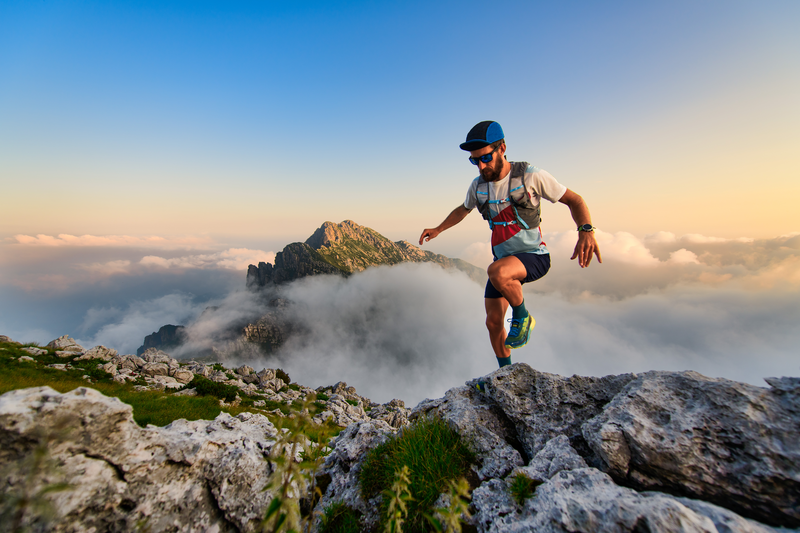
95% of researchers rate our articles as excellent or good
Learn more about the work of our research integrity team to safeguard the quality of each article we publish.
Find out more
PERSPECTIVE article
Front. Pharmacol. , 26 November 2024
Sec. Predictive Toxicology
Volume 15 - 2024 | https://doi.org/10.3389/fphar.2024.1496630
The clinical drug-drug interaction side-effects of Ritonavir, an ingredient in Paxlovid™, have been documented, highlighting the need to explore alternative administration methods for Nirmatrelvir, another drug in the Paxlovid™ combination. In this study, the skin permeability potential of Nirmatrelvir was assessed using various in silico models. The prediction results suggest that Nirmatrelvir could be administrated via the transdermal route, offering a promising avenue to enhance the efficacy of anti-COVID-19 agents.
The first infected case of severe acute respiratory syndrome coronavirus 2 (SARS-CoV-2) was reported and identified in the city of Wuhan, China in December 2019. This initial case marked the onset of a swift outbreak that rapidly spread, leading to the infection of hundreds of million people and claimed millions of lives worldwide. This catastrophic health crisis has become the most severe pandemic in history, as documented by the World Health Organization (WHO) (https://covid19.who.int/). The associated disease caused by SARS-CoV-2 was officially designated as coronavirus disease 2019 (COVID-19) by WHO. The oral administration of Paxlovid™, which is a combination of Nirmatrelvir (PF-07321332) and Ritonavir (NM/r) developed by Pfizer, in the early stages of COVID-19 can reduce the severity of the disease. In fact, Paxlovid has been approved as the first-line small-molecule agent for the treatment of COVID-19 in outpatients (NHS England, 2022), including individuals affected by the Omicron variants. Notably, it is particularly recommended for patients aged 65 years and older. A recent study has demonstrated that the antiviral efficacy of Paxlovid against the latest omicron variants, namely, BA.286 and JN.1 (source: https://data.who.int/dashboards/covid19/variants), remains unchanged (Planas et al., 2024).
Nirmatrelvir was initially derived for the treatment of SARS by inhibiting the 3-chymotrypsin-like cysteine protease enzyme (3CLpro or Mpro), which plays a pivotal role in the viral cycle. Additionally, it has demonstrated potent interaction with the main SARS-CoV-2 protease Mpro and effectively prevent the virus replication (Fenton and Keam, 2022). Ritonavir, on the other hand, was originally developed as an HIV protease inhibitor. It also acts as a cytochrome P450 3A4 (CYP3A4) inhibitor and affects drug efflux, such as P-glycoprotein (P-gp). However, it has been observed that Nirmatrelvir can be metabolized by CYP3A4, which can lead to serious clinical side-effects in immunocompromised patients.
Transplant patients, for instance, often takes cyclosporine-A (CsA) as the first-line immunosuppressive agent to prevent graft rejection. Unfortunately, CsA is also metabolized by CYP3A4, and the inhibitory effect of Ritonavir on CYP3A4 can significantly and rapidly increase the plasma concentrations of CsA, leading to potentially fatal or life-threatening consequences (Fishbane et al., 2022). As such, the CsA dosing regimens for Paxlovid-treated patients should be cautiously monitored (Schuurmans and Hage, 2021). Furthermore, polypharmacy is common among elderly individuals (Khezrian et al., 2020). The administration of Ritonavir can lead to unfavorable interactions with other drugs, known as adverse drug-drug interactions (DDIs) (Ross et al., 2022). Therefore, it is of critical importance for clinicians to consult reliable resources such as https://covid19-druginteractions.org/, to ensure the safe and effective use of these medications, especially in patients with complex medication regimens and potential drug interactions.
It has been estimated that 7%–8% of patients treated with Paxlovid have experienced Paxlovid rebound, which is characterized by the reemergence of COVID-19 symptoms or a positive viral test results after previously testing negative (Birabaharan and Martin, 2022). The observed rebound in cases among Paxlovid-treated patients, occurring at a higher rate compared to untreated counterparts (Cohen and Brown, 2023), is believed to stem from insufficient drug exposure rather than drug resistance or immune impairment against the virus (Carlin et al., 2022). Consequently, there has been a recommendation to extend the duration of the treatment regimen from the original 5 days to 7–10 days. In the event of Paxlovid rebound, it is suggested to administrate additional 3–5 days of treatment (Rao and Singh, 2022). Nevertheless, it is of importance to note that prolonging the duration of Paxlovid treatment may increase the risk of experiencing severe side-effects when used in combination with other medications. Careful consideration of these potential side-effects and individual patient circumstances is crucial when making treatment decisions.
Thus, it can be concluded that the combination of NM/r has indeed yielded various clinical challenges, leading to the strong urge to design novel small antiviral molecules that can be administrated through alternative routes other than oral administration (Fenton and Keam, 2022). By removing Ritonavir from Paxlovid, it is possible to substantially reduce or even eliminate those clinically observed side-effects associated with Paxlovid. Of various alternative administration routes, topical and transdermal drug delivery (TDD) seemingly appear to be attractive options due to their noninvasive nature and self-administration potential. In addition, TDD methods can extend the duration of drug release and target immunogenic Langerhans cells within the skin (Prausnitz and Langer, 2008). These routes offer a pharmacokinetically safer and potentially more effective alternative compared to the oral route (Wu et al., 2022). Nonetheless, it is of importance to note that transdermal drug delivery poses a challenge, as only a limited number of drugs can effectively cross the skin barrier (Yu et al., 2021).
Although various transdermal drug delivery (TDD) methods, namely, ethosomes, microemulsions, iontophoresis, and microneedles, have been developed to enhance transcutaneous permeation, most antiviral agents still struggle to penetrate the skin due to their physicochemical properties (Ita, 2017; Wang et al., 2021). Among these properties, molecular weight (MW) and log P are considered key factors and are widely used to predict the skin permeability of novel compounds (Chen et al., 2018). Specifically, MW should be less than 500 Da, and the log P-value should range between one and 3 (Finnin and Morgan, 1999). These parameters align with Nirmatrelvir’s properties (MW = 499.53; log p = 0.44). Moreover, several antiviral drugs have been successfully delivered via TDD, such as indinavir (MW = 613.79; log p = 2.81), zidovudine (MW = 267.24; log p = −0.3), and acyclovir (MW = 225.21; log p = −1.0), despite having only one of these favorable physicochemical properties (Ita, 2017) (The antiviral drug MW and log P values were retrieved from DrugBank, accessed on 18 October 2024). This suggests that Nirmatrelvir could be a feasible candidate for transdermal delivery.
The skin permeability of Nirmatrelvir was estimated by using various in silico approaches. This estimation was conducted using a previously published hierarchical support (HSVR), which is an innovative machine learning-based model (Wu et al., 2022), along with SwissADME, pkCSM, and Skin Permeation Calculator (SPC), which are available online (Table 1).
Table 1. Predicted log Kp values of Nirmatrelvir by SwissADME, pkCSM, SPC, and HSVR, converted log Pe values, skin permeability classification, and their references.
The predicted values of the logarithm of permeability coefficient or constant (Kp) are listed in Table 1. Although log Kp values have been widely used to quantitatively assess skin permeability, the cut-off value for categorizing skin permeability levels has not yet to be clearly defined. However, Carrer et al. have defined skin permeability using the effective permeability coefficient (Pe) classification as high, medium, and low if log Pe >
It is intriguing to observe that SPC failed to predict the log Kp value of Nirmatrelvir, whereas SwissADME, pkCSM, and HSVR displayed considerable variations in their predicted log Kp values. The failure of SPC can likely be attributed to its limited predictivity due to its narrower applicability domain, as evidenced by the fact that SPC could predict only ca. 80% of compounds in the previous study (Wu et al., 2022). All three predictors, nevertheless, unanimously identified Nirmatrelvir as highly skin permeable after converting log Kp into log Pe and applying skin permeability classification rules.
Thus, it can be concluded that the removal of Ritonavir from the combination of NM/r has the potential to reduce or even eliminate clinically adverse side-effects associated with the administration of Paxlovid. It is plausible to design the next-generation of anti-COVID-19 agent with minimal or no metabolism issues that, inevitably, can be time-consuming. Conversely, delivering Nirmatrelvir through topical or transdermal routes, such as a skin patch, can significantly enhance its clinical efficacy and pharmacokinetic safety while simultaneously addressing the issue of Paxlovid rebound. This approach offers a quick and cost-effective solution. However, further scientific research and clinical trials are required to support these conclusions and determine the effectiveness, safety, and feasibility of the proposed modifications. These steps are essential to ensure the successful development and implementation of such a strategy in the treatment of COVID-19.
The original contributions presented in the study are included in the article/supplementary material, further inquiries can be directed to the corresponding author.
GT: Data curation, Formal Analysis, Investigation, Methodology, Validation, Writing–original draft, Writing–review and editing. ML: Investigation, Methodology, Writing–review and editing, Conceptualization, Data curation, Formal Analysis, Funding acquisition, Project administration, Resources, Software, Supervision, Validation, Visualization, Writing–original draft.
The author(s) declare that financial support was received for the research, authorship, and/or publication of this article. The APC was funded by the National Dong Hwa University (Grant number: 113T2541-03).
Dedicated to Prof. Isaac B. Bersuker for his 96th birthday.
The authors declare that the research was conducted in the absence of any commercial or financial relationships that could be construed as a potential conflict of interest.
All claims expressed in this article are solely those of the authors and do not necessarily represent those of their affiliated organizations, or those of the publisher, the editors and the reviewers. Any product that may be evaluated in this article, or claim that may be made by its manufacturer, is not guaranteed or endorsed by the publisher.
Birabaharan, M., and Martin, T. C. S. (2022). Acute pulmonary emboli following rebound phenomenon after Nirmatrelvir/Ritonavir treatment for COVID-19. Am. J. Emerg. Med. 61, 235.e5–235.e6. doi:10.1016/j.ajem.2022.08.012
Carlin, A. F., Clark, A. E., Chaillon, A., Garretson, A. F., Bray, W., Porrachia, M., et al. (2022). Virologic and immunologic characterization of coronavirus disease 2019 recrudescence after nirmatrelvir/ritonavir treatment. Clin. Infect. Dis. 76, e530–e532. doi:10.1093/cid/ciac496
Chen, C. P., Chen, C. C., Huang, C. W., and Chang, Y. C. (2018). Evaluating molecular properties involved in transport of small molecules in stratum corneum: a quantitative structure-activity relationship for skin permeability. Molecules 23. doi:10.3390/molecules23040911
Carrer, V., Alonso, C., Pont, M., Zanuy, M., Córdoba, M., Espinosa, S., et al. (2020). Effect of propylene glycol on the skin penetration of drugs. Arch. Dermatol. Res. 312, 337–352. doi:10.1007/s00403-019-02017-5
Cohen, M. S., and Brown, E. R. (2023). Rebound of COVID-19 with nirmatrelvir–ritonavir antiviral therapy. Ann. Intern. Med. 176, 1672–1673. doi:10.7326/M23-2887
Fenton, C., and Keam, S. J. (2022). “Emerging small molecule antivirals may fit neatly into COVID-19 treatment,” in Drugs ther. Perspect.
Finnin, B. C., and Morgan, T. M. (1999). Transdermal penetration enhancers: applications, limitations, and potential. J. Pharm. Sci. 88, 955–958. doi:10.1021/js990154g
Fishbane, S., Hirsch, J. S., and Nair, V. (2022). Special considerations for Paxlovid treatment among transplant recipients with SARS-CoV-2 infection. Am. J. Kidney Dis. 79, 480–482. doi:10.1053/j.ajkd.2022.01.001
Ita, K. (2017). Transcutaneous permeation of antiviral agents. J. Drug Deliv. Sci. Technol. 41, 293–302. doi:10.1016/j.jddst.2017.08.002
Khezrian, M., McNeil, C. J., Murray, A. D., and Myint, P. K. (2020). An overview of prevalence, determinants and health outcomes of polypharmacy. Ther. Adv. Drug Saf. 11, 2042098620933741. doi:10.1177/2042098620933741
NHS England (2022). Interim clinical commissioning policy: antivirals or neutralising monoclonal antibodies for non-hospitalised patients with COVID-19 (version 6)
Planas, D., Staropoli, I., Michel, V., Lemoine, F., Donati, F., Prot, M., et al. (2024). Distinct evolution of SARS-CoV-2 Omicron XBB and BA.2.86/JN.1 lineages combining increased fitness and antibody evasion. Nat. Commun. 15, 2254. doi:10.1038/s41467-024-46490-7
Prausnitz, M. R., and Langer, R. (2008). Transdermal drug delivery. Nat. Biotechnol. 26, 1261–1268. doi:10.1038/nbt.1504
Rao, S., and Singh, M. (2022). Paxlovid rebound: proposed strategy to prevent reinfection following treatment of COVID-19 positive nonhospitalized patients. DHR Proceeding 2, 1–9. doi:10.47488/dhrp.v2iS3.67
Ross, S. B., Bortolussi-Courval, É., Hanula, R., Lee, T. C., Goodwin Wilson, M., and McDonald, E. G. (2022). Drug interactions with nirmatrelvir-ritonavir in older adults using multiple medications. JAMA Netw. Open 5, e2220184. e2220184-e2220184. doi:10.1001/jamanetworkopen.2022.20184
Schuurmans, M. M., and Hage, R. (2021). Cyclosporine A and COVID-19 – the COQUIMA cohort. EClinicalMedicine 31, 100680. doi:10.1016/j.eclinm.2020.100680
Wang, F. Y., Chen, Y., Huang, Y. Y., and Cheng, C. M. (2021). Transdermal drug delivery systems for fighting common viral infectious diseases. Drug Deliv. Transl. Res. 11, 1498–1508. doi:10.1007/s13346-021-01004-6
Wu, Y.-W., Ta, G. H., Lung, Y.-C., Weng, C.-F., and Leong, M. K. (2022). In silico prediction of skin permeability using a two-QSAR approach. Pharmaceutics 14, 961. doi:10.3390/pharmaceutics14050961
Keywords: in silico, skin permeability, Paxlovid, nirmatrelvir, COVID-19
Citation: Ta GH and Leong MK (2024) Mitigating Paxlovid™-induced drug‒drug interaction toxicity: an in silico insight. Front. Pharmacol. 15:1496630. doi: 10.3389/fphar.2024.1496630
Received: 15 September 2024; Accepted: 15 November 2024;
Published: 26 November 2024.
Edited by:
Eleonore Fröhlich, Medical University of Graz, AustriaReviewed by:
John Choy, The Catholic University of America, United StatesCopyright © 2024 Ta and Leong. This is an open-access article distributed under the terms of the Creative Commons Attribution License (CC BY). The use, distribution or reproduction in other forums is permitted, provided the original author(s) and the copyright owner(s) are credited and that the original publication in this journal is cited, in accordance with accepted academic practice. No use, distribution or reproduction is permitted which does not comply with these terms.
*Correspondence: Max K. Leong, bGVvbmdAZ21zLm5kaHUuZWR1LnR3
†ORCID: Max K. Leong, orcid.org/0000-0002-6927-1517
Disclaimer: All claims expressed in this article are solely those of the authors and do not necessarily represent those of their affiliated organizations, or those of the publisher, the editors and the reviewers. Any product that may be evaluated in this article or claim that may be made by its manufacturer is not guaranteed or endorsed by the publisher.
Research integrity at Frontiers
Learn more about the work of our research integrity team to safeguard the quality of each article we publish.