- 1Department of Anaesthesiology and Critical Care, Amiens University Hospital, Amiens, France
- 2Department of Anaesthesiology and Critical Care, Centre Hospitalier Regional Universitaire De Dijon, Dijon, France
- 3Université Boulogne Franche Comté, LNC UMR1231, Dijon, France
Introduction: It has been suggested that dynamic arterial elastance (Eadyn) can predict decreases in arterial pressure in response to changing norepinephrine levels. The objective of this study was to determine whether Eadyn is correlated with determinants of the vascular waterfall [critical closing pressure (CCP) and systemic arterial resistance (SARi)] in patients treated with norepinephrine.
Materials and Methods: Patients treated with norepinephrine for vasoplegia following cardiac surgery were studied. Vascular and flow parameters were recorded immediately before the norepinephrine infusion and then again once hemodynamic parameters had been stable for 15 min. The primary outcomes were Eadyn and its associations with CCP and SARi. The secondary outcomes were the associations between Eadyn and vascular/flow parameters.
Results: At baseline, all patients were hypotensive with Eadyn of 0.93 [0.47;1.27]. Norepinephrine increased the arterial blood pressure, cardiac index, CCP, total peripheral resistance (TPRi), arterial elastance, and ventricular elastance and decreased Eadyn [0.40 (0.30;0.60)] and SARi. Eadyn was significantly associated with arterial compliance (CA), CCP, and TPRi (p < 0.05).
Conclusion: In patients with vasoplegic syndrome, Eadyn was correlated with determinants of the vascular waterfall. Eadyn is an easy-to-read functional index of arterial load that can be used to assess the patient’s macro/microcirculatory status.
Clinical Trial Registration: ClinicalTrials.gov #NCT03478709.
Introduction
Arterial hypotension in relation to vasoplegic syndrome is one of the most important causes of acute circulatory failure in the intensive care unit (ICU; Levin et al., 2009; Fischer and Levin, 2010). This condition is usually treated by the intravenous (IV) administration of a vasoactive agent, such as norepinephrine. The assessment of vascular tone is difficult to carry out in daily practice because the vascular parameters used (peripheral resistance and arterial compliance) did not take into account the complexity of the cardiovascular system which includes two pressure systems with a waterfall phenomenon at the microcirculatory level (Maas et al., 2012). It has been suggested that dynamic arterial elastance (Eadyn) is a marker of vascular tone that can help the physician to manage the treatment of acute vasoplegic circulatory failure (Monge Garcia et al., 2011; Pinsky, 2011; Bouchacourt et al., 2013; Cecconi et al., 2015; Guinot et al., 2015). Eadyn is calculated using the ratio of respiratory pulse pressure variation (PPV) over the respiratory stroke volume variation (SVV). Indeed, it has been shown that Eadyn can predict (i) the increase in mean arterial pressure (MAP) following a fluid challenge (Monge Garcia et al., 2011) and (ii) the decrease in blood pressure following a decrease in the norepinephrine dose (Guinot et al., 2015, 2017; Nguyen et al., 2021). It is a simple marker, is easy to read, and does not require additional monitoring than that carried out routinely in intensive care (Nguyen et al., 2021). However, the physiological significance of Eadyn has never been unambiguously demonstrated. Some studies have evidenced an association between Eadyn and several components of blood pressure: MAP, effective arterial elastance (EA), and arterial compliance (CA; Guinot et al., 2017; Monge García et al., 2017; Bar et al., 2018a).
The concept of vascular waterfall introduced the existence of a pressure gradient between the critical closing pressure (CCP) at the arteriolar level and the mean systemic filling pressure (MSP) at the venular level. The CCP has been described on the microcirculatory level and corresponds to the arterial pressure below which blood flow is stopped by occlusion of the arterioles (Maas et al., 2012). The CCP and indexed systemic arterial resistance (SARi) may also be markers of the vascular tone at the junction between the microcirculatory and macrocirculatory systems. It has been shown that the changes in the vascular waterfall induced by sympathetic stimulation and arterial vasodilation are similar to those observed for Eadyn (Gaskell and Krisman, 1958; Sanders et al., 1988). The association between Eadyn and vascular waterfall components (CCP and SARi) in vasoplegic patients in the ICU has not previously been evaluated. Given that vasomotor manipulation changes CCP and SARi, we hypothesized that Eadyn indirectly reflects the status of these vascular waterfall components.
The main objective of the present study was to determine whether Eadyn is associated with the vascular waterfall components in post-operative cardiac surgery patients treated with norepinephrine. The secondary objectives were to assess the putative associations between Eadyn and several arterial load and blood flow variables.
Materials and Methods
Ethics
The study was approved by the local independent ethics committee (Comité de Protection des Personnes Est II, Besançon, France; reference: 17/07, June 19, 2017) and was registered at Clinicaltrials.gov (NCT03478709). The present report was drafted in line with the STARD statement (Bossuyt et al., 2015). The study complied with the tenets of the Declaration of Helsinki. All patients provided their written informed consent to participation.
Patients
We performed a bicentric, prospective, observational study in the cardiothoracic and vascular ICU in two university hospitals. The inclusion criteria were as follows: adult patients admitted to the ICU and for whom the physician had decided, in accordance with the unit’s care protocols, to treat post-operative vasoplegic syndrome; monitoring with a central venous access and an invasive blood pressure measurement; and the presence of a sinus heart rate (HR). Vasoplegic syndrome was defined by persistent arterial hypotension despite fluid resuscitation (Abou-Arab et al., 2018, 2020). The non-inclusion criteria were the presence of a pacemaker, atrial fibrillation, ventricular tachycardia, or ventricular fibrillation; age under 18 years; prior use of vasoactive/inotropic support; or right heart failure.
Hemodynamic Measurements
We measured the systolic blood pressure (SAP), diastolic blood pressure (DAP), and MAP using an invasive arterial catheter.
Echocardiography (using a CX50 ultrasound system and S5-1 Sector Array Ultrasound Probe Transducer, Philips Medical Systems®, Suresnes, France) was performed by a physician who was blinded to the study outcomes. The left ventricular ejection fraction (LVEF) was measured using Simpson’s biplane method with a four-chamber view. The indexed stroke volume (SVi; ml) was calculated by multiplying the left ventricular outflow tract’s (LVOT’s) velocity time integral (VTI) by the aortic area and dividing by body surface area (BSA). The cardiac index (CI) was calculated as SVi × HR.
The respiratory SVV was calculated as (SVmax − SVmin)/[(SVmax + SVmin)/2] × 100.
Mean echocardiographic parameters were calculated from five individual beat measurements (regardless of the respiratory cycle) and analyzed retrospectively by a senior cardiologist with echocardiography certification.
MSP and CCP
The MSP and CCP were measured using the inspiratory breath-hold maneuver described by Maas et al. (2012). The measured venous and arterial pressures were plotted against cardiac output (CO), and a linear regression line was fitted to the data points. The MSP was determined by extrapolation of the central venous pressure (CVP) to zero flow on the venous return curve. The CCP was determined by extrapolation of the arterial pressure to zero flow on the ventricular output curve. Indexed SARi (mmHg ml−1·m−2) was calculated as (MAP − CCP)/CI. Indexed systemic venous resistance (VRi, mmHg ml−1·m−2) was calculated as (MSP − CVP)/CI.
Left Ventricular End-Systolic Elastance, Arterial Elastance, and Ventricular-Arterial Coupling
Left ventricular end-systolic elastance (EV) was estimated at the bedside by using the equation EV = MAP/(ESVi-4; mmHg ml−1·m−2; Tanaka et al., 1998). This measure non-invasively used echocardiographic measurements of end-systolic volume index (ESVi). This method was used in cardiologic studies to assess the effect of vasoactive medication on ventriculo-arterial coupling (Asanoi et al., 1989; Shmuel et al., 2012).
EA is an index that integrates the main components of arterial load, i.e., total peripheral resistance (TPRi), total net CA, characteristic impedance, and systolic and diastolic time intervals (Tanaka et al., 1998; Ky et al., 2013). EA was estimated by using the equation EA = MAP/SVi (mmHg ml−1·m−2). This ratio has been shown to be a robust EA surrogate over a wide range of hemodynamic conditions and is interchangeable in any peripheral artery (Monge Garcia et al., 2018).
Ventricular-arterial coupling was calculated as EA/EV ratio.
TPRi was calculated as (MAP − CVP)/CI (mmHg ml−1·m−2), and CA (ml mmHg−1·m−2) was calculated as SVi/arterial pulse pressure (PP).
Eadyn was calculated as the ratio between the PPV and the SVV (Guinot et al., 2017).
Study Protocol
The indication for treatment with norepinephrine was left to the discretion of the physician in charge of the patient. The following clinical parameters were recorded: age, gender, weight, height, body mass index, Euroscore 2, medical history, operating time, and cardiopulmonary bypass duration. After an equilibration period, baseline measurements of HR, SAP, MAP, DAP, MSP, TPRi, SVi, CI, SVV, PPV, and vascular determinants of waterfall were recorded. A second (post-norepinephrine) set of measurements was recorded after 15 min of hemodynamic stability (defined as a change in MAP of less than 10%). All patients had been sedated by continuous infusion of propofol and were fully adapted to mechanical ventilation. All patients were mechanically ventilated in pressure-controlled mode with a positive end-expiratory pressure (PEEP) of 5 cmH2O. Ventilator settings and the propofol infusion rate were the same for the two sets of measurements.
Statistical Methods
We calculated that a sample size of 14 patients would allow to demonstrate a correlation between Eadyn and CCP higher than 0.5, using Spearman’s correlation coefficient with a risk alpha of 5% and a power of 80%. The distribution of the variables was assessed using the D’Agostino-Pearson test. Data are expressed as proportion (%), mean (standard deviation), or median [IQR] (interquartile range) as appropriate. Paired data were compared using Wilcoxon signed rank test. Linear correlations were tested using Spearman’s rank method. Associations between arterial load and blood flow parameters measurement considering both time points were assessed using mixed linear modeling. Only bivariate models were carried out. Eadyn was the dependent variable; each hemodynamic variable was used as fixed effect, and individual was used as random intercept. The normality of the distribution of random effects and of the model residual were graphically checked. Because this analysis was exploratory, slight disparities between those distributions and normality were tolerated. The threshold for statistical significance was set to 0.05. Statistical analysis was performed using RStudio Version 1.1.447-2009-2018 RStudio, Inc., and SPSS® software (version 24, IBM, New York, NY, United States).
Results
Twenty patients were included during the study period. Three were excluded because of incomplete data, and so 17 were included in the final analysis. Most of the study participants were male (80%). The median age was 68 [50;71], and the median Euroscore 2 was 2.3 [1.5;4.3] (Table 1). The median dose of norepinephrine was 0.08 gamma kg−1·min−1 [0.06;0.11]. None of the patients had been treated with inotropic agents or vasopressors before enrolment in the study.
At baseline, patients had arterial hypotension, with low CCP and CI values, and high SARi, SVV, PPV, and Eadyn values (Table 2). Infusion of norepinephrine significantly increased SAP, DAP, MAP, PP, CVP, CCP, TPRi, VRi, SVV, SVi, CI, EA, and EV and significantly decreased Eadyn, PPV, CA, and SARi (Table 2). These changes did not differ between non-smoker and smoker patients (p = 0.45).
In the overall study population, Eadyn was significantly and negatively correlated with CCP (r = −0.557, p = 0.0017), CCP-MSP (r = −0.515, p = 0.003), SARi (r = −0.487, p = 0.003), SAP (r = −0.402, p = 0.004), DAP (r = −0.484, p = 0.005), MAP (r = −0.498, p = 0.004), EA (r = −0.347, p = 0.05), CA (r = −0.406, p = 0.021), and TPRi (r = −0.396, p = 0.025; Figures 1, 2). Eadyn was not correlated with LVEF (r = 0.012, p = 0.945).
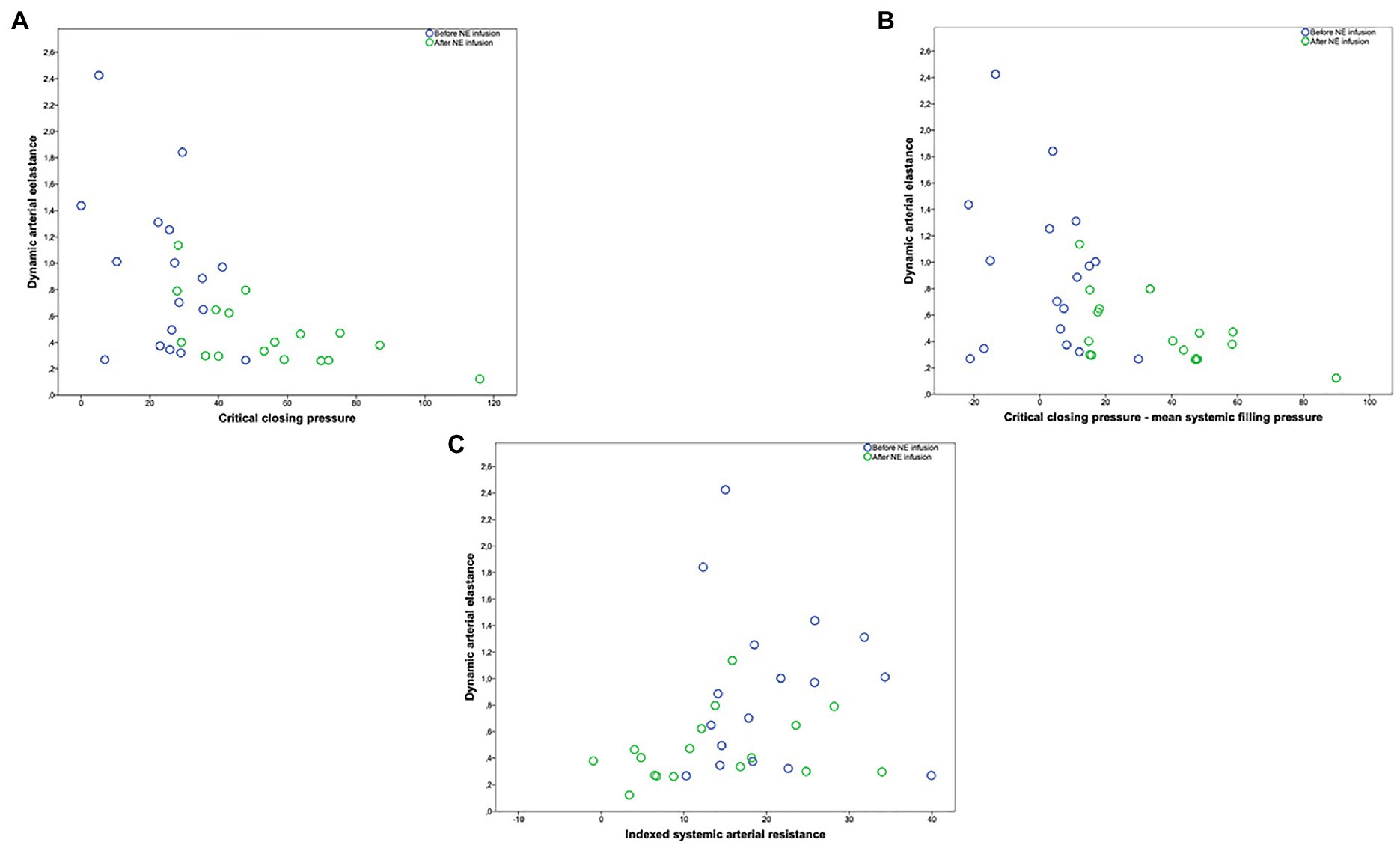
Figure 1. (A) Relationship between dynamic arterial elastance and critical closing pressure (mmHg). (B) Relationship between dynamic arterial elastance and critical closing pressure minus mean systemic filling pressure (mmHg). (C) Relationship between dynamic arterial elastance and indexed systemic arterial resistance (mmHg ml−1·m−2). Circle color refers to before (blue circle)/after norepinephrine (NE) infusion (green circle).
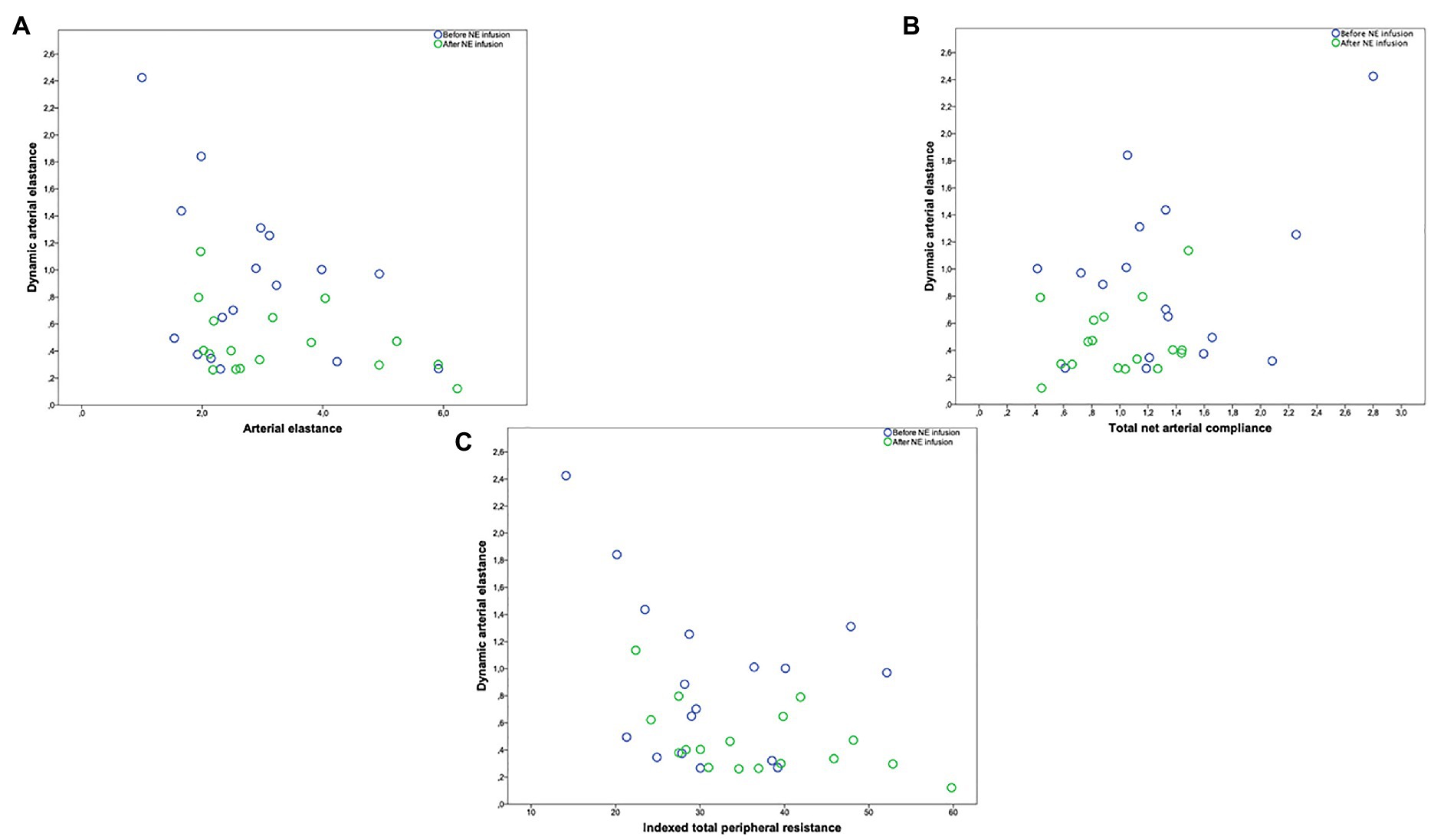
Figure 2. (A) Relationship between dynamic arterial elastance and arterial elastance (mmHg ml−1·m−2). (B) Relationship between dynamic arterial elastance and total net arterial compliance (ml mmHg−1·m−2). (C) Relationship between dynamic arterial elastance and indexed total peripheral resistance (mmHg ml−1·m−2). Circle color refers to before (blue circle)/after norepinephrine (NE) infusion (green circle).
The linear mixed model used to analyze the contribution of arterial and blood flow parameters to Eadyn demonstrated that Eadyn was significantly associated with CA, blood pressure, CCP, and TPRi (Table 3).
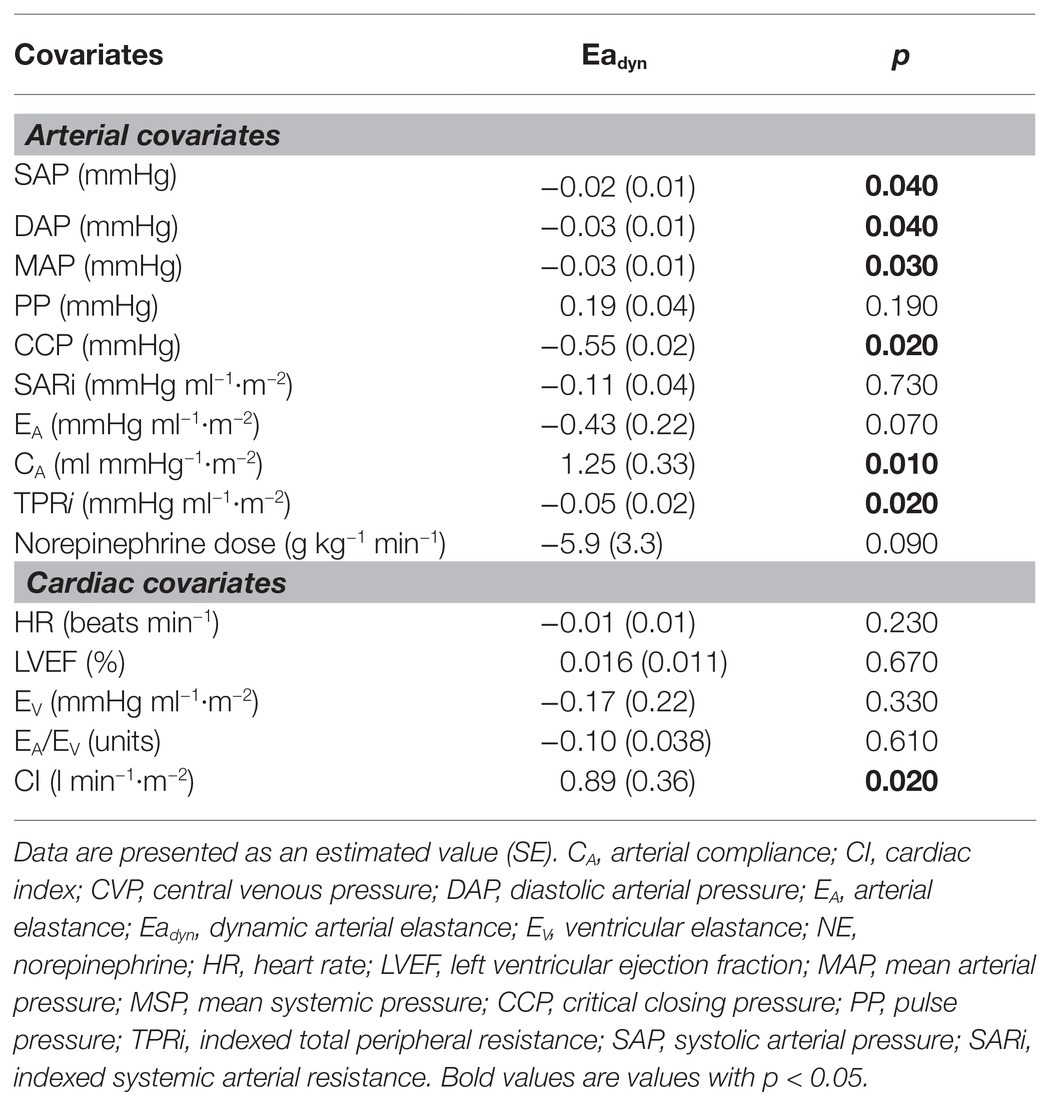
Table 3. Estimated values of fixed effects (arterial load and cardiac parameters) on Eadyn according to a linear mixed-effects model analysis.
Discussion
The present study’s main finding was the association between Eadyn and determinants of the vascular waterfall (CCP and SARi). Furthermore, we confirmed that Eadyn is associated with several components of arterial load (CA and TPRi) and blood pressure (MAP and CCP).
According to the concept of macrocirculatory-microcirculatory coherence, any change in the macrocirculation will affect the microcirculation (Bennett et al., 2018). Hence, resuscitation procedures intended to correct macrocirculatory hemodynamic variables will also modify regional and microcirculatory perfusion and oxygen delivery to the tissues (Ince, 2015). The CCP was described at the microcirculatory level and represents the arterial pressure below which blood flow is stopped by occlusion of the arterioles (Versluis et al., 2001; Maas et al., 2012). The vascular waterfall is thought to maintain tissue perfusion when blood flow is abnormally low. The CCP values observed in the present study were similar to those published in the literature (Jellinek et al., 2000; Schipke et al., 2003; Kottenberg-Assenmacher et al., 2009). As expected, norepinephrine increased CCP, the vascular waterfall (i.e., the CCP-MSP difference), and thus the level of tissue perfusion.
When arterial hypotension occurs, the main adaptive mechanism is the maintenance of blood flow to the tissues. In this respect, it has been suggested that several adaptive hemodynamic factors are activated so as to maintain the blood flow redistribution (De Hert, 2012). The blood pressure increase with norepinephrine treatment might restore the blood pressure and thus diminish the contribution of these hemodynamic factors to changes in tissue perfusion. Hence, we observed a decrease in SARi, which might be due to the redistribution of blood flow to non-perfused capillaries (microvascular recruitment) with norepinephrine, and a decrease in the adaptive phenomena described above (Georger et al., 2010; De Backer et al., 2014).
The changes in Eadyn after norepinephrine infusion contrast with those observed upon norepinephrine weaning in the patients with sepsis or after cardiac surgery (Guinot et al., 2017). Likewise, studies of Eadyn in the operating theater and in the ICU have given conflicting results (Guinot et al., 2015; Lanchon et al., 2017; Bar et al., 2018b). These disparities may be due to several factors, e.g., the non-linearity of the blood pressure-volume relationship (Sanders et al., 1988), the cardiovascular effects of norepinephrine (as an alpha and beta-agonist; Guinot et al., 2018) and the underlying disease (vasoplegia is associated with altered autonomic function; Bernardelli et al., 2016), and thus changes in the interactions between the arterial load and blood pressure. Hence, Eadyn decreased with norepinephrine infusion probably because of a decrease in CA, an increase in left ventricular inotropy (EV), and a decrease in arteriolar vasomotor tone (SARi; Figure 3).
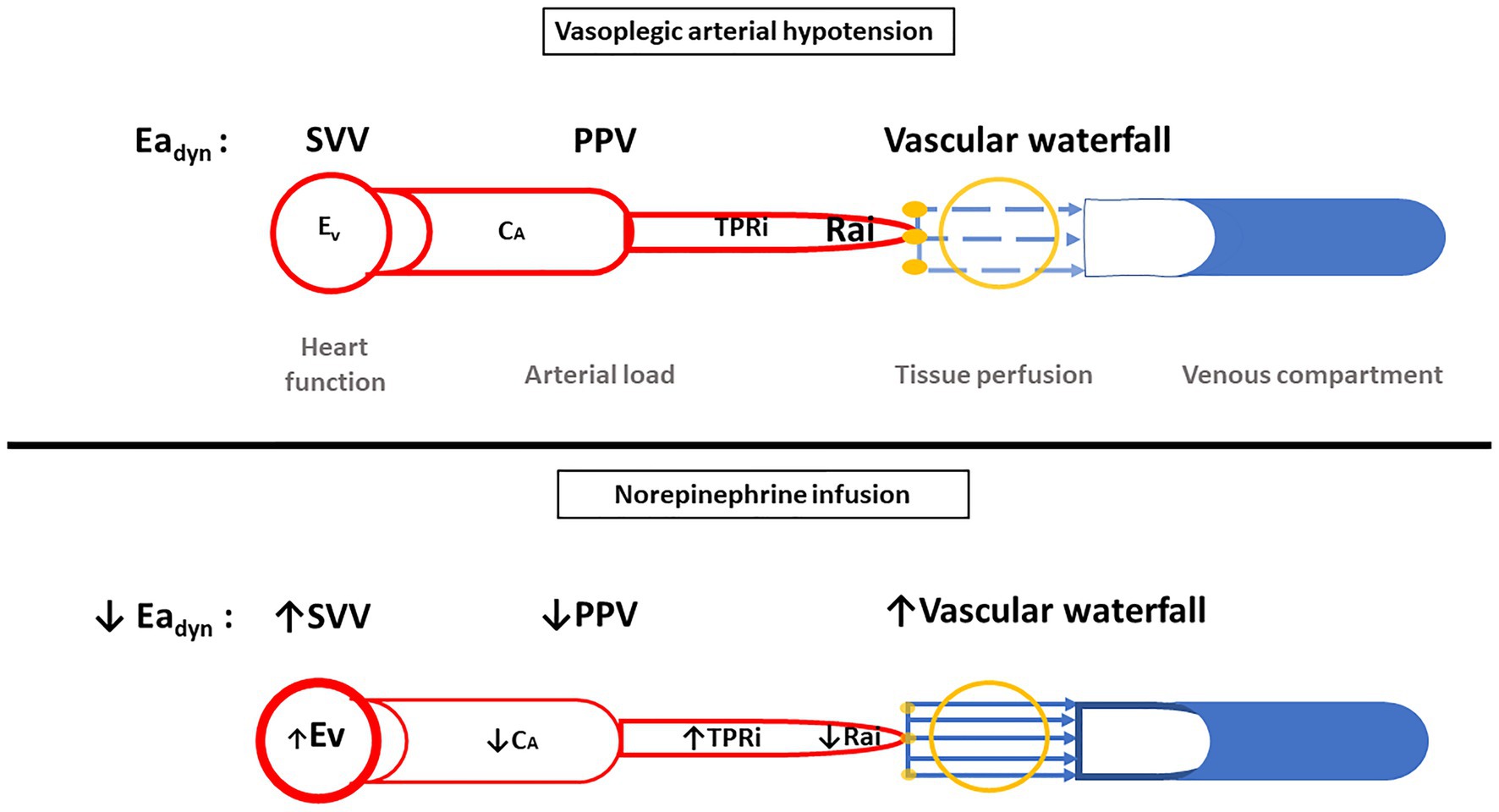
Figure 3. Illustration of the evolution of parameters in cases of vasoplegic arterial hypotension and in case of norepinephrine infusion. CA, arterial compliance; CCP, critical closing pressure; EA, arterial elastance; Eadyn, dynamic arterial elastance; EV, ventricular elastance; PPV, pulse pressure variation; TPRi, indexed total peripheral resistance; Rai, resistance arterial indexed; SVV, stroke volume variation.
As demonstrated by the literature data, CA is probably the main contributor to Eadyn (Monge García et al., 2017; Bar et al., 2018a). It has been shown that norepinephrine infusion can restore CA (Nguyen et al., 2018). Fluid expansion does not change CA in patients treated with norepinephrine but decreases CA in patients not treated with norepinephrine. Likewise, norepinephrine weaning is not associated with a change of CA in non-pressure-responder patients (Bar et al., 2018b). Thus, in norepinephrine-treated patients, changes in the arterial load may mainly be driven by the resistive component (i.e., SARi and TPRi; Bernardelli et al., 2016; Nguyen et al., 2018). One can suppose that in patients treated with vasopressor, Eadyn mainly reflects vascular resistance because CA is fixed. This hypothesis may also explain the ability of Eadyn (when measured with an uncalibrated CO device) to predict arterial pressure changes (Bar et al., 2018b). The arterial load and Eadyn may vary in a complex manner as a function of the therapeutic intervention while decreasing Eadyn’s ability to predict blood pressure changes. The main negative studies concerning Eadyn were performed in the operating theater, and the positive studies were performed in the ICU (i.e., where most of the patients had received vasopressors; Vos et al., 2013; Lanchon et al., 2017; Guarracino et al., 2019). In practice, Eadyn could be considered as an easy-to-read indicator that can help the physician to choose the optimal hemodynamic strategy at the bedside. This index could be used to understand the interaction between cardiac function and arterial load, the effects of hemodynamic treatment on components of arterial load, coherence between macrocirculation and microcirculation (i.e., the vascular waterfall’s status), and thus to determine which treatments could be used or withdrawn. Guarracino et al. (2019) have demonstrated that Eadyn predicts changes in MAP upon fluid expansion or vasopressor administration. In contrast to the complex procedures required to measure the vascular waterfall and arterial load variables, Eadyn is easy to measure with hemodynamic devices – even an uncalibrated pulse contour analysis device (Bar et al., 2018b).
The present study had several limitations. Even though we predetermined the sample size, the present results included a small number of patients and will require external validation in another cohort. Our results were obtained in a group of patients with arterial hypotension treated with norepinephrine and must now be confirmed in patients treated with fluid expansion or inotropes. We did not include a control group, i.e., using IV fluids because fluid infusion may be unable to treat vasoplegia, and it seems difficult to compare the effects of fluid on vasomotor tone and those of norepinephrine in two populations who do not suffer the same hemodynamic underlying disease. To avoid mathematical coupling, we specifically chose to calculate Eadyn from two different signals. The arterial load assessment was based on a two-element Windkessel model and integrative simplification (Westerhof et al., 2009). More precise models (include arterial impedance and wave reflection) have since been developed. However, the performance of these measurements at the bedside would be challenging. The EA calculated from MAP was demonstrated to be a robust surrogate over a wide range of hemodynamic conditions and can be applied to any peripheral artery (Monge Garcia et al., 2018). The method that we used to calculate EV and EA can be criticized because we did not use a high-fidelity catheter for ventricular pressure measurement; however, our approach has been studied previously (Huette et al., 2020; Nguyen et al., 2020). Likewise, we used a standardized inspiratory breath-hold maneuver. This method has been validated in ICU patients, and the values obtained in the present study were similar to those determined with other methods (Maas et al., 2012). Finally, there was heterogeneity in the study participants because their cause for surgery was not the same, but all patients suffer the same hemodynamic disease, and the inclusion criteria allow to homogenize the studied population.
In hypotensive patients treated with norepinephrine, Eadyn may indirectly reflect the vascular waterfall phenomenon and changes in one or both of its determinants (CCP and SARi) following treatment. Hence, one can consider that Eadyn reflects vasomotor tone in hypotensive patients treated with norepinephrine. In view of our present results and the literature data, one can further consider that Eadyn indirectly reflects macro-microcirculatory coherence during acute circulatory failure.
Data Availability Statement
The raw data supporting the conclusions of this article will be made available by the authors, without undue reservation.
Ethics Statement
The studies involving human participants were reviewed and approved by Comité de Protection des Personnes Est II, Besançon, France; reference: 17/07, June 19th, 2017. The patients/participants provided their written informed consent to participate in this study.
Author Contributions
SB, MN, P-GG, OA-A, and BB designed the study and interpreted the data. SB and P-GG analyzed the data. SB, P-GG, and HD drafted the manuscript for important intellectual content. All authors contributed to the article and approved the submitted version.
Conflict of Interest
The authors declare that the research was conducted in the absence of any commercial or financial relationships that could be construed as a potential conflict of interest.
Glossary
References
Abou-Arab, O., Kamel, S., Beyls, C., Huette, P., Bar, S., Lorne, E., et al. (2020). Vasoplegia after cardiac surgery is associated with endothelial glycocalyx alterations. J. Cardiothorac. Vasc. Anesth. 34, 900–905. doi: 10.1053/j.jvca.2019.09.004
Abou-Arab, O., Martineau, L., Bar, S., Huette, P., Amar, A. B., Caus, T., et al. (2018). Postoperative vasoplegic syndrome is associated with impaired endothelial vasomotor response in cardiac surgery: a prospective, observational study. J. Cardiothorac. Vasc. Anesth. 32, 2218–2224. doi: 10.1053/j.jvca.2018.02.006
Asanoi, H., Sasayama, S., and Kameyama, T. (1989). Ventriculoarterial coupling in normal and failing heart in humans. Circ. Res. 65, 483–493. doi: 10.1161/01.RES.65.2.483
Bar, S., Huette, P., Abou-Arab, O., Dupont, H., Lorne, E., and Guinot, P.-G. (2018a). Dynamic arterial elastance might not be an indicator of ventriculo-arterial coupling. Comment on Br J Anaesth 2017; 118: 938–46. Br. J. Anaesth. 121, 672–673. doi: 10.1016/j.bja.2018.03.027
Bar, S., Leviel, F., Abou Arab, O., Badoux, L., Mahjoub, Y., Dupont, H., et al. (2018b). Dynamic arterial elastance measured by uncalibrated pulse contour analysis predicts arterial-pressure response to a decrease in norepinephrine. Br. J. Anaesth. 121, 534–540. doi: 10.1016/j.bja.2018.01.032
Bennett, V. A., Vidouris, A., and Cecconi, M. (2018). Effects of fluids on the macro- and microcirculations. Crit. Care 22:74. doi: 10.1186/s13054-018-1993-1
Bernardelli, A. K., Da Silva, R. d. C. V. d. A. F., Corrêa, T., and Da Silva-Santos, J. E. (2016). Vasoplegia in sepsis depends on the vascular system, vasopressor, and time-point: a comparative evaluation in vessels from rats subjected to the cecal ligation puncture model. Can. J. Physiol. Pharmacol. 94, 1227–1236. doi: 10.1139/cjpp-2015-0514
Bossuyt, P. M., Reitsma, J. B., Bruns, D. E., Gatsonis, C. A., Glasziou, P. P., Irwig, L., et al. (2015). STARD 2015: an updated list of essential items for reporting diagnostic accuracy studies. BMJ 351, 1–9. doi: 10.1136/bmj.h5527
Bouchacourt, J. P., Riva, J. A., and Grignola, J. C. (2013). The increase of vasomotor tone avoids the ability of the dynamic preload indicators to estimate fluid responsiveness. BMC Anesthesiol. 13:41. doi: 10.1186/1471-2253-13-41
Cecconi, M., García, M. I. M., Romero, M. G., Mellinghoff, J., Caliandro, F., Grounds, R. M., et al. (2015). The use of pulse pressure variation and stroke volume variation in spontaneously breathing patients to assess dynamic arterial elastance and to predict arterial pressure response to fluid administration. Anesth. Analg. 120, 76–84. doi: 10.1213/ANE.0000000000000442
De Backer, D., Orbegozo Cortes, D., Donadello, K., and Vincent, J.-L. (2014). Pathophysiology of microcirculatory dysfunction and the pathogenesis of septic shock. Virulence 5, 73–79. doi: 10.4161/viru.26482
De Hert, S. (2012). Physiology of hemodynamic homeostasis. Best Pract. Res. Clin. Anaesthesiol. 26, 409–419. doi: 10.1016/j.bpa.2012.10.004
Fischer, G. W., and Levin, M. A. (2010). Vasoplegia during cardiac surgery: current concepts and management. Semin. Thorac. Cardiovasc. Surg. 22, 140–144. doi: 10.1053/j.semtcvs.2010.09.007
Gaskell, P., and Krisman, A. M. (1958). Critical closing pressure of vessels supplying the capillary loops of the nailfold. Circ. Res. 6, 461–467. doi: 10.1161/01.RES.6.4.461
Georger, J.-F., Hamzaoui, O., Chaari, A., Maizel, J., Richard, C., and Teboul, J.-L. (2010). Restoring arterial pressure with norepinephrine improves muscle tissue oxygenation assessed by near-infrared spectroscopy in severely hypotensive septic patients. Intensive Care Med. 36, 1882–1889. doi: 10.1007/s00134-010-2013-3
Guarracino, F., Bertini, P., and Pinsky, M. R. (2019). Cardiovascular determinants of resuscitation from sepsis and septic shock. Crit. Care 23:118. doi: 10.1186/s13054-019-2414-9
Guinot, P.-G., Abou-Arab, O., Guilbart, M., Bar, S., Zogheib, E., Daher, M., et al. (2017). Monitoring dynamic arterial elastance as a means of decreasing the duration of norepinephrine treatment in vasoplegic syndrome following cardiac surgery: a prospective, randomized trial. Intensive Care Med. 43, 643–651. doi: 10.1007/s00134-016-4666-z
Guinot, P.-G., Bernard, E., Levrard, M., Dupont, H., and Lorne, E. (2015). Dynamic arterial elastance predicts mean arterial pressure decrease associated with decreasing norepinephrine dosage in septic shock. Crit. Care 19:14. doi: 10.1186/s13054-014-0732-5
Guinot, P.-G., Longrois, D., Kamel, S., Lorne, E., and Dupont, H. (2018). Ventriculo-arterial coupling analysis predicts the hemodynamic response to norepinephrine in hypotensive postoperative patients: a prospective observational study. Crit. Care Med. 46, e17–e25. doi: 10.1097/CCM.0000000000002772
Huette, P., Abou-Arab, O., Longrois, D., and Guinot, P.-G. (2020). Fluid expansion improve ventriculo-arterial coupling in preload-dependent patients: a prospective observational study. BMC Anesthesiol. 20:171. doi: 10.1186/s12871-020-01087-7
Ince, C. (2015). Hemodynamic coherence and the rationale for monitoring the microcirculation. Crit. Care 19, 1–13. doi: 10.1186/cc14726
Jellinek, H., Krenn, H., Oczenski, W., Veit, F., Schwarz, S., and Fitzgerald, R. D. (2000). Influence of positive airway pressure on the pressure gradient for venous return in humans. J. Appl. Physiol. 88, 926–932. doi: 10.1152/jappl.2000.88.3.926
Kottenberg-Assenmacher, E., Aleksic, I., Eckholt, M., Lehmann, N., and Peters, J. (2009). Critical closing pressure as the arterial downstream pressure with the heart beating and during circulatory arrest. Anesthesiology 110, 370–379. doi: 10.1097/ALN.0b013e3181942c99
Ky, B., French, B., May Khan, A., Plappert, T., Wang, A., Chirinos, J. A., et al. (2013). Ventricular-arterial coupling, remodeling, and prognosis in chronic heart failure. J. Am. Coll. Cardiol. 62, 1165–1172. doi: 10.1016/j.jacc.2013.03.085
Lanchon, R., Nouette-Gaulain, K., Stecken, L., Sesay, M., Lefrant, J.-Y., and Biais, M. (2017). Dynamic arterial elastance obtained using arterial signal does not predict an increase in arterial pressure after a volume expansion in the operating room. Anaesthesia Critical Care & Pain Medicine 36, 377–382. doi: 10.1016/j.accpm.2017.05.001
Levin, M. A., Lin, H.-M., Castillo, J. G., Adams, D. H., Reich, D. L., and Fischer, G. W. (2009). Early on-cardiopulmonary bypass hypotension and other factors associated with vasoplegic syndrome. Circulation 120, 1664–1671. doi: 10.1161/CIRCULATIONAHA.108.814533
Maas, J. J., de Wilde, R. B., Aarts, L. P., Pinsky, M. R., and Jansen, J. R. (2012). Determination of vascular waterfall phenomenon by bedside measurement of mean systemic filling pressure and critical closing pressure in the intensive care unit. Anesth. Analg. 114, 803–810. doi: 10.1213/ANE.0b013e318247fa44
Monge Garcia, M. I., Gil Cano, A., and Gracia Romero, M. (2011). Dynamic arterial elastance to predict arterial pressure response to volume loading in preload-dependent patients. Crit. Care 15:R15. doi: 10.1186/cc9420
Monge García, M. I., Guijo González, P., Gracia Romero, M., Gil Cano, A., Rhodes, A., Grounds, R. M., et al. (2017). Effects of arterial load variations on dynamic arterial elastance: an experimental study. Br. J. Anaesth. 118, 938–946. doi: 10.1093/bja/aex070
Monge Garcia, M. I., Jian, Z., Settels, J. J., Hatib, F., Cecconi, M., and Pinsky, M. R. (2018). Reliability of effective arterial elastance using peripheral arterial pressure as surrogate for left ventricular end-systolic pressure. J. Clin. Monit. Comput. 33, 803–813. doi: 10.1007/s10877-018-0236-y
Nguyen, M., Abou-Arab, O., Bar, S., Dupont, H., Bouhemad, B., and Guinot, P.-G. (2021). Echocardiographic measure of dynamic arterial elastance predict pressure response during norepinephrine weaning: an observational study. Sci. Rep. 11:2853. doi: 10.1038/s41598-021-82408-9
Nguyen, M., Berhoud, V., Bartamian, L., Martin, A., Ellouze, O., Bouhemad, B., et al. (2020). Agreement between different non-invasive methods of ventricular elastance assessment for the monitoring of ventricular–arterial coupling in intensive care. J. Clin. Monit. Comput. 34, 893–901. doi: 10.1007/s10877-019-00397-7
Nguyen, M., Fischer, M.-O., Mallat, J., Marc, J., Lorne, E., Bouhemad, B., et al. (2018). Fluid responsiveness depend on arterial load and vasopressor usein ICU patients. ESICM LIVES 2018: Paris, France. 20-24 October 2018. Intensive Care Medicine Experimental 6:59. doi: 10.1186/s40635-018-0201-6
Pinsky, M. R. (2011). Defining the boundaries of bedside pulse contour analysis: dynamic arterial elastance. Crit. Care 15:120. doi: 10.1186/cc9986
Sanders, J. S., Ferguson, D. W., and Mark, A. L. (1988). Arterial baroreflex control of sympathetic nerve activity during elevation of blood pressure in normal man: dominance of aortic baroreflexes. Circulation 77, 279–288. doi: 10.1161/01.CIR.77.2.279
Schipke, J. D., Heusch, G., Sanii, A. P., Gams, E., and Winter, J. (2003). Static filling pressure in patients during induced ventricular fibrillation. Am. J. Phys. Heart Circ. Phys. 285, H2510–H2515. doi: 10.1152/ajpheart.00604.2003
Shmuel, S., Redfield, M. M., From, A. M., Paul, S., Nishimura, R. A., and Borlaug, B. A. (2012). Effects of vasodilation in heart failure with preserved or reduced ejection fraction. J. Am. Coll. Cardiol. 59, 442–451. doi: 10.1016/j.jacc.2011.09.062
Tanaka, K., Oshita, S., Kitahata, H., Kimura, H., Kawahito, S., Park, Y. C., et al. (1998). Effects of nicardipine on ventriculo-arterial coupling in humans. Br. J. Anaesth. 81, 180–185. doi: 10.1093/bja/81.2.180
Versluis, J. P., Heslinga, J. W., Sipkema, P., and Westerhof, N. (2001). Microvascular pressure measurement reveals a coronary vascular waterfall in arterioles larger than 110 μm. Am. J. Phys. Heart Circ. Phys. 281, H1913–H1918. doi: 10.1152/ajpheart.2001.281.5.H1913
Vos, J. J., Kalmar, A. F., Struys, M. M. R. F., Wietasch, J. K. G., Hendriks, H. G. D., and Scheeren, T. W. L. (2013). Comparison of arterial pressure and plethysmographic waveform-based dynamic preload variables in assessing fluid responsiveness and dynamic arterial tone in patients undergoing major hepatic resection. Br. J. Anaesth. 110, 940–946. doi: 10.1093/bja/aes508
Keywords: dynamic arterial elastance, norepinephrine, waterfall phenomenon, vascular resistance, cardiac output
Citation: Bar S, Nguyen M, Abou-Arab O, Dupont H, Bouhemad B and Guinot P-G (2021) Dynamic Arterial Elastance Is Associated With the Vascular Waterfall in Patients Treated With Norepinephrine: An Observational Study. Front. Physiol. 12:583370. doi: 10.3389/fphys.2021.583370
Edited by:
Lacolley Patrick, Institut National de la Santé et de la Recherche Médicale (INSERM), FranceReviewed by:
Dimitrios Terentes-Printzios, University of Oxford, United KingdomThomas Desaive, University of Liège, Belgium
Copyright © 2021 Bar, Nguyen, Abou-Arab, Dupont, Bouhemad and Guinot. This is an open-access article distributed under the terms of the Creative Commons Attribution License (CC BY). The use, distribution or reproduction in other forums is permitted, provided the original author(s) and the copyright owner(s) are credited and that the original publication in this journal is cited, in accordance with accepted academic practice. No use, distribution or reproduction is permitted which does not comply with these terms.
*Correspondence: Stéphane Bar, stephane.bar.sb@gmail.com