- 1School of Physics and Materials Science, Guangzhou University, Guangzhou, China
- 2Research Center for Advanced Information Materials (CAIM), Guangzhou University, Guangzhou, China
Laser-induced magnetization dynamics in a perpendicularly exchange-coupled TbFeCo/GdFeCo bilayer film are studied by using pump-probe magneto-optical Kerr spectroscopy. An ultrafast modulation effect on local magnetization orientation is observed. Such ultrafast magnetization reorientation in the GdFeCo layer is revealed to be triggered by the femtosecond laser pulse and driven by the effective exchange field. These processes occur within a timescale of hundreds of picoseconds, in which the field- and fluence-dependent dynamical behaviors are demonstrated. In addition, an atomistic Heisenberg model is proposed for studying the laser-induced magnetization dynamics by using micromagnetic simulation. The simulated results agree with the experimental phenomena and further reveal the underlying mechanism. These results show an approach for ultrafast manipulation of the local magnetization orientation in perpendicularly exchange-coupled structures.
1 INTRODUCTION
Exchange-coupled composites (ECCs) have attracted much interest due to their designable magnetic properties from combining individual material with different properties [1, 2] and also potential applications in magnetic recording [3, 4], magnetic sensors [5, 6] and magnonic devices [7, 8]. In particular, many research works focused on the perpendicular ECCs which have high thermal stability, reduced switching field, and thus excellent applied potential for ultrahigh-density magnetic recording [9–11]. On the other hand, laser-induced magnetization dynamics in magnetic system is also an active field. Introduction of the ultrashort laser pulses can lead to infusive effects, such as further reduce coercivity and remarkably accelerate magnetization reversal for magnetic recording [12–15]. Therefore, studying laser-induced or -modulated ultrafast magnetization dynamics in perpendicular ECCs is demanded for developing related applications.
Amorphous ferrimagnetic rare earth-transition metal (RE-TM) alloy films, such as GdFeCo and TbFeCo, are one kind of the most-concerned materials for ultrafast magnetic applications [16–18]. Typically, GdFeCo and TbFeCo can be used as the free and pinning layers, respectively, in perpendicular ECCs due to their dissimilar spin-orbit coupling and perpendicular magnetic anisotropy (PMA) [2, 19]. RE-TM ECCs have attracted attentions also due to their applied potential in magnetic super resolution (MSR) for magneto-optical readout [20–22], but observation of the related dynamical process has not been reported.
In this paper, we investigate the laser-induced magnetization dynamics in a perpendicularly exchange-coupled TbFeCo/GdFeCo bilayer film by using pump-probe magneto-optical polar Kerr spectroscopy. We observe an ultrafast modulation effect on local magnetization orientation in the GdFeCo layer which is triggered by the femtosecond laser pulse and driven by the effective exchange field, and also first demonstrate the dynamics. In addition, an atomistic Heisenberg model is proposed for studying these laser-induced magnetization dynamics by using micromagnetic simulation.
2 EXPERIMENT
The sample studied here is a TbFeCo(40 nm)/GdFeCo(50 nm) coupled bilayer film prepared on a glass substrate by magnetron sputtering. The TbFeCo layer with high PMA has a strong coercivity (Hc) of ∼7 kOe, while the GdFeCo layer has weak magnetocrystalline anisotropy and hence possesses in-plane magnetization.
The laser-induced magnetization dynamics are measured by using a time-resolved pump-probe magneto-optical Kerr configuration. Linearly polarized laser pulse train with a central wavelength of 800 nm, a duration of 100 fs, and a repetition rate of 1 kHz is generated from a Ti:sapphire regenerative amplifier and split into the pump and probe beams. Both the two beams are almost incident normally on the surface of GdFeCo layer with a pulse fluence ratio of pump to probe larger than 30. The focused spot diameter of the pump beam is ∼150 μm, while the probe spot located at the center of the pump spot is set to be nearly a half smaller to decrease the temperature gradient within the probed area. The probe beam reflected from the sample is divided into two orthogonally polarized components by a Glan prism to measure the polar Kerr rotation by using a differential detector combined with a lock-in amplifier. A variable magnetic field generated by an electromagnet is applied perpendicularly to the sample plane. All measurements are performed at room temperature.
3 RESULTS AND DISCUSSION
Considering the light penetration depth, the polar Kerr signal in our experiment mainly comes from the out-of-plane magnetization component in the upper half part of GdFeCo layer. Note that for RE-TM materials, the Kerr signal probed at 800 nm is contributed from the magnetic moment of TM atoms [18], namely FeCo atoms here. As shown in Figure 1A, the out-of-plane polar Kerr hysteresis loop presents the hard-axis hysteresis of GdFeCo layer. Due to the competition between the demagnetizing field and the effective bias field from exchange coupling [2, 10], a nonuniform magnetization distribution is formed in the GdFeCo layer. The slight hysteresis under small external field just presents the different magnetization states of GdFeCo layer originated from the opposite saturation states of TbFeCo layer.
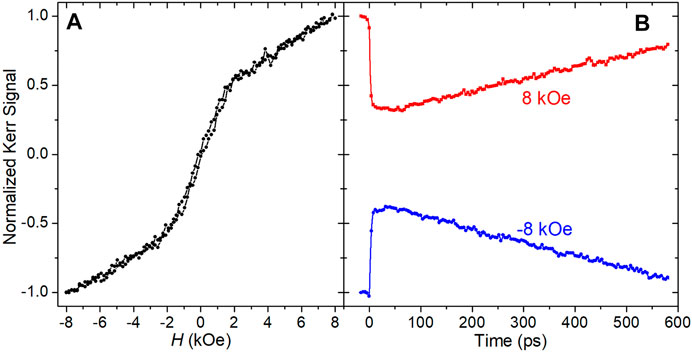
FIGURE 1. (A) Normalized polar Kerr hysteresis loop of the GdFeCo layer. (B) Laser-induced magnetization dynamics measured under H = ±8 kOe and pump fluence of 9.8 mJ/cm2. The Kerr signal is normalized to the static Kerr value at H = ±8 kOe.
Figure 1B shows the laser-induced magnetization dynamics measured under pump fluence of 9.8 mJ/cm2 and external field (H) of ±8 kOe which is larger than Hc of TbFeCo layer. Only ultrafast demagnetization and magnetization recovery can be observed in the dynamical process. The magnetization recovery time is ∼460 ps, showing a slow heat-diffusion process.
Next, field-dependent magnetization dynamics are measured. Anomalous dynamical phenomena are observed in a range of small field. In Figure 2A, it seems that the trace for H = 1.6 kOe still only presents the typical dynamical processes of ultrafast demagnetization and magnetization recovery. However, the decay curves for −800 Oe, −320 Oe, and 0 Oe all cross their initial magnetization states. With decreasing H, the crossing amplitude increases, while the crossing time decreases from 462 to 176 ps. In the case of 0 Oe (without external field applied), the crossing amplitude seems even larger than the demagnetization amplitude. This anomalous behavior could not be attributed to the magnetization precession, a strong evidence is that the crossing time increases with increasing H, whereas the time period of magnetization precession should decrease with increasing H [23]. Then, what does it originate from?
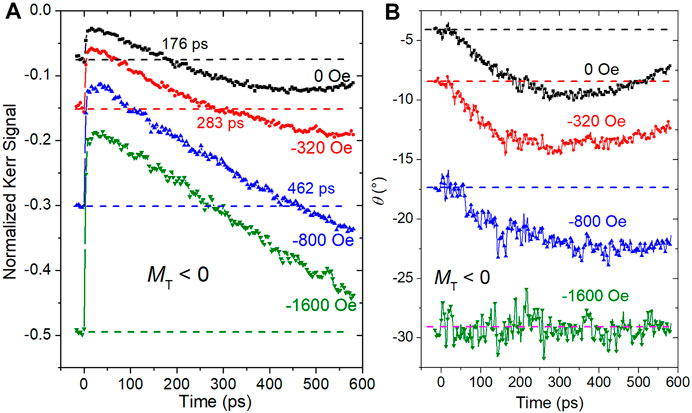
FIGURE 2. (A) Laser-induced magnetization dynamics measured under different external field and a constant pump fluence of 9.8 mJ/cm2. (B) Average magnetization orientation angle
As mentioned above, because of the limited light penetration depth, direct laser excitation on the TbFeCo layer can be neglected. The femtosecond laser pulse mainly decreases the transient magnetization of GdFeCo layer, and simultaneously reduce the demagnetizing energy (Ed) and the exchange energy (EA) [10]. These would change the equilibrium distribution of the magnetization orientation in GdFeCo layer, leading to the transient magnetization reorientation. Mostly in RE-TM layer, the reduction of Ed is more obvious, so the magnetization reorientation is expected to toward the orientation of TbFeCo magnetization driven by the exchange field. Note that along the vertical direction, the demagnetizing field in GdFeCo layer is nonuniform, the vertical diffusion of laser heating should increase the transient influence on demagnetizing field, and thus further singularize the role of exchange field. Especially, if the transient temperature is around the magnetization compensation point [16], a remarkable reduction of Ed should also significantly enhance this effect. Moreover, with H increased, the role of exchange field in the total effective field become minor, and thus the magnetization reorientation effect would be gradually submerged by the magnetization recovery, leading to smaller crossing amplitude and shorter crossing time.
Time dependence of the magnetization orientation can be estimated via the relation
To further demonstrate the role of exchange field in the dynamics, we compare the transient traces measured with opposite saturated magnetization states of the TbFeCo layer (denoted by the sign of MT) and opposite directions of H, respectively, as shown in Figure 3A. As expected, opposite MT results in opposite direction of magnetization reorientation, even with the same condition of external field and laser fluence. This is also another strong evidence for excluding the magnetization precession as the origin of the observed dynamical phenomena. It is clear that the initial state of magnetization in the probed area is dominated by H, while the direction of magnetization reorientation is controlled by MT, implying that just the exchange field drives the magnetization reorientation after laser excitation. This result agrees with that of the steady measurements for MSR [20], but here we first reveal the dynamics and related timescale.
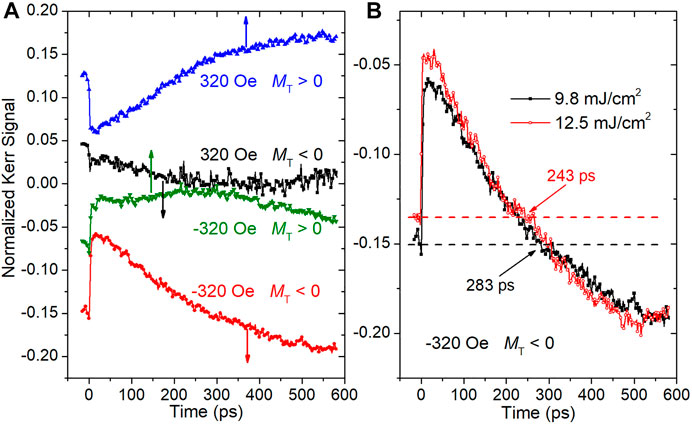
FIGURE 3. (A) Laser-induced magnetization dynamics depended on the directions of exchange bias field and external field. The sign of MT shows the saturated state of the TbFeCo magnetization which reflects the direction of exchange bias field. The arrows denote the direction of magnetization reorientation. (B) Comparison of magnetization dynamics measured under different excitation fluence.
Figure 3B shows the dynamics measured under the same H and MT but different laser fluence. The higher fluence not only leads to a more remarkable demagnetization, but also a larger crossing amplitude and a shorter crossing time, showing that increase of the laser excitation energy can accelerate the magnetization reorientation. This result further demonstrates the laser modulation effect of the local magnetization orientation.
4 MICROMAGNETIC SIMULATION
In order to further understand the mechanism of above experimental phenomena, we construct an atomistic Heisenberg model for describing the magnetic states of FeCo atoms in GdFeCo layer of the sample, which comprises of nearest ferromagnetic exchange interaction (
where the magnetic moments are imposed on a two-dimensional square lattice with periodic boundary conditions, with
In the simulations, we first study the temperature dependences of equilibrium magnetic properties of GdFeCo layer using the Langevin Landau-Lifshitz-Gilbert stochastic equation [24, 25] (see Simulation methods in the Supplementary Information). The temperature-dependent behaviors are monitored by evaluating the thermal-averaged magnetization
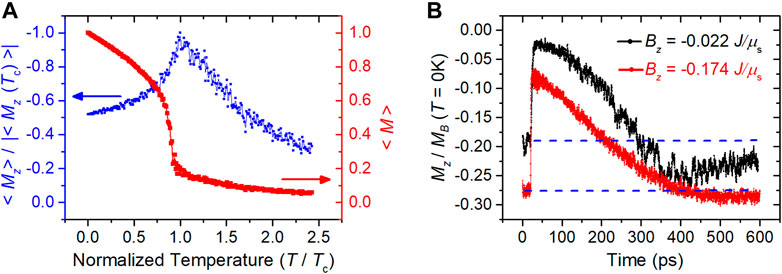
FIGURE 4. (A) Simulated thermal-averaged out-of-plane magnetization component
To proceed, we simulate the laser-induced ultrafast magnetization dynamics by employing the Landau–Lifshitz–Bloch (LLB) equation [29–32], in which the temperature of atomic spins are determined by using the three-temperature model [33–35] (see Simulation methods in the Supplementary Information). In this scheme, the system is first relaxed by solving the LLB equation under the effective magnetic field Bz for reaching the equilibrium state. Then we carefully tracked the dynamics of out-of-plane components of magnetization (
Figure 4B presents the evolutions of
In our experiments,
5 CONCLUSION
In summary, the laser-induced magnetization dynamics and the ultrafast modulation effect on local magnetization orientation in a perpendicularly coupled TbFeCo/GdFeCo film are studied by using time-resolved magneto-optical Kerr spectroscopy. The magnetization reorientation in the GdFeCo layer is triggered by the femtosecond laser pulse and driven by the effective exchange field. These processes occur within a timescale of hundreds of ps. We discuss the field- and fluence-dependent dynamical behaviors, and propose an atomistic Heisenberg model to study the dynamics by using micromagnetic simulation. The simulation agrees with the experimental phenomena and further reveals the underlying mechanism. These results show an approach for ultrafast manipulation to the local magnetization orientation in perpendicularly exchange-coupled structures via changing exchange bias state and laser fluence.
Data Availability Statement
The original contributions presented in the study are included in the article/Supplementary Material, further inquiries can be directed to the corresponding authors.
Author Contributions
ZX and ZC designed the experiment, set up the configuration and carried out the measurement. JZ, YC, and JP contributed to the experiment. JZ and ZC finished the data analysis with supports from WZ. JC constructed the model and performed the computations. ZX, JC, and ZC prepared the manuscript. All authors commented on the manuscript.
Funding
This work was partially supported by the National Natural Science Foundation of China under Grant Nos. 11204044, 11604059, and 21903017; the Natural Science Foundation of Guangdong Province under Grant Nos. 2020A1515010411, 2019A1515010783, and 2017A030313020; and the Key Research Project of Guangzhou University under Grant No. YK2020003.
Conflict of Interest
The authors declare that the research was conducted in the absence of any commercial or financial relationships that could be construed as a potential conflict of interest.
Publisher’s Note
All claims expressed in this article are solely those of the authors and do not necessarily represent those of their affiliated organizations, or those of the publisher, the editors and the reviewers. Any product that may be evaluated in this article, or claim that may be made by its manufacturer, is not guaranteed or endorsed by the publisher.
Supplementary Material
The Supplementary Material for this article can be found online at: https://www.frontiersin.org/articles/10.3389/fphy.2021.755081/full#supplementary-material
References
1. Blanco-Roldán C, Quirós C, Sorrentino A, Hierro-Rodríguez A, Álvarez-Prado LM, Valcárcel R, et al. Nanoscale Imaging of Buried Topological Defects with Quantitative X-ray Magnetic Microscopy. Nat Commun (2015) 6:8196. doi:10.1038/ncomms9196
2. Wang K, Wang Y, Ling F, Xu Z. Perpendicular Exchange Coupling Effects in Ferrimagnetic TbFeCo/GdFeCo Hard/soft Structures. J Magnetism Magn Mater (2018) 452:153–6. doi:10.1016/j.jmmm.2017.12.061
3. Wang J-P, Shen WK, Bai JM, Victora RH, Judy JH, Song WL. Composite Media (dynamic tilted media) for Magnetic Recording. Appl Phys Lett (2005) 86:142504. doi:10.1063/1.1896431
4. Xu Z, Zhou SM, Ge JJ, Du J, Sun L. Magnetization reversal mechanism of perpendicularly exchange-coupled composite L10-FePt/CoCrPt bilayers. J Appl Phys (2009) 105:123903. doi:10.1063/1.3148300
5. Radu F, Abrudan R, Radu I, Schmitz D, Zabel H. Perpendicular Exchange Bias in Ferrimagnetic Spin Valves. Nat Commun (2012) 3:715. doi:10.1038/ncomms1728
6. Hebler B, Reinhardt P, Katona GL, Hellwig O, Albrecht M. Double Exchange Bias in Ferrimagnetic Heterostructures. Phys Rev B (2017) 95:104410. doi:10.1103/PhysRevB.95.104410
7. Zhang Z, Cui B, Wang G, Ma B, Jin QY, Liu Y. Ultrafast Laser-induced Magnetization Precession Dynamics in FePt/CoFe Exchange-coupled Films. Appl Phys Lett (2010) 97:172508. doi:10.1063/1.3510473
8. Li S-F, He P, Cheng C-Y, Zhou S-M, Lai T-S. Spin-Wave Modes in Exchange-Coupled FePt/FeNi Bilayer Films. Chin Phys. Lett. (2014) 31:017502. doi:10.1088/0256-307X/31/1/017502
9. Makarov D, Lee J, Brombacher C, Schubert C, Fuger M, Suess D, et al. Perpendicular FePt-based Exchange-coupled Composite Media. Appl Phys Lett (2010) 96:062501. doi:10.1063/1.3309417
10. Chen Z, Li S, Lai T. Laser-induced transient strengthening of coupling in L10-FePt/FeNi exchange-spring film. J Phys D: Appl Phys (2015) 48:145002. doi:10.1088/0022-3727/48/14/145002
11. Li ZW, Jiao JY, Luo Z, Ma TY, Qiao L, Wang Y, et al. Microstructure and Magnetic Properties of Exchange-Coupled Co72Pt28/Pt/Co81Ir19 Composite Media for Perpendicular Magnetic Recording. J Supercond Nov Magn (2019) 32:2229–33. doi:10.1007/s10948-018-4953-8
12. Xu C, Chen Z, Chen D, Zhou S, Lai T. Origin of Anomalous Hysteresis Loops Induced by Femtosecond Laser Pulses in GdFeCo Amorphous Films. Appl Phys Lett (2010) 96:092514. doi:10.1063/1.3339878
13. Vahaplar K, Kalashnikova AM, Kimel AV, Hinzke D, Nowak U, Chantrell R, et al. Ultrafast Path for Optical Magnetization Reversal via a Strongly Nonequilibrium State. Phys Rev Lett (2009) 103:117201. doi:10.1103/PhysRevLett.103.117201
14. Stanciu CD, Tsukamoto A, Kimel AV, Hansteen F, Kirilyuk A, Itoh A, et al. Subpicosecond Magnetization Reversal across Ferrimagnetic Compensation Points. Phys Rev Lett (2007) 99:217204. doi:10.1103/PhysRevLett.99.217204
15. Chen Z, Gao R, Wang Z, Xu C, Chen D, Lai T. Field-dependent Ultrafast Dynamics and Mechanism of Magnetization Reversal Across Ferrimagnetic Compensation Points in GdFeCo Amorphous Alloy Films. J Appl Phys (2010) 108:023902. doi:10.1063/1.3462429
16. Stanciu CD, Kimel AV, Hansteen F, Tsukamoto A, Itoh A, Kirilyuk A, et al. Ultrafast spin dynamics across compensation points in ferrimagnetic GdFeCo: The role of angular momentum compensation. Phys Rev B (2006) 73:220402. doi:10.1103/PhysRevB.73.220402
17. Ogasawara T, Iwata N, Murakami Y, Okamoto H, Tokura Y. Submicron-scale Spatial Feature of Ultrafast Photoinduced Magnetization Reversal in TbFeCo Thin Film. Appl Phys Lett (2009) 94:162507. doi:10.1063/1.3123256
18. Chen Z, Li S, Zhou S, Lai T. Ultrafast dynamics of 4f electron spins in TbFeCo film driven by inter-atomic 3d-5d-4f exchange coupling. New J Phys (2019) 21:123007. doi:10.1088/1367-2630/ab5aa4
19. Ye L-X, Lee C-M, Lai J-H, Canizo-Cabrera A, Chen W-J, Wu Th. Magnetic Properties of MgO-based RE-TM Perpendicular Magnetic Tunnel Junctions. J Magnetism Magn Mater (2010) 322:L9–L11. doi:10.1016/j.jmmm.2009.11.021
20. Peng C, Mansuripur M. Noise and Coupling in Magnetic Super-resolution Media for Magneto-optical Readout. J Appl Phys (1999) 85:6323–30. doi:10.1063/1.370133
21. Wang XY, Zhang YP, Li ZY, Shen DF, Gan FX. Temperature-Induced Magnetization Reorientation in GdFeCo/TbFeCo Exchange-Coupled Double Layer Films. Chin Phys Lett (2003) 20:1359–61. doi:10.3321/j.issn:0256-307X.2003.08.052
22. Tani M. Domain Expansion Readout for Magnetic Amplifying Magneto-Optical System. J Magn Soc Jpn (2008) 32:43–9. doi:10.3379/msjmag.32.43
23. Chen Z, Yi M, Chen M, Li S, Zhou S, Lai T. Spin Waves and Small Intrinsic Damping in an in-plane Magnetized FePt Film. Appl Phys Lett (2012) 101:222402. doi:10.1063/1.4768787
24. Ostler TA, Barker J, Evans RFL, Chantrell RW, Atxitia U, Chubykalo-Fesenko O, et al. Ultrafast Heating as a Sufficient Stimulus for Magnetization Reversal in a Ferrimagnet. Nat Commun (2012) 3:6. doi:10.1038/ncomms1666
25. Radu I, Vahaplar K, Stamm C, Kachel T, Pontius N, Dürr HA, et al. Transient Ferromagnetic-like State Mediating Ultrafast Reversal of Antiferromagnetically Coupled Spins. Nature (2011) 472:205–8. doi:10.1038/nature09901
26. MacIsaac AB, De'Bell K, Whitehead JP. Simulation of the Reorientation Transition in Ultrathin Magnetic Films with Striped and Tetragonal Phases. Phys Rev Lett (1998) 80:616–9. doi:10.1103/PhysRevLett.80.616
27. De’Bell K, MacIsaac AB, Whitehead JP. Dipolar Effects in Magnetic Thin Films and Quasi-two-dimensional Systems. Rev Mod Phys (2000) 72:225–57. doi:10.1103/RevModPhys.72.225
28. Chen JP, Wang ZQ, Gong JJ, Qin MH, Zeng M, Gao XS, et al. Stripe-vortex Transitions in Ultrathin Magnetic Nanostructures. J Appl Phys (2013) 113:054312. doi:10.1063/1.4790483
29. Evans RFL, Hinzke D, Atxitia U, Nowak U, Chantrell RW, Chubykalo-Fesenko O. Stochastic Form of the Landau-Lifshitz-Bloch Equation. Phys Rev B (2012) 85:9. doi:10.1103/PhysRevB.85.014433
30. Vogler C, Abert C, Bruckner F, Suess D. Landau-Lifshitz-Bloch Equation for Exchange-Coupled Grains. Phys Rev B (2014) 90:10. doi:10.1103/PhysRevB.90.214431
31. Mendil J, Nieves P, Chubykalo-Fesenko O, Walowski J, Santos T, Pisana S, et al. Resolving the Role of Femtosecond Heated Electrons in Ultrafast Spin Dynamics. Sci Rep (2014) 4:7. doi:10.1038/srep03980
32. Kazantseva N, Hinzke D, Nowak U, Chantrell RW, Atxitia U, Chubykalo-Fesenko O. Towards Multiscale Modeling of Magnetic Materials: Simulations of FePt. Phys Rev B (2008) 77:7. doi:10.1103/PhysRevB.77.184428
33. Beaurepaire E, Merle J-C, Daunois A, Bigot J-Y. Ultrafast Spin Dynamics in Ferromagnetic Nickel. Phys Rev Lett (1996) 76:4250–3. doi:10.1103/PhysRevLett.76.4250
34. Kim J-W, Vomir M, Bigot J-Y. Ultrafast Magnetoacoustics in Nickel Films. Phys Rev Lett (2012) 109:5. doi:10.1103/PhysRevLett.109.166601
Keywords: magnetization dynamics, exchange coupling, time-resolved magneto-optical Kerr spectroscopy, magnetization reorientation, femtosecond laser, micromagnetic simulation
Citation: Xie Z, Zhou J, Cai Y, Chen J, Zhang W, Peng J and Chen Z (2021) Ultrafast Laser Modulation of Local Magnetization Orientation in Perpendicularly Exchange-Coupled Bilayer. Front. Phys. 9:755081. doi: 10.3389/fphy.2021.755081
Received: 07 August 2021; Accepted: 10 September 2021;
Published: 01 November 2021.
Edited by:
Feng Chi, University of Electronic Science and Technology of China, ChinaReviewed by:
Yanxiong Du, South China Normal University, ChinaLihua Teng, Qingdao University of Science and Technology, China
Copyright © 2021 Xie, Zhou, Cai, Chen, Zhang, Peng and Chen. This is an open-access article distributed under the terms of the Creative Commons Attribution License (CC BY). The use, distribution or reproduction in other forums is permitted, provided the original author(s) and the copyright owner(s) are credited and that the original publication in this journal is cited, in accordance with accepted academic practice. No use, distribution or reproduction is permitted which does not comply with these terms.
*Correspondence: Zhifeng Chen, Y2hlbnpmQGd6aHUuZWR1LmNu; Jipei Chen, Y2hlbmpwQGd6aHUuZWR1LmNu
†These authors have contributed equally to this work