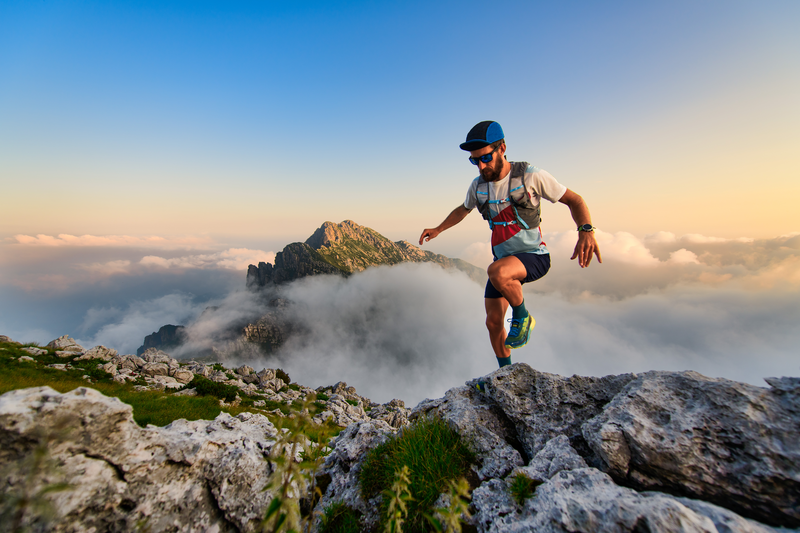
95% of researchers rate our articles as excellent or good
Learn more about the work of our research integrity team to safeguard the quality of each article we publish.
Find out more
ORIGINAL RESEARCH article
Front. Phys. , 13 July 2023
Sec. Interdisciplinary Physics
Volume 11 - 2023 | https://doi.org/10.3389/fphy.2023.1228006
This article is part of the Research Topic Lost Circulation Control During Drilling and Completion in Complex Formations View all 10 articles
For unconventional oil and gas reservoirs and deep carbonate reservoirs, temporary plugging fracturing is an important technology to improve oil and gas production. At present, the research of temporary plugging fracturing is mainly focused on the development of high-performance temporary plugging agent, the simulation of fracture growth morphology and the test of plugging ability of temporary plugging agent. There is limited research on the migration law of temporary plugging agents in fractures, which affects the optimization of temporary plugging processes and parameters. Through a crack sealing experimental device, taking into account the influence of rough cracks and crack width, a temporary plugging agent sealing pressure test was conducted. The sealing pressure and the thickness of the dense layer formed by the temporary plugging agent were used to quantitatively characterize the migration law of the temporary plugging agent. This article elucidates the influence of different types, combinations, and concentrations of temporary plugging agents on the sealing pressure. The research results show that the granular temporary plugging agent has better plugging ability than fiber, and it is easier to plug the fractures with small width. Increasing the concentration of temporary plugging agent is beneficial to plugging fractures, and high concentration of temporary plugging agent can achieve higher plugging pressure compared with low concentration of temporary plugging agent. At the same concentration, the plugging pressure of the temporary plugging agent combined with fiber and small particles is lower than that of the temporary plugging agent combined with fiber, small particles and large particles. Increasing the fiber concentration in the composite temporary plugging agent can effectively increase the temporary plugging pressure and shorten the pressure starting time. Compared to smooth fractures, temporary plugging agents are more likely to accumulate and seal in rough fractures. Temporary plugging fracturing technology has been widely used in unconventional fields such as tight gas and shale oil in the Sichuan Basin. The analysis of fracturing injection pressure data shows that fractures will turn several times in the formation after the temporary plugging agent is added to the fracturing fluid, thus increasing the stimulation range.
China has a large number of unconventional oil and gas resources, and unconventional resources such as tight gas, shale oil and shale gas are playing an increasingly important role in oil and gas production [1–3]. Unconventional oil and gas reservoirs are often characterized by low porosity, low permeability and strong heterogeneity. Hydraulic fracturing is an important technology to improve the utilization of unconventional oil and gas resources [4–8]. Affected by reservoir heterogeneity and mutual interference between fractures, hydraulic fractures often expand unevenly, which seriously affects oil and gas production [9]. Improving the uniformity of multiple fractures is the key to unconventional fracturing technology. By adding temporary plugging agent to the fracturing fluid, temporary plugging fracturing can form a plugging layer in the fracture, change the flow direction of subsequent fracturing fluid, and open a new hydraulic fracture in the horizontal section [10, 11]. This technology can greatly improve the uniform expansion of the fracture, thus improving the stimulation effect. Temporary plugging fracturing can form a plugging layer in the fracture by adding temporary plugging agent to the fracturing fluid, forcing the subsequent fracturing fluid to change the flow direction and open a new hydraulic fracture in the horizontal section [12, 13]. This technology can greatly improve the uniform expansion of the fracture, thus improving the stimulation effect.
Yang Wang et al. [14] evaluated the applicability of fiber and granular temporary plugging agent in acid fracturing of ultra-deep carbonate reservoir, and optimized the formula of temporary plugging agent with plugging pressure greater than 15 MPa. Yang Wang et al. [15] proposed a compound staged acid fracturing method for horizontal wells using packers and temporary plugging agents, which greatly increased the stimulation range by adding temporary plugging agents several times. Lishan Yuan et al. [16] used 3D printing technology to create rough fractures and evaluated the migration law of 1 mm fibers and particles in the fractures, and the experimental results shows that fibers and particles are easy to accumulate in the narrow area of fractures. Wei Feng et al. [17] proposed new parameters to analyze fracture morphology and introduced an integrated experimental method as well to study plugging behavior within fractures with different morphologies. Hao Qin et al. [18] used the coupling method of computational fluid dynamics (CFD) and discrete element method (DEM) to establish the mathematical model of temporary plugging agent migration, through which the migration law of temporary plugging agent in artificial fractures during temporary plugging and fracturing of hot and dry rocks can be accurately grasped. Daobing Wang et al. [19] first present a comprehensive workflow to model hydraulic fracture by accounting for interactions with numerous crosscutting natural fracture or joint sets, as well as the effect of temporary plugging in opened fractures. This model is a fully coupled seepage flow in porous media, fluid flow in fractures, and rock deformation finite element model with adaptive insertion of cohesive elements as crosscutting natural fracture or joint sets. Bo Wang et al; [20] modified the commonly used true triaxial hydraulic fracturing system to study the influence of various factors in the process of diversion on the injection pressure response and fracture geometry. According to the physical and mechanical parameters of tight rocks, Yin Zhang et al; [21] poured artificial simulated rock samples (AARS) of sandstone reservoirs in Shengli Oilfield. The true triaxial TP fracturing test under different TP is concentrated on AARS. With the aid of nano-boron crosslinker (NBC) and sleeve, the initiation and propagation of hydraulic fractures are monitored by acoustic emission (AE) system. Chen Yang et al. [22] established a large-scale visual experimental system based on similarity criteria, which can observe the dynamic plugging performance of fibers and particles. The experimental results show that the plugging process begins with the fiber adhering to the bottom, top and surface of the crack, and then the attached fiber continuously captures the flowing particles to form a dispersed blocking area, and finally forms a flow channel and gradually narrows until it is completely blocked. The fiber causes plugging, and the particles as the skeleton accelerate the subsequent plugging process. Lufeng Zhang et al; [23] carried out experimental research on plugging behavior of degradable fibers and particles in acid-etched fractures, and the results showed that the fracture surface morphology affected the formation time of temporary plugging, but did not affect whether temporary plugging was formed. Ahmed M. Gomaa et al; [24] evaluated the temporary plugging agent suitable for far field (FF) and near well (NW) applications through bridging test, filling permeability test and dissolution test under static and dynamic conditions. Based on Jimusar shale, Minghui Li et al. [25] investigated the plugging performance of fibers and particles and diverting fracturing behavior under three different TPDF application scenarios: near-wellbore in-plane TPDF, multistage TPDF in the horizontal wellbore, and far-field TPDF within fractures. C. E. Cohen et al; [26] developed an acid containing fiber and has been successfully used in matrix acidizing of highly heterogeneous carbonate formations. The fiber is designed to be inert under surface and pumping conditions. Its geometry enables it to form a strong and stable fiber network, which can effectively cross natural cracks, wormholes, and perforated tunnels. Finally, the fiber degrades into water-soluble organic liquid, which will return to the surface during the reflux process. Vanessa Williams et al; [27] proposed an acid fracturing technology that uses solid particles to improve the stimulation effect and enhance the far-field conductivity. This method can not only enhance the conductivity of fractures, but also increase the complexity of fractures. Wang Liwei et al; [28] used the physical simulation method and combined with the on-site temporary plugging process to study the indoor verification of the opening and coupling extension of natural fractures at different levels. The experimental results show that the fracture aperture has a great influence on the temporary plugging steering pressure, and not all fractures with any apertures can be temporarily plugged and steered into new fractures. Ruxin Zhang et al; [29] used five shale outcrops to carry out large-scale real triaxial temporary plugging fracturing simulation research, and the results showed that the temporary plugging agent effectively plugs up induced fractures at two different positions, which are its heel or tip, and results in three main fracture diversion patterns: fracture diversion at the old fracture heel, fracture diversion with an old fracture, and a new fracture induced at a new position. Based on past experience, Mary S. Van Domelen et al; [30] optimized modern diversion practices with self degradable particles and developed diverter design guidelines that provide a basis for temporary plugging fracturing design. The research of temporary plugging fracturing mainly focuses on two aspects: performance evaluation of temporary plugging agent and numerical simulation of fracture propagation, among which performance evaluation of temporary plugging agent is an important component of temporary plugging fracturing technology. The performance evaluation of temporary plugging agents often uses smooth steel plates instead of fracturing fractures to test the plugging pressure of temporary plugging agents under different width fracture conditions. However, the fracturing fracture walls in the formation are actually not smooth, and they often present a rough and uneven state. Therefore, using smooth steel plates to conduct experiments has certain errors, which cannot accurately characterize the impact of rough walls on the plugging process of temporary plugging agents. Chengyuan Xu et al; [31] used a microscopic visualization experimental device formed by the blockage zone to observe the dynamic blockage performance of spherical materials, sheet materials, and fibers in fractures. Chengyuan Xu et al; [32] used the microscopic visualization experimental device formed by the crack plugging zone to analyze the plugging behavior of different types and different concentrations of irregular shape plugging materials in the fracture. The experimental results show that the main factors affecting the formation of fracture plugging zone are flatness, roundness, convexity and concentration. Huang Liuke et al; [33] studied the effects of rock intrinsic heterogeneity and grain size on the initiation and propagation of hydraulic fractures under different propagation modes by using a two-dimensional discrete element model. Yixin Chen et al; [34] proposed the liquid solid phase transition self-generated proppant fracturing fluid system (LSPCAP), which converts into solid particles at formation temperature to resist closure stress in fractures. Daobing Wang et al. [35] established a discrete network model of complex fracture distribution in HDR reservoirs. The numerical simulation results show that the main factors affecting the thermal recovery efficiency of HDR reservoirs include fracture width, fracture density, fracture permeability and matrix permeability. Daobing Wang et al. [36] used the extended finite element method to study the repeated fracture propagation paths at different initiation angles. The rich function method and magic mode technique are introduced into the repeated crack model, which ensures that the repeated crack can spread freely on the structural grid without any refinement near the crack tip.
Through literature research, it can be found that the research on temporary plugging fracturing of Shale oil mainly focuses on the development of high-performance temporary plugging agent, numerical simulation of fracture growth, and the distribution of temporary plugging agent in the petrophysical model, lacking the research on the migration law of temporary plugging agent in fractures. It is necessary to accurately understand the migration law of temporary plugging agents in rough fractures, as it can provide important basis for the selection of temporary plugging materials and the parameter design of temporary plugging fracturing.
Through a fracture plugging experimental device, taking into account the impact of rough fractures and fracture widths on temporary plugging effectiveness, this paper conducted a plugging pressure test of temporary plugging agents, demonstrating in detail the impact of different types, combinations, and concentrations of temporary plugging agents on plugging pressure, and revealing the migration and bridging process of temporary plugging agents under rough fracture conditions. This study has guiding significance for improving temporary plugging fracturing technology, which has been widely used in unconventional oil and gas reservoirs such as shale oil, tight gas, and shale gas in the Sichuan Basin, achieving good stimulation effects and improving the development effect of gas reservoirs.
Sichuan Basin is rich in conventional and unconventional oil and gas resources [37]. Its main shale gas production layer is the Wufeng Formation-Longmaxi Formation. Shale oil is mainly distributed in the Jurassic Daanzhai and Lianggaoshan Formations [38], and the tight gas production layer is the Jurassic Shaximiao Formation [39]. The unconventional oil and gas resources in the Sichuan Basin are characterized by low porosity, low permeability, and strong heterogeneity [40, 41]. Hydraulic fracturing is an important technology to enhance unconventional oil and gas production.
Taking the Jurassic shale oil as an example, the Jurassic reservoir is mainly composed of limestone and sandstone, with an average porosity of 5.7%, an average permeability is 0.42 mD, organic carbon content of 1.2%–2.4%, movable hydrocarbon content of 0.6 mg/g–2.5 mg/g, and brittle mineral content of 50%–60%. The compressive strength of shale is 165 MPa, the compressive strength of sandstone is 415 MPa, the elastic modulus of shale is 5.2 × 104MPa, the elastic modulus of sandstone is 6.1 × 104MPa, the horizontal stress difference of reservoir is 6.6–10.4 MPa, and the stress difference coefficient is 0.14–0.18. As can be seen from Figure 1, the reservoir structure of Well XQ1 is composed of residual intergranular pores, clay mineral intergranular pores, and microfractures.
This experiment uses a crack sealing tester, which is composed of an injection system, a fracture simulation system, and a data acquisition system. The injection system consists of a high-pressure displacement pump, with an upper limit of 300 mL/min. The fracture simulation system uses a set of parallel steel plates to simulate fracturing cracks, and simulates fractures of different widths by adjusting the gap between two steel plates. Because the fracture wall in the formation is rough, in order to truly simulate the rough fracture in the formation, we first obtained the fracture wall data of Shale oil core through 3D scanning technology, and then polished the steel plate according to the obtained 3D laser scanning data, so as to ensure that the roughness of the simulated fracture is consistent with that of the fracture in the real formation. The data collection system mainly records the injection pressure and displacement in real-time through high-performance pressure sensors.
Figure 2 is a scanned cloud map of the wall of a hydraulic fracturing fracture. From this image, it can be seen that the wall of the fracturing fracture is not smooth, but rather very rough and uneven. Using rough wall fractures for experiments is helpful to truly reflect the migration law of temporary plugging agents in fracturing fractures. We create a simulated crack system based on the crack wall data in Figure 2.
Linear gel is a commonly used fracturing fluid, mainly composed of guar gum and water. In this experiment, a linear gel with a viscosity of 30 mPas was selected as the carrier fluid, and fibers and granular temporary plugging agents of different concentrations and proportions were mixed in the linear gel. The mixed liquid was injected into the simulated fracture through a displacement pump, and the injection pressure and displacement were recorded in real-time through a data acquisition system. The chemical composition of fiber, 100 mesh and 10/40 mesh particles in the experiment is polyvinyl alcohol resin modified by poly lotion. The length of the fiber is 6 mm, the diameter of 100 mesh particles is 0.15 mm, and the diameter of 10/40 mm particles is 0.425 mm–2 mm. The density of fibers and particles is 1.26 g/cm3, and the above temporary plugging agents were all purchased from Southwest Oil and Gas Field Company.
We inject 0.5% fiber, 0.5% 100 mesh particulate temporary plugging agent, and 0.5% 10/40 mesh particulate temporary plugging agent into a simulated rough fracture with a width of 4 mm through an injection system, while recording the plugging pressure through a data acquisition system.
As can be seen from Figure 3 and Table 1, compared to fiber and 10/40 mesh granular temporary plugging agent, the peak plugging pressure of the 100 mesh granular temporary plugging agent is the highest, and the plugging pressure increases rapidly after the injection of the fiber, with the shortest pressure build-up time. The peak plugging pressure of 10/40 mesh granular temporary plugging agent can reach 6.78 MPa, which is 136% larger than the peak fiber plugging pressure.
TABLE 1. Comparison of plugging peak pressure and starting time of different forms of temporary plugging agents.
Compared to fiber and 100 mesh granular temporary plugging agent, the reason why 10/40 mesh granular temporary plugging agent has the best plugging effect is that large particle temporary plugging agent has a good match with the fracture [42], which can quickly fill and accumulate at the fracture opening to form a sealing layer, thereby increasing the plugging pressure and shortening the plugging pressure rise time.
We injected different concentrations of fiber, 100 mesh granular temporary plugging agent, and 10/40 mesh granular temporary plugging agent into the simulated rough fractures, with a fracture width of 4 mm and a carrier fluid injection displacement of 250 mL/min. In the experiment, the plugging pressure data was recorded by the data acquisition system. After the experiment, the parallel steel plate used to simulate the crack was opened, and the thickness of the dense layer formed by the temporary plugging agent in the crack was measured by the vernier caliper. The migration of the temporary plugging agent in the fracture is judged by the plugging pressure and the thickness of the dense layer.
As can be seen from Figure 4, as the fiber concentration increases from 0.5% to 1.5%, the peak plugging pressure of the fiber increases, but the increase in plugging pressure is not significant. Especially when the fiber concentration is 1.5%, the peak plugging pressure is 3.71 MPa, which is only 0.84 MPa more than the peak plugging pressure when the fiber concentration is 0.5%. Shale oil and gas reservoirs in Sichuan Basin have strong heterogeneity, with a horizontal two-way stress difference of over 10 MPa. The plugging pressure of fibers is low and cannot meet the requirements for temporary plugging and fracturing of shale oil and gas reservoirs.
As can be seen from Table 2, with the increase in fiber concentration, the peak plugging pressure gradually increases, while the thickness of the sealing layer gradually increases. The fiber concentration has a significant impact on the performance of the plugging layer. The sealing layer formed by compaction of high concentration fibers is very dense, and the pressure bearing capacity of the sealing layer is significantly improved.
As can be seen from Figure 5, with the concentration of 100 mesh granular temporary plugging agent increasing from 0.5% to 1.5%, the peak plugging pressure does not increase significantly. Especially, the peak plugging pressure of 100 mesh granular temporary plugging agent with a concentration of 1.5% is only 0.91 MPa higher than the peak plugging pressure of 100 mesh granular temporary plugging agent with a concentration of 0.5%, and the peak plugging pressure of 100 mesh granular temporary plugging agent with a high concentration is still very low, which is only 5.67 MPa, It still cannot meet the requirements for temporary plugging and fracturing of shale oil and gas reservoirs in the Sichuan Basin.
FIGURE 5. Plugging pressure curve of 100 mesh particles plugging agents with different concentrations.
As can be seen from Figure 6, under the same concentration, the plugging pressure of 10/40 mesh granular temporary plugging agent is much greater than that of fiber and 100 mesh granular temporary plugging agent. The plugging pressure of 10/40 mesh granular temporary plugging agent with a concentration of 1.5% is the highest, which can reach 9.42 MPa. However, temporary plugging fracturing of shale oil and gas reservoirs in Sichuan Basin requires a plugging pressure of more than 15 MPa, and using fiber or particles cannot meet the reservoir stimulation requirements.
Compared to fiber and 100 mesh granular temporary plugging agent, the reason why the plugging pressure of 10/40 mesh granular temporary plugging agent is high is that the temporary plugging agent has a large size and can quickly form accumulation at the simulated fracture opening with a width of 4 mm to improve the plugging pressure. However, due to the obvious gap between various particles in the accumulation process of granular temporary plugging agents (Figure 7), the plugging layer formed by the granular temporary plugging agent has obvious gaps, which makes the liquid easily flow through the gaps, resulting in the low plugging pressure of the granular temporary plugging agent.
Tight gas, shale oil, and ultra-deep carbonate reservoirs in the Sichuan Basin have strong heterogeneity and large stress differences. Temporary plugging fracturing requires high plugging performance of temporary plugging agents. Neither fiber nor granular temporary plugging agent can meet the requirements of temporary plugging and fracturing in the Sichuan Basin. Composite fiber and granular temporary plugging agent can improve the plugging pressure [43]. This paper evaluates the plugging effect of composite fiber and granular temporary plugging agent by plugging pressure testing.
We maintain the concentration of temporary plugging agent at 2% and adjust the proportion of fiber, 100 mesh granular temporary plugging agent, and 10/40 mesh granular temporary plugging agent. The experiment injected a mixture of fiber and granular temporary plugging agent into the simulated rough fractures, and recorded the change in plugging pressure in real time. The fracture width was 4 mm, and the carrier liquid injection displacement was 250 mL/min.
From Figure 8 and Table 3, it can be seen that the plugging pressure increases significantly after mixing fibers and particles together, especially when the proportion of granular temporary plugging agents is high. As the concentration of 10/40 mesh granular temporary plugging agent increases, the peak plugging pressure of the dense layer formed by the mixture of fiber and granular temporary plugging agent significantly increases, especially when the concentration of 10/40 mesh granular temporary plugging agent is 1.5%, the peak plugging pressure is 37.5% higher than the peak plugging pressure when the concentration of 10/40 mesh granular temporary plugging agent is 0.5%.
The strength of the sealing layer is characterized by the sealing pressure, and the higher the sealing pressure, the greater the strength of the sealing layer. As can be seen from Figure 9, the mixture of fiber and granular temporary plugging agent forms a very thick dense layer at the fracture opening, in which the fiber and granular temporary plugging agent are wrapped together, especially in the interior of the fracture, where a large amount of the mixture of fiber and granular temporary plugging agent is also filled. By comparing Figure 7, it can be seen that if only granular temporary plugging agents are used, there are obvious gaps between the particles, and using a mixture of fibers and particles can well compensate for this disadvantage. Fibers capture granular temporary plugging agents like fishing nets. After the granular temporary plugging agent is wrapped in fibers, the strength of the plugging layer is further improved, while fibers can be filled in the gaps between the granular temporary plugging agents, which improves the compactness of the plugging layer. The plugging pressure formed by fibers and particles is higher than that of a single fiber or particle, mainly because when fibers and particles are mixed together, they can wrap and fill each other, thereby reducing the gap in the sealing layer.
FIGURE 9. Plugging pressure curve of composite fiber and granular temporary plugging agent. (A). Image of the accumulation of a mixture of 10/40 mesh granular temporary plugging agent and fibers at the fracture opening. (B). Image of the accumulation of a mixture of 10/40 mesh granular temporary plugging agent and fibers inside the fracture.
We used a mixture of fiber, 100 mesh granular temporary plugging agent, and 10/40 mesh granular temporary plugging agent to conduct plugging tests. The plugging layer consists of the above three temporary plugging agents. We adjusted the ratio of fiber, 100 mesh granular temporary plugging agent, and 10/40 mesh granular temporary plugging agent to test the plugging performance of different combinations of temporary plugging agents. In the experiment, the simulated fracture width is 4 mm, and the carrier liquid injection displacement is 250 mL/m.
As can be seen from Figure 10, when the concentration of fiber is 0.5%, increasing the concentration of 10/40 mesh granular temporary plugging agent is beneficial to improving the plugging pressure, especially when the concentration of 10/40 mesh granular temporary plugging agent increases from 0.5% to 1.0%, the peak plugging pressure increases from 13.54 MPa to 18.12 MPa. In the experiment, the fiber concentration was increased from 0.5% to 1.0%, and the peak plugging pressure was increased from 18.12 MPa to 19.86 MPa. The peak plugging pressure was not significantly increased after increasing the fiber concentration, but the pressure onset time was shortened from 20 s to 10 s. Increasing the fiber concentration is conducive to the formation of a network structure between fibers, which can quickly capture granular temporary plugging agents and shorten the formation time of the plugging layer.
We injected a mixture of 100 mesh temporary plugging agent with a concentration of 0.1% and 10/40 mesh temporary plugging agent with a concentration of 0.1% into the simulated rough fractures. The widths of fractures are 2 mm, 4 mm, and 6 mm, respectively, while carefully recording the changes in plugging pressure. The displacement of this experiment is 250 mL/min.
As can be seen from Figure 11, temporary plugging agents have a better sealing effect on narrow fractures. As the fracture width decreases, the peak sealing pressure increases rapidly, and the pressure onset time becomes shorter. During the plugging process of temporary plugging agent, the larger the width of the fracture, the larger the cross section of temporary plugging agent migration, and the greater the difficulty of temporary plugging agent bridging. In order to achieve rapid and high-strength plugging, it is recommended to reduce the injection displacement before injecting temporary plugging agent in acid fracturing, thereby reducing the fracture width, and improving the plugging pressure and effectiveness.
The wall of a fracture formed by hydraulic fracturing is rough and uneven. We use two types of roughness to simulate the fracture for experiments, one is a simulation fracture with a smoother wall surface, and the other is a simulation fracture with a very rough wall surface. Through experiments, we evaluate the impact of fracture roughness on the plugging effect of temporary plugging agents. The experiment uses a mixture of 0.5% fiber, 1.0% 100 mesh granular temporary plugging agent, and 0.5% 10/40 mesh granular temporary plugging agent. The simulated fracture width is 4 mm, and the injected liquid displacement is 250 mL/min.
As can be seen from Figure 12, compared to the smooth fractures, temporary plugging agents have a better plugging effect on the rough fractures, mainly because there are a large number of bumps on the surface of rough fractures, which will intensify the collision of granular temporary plugging agents. The granular temporary plugging agent will stop moving and accumulate at the depressed position of the fracture, while the bumps on the fracture wall will slow down the migration speed of fibers within the fracture, which will further slow down the migration speed of granular temporary plugging agent, so as to achieve effective plugging [44].
More than 40 wells in the Sichuan Basin have undergone temporary plugging fracturing, which has significantly improved the stimulation effect of tight gas, shale oil and gas, and ultra-deep carbonate oil and gas reservoirs. Well SC1 is a horizontal well in the Daanzhai section of the Sichuan Basin, with a reservoir temperature of 89°C and a reservoir pressure coefficient of 1.65. The horizontal section of SC1 is 1000 m long, with strong reservoir heterogeneity and significant differences in porosity and permeability. In order to improve the complexity of fracturing fractures, temporary plugging fracturing technology was used in Well SC1. A total of 1700 kg of fiber and 1700 kg of granular temporary plugging agent were added to the fracturing of Well SC1, and among them, there is 850 kg of 100 mesh granular temporary plugging agent, and 850 kg of 10/40 mesh granular temporary plugging agent. During the fracturing process, the temporary plugging agent is uniformly mixed in the linear glue with a viscosity of 30 mPas, and the mixed liquid is injected into the formation through the fracturing pump truck with an injection displacement of 1 m3/min.
After adding the temporary plugging agent, the fracturing pressure increased by an average of 6 MPa.The pump injection pressure analysis of well SC1 (Figure 13) shows that the G function exhibits multiple jumping peaks and troughs, indicating that the fracturing fracture has undergone multiple deflections and expansion in the formation, which proves that the research results in this paper can be used to guide the fracturing design of Shale oil.
In this study, we clarified the migration rule of temporary plugging agent in rough fractures through visualization and plugging pressure testing, and confirmed that using fiber, 100 mesh granular temporary plugging agent, and 10/40 mesh granular temporary plugging agent can achieve rapid and high-strength plugging. Especially when the fiber concentration is increased, the pressure onset time is significantly shortened, which is conducive to the rapid formation of a sealing layer to force the fracture to turn. This study has been widely used in the stimulation of tight gas, shale oil and gas, and ultra deep carbonate oil and gas reservoirs in the Sichuan Basin, and has achieved good stimulation effects. Although this paper has studied the migration law of temporary plugging agent in the fracture, it still needs to be further improved. For example, it is suggested to use high-strength transparent resin to make a fracture seal pressure test system, and to be equipped with high-speed cameras to capture the migration state of temporary sealing agent in the fracture in real time, so as to have a more intuitive understanding of the results.
The original contributions presented in the study are included in the article/supplementary material, further inquiries can be directed to the corresponding author.
YW: Conceptualization, funding acquisition, project administration, re-sources, writing—original draft and software, formal analysis, methodology, writing—review and editing project administration. YF and XW: Investigation, Data curation funding. All authors contributed to the article and approved the submitted version.
This work was financially supported by the project of the PetroChina Southwest Oil and Gas Field Company (Grant Nos. 20200302-14, 20210302-19, and 20220302-11).
Authors YW, YF, and XW were employed by Engineering Technology Research Institute of Southwest Oil and Gas Field Company, PetroChina.
All claims expressed in this article are solely those of the authors and do not necessarily represent those of their affiliated organizations, or those of the publisher, the editors and the reviewers. Any product that may be evaluated in this article, or claim that may be made by its manufacturer, is not guaranteed or endorsed by the publisher.
1. Suyun HU, Jianzhong LI, Tongshan W, Zecheng WA, Tao YA, Xin LI, et al. CNPC oil and gas resource potential and exploration target selection. P Geo Exp (2020) 42(5):813–23.
2. Li L, Tan J, Wood DA, Zhao Z, Becker D, Lyu Q, et al. A review of the current status of induced seismicity monitoring for hydraulic fracturing in unconventional tight oil and gas reservoirs. Fuel (2019) 242:195–210. doi:10.1016/j.fuel.2019.01.026
3. Chen S, Xu G, Yang J, Li Y-F, Zhang J, Zhao H-W, et al. Foundational geological survey for oil and gas resources in Songliao Basin and its periphery areas: Progress and prospect. Geology Resour (2021) 30(3):221–31.
4. Guoxin L, Rukai Z. Progress, challenges and key issues in the unconventional oil and gas development of CNPC. China Pet Exploration (2020) 25(2):1. doi:10.3969/j.issn.1672-7703.2020.02.001
5. Qin L, Zhang X, Zhai C, Lin H, Lin S, Wang P, et al. Advances in liquid nitrogen fracturing for unconventional oil and gas development: A review. Energy and Fuels (2022) 36(6):2971–92. doi:10.1021/acs.energyfuels.2c00084
6. Boak J, Kleinberg R. Shale gas, tight oil, shale oil and hydraulic fracturing. Future Energy (2020) 67–95. doi:10.1016/b978-0-08-102886-5.00004-9
7. Aslannezhad M, Kalantariasl A, You Z, Iglauer S, Keshavarz A. Micro-proppant placement in hydraulic and natural fracture stimulation in unconventional reservoirs: A review. Energ Rep (2021) 7:8997–9022. doi:10.1016/j.egyr.2021.11.220
8. Wang D, Wang X, Ge H, Sun D, Yu B. Insights into the effect of spontaneous fluid imbibition on the formation mechanism of fracture networks in brittle shale: An experimental investigation. ACS omega (2020) 5(15):8847–57. doi:10.1021/acsomega.0c00452
9. Wang D, Dahi Taleghani A, Yu B, Wang M, He C. Numerical simulation of fracture propagation during refracturing. Sustainability (2022) 14(15):9422. doi:10.3390/su14159422
10. Wang Y, Yang J, Wang T, Hu Q, Lv Z, He T. Visualization experiment of multi-stage alternating injection acid fracturing. Energ Rep (2022) 8:9094–103. doi:10.1016/j.egyr.2022.07.031
11. Wang Y, Zhou C, Zhang H, He T, Tang X, Peng H, et al. Research and application of segmented acid fracturing technology in horizontal wells of ultra deep carbonate gas reservoirs in southwest China. In: International Petroleum Technology Conference; March 2021. OnePetro (2021).
12. Wang D, Qin H, Zheng C, Sun D, Yu B. Transport mechanism of temporary plugging agent in complex fractures of hot dry rock: A numerical study. Geothermics (2023) 111:102714. doi:10.1016/j.geothermics.2023.102714
13. Wang Y, Zhou C, Yi X, Li L, Chen W, Han X. Technology and application of segmented temporary plugging acid fracturing in highly deviated wells in ultradeep carbonate reservoirs in southwest China. ACS omega (2020) 5(39):25009–15. doi:10.1021/acsomega.0c01008
14. Wang Y, Fan Y, Zhou C, Luo Z, Chen W, He T, et al. Research and application of segmented acid fracturing by temporary plugging in ultradeep carbonate reservoirs. ACS omega (2021) 6(43):28620–9. doi:10.1021/acsomega.1c03021
15. Wang Y, Fan Y, Wang T, Ye J, Luo Z. A new compound staged gelling acid fracturing method for ultra-deep horizontal wells. Gels (2022) 8(7):449. doi:10.3390/gels8070449
16. Yuan L, Zhou F, Li B, Gao J, Yang X, Cheng J, et al. Experimental study on the effect of fracture surface morphology on plugging efficiency during temporary plugging and diverting fracturing. J Nat Gas Sci Eng (2020) 81:103459. doi:10.1016/j.jngse.2020.103459
17. Feng W, Yang C, Zhou F. Experimental study on surface morphology and relevant plugging behavior within acid-etched and unetched fractures. J Nat Gas Sci Eng (2021) 88:103847. doi:10.1016/j.jngse.2021.103847
18. Qin H, Wang D, Deng Y, Han D, Yu B, Sun D. Study on transport law of temporary plugging agent in artificial fractures of hot dry rocks. J Eng Thermophys 43(9):2397–403.
19. Wang D, Dong Y, Sun D, Yu B. A three-dimensional numerical study of hydraulic fracturing with degradable diverting materials via CZM-based FEM. Eng Fracture Mech (2020) 237:107251. doi:10.1016/j.engfracmech.2020.107251
20. Wang B, Zhou F, Yang C, Wang D, Yang K, Liang T. Experimental study on injection pressure response and fracture geometry during temporary plugging and diverting fracturing. SPE J (2020) 25(2):573–86. doi:10.2118/199893-pa
21. Zhang Y, Yu R, Yang W, Tian Y, Shi Z, Sheng C, et al. Effect of temporary plugging agent concentration and fracturing fluid infiltration on initiation and propagation of hydraulic fractures in analogue tight sandstones. J Pet Sci Eng (2022) 210:110060. doi:10.1016/j.petrol.2021.110060
22. Yang C, Zhou F, Feng W, Tian Z, Yuan L, Gao L. Plugging mechanism of fibers and particulates in hydraulic fracture. J Pet Sci Eng (2019) 176:396–402. doi:10.1016/j.petrol.2019.01.084
23. Zhang L, Zhou F, Feng W, Pournik M, Li Z, Li X. Experimental study on plugging behavior of degradable fibers and particulates within acid-etched fracture. J Pet Sci Eng (2020) 185:106455. doi:10.1016/j.petrol.2019.106455
24. Gomaa AM, Nino-Penaloza A, Castillo D, McCartney E, Mayor J. Experimental investigation of particulate diverter used to enhance fracture complexity. In: SPE International Conference and Exhibition on Formation Damage Control; February 24-26, 2016; Louisiana, USA. OnePetro (2016).
25. Li M, Zhou F, Sun Z, Dong E, Zhuang X, Yuan L, et al. Experimental study on plugging performance and diverted fracture geometry during different temporary plugging and diverting fracturing in Jimusar shale. J Pet Sci Eng (2022) 215:110580. doi:10.1016/j.petrol.2022.110580
26. Cohen CE, Tardy PM, Lesko T, Lecerf B, Pavlova S, Voropaev S, et al. Understanding diversion with a novel fiber-laden acid system for matrix acidizing of carbonate formations. In: SPE annual technical conference and exhibition; September 19–22, 2010; Florence, Italy. OnePetro (2010).
27. Williams V, McCartney E, Nino-Penaloza A. Far-field diversion in hydraulic fracturing and acid fracturing: Using solid particulates to improve stimulation efficiency. In: SPE Asia Pacific hydraulic fracturing conference; August 24–26, 2016; Beijing, China. OnePetro (2016).
28. Liwei W, Xiuling H, Chunming X, Bo W, Zhanwei Y, Ying G. Multi-fracture temporary blocking steering mechanism and experimental research in ultra—deep fractured reservoir. In: 5th ISRM Young Scholars’ Symposium on Rock Mechanics and International Symposium on Rock Engineering for Innovative Future; December 1–4, 2019; Okinawa, Japan. OnePetro (2019).
29. Zhang R, Hou B, Tan P, Muhadasi Y, Fu W, Dong X, et al. Hydraulic fracture propagation behavior and diversion characteristic in shale formation by temporary plugging fracturing. J Pet Sci Eng (2020) 190:107063. doi:10.1016/j.petrol.2020.107063
30. Van Domelen MS. A practical guide to modern diversion technology. In: Spe oklahoma city oil and gas symposium; March 27–31, 2017; Oklahoma, OK. OnePetro (2017).
31. Xu C, Zhang H, Kang Y, Zhang J, Bai Y, Zhang J, et al. Physical plugging of lost circulation fractures at microscopic level. Fuel (2022) 317:123477. doi:10.1016/j.fuel.2022.123477
32. Xu C, Zhang H, She J, Jiang G, Peng C, You Z. Experimental study on fracture plugging effect of irregular-shaped lost circulation materials. Energy (2023) 276:127544. doi:10.1016/j.energy.2023.127544
33. Huang L, Liu J, Zhang F, Dontsov E, Damjanac B. Exploring the influence of rock inherent heterogeneity and grain size on hydraulic fracturing using discrete element modeling. Int J Sol Structures (2019) 176:207–20. doi:10.1016/j.ijsolstr.2019.06.018
34. Chen Y, Sang Y, Guo J, Yang J, Chen W, Zeng J, et al. Experimental study on a liquid–solid phase-change autogenous proppant fracturing fluid system. ACS omega (2023) 8(10):9101–10. doi:10.1021/acsomega.2c04853
35. Wang D, Zhu H, Micheal M, Tang X, Li Q, Yi X, et al. Coupled heat-fluid-solid numerical study on heat extraction potential of hot dry rocks based on discrete fracture network model. Energ Geosci (2023):100159. doi:10.1016/j.engeos.2023.100159
36. Wang DB, Zhou FJ, Li YP, Yu B, Martyushev D, Liu XF, et al. Numerical simulation of fracture propagation in Russia carbonate reservoirs during refracturing. Pet Sci (2022) 19(6):2781–95. doi:10.1016/j.petsci.2022.05.013
37. Guo X, Hu D, Huang R, Wei Z, Duan J, Wei X, et al. Deep and ultra-deep natural gas exploration in the Sichuan Basin: Progress and prospect. Nat Gas Industry B (2020) 7(5):419–32. doi:10.1016/j.ngib.2020.05.001
38. Chen S, Zhu Y, Wang H, Liu H, Wei W, Fang J. Shale gas reservoir characterisation: A typical case in the southern Sichuan Basin of China. Energy (2011) 36(11):6609–16. doi:10.1016/j.energy.2011.09.001
39. Zhang X, Fu M, Deng H, Li Z, Zhao S, Gluyas JG, et al. The differential diagenesis controls on the physical properties of lithofacies in sandstone reservoirs from the Jurassic Shaximiao Formation, Western Sichuan depression, China. J Pet Sci Eng (2020) 193:107413. doi:10.1016/j.petrol.2020.107413
40. Dai J, Zou C, Liao S, Dong D, Ni Y, Huang J, et al. Geochemistry of the extremely high thermal maturity Longmaxi shale gas, southern Sichuan Basin. Org Geochem (2014) 74:3–12. doi:10.1016/j.orggeochem.2014.01.018
41. Liang C, Jiang Z, Yiting Y, Wei X. Shale lithofacies and reservoir space of the wufeng–longmaxi formation, Sichuan Basin, China. Pet Exploration Dev (2012) 39(6):736–43. doi:10.1016/s1876-3804(12)60098-6
42. Wang D, Zhou F, Ge H, Shi Y, Yi X, Xiong C, et al. An experimental study on the mechanism of degradable fiber-assisted diverting fracturing and its influencing factors. J Nat Gas Sci Eng (2015) 27:260–73. doi:10.1016/j.jngse.2015.08.062
43. Al-Enezi A, Al-Othman M, Al-Shtail M, Sadeeqi YA, Bhatia K, Alboueshi A, et al. Application of integrated far-field diversion technology in multistage acid-fracturing: Lesson learnings from unconventional field north Kuwait. In: SPE International Hydraulic Fracturing Technology Conference and Exhibition; January 11–13, 2022; Muscat, Oman. OnePetro (2022).
Keywords: migration, multi-scale, temporary plugging agent, rough fracture, fracturing
Citation: Wang Y, Fan Y and Wang X (2023) Study on migration law of multiscale temporary plugging agent in rough fractures of shale oil reservoirs. Front. Phys. 11:1228006. doi: 10.3389/fphy.2023.1228006
Received: 24 May 2023; Accepted: 03 July 2023;
Published: 13 July 2023.
Edited by:
Chengyuan Xu, Southwest Petroleum University, ChinaReviewed by:
Xiaohui Mao, University of Alberta, CanadaCopyright © 2023 Wang, Fan and Wang. This is an open-access article distributed under the terms of the Creative Commons Attribution License (CC BY). The use, distribution or reproduction in other forums is permitted, provided the original author(s) and the copyright owner(s) are credited and that the original publication in this journal is cited, in accordance with accepted academic practice. No use, distribution or reproduction is permitted which does not comply with these terms.
*Correspondence: Yang Wang, d2FuZ3lhbmcwOTk2QHBldHJvY2hpbmEuY29tLmNu
Disclaimer: All claims expressed in this article are solely those of the authors and do not necessarily represent those of their affiliated organizations, or those of the publisher, the editors and the reviewers. Any product that may be evaluated in this article or claim that may be made by its manufacturer is not guaranteed or endorsed by the publisher.
Research integrity at Frontiers
Learn more about the work of our research integrity team to safeguard the quality of each article we publish.