCs; however, miR-34a inhibitor (morpholino) obstructed this process and improved hepatic fibrosis, which indicating that miR-34a plays a role in promoting hepatocytes senescence and reducing HSCs senescence (Wan et al., 2017). Not only can miR-34a regulates epithelial cell senescence and induce fibroblast to differentiate into myofibroblast, but also inhibits fibroblast senescence, promotes fibroblast proliferation, and aggravates the fibrosis process. Therefore, cell senescence plays an important role in the process of miR-34a participating in fibrosis.
Regulation MicroRNA-34a on Typical Target Genes
MiRNA-34a regulates growth, differentiation and metabolism by negatively regulating typical target genes. Previous studies have revealed that miR-34a can combined with multiple target genes to regulate fibrosis in many ways.
ACSL1 is a member of Acyl-CoA synthetase long-chain (ACSL) family. ACSL1 is an important gene in liver lipid metabolism. The luciferase reporter assay confirmed that ACSL1 was the target gene of miR-34a (Li et al., 2011). In the research of hepatic fibrosis, miR-34a specifically bound to the 3′-UTR of ACSL1, which negatively regulated the expression of ACSL1 mRNA and protein, promoted the activation and proliferation of HSCs, and lead to upregulation of ECM-related indicators (COL 1, a-SMA); in contrast, silencing of the miR-34a gene increased the expression of ACSL1, decreased the expression of ECM-related proteins, and affected HSCs activation (Yan et al., 2015; Yan, 2016). Thus, ACSL1 is one of the factors by which miR-34a promotes hepatic fibrosis.
Protooncogene c-ski, a transcriptional corepressor, is a negative regulator of TGF-β/Smad signaling (Cunnington et al., 2009), and can inhibit TGF-β1-induced activation of cardiac fibroblasts and ECM deposition (Wang J. et al., 2017). In vitro and in vivo studies on myocardial fibrosis in rats, it was found that miR-34a could target and inhibit the expression of c-ski, and the levels of collagen I and a—SMA were significantly increased; Inhibition of miR-34a significantly increased the expression of c-ski protein and decreased the levels of COL one and a-SMA protein (Zhang et al., 2018). It can be seen c-ski mediates miR-34a to promote the proliferation and ECM deposition of TGF-β1-induced primary cultured rat cardiac fibroblasts, which contribute to myocardial fibrosis.
Klotho, a specific antiaging protein of kidney, is mainly expressed in renal tubular epithelial cells and has a significant anti-fibrosis effect (Guan et al., 2014; Ding et al., 2019). The luciferase reporter assay showed that miR-34a directly down-regulated the expression of Klotho. In renal fibrosis, the increased expression of miR-34a is accompanied by the sharp downregulation of Klotho, the increase of a—SMA and fibronectin, and the decrease of E-cadherin, which promote the process of epithelial mesenchymal transformation (EMT); however, the expression of Klotho was significantly increased and EMT was inhibited in miR-34a−/− mice, so miR-34a negatively regulates Klotho to promote EMT and induce renal fibrosis (Liu et al., 2019).
In addition, there were other miR-34a target genes, including PPAR-γ, PNUTS, RXRa, Notch1, c-Met, nectin-1, and Abca3, have been found to affect the fibrosis process by regulating cell proliferation, the EMT process and collagen synthesis (Du et al., 2012; Boon et al., 2013; Oda et al., 2014; Simone et al., 2014; Li et al., 2015; Takano et al., 2017). In various organ fibrosis, miR-34a affects the process of fibrosis by targeting different protein-coding genes.
Role of MicroRNA-34a in Transforming Growth Factor-β1/Smad Signaling Pathway
Transforming growth factor-β1 (TGF-β1) is a key cytokine involved in the formation of fibrosis (Ghosh et al., 2013) that not only plays an important role in the transdifferentiation of fibroblasts into myofibroblasts but also triggers the EMT, mesothelial-to-mesenchymal transition (MMT) and endothelial-to-mesenchymal-transition (EndoMT) processes, controls the extracellular matrix (ECM) synthesis, and participates in the pathogenesis of fibrosis (Wu et al., 2013; Weiskirchen et al., 2019). There is a certain correlation between miR-34a disorders and TGF-β pathway in fibrotic diseases (Xie et al., 2011; Zhang et al., 2014; Zhang J. et al., 2021).
Firstly, Bin Zhou found that eight miRNAs and seven mRNA were involved in TGF-β signal pathway, including miR-34a, in systemic sclerosis (SSc) by Gene Expression Omnibus (GEO) analysis (Zhou et al., 2017), this was a direct evidence that miR-34a targets fibrosis through TGF-β signaling pathway. Smad transcription factors are the core of TGF-β pathway (Massague et al., 2005). TGF-β1/Smad signaling pathway has been widely recognized as a typical pathway in fibrosis (Zhang et al., 2019; Lv et al., 2020). The expression of miR-34a was increased in mice with cardiac fibrosis, and the degree of fibrosis was inhibited by miR-34a antagonist; miR-34a directly targets Smad4 mRNA according to luciferase reporter assay; when the fibroblasts are transfected with Smad4 siRNA, the expression of type I collagen, TGF-β1 and a-SMA was suppressed. The study indicated that TGF-β1 induces the expression of miR-34a, which in turn promotes the activation of TGF-β1-induced myocardial fibroblasts and the formation of cardiac fibrosis by targeting Smad4 (Huang et al., 2014). In carbon tetrachloride (CCl4)-induced hepatic fibrosis mice, miR-34a imbalance was also found to promote liver fibrosis via targeting Smad4 and activation TGF-β1/Smad3 pathway (Feili et al., 2018).
Besides, miR-34a/SIRT1/p53 loop is also involved in the EMT mediated by TGF-β1/Smad signaling pathway. Activated p53 (ac-p53 and p-p53) combines with Smad3 to form a multiprotein complex to promote TGF-β1-induced EMT process (Piccolo, 2008; Termén et al., 2013). In rat model of hepatic fibrosis, it was found that miR-34a was overexpressed, SIRT1 was down-regulated, p53 and ac-p53 were increased, with activated TGF-β1/Smad signal pathway; miR-34a inhibitor and p53 siRNA significantly prevented TGF-β1-induced EMT in hepatocytes, and alleviated the degree of hepatic fibrosis (Song et al., 2019). Therefore, these results suggest that TGF-β1/Smad signaling pathway mediates the process of miR-34a-induced fibrosis.
MiRNA-34a as Therapeutic Targets of Fibrosis
As described, miR-34a is a key regulator of FB-related molecules. In recent years, miR-34a or miR-34a-targeted gene have been used as new intervention targets in the treatment of FB, which have better effectiveness. Therefore, the regulation of miR-34a and related molecules are expected to be new therapeutic targets for FB (Table 2).
MicroRNA-34a Inhibitors
In most studies, miR-34a inhibitors were used to improve the degree of fibrosis. At the cellular level, miR-34a inhibitor was transfected into renal tubular cells incubated with TGF-β1 to induce the upregulation of Bcl-2, inhibit the apoptosis of renal tubular cells and improve the degree of renal fibrosis (Zhou et al., 2014). Transfection of miR-34a silencing vector using Lipofectamine2000 into activated HSCs increased the expression of ACSL1 and promoted lipogenesis, thereby inhibiting HSCs activation and hepatic fibrosis (Yan et al., 2015). MiR-34a inhibitor was also found to increase PPAR γ, decrease a-SMA, and improve the process of liver fibrosis (Li et al., 2015). Transfection of miR-34a inhibitor in primary hepatocytes increased SIRT1 and p65/p53 deacetylation levels, decreased the expression of proinflammatory cytokines and improved liver inflammatory response (Kim et al., 2015). MiR-34a inhibitor could reduce the EMT process and fibrosis activity of human intrahepatic biliary epithelial cells, and improved liver fibrosis (Pan et al., 2021). In cardiac fibroblasts, a miR-34a antagonist improved cardiac fibrosis by inhibiting TGF-β1 signaling (Huang et al., 2014). In vivo study, Subcutaneous injection of locked nucleic acid (LNA)-antimiR-34a (initial dose 25 mg/kg, maintenance dose 10 mg/kg every other day, 3 times a week for 6 weeks) can improved the cardiac function of female mice with dilated cardiomyopathy, characterized by attenuated heart enlargement and lung congestion, inhibit the expression of cardiac stress genes, and alleviate myocardial fibrosis (Bernardo et al., 2016). Besides, miR-34a inhibitors can improve myocardial fibrosis and reduce scar area in myocardial infarction rats (Zhang F. et al., 2021). In the mice of CCl4-induced liver fibrosis, miR-34a siRNA significantly reduced the express of TGF-β, a-SMA, and MCP-1, further inhibited the fibrosis of HSCs (Zhang J. et al., 2021). It has also been found that ablation of miR-34a protected aged animals from developing experimental lung fibrosis (Cui et al., 2017b).
In addition, there are some compounds acting on miR-34a to intervene in FB. Hydrogen sulfide and astragaloside IV(AS-IV) were found to reverse myocardial fibrosis, which may be related to the down-regulation of miR-34a to activate autophagy (Liu et al., 2018; Zhu et al., 2019). Prunella vulgaris aqueous extract (PVAE) can downregulate miR-34a level, inhibit the activation of HSCs, and regulate the expression of TIMP-1, MMP-2, and MMP-13, promoting the degradation of collagen, and alleviating hepatic fibrosis (Hu et al., 2016); Paclitaxel has been applied to treat fibrosis by downregulating miR-34a, upregulating SIRT1, and inhibiting p53 activation and TGF-β1/Smads signal pathway (Song et al., 2019). Atorvastatin also inhibited miR-34a and upregulated SIRT1 to improve myocardial fibrosis (Tabuchi et al., 2012). Therefore, the above study shows that the downregulation of miR-34a has therapeutic effect on FB.
The Biological Agents of MicroRNA-34a-Related Molecules
The target gene of miR-34a has been used as the therapeutic target for fibrosis in some researches. SRT1720, the SIRT1activator, inhibited hepatocyte apoptosis and improved liver fibrosis by reducing the expression of miR-34a and the acylation of p53 (Tian et al., 2016). Resveratrol, another SIRT1 activator, was often used as an inhibitor in fibrosis researches (Chávez et al., 2008; Hong et al., 2010; Li et al., 2010). P53 inhibitor, pifithrin—a (PFT), decreased the level of miR-34a and played a protective role in hepatic ischemia/reperfusion mice (Kim et al., 2015). In addition, PPAR γ activators blocked the activation of HSCs in hepatic fibrosis (Attia et al., 2013; Sharvit et al., 2013). Smad4 siRNA downregulated the mRNA and protein expression of Col I, a-SMA, and TGF-β1, and inhibited myocardial fibrosis (Huang et al., 2014). Jagged1 siRNA and Notch 1 siRNAs effectively inhibited EMT in renal tubular epithelial cells (Du et al., 2012). LGR4 is the direct target of miR-34a, LGR4 siRNA significantly inhibited the proliferation and migration of retinal pigmented epithelial cell line ARPE-19 (Hou et al., 2016). As a novel direct miR-34a target, PNUTS improved the functional recovery after acute myocardial infarction by reducing telomere shortening, DNA damage response and cardiomyocyte apoptosis (Boon et al., 2013). These results suggest that miR-34a-related molecules also plays an important role in the treatment of FB, which may provide guiding significance for clinical research.
Limitation of MicroRNA-34a as Therapeutic Targets of Fibrosis
Currently there are no FDA-approved miRNAs, but many miRNA therapies have achieved substantial preclinical efficacy, even entered in clinical trials (Wang et al., 2021; Smith et al., 2022; Zogg et al., 2022). For example, miravirsen (miR-122 inhibitor) has completed Phase II clinical trials for the treatment of Hepatitis C (Janssen et al., 2013; Panigrahi et al., 2022). The Phase I clinical trials of MRG-110 (miR-92a inhibitor) to improve wound healing has been completed (Gallant-Behm et al., 2018; Abplanalp et al., 2020). A Phase I/IIa clinical trial has demonstrated the potential of RG-125 (AZD4076) (miR-103/107 inhibitor) for the treatment of type 2 diabetes and non-alcoholic fatty liver disease (Rottiers and Naar, 2012). A Phase 1b clinical trial of RGLS4326 (miR-17 inhibitor) in patients with autosomal dominant polycystic kidney disease is under way (Kim and Park, 2016). A Phase I clinical trials have shown that CDR132L inhibits miR-132 in patients with heart failure (Ucar et al., 2012). Moreover, TargomiRs, a miR-16 mimic, has been considered as a second- or third-line treatment for recurrent malignant pleural mesothelioma and non-small cell lung cancer (van Zandwijk et al., 2017). Therefore, the therapeutic potential of miRNAs is limitless.
Based on the existing research, miR-34a plays a complex and important role in fibrotic diseases. It will be a new target for the treatment of FB, but there are still many practical problems for miR-34a as a therapeutic target. At present, the anti-fibrosis effect of miR-34a and its target molecules have been explored mainly at the cellular level in vitro, perhaps because of the functional complexity of miR-34a and the non-target effect in vivo. There are still some problems in the preparation of miR-34a inhibitors. Although liposome transfection has been used in some experiments, it has the disadvantage of immunogenicity (Simone et al., 2014; Yan et al., 2015). Viral delivery enables long-term, persistent, and high expression of miRNAs, but it also has the disadvantage of nonspecific binding, so it cannot transport miRNAs to the designated site. Microvesicles, a new cell signaling vector for short- or long-range delivery, contains protein, mRNA and miRNA (Martins et al., 2013; Recep et al., 2017). In a study of renal fibrosis, it has been found that (Zhou et al., 2014; Li et al., 2019) renal interstitial fibroblasts can secrete microvesicles containing miR-34a to transport to renal tubular epithelial cells and promote their apoptosis; then the microbubbles in fibroblasts can be extracted and injected into cells or mice to imitate the mechanism of miR-34a in renal fibrosis. With the development of science and technology, other better biological agents will likely be found in the future, further improving the treatment of FB.
Conclusion
In summary, although there have been many studies on the pathogenesis of fibrosis, there are still many deficiencies in the treatment of fibrosis. Various types of fibrosis, such as pulmonary fibrosis, cardiac fibrosis, liver fibrosis, renal fibrosis, etc., involve the same or different internal signal network. Therefore, it is very difficult to find the common target of fibrosis. Although there were two drugs (pirfenidone and nintedanib) have been approved for the treatment of idiopathic pulmonary fibrosis, they can only improve lung capacity and survival rate, and do not show beneficial histological changes in pulmonary fibrosis (Martinez et al., 2017). Therefore, it is urgent to develop new anti-fibrosis therapy for other fibrotic diseases. MiR-34a can regulate the expression of many genes and proteins, and participate in complex signal mechanism. Compared with traditional cytokines and signal molecules, miR-34a is more suitable as a common target for the regulation of organ FB. MiR-34a is a key regulator of fibrosis, which is involved in the regulation of apoptosis, senescence, autophagy, and TGF-β1 signaling pathway in epithelial cells and fibroblasts to affect the excessive repair; moreover, target genes of miR-34a also regulate the process of fibrosis in many ways; the application of miR-34a inhibitor has also been found to significantly improve the degree of fibrosis. So miR-34a is expected to become a new target for the treatment of fibrosis. However, those vivo or clinical studies on the treatment of fibrosis with miR-34a are still little and incomplete, so the specific mechanism and efficacy need to be further verified.
Author Contributions
MZ wrote the manuscript. QQ, SL, and RH collect related literature; JS, YZ, JC, and HZ revised the manuscript; HW and HL contributed to conception and design of the article. All the authors reviewed the manuscript and agreed for submission.
Funding
This study supported by the National Natural Sciences Foundation of China (81873374); the Science and Technology Commission of Shanghai (21ZR1460000); Shanghai Sailing Program (20YF1445400), and Shanghai Clinical Research Center for Acupuncture and Moxibustion (No. 20MC1920500).
Conflict of Interest
The authors declare that the research was conducted in the absence of any commercial or financial relationships that could be construed as a potential conflict of interest.
Publisher’s Note
All claims expressed in this article are solely those of the authors and do not necessarily represent those of their affiliated organizations, or those of the publisher, the editors and the reviewers. Any product that may be evaluated in this article, or claim that may be made by its manufacturer, is not guaranteed or endorsed by the publisher.
References
Abplanalp W. T., Fischer A., John D., Zeiher A. M., Gosgnach W., Darville H. (2020). Efficiency and Target Derepression of Anti-miR-92a: Results of a First in Human Study. Nucleic Acid. Ther. 30(6), 335–345. doi:10.1089/nat.2020.0871
Alivernini S., Bosello S. L., Luca G. D., Canestri S., Mario C. D., Gigante M. R., et al. (2014). A3.21 MicroRNA-34a and microRNA-155 in Systemic Sclerosis: Possible Epigenetic Biomarkers of Endothelial Dysfunction in VEDOSS and Long-Standing Disease. 73 (Suppl. 1), A50. doi:10.1136/annrheumdis-2013-205124.114
Álvarez D., Cárdenes N., Sellarés J., Bueno M., Corey C., Hanumanthu V. S., et al. (2017). IPF Lung Fibroblasts Have a Senescent Phenotype. Am. J. physiology. Lung Cell. Mol. physiology 313 (6), L1164–L1173. doi:10.1152/ajplung.00220.2017
Aoki M., Fujishita T. (2017). Oncogenic Roles of the PI3K/AKT/mTOR Axis. Curr. Top. Microbiol. Immunol. 407, 153–189. doi:10.1007/82_2017_6
Attia Y. M., Elalkamy E. F., Hammam O. A., Mahmoud S. S., El-Khatib A. S. (2013). Telmisartan, an AT1 Receptor Blocker and a PPAR Gamma Activator, Alleviates Liver Fibrosis Induced Experimentally by Schistosoma Mansoni Infection. Parasites vectors 6, 199. doi:10.1186/1756-3305-6-199
Bernardo B. C., Ooi J. Y. Y., Matsumoto A., Tham Y. K., Singla S., Kiriazis H., et al. (2016). Sex Differences in Response to miRNA-34a Therapy in Mouse Models of Cardiac Disease: Identification of Sex-, Disease- and Treatment-Regulated miRNAs. J. physiology 594 (20), 5959–5974. doi:10.1113/JP272512
Bhandary Y. P., Shetty S. K., Marudamuthu A. S., Gyetko M. R., Idell S., Gharaee-Kermani M., et al. (2012). Regulation of Alveolar Epithelial Cell Apoptosis and Pulmonary Fibrosis by Coordinate Expression of Components of the Fibrinolytic System. Am. J. physiology. Lung Cell. Mol. physiology 302 (5), L463–L473. doi:10.1152/ajplung.00099.2011
Bommer G. T., Gerin I., Feng Y., Kaczorowski A. J., Kuick R., Love R. E., et al. (2007). p53-mediated Activation of miRNA34 Candidate Tumor-Suppressor Genes. Curr. Biol. 17 (15), 1298–1307. doi:10.1016/j.cub.2007.06.068
Boon R. A., Iekushi K., Lechner S., Seeger T., Fischer A., Heydt S., et al. (2013). MicroRNA-34a Regulates Cardiac Ageing and Function. Nature 495 (7439), 107–110. doi:10.1038/nature11919
Cai X., Wang J., Wang J., Zhou Q., Yang B., He Q., et al. (2020). Intercellular Crosstalk of Hepatic Stellate Cells in Liver Fibrosis: New Insights into Therapy. Pharmacol. Res. 155, 104720. doi:10.1016/j.phrs.2020.104720
Castro R. E., Ferreira D. M., Afonso M. B., Borralho P. M., Machado M. V., Cortez-Pinto H., et al. (2013). miR-34a/SIRT1/p53 Is Suppressed by Ursodeoxycholic Acid in the Rat Liver and Activated by Disease Severity in Human Non-alcoholic Fatty Liver Disease. J. Hepatol. 58 (1), 119–125. doi:10.1016/j.jhep.2012.08.008
Chávez E., Reyes-Gordillo K., Segovia J., Shibayama M., Tsutsumi V., Vergara P., et al. (2008). Resveratrol Prevents Fibrosis, NF-kappaB Activation and TGF-Beta Increases Induced by Chronic CCl4 Treatment in Rats. J. Appl. Toxicol. JAT 28 (1), 35–43. doi:10.1002/jat.1249
Chen F., Hu S. (2012). Effect of microRNA-34a in Cell Cycle, Differentiation, and Apoptosis: a Review. J. Biochem. Mol. Toxicol. 26 (2), 79–86. doi:10.1002/jbt.20412
Cui H., Ge J., Xie N., Banerjee S., Zhou Y., Antony V. B., et al. (2017a). miR-34a Inhibits Lung Fibrosis by Inducing Lung Fibroblast Senescence. Am. J. Respir. Cell Mol. Biol. 56 (2), 168–178. doi:10.1165/rcmb.2016-0163OC
Cui H., Ge J., Xie N., Banerjee S., Zhou Y., Liu R.-M., et al. (2017b). miR-34a Promotes Fibrosis in Aged Lungs by Inducing Alveolarepithelial Dysfunctions. Am. J. physiology. Lung Cell. Mol. physiology 312 (3), L415–L424. doi:10.1152/ajplung.00335.2016
Cunnington R. H., Nazari M., Dixon I. M. C. (2009). c-Ski, Smurf2, and Arkadia as Regulators of TGF-Beta Signaling: New Targets for Managing Myofibroblast Function and Cardiac Fibrosis. Can. J. physiology Pharmacol. 87 (10), 764–772. doi:10.1139/Y09-076
Ding J., Tang Q., Luo B., Zhang L., Lin L., Han L., et al. (2019). Klotho Inhibits Angiotensin II-Induced Cardiac Hypertrophy, Fibrosis, and Dysfunction in Mice through Suppression of Transforming Growth Factor-Beta1 Signaling Pathway. Eur. J. Pharmacol. 859, 172549. doi:10.1016/j.ejphar.2019.172549
Ding Y., Choi M. E. (2014). Regulation of Autophagy by TGF-β: Emerging Role in Kidney Fibrosis. Seminars Nephrol. 34 (1), 62–71. doi:10.1016/j.semnephrol.2013.11.009
Disayabutr S., Kim E. K., Cha S.-I., Green G., Naikawadi R. P., Jones K. D., et al. (2016). miR-34 miRNAs Regulate Cellular Senescence in Type II Alveolar Epithelial Cells of Patients with Idiopathic Pulmonary Fibrosis. PloS one 11 (6), e0158367. doi:10.1371/journal.pone.0158367
Docherty N. G., O'Sullivan O. E., Healy D. A., Fitzpatrick J. M., Watson R. W. G. (2006). Evidence that Inhibition of Tubular Cell Apoptosis Protects against Renal Damage and Development of Fibrosis Following Ureteric Obstruction. Am. J. physiology. Ren. physiology 290 (1), F4–F13. doi:10.1152/ajprenal.00045.2005
Du R., Sun W., Xia L., Zhao A., Yu Y., Zhao L., et al. (2012). Hypoxia-induced Down-Regulation of microRNA-34a Promotes EMT by Targeting the Notch Signaling Pathway in Tubular Epithelial Cells. PloS one 7 (2), e30771. doi:10.1371/journal.pone.0030771
Feili X., Wu S., Ye W., Tu J., Lou L. (2018). MicroRNA-34a-5p Inhibits Liver Fibrosis by Regulating TGF-β1/Smad3 Pathway in Hepatic Stellate Cells. Cell Biol. Int. 42 (10), 1370–1376. doi:10.1002/cbin.11022
Fu S., Hu X., Ma Z., Wei Q., Xiang X., Li S., et al. (2022). p53 in Proximal Tubules Mediates Chronic Kidney Problems after Cisplatin Treatment. Cells 11 (4), 712. doi:10.3390/cells11040712
Gallant-Behm C. L., Piper J., Dickinson B. A., Dalby C. M., Pestano L. A., Jackson A. L. (2018). A Synthetic microRNA-92a Inhibitor (MRG-110) Accelerates Angiogenesis and Wound Healing in Diabetic and Nondiabetic Wounds. Wound Repair Regen. 26 (4), 311–323. doi:10.1111/wrr.12660
Ghosh A. K., Quaggin S. E., Vaughan D. E. (2013). Molecular Basis of Organ Fibrosis: Potential Therapeutic Approaches. Exp. Biol. Med. (Maywood, N.J.) 238 (5), 461–481. doi:10.1177/1535370213489441
Gieseck R. L., Wilson M. S., Wynn T. A. (2018). Type 2 Immunity in Tissue Repair and Fibrosis. Nat. Rev. Immunol. 18 (1), 62–76. doi:10.1038/nri.2017.90
Guan X., Nie L., He T., Yang K., Xiao T., Wang S., et al. (2014). Klotho Suppresses Renal Tubulo-Interstitial Fibrosis by Controlling Basic Fibroblast Growth Factor-2 Signalling. J. Pathol. 234 (4), 560–572. doi:10.1002/path.4420
Harding K. G., Moore K., Phillips T. J. (2005). Wound Chronicity and Fibroblast Senescence-Iimplications for Treatment. Int. wound J. 2 (4), 364–368. doi:10.1111/j.1742-4801.2005.00149.x
Harries L. W. (2014). MicroRNAs as Mediators of the Ageing Process. Genes (Basel) 5 (3), 656–670. doi:10.3390/genes5030656
Henderson N. C., Rieder F., Wynn T. A. (2020). Fibrosis: from Mechanisms to Medicines. Nature 587 (7835), 555–566. doi:10.1038/s41586-020-2938-9
Hermeking H. (2010). The miR-34 Family in Cancer and Apoptosis. Cell death Differ. 17 (2), 193–199. doi:10.1038/cdd.2009.56
Hong S.-W., Jung K. H., Zheng H.-M., Lee H.-S., Suh J.-K., Park I.-S., et al. (2010). The Protective Effect of Resveratrol on Dimethylnitrosamine-Induced Liver Fibrosis in Rats. Archives pharmacal Res. 33 (4), 601–609. doi:10.1007/s12272-010-0415-y
Hou Q., Zhou L., Tang J., Ma N., Xu A., Tang J., et al. (2016). LGR4 Is a Direct Target of MicroRNA-34a and Modulates the Proliferation and Migration of Retinal Pigment Epithelial ARPE-19 Cells. PloS one 11 (12), e0168320. doi:10.1371/journal.pone.0168320
Hu Y., Yu C., Wu F., Yu W., Zhong Y., Ying H., et al. (2016). Antihepatofibrotic Effects of Aqueous Extract of Prunella Vulgaris on Carbon Tetrachloride-Induced Hepatic Fibrosis in Rats. Planta medica. 82 (1-2), 97–105. doi:10.1055/s-0035-1558112
Huang Y., Qi Y., Du J., Zhang D. (2014). MicroRNA-34a Regulates Cardiac Fibrosis after Myocardial Infarction by Targeting Smad4. Expert Opin. Ther. targets 18 (12), 1355–1365. doi:10.1517/14728222.2014.961424
Janssen H. L., Reesink H. W., Lawitz E. J., Zeuzem S., Rodriguez-Torres M., Patel K., et al. (2013). Treatment of HCV Infection by Targeting microRNA. N. Engl. J. Med. 368 (18), 1685–1694. doi:10.1056/NEJMoa1209026
Jesus C.-R., Francisco C., Dulce C. M.-C., Laura G.-F., Dolores O.-M., Juan V. E., et al. (2019). Autophagy Stimulation as a Potential Strategy against. Intest. Fibros. 8 (9), 1078. doi:10.3390/cells8091078
Jun J.-I., Lau L. F. (2018). Resolution of Organ Fibrosis. J. Clin. investigation 128 (1), 97–107. doi:10.1172/JCI93563
Jun J. I., Lau L. F. (2010). The Matricellular Protein CCN1/CYR61 Induces Fibroblast Senescence and Restricts Fibrosis in Cutaneous Wound Healing. Nat. Cell Biol. 12 (7), 676–685. doi:10.1038/ncb2070
Kim D. Y., Park J. H. (2016). Genetic Mechanisms of ADPKD. Adv. Exp. Med. Biol. 933, 13–22. doi:10.1007/978-981-10-2041-4_2
Kim H. J., Joe Y., Yu J. K., Chen Y., Jeong S. O., Mani N., et al. (2015). Carbon Monoxide Protects against Hepatic Ischemia/reperfusion Injury by Modulating the miR-34a/SIRT1 Pathway. Biochimica biophysica acta 1852 (7), 1550–1559. doi:10.1016/j.bbadis.2015.04.017
Kota A., Deshpande D. A., Haghi M., Oliver B., Sharma P. (2017). Autophagy and Airway Fibrosis: Is There a Link? F1000Res 6, 409. doi:10.12688/f1000research.11236.2
Krizhanovsky V., Yon M., Dickins R. A., Hearn S., Simon J., Miething C., et al. (2008). Senescence of Activated Stellate Cells Limits Liver Fibrosis. Cell 134 (4), 657–667. doi:10.1016/j.cell.2008.06.049
Kumamoto K., Spillare E. A., Fujita K., Horikawa I., Yamashita T., Appella E., et al. (2008). Nutlin-3a Activates P53 to Both Down-Regulate Inhibitor of Growth 2 and Up-Regulate Mir-34a, Mir-34b, and Mir-34c Expression, and Induce Senescence. Cancer Res. 68 (9), 3193–3203. doi:10.1158/0008-5472.CAN-07-2780
Kyle L.-W., Claire J. C., Nigel B. J., Karin A. O., Keith W. N. (2009). Pathway Analysis of Senescence-Associated miRNA Targets Reveals Common Processes to Different Senescence Induction Mechanisms. Biochimica Biophysica Acta 1792 (4), 341–352. doi:10.1016/j.bbadis.2009.02.003
Lehmann M., Korfei M., Mutze K., Klee S., Skronska-Wasek W., Alsafadi H. N., et al. (2017). Senolytic Drugs Target Alveolar Epithelial Cell Function and Attenuate Experimental Lung Fibrosis Ex Vivo. Eur. Respir. J. 50 (2), 1602367. doi:10.1183/13993003.02367-2016
Li A., Peng R., Sun Y., Liu H., Peng H., Zhang Z. (2018). LincRNA 1700020I14Rik Alleviates Cell Proliferation and Fibrosis in Diabetic Nephropathy via miR-34a-5p/Sirt1/HIF-1alpha Signaling. Cell Death Dis. 9 (5), 461. doi:10.1038/s41419-018-0527-8
Li B., Li F., Gu T., Guo Y., Shen B., Xu X., et al. (2022). Specific Knockdown of Y-Box Binding Protein 1 in Hepatic Progenitor Cells Inhibits Proliferation and Alleviates Liver Fibrosis. Eur. J. Pharmacol. 921, 174866. doi:10.1016/j.ejphar.2022.174866
Li H., Xu Y., Zhang Q., Xu H., Xu Y., Ling K. (2019). Microvesicles Containing miR-34a Induce Apoptosis of Proximal Tubular Epithelial Cells and Participate in Renal Interstitial Fibrosis. Exp. Ther. Med. 17 (3), 2310–2316. doi:10.3892/etm.2019.7197
Li J., Qu X., Ricardo S. D., Bertram J. F., Nikolic-Paterson D. J. (2010). Resveratrol Inhibits Renal Fibrosis in the Obstructed Kidney: Potential Role in Deacetylation of Smad3. Am. J. pathology 177 (3), 1065–1071. doi:10.2353/ajpath.2010.090923
Li W., Chen C., Xu M., Guo J., Li Y., Xia Q., et al. (2011). The Rno-miR-34 Family Is Upregulated and Targets ACSL1 in Dimethylnitrosamine-Induced Hepatic Fibrosis in Rats. FEBS J. 278 (9), 1522–1532. doi:10.1111/j.1742-4658.2011.08075.x
Li X., Chen Y., Wu S., He J., Lou L., Ye W., et al. (2015). microRNA-34a and microRNA-34c Promote the Activation of Human Hepatic Stellate Cells by Targeting Peroxisome Proliferator-Activated Receptor γ. Mol. Med. Rep. 11 (2), 1017–1024. doi:10.3892/mmr.2014.2846
Liu M., Li Z., Liang B., Li L., Liu S., Tan W., et al. (2018). Hydrogen Sulfide Ameliorates Rat Myocardial Fibrosis Induced by Thyroxine through PI3K/AKT Signaling Pathway. Endocr. J. 65 (7), 769–781. doi:10.1507/endocrj.EJ17-0445
Liu Y., Bi X., Xiong J., Han W., Xiao T., Xu X., et al. (2019). MicroRNA-34a Promotes Renal Fibrosis by Downregulation of Klotho in Tubular Epithelial Cells. Mol. Ther. J. Am. Soc. Gene Ther. 27 (5), 1051–1065. doi:10.1016/j.ymthe.2019.02.009
Lv X., Liu S., Hu Z. (2017). Autophagy-inducing Natural Compounds: a Treasure Resource for Developing Therapeutics against Tissue Fibrosis. J. Asian Nat. Prod. Res. 19 (2), 101–108. doi:10.1080/10286020.2017.1279151
Lv Y., Bing Q., Lv Z., Xue J., Li S., Han B., et al. (2020). Imidacloprid-induced Liver Fibrosis in Quails via Activation of the TGF-beta1/Smad Pathway. Sci. Total Environ. 705, 135915. doi:10.1016/j.scitotenv.2019.135915
Madesh M., Zong W. X., Hawkins B. J., Ramasamy S., Venkatachalam T., Mukhopadhyay P., et al. (2009). Execution of Superoxide-Induced Cell Death by the Proapoptotic Bcl-2-Related Proteins Bid and Bak. Mol. Cell Biol. 29 (11), 3099–3112. doi:10.1128/MCB.01845-08
Martinez F. J., Collard H. R., Pardo A., Raghu G., Richeldi L., Selman M., et al. (2017). Idiopathic Pulmonary Fibrosis. Nat. Rev. Dis. Prim. 3, 17074. doi:10.1038/nrdp.2017.74
Martins V. R., Dias M. S., Hainaut P. (2013). Tumor-cell-derived Microvesicles as Carriers of Molecular Information in Cancer. Curr. Opin. Oncol. 25 (1), 66–75. doi:10.1097/CCO.0b013e32835b7c81
Massague J., Seoane J., Wotton D. (2005). Smad Transcription Factors. Genes Dev. 19 (23), 2783–2810. doi:10.1101/gad.1350705
Meng F., Glaser S. S., Francis H., Yang F., Han Y., Stokes A., et al. (2012). Epigenetic Regulation of miR-34a Expression in Alcoholic Liver Injury. Am. J. pathology 181 (3), 804–817. doi:10.1016/j.ajpath.2012.06.010
Oda Y., Nakajima M., Tsuneyama K., Takamiya M., Aoki Y., Fukami T., et al. (2014). Retinoid X Receptor α in Human Liver Is Regulated by miR-34a. Biochem. Pharmacol. 90 (2), 179–187. doi:10.1016/j.bcp.2014.05.002
Overstreet J. M., Samarakoon R., Meldrum K. K., Higgins P. J. (2014). Redox Control of P53 in the Transcriptional Regulation of TGF-Beta1 Target Genes through SMAD Cooperativity. Cell Signal 26 (7), 1427–1436. doi:10.1016/j.cellsig.2014.02.017
Pan Y., Wang J., He L., Zhang F. (2021). MicroRNA-34a Promotes EMT and Liver Fibrosis in Primary Biliary Cholangitis by Regulating TGF-Beta1/smad Pathway. J. Immunol. Res. 2021, 6890423. doi:10.1155/2021/6890423
Panigrahi M., Thibault P. A., Wilson J. A. (2022). MicroRNA 122 Affects Both the Initiation and the Maintenance of Hepatitis C Virus Infections. J. Virol. 96 (4), e0190321. doi:10.1128/JVI.01903-21
Piccolo S. (2008). p53 Regulation Orchestrates the TGF-Beta Response. Cell 133 (5), 767–769. doi:10.1016/j.cell.2008.05.013
Qi Y., Zhao A., Yang P., Jin L., Hao C. (2020). miR-34a-5p Attenuates EMT through Targeting SMAD4 in Silica-Induced Pulmonary Fibrosis. J. Cell Mol. Med. 24 (20), 12219–12224. doi:10.1111/jcmm.15853
Ramos K. J., Smith P. J., McKone E. F., Pilewski J. M., Lucy A., Hempstead S. E., et al. (2019). Lung Transplant Referral for Individuals with Cystic Fibrosis: Cystic Fibrosis Foundation Consensus Guidelines. J. Cyst. Fibros. 18 (3), 321–333. doi:10.1016/j.jcf.2019.03.002
Recep B., Katrien V. R., George A. C. (2017). Cell-to-cell Communication: microRNAs as Hormones. Mol. Oncol. 11 (12), 1673–1686. doi:10.1002/1878-0261.12144
Richeldi L., Collard H. R., Jones M. G. (2017). Idiopathic Pulmonary Fibrosis. Lancet 389 (10082), 1941–1952. doi:10.1016/s0140-6736(17)30866-8
Rieder F., Kessler S., Sans M., Fiocchi C. (2012). Animal Models of Intestinal Fibrosis: New Tools for the Understanding of Pathogenesis and Therapy of Human Disease. Am. J. physiology. Gastrointest. liver physiology 303 (7), G786–G801. doi:10.1152/ajpgi.00059.2012
Rottiers V., Naar A. M. (2012). MicroRNAs in Metabolism and Metabolic Disorders. Nat. Rev. Mol. Cell Biol. 13 (4), 239–250. doi:10.1038/nrm3313
Salminen A., Kaarniranta K. (2009). SIRT1: Regulation of Longevity via Autophagy. Cell Signal 21 (9), 1356–1360. doi:10.1016/j.cellsig.2009.02.014
Sharvit E., Abramovitch S., Reif S., Bruck R. (2013). Amplified Inhibition of Stellate Cell Activation Pathways by PPAR-γ, RAR and RXR Agonists. PloS one 8 (10), e76541. doi:10.1371/journal.pone.0076541
Shetty S. K., Tiwari N., Marudamuthu A. S., Puthusseri B., Bhandary Y. P., Fu J., et al. (2017). p53 and miR-34a Feedback Promotes Lung Epithelial Injury and Pulmonary Fibrosis. Am. J. pathology 187 (5), 1016–1034. doi:10.1016/j.ajpath.2016.12.020
Shi B., Deng W., Long X., Zhao R., Wang Y., Chen W., et al. (2017). miR-21 Increases C-Kit(+) Cardiac Stem Cell Proliferation In Vitro through PTEN/PI3K/Akt Signaling. PeerJ 5, e2859. doi:10.7717/peerj.2859
Simic P., Williams E. O., Bell E. L., Gong J. J., Bonkowski M., Guarente L. (2013). SIRT1 Suppresses the Epithelial-To-Mesenchymal Transition in Cancer Metastasis and Organ Fibrosis. Cell Rep. 3 (4), 1175–1186. doi:10.1016/j.celrep.2013.03.019
Simone B. A., Ly D., Savage J. E., Hewitt S. M., Dan T. D., Ylaya K., et al. (2014). microRNA Alterations Driving Acute and Late Stages of Radiation-Induced Fibrosis in a Murine Skin Model. Int. J. Radiat. Oncol. Biol. Phys. 90 (1), 44–52. doi:10.1016/j.ijrobp.2014.05.003
Smith E. S., Whitty E., Yoo B., Moore A., Sempere L. F., Medarova Z. (2022). Clinical Applications of Short Non-Coding RNA-Based Therapies in the Era of Precision Medicine. Cancers (Basel) 14 (6), 1588. doi:10.3390/cancers14061588
Song L., Chen T., Zhao X., Xu Q., Jiao R., Li J., et al. (2019). Pterostilbene Prevents Hepatocyte Epithelial-Mesenchymal Transition in Fructose-Induced Liver Fibrosis through Suppressing miR-34a/Sirt1/p53 and TGF-β1/Smads Signalling. Br. J. Pharmacol. 176 (11), 1619–1634. doi:10.1111/bph.14573
Sutton T. A., Hato T., Mai E., Yoshimoto M., Kuehl S., Anderson M., et al. (2013). p53 Is Renoprotective after Ischemic Kidney Injury by Reducing Inflammation. J. Am. Soc. Nephrol. 24 (1), 113–124. doi:10.1681/ASN.2012050469
Tabuchi T., Satoh M., Itoh T., Nakamura M. (2012). MicroRNA-34a Regulates the Longevity-Associated Protein SIRT1 in Coronary Artery Disease: Effect of Statins on SIRT1 and microRNA-34a Expression. Clin. Sci. Lond. 123 (3), 161–171. doi:10.1042/CS20110563
Takano M., Nekomoto C., Kawami M., Yumoto R. (2017). Role of miR-34a in TGF-β1- and Drug-Induced Epithelial-Mesenchymal Transition in Alveolar Type II Epithelial Cells. J. Pharm. Sci. 106 (9), 2868–2872. doi:10.1016/j.xphs.2017.04.002
Tang G., Shen X., Lv K., Wu Y., Bi J., Shen Q. (2015). Different Normalization Strategies Might Cause Inconsistent Variation in Circulating microRNAs in Patients with Hepatocellular Carcinoma. Med. Sci. Monit. Int. Med. J. Exp. Clin. Res. 21, 617–624. doi:10.12659/MSM.891028
Tarasov V., Jung P., Verdoodt B., Lodygin D., Epanchintsev A., Menssen A., et al. (2007). Differential Regulation of microRNAs by P53 Revealed by Massively Parallel Sequencing: miR-34a Is a P53 Target that Induces Apoptosis and G1-Arrest. Cell cycleGeorget. Tex.) 6 (13), 1586–1593. doi:10.4161/cc.6.13.4436
Termén S., Tan E. J., Heldin C.-H., Moustakas A. (2013). p53 Regulates Epithelial-Mesenchymal Transition Induced by Transforming Growth Factor β. J. Cell. physiology 228 (4), 801–813. doi:10.1002/jcp.24229
Tian X., Ji F., Zang H., Cao H. (2016). Activation of the miR-34a/SIRT1/p53 Signaling Pathway Contributes to the Progress of Liver Fibrosis via Inducing Apoptosis in Hepatocytes but Not in HSCs. PloS one 11 (7), e0158657. doi:10.1371/journal.pone.0158657
Tsou P.-S., Haak A. J., Khanna D., Neubig R. R. (2014). Cellular Mechanisms of Tissue Fibrosis. 8. Current and Future Drug Targets in Fibrosis: Focus on Rho GTPase-Regulated Gene Transcription. Am. J. physiology. Cell physiology 307 (1), C2–C13. doi:10.1152/ajpcell.00060.2014
Ucar A., Gupta S. K., Fiedler J., Erikci E., Kardasinski M., Batkai S., et al. (2012). The miRNA-212/132 Family Regulates Both Cardiac Hypertrophy and Cardiomyocyte Autophagy. Nat. Commun. 3, 1078. doi:10.1038/ncomms2090
Uhal B. D. (2002). Apoptosis in Lung Fibrosis and Repair. Chest 122 (6 Suppl. l), 293S–298S. doi:10.1378/chest.122.6_suppl.293s
Valentijn F. A., Knoppert S. N., Pissas G., Rodrigues-Diez R. R., Marquez-Exposito L., Broekhuizen R., et al. (2021). CCN2 Aggravates the Immediate Oxidative Stress-DNA Damage Response Following Renal Ischemia-Reperfusion Injury. Antioxidants (Basel) 10(12). 2020. doi:10.3390/antiox10122020
van Zandwijk N., Pavlakis N., Kao S. C., Linton A., Boyer M. J., Clarke S., et al. (2017). Safety and Activity of microRNA-Loaded Minicells in Patients with Recurrent Malignant Pleural Mesothelioma: a First-In-Man, Phase 1, Open-Label, Dose-Escalation Study. Lancet Oncol. 18 (10), 1386–1396. doi:10.1016/S1470-2045(17)30621-6
Villac Adde F., Vidal Campos S., de Oliveira Braga Teixeira R. H., Rodrigues J. C. (2018). Indications for Lung Resection Surgery and Lung Transplant in South American Children with Cystic Fibrosis. Paediatr. Respir. Rev. 25, 37–42. doi:10.1016/j.prrv.2017.02.001
Wan Y., McDaniel K., Wu N., Ramos-Lorenzo S., Glaser T., Venter J., et al. (2017). Regulation of Cellular Senescence by miR-34a in Alcoholic Liver Injury. Am. J. pathology 187 (12), 2788–2798. doi:10.1016/j.ajpath.2017.08.027
Wang B. B., Xie H., Wu T., Xie N., Wu J., Gu Y., et al. (2017a). Controlled-release Mitomycin C-Polylactic Acid Film Prevents Epidural Scar Hyperplasia after Laminectomy by Inducing Fibroblast Autophagy and Regulating the Expression of miRNAs. Eur. Rev. Med. Pharmacol. Sci. 21 (10), 2526–2537. doi:10.7666/d.Y3072421
Wang J., Guo L., Shen D., Xu X., Wang J., Han S., et al. (2017b). The Role of C-SKI in Regulation of TGFbeta-Induced Human Cardiac Fibroblast Proliferation and ECM Protein Expression. J. Cell Biochem. 118 (7), 1911–1920. doi:10.1002/jcb.25935
Wang P., Zhou Y., Richards A. M. (2021). Effective Tools for RNA-Derived Therapeutics: siRNA Interference or miRNA Mimicry. Theranostics 11 (18), 8771–8796. doi:10.7150/thno.62642
Waters D. W., Blokland K. E. C., Pathinayake P. S., Burgess J. K., Mutsaers S. E., Prele C. M., et al. (2018). Fibroblast Senescence in the Pathology of Idiopathic Pulmonary Fibrosis. Am. J. Physiol. Lung Cell Mol. Physiol. 315 (2), L162–L172. doi:10.1152/ajplung.00037.2018
Weiskirchen R., Weiskirchen S., Tacke F. (2019). Organ and Tissue Fibrosis: Molecular Signals, Cellular Mechanisms and Translational Implications. Mol. Asp. Med. 65, 2–15. doi:10.1016/j.mam.2018.06.003
Wu C., Chiang W., Lai C., Chang F., Chen Y., Chou Y., et al. (2013). Transforming Growth Factor β-1 Stimulates Profibrotic Epithelial Signaling to Activate Pericyte-Myofibroblast Transition in Obstructive Kidney Fibrosis. Am. J. pathology 182 (1), 118–131. doi:10.1016/j.ajpath.2012.09.009
Xie T., Liang J., Guo R., Liu N., Noble P. W., Jiang D. (2011). Comprehensive microRNA Analysis in Bleomycin-Induced Pulmonary Fibrosis Identifies Multiple Sites of Molecular Regulation. Physiol. genomics 43 (9), 479–487. doi:10.1152/physiolgenomics.00222.2010
Xue M., Li Y., Hu F., Jia Y., Zheng Z., Wang L., et al. (2018). High Glucose Up-Regulates microRNA-34a-5p to Aggravate Fibrosis by Targeting SIRT1 in HK-2 Cells. Biochem. biophysical Res. Commun. 498 (1), 38–44. doi:10.1016/j.bbrc.2017.12.048
Yamakuchi M., Ferlito M., Lowenstein C. J. (2008). miR-34a Repression of SIRT1 Regulates Apoptosis. Proc. Natl. Acad. Sci. U. S. A. 105 (36), 13421–13426. doi:10.1073/pnas.0801613105
Yan G., Li B., Xin X., Xu M., Ji G., Yu H. (2015). MicroRNA-34a Promotes Hepatic Stellate Cell Activation via Targeting ACSL1. Med. Sci. Monit. Int. Med. J. Exp. Clin. Res. 21, 3008–3015. doi:10.12659/MSM.894000
Yan G. (2016). MicroRNA-34a Bind ACSL1 to Affect Hepatic Fibrosis. Shanghai: The Second Military Medical University.
Yang J., Chen D., He Y., Meléndez A., Feng Z., Hong Q., et al. (2013). MiR-34 Modulates Caenorhabditis elegans Lifespan via Repressing the Autophagy Gene Atg9. Age Dordr. Neth. 35 (1), 11–22. doi:10.1007/s11357-011-9324-3
Yang L., Besschetnova T. Y., Brooks C. R., Shah J. V., Bonventre J. V. (2010). Epithelial Cell Cycle Arrest in G2/M Mediates Kidney Fibrosis after Injury. Nat. Med. 16 (5), 535–543. doi:10.1038/nm.2144
Zhang C., Zhang Y., Zhu H., Hu J., Xie Z. (2018). MiR-34a/miR-93 Target C-Ski to Modulate the Proliferaton of Rat Cardiac Fibroblasts and Extracellular Matrix Deposition In Vivo and In Vitro. Cell. Signal. 46, 145–153. doi:10.1016/j.cellsig.2018.03.005
Zhang F., Gao F., Wang K., Liu X., Zhang Z. (2021a). MiR-34a Inhibitor Protects Mesenchymal Stem Cells from Hyperglycaemic Injury through the Activation of the SIRT1/FoxO3a Autophagy Pathway. Stem Cell Res. Ther. 12 (1), 115. doi:10.1186/s13287-021-02183-2
Zhang G., Oldroyd S. D., Huang L. H., Yang B., Li Y., Ye R., et al. (2001). Role of Apoptosis and Bcl-2/Bax in the Development of Tubulointerstitial Fibrosis during Experimental Obstructive Nephropathy. Exp. Nephrol. 9 (2), 71–80. doi:10.1159/000052597
Zhang J., Tian X., Zhang H., Teng Y., Li R., Bai F., et al. (2014). TGF-β-induced Epithelial-To-Mesenchymal Transition Proceeds through Stepwise Activation of Multiple Feedback Loops. Sci. Signal. 7 (345), ra91. doi:10.1126/scisignal.2005304
Zhang J., Wang H., Yao L., Zhao P., Wu X. (2021b). MiR-34a Promotes Fibrosis of Hepatic Stellate Cells via the TGF-Beta Pathway. Ann. Transl. Med. 9 (20), 1520. doi:10.21037/atm-21-5005
Zhang Q., Chang X., Wang H., Liu Y., Wang X., Wu M., et al. (2019). TGF-beta1 Mediated Smad Signaling Pathway and EMT in Hepatic Fibrosis Induced by Nano NiO In Vivo and In Vitro. Environ. Toxicol. 35 (4), 419–429. doi:10.1002/tox.22878
Zhao X., Kwan J. Y. Y., Yip K., Liu P. P., Liu F. F. (2020). Targeting Metabolic Dysregulation for Fibrosis Therapy. Nat. Rev. Drug Discov. 19 (1), 57–75. doi:10.1038/s41573-019-0040-5
Zhou B., Zuo X. X., Li Y. S., Gao S. M., Dai X. D., Zhu H. L., et al. (2017). Integration of microRNA and mRNA Expression Profiles in the Skin of Systemic Sclerosis Patients. Sci. Rep. 7, 42899. doi:10.1038/srep42899
Zhou Y., Xiong M., Niu J., Sun Q., Su W., Zen K., et al. (2014). Secreted Fibroblast-Derived miR-34a Induces Tubular Cell Apoptosis in Fibrotic Kidney. J. Cell Sci. 127 (Pt 20), 4494–4506. doi:10.1242/jcs.155523
Zhu Y., Qian X., Li J., Lin X., Luo J., Huang J., et al. (2019). Astragaloside-IV Protects H9C2(2-1) Cardiomyocytes from High Glucose-Induced Injury via miR-34a-Mediated Autophagy Pathway. Artif. cells, nanomedicine, Biotechnol. 47 (1), 4172–4181. doi:10.1080/21691401.2019.1687492
Zogg H., Singh R., Ro S. (2022). Current Advances in RNA Therapeutics for Human Diseases. Int. J. Mol. Sci. 23 (5), 2736. doi:10.3390/ijms23052736
Keywords: microRNA-34a, fibrosis, apoptosis, autophagy, senescence, TGF-β1/Smad signal pathway, target genes
Citation: Zhao M, Qi Q, Liu S, Huang R, Shen J, Zhu Y, Chai J, Zheng H, Wu H and Liu H (2022) MicroRNA-34a: A Novel Therapeutic Target in Fibrosis. Front. Physiol. 13:895242. doi: 10.3389/fphys.2022.895242
Received: 13 March 2022; Accepted: 30 May 2022;
Published: 20 June 2022.
Edited by:
Zhihong Yang, Université de Fribourg, SwitzerlandReviewed by:
Paul J. Higgins, Albany Medical College, United StatesAmr M. Abdelhamid, October University for Modern Sciences and Arts (MSA), Egypt
Copyright © 2022 Zhao, Qi, Liu, Huang, Shen, Zhu, Chai, Zheng, Wu and Liu. This is an open-access article distributed under the terms of the Creative Commons Attribution License (CC BY). The use, distribution or reproduction in other forums is permitted, provided the original author(s) and the copyright owner(s) are credited and that the original publication in this journal is cited, in accordance with accepted academic practice. No use, distribution or reproduction is permitted which does not comply with these terms.
*Correspondence: Huangan Wu, wuhuangan@126.com; Huirong Liu, lhr_tcm@139.com