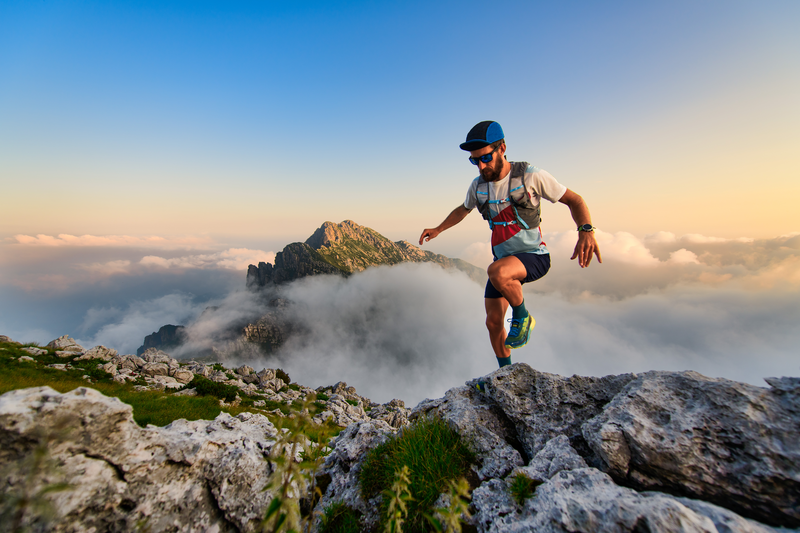
94% of researchers rate our articles as excellent or good
Learn more about the work of our research integrity team to safeguard the quality of each article we publish.
Find out more
REVIEW article
Front. Pharmacol. , 12 February 2025
Sec. Integrative and Regenerative Pharmacology
Volume 16 - 2025 | https://doi.org/10.3389/fphar.2025.1548837
This article is part of the Research Topic Innovative Approaches for Wound Treatment View all 5 articles
The unique properties of sericin and silk fibroin (SF) favor their widespread application in biopharmaceuticals, particularly in wound treatment and bone repair. The immune response directly influences wound healing cycle, and the extensive immunomodulatory functions of silk-based nanoparticles and hydrogels have attracted wide attention. However, different silk-processing methods may trigger intense immune system resistance after implantation into the body. In this review, we elaborate on the inflammation and immune responses caused by the implantation of sericin and SF and also explore their anti-inflammatory properties and immune regulatory functions. More importantly, we describe the latest research progress in enhancing the immunotherapeutic and anti-inflammatory effects of composite materials prepared from silk from a mechanistic perspective. This review will provide a useful reference for using the correct processes to exploit silk-based biomaterials in different wound treatments.
Silk is a natural fiber with a long history. It is formed by the solidification of the silk liquid secreted by the mature silkworm during the cocoon phase. Silk is mainly composed of hydrophobic silk fibroin (SF), which forms the core fiber, and hydrophilic sericin, a globular protein that binds to SF (Johari et al., 2022; Silva et al., 2022). SF is rich in glycine, alanine and serine, while sericin mainly contains serine and threonine (Silva et al., 2022; Takahashi et al., 1999).
Initially, sericin was often discarded in large quantities as textile waste, resulting in environmental pollution and wastage of natural resources (Aramwit et al., 2012). With the rapid development of biomaterials, the use of sericin in medicines has received increasing attention. Sericin possesses outstanding antioxidant, anti-inflammatory, antibacterial, antiviral, and biological characteristics that promote tissue regeneration, making it important in curing diseases such as hypertension, cancer, and diabetes (Hu et al., 2022). In addition, sericin biomaterials exhibit good biocompatibility and low immunogenicity, and have been engineered into various biomaterials, including films, hydrogels, scaffolds, fiber pads, particles, coatings, conduits, and nanoparticles for tissue repair, regeneration, and disease cure (Qi et al., 2018; Shah et al., 2019; Li et al., 2020; Wang et al., 2021; Xu et al., 2022; Deng Y. et al., 2022; Ghensi et al., 2019; Fu et al., 2022). Over the past decade, sericin-based biomaterials have developed rapidly in drug-delivery and tissue engineering.
SF has superior biocompatibility, marked mechanical properties, controlled biodegradation rates, and ideal cellular-SF interactions (Gholipourmalekabadi et al., 2020; Wani et al., 2022; Wang et al., 2008). The application of silk in the textile industry has a long history and the preparation technology for silk is very mature; therefore, the source of SF is rich (Huang W. et al., 2018). These characteristics have made SF emerge among biomaterial applications in recent years. SF can be transformed into scaffolds, hydrogels, films, microspheres, and nanoparticles alone or in combination with other materials for tissue engineering such as nerves, bone and muscles, and can also be used in drug delivery systems for skin wound treatment, tumor immunotherapy, and other medical treatments (Cui et al., 2020; Wang D. et al., 2022; Wenhao et al., 2020; Del Bianco et al., 2022; Cai et al., 2023; Hassan et al., 2024).
The body possesses intricate and precise protective mechanisms. When silk is used as a medical material, the immune system often faces challenges. Silk medical materials require different processing techniques before implantation into the body, which can cause significantly alteration of the protein structure of the original silk, resulting in varying levels of immune system activation. This difference may be due to surface chemistry, protein conformation, and polymer formation with other proteins in the treated silk-based materials (Majumder et al., 2024). This has expanded the application of silk in the medical field and has extended the proinflammatory effects to anti-tumor, antibacterial, and vaccine adjuvant fields, although a low immune response contributes to bone, skin, and vascular repair. In this review, we aimed to provide profound discussion of the activation of a wide range of immune responses in vivo using silk-based biomaterials and describe the rigorous and accurate processing methods required for different clinical applications in subsequent research.
One of the most important characteristics of biomaterials is their biocompatibility, the initiation of the innate immune cells is the decisive factor in the biocompatibility of biomaterials. This process usually causes an inflammatory response, and the degree of the response resides mainly on the properties of the biomaterial (Ekdahl et al., 2011). In this section, we summarize and discuss the biosafety, immunogenicity and immunomodulatory properties of sericin and SF.
The biosafety of sericin has long been controversial. Studies have confirmed that sericin has good biocompatibility from the three standpoints of inflammation, allergy, and immunogenicity: (i) sericin only causes low response of inflammatory cells in vivo (macrophages and neutrophils) (Jiao et al., 2017; Li et al., 2015) (Figures 1A, B); (ii) neglected allergens (Jiao et al., 2017) and (iii) sericin only causes mild innate and adaptive immune responses (Jiao et al., 2017; Zhang et al., 2006; Zhang Y. et al., 2021; Panilaitis et al., 2003). Furthermore, the addition of sericin to a mixture of chitosan and silver nanoparticles (AgNPs) can reduce their immunogenicity (Nayak et al., 2021). Various forms of materials such as nanoparticles, hydrogels, scaffolds, sponges and films prepared from sericin have also not been found to cause marked immune responses or inflammatory reactions (such as mast cell degranulation) (Hassan et al., 2024; Ampawong and Aramwit, 2016). An important reason for the low immunogenicity of serine is that it is rich in hydrophilic amino acids. Inspired by this, poly-β-homoserineand poly-DL-serine materials can substantially reduce foreign body reactions and are expected to replace polyethylene glycol as an ideal implantable biological material (Zhang et al., 2020; Zhang D. et al., 2021) (Table 1).
Figure 1. Sericin presents characteristics of good biocompatibility, low immunogenicity, inhibition of inflammatory responses, and good immune regulatory function. (A) Hematoxylin and eosin (H&E) staining following 10 days of implantation of AL, SF, SS1 and CH. AL, alginate; SF, silk fibroin; SS, sericin; CH, chitosan; MU, mouse muscles; HY, hydrogel-implanted sites. (B) ELISA results measuring total IgE, allergen-specific IgE, IgG (total) and induced by sericin, fibrinogen, PBS, and ovalbumin (OVA). Reprinted with permission from (Jiao et al., 2017). Copyright (2017) Wiley-VCH. (C) mRNA expression and cytokine production of proinflammatory factor in hPBMC from the psoriasis patients, who exposure to naringin (20 μg/mL), sericin (100 μg/mL), or sericin/naringin. Reprinted with permission from (Deenonpoe et al., 2019). Copyright (2019) BMC. (D) Expression of microphthalmia-associated transcription factor (MITF) and IL-4, IL-10, and TGF-β on melanocytes and DC 48 h after allergy induction treated with PEG or sericin (5, 10 and 20 μg/mL) + PEG. Reprinted with permission from (Aramwit et al., 2018). Copyright (2018) BMC. (E) Western blotting results of IL-10, IL-1β, and TNF-α in the young mice, adult mice, and older adult mice + oral sericin treatment groups (250 mg/kg, 21 days). Reprinted with permission from (Seyedaghamiri et al., 2021). Copyright (2021) Springer nature. (F) Arg-1 and iNOS immunofluorescence staining of macrophage treated with 0.1 mg/mL, 1 mg/mL LMW-sericin (<10 kDa) and 10 ng/mL lipopolysaccharide (LPS). Reprinted with permission from (Cherng et al., 2022).Copyright (2022) Frontiers.
Inflammation is the body’s defense response to injury or infection and involves a variety of cellular and molecular mechanisms. In the process of tissue healing, inflammatory cells such as macrophages and neutrophils are first recruited to the injury site and release pro-inflammatory factors such as interleukin-1 beta (IL-1β), IL-6, tumor necrosis factor α (TNF-α), etc. These factors promote vascular dilation and increased permeability, attracting more immune cells to participate in the inflammatory response. It also activates the degradation and remodeling of extracellular matrix. Subsequently, anti-inflammatory factors such as IL-4 and IL-10 begin to play a role, inhibiting the production of pro-inflammatory factors, reducing the activity of inflammatory cells, and promoting tissue repair and regeneration (Eming et al., 2017). The balance of inflammation and inflammatory factors is crucial for tissue healing. Excessive inflammatory response may lead to increased tissue damage, while insufficient anti-inflammatory factors may delay the healing process.
Sericin preparations have found application in skin repair, blood sugar reduction, and treatment of acute myocardial infarction (Wang D. et al., 2022; Aramwit et al., 2013; Tuentam et al., 2022; Song et al., 2016) (Figure 1C). There are three main mechanisms through which sericin inhibits the inflammatory response: (i) inhibits infiltration and proliferation of inflammatory cells (Zhaorigetu et al., 2003; Chlapanidas et al., 2013); (ii) inhibits expression of IL-1β, IL-6, IL-23, etc (Song et al., 2016; Chlapanidas et al., 2013; Deenonpoe et al., 2019; Kumar J. P. et al., 2018; Dong et al., 2020; Farajdokht et al., 2021); and (iii) increases the expression of IL-4 andIL-10, etc (Seyedaghamiri et al., 2021; Aramwit et al., 2018) (Figures 1D, E). Notably, Sun et al. revealed the mechanism of sericin inhibiting lipopolysaccharide (LPS) induced inflammation by multi omics integration: (i) sericin inhibits LPS-activated PRRs, Toll-like receptors and NOD-like receptors pathways; (ii) sericin significantly downregulates the expression of the MyD88 and NOD1; (iii) sericin decreases the expression of IL-1β, IL-6, INOS, etc (Sun et al., 2022). Interestingly, I-sericin is induced by γ-irradiation of sericin, and exhibits potent anti-inflammatory activities as the parent molecule, including reduction of oxidative stress-induced inflammatory cytokines cyclooxygenase-2 (COX-2), inducible nitric oxide synthase (iNOS), TNF-α, IL-1β and alleviation of LPS-induced inflammation (Choi et al., 2023; Song et al., 2020).
Sericin reportedly modulates epidermal immune responses in patients with psoriasis by reducing cytokine production by Th17 cells, upregulating galectin-3 (Lgals3) and down-regulating sphingosine-1-phospholyase 1 (Sgpl1) (Rujimongkon et al., 2021). As recently shown, low molecular weight (LMW) sericin (<10 kDa) enhances immune regulation in vitro: LMW-sericin (0.1 mg/mL) can upregulate the expression of CXCL9, IL-12A, BMP-7, and IL-10 in macrophages; balance Th1 and Th2 levels; and induce M2 polarization of macrophages. Sericin regulates macrophage proliferation to achieve immune regulation (Cherng et al., 2022) (Figure 1F). In addition, I-sericin has immune-enhancing effects, manifested as a significant increase in lymphocyte proliferation and activation of NK cells (Song et al., 2020). In addition, biosynthetic sericin 1-like protein can induce tolerant dendritic cells (DCs), which have excellent immunomodulatory capabilities. The purity of sericin 1- like protein is positively correlated with the anti-inflammatory effect of sericin; therefore, it is expected to be developed as an immune modulator (Song et al., 2020; Ritprajak et al., 2021).
In summary, the good biocompatibility and low immunogenicity of sericin are now widely recognized, making it a new avenue for drug delivery and tissue engineering (Wang C. et al., 2022; Sapru et al., 2021). Moreover, the anti-inflammatory properties and good immune regulatory functions of sericin will greatly expand its clinical applications.
SF exhibits good biocompatibility and low immunogenicity; therefore, it is favored for application in biological materials. Many studies have explored inflammatory processes in vitro or in vivo of SF-based biomaterials in the form of hydrogels, scaffolds, films, and nanoparticles (Table 2).
SF can achieve solution-gel transition by altering the pH, temperature, and solvation state, or by increasing biopolymer dynamics (Matsumoto et al., 2006). Studies on the biocompatibility and low immunogenicity of hydrogels are often based on the histological evaluation of tissue reactions, with the main focus of observing changes in inflammatory markers, such as neutrophils, eosinophils, and macrophages, in the SF hydrogel and the surrounding tissues (Fu et al., 2022; Etienne et al., 2009; Maity et al., 2022) (Figure 2A). However, these methods are not sufficiently precise to determine the biocompatibility and low immunogenicity of SF hydrogels. Therefore, advanced equipment and technical means are required to explore the inflammatory reaction caused by SF hydrogels in detail. Recently, using noninvasive bioluminescence imaging, Gorenkova et al. demonstrated that SF hydrogels elicited an acute but mild local inflammatory response in vivo, which elicited an innate immune response similar to that elicited by polyethylene glycol (PEG) hydrogels (Gorenkova et al., 2021) (Figure 2B). The Forster/fluorescence resonance energy transfer (FRET)-based sensor experiment developed by Kambe et al. first exposed the initial immune response of SF hydrogels. SF hydrogels are surrounded or invaded by matrix metalloproteinases (MMP) within 24 h after implantation and undergo biodegradation within 3 h after implantation, which may favor immune cells (macrophages, foreign body giant cells) to achieve major degradation of the hydrogel over a period of weeks (Kambe and Yamaoka, 2021; Janani et al., 2022) (Figure 2C).
Figure 2. Biomaterials constructed with SF causes a modest inflammatory response and has low immunogenicity and anti-inflammatory properties. (A) The H&E staining of skin tissue from the backs of mice subcutaneously transplanted with SF after 12 weeks. Reprinted with permission from (Etienne et al., 2009). Copyright (2009) Wiley-VCH. (B) Non-invasive imaging of the acute and chronic inflammatory response towards implanted SF and PEG hydrogels. Reprinted with permission from (Gorenkova et al., 2021). Copyright (2021) the ROYAL SOCIETY of CHEMISTRY. (C) Relative expression of proinflammatory and pro-remodeling genes after exposure to degradation products of silk (Bombyx mori (BM) silk; Antheraea assamensi (AA) silk, and BA silk and ECM (liver ECM (LECM) and small intestinal submucosa ECM (SIS)) bioscaffolds on proinflammatory activated macrophages. Reprinted with permission from (Janani et al., 2022). Copyright (2022) Elsevier. (D) H&E staining of colon in non-colitic, colitic control, SFNs and RGD-SFNs group. Reprinted with permission from (Rodriguez-Nogales et al., 2016) Copyright (2016) Dovepress. (E) Proinflammatory gene expression of diabetic wounds treated with PBS, nanosilk (made from SF), and nanosilk + cerium oxide nanoparticle-microRNA146a (CNP-miR146a) after 7 days. Reprinted with permission from (Niemiec et al., 2020). Copyright (2020) Frontiers. (F) H&E and Masson trichrome staining were performed on the control group, porous SF membrane group, and non porous SF membrane group at 1 and 4 weeks postoperatively. Reprinted with permission from (Yao et al., 2019). Copyright (2019) Wiley-VCH.
The surface morphology, physical structure, and chemical structure of the scaffold play a decisive role in the reaction with immune cells, and these features also regulate macrophage polarization at the host tissue implant interface (Antmen et al., 2021). The SF scaffold reportedly has good biocompatibility and low immunogenicity through histological and immunofluorescence staining analysis (Fu et al., 2022; Wang et al., 2008; Guan et al., 2013; Yang et al., 2021; Ge et al., 2012; Suzuki et al., 2019; Singh et al., 2018). Recent studies have revealed the effects of SF scaffolds on macrophages. SF scaffold implants can inhibit classical activated macrophages (M1) and stimulate alternatively activated macrophages (M2) to regulate local inflammatory responses (Janani et al., 2022; Yang et al., 2021). Mast cells, an important type of immune cells, can be activated by biomaterials, which can trigger inflammation by releasing histamine, cytokines and other mediators, promote recruitment and activation of macrophages and other immune cells, and affect the immune microenvironment at the transplant site of biomaterials. Garg K et al. found that SF scaffolds were not conducive to mast cell adhesion and proliferation, suggesting that they were largely immune inert (Garg et al., 2011). The origin, protein conformation, amino acid sequence, fiber thickness, and porosity of SF play important roles in determining the macrophage phenotype, monocyte responsiveness, and T-cell activity (Janani et al., 2022; Yang et al., 2021; Bhattacharjee et al., 2013). Therefore, it is necessary to consider factors that affect the immune response when designing SF scaffolds.
Evaluation of the innate and adaptive immunity of SF nanoparticles (SFNPs) in vivo demonstrate low immunogenicity and anti-inflammatory properties (Zhang Y. et al., 2021). SFNPs play an anti-inflammatory role and immunomodulatory properties in intestine of trinitrophenyl sulfonic acid-induced experimental colitis in rats, which is specifically manifested as reducing neutrophil infiltration, decreasing the expression of IL-1β and promoting the expression of IL-10, while functionalization of arginine–glycine–aspartic acid (RGD) peptide can significantly improve its anti-inflammatory properties (Rodriguez-Nogales et al., 2016) (Figure 2D). In addition, a SF nanosilk solution reportedly reduces gene expression of the proinflammatory factor IL-6 accompanied by a tendency to reduce inflammatory cell infiltration early in the healing process (Niemiec et al., 2020) (Figure 2E).
SF films are also biocompatible, have low immunogenicity, and can reduce the infiltration of inflammatory cells. SF films are mainly used in wound healing and tissue repair (Ge et al., 2012; Arthe et al., 2020; Yao et al., 2019) (Figure 2F). In addition, the transparent artificial dura made from SF effectively reduces the expression of IL-1β, IL-6, and TNF-α (Kim et al., 2011).
Notably, peptides produced by SF hydrolysis exhibit anti-inflammatory potential. SF peptide alone inhibits TPA-induced increase in COX-2, IL-6, IL-1β, and TNF-α levels, and significantly enhances the anti-inflammatory activity of Tat-SOD and PEP-1-FK506 binding proteins (Kim et al., 2011; Kim et al., 2012). γ-irradiated SF significantly enhances various aspects of the immune systems by activating NK cells, T-cell proliferation, NO production, and increasing cytokine levels (Byun et al., 2010). The anti-inflammatory potential of the SF hydrolysate and irradiation products suggests that further processing and treatment of SF will be a new strategy to explore its value.
Compared with the current artificial materials, such as polylactic acid, PEG, etc., the degradation products of sericin and SF are small molecular amino acids, and possess lower inflammatory response and better biocompatibility, while the degradation products of artificial materials such as polylactic acid will produce obvious inflammatory response by reducing the pH value of the environment (Ma et al., 2024). Chemical crosslinking is an important strategy to reduce the immunogenicity of biomaterials. Based on the active groups on various amino acid residues in sericin and SF, it can be chemically modified to meet the needs of different applications. For instance, by introducing methacrylic acid group into the amino acid side chain of SF protein, the water solubility of SF protein can be improved, thus reducing the immunogenicity (Kim et al., 2021). Reducing the antigenic epitopes exposed by material surface modification is another important strategy to reduce the immunogenicity. For example, the introduction of specific bioactive molecules, such as PEG, on the surface of fibroin proteins can reduce the immunogenicity of SF (Wei et al., 2020).
Biomaterials implanted in the body will cause FBR, including local aseptic inflammatory responses, such as inflammatory cell infiltration, including macrophages, lymphocytes, neutrophils, etc. Over time, foreign-body giant cell form and eventually lead to fibrosis (Cai et al., 2024). In order to overcome the FBR, it is necessary to pay attention to the following characteristics when preparing sericin-based and SF-based biomaterials. Firstly, the mechanical properties of the hydrogels should be suitable for the implant site, and the lower stiffness helps to mitigate FBR (Blakney et al., 2012). Secondly, preventing protein adsorption by binding hydrogels with PEG or other novel anti-fouling biomaterials (such as zwitterionic or hydroxyl-rich polypeptides) is another attractive option (Zhou et al., 2024). Another method to mitigate FBR is to use biomimetic materials (such as decellularized extracellular matrix and mucins) mimic the extracellular matrix (ECM) of native tissues (Bhunia et al., 2023; Werlang et al., 2024). In regard to nanoparticles, surface functionalized by different chemical groups can affect the intensity of the FBR (Huang Y. J. et al., 2018; Huang et al., 2015). It is noteworthy that the membrane encapsulation to reduce the FBR of the nanoparticle has become another novel way (Fan et al., 2018).
Finally, the composition and MW of sericin and SF also play a key role in its immunogenicity. SF is a fibrous protein consisting of a heavy chain (H chain) (390 kDa), a light chain (L chain) (26 kDa), and a glycoprotein P25 (30 kDa), which are assembled in a ratio of 6:6:1 (Inoue et al., 2000). Sericin is also a macromolecular protein, with MW ranges from about 10 kDa to over 300 kDa (Zhang, 2002). The MW of them is greatly affected by the extraction conditions. LMW-sericin (below 10 kDa) not only displays good biocompatibility, but also owns good anti-inflammatory ability to regulate macrophage polarization towards the M2 phenotype (Cherng et al., 2022). Therefore, it is another way to reduce the immunogenicity of sericin-based and SF-based biomaterials by optimizing the extraction and purification process to obtain the appropriate MW of sericin and SF.
Due to the good biocompatibility, low immunogenicity and outstanding immunomodulatory properties, sericin is highly favored in the biomedical field. Sericin-based biomaterials have shown excellent effects in improving immunotherapy and anti-inflammatory.
Immunotherapy is mainly a method of treating diseases by artificially enhancing or inhibiting the body’s immune function. It is suitable for treating various diseases, including cancer and autoimmune diseases. Currently, the drug delivery system using sericin as a biological material for immune agents become a very promising method for immunotherapy.
Small interfering RNA (siRNA) is essential for the effective inhibition of tumorigenesis, targeting of tumor metastasis, and activation of tumor-associated immune cells via silencing the specific gene (such as p65 and PD-L1) responsible for different cancer hallmarks (Ngamcherdtrakul and Yantasee, 2019). Using an effective delivery system, siRNAs can be selectively targeted to tumor microenvironment (TME), sent to regulatory T cells (Tregs), macrophages, myeloid-derived suppressor cells, and other cells to “silence” immunosuppressive cells, and enhance therapeutic immunotherapy (Deng K. et al., 2022; Qian H. et al., 2022; Li S. Y. et al., 2016; Hossain et al., 2015). Currently, sericin as a biological material has been designed as a delivery system for targeted delivery of siRNA. For example, a system consisting of superparamagnetic iron oxide nanoparticles (SPIONs) modified with sericin can target triple-negative breast cancer, accelerate tumor necrosis, and inhibit tumor proliferative growth (Shirangi et al., 2022). Furthermore, hyaluronic acid/poly-L-lysine-siRNA/albumin-sericin (2:1) nanoparticles can be used as siRNA delivery system for laryngeal cancer treatment (Yalcin et al., 2019). Therefore, it is expected that, in the future, serine will be increasingly used as a biological material to design a drug delivery system to target siRNA to immunosuppressive cells in the TME, which will be a potential new direction for enhancing immunotherapy.
Photothermal therapy (PTT) and photodynamic therapy (PDT) are two novel cancer treatments. Their anti-tumor efficacy can be improved by inducing non-invasive pyroptosis of cancer cells and stimulating anti-tumor immune responses. For example, recently prepared VB12-Sericin-PBLG-IR780 nanomicelles not only trigger programmed pyroptosis in cancer cells but also activate DC maturation, initiate T-cell recruitment, and play a key role in anti-tumor processes (Guo et al., 2022) (Figure 3A).
Figure 3. Application of sericin-based biomedical composites in immune regulation. (A) The preparation process and mechanism of VB12-sericin-PBLG-IR780 nanomicelles mediated pyroptosis are related to DC maturation, T cell recruitment, and tumor inhibition efficiency. Reprinted with permission from (Guo et al., 2022). Copyright (2022) ACS. (B) Treatment of atopic dermatitis in mice with siRelA sericin (SC) hydrogel. Observation of ear swelling (HE staining) and mast cell infiltration (TB staining) in tissue sections through optical microscopy. Reprinted with permission from (Kanazawa et al., 2015). Copyright (2015) MDPI. (C) Se-CuSrHA@EGCG Preparation and functional model diagram of nanoplatforms, including clearance of ROS, regulation of immune response, angiogenesis, and bone formation. Reprinted with permission from (Ming et al., 2025). Copyright (2025) Elsevier. (D) Chitosan (CS) - NPs gel, CS Lupeol (L) - NPs gel, CS Ag NPs gel and CS Ag L-NPs gel were used to treat skin wounds. The expression of TNF - α and IL-1 β in tissues was detected by immunohistochemistry. Reprinted with permission from (Chu et al., 2023). Copyright (2023) Elsevier. (E) Treated with IL-4 and alginate/sericin/graphene oxide (Alg/Ser/GO) hydrogel, the frequency of CD206+CD163+and CD11b+CD80+cells was analyzed by flow cytometry. Reprinted with permission from (Jiang et al., 2021a). Copyright (2021) Elsevier.
In addition, during the treatment for tumors, although the use of chemotherapy drugs inhibits tumor formation and development, it can also have a negative impact on the immune system. Lactoferrin (LF), a common iron-binding glycoprotein, not only has the function of regulating iron metabolism, but also plays a crucial role in antibacterial, antiviral, anti-tumor and immune regulation (Jańczuk et al., 2022). A sericin hydrogel system for the delivery of recombinant human LF (SSH-rhLF) can prolong the bioactivity and bioavailability of rhLF and may have a therapeutic effect on the cyclophosphamide (CTX) induced immunosuppression mice by enhancing the function of liver macrophages, promoting the expression of immunoregulatory factors (IL-2, IL-21, IL-18, and CD-3), and promoting the proliferation of splenic lymphocytes (Xu et al., 2021). This provides a new strategy for enhancing immunity in patients undergoing cancer chemotherapy.
Atopic dermatitis (AD) is a common chronic inflammatory disease, for which immunotherapy is an important treatment (Li and Man, 2022). Sericin prepared in emulsion gel and hydrogel forms is very effective in correcting the abnormal immune response caused by AD by loading levocetirizine (an antihistamine), anti-RelA siRNA, and functional peptides (Pal et al., 2019; Kanazawa et al., 2015) (Figure 3B). Intra-articular injection of sericin hydrogel preparations containing anti-RelA siRNA also has great therapeutic potential for the treatment of rheumatoid arthritis (RA) (Kanazawa et al., 2017). Moreover, sericin-based microspheres loaded with racemic flavanone naringenin (a TNF-α blocker) helped to suppress LPS-induced serum TNF-α levels, which mediate immune disorders in psoriasis, and thus are expected to be a new modality for psoriasis immunotherapy (Chlapanidas et al., 2014).
Sericin has good adhesion and hydrophilicity, which helps to regulate the mechanical properties of biomaterials, enhance their degradation ability, promote cell adhesion and proliferation, and facilitate the sustained release of anti-inflammatory drugs, thereby enhancing their anti-inflammatory effects. From a mechanistic perspective, the drug delivery system and composite biomaterials involved in sericin play an anti-inflammatory role, mainly by promoting the M2 polarization of macrophages, inhibiting the proliferation and infiltration of inflammatory cells, and regulating the secretion of inflammatory mediators.
Macrophages are among the first cells to arrive at and interact with implanted materials and involved in regulating the resolution of inflammation, promoting tissue repair and regeneration. Mature macrophages are polarized into M1 or M2 subtypes. Classically activated M1 induced by IFN-γ, exhibit a proinflammatory phenotype. Activated M2 induced by IL-4 or IL-13 exhibit an anti-inflammatory phenotype (Kumar et al., 2016). During tissue repair, excessive and prolonged activation of proinflammatory M1 macrophages in the early stages of inflammation can lead to increased inflammation, thereby impairing tissue repair and regeneration (Martin and García, 2021; Bessa-Gonçalves et al., 2023). Therefore, accelerating the change in the macrophage from M1 to M2 is an important strategy in both biomaterial development and tissue repair/regeneration.
The polarization response of macrophages to biomaterials is currently being explored in three main approaches: (i) immunofluorescence staining using M1 surface markers (such as chemokine receptors 7, CCR7) and M2 surface markers (such as CD206) and observing results (Wang et al., 2020); (ii) determining cytokine secretion profiles of macrophages, which indirectly reflect macrophage phenotype (Wang et al., 2020; Chachlioutaki et al., 2022); (iii) examining the expression of M1 and M2 genes (Wang et al., 2020; Zhang et al., 2017; Jiang et al., 2021a). Sericin composite biomaterials in the form of scaffolds, films, hydrogels, and other treatments combined with drugs such as ketoprofen (an anti-inflammatory agent), nerve growth factor, and exosomes inhibit inflammatory responses by promoting macrophage M2/M1 conversion, which is exploited for tissue and peripheral nerve repair (Chachlioutaki et al., 2022; Zhang et al., 2017; Jiang et al., 2021a). Recently, the bone immune microenvironment, in which macrophages play a key role in reprogramming of the bone regeneration and immune microenvironment, has attracted increasing attention. Sericin released in an injectable alginate/serin/graphene oxide (Alg/Ser/GO) hydrogel promotes M2 polarization and migration through nuclear factor kappa-B (NF-κB) and mitogen-activated protein kinase (MAPK) signaling, inducing osteogenic differentiation and bone regeneration (Jiang et al., 2021a). Se-CuSrHA@ (epigallocatechin -3- gallate) EGCG (Figure 3C), a sericin-based nanoplatform, increases mRNA level of Arg-1 and CD206, increases the secretion of anti-inflammatory cytokines, inhibit M1 while promoting the polarization of M2, balanced immune homeostasis, and accelerating bone regeneration (Ming et al., 2025). In another study, serine incorporated into a gelatin sponge polarizes M1 macrophages and promotes bone morphogenetic protein 2(BMP-2) secretion to enhance osteogenesis (Jo et al., 2021). Therefore, the factors through which sericin materials determine macrophage phenotypes may be complex and require further exploration.
Sericin-composite biomaterials can also inhibit inflammatory cell proliferation and infiltration in the treatment of inflammatory diseases. Sericin-loaded alginate nanoparticles significantly reduce polymorphonuclear cell (PMN) infiltration and inhibit carrageenan-induced paw edema (Khampieng et al., 2015). The sericin/proanthocyanidin (PAC) composite reverses histological damage, including inflammatory cell infiltration and goblet cell loss, slowing disease progression and is a promising alternative therapeutic strategy for dextran sulfate sodium (DSS) -induced ulcerative colitis (UC) (Wang C. et al., 2022). In addition, the reduction of inflammatory cells in the surrounding tissues was observed at the initial stages of implantation of the alginate/sericin/graphene oxide (Alg/Ser/GO) hydrogel, which is conducive to bone regeneration (Jiang et al., 2021a). Sericin can promote chondrocyte differentiation and growth by activating the Smad2/3/TGF-β pathway and regulate local proinflammatory responses (Fongsodsri et al., 2024).
In terms of wound treatment, carboxymethyl cellulose/sericin-based hydrogel dressing can downregulate the IL-1β, IL-6, and TNF-α to improve the pro-inflammatory response at the diabetic wound site (El-Samad et al., 2022; Bai et al., 2023). Hydrogels made of fibroin and sericin are excellent at promoting wound healing and reducing inflammation (Zhang D. et al., 2024; Ashraaf et al., 2023). Using sericin as a biological template, CuS@Ser NPs stimulate angiogenesis and inhibited inflammation, thereby facilitating rapid wound healing (Guo et al., 2023).
Another bacterial cellulose wound dressing made from sericin/polyhexamethylene biguanide has a strong ability to promote tissue secretion of IL-4 and TGF- β, thereby achieving a more efficient regulatory ability to promote wound treatment (Napavichayanun et al., 2018). In terms of psoriasis treatment, the combination of naringin and sericin in equal proportions significantly reduced the production IL-6, TNF-α and IL-23 in patients (Deenonpoe et al., 2019). Sericin-based poly (vinyl) alcohol (SS/PVA) hydrogel alleviates the development of psoriasis symptoms by up-regulating nuclear factor erythroid 2 related factor 2 (Nrf2), IL-10, and down-regulating TNF-α and IL-20 (Tuentam et al., 2022). In terms of colitis treatment, sericin composite biomaterials can alleviate UC by reducing the levels of proinflammatory cytokines TNF-α, IL2, IL-6, IL-17, and IL-12 and upregulating the levels of the anti-inflammatory cytokine IL-10 (Xu et al., 2022; Fongsodsri et al., 2024; Ma et al., 2019). The mixture of sericin and curcumin can inhibit carrageenan-induced foot edema in mice by suppressing the release of IL-1 β, promoting the secretion of IL-4 and IL-10 (Ashraaf et al., 2023).
The sericin composite biomaterials loaded with drugs can be used to treat inflammatory diseases by reducing the release of proinflammatory mediators, upregulating anti-inflammatory cytokines and macrophage polarization (Jiang et al., 2021a; Chu et al., 2023) (Figures 3D, E). Sericin base biomaterial is a new and gradually emerging therapeutic strategy for wound treatment.
Compared with sericin, SF is more widely used in the field of tissue engineering and regenerative medicine attributed to its better mechanical properties and versatile processing capabilities. SF-based biomaterials have also played an outstanding role in enhancing immunotherapy and anti-inflammatory.
SF has biocompatibility, controlled-release characteristics, and excellent mechanical and biological properties, such as immunomodulatory and anti-inflammatory properties, making it an excellent choice for the construction of composite biomaterials for tumor immunotherapy. Surface-engineered SF nanocomposites not only exhibit excellent anti-tumor functions but can also be combined with different types of tumor therapies to enhance tumor immunotherapy. The related mechanisms mainly include: (i) reversal of the immunosuppressive TME, including inducing the transformation of tumor-associated macrophages from M2 to M1 and reducing the number of Tregs (Zhang X. et al., 2022; Yu et al., 2022a; Tan et al., 2020; Yu et al., 2023) (Figures 4A, B); (ii) induction of immunogenic cell death (ICD) to stimulate anti-tumor immunity (Zhang X. et al., 2022; Yu et al., 2022a; Tan et al., 2020; Yu et al., 2023; Tkach et al., 2017); and (iii) promotion of the maturation of DCs, the formation of effector T cells and effector memory T cells to improve immune activation and promote immune memory (Zhang X. et al., 2022; Yu et al., 2022a; Tan et al., 2020; Tkach et al., 2017; Cao et al., 2022) (Figures 4A–C).
Figure 4. Application of SF-based biomedical composites in anti-tumor immune response and enhancing the immune response to vaccines. (A) Flow cytometry analysis showing the frequency of M1 and M2 macrophages after various treatments: ultrasound (US), bovine serum albumin (BSA)+US, SF + US, Au/SF@Cu2-xS nanoreactor (ASC), and ASC + US. Reprinted with permission from (Yu et al., 2022). Copyright (2023) Elsevier. (B) Schematic illustration of the synthetic route of NST NPs (metal-organic framework nanosystem (NMOF)+SF + tirapazamine (TPZ)) and the mechanism of synergistic induction of strong immune response by deoxygenation driven chemotherapy in the treatment of tumor specific redox imbalance. Reprinted with permission from (Yu et al., 2022a). (C) Impact of different types of oral nanomotors (NMs)-embedded hydrogel plus anti-PD-L1 on DC maturation, the ratio of CD8+/CD4+ T cells and changes, central memory cells (TCM), effector memory T cells in the mouse spleen and variations in cytokine levels (e.g., TNF-α and IFN-γ) in the mice serum. Reprinted with permission from (Cao et al., 2022). Copyright (2022) Wiley-VCH. (D) (a) Schematic of the fabrication and application to skin of SF/poly (acrylic acid) (PAA) composite microneedles. (b) ELISA analyses of anti-trimer-His serum IgG responses over time. Reprinted with permission from (Boopathy et al., 2019). Copyright (2018) PNAS. (E) Kaplan–Meier survival curves showing that MIMIX (SF-based microneedle patch) reduced mortality relative to control, whereas bolus vaccination vaccines did not significantly improve survival rates. Reprinted with permission from (Stinson et al., 2021). Copyright (2022) Elsevier. (F) Immunofluorescence staining in acute or steady-state conditions: Adding SF to vaccines can induce stronger immune responses and increase the distribution of CD4+T cells in the gastric mucosa. Reprinted with permission from (Hu et al., 2020). Copyright (2020) Taylor and Francis.
Transcutaneous immunization (TCI) enhances tumor immunotherapy by delivering antigens to DCs through skin. Compared to traditional oral or injection vaccinations, it has the advantages of excellent immunogenicity, avoidance of the liver first-pass effect, good compliance, safety, high efficiency, non-invasiveness, and ease of use (Karande and Mitragotri, 2010). However, the presence of a cuticular barrier leads to a low transdermal delivery efficiency of TCI, thereby limiting its large-scale clinical application. SF has good biocompatibility, air permeability, and skin affinity, and can mimic the extracellular matrix, making it a good biological material for constructing transdermal drug delivery systems. Composite biomaterials constructed using SF can Stimulate cellular and humoral immune responses and induce systemic anti-tumor response by improving the transdermal properties of transdermal carriers, targeting, and inducing DCs maturation (Yang et al., 2020; Song et al., 2022). According to recent researches, the combination of SF-constructed percutaneous tumor immune materials and immune checkpoint blockers, such as programmed cell death protein 1 monoclonal antibody (aPD-1) which can enhance T-cell responses and mediate preclinical antitumor activity by blocking the binding of PD-1 on T cell with PD-L1 on cancer cells, may be a new strategy for effectively enhancing tumor immunotherapy. The involved mechanisms include: (i) promotion of the infiltration of CD4 and CD8 T cells into the tumor tissue, and (ii) promotion of the expression of IL-12, IFN-γ, and TNF-α (Song et al., 2022; Hong et al., 2021).
It is also of interest that SF has been used as a vaccine carrier for cancer immunotherapy. For example, Lei et al. recently developed an injectable SF microsphere loaded with an antigen and an immune adjuvant. Its macroporous structure is conducive for the recruitment of immune cells and can promote the activation of DCs to forms a favorable immune microenvironment. In turn, strong humoral and cellular immunity is induced. In addition, an enhanced vaccine modified by adsorbing antigens on SF microspheres effectively inhibits tumor growth by improving the cytotoxic T lymphocyte (CTL) response (Lei et al., 2022), properties that encourage a new approach for the future development of tumor immunotherapy vaccines.
Antigen delivery dynamics can influence the immune response to vaccines. For example, vaccine antigens can induce sustained humoral immunity after they are delivered to the lymph nodes to trigger naïve B cell response, whereas traditionally injected immunization can rapidly eliminate antigens, which is not conducive to the establishment of humoral and cellular immunity. Improving the immunogenicity of vaccine antigens and maintaining their slow release are new strategies for enhancing vaccine efficacy. The ability of the SF matrix to enclose and release intact and bioactive immunologically active materials has attracted much attention in the construction of slow-release novel vaccines (Reeves et al., 2015; Guziewicz et al., 2011; Kumar M. et al., 2018) (Figure 4D). The microneedle patch combined with the SF matrix can achieve the continuous release of vaccine antigens, enhance the immunogenicity of the vaccine, and thereby significantly enhance the degree, duration, and breadth of the humoral and cellular immune responses caused by the vaccine. Therefore, this represents a promising vaccine delivery strategy at present (Boopathy et al., 2019; Stinson et al., 2021; DeMuth et al., 2014) (Figure 4E).
SFNPs can enhance antigen target delivery, immunogenicity, and stability and can release antigens slowly and continuously, making them a promising new vaccine preparation. SFNPs was used as nanoadjuvants to deliver recombinant hepatitis B surface antigen (HBsAg) and FimH-IutA antigen, with the resulting vaccine significantly increasing the content of specific antibody IgG and promoting humoral and cellular immune responses (Rezaei et al., 2021; Hasanzadeh et al., 2020). Similarly, SF, which presents the advantages of sustained release, absorption, and in situ gelation in various tissues, has been used as a mucosal vaccine carrier. This vaccine carrier not only alleviates gastric injury but also leads to significant infiltration and generation of CD4 tissue-resident memory T (TRM) cellsin gastric epithelial tissues, which are the key mediators of anti-infection immunity in various tissues and have recently been shown to boost local stomach immunity (Schenkel et al., 2014; Xu et al., 2019; Hu et al., 2020) (Figure 4F).
CpG oligodeoxynucleotides (CpG ODNs) are short single-stranded synthetic DNA molecules which are designed to mimic bacterial DNA. These molecules are recognized by Toll-like receptor 9 (TLR9), which is expressed in certain immune cells such as myeloid cells, thus possess potent immune-stimulatory properties (Hekmatshoar et al., 2019). SFNPs have been used as effective carriers of CpG ODNs, which can significantly improve the delivery of CpG ODNs, as shown by the significantly enhanced cellular uptake and significantly increased levels of cytokines and nitric oxide produced following CpG ODN stimulation (Zhang et al., 2019).
SF has particularly excellent biocompatibility and can slowly degrade in vivo, has an excellent mechanical strength, and increases cell adhesion. Injectable hydrogels prepared by combining SF with other materials achieve controlled biodegradation and low mass loss and can be loaded with immunosuppressive agents such as methylprednisolone and betamethasone for cartilage regeneration and the treatment of rheumatoid arthritis (Phan et al., 2022; Oliveira et al., 2020).
Human bone marrow mesenchymal stem cells (hBMSC) are widely used in cell therapy because of their powerful proliferative and immune-regulatory abilities. SF films reportedly preserve not only the immunosuppressive effects of hBMSCs on T-cell proliferation and cytokine release, but also IL-6 secretion, and the immunophenotypes of hBMSCs (Luan et al., 2009).
M2 macrophage polarization is an important immune regulatory event that reduces inflammation during wound repair, bone regeneration and repair, and colitis repair. Many composite biomaterials targeting this key event have been developed based on the excellent mechanical properties, biocompatibility, and bioactivity of SF. For example, Silk-6/ε-PL@Exo (constructed from SF/poly-L-lysine hydrogel) controls inflammation, inhibits glycolysis and lactic acid accumulation by targeting M1 macrophages, and promotes the polarization of macrophages from M1 to M2 (Jin et al., 2024). The inflammatory response induced by SF is not fixed: SF/nano-hydroxyapatite scaffolds trigger a proinflammatory response by M1 macrophages on the first day, whereas SF degradation products induce an anti-inflammatory response by M2 macrophages on the day 24 of treatment (Wong et al., 2024). Lv et al. found that SF treated with reagents such as acetic acid and sodium hydroxide increased the expression of proinflammatory cytokines in rats and accelerated their degradation in vivo. Overall, most studies suggest that SF has good biocompatibility and anti-inflammatory properties.
Owing to the dissolution of the scaffold by protease K in the body, the secondary/tertiary structure of SF is altered, leading to significantly different immune responses. Maintaining the stability of SF in the body is of great clinical value, and bioactive gold cluster sutures (clusters assembled on the SF surface) ensure the structural stability of SF for 15 months without degradation in vivo (Tian et al., 2024).
Wound treatment promotes M2 polarization of macrophages to establish anti-inflammatory niche required for tissue healing, which is critical for skin wound treatment. SF hydrogels can significantly increase the expression of the anti-inflammatory marker CD163 in M2 macrophages in the early stage, accelerating the transition from inflammation to the proliferation stage of wound repair (Chouhan et al., 2018). Considering that the SF hydrogel system has a controlled drug delivery capacity, good mechanical properties, skin tissue adhesion, and bioactivity, the combination of other biomaterials and therapeutic drugs will not only be beneficial for promoting angiogenesis in the wound area but will also induce M2 polarization in the wound area to create a pro-healing anti-inflammatory microenvironment. For example, after in situ photocuring, methacrylonyloxylated SF hydrogel showed good adhesion and sealing properties. Currently, two methacryloxylated SF hydrogel systems loaded with borosilicate and metformin have been prepared, both of which can regulate inflammation by inducing macrophage polarization towards the anti-inflammatory phenotype M2 and support diabetic wound treatment (Pang et al., 2021; Mei et al., 2022; Wu et al., 2021; Wang et al., 2023; Li et al., 2023). In addition, novel glycyrrhizic acid and inorganic zinc in the immunoregulatory SF/novel glycyrrhizic acid/inorganic Zn2+ (SF/GA/Zn) hydrogels synergistically reduce the activation of M1-type macrophages, induced an M2 phenotype shift, and accelerate the three stages of diabetic wound repair (Qian Y. et al., 2022) (Figure 5A).
Figure 5. Application of SF-based biomedical composites in inhibiting inflammation. (A) Preparation of SF/GA/Zn hybrid hydrogel and its immunomodulatory mechanism in wound treatment of diabetes. Reprinted with permission from (Qian Y. et al., 2022). Copyright (2022) Wiley-VCH. (B) Schematic of the osteo-immunomodulatory effects of three-dimensional-printed biodegradable cellulose nanoparticles-reinforced chitosan/silk fibroin (CS/SF/CNPs) scaffolds. Reprinted with permission from (Patel et al., 2022). Copyright (2022) Elsevier. (C) The CD68 antibody fluorescence staining image confirmed the inhibitory effect of the hybrid poly (glycolide-co-ε-caprolactone) (PGCL)/SF-Tubasatin A (TUBA) multi-channel bioactive filament nanofiber catheters on inflammation after SCI. Reprinted with permission from (Liao et al., 2022). Copyright (2022) Elsevier. (D) RT-PCR displays the expression levels of genes MMP-1, MMP-13, iNOS, and TNF-α2 activated by IL-1β, were dramatically lower in the silk-chondroitin sulfate (CS) scaffolds compared with the silk scaffold. Reprinted with permission from (Zhou et al., 2017). Copyright (2017) Elsevier. (E) The expression of IL-6, TNF-α, and IL-10 showed that the implantation of collagen/SF scaffold combined with human umbilical cord mesenchymal stem cells (hUCMSCs) regulated systemic inflammatory factor levels in the acute and chronic stages of traumatic brain injury. Reprinted with permission from (Jiang J. et al., 2021). Copyright (2021) Theranostics.
SF can co-self-assemble with VEGF-mimicking peptides to construct an immunoregulatory hydrogel, QK-SF, which supports tissue repair and wound healing by regulating macrophage polarization and promoting angiogenesis (Chen Z. et al., 2023). Similarly, gelatin methacrylate/silk fibroin glycidyl methacrylate/mesoporous silica NP-resveratrol/platelet-derived extracellular vesicles (GelMA/SFMA/MSN-RES/PDEVs) hydrogels have appropriate mechanical properties and swelling ratios, which allow sustainable release of MS-RES and PDEVs to regulate macrophage to M2 phenotype conversion, promote angiogenesis, and accelerate the diabetic wound treatment process (Zhu et al., 2022).
During the process of fracture healing, the first stage is acute inflammation, followed by a transition to repair and regeneration. Therefore, the development of bone immunoregulatory biomaterials that favor polarization of the M2-phenotype macrophages is a novel strategy for bone regeneration and repair (Hu et al., 2018). SFNPs improve the bioavailability of hydrophobic anti-inflammatory drugs. Ti-MAO/Sr/LBLWNP prepared according to this strategy can continuously release wogonin, which can transform M1 macrophages into M2 macrophages, regulate the ratio of M1/M2 macrophages, and promote osteoblast differentiation (Wang D. et al., 2022). SF also has the ability to release cytokines locally and can be used for the local release of sitagliptin to induce macrophages to polarize to the M2 phenotype and effectively recruit M2 macrophages to the titanium implant site to support bone regeneration (Xiang et al., 2021). In addition, the addition of SF/cellulose nanofibrils (CNFs) makes three-dimensional printing of chitosan/SF/cellulose nanoparticle scaffolds, and on activation of the M2 phenotype, macrophage polarization and immune regulation contribute to bone regeneration (Patel et al., 2022) (Figure 5B). A composite hydrogel composed of photoresponsive methacrylate SF, laponite nanocomposite, and tannic acid has the ability to resist oxidation and inflammation and induce bone formation (Wang et al., 2024). SF/black phosphorus/lycrhizic acid nanocomposite hydrogels can weaken the damage caused by oxygen free radicals, promote macrophage polarization towards M2, inhibit proinflammatory effects, and enhance the repair of damaged bone marrow (Zhang B. et al., 2024). Multilayered regenerated SF (RSF) on the surface of PET artificial ligaments regulates the inflammatory response and promotes the maturation of intra-articular grafts (Chen N. et al., 2023).
Oral nanoparticles have been used to treat ulcerative (UC) as they can deliver drugs directly to the colonic region and are more convenient, achieving high patient compliance and safety (Zu et al., 2021). Therapeutic strategies for the treatment of UC include enhancing inflammation resolution, alleviating oxidative stress, promoting colonic mucosal repair, and regulating the intestinal flora. Macrophage M2 polarization is conducive to resolving inflammation and promoting mucosal healing, and has recently been shown to involved in the treatment of inflammatory bowel disease (IBD) (Koelink et al., 2020; Sun et al., 2020). At present, improving the targeting and co-treatment of UC from multiple aspects is the most recent strategy for preparing UC therapeutic drug delivery systems. Specific ideas include (i) modifying oral nanoparticles with ligands that specifically target colonic epithelial cells or macrophages (Li et al., 2022) and (ii) using drugs with multiple therapeutic functions (Zu et al., 2021). In line with this strategy, SFNPs have been designed as oral nanodrug delivery systems to exert multiple therapeutic effects (such as promoting macrophage M2 polarization) by the targeted delivery of therapeutic agents to the colonic mucosa and to significantly alleviate UC symptoms (Liu et al., 2022; Du et al., 2022; Ma et al., 2022; Gou et al., 2019).
In addition, the prepared SF composite biomaterials with immune regulatory functions can regulate macrophage M2 polarization, which is used to promote tendon repair, prevent tendon adhesion, and promote pelvic floor tissue repair (Cai et al., 2023; Dong et al., 2021; Yin et al., 2022).
Composite biomaterial systems in the form of hydrogels, scaffolds, and nanoparticles prepared using SF can be loaded with anti-inflammatory drugs, endowing them with anti-inflammatory activity by inhibiting the infiltration and proliferation of inflammatory immune cells. For example, SF hydrogels loaded with EGCG, rhein, and glycyrrhizic acid can effectively reduce the infiltration and proliferation of inflammatory cells (Qian Y. et al., 2022; Yin et al., 2022; Lee et al., 2022; Zhang F. et al., 2022). For the scaffold system, a gelatin sponge scaffold modified with neurotrophin-3 (NT-3)/SF can achieve a controlled-artificial release system with significant inflammatory inhibitory activity in the rat spinal cord injury (SCI) model, as shown by a significant reduction in the number of IBA-1 positive and CD68 positive macrophages/microglia (Li et al., 2018; Li G. et al., 2016). SF NP drug delivery systems loaded with bromelain and ZnO NPs, EGCG and Tubasatin A is effective in reducing the massive infiltration and proliferation of inflammatory cells (Liu et al., 2022; Hasannasab et al., 2021; Xie et al., 2022; Liao et al., 2022) (Figure 5C).
In particular, it is worth noting that SFNPs have anti-inflammatory properties, which can inhibit the infiltration and proliferation of inflammatory immune cells and can cooperate with anti-inflammatory drugs to exert anti-inflammatory effects. Therefore, SFNPs are a suitable choice for the preparation of anti-inflammatory composite biomaterials.
SF exhibits excellent biocompatibility, very low immunogenicity, great modification potential, and can regulate wound treatment process through the NF-κB signaling pathway, and thus, it has attracted much attention (Chouhan and Mandal, 2020). However, SF materials are brittle and have rapid enzymatic biodegradability, which limits their application in wound healing. Therefore, they are usually complemented with other polymers to optimize their effects for wound treatment (Shen et al., 2022). SF hydrogels, scaffolds, and nanofibrous membrane systems have been developed to promote wound treatment by loading anti-inflammatory drugs and regulating the release of inflammatory factors to repair immune homeostasis. The SF hydrogel system can reduce the expression of IL-1, IL-6, IL-8 and TNF-α, and can increase the expression of IL-10, TGF-β, and Arg-1 by loading resveratrol, novel glycyrrhiza, CNP-miR146a, and borosilicate (BS). Inhibition of inflammation in the wound microenvironment accelerates the transition from the inflammatory to proliferative phase, thereby accelerating diabetic wound treatment (Niemiec et al., 2020; Pang et al., 2021; Qian Y. et al., 2022; Zhu et al., 2022; Yang et al., 2024). In addition, SF composite biomaterials loaded with rhein and puerarin promote the inflammatory stage of the wound by reducing the levels of IL-6, Inos, COX-2 and TNF-α, and correspondingly increasing the levels of IL-10, thereby promoting wound treatment process (Liu et al., 2022; Yin et al., 2022).
IL-1β is a proinflammatory factor, which induces inflammation and hinders articular cartilage repair (Wojdasiewicz et al., 2014). Therefore, it is an effective therapeutic strategy to use SF composite biomaterials system to deliver anti-inflammatory drugs to inhibit IL-1β and create an anti-inflammatory microenvironment to promote the repair of articular cartilage. At present, ginsenoside Rb1/TGF-β1-loaded biodegradable SF-gelatin scaffolds, SF-chondroitin sulfate scaffolds and injectable SF hydrogels containing articular chondrocytes (ACs) and hypoxic preconditioned exosomes (H-Exos) (SF/ACs/H-Exos) have been developed, all of which can reduce the inflammatory response of chondrocytes induced by IL-1β and support cartilage regeneration (Shen et al., 2022; Zhou et al., 2017; Wu et al., 2020) (Figure 5D).
As a non-toxic drug carrier with good biocompatibility, immunogenicity, and low biodegradability, SF can effectively treat colitis by preparing a NP system to deliver anti-inflammatory drugs to the inflamed parts of the colon. It is reported that SFNPs system loaded with pluronic F127 (PF127) -modified resveratrol (RSV), EGCG, patchouli alcohol (PA), and curcumin (CUR) can downregulate proinflammatory cytokines such as IL-1β, IL-6, IL-12, and TNF-α, upregulate anti-inflammatory cytokines such as IL-10, thus effectively alleviating the inflammatory response (Liu et al., 2022; Du et al., 2022; Gou et al., 2019; Xie et al., 2022).
Stem cell transplantation and biological scaffold implantation are considered effective methods for nerve regeneration. Safety and biocompatibility are two key factors in material selection for nerve regeneration research. SF is an excellent carrier for cell and growth factor delivery, a natural material with good biocompatibility, good mechanical properties, and biodegradability, and is reportedly a favorable choice for the repair of SCI and traumatic brain injury (Xu et al., 2016; Jiang et al., 2020). The inflammatory response in the injured area of the nervous system will inhibit nerve regeneration; thus, SF scaffolds with anti-inflammatory effects have also been developed, mainly by reducing the proinflammatory cytokines IL-6 and TNF-α, and increasing the anti-inflammatory cytokines IL-10 to inhibit inflammation and promote nerve regeneration (Li G. et al., 2016; Jiang J. et al., 2021) (Figure 5E).
SF hydrogel significantly improves skin penetration and the anti-keratinization ability of curcumin-loaded NPs (CUR-NPs), and can prolong the release of curcumin-loaded NPs; thus, inhibition of inflammatory cytokines (TNF-αand IL-6) is achieved to a greater extent with improvements in the therapeutic efficacy of curcumin on psoriasis mouse model (Mao et al., 2017). In addition, neutrophil membrane-coated SF-NPs can enhance the bioavailability and solubility of ferulic acid (FA), improve its pharmacological characteristics and targeted delivery, thereby significantly reducing proinflammatory cytokines IL-6, IL-1β and TNF-α (Hassanzadeh et al., 2021). Similarly, liquid SF along with the RES-SFN treatment achieved better results than each of these treatments used separately, and showed a more significant reduction in the proinflammatory cytokines IL-1β and TGF-β (Giménez-Siurana et al., 2020).
SF and sericin have been widely used in wound treatment, tissue engineering, and other fields in the form of hydrogels, scaffolds, films, and nanoparticles in recent decades. These different processing methods enable them to play different roles in the wound treatment process (proinflammatory and antibacterial stage or anti-inflammatory and healing promoting stage).
Our review focused on the anti-inflammatory and immunoregulatory effects of SF and sericin as biomaterials, particularly in the field of wound treatment. SF and sericin have been shown to be safe, biocompatible, and to exhibit low immunogenicity, and can elicit appropriate and acceptable immune responses in vivo, including innate and adaptive immune responses, when used alone or prepared in various forms of biomaterials. The anti-inflammatory and immune regulatory properties of sericin and SF make them widely used in skin wound treatment, UC, articular cartilage repair, and psoriasis. In addition to individual applications, they can also be combined with other materials to make composite materials using their modifiability, controllable biodegradability, and good mechanical properties, which can not only be used to improve the properties of single anti-inflammatory drugs, but also give full play to their own immune regulation and anti-inflammatory ability. These composites can improve existing immunotherapy methods, such as delivery of siRNA, enhancement of cellular immunity, and can be exploited as vaccine carriers to exert immune regulation. SF and sericin composites can also exert anti-inflammatory effects by promoting M2 polarization of macrophages, inhibiting the proliferation and infiltration of inflammatory cells, and regulating the release of inflammatory factors (Figure 6).
Figure 6. Immune response induced by (A) sericin/(B) SF-based biomaterials in vivo and their application.
There are still many challenges to be faced regarding the application of sericin-based and SF-based biomaterials in the field of medical biomedicine. For instance, due to the lack of standardized methods for assessing immune responses, it is difficult to make a comprehensive assessment of implant-induced immune responses (Kaprin et al., 2022). Additionally, it has also been well-known that epithelial cells and other types of immune cells (such as mast cells) equally play a significant role in the direct immune responses. However, there are few researches have explored their inflammatory response to sericin-based and SF-based biomaterials. Last but not least, there are some limitations of sericin-based and SF-based biomaterials in wound applications: (i) The extraction and purification process of SF and sericin is complicated, and other substances are easy to remain, which affects the purity and properties of the biomaterials; (ii) Compared to sericin, SF lacks antibacterial and antioxidant properties, so it is not effective in preventing wound infection.
In conclusion, SF and sericin exhibit good biocompatibility, low immunogenicity, controllable biodegradability, good mechanical properties, and excellent anti-inflammatory and immunomodulatory properties. A profound understanding of the different ways in which SF/sericin acts as a biomaterial and induces either proinflammatory or hypoinflammatory responses in the body will greatly improve utilization rate of silk biomaterials, especially in the field of wound treatment.
ZT: Writing–original draft, Writing–review and editing. HC: Writing–original draft. PZ: Conceptualization, Data curation, Writing–review and editing.
The author(s) declare that financial support was received for the research, authorship, and/or publication of this article. The National Natural Science Foundation of China (No. 32172798), The Natural Science Foundation of Chongqing (No. CSTB2024NSCQ-MSX0517) and Medical and Health Science and Technology Program of Zhejiang (No. 2025KY1192) provided support for this work.
We would like to thank Editage (www.editage.cn) for English language editing.
The authors declare that the research was conducted in the absence of any commercial or financial relationships that could be construed as a potential conflict of interest.
The author(s) declare that no Generative AI was used in the creation of this manuscript.
All claims expressed in this article are solely those of the authors and do not necessarily represent those of their affiliated organizations, or those of the publisher, the editors and the reviewers. Any product that may be evaluated in this article, or claim that may be made by its manufacturer, is not guaranteed or endorsed by the publisher.
CH, chitosan; SF, silk fibroin; SS1, sericin; HBsAg, hepatitis B surface antigen; IL-1/5/8/12/16/23, interleukin-1/5/8/12/16/23; TNF-α, tumor necrosis factor alpha; iNOS, inducible nitric oxide synthase; COX-2, Cyclooxygenase-2; LMW, low molecular weight; MMP, metalloproteinases; M1, classical activated macrophages; M2, Alternatively activated macrophages; BMP, morphogenetic protein; UC, ulcerative colitis; RA, rheumatoid arthritis; NF-κB, nuclear factor kappa-B; MAPK, mitogen-activated protein kinase; BM, Bombyx mori; PEG, polyethylene glycol; SCI, spinal cord injury.
Ampawong, S., and Aramwit, P. (2016). Tolerogenic responses of CD206+, CD83+, FOXP3+, and CTLA-4 to sericin/polyvinyl alcohol/glycerin scaffolds relevant to IL-33 and HSP60 activity. Histol. Histopathol. 31 (9), 1011–1027. doi:10.14670/hh-11-733
Antmen, E., Vrana, N. E., and Hasirci, V. (2021). The role of biomaterials and scaffolds in immune responses in regenerative medicine: macrophage phenotype modulation by biomaterial properties and scaffold architectures. Biomater. Sci. 9 (24), 8090–8110. doi:10.1039/d1bm00840d
Aramwit, P., Luplertlop, N., Kanjanapruthipong, T., and Ampawong, S. (2018). Effect of urea-extracted sericin on melanogenesis: potential applications in post-inflammatory hyperpigmentation. Biol. Res. 51 (1), 54. doi:10.1186/s40659-018-0204-5
Aramwit, P., Siritientong, T., and Srichana, T. (2012). Potential applications of silk sericin, a natural protein from textile industry by-products. Waste Manag. Res. 30 (3), 217–224. doi:10.1177/0734242x11404733
Aramwit, P., Towiwat, P., and Srichana, T. (2013). Anti-inflammatory potential of silk sericin. Nat. Prod. Commun. 8 (4), 501–504. doi:10.1177/1934578x1300800424
Arthe, R., Arivuoli, D., and Ravi, V. (2020). Preparation and characterization of bioactive silk fibroin/paramylon blend films for chronic wound healing. Int. J. Biol. Macromol. 154, 1324–1331. doi:10.1016/j.ijbiomac.2019.11.010
Ashraaf, S., Tahir, H. M., Raza, C., Awad, E. M., Ali, S., Khan, S. Y., et al. (2023). Synergistic effect of silk sericin and curcumin to treat an inflammatory condition. J. Burn Care Res. 44 (1), 106–113. doi:10.1093/jbcr/irac157
Bai, L., Zhang, X., Li, X., Wang, S., Zhang, Y., and Xu, G. (2023). Impact of a novel hydrogel with injectable platelet-rich fibrin in diabetic wound healing. J. Diabetes Res. 2023, 7532637. doi:10.1155/2023/7532637
Bessa-Gonçalves, M., Ribeiro-Machado, C., Costa, M., Ribeiro, C. C., Barbosa, J. N., Barbosa, M. A., et al. (2023). Magnesium incorporation in fibrinogen scaffolds promotes macrophage polarization towards M2 phenotype. Acta Biomater. 155, 667–683. doi:10.1016/j.actbio.2022.10.046
Bhattacharjee, M., Schultz-Thater, E., Trella, E., Miot, S., Das, S., Loparic, M., et al. (2013). The role of 3D structure and protein conformation on the innate and adaptive immune responses to silk-based biomaterials. Biomaterials 34 (33), 8161–8171. doi:10.1016/j.biomaterials.2013.07.018
Bhunia, B. K., Bandyopadhyay, A., Dey, S., and Mandal, B. B. (2023). Silk-hydrogel functionalized with human decellularized Wharton's jelly extracellular matrix as a minimally invasive injectable hydrogel system for potential nucleus pulposus tissue replacement therapy. Int. J. Biol. Macromol. 254, 127686. doi:10.1016/j.ijbiomac.2023.127686
Blakney, A. K., Swartzlander, M. D., and Bryant, S. J. (2012). The effects of substrate stiffness on the in vitro activation of macrophages and in vivo host response to poly(ethylene glycol)-based hydrogels. J. Biomed. Mater Res. A 100 (6), 1375–1386. doi:10.1002/jbm.a.34104
Boopathy, A. V., Mandal, A., Kulp, D. W., Menis, S., Bennett, N. R., Watkins, H. C., et al. (2019). Enhancing humoral immunity via sustained-release implantable microneedle patch vaccination. Proc. Natl. Acad. Sci. U. S. A. 116 (33), 16473–16478. doi:10.1073/pnas.1902179116
Byun, E. B., Sung, N. Y., Kim, J. H., Choi, J. I., Matsui, T., Byun, M. W., et al. (2010). Enhancement of anti-tumor activity of gamma-irradiated silk fibroin via immunomodulatory effects. Chem. Biol. Interact. 186 (1), 90–95. doi:10.1016/j.cbi.2010.03.032
Cai, F., Jiang, B., and He, F. (2024). Formation and biological activities of foreign body giant cells in response to biomaterials. Acta Biomater. 188, 1–26. doi:10.1016/j.actbio.2024.08.034
Cai, J., Liu, J., Xu, J., Li, Y., Zheng, T., Zhang, T., et al. (2023). Constructing high-strength nano-micro fibrous woven scaffolds with native-like anisotropic structure and immunoregulatory function for tendon repair and regeneration. Biofabrication 15, 025002. doi:10.1088/1758-5090/acb106
Cao, Y., Liu, S., Ma, Y., Ma, L., Zu, M., Sun, J., et al. (2022). Oral nanomotor-enabled mucus traverse and tumor penetration for targeted chemo-sono-immunotherapy against colon cancer. Small 18 (42), e2203466. doi:10.1002/smll.202203466
Chachlioutaki, K., Karavasili, C., Adamoudi, E., Bouropoulos, N., Tzetzis, D., Bakopoulou, A., et al. (2022). Silk sericin/PLGA electrospun scaffolds with anti-inflammatory drug-eluting properties for periodontal tissue engineering. Biomater. Adv. 133, 112723. doi:10.1016/j.msec.2022.112723
Chen, N., Jin, W., Gao, H., Hong, J., Sun, L., Yao, J., et al. (2023b). Sequential intervention of anti-inflammatory and osteogenesis with silk fibroin coated polyethylene terephthalate artificial ligaments for anterior cruciate ligament reconstruction. J. Mater Chem. B 11 (34), 8281–8290. doi:10.1039/d3tb00911d
Chen, Z., Wang, L., Guo, C., Qiu, M., Cheng, L., Chen, K., et al. (2023a). Vascularized polypeptide hydrogel modulates macrophage polarization for wound healing. Acta Biomater. 155, 218–234. doi:10.1016/j.actbio.2022.11.002
Cherng, J. H., Chang, S. J., Chiu, Y. K., Chiu, Y. H., Fang, T. J., and Chen, H. C. (2022). Low molecular weight sericin enhances the in vitro of immunological modulation and cell migration. Front. Bioeng. Biotechnol. 10, 925197. doi:10.3389/fbioe.2022.925197
Chlapanidas, T., Faragò, S., Lucconi, G., Perteghella, S., Galuzzi, M., Mantelli, M., et al. (2013). Sericins exhibit ROS-scavenging, anti-tyrosinase, anti-elastase, and in vitro immunomodulatory activities. Int. J. Biol. Macromol. 58, 47–56. doi:10.1016/j.ijbiomac.2013.03.054
Chlapanidas, T., Perteghella, S., Leoni, F., Faragò, S., Marazzi, M., Rossi, D., et al. (2014). TNF-α blocker effect of naringenin-loaded sericin microparticles that are potentially useful in the treatment of psoriasis. Int. J. Mol. Sci. 15 (8), 13624–13636. doi:10.3390/ijms150813624
Choi, J. H., Lee, S., Han, H. J., and Kwon, J. (2023). Antioxidation and anti-inflammatory effects of gamma-irradiated silk sericin and fibroin in H2O2-induced HaCaT Cell. Korean J. Physiol. Pharmacol. 27 (1), 105–112. doi:10.4196/kjpp.2023.27.1.105
Chouhan, D., Lohe, T. U., Samudrala, P. K., and Mandal, B. B. (2018). In situ forming injectable silk fibroin hydrogel promotes skin regeneration in full thickness burn wounds. Adv. Healthc. Mater 7 (24), e1801092. doi:10.1002/adhm.201801092
Chouhan, D., and Mandal, B. B. (2020). Silk biomaterials in wound healing and skin regeneration therapeutics: from bench to bedside. Acta Biomater. 103, 24–51. doi:10.1016/j.actbio.2019.11.050
Chu, W., Wang, P., Ma, Z., Peng, L., Guo, C., Fu, Y., et al. (2023). Lupeol-loaded chitosan-Ag(+) nanoparticle/sericin hydrogel accelerates wound healing and effectively inhibits bacterial infection. Int. J. Biol. Macromol. 243, 125310. doi:10.1016/j.ijbiomac.2023.125310
Cui, T., Yu, J., Li, Q., Wang, C. F., Chen, S., Li, W., et al. (2020). Large-scale fabrication of robust artificial skins from a biodegradable sealant-loaded nanofiber scaffold to skin tissue via microfluidic blow-spinning. Adv. Mater 32 (32), e2000982. doi:10.1002/adma.202000982
Deenonpoe, R., Prayong, P., Thippamom, N., Meephansan, J., and Na-Bangchang, K. (2019). Anti-inflammatory effect of naringin and sericin combination on human peripheral blood mononuclear cells (hPBMCs) from patient with psoriasis. BMC Complement. Altern. Med. 19 (1), 168. doi:10.1186/s12906-019-2535-3
Del Bianco, L., Spizzo, F., Yang, Y., Greco, G., Gatto, M. L., Barucca, G., et al. (2022). Silk fibroin films with embedded magnetic nanoparticles: evaluation of the magneto-mechanical stimulation effect on osteogenic differentiation of stem cells. Nanoscale 14 (39), 14558–14574. doi:10.1039/d2nr03167a
DeMuth, P. C., Min, Y., Irvine, D. J., and Hammond, P. T. (2014). Implantable silk composite microneedles for programmable vaccine release kinetics and enhanced immunogenicity in transcutaneous immunization. Adv. Healthc. Mater 3 (1), 47–58. doi:10.1002/adhm.201300139
Deng, K., Yang, D., and Zhou, Y. (2022b). Nanotechnology-based siRNA delivery systems to overcome tumor immune evasion in cancer immunotherapy. Pharmaceutics 14 (7), 1344. doi:10.3390/pharmaceutics14071344
Deng, Y., Yang, C., Zhu, Y., Liu, W., Li, H., Wang, L., et al. (2022a). Lamprey-teeth-Inspired oriented antibacterial sericin microneedles for infected wound healing improvement. Nano Lett. 22 (7), 2702–2711. doi:10.1021/acs.nanolett.1c04573
Dong, L., Li, L., Song, Y., Fang, Y., Liu, J., Chen, P., et al. (2021). MSC-derived immunomodulatory extracellular matrix functionalized electrospun fibers for mitigating foreign-body reaction and tendon adhesion. Acta Biomater. 133, 280–296. doi:10.1016/j.actbio.2021.04.035
Dong, X., Zhao, S. X., Yin, X. L., Wang, H. Y., Wei, Z. G., and Zhang, Y. Q. (2020). Silk sericin has significantly hypoglycaemic effect in type 2 diabetic mice via anti-oxidation and anti-inflammation. Int. J. Biol. Macromol. 150, 1061–1071. doi:10.1016/j.ijbiomac.2019.10.111
Du, Y., Shi, J., Duan, R., Tsim, K. W. K., Shen, L., Zhang, N., et al. (2022). cRGD peptide incorporated with patchouli alcohol loaded silk fibroin nanoparticles for enhanced targeting of inflammatory sites in colitis. Biomater. Adv. 140, 213069. doi:10.1016/j.bioadv.2022.213069
Ekdahl, K. N., Lambris, J. D., Elwing, H., Ricklin, D., Nilsson, P. H., Teramura, Y., et al. (2011). Innate immunity activation on biomaterial surfaces: a mechanistic model and coping strategies. Adv. Drug Deliv. Rev. 63 (12), 1042–1050. doi:10.1016/j.addr.2011.06.012
El-Samad, L. M., Hassan, M. A., Basha, A. A., El-Ashram, S., Radwan, E. H., Abdul Aziz, K. K., et al. (2022). Carboxymethyl cellulose/sericin-based hydrogels with intrinsic antibacterial, antioxidant, and anti-inflammatory properties promote re-epithelization of diabetic wounds in rats. Int. J. Pharm. 629, 122328. doi:10.1016/j.ijpharm.2022.122328
Eming, S. A., Wynn, T. A., and Martin, P. (2017). Inflammation and metabolism in tissue repair and regeneration. Science 356 (6342), 1026–1030. doi:10.1126/science.aam7928
Etienne, O., Schneider, A., Kluge, J. A., Bellemin-Laponnaz, C., Polidori, C., Leisk, G. G., et al. (2009). Soft tissue augmentation using silk gels: an in vitro and in vivo study. J. Periodontol. 80 (11), 1852–1858. doi:10.1902/jop.2009.090231
Fan, Z., Li, P. Y., Deng, J., Bady, S. C., and Cheng, H. (2018). Cell membrane coating for reducing nanoparticle-induced inflammatory responses to scaffold constructs. Nano Res. 11 (10), 5573–5583. doi:10.1007/s12274-018-2084-y
Farajdokht, F., Vatandoust, S. M., Hosseini, L., Fekri, K., Rahigh Aghsan, S., Majdi, A., et al. (2021). Sericin protects against acute sleep deprivation-induced memory impairment via enhancement of hippocampal synaptic protein levels and inhibition of oxidative stress and neuroinflammation in mice. Brain Res. Bull. 174, 203–211. doi:10.1016/j.brainresbull.2021.06.013
Fongsodsri, K., Tiyasatkulkovit, W., Chaisri, U., Reamtong, O., Adisakwattana, P., Supasai, S., et al. (2024). Sericin promotes chondrogenic proliferation and differentiation via glycolysis and Smad2/3 TGF-β signaling inductions and alleviates inflammation in three-dimensional models. Sci. Rep. 14 (1), 11553. doi:10.1038/s41598-024-62516-y
Fu, Z., Li, W., Wei, J., Yao, K., Wang, Y., Yang, P., et al. (2022). Construction and biocompatibility evaluation of fibroin/sericin-based scaffolds. ACS Biomater. Sci. Eng. 8 (4), 1494–1505. doi:10.1021/acsbiomaterials.1c01426
Garg, K., Ryan, J. J., and Bowlin, G. L. (2011). Modulation of mast cell adhesion, proliferation, and cytokine secretion on electrospun bioresorbable vascular grafts. J. Biomed. Mater Res. A 97 (4), 405–413. doi:10.1002/jbm.a.33073
Ge, Z., Yang, Q., Xiang, X., and Liu, K. Z. (2012). Assessment of silk fibroin for the repair of buccal mucosa in a rat model. Int. J. Oral Maxillofac. Surg. 41 (5), 673–680. doi:10.1016/j.ijom.2011.11.016
Ghensi, P., Bettio, E., Maniglio, D., Bonomi, E., Piccoli, F., Gross, S., et al. (2019). Dental implants with anti-biofilm properties: a pilot study for developing a new sericin-based coating. Mater. (Basel) 12 (15), 2429. doi:10.3390/ma12152429
Gholipourmalekabadi, M., Sapru, S., Samadikuchaksaraei, A., Reis, R. L., Kaplan, D. L., and Kundu, S. C. (2020). Silk fibroin for skin injury repair: where do things stand? Adv. Drug Deliv. Rev. 153, 28–53. doi:10.1016/j.addr.2019.09.003
Giménez-Siurana, A., Gómez García, F., Pagan Bernabeu, A., Lozano-Pérez, A. A., Aznar-Cervantes, S. D., Cenis, J. L., et al. (2020). Chemoprevention of experimental periodontitis in diabetic rats with silk fibroin nanoparticles loaded with resveratrol. Antioxidants (Basel) 9 (1), 85. doi:10.3390/antiox9010085
Gorenkova, N., Maitz, M. F., Böhme, G., Alhadrami, H. A., Jiffri, E. H., Totten, J. D., et al. (2021). The innate immune response of self-assembling silk fibroin hydrogels. Biomater. Sci. 9 (21), 7194–7204. doi:10.1039/d1bm00936b
Gou, S., Huang, Y., Wan, Y., Ma, Y., Zhou, X., Tong, X., et al. (2019). Multi-bioresponsive silk fibroin-based nanoparticles with on-demand cytoplasmic drug release capacity for CD44-targeted alleviation of ulcerative colitis. Biomaterials 212, 39–54. doi:10.1016/j.biomaterials.2019.05.012
Guan, L., Ge, H., Tang, X., Su, S., Tian, P., Xiao, N., et al. (2013). Use of a silk fibroin-chitosan scaffold to construct a tissue-engineered corneal stroma. Cells Tissues Organs 198 (3), 190–197. doi:10.1159/000355944
Guo, W., Li, Z., Huang, H., Xu, Z., Chen, Z., Shen, G., et al. (2022). VB12-Sericin-PBLG-IR780 nanomicelles for programming cell pyroptosis via photothermal (PTT)/Photodynamic (PDT) effect-induced mitochondrial DNA (mitoDNA) oxidative damage. ACS Appl. Mater Interfaces 14 (15), 17008–17021. doi:10.1021/acsami.1c22804
Guo, Y., Xie, B., Jiang, M., Yuan, L., Jiang, X., Li, S., et al. (2023). Facile and eco-friendly fabrication of biocompatible hydrogel containing CuS@Ser NPs with mechanical flexibility and photothermal antibacterial activity to promote infected wound healing. J. Nanobiotechnology 21 (1), 266. doi:10.1186/s12951-023-02035-6
Guziewicz, N., Best, A., Perez-Ramirez, B., and Kaplan, D. L. (2011). Lyophilized silk fibroin hydrogels for the sustained local delivery of therapeutic monoclonal antibodies. Biomaterials 32 (10), 2642–2650. doi:10.1016/j.biomaterials.2010.12.023
Hasannasab, M., Nourmohammadi, J., Dehghan, M. M., and Ghaee, A. (2021). Immobilization of bromelain and ZnO nanoparticles on silk fibroin nanofibers as an antibacterial and anti-inflammatory burn dressing. Int. J. Pharm. 610, 121227. doi:10.1016/j.ijpharm.2021.121227
Hasanzadeh, S., Farokhi, M., Habibi, M., Shokrgozar, M. A., Ahangari Cohan, R., Rezaei, F., et al. (2020). Silk fibroin nanoadjuvant as a promising vaccine carrier to deliver the FimH-IutA antigen for urinary tract infection. ACS Biomater. Sci. Eng. 6 (8), 4573–4582. doi:10.1021/acsbiomaterials.0c00736
Hassan, M. A., Basha, A. A., Eraky, M., Abbas, E., and El-Samad, L. M. (2024). Advancements in silk fibroin and silk sericin-based biomaterial applications for cancer therapy and wound dressing formulation: a comprehensive review. Int. J. Pharm. 662, 124494. doi:10.1016/j.ijpharm.2024.124494
Hassanzadeh, P., Arbabi, E., and Rostami, F. (2021). Coating of ferulic acid-loaded silk fibroin nanoparticles with neutrophil membranes: a promising strategy against the acute pancreatitis. Life Sci. 270, 119128. doi:10.1016/j.lfs.2021.119128
Hekmatshoar, Y., Rahbar Saadat, Y., Hosseiniyan Khatibi, S. M., Ozkan, T., Zununi Vahed, F., Nariman-Saleh-Fam, Z., et al. (2019). The impact of tumor and gut microbiotas on cancer therapy: beneficial or detrimental? Life Sci. 233, 116680. doi:10.1016/j.lfs.2019.116680
Hong, H., Wang, X., Song, X., Fawal, G. E., Wang, K., Jiang, D., et al. (2021). Yp, Zw and Hw: transdermal delivery of interleukin-12 gene targeting dendritic cells enhances the anti-tumour effect of programmed cell death protein 1 monoclonal antibody. Biomater. Transl. 2 (2), 151–164. doi:10.12336/biomatertransl.2021.02.005
Hossain, D. M., Pal, S. K., Moreira, D., Duttagupta, P., Zhang, Q., Won, H., et al. (2015). TLR9-Targeted STAT3 silencing abrogates immunosuppressive activity of myeloid-derived suppressor cells from prostate cancer patients. Clin. Cancer Res. 21 (16), 3771–3782. doi:10.1158/1078-0432.Ccr-14-3145
Hu, C., Liu, W., Xu, N., Huang, A., Zhang, Z., Fan, M., et al. (2020). Silk fibroin hydrogel as mucosal vaccine carrier: induction of gastric CD4+TRM cells mediated by inflammatory response induces optimal immune protection against Helicobacter felis. Emerg. Microbes Infect. 9 (1), 2289–2302. doi:10.1080/22221751.2020.1830719
Hu, D., Li, T., Liang, W., Wang, Y., Feng, M., and Sun, J. (2022). Silk sericin as building blocks of bioactive materials for advanced therapeutics. J. Control Release 353, 303–316. doi:10.1016/j.jconrel.2022.11.019
Hu, Z., Ma, C., Rong, X., Zou, S., and Liu, X. (2018). Immunomodulatory ECM-like microspheres for accelerated bone regeneration in diabetes mellitus. ACS Appl. Mater Interfaces 10 (3), 2377–2390. doi:10.1021/acsami.7b18458
Huang, W., Ling, S., Li, C., Omenetto, F. G., and Kaplan, D. L. (2018a). Silkworm silk-based materials and devices generated using bio-nanotechnology. Chem. Soc. Rev. 47 (17), 6486–6504. doi:10.1039/c8cs00187a
Huang, Y. J., Hung, K. C., Hsieh, F. Y., and Hsu, S. H. (2015). Carboxyl-functionalized polyurethane nanoparticles with immunosuppressive properties as a new type of anti-inflammatory platform. Nanoscale 7 (48), 20352–20364. doi:10.1039/c5nr06379e
Huang, Y. J., Hung, K. C., Hung, H. S., and Hsu, S. H. (2018b). Modulation of macrophage phenotype by biodegradable polyurethane nanoparticles: possible relation between macrophage polarization and immune response of nanoparticles. ACS Appl. Mater Interfaces 10 (23), 19436–19448. doi:10.1021/acsami.8b04718
Inoue, S., Tanaka, K., Arisaka, F., Kimura, S., Ohtomo, K., and Mizuno, S. (2000). Silk fibroin of Bombyx mori is secreted, assembling a high molecular mass elementary unit consisting of H-chain, L-chain, and P25, with a 6:6:1 molar ratio. J. Biol. Chem. 275 (51), 40517–40528. doi:10.1074/jbc.M006897200
Janani, G., Zhang, L., Badylak, S. F., and Mandal, B. B. (2022). Silk fibroin bioscaffold from Bombyx mori and Antheraea assamensis elicits a distinct host response and macrophage activation paradigm in vivo and in vitro. Biomater. Adv. 145, 213223. doi:10.1016/j.bioadv.2022.213223
Jańczuk, A., Brodziak, A., Czernecki, T., and Król, J. (2022). Lactoferrin-the health-promoting properties and contemporary application with genetic aspects. Foods 12 (1), 70. doi:10.3390/foods12010070
Jiang, J., Dai, C., Liu, X., Dai, L., Li, R., Ma, K., et al. (2021b). Implantation of regenerative complexes in traumatic brain injury canine models enhances the reconstruction of neural networks and motor function recovery. Theranostics 11 (2), 768–788. doi:10.7150/thno.50540
Jiang, J. P., Liu, X. Y., Zhao, F., Zhu, X., Li, X. Y., Niu, X. G., et al. (2020). Three-dimensional bioprinting collagen/silk fibroin scaffold combined with neural stem cells promotes nerve regeneration after spinal cord injury. Neural Regen. Res. 15 (5), 959–968. doi:10.4103/1673-5374.268974
Jiang, L.-B., Ding, S.-L., Ding, W., Su, D.-H., Zhang, F.-X., Zhang, T.-W., et al. (2021a). Injectable sericin based nanocomposite hydrogel for multi-modal imaging-guided immunomodulatory bone regeneration. Chem. Eng. J. 418, 129323. doi:10.1016/j.cej.2021.129323
Jiao, Z., Song, Y., Jin, Y., Zhang, C., Peng, D., Chen, Z., et al. (2017). In vivo characterizations of the immune properties of sericin: an ancient material with emerging value in biomedical applications. Macromol. Biosci. 17 (12). doi:10.1002/mabi.201700229
Jin, J., Yang, Y., Yang, J., Sun, Z., Wang, D., Qin, Y., et al. (2024). Macrophage metabolic reprogramming-based diabetic infected bone defect/bone reconstruction though multi-function silk hydrogel with exosome release. Int. J. Biol. Macromol. 278 (Pt 4), 134830. doi:10.1016/j.ijbiomac.2024.134830
Jo, Y. Y., Kweon, H., Kim, D. W., Baek, K., Chae, W. S., Kang, Y. J., et al. (2021). Silk sericin application increases bone morphogenic protein-2/4 expression via a toll-like receptor-mediated pathway. Int. J. Biol. Macromol. 190, 607–617. doi:10.1016/j.ijbiomac.2021.09.021
Johari, N., Khodaei, A., Samadikuchaksaraei, A., Reis, R. L., Kundu, S. C., and Moroni, L. (2022). Ancient fibrous biomaterials from silkworm protein fibroin and spider silk blends: biomechanical patterns. Acta Biomater. 153, 38–67. doi:10.1016/j.actbio.2022.09.030
Kambe, Y., and Yamaoka, T. (2021). Initial immune response to a FRET-based MMP sensor-immobilized silk fibroin hydrogel in vivo. Acta Biomater. 130, 199–210. doi:10.1016/j.actbio.2021.05.030
Kanazawa, T., Shizawa, Y., Takeuchi, M., Tamano, K., Ibaraki, H., Seta, Y., et al. (2015). Topical anti-nuclear factor-kappa B small interfering RNA with functional peptides containing sericin-based hydrogel for atopic dermatitis. Pharmaceutics 7 (3), 294–304. doi:10.3390/pharmaceutics7030294
Kanazawa, T., Tamano, K., Sogabe, K., Endo, T., Ibaraki, H., Takashima, Y., et al. (2017). Intra-articular retention and anti-arthritic effects in collagen-induced arthritis model mice by injectable small interfering RNA containing hydrogel. Biol. Pharm. Bull. 40 (11), 1929–1933. doi:10.1248/bpb.b17-00481
Kaprin, A. D., Shegay, P. V., Krasilnikova, M., Baranovskii, D. S., Klabukov, I. D., and Shestakova, V. A. (2022). Assessment of immunological responses - a novel challenge in tissue engineering and regenerative medicine. Biomed. Res. Ther. 9 (11), 5384–5386. doi:10.15419/bmrat.v9i11.776
Karande, P., and Mitragotri, S. (2010). Transcutaneous immunization: an overview of advantages, disease targets, vaccines, and delivery technologies. Annu. Rev. Chem. Biomol. Eng. 1, 175–201. doi:10.1146/annurev-chembioeng-073009-100948
Khampieng, T., Aramwit, P., and Supaphol, P. (2015). Silk sericin loaded alginate nanoparticles: preparation and anti-inflammatory efficacy. Int. J. Biol. Macromol. 80, 636–643. doi:10.1016/j.ijbiomac.2015.07.018
Kim, D. W., Eum, W. S., Jang, S. H., Park, J., Heo, D. H., Sheen, S. H., et al. (2011). A transparent artificial dura mater made of silk fibroin as an inhibitor of inflammation in craniotomized rats. J. Neurosurg. 114 (2), 485–490. doi:10.3171/2010.9.Jns091764
Kim, D. W., Hwang, H. S., Kim, D. S., Sheen, S. H., Heo, D. H., Hwang, G., et al. (2012). Enhancement of anti-inflammatory activity of PEP-1-FK506 binding protein by silk fibroin peptide. J. Microbiol. Biotechnol. 22 (4), 494–500. doi:10.4014/jmb.1111.11024
Kim, S. H., Hong, H., Ajiteru, O., Sultan, M. T., Lee, Y. J., Lee, J. S., et al. (2021). 3D bioprinted silk fibroin hydrogels for tissue engineering. Nat. Protoc. 16 (12), 5484–5532. doi:10.1038/s41596-021-00622-1
Koelink, P. J., Bloemendaal, F. M., Li, B., Westera, L., Vogels, E. W. M., van Roest, M., et al. (2020). Anti-TNF therapy in IBD exerts its therapeutic effect through macrophage IL-10 signalling. Gut 69 (6), 1053–1063. doi:10.1136/gutjnl-2019-318264
Kumar, J. P., Alam, S., Jain, A. K., Ansari, K. M., and Mandal, B. B. (2018a). Protective activity of silk sericin against UV radiation-induced skin damage by downregulating oxidative stress. ACS Appl. Bio Mater 1 (6), 2120–2132. doi:10.1021/acsabm.8b00558
Kumar, M., Coburn, J., Kaplan, D. L., and Mandal, B. B. (2016). Immuno-informed 3D silk biomaterials for tailoring biological responses. ACS Appl. Mater Interfaces 8 (43), 29310–29322. doi:10.1021/acsami.6b09937
Kumar, M., Gupta, P., Bhattacharjee, S., Nandi, S. K., and Mandal, B. B. (2018b). Immunomodulatory injectable silk hydrogels maintaining functional islets and promoting anti-inflammatory M2 macrophage polarization. Biomaterials 187, 1–17. doi:10.1016/j.biomaterials.2018.09.037
Lee, G., Ko, Y. G., Bae, K. H., Kurisawa, M., Kwon, O. K., and Kwon, O. H. (2022). Green tea catechin-grafted silk fibroin hydrogels with reactive oxygen species scavenging activity for wound healing applications. Biomater. Res. 26 (1), 62. doi:10.1186/s40824-022-00304-3
Lei, F., Cai, J., Lyu, R., Shen, H., Xu, Y., Wang, J., et al. (2022). Efficient tumor immunotherapy through a single injection of injectable antigen/adjuvant-loaded macroporous silk fibroin microspheres. ACS Appl. Mater Interfaces 14 (38), 42950–42962. doi:10.1021/acsami.2c11286
Li, D. F., Yang, M. F., Xu, H. M., Zhu, M. Z., Zhang, Y., Tian, C. M., et al. (2022). Nanoparticles for oral delivery: targeted therapy for inflammatory bowel disease. J. Mater Chem. B 10 (31), 5853–5872. doi:10.1039/d2tb01190e
Li, G., Che, M. T., Zeng, X., Qiu, X. C., Feng, B., Lai, B. Q., et al. (2018). Neurotrophin-3 released from implant of tissue-engineered fibroin scaffolds inhibits inflammation, enhances nerve fiber regeneration, and improves motor function in canine spinal cord injury. J. Biomed. Mater Res. A 106 (8), 2158–2170. doi:10.1002/jbm.a.36414
Li, G., Che, M. T., Zhang, K., Qin, L. N., Zhang, Y. T., Chen, R. Q., et al. (2016b). Graft of the NT-3 persistent delivery gelatin sponge scaffold promotes axon regeneration, attenuates inflammation, and induces cell migration in rat and canine with spinal cord injury. Biomaterials 83, 233–248. doi:10.1016/j.biomaterials.2015.11.059
Li, L., Qian, Y., Lin, C., Li, H., Jiang, C., Lv, Y., et al. (2015). The effect of silk gland sericin protein incorporation into electrospun polycaprolactone nanofibers on in vitro and in vivo characteristics. J. Mater Chem. B 3 (5), 859–870. doi:10.1039/c4tb00653d
Li, Q., Hu, W., Huang, Q., Yang, J., Li, B., Ma, K., et al. (2023). MiR146a-loaded engineered exosomes released from silk fibroin patch promote diabetic wound healing by targeting IRAK1. Signal Transduct. Target Ther. 8 (1), 62. doi:10.1038/s41392-022-01263-w
Li, S. Y., Liu, Y., Xu, C. F., Shen, S., Sun, R., Du, X. J., et al. (2016a). Restoring anti-tumor functions of T cells via nanoparticle-mediated immune checkpoint modulation. J. Control Release 231, 17–28. doi:10.1016/j.jconrel.2016.01.044
Li, W., and Man, X. Y. (2022). Immunotherapy in atopic dermatitis. Immunotherapy 14 (14), 1149–1164. doi:10.2217/imt-2022-0054
Li, X., Yang, W., Xie, H., Wang, J., Zhang, L., Wang, Z., et al. (2020). CNT/Sericin conductive nerve guidance conduit promotes functional recovery of transected peripheral nerve injury in a rat model. ACS Appl. Mater Interfaces 12 (33), 36860–36872. doi:10.1021/acsami.0c08457
Liao, S., Liu, Y., Kong, Y., Shi, H., Xu, B., Tang, B., et al. (2022). A bionic multichannel nanofiber conduit carrying Tubastatin A for repairing injured spinal cord. Mater Today Bio 17, 100454. doi:10.1016/j.mtbio.2022.100454
Liu, S., Cao, Y., Ma, L., Sun, J., Ramos-Mucci, L., Ma, Y., et al. (2022). Oral antimicrobial peptide-EGCG nanomedicines for synergistic treatment of ulcerative colitis. J. Control Release 347, 544–560. doi:10.1016/j.jconrel.2022.05.025
Luan, X. Y., Huo, G. H., Li, M. Z., Lu, S. Z., and Zhang, X. G. (2009). Antheraea pernyi silk fibroin maintains the immunosupressive properties of human bone marrow mesenchymal stem cells. Cell Biol. Int. 33 (11), 1127–1134. doi:10.1016/j.cellbi.2009.07.005
Ma, T., Wu, J., Chen, S., Bian, J., Gao, G., and Nong, L. (2024). pH-Responsive modified HAMA microspheres regulate the inflammatory microenvironment of intervertebral discs. ACS Appl. Mater Interfaces 16 (46), 63295–63305. doi:10.1021/acsami.4c14475
Ma, Y., Duan, L., Sun, J., Gou, S., Chen, F., Liang, Y., et al. (2022). Oral nanotherapeutics based on Antheraea pernyi silk fibroin for synergistic treatment of ulcerative colitis. Biomaterials 282, 121410. doi:10.1016/j.biomaterials.2022.121410
Ma, Y., Tong, X., Huang, Y., Zhou, X., Yang, C., Chen, J., et al. (2019). Oral administration of hydrogel-embedding silk sericin alleviates ulcerative colitis through wound healing, anti-inflammation, and anti-oxidation. ACS Biomater. Sci. Eng. 5 (11), 6231–6242. doi:10.1021/acsbiomaterials.9b00862
Maity, B., Alam, S., Samanta, S., Prakash, R. G., and Govindaraju, T. (2022). Antioxidant silk fibroin composite hydrogel for rapid healing of diabetic wound. Macromol. Biosci. 22 (9), e2200097. doi:10.1002/mabi.202200097
Majumder, N., Bhattacharjee, M., Spagnoli, G. C., and Ghosh, S. (2024). Immune response profiles induced by silk-based biomaterials: a journey from 'immunogenicity' towards 'immuno-compatibility. J. Of Mater. Chem. B 12 (38), 9508–9523. doi:10.1039/d4tb01231c
Mao, K. L., Fan, Z. L., Yuan, J. D., Chen, P. P., Yang, J. J., Xu, J., et al. (2017). Skin-penetrating polymeric nanoparticles incorporated in silk fibroin hydrogel for topical delivery of curcumin to improve its therapeutic effect on psoriasis mouse model. Colloids Surf. B Biointerfaces 160, 704–714. doi:10.1016/j.colsurfb.2017.10.029
Martin, K. E., and García, A. J. (2021). Macrophage phenotypes in tissue repair and the foreign body response: implications for biomaterial-based regenerative medicine strategies. Acta Biomater. 133, 4–16. doi:10.1016/j.actbio.2021.03.038
Matsumoto, A., Chen, J., Collette, A. L., Kim, U. J., Altman, G. H., Cebe, P., et al. (2006). Mechanisms of silk fibroin sol-gel transitions. J. Phys. Chem. B 110 (43), 21630–21638. doi:10.1021/jp056350v
Mei, J., Zhou, J., Kong, L., Dai, Y., Zhang, X., Song, W., et al. (2022). An injectable photo-cross-linking silk hydrogel system augments diabetic wound healing in orthopaedic surgery through spatiotemporal immunomodulation. J. Nanobiotechnology 20 (1), 232. doi:10.1186/s12951-022-01414-9
Ming, P., Li, B., Li, Q., Yuan, L., Jiang, X., Liu, Y., et al. (2025). Multifunctional sericin-based biomineralized nanoplatforms with immunomodulatory and angio/osteo-genic activity for accelerated bone regeneration in periodontitis. Biomaterials 314, 122885. doi:10.1016/j.biomaterials.2024.122885
Napavichayanun, S., Ampawong, S., Harnsilpong, T., Angspatt, A., and Aramwit, P. (2018). Inflammatory reaction, clinical efficacy, and safety of bacterial cellulose wound dressing containing silk sericin and polyhexamethylene biguanide for wound treatment. Arch. Dermatol Res. 310 (10), 795–805. doi:10.1007/s00403-018-1871-3
Nayak, D., Thathapudi, N. C., Ashe, S., and Nayak, B. (2021). Bioengineered ethosomes encapsulating AgNPs and Tasar silk sericin proteins for non melanoma skin carcinoma (NMSC) as an alternative therapeutics. Int. J. Pharm. 596, 120265. doi:10.1016/j.ijpharm.2021.120265
Ngamcherdtrakul, W., and Yantasee, W. (2019). siRNA therapeutics for breast cancer: recent efforts in targeting metastasis, drug resistance, and immune evasion. Transl. Res. 214, 105–120. doi:10.1016/j.trsl.2019.08.005
Niemiec, S. M., Louiselle, A. E., Hilton, S. A., Dewberry, L. C., Zhang, L., Azeltine, M., et al. (2020). Nanosilk increases the strength of diabetic skin and delivers CNP-miR146a to improve wound healing. Front. Immunol. 11, 590285. doi:10.3389/fimmu.2020.590285
Oliveira, I. M., Gonçalves, C., Shin, M. E., Lee, S., Reis, R. L., Khang, G., et al. (2020). Anti-inflammatory properties of injectable betamethasone-loaded tyramine-modified gellan gum/silk fibroin hydrogels. Biomolecules 10 (10), 1456. doi:10.3390/biom10101456
Pal, R. R., Parashar, P., Singh, I., and Saraf, S. A. (2019). Tamanu oil potentiated novel sericin emulgel of levocetirizine: repurposing for topical delivery against DNCB-induced atopic dermatitis, QbD based development and in vivo evaluation. J. Microencapsul. 36 (5), 432–446. doi:10.1080/02652048.2019.1637474
Pang, L., Tian, P., Cui, X., Wu, X., Zhao, X., Wang, H., et al. (2021). In situ photo-cross-linking hydrogel accelerates diabetic wound healing through restored hypoxia-inducible factor 1-alpha pathway and regulated inflammation. ACS Appl. Mater Interfaces 13 (25), 29363–29379. doi:10.1021/acsami.1c07103
Panilaitis, B., Altman, G. H., Chen, J., Jin, H. J., Karageorgiou, V., and Kaplan, D. L. (2003). Macrophage responses to silk. Biomaterials 24 (18), 3079–3085. doi:10.1016/s0142-9612(03)00158-3
Patel, D. K., Dutta, S. D., Hexiu, J., Ganguly, K., and Lim, K. T. (2022). 3D-printable chitosan/silk fibroin/cellulose nanoparticle scaffolds for bone regeneration via M2 macrophage polarization. Carbohydr. Polym. 281, 119077. doi:10.1016/j.carbpol.2021.119077
Phan, V. H. G., Murugesan, M., Nguyen, P. P. T., Luu, C. H., Le, N. H., Nguyen, H. T., et al. (2022). Biomimetic injectable hydrogel based on silk fibroin/hyaluronic acid embedded with methylprednisolone for cartilage regeneration. Colloids Surf. B Biointerfaces 219, 112859. doi:10.1016/j.colsurfb.2022.112859
Qi, C., Liu, J., Jin, Y., Xu, L., Wang, G., Wang, Z., et al. (2018). Photo-crosslinkable, injectable sericin hydrogel as 3D biomimetic extracellular matrix for minimally invasive repairing cartilage. Biomaterials 163, 89–104. doi:10.1016/j.biomaterials.2018.02.016
Qian, H., Zhou, T., Fu, Y., Guo, M., Yang, W., Zhang, D., et al. (2022a). Self-assembled tetrahedral framework nucleic acid mediates tumor-associated macrophage reprogramming and restores antitumor immunity. Mol. Ther. Nucleic Acids 27, 763–773. doi:10.1016/j.omtn.2021.12.036
Qian, Y., Zheng, Y., Jin, J., Wu, X., Xu, K., Dai, M., et al. (2022b). Immunoregulation in diabetic wound repair with a photoenhanced glycyrrhizic acid hydrogel scaffold. Adv. Mater 34 (29), e2200521. doi:10.1002/adma.202200521
Reeves, A. R., Spiller, K. L., Freytes, D. O., Vunjak-Novakovic, G., and Kaplan, D. L. (2015). Controlled release of cytokines using silk-biomaterials for macrophage polarization. Biomaterials 73, 272–283. doi:10.1016/j.biomaterials.2015.09.027
Rezaei, F., Keshvari, H., Shokrgozar, M. A., Doroud, D., Gholami, E., Khabiri, A., et al. (2021). Nano-adjuvant based on silk fibroin for the delivery of recombinant hepatitis B surface antigen. Biomater. Sci. 9 (7), 2679–2695. doi:10.1039/d0bm01518k
Ritprajak, P., Sirithanakorn, C., Nguyen, T. N., Sereemaspun, A., and Aramwit, P. (2021). Biosynthetic sericin 1-like protein skews dendritic cells to tolerogenic-like phenotype. Biotechnol. Appl. Biochem. 68 (6), 1508–1517. doi:10.1002/bab.2072
Rodriguez-Nogales, A., Algieri, F., De Matteis, L., Lozano-Perez, A. A., Garrido-Mesa, J., Vezza, T., et al. (2016). Intestinal anti-inflammatory effects of RGD-functionalized silk fibroin nanoparticles in trinitrobenzenesulfonic acid-induced experimental colitis in rats. Int. J. Nanomedicine 11, 5945–5958. doi:10.2147/ijn.S116479
Rujimongkon, K., Ampawong, S., Reamtong, O., Buaban, T., and Aramwit, P. (2021). The therapeutic effects of Bombyx mori sericin on rat skin psoriasis through modulated epidermal immunity and attenuated cell proliferation. J. Tradit. Complement. Med. 11 (6), 587–597. doi:10.1016/j.jtcme.2021.06.007
Sapru, S., Das, S., Mandal, M., Ghosh, A. K., and Kundu, S. C. (2021). Sericin-chitosan-glycosaminoglycans hydrogels incorporated with growth factors for in vitro and in vivo skin repair. Carbohydr. Polym. 258, 117717. doi:10.1016/j.carbpol.2021.117717
Schenkel, J. M., Fraser, K. A., Beura, L. K., Pauken, K. E., Vezys, V., and Masopust, D. (2014). T cell memory. Resident memory CD8 T cells trigger protective innate and adaptive immune responses. Science 346 (6205), 98–101. doi:10.1126/science.1254536
Seyedaghamiri, F., Farajdokht, F., Vatandoust, S. M., Mahmoudi, J., Khabbaz, A., and Sadigh-Eteghad, S. (2021). Sericin modulates learning and memory behaviors by tuning of antioxidant, inflammatory, and apoptotic markers in the hippocampus of aged mice. Mol. Biol. Rep. 48 (2), 1371–1382. doi:10.1007/s11033-021-06195-2
Shah, A., Ali Buabeid, M., Arafa, E. A., Hussain, I., Li, L., and Murtaza, G. (2019). The wound healing and antibacterial potential of triple-component nanocomposite (chitosan-silver-sericin) films loaded with moxifloxacin. Int. J. Pharm. 564, 22–38. doi:10.1016/j.ijpharm.2019.04.046
Shen, K., Duan, A., Cheng, J., Yuan, T., Zhou, J., Song, H., et al. (2022). Exosomes derived from hypoxia preconditioned mesenchymal stem cells laden in a silk hydrogel promote cartilage regeneration via the miR-205-5p/PTEN/AKT pathway. Acta Biomater. 143, 173–188. doi:10.1016/j.actbio.2022.02.026
Shirangi, A., Mottaghitalab, F., Dinarvand, S., and Atyabi, F. (2022). Theranostic silk sericin/SPION nanoparticles for targeted delivery of ROR1 siRNA: synthesis, characterization, diagnosis and anticancer effect on triple-negative breast cancer. Int. J. Biol. Macromol. 221, 604–612. doi:10.1016/j.ijbiomac.2022.09.020
Silva, A. S., Costa, E. C., Reis, S., Spencer, C., Calhelha, R. C., Miguel, S. P., et al. (2022). Silk sericin: a promising sustainable biomaterial for biomedical and pharmaceutical applications. Polym. (Basel) 14 (22), 4931. doi:10.3390/polym14224931
Singh, Y. P., Moses, J. C., Bhunia, B. K., Nandi, S. K., and Mandal, B. B. (2018). Hierarchically structured seamless silk scaffolds for osteochondral interface tissue engineering. J. Mater Chem. B 6 (36), 5671–5688. doi:10.1039/c8tb01344f
Song, I. B., Han, H. J., and Kwon, J. (2020). Immune-enhancing effects of gamma-irradiated sericin. Food Sci. Biotechnol. 29 (7), 969–976. doi:10.1007/s10068-020-00734-6
Song, X., Jiang, Y., Zhang, W., Elfawal, G., Wang, K., Jiang, D., et al. (2022). Transcutaneous tumor vaccination combined with anti-programmed death-1 monoclonal antibody treatment produces a synergistic antitumor effect. Acta Biomater. 140, 247–260. doi:10.1016/j.actbio.2021.11.033
Song, Y., Zhang, C., Zhang, J., Sun, N., Huang, K., Li, H., et al. (2016). An injectable silk sericin hydrogel promotes cardiac functional recovery after ischemic myocardial infarction. Acta Biomater. 41, 210–223. doi:10.1016/j.actbio.2016.05.039
Stinson, J. A., Boopathy, A. V., Cieslewicz, B. M., Zhang, Y., Hartman, N. W., Miller, D. P., et al. (2021). Enhancing influenza vaccine immunogenicity and efficacy through infection mimicry using silk microneedles. Vaccine 39 (38), 5410–5421. doi:10.1016/j.vaccine.2021.07.064
Sun, T., Kwong, C. H. T., Gao, C., Wei, J., Yue, L., Zhang, J., et al. (2020). Amelioration of ulcerative colitis via inflammatory regulation by macrophage-biomimetic nanomedicine. Theranostics 10 (22), 10106–10119. doi:10.7150/thno.48448
Sun, Y., Shi, W., Zhang, Q., Guo, H., Dong, Z., Zhao, P., et al. (2022). Multi-omics integration to reveal the mechanism of sericin inhibiting LPS-induced inflammation. Int. J. Mol. Sci. 24 (1), 259. doi:10.3390/ijms24010259
Suzuki, S., Shadforth, A. M. A., McLenachan, S., Zhang, D., Chen, S. C., Walshe, J., et al. (2019). Optimization of silk fibroin membranes for retinal implantation. Mater Sci. Eng. C Mater Biol. Appl. 105, 110131. doi:10.1016/j.msec.2019.110131
Takahashi, Y., Gehoh, M., and Yuzuriha, K. (1999). Structure refinement and diffuse streak scattering of silk (Bombyx mori). Int. J. Biol. Macromol. 24 (2-3), 127–138. doi:10.1016/s0141-8130(98)00080-4
Tan, M., Chen, Y., Guo, Y., Yang, C., Liu, M., Guo, D., et al. (2020). A low-intensity focused ultrasound-assisted nanocomposite for advanced triple cancer therapy: local chemotherapy, therapeutic extracellular vesicles and combined immunotherapy. Biomater. Sci. 8 (23), 6703–6717. doi:10.1039/d0bm00804d
Tian, F., Zhang, S., Wang, M., Yan, Y., Cao, Y., Wang, Y., et al. (2024). Clinical grade fibroin sutures with bioactive gold clusters enhance surgical wound healing via inflammation modulation. ACS Appl. Mater Interfaces. doi:10.1021/acsami.4c10451
Tkach, M., Kowal, J., Zucchetti, A. E., Enserink, L., Jouve, M., Lankar, D., et al. (2017). Qualitative differences in T-cell activation by dendritic cell-derived extracellular vesicle subtypes. Embo J. 36 (20), 3012–3028. doi:10.15252/embj.201696003
Tuentam, K., Aramwit, P., Reamtong, O., Supasai, S., Chaisri, U., Fongsodsri, K., et al. (2022). Sericin-based poly(vinyl) alcohol relieves plaque and epidermal lesions in psoriasis; a chance for dressing development in a specific area. Int. J. Mol. Sci. 24 (1), 145. doi:10.3390/ijms24010145
Wang, C., Li, J., Han, X., Liu, S., Gao, X., Guo, C., et al. (2022b). Silk sericin stabilized proanthocyanidins for synergetic alleviation of ulcerative colitis. Int. J. Biol. Macromol. 220, 1021–1030. doi:10.1016/j.ijbiomac.2022.08.134
Wang, D., Chen, M. W., Wei, Y. J., Geng, W. B., Hu, Y., Luo, Z., et al. (2022a). Construction of wogonin nanoparticle-containing strontium-doped nanoporous structure on titanium surface to promote osteoporosis fracture repair. Adv. Healthc. Mater 11 (21), e2201405. doi:10.1002/adhm.202201405
Wang, J., Li, X., Song, Y., Su, Q., Xiaohalati, X., Yang, W., et al. (2021). Injectable silk sericin scaffolds with programmable shape-memory property and neuro-differentiation-promoting activity for individualized brain repair of severe ischemic stroke. Bioact. Mater 6 (7), 1988–1999. doi:10.1016/j.bioactmat.2020.12.017
Wang, R., He, X., Su, S., Bai, J., Liu, H., and Zhou, F. (2024). Multifunctional tannic acid-based nanocomposite methacrylated silk fibroin hydrogel with the ability to scavenge reactive oxygen species and reduce inflammation for bone regeneration. Int. J. Biol. Macromol. 266 (Pt 2), 131357. doi:10.1016/j.ijbiomac.2024.131357
Wang, S., Zhang, Y., Shi, Y., He, Q., Tan, Q., Peng, Z., et al. (2023). Rhubarb charcoal-crosslinked chitosan/silk fibroin sponge scaffold with efficient hemostasis, inflammation, and angiogenesis for promoting diabetic wound healing. Int. J. Biol. Macromol. 253 (Pt 2), 126796. doi:10.1016/j.ijbiomac.2023.126796
Wang, Y., Rudym, D. D., Walsh, A., Abrahamsen, L., Kim, H. J., Kim, H. S., et al. (2008). In vivo degradation of three-dimensional silk fibroin scaffolds. Biomaterials 29 (24-25), 3415–3428. doi:10.1016/j.biomaterials.2008.05.002
Wang, Y., Yao, D., Li, L., Qian, Z., He, W., Ding, R., et al. (2020). Effect of electrospun silk fibroin-silk sericin films on macrophage polarization and vascularization. ACS Biomater. Sci. Eng. 6 (6), 3502–3512. doi:10.1021/acsbiomaterials.0c00175
Wani, S. U. D., Zargar, M. I., Masoodi, M. H., Alshehri, S., Alam, P., Ghoneim, M. M., et al. (2022). Silk fibroin as an efficient biomaterial for drug delivery, gene therapy, and wound healing. Int. J. Mol. Sci. 23 (22), 14421. doi:10.3390/ijms232214421
Wei, W., Liu, J., Peng, Z., Liang, M., Wang, Y., and Wang, X. (2020). Gellable silk fibroin-polyethylene sponge for hemostasis. Artif. Cells Nanomed Biotechnol. 48 (1), 28–36. doi:10.1080/21691401.2019.1699805
Wenhao, Z., Zhang, T., Yan, J., Li, Q., Xiong, P., Li, Y., et al. (2020). In vitro and in vivo evaluation of structurally-controlled silk fibroin coatings for orthopedic infection and in-situ osteogenesis. Acta Biomater. 116, 223–245. doi:10.1016/j.actbio.2020.08.040
Werlang, C. A., Sahoo, J. K., Cárcarmo-Oyarce, G., Stevens, C., Uzun, D., Putnik, R., et al. (2024). Selective biofilm inhibition through mucin-inspired engineering of silk glycopolymers. J. Am. Chem. Soc. 146 (50), 34661–34668. doi:10.1021/jacs.4c12945
Wojdasiewicz, P., Poniatowski, A., and Szukiewicz, D. (2014). The role of inflammatory and anti-inflammatory cytokines in the pathogenesis of osteoarthritis. Mediat. Inflamm. 2014, 561459. doi:10.1155/2014/561459
Wong, K., Tan, X. H., Li, J., Hui, J. H. P., and Goh, J. C. H. (2024). An in vitro macrophage response study of silk fibroin and silk fibroin/nano-hydroxyapatite scaffolds for tissue regeneration application. ACS Biomater. Sci. Eng. 10 (11), 7073–7085. doi:10.1021/acsbiomaterials.4c00976
Wu, T., Chen, Y., Liu, W., Tong, K. L., Suen, C. W., Huang, S., et al. (2020). Ginsenoside Rb1/TGF-β1 loaded biodegradable silk fibroin-gelatin porous scaffolds for inflammation inhibition and cartilage regeneration. Mater Sci. Eng. C Mater Biol. Appl. 111, 110757. doi:10.1016/j.msec.2020.110757
Wu, X., Guo, H., Jia, Y., Wang, Q., Wang, J., Sun, X., et al. (2022). Adipose mesenchymal stem cell-based tissue engineering mesh with sustained bFGF release to enhance tissue repair. Biomater. Sci. 10 (12), 3110–3121. doi:10.1039/d1bm01893k
Wu, X., Zhou, M., Jiang, F., Yin, S., Lin, S., Yang, G., et al. (2021). Marginal sealing around integral bilayer scaffolds for repairing osteochondral defects based on photocurable silk hydrogels. Bioact. Mater 6 (11), 3976–3986. doi:10.1016/j.bioactmat.2021.04.005
Xiang, G., Liu, K., Wang, T., Hu, X., Wang, J., Gao, Z., et al. (2021). In situ regulation of macrophage polarization to enhance osseointegration under diabetic conditions using injectable silk/sitagliptin gel scaffolds. Adv. Sci. (Weinh) 8 (3), 2002328. doi:10.1002/advs.202002328
Xie, D., Zhou, X., Xiao, B., Duan, L., and Zhu, Z. (2022). Mucus-Penetrating silk fibroin-based nanotherapeutics for efficient treatment of ulcerative colitis. Biomolecules 12 (9), 1263. doi:10.3390/biom12091263
Xu, N., Ruan, G., Liu, W., Hu, C., Huang, A., Zeng, Z., et al. (2019). Vaccine-induced gastric CD4(+) tissue-resident memory T cells proliferate in situ to amplify immune response against Helicobacter pylori insult. Helicobacter 24 (5), e12652. doi:10.1111/hel.12652
Xu, S., Tan, H., Yang, Q., Wang, R., Tian, C., Ji, Y., et al. (2021). Fabrication of a silk sericin hydrogel system delivering human lactoferrin using genetically engineered silk with improved bioavailability to alleviate chemotherapy-induced immunosuppression. ACS Appl. Mater Interfaces 13 (38), 45175–45190. doi:10.1021/acsami.1c08409
Xu, S., Yang, Q., Wang, R., Tian, C., Ji, Y., Tan, H., et al. (2022). Genetically engineered pH-responsive silk sericin nanospheres with efficient therapeutic effect on ulcerative colitis. Acta Biomater. 144, 81–95. doi:10.1016/j.actbio.2022.03.012
Xu, Y., Zhang, Z., Chen, X., Li, R., Li, D., and Feng, S. (2016). A silk fibroin/collagen nerve scaffold seeded with a Co-culture of schwann cells and adipose-derived stem cells for sciatic nerve regeneration. PLoS One 11 (1), e0147184. doi:10.1371/journal.pone.0147184
Yalcin, E., Kara, G., Celik, E., Pinarli, F. A., Saylam, G., Sucularli, C., et al. (2019). Preparation and characterization of novel albumin-sericin nanoparticles as siRNA delivery vehicle for laryngeal cancer treatment. Prep. Biochem. Biotechnol. 49 (7), 659–670. doi:10.1080/10826068.2019.1599395
Yang, H., Xu, H., Lv, D., Li, S., Rong, Y., Wang, Z., et al. (2024). The naringin/carboxymethyl chitosan/sodium hyaluronate/silk fibroin scaffold facilitates the healing of diabetic wounds by restoring the ROS-related dysfunction of vascularization and macrophage polarization. Int. J. Biol. Macromol. 260 (Pt 1), 129348. doi:10.1016/j.ijbiomac.2024.129348
Yang, N., Moore, M. J., Michael, P. L., Santos, M., Lam, Y. T., Bao, S., et al. (2021). Silk fibroin scaffold architecture regulates inflammatory responses and engraftment of bone marrow-mononuclear cells. Adv. Healthc. Mater 10 (16), e2100615. doi:10.1002/adhm.202100615
Yang, X., Wang, X., Hong, H., Elfawal, G., Lin, S., Wu, J., et al. (2020). Galactosylated chitosan-modified ethosomes combined with silk fibroin nanofibers is useful in transcutaneous immunization. J. Control Release 327, 88–99. doi:10.1016/j.jconrel.2020.07.047
Yao, S., Xie, Y., Xiao, L., Cai, L., and Ma, Z. (2019). Porous and nonporous silk fibroin (SF) membranes wrapping for Achilles tendon (AT) repair: which one is a better choice? J. Biomed. Mater Res. B Appl. Biomater. 107 (3), 733–740. doi:10.1002/jbm.b.34167
Yin, C., Han, X., Lu, Q., Qi, X., Guo, C., and Wu, X. (2022). Rhein incorporated silk fibroin hydrogels with antibacterial and anti-inflammatory efficacy to promote healing of bacteria-infected burn wounds. Int. J. Biol. Macromol. 201, 14–19. doi:10.1016/j.ijbiomac.2021.12.156
Yu, H., He, M., Li, Y., Liu, Y., Xu, Z., Zhang, L., et al. (2023). A robust Au@Cu(2-x)S nanoreactor assembled by silk fibroin for enhanced intratumoral glucose depletion and redox dyshomeostasis. Biomaterials 293, 121970. doi:10.1016/j.biomaterials.2022.121970
Yu, H., Li, Y., Zhang, Z., Ren, J., Zhang, L., Xu, Z., et al. (2022). Silk fibroin-capped metal-organic framework for tumor-specific redox dyshomeostasis treatment synergized by deoxygenation-driven chemotherapy. Acta Biomater. 138, 545–560. doi:10.1016/j.actbio.2021.11.009
Zhang, B., Wang, W., Gao, P., Li, X., Chen, L., Lin, Z., et al. (2024b). Injectable, electroconductive, free radical scavenging silk fibroin/black phosphorus/glycyrrhizic acid nanocomposite hydrogel for enhancing spinal cord repair. Adv. Healthc. Mater 13 (18), e2304300. doi:10.1002/adhm.202304300
Zhang, D., Chen, Q., Bi, Y., Zhang, H., Chen, M., Wan, J., et al. (2021b). Bio-inspired poly-DL-serine materials resist the foreign-body response. Nat. Commun. 12 (1), 5327. doi:10.1038/s41467-021-25581-9
Zhang, D., Chen, Q., Zhang, W., Liu, H., Wan, J., Qian, Y., et al. (2020). Silk-Inspired β-peptide materials resist fouling and the foreign-body response. Angew. Chem. Int. Ed. Engl. 59 (24), 9586–9593. doi:10.1002/anie.202000416
Zhang, D., Zhao, L., Cui, X., Li, X., Qian, Z., Zhou, X., et al. (2024a). Silkworm cocoon bionic design in wound dressings: a novel hydrogel with self-healing and antimicrobial properties. Int. J. Biol. Macromol. 280 (Pt 4), 136114. doi:10.1016/j.ijbiomac.2024.136114
Zhang, F., Yin, C., Qi, X., Guo, C., and Wu, X. (2022b). Silk fibroin crosslinked glycyrrhizic acid and silver hydrogels for accelerated bacteria-infected wound healing. Macromol. Biosci. 22 (4), e2100407. doi:10.1002/mabi.202100407
Zhang, H., Lai, L., Wang, Y., Ye, B., Deng, S., Ding, A., et al. (2019). Silk fibroin for CpG oligodeoxynucleotide delivery. ACS Biomater. Sci. Eng. 5 (11), 6082–6088. doi:10.1021/acsbiomaterials.9b01413
Zhang, L., Yang, W., Tao, K., Song, Y., Xie, H., Wang, J., et al. (2017). Sustained local release of NGF from a chitosan-sericin composite scaffold for treating chronic nerve compression. ACS Appl. Mater Interfaces 9 (4), 3432–3444. doi:10.1021/acsami.6b14691
Zhang, X., He, Q., Sun, J., Gong, H., Cao, Y., Duan, L., et al. (2022a). Near-infrared-enpowered nanomotor-mediated targeted chemotherapy and mitochondrial phototherapy to boost systematic antitumor immunity. Adv. Healthc. Mater 11 (14), e2200255. doi:10.1002/adhm.202200255
Zhang, Y., Shi, N., He, L., Wang, S., Li, X., Lu, S., et al. (2021a). Silk sericin activates mild immune response and increases antibody production. J. Biomed. Nanotechnol. 17 (12), 2433–2443. doi:10.1166/jbn.2021.3206
Zhang, Y. Q. (2002). Applications of natural silk protein sericin in biomaterials. Biotechnol. Adv. 20 (2), 91–100. doi:10.1016/s0734-9750(02)00003-4
Zhang, Y. Q., Ma, Y., Xia, Y. Y., Shen, W. D., Mao, J. P., and Xue, R. Y. (2006). Silk sericin-insulin bioconjugates: synthesis, characterization and biological activity. J. Control Release 115 (3), 307–315. doi:10.1016/j.jconrel.2006.08.019
Zhaorigetu, S., Yanaka, N., Sasaki, M., Watanabe, H., and Kato, N. (2003). Silk protein, sericin, suppresses DMBA-TPA-induced mouse skin tumorigenesis by reducing oxidative stress, inflammatory responses and endogenous tumor promoter TNF-alpha. Oncol. Rep. 10 (3), 537–543. doi:10.3892/or.10.3.537
Zhou, F., Zhang, X., Cai, D., Li, J., Mu, Q., Zhang, W., et al. (2017). Silk fibroin-chondroitin sulfate scaffold with immuno-inhibition property for articular cartilage repair. Acta Biomater. 63, 64–75. doi:10.1016/j.actbio.2017.09.005
Zhou, X., Wang, Y., Ji, J., and Zhang, P. (2024). Materials strategies to overcome the foreign body response. Adv. Healthc. Mater 13 (18), e2304478. doi:10.1002/adhm.202304478
Zhu, W., Dong, Y., Xu, P., Pan, Q., Jia, K., Jin, P., et al. (2022). A composite hydrogel containing resveratrol-laden nanoparticles and platelet-derived extracellular vesicles promotes wound healing in diabetic mice. Acta Biomater. 154, 212–230. doi:10.1016/j.actbio.2022.10.038
Keywords: biopharmaceuticals, nanomedicine, sericin, silk fibroin (SF), wound treatment
Citation: Tian Z, Chen H and Zhao P (2025) Compliant immune response of silk-based biomaterials broadens application in wound treatment. Front. Pharmacol. 16:1548837. doi: 10.3389/fphar.2025.1548837
Received: 20 December 2024; Accepted: 23 January 2025;
Published: 12 February 2025.
Edited by:
Jinlong Ma, Shandong Second Medical University, ChinaReviewed by:
Jatuporn Ngoenkam, Naresuan University, ThailandCopyright © 2025 Tian, Chen and Zhao. This is an open-access article distributed under the terms of the Creative Commons Attribution License (CC BY). The use, distribution or reproduction in other forums is permitted, provided the original author(s) and the copyright owner(s) are credited and that the original publication in this journal is cited, in accordance with accepted academic practice. No use, distribution or reproduction is permitted which does not comply with these terms.
*Correspondence: Ping Zhao, emhhb3BAc3d1LmVkdS5jbg==
†These authors have contributed equally to this work
Disclaimer: All claims expressed in this article are solely those of the authors and do not necessarily represent those of their affiliated organizations, or those of the publisher, the editors and the reviewers. Any product that may be evaluated in this article or claim that may be made by its manufacturer is not guaranteed or endorsed by the publisher.
Research integrity at Frontiers
Learn more about the work of our research integrity team to safeguard the quality of each article we publish.