- 1Department of Orthopedics, Sports Medicine and Arthroscopy, Affiliated Fuyang People’s Hospital of Anhui Medical University, Fuyang, Anhui, China
- 2Shanghai Jiao Tong University School of Medicine, Shanghai, China
Diabetes is one of the most common metabolic diseases worldwide, leading to complications, mortality, and significant healthcare expenditures, which impose a substantial social and financial burden globally. A diabetic environment can induce metabolic changes, negatively affecting tendon homeostasis, leading to alterations in biomechanical properties and histopathology. Numerous studies have investigated the mechanisms through which diabetes exerts pathological effects on tendons, including increased free radical production, oxidative stress, inflammatory responses, deposition of advanced glycation end products (AGEs), and microvascular changes. These metabolic changes damages tendon structure, biomechanics, and tendon repair processes. The proliferation of tendon stem cells decreases, apoptosis increases, and abnormal differentiation, along with abnormal expression of myofibroblasts, ultimately lead to insufficient tendon repair, fibrosis, and remodeling. Although researches unveiling the effects of diabetes on tendinopathy, fibrosis or contracture, and tendon injury healing are growing, systematic understanding is still lacking. Therefore, this review summarizes the current research status and provides a comprehensive overview, offering theoretical guidance for future in-depth exploration of the impact of diabetes on tendons and the development of treatments for diabetes-related tendon diseases.
1 Introduction
Diabetes mellitus (DM) is a metabolic disorder characterized by hyperglycemia, primarily caused by insufficient insulin secretion (type 1 diabetes, T1DM) or insulin resistance (type 2 diabetes, T2DM) (DiMeglio et al., 2018; Chatterjee et al., 2017). The latter accounts for approximately 90% of all diabetes cases and is one of the most prevalent metabolic diseases worldwide (Chatterjee et al., 2017; Giha et al., 2022). By 2045, the prevalence is projected to rise to 12.2% of the population worldwide (Sun et al., 2022). The high prevalence of DM has significant social, economic, and developmental implications (Chatterjee et al., 2017; Vasiljević et al., 2022). Complications, mortality, and healthcare costs associated with DM impose a considerable social and financial burden (Cho et al., 2018; Nichols et al., 2019).
DM induces metabolic changes in microenvironment, such as increased free radical production, oxidative stress, abnormal expression of inflammatory factors (Vasiljević et al., 2022), copper metabolism abnormalities (Jia et al., 2024a), and the deposition of advanced glycation end products (AGEs) (Lee and Veres, 2019; Haus et al., 2007) These diabetes-related microenvironmental changes lead to numerous clinical complications, such microvascular diseases (Puri et al., 2022) and macrovascular diseases (Puri et al., 2022; Kato et al., 2024). In addition, musculoskeletal abnormalities, including tendon dysfunction, are also common complications of diabetes (Giha et al., 2022; Shalit et al., 2024).
Tendons connect muscles and bones, effectively transmitting muscle forces during musculoskeletal movements (Lu et al., 2020; Sharma and Maffulli, 2005; Singh et al., 2022). While there are extensive research on the impact of DM on musculoskeletal disorders, including arthritis (Wang et al., 2024a; Banu and Köseoğlu, 2023), osteoporosis (Li et al., 2024a), skeletal muscle atrophy (Atala et al., 2021; Cruz-Jentoft et al., 2019), and fibrosis (Singh et al., 2022; Wu et al., 2024), recent years witnesses an increasing number of investigations on the effects of DM on tendon homeostasis, providing knowledgeable foundation for further studies.
2 Impact of DM on normal tendons
The primary components of tendons are dense fibrous connective tissue and collagen, connecting muscles to bones and efficiently transmits forces during movements (Lu et al., 2020; Sharma and Maffulli, 2005). T2DM leads to impaired cellular glucose uptake and chronic hyperglycemia, exposing tissues to abnormally high glucose concentrations (Chatterjee et al., 2017). In both basic and clinical studies, the impact of T2DM on tendon homeostasis is generally overlooked, possibly due to a lack of recognition of the chronic pathological changes in tendon structure caused by T2DM (Nichols et al., 2019; Kim et al., 2022; Filgueiras et al., 2022).
DM alters muscle microcirculation and metabolic responses. In diabetic patients with a high risk of peripheral arterial or neurological disease, microcirculation deterioration is present in muscles and tendons, and tendon homeostasis may be affected by hyperglycemia (Kim et al., 2022; Kim et al., 2021; Panji Sananta et al., 2019), leading to structural changes and inflammation (Nopparat et al., 2023). Furthermore, T2DM is associated with increased oxidative stress (OS), which negatively affects tendon conditions (Vasiljević et al., 2022; Atala et al., 2021; Alabadi et al., 2023). Advanced glycation end products (AGEs) are compounds formed by aging and DM, which activate NADPH oxidase (NOX), increase reactive oxygen species (ROS) production and leads to OS (Kato et al., 2023; Shinohara et al., 2022a). AGEs also induces OS and triggers inflammatory responses (Shinohara et al., 2022a). The accumulation of AGEs, combined with other systemic and behavioral factors, further complicates tendon dysfunction (Singh et al., 2022; Zellers et al., 2021). AGEs, formed by non-enzymatic reactions, bind to membrane receptors to exacerbate inflammation and accelerate protein degradation (Puri et al., 2022; Cruz-Jentoft et al., 2019).
Structural changes in tendons of DM patients include collagen fiber disorder and micro-tears (Lo et al., 2013; Zaib et al., 2024; Chang et al., 2022). The metabolic changes in the microenvironment affect tendon stiffness, collagen composition, and physiology (Lee and Veres, 2019; Shi et al., 2021), which may be associated with AGEs (Fessel et al., 2014; Li et al., 2013). Research has shown that the crosslinking of AGEs in DM tendon inhibits the biomechanical plasticity and significantly disrupts tissue morphology (Lee and Veres, 2019; Indyk et al., 2021). The accumulated AGEs not only crosslinks adjacent collagen molecules to weaken biomechanics (Lee and Veres, 2019), but also induces inflammatory responses (Indyk et al., 2021). Moreover, pro-inflammatory chemokines, such as CCL-1, 2, 4, and 5, are highly expressed in the circulation of T2DM, further mediating inflammation (Mir et al., 2024).
Degenerative changes in tendons are common in DM patients (Abate et al., 2010). For example, histological studies confirm that hyperglycemia caused by DM is associated with degeneration of the rotator cuff or Achilles tendons (Kim et al., 2022; Kent and Bailey, 1985). Even asymptomatic DM patients may exhibit morphological abnormalities in the Achilles tendon (Afolabi et al., 2020), such as thickening, collagen disorder, or calcific changes at the tendon-bone junction (Harish et al., 2020; Vaidya et al., 2022; Xu et al., 2022). Specifically, Sneha et al. (Harish et al., 2020) evaluated the Achilles tendons of 61 healthy volunteers and 81 T2DM patients using ultrasound. Compared with healthy volunteers, the Achilles in T2DM patients was thickened and softened. DM can also lead to increased risk of Achilles tendon and plantar fascia contracture, impairing foot biomechanics and contributing to foot ulcers (Zellers et al., 2021; Harish et al., 2020; Ra and Hn, 2022). These changes reduce the extensibility of normal tendons and the strain energy of rupture (Lopez-Pedrosa et al., 2024; Su et al., 2024).
3 Impact of DM on tendinopathy
Tendinopathy is a common connective tissue disease, widely described as involving cellular proliferation, changes in extracellular matrix (ECM) turnover/synthesis, and inflammation associated with chronic tendon pathology (Sikes et al., 2021). The etiology is multifactorial and not yet fully understood (Giha et al., 2022; Xu et al., 2022). Tendinopathy is usually caused by overuse, metabolic disorders, and other metabolic factors related to micro-injuries in tendons. Tendinopathy is a challenging complication in diabetic patients (Shi et al., 2021; Cannata et al., 2020), often leading to chronic pain, restricted joint mobility, and even tendon rupture. DM, especially hyperglycemia, leads to elevated levels of acetylated p53, promoting cell apoptosis and OS, shifting the response of tenocytes from anabolic to pathogenic (Chang et al., 2022; Shinohara et al., 2022b), increasing the risk of developing tendinopathy (Panji Sananta et al., 2019; Harish et al., 2020). The potential pathogenic mechanisms by which DM leads to tendinopathy can generally be categorized into several aspects (Figure 1).
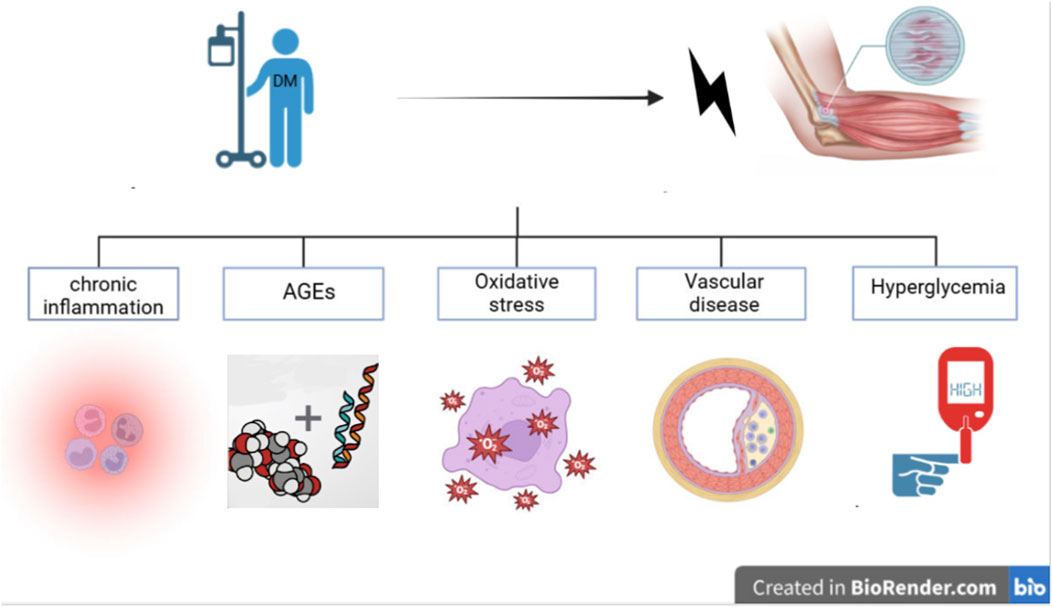
Figure 1. Schematic illustration of the potential mechanisms by whcich diabetes mellitus triggers tendinopathy.
Chronic Inflammation: It is well known that diabetic patients are in a pro-inflammatory state, and the hyperglycemic environment in diabetes may lead to chronic inflammation in tendons, eventually progressing to tendinopathy (Kwan et al., 2020). Diabetic patients typically exhibit elevated levels of pro-inflammatory cytokines, such as prostaglandins, tumor necrosis factor-α (TNF-α), interleukin-6 (IL-6), and leukotriene B4, which are significantly elevated in the serum of diabetic patients (Vasiljević et al., 2022; Zaib et al., 2024). These elevated levels of pro-inflammatory cytokines and chemokines may contribute to the chronic development of tendinopathy (Indyk et al., 2021; Xu et al., 2022; Kwan et al., 2020). Evidence indicates that the chronic inflammation observed in tendinopathy may be due to the reduced proteolytic response of tendon-derived stem cells (TDSCs) in tendinopathy, where the hyperglycemic environment may stimulate chronic inflammation and reduced proteolytic response, leading to tendinopathy (Kwan et al., 2020). Studies on the role of T2DM in rotator cuff tendinopathy suggest that persistent hyperglycemia may impair the proliferation and autophagy of tenocytes, further leading to increased expression of pro-inflammatory and pro-fibrotic mediators (Song et al., 2022).
Excessive Production of AGEs: AGEs can alter collagen within tendons, increase collagen crosslinking, reduce tendon fiber sliding and viscoelasticity, inhibit the biomechanical plasticity of natural tendons, and disrupt tendon morphology (Lee and Veres, 2019; Indyk et al., 2021). TDSCs are involved in tendinopathy, and AGEs can alter the pathophysiology of tendons in diabetic patients by regulating the proliferation and differentiation of TSPCs (Lu et al., 2020). However, other studies suggest that the relationship between AGE content and tendon tensile mechanics may be obscured by collagen disorder (Zellers et al., 2021).
OS: Diabetic patients may experience impaired angiogenesis, promoting tissue hypoxia and the production of ROS, leading to OS and pathological damage (Abu Khadra et al., 2024). In addition, DM patients have lower levels of catalase (CAT) activity, with an imbalance between oxidants and antioxidants, which increases OS to induce cell death and trigger tendinopathy (Lu et al., 2020; Abu Khadra et al., 2024; Yoon et al., 2024).
Vascular Changes: Vascular disease is one of the most common long-term complications of poorly DM, leading to functional and structural changes in the macrovascular and microvascular systems of tendons (Panji Sananta et al., 2019). These biochemical and structural abnormalities are also observed in various organs and tissues, including nephropathy, retinopathy, peripheral neuropathy, atherosclerosis, etc. (Kato et al., 2024; Tavares et al., 2021; Zheng et al., 2021). Diabetes-induced endothelial cell damage reduces the synthesis and secretion of protective factors, resulting in vasoconstriction and inflammation (Sharma and Maffulli, 2005).
Circulating AGEs are associated with vascular complications (Kato et al., 2024). Impaired vascular supply may also reduce the nutrients and oxygen supply to connective tissues, leading to degenerative changes and hindering tendon healing, thus promoting tendinopathy (Kato et al., 2024; Indyk et al., 2021; Abu Khadra et al., 2024). Some studies suggest that dysregulated glucose and lipid metabolism exacerbate the aging of TDSCs and promote osteogenic differentiation (Chen et al., 2024).
Calcific tendinopathy of the Achilles tendon is common, but most patients are asymptomatic. The incidence of Achilles tendon insertional calcific tendinopathy increases with age and is significantly higher in diabetic patients (Giai Via et al., 2022). Research shows that the risk of developing calcific tendinopathy of the shoulder increases by 27% at 8 years following DM diagnosis (Su et al., 2021). On the other hand, the etiology and pathogenesis of calcific tendinopathy remain unclear. Riley et al. (1994). proposed a theory suggesting that ischemic injury and rotator cuff degeneration associated with metabolic diseases lead to further calcification, indicating that metabolic diseases may be related to calcific tendinopathy. Chen et al. (2024) demonstrated that dysregulated glucose and lipid metabolism can activate the CXCL13-CXCR5 axis in aged TDSCs, thereby promoting ectopic ossification.
Hyperglycemia, inflammatory responses, AGEs, OS, and diabetic vascular changes can all influence tendon cell behavior. However, the extent to which these specific changes lead to diabetic tendinopathy and impaired healing remains unclear (Vaidya et al., 2022). Antidiabetic drugs may have beneficial effects on diabetic tendinopathy. Pioglitazone improves TDSC dysfunction caused by AGEs through autophagy promotion, and pioglitazone has been identified as a potential pharmacological option for tendinopathy (Xu et al., 2020). Research on metformin suggests that it may affect gene expression of myogenesis and adipogenesis, while whether metformin benefits tendinopathy remain unclear (Chang et al., 2022). Further efforts are required to develop effective therapeutics.
4 Impact of DM on tendon fibrosis
DM is associated with several fibrotic conditions, such as frozen shoulder, Dupuytren’s contracture, trigger finger, Achilles tendon contracture, and plantar fasciitis, which limit the range of motion of the affected joints, impairing function and the ability to perform daily activities (Abate et al., 2013; Al-Matubsi et al., 2011). Fibrosis is characterized by the accumulation of ECM, usually involving changes in ECM quality. The morphological and biochemical disruption of the ECM is directly related to the loss of target organ function (Primadhi and Herman, 2021; Ramirez et al., 2024). The excessive production of AGEs under hyperglycemic conditions can alter collagen within tendons, increase collagen crosslinking, reduce tendon fiber sliding and viscoelasticity, inhibit the biomechanical plasticity of natural tendons, and disrupt tendon morphology (Lee and Veres, 2019; Indyk et al., 2021; Gautieri and Silván, 2016). By stimulating transforming growth factor-beta (TGF-β) pathway, AGEs and ROS regulate the expression of various matrix proteins, forming fibrotic tissue (Primadhi and Herman, 2021; Li et al., 2024b; Noonin and Thongboonkerd, 2024). Myofibroblasts, the main producers and organizers of collagen/ECM during tissue healing, are also sensitive to DM related pathological changes, initiating hypertrophic scar formation and tissue fibrosis (Schuster et al., 2023). Given the aberrant fibrogenesis process, T2DM significantly impairs tendon healing by inducing scar formation (Zhao et al., 2017).
Tendon injuries can occur at the muscle-tendon junction (e.g., gastrocnemius, quadriceps), within the tendon itself (e.g., Achilles tendon), and at the tendon-bone interface (e.g., rotator cuff) (Sharma and Maffulli, 2005; Tavares et al., 2021; Takahashi et al., 2021; Yuan et al., 2024). Tendon healing occurs in three overlapping phases: the initial inflammatory phase, where erythrocytes and inflammatory cells, particularly neutrophils, infiltrate the injury site, with monocytes and macrophages predominating within the first 24 h, leading to the phagocytosis of necrotic material; a few days later, the proliferative phase begins and lasts for several weeks, during which the synthesis of type III collagen peaks; approximately 6 weeks later, the remodeling phase begins, characterized by a reduction in cell numbers, and decreased collagen and glycosaminoglycan synthesis. The remodeling phase can be divided into a consolidation phase, beginning around 6 weeks and lasting up to 10 weeks, and a maturation phase, starting 10 weeks after injury and continuing for up to a year, during which fibrous tissue gradually transforms into scar-like tendon tissue (Sharma and Maffulli, 2005; Farkas et al., 1973; Adawhhflf et al., 1983).
The increased risk of rotator cuff tears (RCTs) in diabetic patients may be related to impaired microcirculation (Yuan et al., 2024). Studies have shown that sodium-glucose cotransporter 2 inhibitors (SGLT2is) promote systemic anti-inflammatory effects by increasing fat utilization and regulating macrophage-mediated inflammatory pathways. SGLT2 inhibitors may prevent rotator cuff tears and subsequent repairs by reducing inflammation (Su et al., 2024). Diabetes leads to severe damage to the inflammatory, angiogenic, and proliferative processes, which may adversely affect tendon healing or remodeling after injury (Chbinou and Frenette, 2004).
Diabetic patients are at a higher risk of requiring tendon repair surgery (Cho et al., 2015), and diabetes can affect tendon healing post-operatively (Tavares et al., 2021; Takahashi et al., 2021; Griffith et al., 2022). Elevated hemoglobin A1c levels 3–6 months after rotator cuff repair surgery in diabetic patients are associated with an increased rate of re-tears (Kim et al., 2023). Nevertheless, for diabetic patients with perioperative glycemic control, the re-tear rate following rotator cuff repair is observed to be comparable to that of non-diabetic patients (Smith et al., 2021), underlying the importance of blood glucose control.
Tendon-bone healing is a challenging process in orthopedics and sports medicine (Wang et al., 2024b), while DM is a significant risk factor for poor tendon-to-bone healing. The hyperglycemic microenvironment inhibits TDSCs proliferation and inducing osteochondral differentiation, a potential mechanism by which diabetes impairs tendon-to-bone healing (Cao et al., 2022). Additionally, diabetes-induced hyperglycemia increases the expression of AGE and RAGE, resulting in significantly elevated mRNA expression levels of NOX1, NOX4, IL-6, RAGE, type III collagen, MMP2, TIMP1, and TIMP2 in the rotator cuff tendon, along with an increase in ROS-positive cells and apoptotic cells (Lee and Veres, 2019; Shinohara et al., 2022b; Yoshikawa et al., 2022). These inflammatory factors also induce a crosstalk between immune cells and tenocytes/TDSCs, while breaking this vicious cycle has therapeutic potential against this condition (Peng et al., 2024). Fibroblasts is closely correlated with collagen levels, and a hyperglycemic environment negatively impacts fibroblast quantity, adversely affecting tendon healing (Panji Sananta et al., 2019). AGEs-related increased expression of inflammatory factors can result in insufficient type I collagen synthesis of fibroblasts, delaying recovery process (Yoshikawa et al., 2022; Jia et al., 2024b).
5 Potential therapies under development
The ability to manage targets related to tendinopathy/tendon healing and strictly control diabetes may be effective in treating tendon pathology in diabetic patients (Yoon et al., 2024). However, the cellular and molecular components involved in various aspects of tendons disrupted by diabetes remain to be elucidated (Yoon et al., 2024). AGE inhibitors that prevent AGE formation could be a novel approach to treating diabetic tendon-to-bone healing (Jud and Sourij, 2019) (Menè and Pugliese, 2003). These therapeutic options include AGE crosslink breakers, AGE inhibitors, RAGE antagonists, clinically approved drugs for various indications (e.g., antidiabetic and antihypertensive drugs, or statins), and dietary and herbal treatments (Jud and Sourij, 2019). Direct AGE inhibitors include pyridoxamine and aminoguanidine, which reduce AGE/RAGE by increasing activation of the detoxifying enzyme Glo-1 and inhibiting ROS derived from NOX, as well as by inhibiting the formation of reactive dicarbonyl compounds (such as methylglyoxal) (Sourris et al., 2020). Hyperglycemic conditions increase intracellular ROS levels, a major cause of OS, which may interfere with the repair capacity of damaged or degenerated tendons under hyperglycemic conditions (Yoon et al., 2024; Osonoi et al., 2020). Inhibiting OS and improving mitochondrial function is another manner to facilitate tissue repair (Li et al., 2024c; Quetglas-Llabrés et al., 2024). Dietary polyphenols is noticed to mitigate OS and mitochondrial dysfunction in the crosstalk between type 2 diabetes and tendinopathy (Wang et al., 2024c). Polyphenols, such as pomegranate peel extract, have also shown beneficial effects on inflammatory states and OS biomarkers in T2DM (Vasiljević et al., 2022).
The decline in regenerative function of adipose-derived stem cells is partly mediated by the OS and inflammatory environment induced by diabetes. The induction of antioxidant stress factors in adipose-derived stem cells may represent an adaptive mechanism to cope with the increased OS in the diabetic microenvironment (Ahmed et al., 2024). After applying adipose tissue-derived stromal vascular fraction (SVF) in diabetic rats, the number of tenocytes, capillaries, and collagen increased, improving Achilles tendon rupture healing (Panji Sananta et al., 2019). 3D-printed biological scaffolds have the potential to improve rotator cuff healing by enhancing osteogenesis, reducing inflammation, and promoting macrophage polarization (Wang et al., 2024b). Some studies also suggest that antidiabetic drugs may have beneficial effects on tendon healing. For example, pioglitazone can prevent the harmful effects of AGEs on Achilles tendon healing, improving the biomechanical properties of the Achilles tendon (Jia et al., 2024b). Pioglitazone is a peroxisome proliferator-activated receptor-gamma (PPAR-γ) agonist widely used in clinical practice to treat T2DM. It can also reduce RAGE expression and block its downstream signaling pathways, thereby alleviating OS and inflammation in tissues (Xu et al., 2020; Yuan et al., 2011). Diabetes has adverse effects on the neurotrophic pathways in tendon regeneration. Therefore, new therapeutic strategies for regenerating tendons after injury in diabetic patients may include the modulation of neurotrophic pathway molecules, such as NGF and its receptors (Quaini et al., 2017).
6 Conclusion
In summary, DM alters the microcirculation and metabolic responses in tendons, leading to negative changes that affect the biomechanical properties and histopathology. Specifically, increased free radical production, OS, inflammatory responses, and the deposition of AGEs collectively damage tendon structure, biomechanics, and tendon fibrosis and repair. The decreased proliferation of tendon stem cells, increased apoptosis, and incorrect differentiation ultimately result in insufficient tendon repair, maintenance, and remodeling. Although current research has explored the impact of diabetes on tendons, tendinopathy, and tendon injury healing, detailed evidence on the underlying mechanisms remains to be revealed. Future researches are needed to delve deeper into the mechanisms DM-associated tendon pathology to provide references for developing treatment methods against this disorder.
Author contributions
JX: Writing–original draft, Writing–review and editing. JW: Writing–original draft, Writing–review and editing. YJ: Conceptualization, Writing–original draft. YL: Data curation, Investigation, Writing–original draft. JJ: Methodology, Writing–review and editing. YaW: Formal Analysis, Writing–original draft. XC: Methodology, Writing–review and editing. YuW: Funding acquisition, Writing–original draft. BG: Writing–review and editing. HY: Writing–original draft, Writing–review and editing.
Funding
The author(s) declare that financial support was received for the research, authorship, and/or publication of this article. This study was supported by the Research Project of Fuyang Science and Technology Bureau (FK202081024), Key Research and Development Plan Project of Fuyang Science and Technology Bureau (2024), and the Science Research Project of Bengbu Medical College (2022byzd172).
Conflict of interest
The authors declare that the research was conducted in the absence of any commercial or financial relationships that could be construed as a potential conflict of interest.
Publisher’s note
All claims expressed in this article are solely those of the authors and do not necessarily represent those of their affiliated organizations, or those of the publisher, the editors and the reviewers. Any product that may be evaluated in this article, or claim that may be made by its manufacturer, is not guaranteed or endorsed by the publisher.
References
Abate, M., Schiavone, C., and Salini, V. (2010). Sonographic evaluation of the shoulder in asymptomatic elderly subjects with diabetes. BMC Musculoskelet. Disord. 11 (1), 278. doi:10.1186/1471-2474-11-278
Abate, M., Schiavone, C., Salini, V., and Andia, I. (2013). Management of limited joint mobility in diabetic patients. Diabetes, Metabolic Syndrome Obes. Targets Ther. 6, 197–207. doi:10.2147/DMSO.S33943
Abu Khadra, K. M., Bataineh, M. I., Khalil, A., and Saleh, J. (2024). Oxidative stress and type 2 diabetes: the development and the pathogenesis, Jordanian cross-sectional study. Eur. J. Med. Res. 29 (1), 370. doi:10.1186/s40001-024-01906-4
Adawhhflf, C., Akeson, W. H., Harwood, F. L., and Frank, C. B. (1983). Stress deprivation effect on metabolic turnover of the medial collateral ligament collagen. A comparison between nine- and 12-week immobilization. Clin. Orthop. Relat. R. 172, 265–270. doi:10.1097/00003086-198301000-00042
Afolabi, B. I., Idowu, B. M., and Onigbinde, S. O. (2020). Achilles tendon degeneration on ultrasound in type 2 diabetic patients. J. Ultrasonogr. 20 (83), 291–299. doi:10.15557/JoU.2020.0051
Ahmed, S. M., Elkhenany, H. A., Ahmed, T. A., Ghoneim, N. I., Elkodous, M. A., Mohamed, R. H., et al. (2024). Diabetic microenvironment deteriorates the regenerative capacities of adipose mesenchymal stromal cells. Diabetology & Metabolic Syndrome 16 (1), 131. doi:10.1186/s13098-024-01365-1
Alabadi, B., Civera, M., De la Rosa, A., Martinez-Hervas, S., Gomez-Cabrera, M. C., and Real, J. T. (2023). Low muscle mass is associated with poorer glycemic control and higher oxidative stress in older patients with type 2 diabetes. Nutrients 15 (14), 3167. doi:10.3390/nu15143167
Al-Matubsi, H. Y., Hamdan, F., AlHanbali, O. A., Oriquat, G. A., and Salim, M. (2011). Diabetic hand syndromes as a clinical and diagnostic tool for diabetes mellitus patients. Diabetes Res. Clin. Pract. 94 (2), 225–229. doi:10.1016/j.diabres.2011.07.012
Atala, N. A., Bongiovanni, S. L., Galich, A. M., Bruchmann, M. G., Rossi, L. A., Tanoira, I., et al. (2021). Is sarcopenia a risk factor for rotator cuff tears? J. Shoulder Elb. Surg. 30 (8), 1851–1855. doi:10.1016/j.jse.2020.10.001
Banu, A. Z., and Köseoğlu, D. (2023). Ultrasonographic assessment of knee joint in patients with diabetes mellitus: comparison of sonographic changes in distal femoral cartilage and tendon structures with non-diabetics patients. Eur. Rev. Med. Pharm. 27 (15), 7180–7187. doi:10.26355/eurrev_202308_33291
Cannata, F., Vadalà, G., Ambrosio, L., Napoli, N., Papalia, R., Denaro, V., et al. (2020). The impact of type 2 diabetes on the development of tendinopathy. Diabetes/Metabolism Res. Rev. 37 (6), e3417. doi:10.1002/dmrr.3417
Cao, T., Hong, J., Qi, F., Zheng, B., Chen, G., Yu, B., et al. (2022). A hyperglycemic microenvironment inhibits tendon-to-bone healing through the let-7b-5p/CFTR pathway. Comput. Math. Methods Med. 2022, 8268067–8268110. doi:10.1155/2022/8268067
Chang, R., Tu, T.-Y., Hung, Y.-M., Huang, J.-Y., Chou, M.-C., and Wei, J. C.-C. (2022). Metformin use is associated with a lower risk of rotator cuff disease in patients with Type 2 diabetes mellitus. Diabetes & Metabolism 48 (5), 101368. doi:10.1016/j.diabet.2022.101368
Chatterjee, S., Khunti, K., and Davies, M. J. (2017). Type 2 diabetes. Lancet 389 (10085), 2239–2251. doi:10.1016/S0140-6736(17)30058-2
Chbinou, N., and Frenette, J. (2004). Insulin-dependent diabetes impairs the inflammatory response and delays angiogenesis following Achilles tendon injury. Am. J. Physiology-Regulatory, Integr. Comp. Physiology 286 (5), R952–R957. doi:10.1152/ajpregu.00536.2003
Chen, Y., Wu, J., Wong, C., Gao, W., Qi, X., and Zhou, H. (2024). Disturbed glycolipid metabolism activates CXCL13-CXCR5 axis in senescent TSCs to promote heterotopic ossification. Cell. Mol. Life Sci. 81 (1), 265. doi:10.1007/s00018-024-05302-3
Cho, N. H., Shaw, J. E., Karuranga, S., Huang, Y., da Rocha Fernandes, J. D., Ohlrogge, A. W., et al. (2018). IDF Diabetes Atlas: global estimates of diabetes prevalence for 2017 and projections for 2045. Diabetes Res. Clin. Pract. 138, 271–281. doi:10.1016/j.diabres.2018.02.023
Cho, N. S., Moon, S. C., Jeon, J. W., and Rhee, Y. G. (2015). The influence of diabetes mellitus on clinical and structural outcomes after arthroscopic rotator cuff repair. Am. J. Sports Med. 43 (4), 991–997. doi:10.1177/0363546514565097
Cruz-Jentoft, A. J., Bahat, G., Bauer, J., Boirie, Y., Bruyère, O., Cederholm, T., et al. (2019). Sarcopenia: revised European consensus on definition and diagnosis. Age Ageing 48 (1), 601–631. doi:10.1093/ageing/afz046
DiMeglio, L. A., Evans-Molina, C., and Oram, R. A. (2018). Type 1 diabetes. Lancet 391 (10138), 2449–2462. doi:10.1016/S0140-6736(18)31320-5
Farkas, L. G. M. W. G., Sweeney, P., Wilson, W., Hurst, L. N., and Lindsay, W. K. (1973). An experimental study of the changes following silastic rod preparation of a new tendon sheath and subsequent tendon grafting. J. BONE Jt. Surg. Am. 55 (6), 1149–1158. doi:10.2106/00004623-197355060-00002
Fessel, G. L., Yufei, D., Vincent, G., Sicairos, M., Schneider, P., Sell, D. R., et al. (2014). Advanced glycation end-products reduce collagen molecular sliding to affect collagen fibril damage mechanisms but not stiffness. PLoS One 9 (11), e110948. doi:10.1371/journal.pone.0110948
Filgueiras, J. R., Sales, C. P., da Silva, I. G., Dos Santos, C. M., Neto, E. C. M., da Rocha, R. B., et al. (2022). Morphological and functional changes in skeletal muscle in type 2 diabetes mellitus: a systematic review and meta-analysis. Physiother. Theory Pract. 39 (9), 1789–1815. doi:10.1080/09593985.2022.2057375
Gautieri, A. P. F., and Silván, U. (2016). Advanced glycation end-products: mechanics of aged collagen from molecule to tissue. MATRIX Biol. 59, 95–108. doi:10.1016/j.matbio.2016.09.001
Giai Via, A., Oliva, F., Padulo, J., Oliva, G., and Maffulli, N. (2022). Insertional calcific tendinopathy of the Achilles tendon and dysmetabolic diseases: an epidemiological survey. Clin. J. Sport Med. 32 (1), e68–e73. doi:10.1097/JSM.0000000000000881
Giha, H. A., Sater, M. S., and Alamin, O. A. O. (2022). Diabetes mellitus tendino-myopathy: epidemiology, clinical features, diagnosis and management of an overlooked diabetic complication. Acta Diabetol. 59 (7), 871–883. doi:10.1007/s00592-022-01860-9
Griffith, K. M., Hammer, L. C., Iannuzzi, N. P., Takatani, K. C., Hsu, J. E., Cotton, J. D., et al. (2022). Review of human supraspinatus tendon mechanics. Part II: tendon healing response and characterization of tendon health. J. Shoulder Elb. Surg. 31 (12), 2678–2682. doi:10.1016/j.jse.2022.05.030
Harish, C. S., Dixit, R., Singh, S., and Garg, S. (2020). Sonoelastographic evaluation of the Achilles tendon in patients with type 2 diabetes mellitus. Ultrasound Med. & Biol. 46 (11), 2989–2997. doi:10.1016/j.ultrasmedbio.2020.07.023
Haus, J. M., Carrithers, J. A., Trappe, S. W., and Trappe, T. A. (2007). Collagen, cross-linking, and advanced glycation end products in aging human skeletal muscle. J. Appl. Physiology 103 (6), 2068–2076. doi:10.1152/japplphysiol.00670.2007
Indyk, D., Bronowicka-Szydełko, A., Gamian, A., and Kuzan, A. (2021). Advanced glycation end products and their receptors in serum of patients with type 2 diabetes. Sci. Rep. 11 (1), 13264. doi:10.1038/s41598-021-92630-0
Jia, D., Liu, L., Liu, W., Li, J., Jiang, X., and Xin, Y. (2024a). Copper metabolism and its role in diabetic complications: a review. Pharmacol. Res., 206. doi:10.1016/j.phrs.2024.107264
Jia, G., Jia, X., Yang, J., Shi, T., Qiang, M., and Chen, Y. (2024b). Pioglitazone antagonized the effects of advanced glycation end products on Achilles tendon healing and improved the recovery of tendon biomechanical properties. Cell. Mol. Bioeng. 17 (3), 219–228. doi:10.1007/s12195-024-00800-7
Jud, P., and Sourij, H. (2019). Therapeutic options to reduce advanced glycation end products in patients with diabetes mellitus: a review. Diabetes Res. Clin. Pract. 148, 54–63. doi:10.1016/j.diabres.2018.11.016
Kato, S., Matsumura, T., Sugawa, H., and Nagai, R. (2024). Correlation between serum advanced glycation end-products and vascular complications in patient with type 2 diabetes. Sci. Rep. 14 (1), 18722. doi:10.1038/s41598-024-69822-5
Kato, T., Shinohara, I., Mifune, Y., Inui, A., Nishimoto, H., Yoshikawa, T., et al. (2023). Intra-articular site-specific distribution of advanced glycation end products in the shoulder of patients with diabetes mellitus having rotator cuff tears. Mol. Biol. Rep. 50 (12), 10339–10349. doi:10.1007/s11033-023-08861-z
Kent, M. J. L., and Bailey, A. J. (1985). Evidence for glucose-mediated covalent cross-linking of collagen after glycosylation in vitro. Biochem. J. 225 (3), 745–752. doi:10.1042/bj2250745
Kim, D. H., Min, S. G., Kim, H.-M., Kang, H. R., Choi, J.-H., Lee, H. J., et al. (2022). Comparison of the characteristics of rotator cuff tissue in a diabetic rat model. Orthopedics. 45 (3), e154–e161. doi:10.3928/01477447-20220128-08
Kim, D.-H., Noh, S.-U., Chae, S.-W., Kim, S.-J., and Lee, Y.-T. (2021). Altered differentiation of tendon-derived stem cells in diabetic conditions mediated by macrophage migration inhibitory factor. Int. J. Mol. Sci. 22 (16), 8983. doi:10.3390/ijms22168983
Kim, M. S., Rhee, S. M., and Cho, N. S. (2023). Increased HbA1c levels in diabetics during the postoperative 3-6 Months after rotator cuff repair correlated with increased retear rates. Arthrosc. J. Arthrosc. & Relat. Surg. 39 (2), 176–182. doi:10.1016/j.arthro.2022.08.021
Kwan, C.-K., Fu, S.-C., and Yung, P. S.-h (2020). A high glucose level stimulate inflammation and weaken pro-resolving response in tendon cells – a possible factor contributing to tendinopathy in diabetic patients. Asia-Pacific J. Sports Med. Arthrosc. Rehabilitation Technol. 19, 1–6. doi:10.1016/j.asmart.2019.10.002
Lee, J. M., and Veres, S. P. (2019). Advanced glycation end-product cross-linking inhibits biomechanical plasticity and characteristic failure morphology of native tendon. J. Appl. Physiology 126 (4), 832–841. doi:10.1152/japplphysiol.00430.2018
Li, F., Wang, Y., Cao, J., Chen, Q., Gao, Y., Li, R., et al. (2024a). Integrated analysis of genes shared between type 2 diabetes mellitus and osteoporosis. Front. Pharmacol. 15, 1388205. doi:10.3389/fphar.2024.1388205
Li, L., Zou, J., Zhou, M., Li, H., Zhou, T., Liu, X., et al. (2024c). Phenylsulfate-induced oxidative stress and mitochondrial dysfunction in podocytes are ameliorated by Astragaloside IV activation of the SIRT1/PGC1α/Nrf1 signaling pathway. Biomed. & Pharmacother. 177, 117008. doi:10.1016/j.biopha.2024.117008
Li, Q., Wang, Y., Yan, J., Yuan, R., Zhang, J., Guo, X., et al. (2024b). Osthole ameliorates early diabetic kidney damage by suppressing oxidative stress, inflammation and inhibiting TGF-β1/Smads signaling pathway. Int. Immunopharmacol. 133, 112131. doi:10.1016/j.intimp.2024.112131
Li, Y. F. G., Georgiadis, M., and Snedeker, J. G. (2013). Advanced glycation end-products diminish tendon collagen fiber sliding. MATRIX Biol. 32 (3-4), 169–177. doi:10.1016/j.matbio.2013.01.003
Lo, A. W. I., Oliveira, R. R., Martins, C. S., Rocha, Y. R., Braga, A. B. R., Mattos, R. M., et al. (2013). Experimental diabetes induces structural, inflammatory and vascular changes of Achilles tendons. PLoS ONE 8 (10), e74942. doi:10.1371/journal.pone.0074942
Lopez-Pedrosa, J. M., Camprubi-Robles, M., Guzman-Rolo, G., Lopez-Gonzalez, A., Garcia-Almeida, J. M., Sanz-Paris, A., et al. (2024). The vicious cycle of type 2 diabetes mellitus and skeletal muscle atrophy: clinical, biochemical, and nutritional bases. Nutrients 16 (1), 172. doi:10.3390/nu16010172
Lu, P.-P., Chen, M.-H., Dai, G.-C., Li, Y.-J., Shi, L., and Rui, Y.-F. (2020). Understanding cellular and molecular mechanisms of pathogenesis of diabetic tendinopathy. World J. Stem Cells 12 (11), 1255–1275. doi:10.4252/wjsc.v12.i11.1255
Menè, P. F. F., and Pugliese, F. (2003). Clinical potential of advanced glycation end-product inhibitors in diabetes mellitus. Am. J. CARDIOVASC DRUG 3 (5), 315–320. doi:10.2165/00129784-200303050-00002
Mir, M. M., Alfaifi, J., Sohail, S. K., Rizvi, S. F., Akhtar, M. T., Alghamdi, M. A. A., et al. (2024). The role of pro-inflammatory chemokines CCL-1, 2, 4, and 5 in the etiopathogenesis of type 2 diabetes mellitus in subjects from the asir region of Saudi arabia: correlation with different degrees of obesity. J. Personalized Med. 14 (7), 743. doi:10.3390/jpm14070743
Nichols, A. E. C., Oh, I., and Loiselle, A. E. (2019). Effects of type II diabetes mellitus on tendon homeostasis and healing. J. Orthop. Res. 38 (1), 13–22. doi:10.1002/jor.24388
Noonin, C., and Thongboonkerd, V. (2024). Curcumin prevents high glucose-induced stimulatory effects of renal cell secretome on fibroblast activation via mitigating intracellular free radicals and TGF-β secretion. Biomed. & Pharmacother. 174, 116536. doi:10.1016/j.biopha.2024.116536
Nopparat, J., Khimmaktong, W., Pholpramool, C., and Tipbunjong, C. (2023). Hypoglycemic effect of flavonoid glabridin prevents homeostatic disruption of native Achilles tendon in streptozotocin-induced type I diabetic rats. Sains Malays. 52 (2), 579–588. doi:10.17576/jsm-2023-5202-19
Osonoi, S., Mizukami, H., Itabashi, C., Wada, K., Kudoh, K., Igawa, A., et al. (2020). Increased oxidative stress underlies abnormal pain threshold in a normoglycemic Japanese population. Int. J. Mol. Sci. 21 (21), 8306. doi:10.3390/ijms21218306
Panji Sananta, L. S., Sintong, L., Andarini, S., Putera, M. A., and Dradjat, R. S. (2019). Increasing number of fibroblast, capillary and collagen amount in Achilles tendons of rats with diabetic mellitus after application of stromal vascular fraction derived from adipose tissue from adipose tissue. Curr. Orthop. Pract. 30(5):465–470. doi:10.1097/bco.0000000000000789
Peng, Z., Qi, B., Luo, Z., Sun, Y., Zhang, X., Lin, J., et al. (2024). Agomir-122-loaded nanoparticles coated with cell membrane of activated fibroblasts to treat frozen shoulder based on homologous targeting. J. nanobiotechnology 22 (1), 165. doi:10.1186/s12951-024-02403-w
Primadhi, R. A., and Herman, H. (2021). Diabetic foot: which one comes first, the ulcer or the contracture? World J. Orthop. 12 (2), 61–68. doi:10.5312/wjo.v12.i2.61
Puri, D., Kaur, J., Gaur, N., and Kodidala, S. R. (2022). Role of glycated hemoglobin in microvascular complications in type 2 diabetes mellitus: cross sectional study. Int. J. Endocrinol. (Ukraine) 18 (6), 319–323. doi:10.22141/2224-0721.18.6.2022.1201
Quaini, F., Ahmed, A. S., Li, J., Abdul, A. M. D., Ahmed, M., Östenson, C.-G., et al. (2017). Compromised neurotrophic and angiogenic regenerative capability during tendon healing in a rat model of type-II diabetes. Plos One 12 (1), e0170748. doi:10.1371/journal.pone.0170748
Quetglas-Llabrés, M. M., Monserrat-Mesquida, M., Bouzas, C., García, S., Mateos, D., Ugarriza, L., et al. (2024). Long-term impact of nutritional intervention with increased polyphenol intake and physical activity promotion on oxidative and inflammatory profiles in patients with metabolic syndrome. Nutrients 16 (13), 2121. doi:10.3390/nu16132121
Ra, P., and Hn, R. (2022). Is elevated Hba1c level associated with Achilles tendon contracture development in diabetic foot patients? Malays. Orthop. J. 16 (1), 70–75. doi:10.5704/MOJ.2203.010
Ramirez, M., Bastien, E., Chae, H., Gianello, P., Gilon, P., and Bouzin, C. (2024). 3D evaluation of the extracellular matrix of hypoxic pancreatic islets using light sheet fluorescence microscopy. Islets 16 (1), 2298518. doi:10.1080/19382014.2023.2298518
Riley, G., Harrall, R. L., Constant, C. R., Chard, M. D., Cawston, T. E., and Hazleman, B. L. (1994). Tendon degeneration and chronic shoulder pain:changes in the collagen composition of the humanrotator cuff tendons in rotator cuff tendinitis. Ann. Rheum. Dis. 53 (6), 359–366. doi:10.1136/ard.53.6.359
Schuster, R., Younesi, F., Ezzo, M., and Hinz, B. (2023). The role of myofibroblasts in physiological and pathological tissue repair. Cold Spring Harb. Perspect. Biol. 15 (1), a041231. doi:10.1101/cshperspect.a041231
Shalit, A., Gerontiti, E., Boutzios, G., Korakianiti, E., Kanouta, F., Vasileiou, V., et al. (2024). Nutrition of aging people with diabetes mellitus: focus on sarcopenia. Maturitas 185, 107975. doi:10.1016/j.maturitas.2024.107975
Sharma, P., and Maffulli, N. (2005). Tendon injury and tendinopathy: healing and repair. J. BONE Jt. Surg. Am. 87 (1), 187–202. doi:10.2106/JBJS.D.01850
Shi, L., Lu, P.-P., Dai, G.-C., Li, Y.-J., and Rui, Y.-F. (2021). Advanced glycation end productions and tendon stem/progenitor cells in pathogenesis of diabetic tendinopathy. World J. Stem Cells 13 (9), 1338–1348. doi:10.4252/wjsc.v13.i9.1338
Shinohara, I., Mifune, Y., Inui, A., Nishimoto, H., Yamaura, K., Mukohara, S., et al. (2022a). Advanced glycation end products are associated with limited range of motion of the shoulder joint in patients with rotator cuff tears associated with diabetes mellitus. BMC Musculoskelet. Disord. 23 (1), 271. doi:10.1186/s12891-022-05229-5
Shinohara, I., Mifune, Y., Inui, A., Nishimoto, H., Yamaura, K., Mukohara, S., et al. (2022b). Biochemical markers of aging (advanced glycation end products) and degeneration are increased in type 3 rotator cuff tendon stumps with increased signal intensity changes on MRI. Am. J. Sports Med. 50 (7), 1960–1970. doi:10.1177/03635465221090649
Sikes, K. J., McConnell, A., Serkova, N., Cole, B., and Frisbie, D. (2021). Untargeted metabolomics analysis identifies creatine, myo-inositol, and lipid pathway modulation in a murine model of tendinopathy. J. Orthop. Res. 40 (4), 965–976. doi:10.1002/jor.25112
Singh, K., Huang, S., Xiang, C., and Song, Y. (2022). Identification of the shared gene signatures and pathways between sarcopenia and type 2 diabetes mellitus. Plos One 17 (3), e0265221. doi:10.1371/journal.pone.0265221
Smith, K. M., Presson, A. P., Zhang, C., Horns, J. J., Hotaling, J. M., Tashjian, R. Z., et al. (2021). Does diabetes mellitus predispose to both rotator cuff surgery and subsequent failure? JSES Int. 5 (4), 636–641. doi:10.1016/j.jseint.2021.03.002
Song, F.-C., Yuan, J.-Q., Zhu, M.-D., Li, Q., Liu, S.-H., Zhang, L., et al. (2022). High glucose represses the proliferation of tendon fibroblasts by inhibiting autophagy activation in tendon injury. Biosci. Rep. 42 (3). doi:10.1042/BSR20210640
Sourris, K. C., Watson, A., and Jandeleit-Dahm, K. (2020). “Inhibitors of advanced glycation end product (AGE) formation and accumulation. Reactive oxygen species,” in Handbook of experimental Pharmacology2020, 395–423.
Su, Y. C., Chung, C. H., Ke, M. J., Chen, L. C., Chien, W. C., and Wu, Y. T. (2021). Increased risk of shoulder calcific tendinopathy in diabetes mellitus: a nationwide, population-based, matched cohort study. Int. J. Clin. Pract. 75 (10), e14549. doi:10.1111/ijcp.14549
Su, Y.-C., Hsieh, P.-C., Lai, E. C.-C., and Lin, Y.-C. (2024). Risk of rotator cuff tear and rotator cuff repair surgery comparison between sodium-glucose cotransporter 2 inhibitors and glucagon like peptide-1 receptor agonists: a real-world study. Diabetes & Metabolism 50 (2), 101522. doi:10.1016/j.diabet.2024.101522
Sun, H., Saeedi, P., Karuranga, S., Pinkepank, M., Ogurtsova, K., Duncan, B. B., et al. (2022). IDF Diabetes Atlas: global, regional and country-level diabetes prevalence estimates for 2021 and projections for 2045. Diabetes Res. Clin. Pract., 183. doi:10.1016/j.diabres.2023.110945
Takahashi, R., Kajita, Y., Harada, Y., Iwahori, Y., and Deie, M. (2021). Clinical results of arthroscopic rotator cuff repair in diabetic and non-diabetic patients. J. Orthop. Sci. 26 (2), 213–218. doi:10.1016/j.jos.2020.03.013
Tavares, B. A. P., Antunes, L. C., Guerrero, S. J. S., de Faria, Â. J. N., Carvalho, R. A., and Amorim, R. D. (2021). Ruptura bilateral simultânea do tendão quadricipital em paciente portador de diabetes mellitus do tipo II após trauma de baixa energia: Relato de caso. Rev. Bras. Ortop. 59 (01), e136–e8. doi:10.1055/s-0040-1722584
Vaidya, R., Lake, S. P., and Zellers, J. A. (2022). Effect of diabetes on tendon structure and function: not limited to collagen crosslinking. J. Diabetes Sci. Technol. 17 (1), 89–98. doi:10.1177/19322968221100842
Vasiljević, N., Bigović, D., Šavikin, K., Suručić, R., Djuric, D. M., Jakovljević, V., et al. (2022). A prospective, randomized, double-blind, placebo-controlled trial of polyphenols on the outcomes of inflammatory factors and oxidative stress in patients with type 2 diabetes mellitus. Rev. Cardiovasc. Med. 23 (2), 57. doi:10.31083/j.rcm2302057
Wang, J., Zhang, J., Yu, Z.-L., Chung, S. K., and Xu, B. (2024c). The roles of dietary polyphenols at crosstalk between type 2 diabetes and Alzheimer's disease in ameliorating oxidative stress and mitochondrial dysfunction via PI3K/Akt signaling pathways. Ageing Res. Rev. 99, 102416. doi:10.1016/j.arr.2024.102416
Wang, T., Yu, Z., Lin, S., Chen, Z., Jin, H., Liang, L., et al. (2024b). 3D-printed Mg-incorporated PCL-based scaffolds improves rotator cuff tendon-bone healing through regulating macrophage polarization. Front. Bioeng. Biotechnol. 12. doi:10.3389/fbioe.2024.1407512
Wang, X., Gao, Z., Chen, K., Huang, C., and Li, Y. (2024a). Diabetes mellitus and intervertebral disc degeneration: a meta-analysis. World Neurosurg. 188, e81–e92. doi:10.1016/j.wneu.2024.05.043
Wu, J., Wang, S., Zhuang, H., Wang, W., Wang, Y., Chen, Y., et al. (2024). Proteomics analysis provides insights into the role of lipid metabolism in t2dm-related sarcopenia. ACS Omega 9 (31), 34056–34069. doi:10.1021/acsomega.4c04668
Xu, K., Zhang, L., Ren, Z., Wang, T., Zhang, Y., Zhao, X., et al. (2022). Evaluating the role of type 2 diabetes mellitus in rotator cuff tendinopathy: development and analysis of a novel rat model. Front. Endocrinol. 13, 1042878. doi:10.3389/fendo.2022.1042878
Xu, L., Xu, K., Wu, Z., Chen, Z., He, Y., Ma, C., et al. (2020). Pioglitazone attenuates advanced glycation end products-induced apoptosis and calcification by modulating autophagy in tendon-derived stem cells. J. Cell. Mol. Med. 24 (3), 2240–2251. doi:10.1111/jcmm.14901
Yoon, J. P., Park, S.-J., Choi, Y. S., Kim, D.-H., Lee, H. J., Park, E. J. J., et al. (2024). Current research trends on the effect of diabetes mellitus on rotator cuff tendon healing/tendinopathy. Archives Orthop. Trauma Surg. 144 (6), 2491–2500. doi:10.1007/s00402-024-05350-1
Yoshikawa, T., Mifune, Y., Inui, A., Nishimoto, H., Yamaura, K., Mukohara, S., et al. (2022). Influence of diabetes-induced glycation and oxidative stress on the human rotator cuff. Antioxidants 11 (4), 743. doi:10.3390/antiox11040743
Yuan, X. Z., Zhengang, G., Kaizheng, Z., Pei, Q., and Liu, N. (2011). Inhibition of reactive oxygen species/extracellular signal-regulated kinases pathway by pioglitazone attenuates advanced glycation end products-induced proliferation of vascular smooth muscle cells in rats. Biol. Pharm. Bull. 34 (5), 618–623. doi:10.1248/bpb.34.618
Yuan, Z., Zhu, X., Dai, Y., Shi, L., Feng, Z., Li, Z., et al. (2024). Analysis of differentially expressed genes in torn rotator cuff tendon tissues in diabetic patients through RNA-sequencing. BMC Musculoskelet. Disord. 25 (1), 31. doi:10.1186/s12891-023-07149-4
Zaib, S., Ahmad, S., Khan, I., Bin Jardan, Y. A., and Fentahun, W. G. (2024). An evaluation of inflammatory and endothelial dysfunction markers as determinants of peripheral arterial disease in those with diabetes mellitus. Sci. Rep. 14 (1), 15348. doi:10.1038/s41598-024-65188-w
Zellers, J. A., Eekhoff, J. D., Walk, R. E., Hastings, M. K., Tang, S. Y., and Lake, S. P. (2021). Human Achilles tendon mechanical behavior is more strongly related to collagen disorganization than advanced glycation end-products content. Sci. Rep. 11 (1), 24147. doi:10.1038/s41598-021-03574-4
Zhao, C., Ackerman, J. E., Geary, M. B., Orner, C. A., Bawany, F., and Loiselle, A. E. (2017). Obesity/Type II diabetes alters macrophage polarization resulting in a fibrotic tendon healing response. Plos One 12 (7), e0181127. doi:10.1371/journal.pone.0181127
Keywords: diabetes, tendon, tendinopathy, pathology, fibrosis
Citation: Xu J, Wang J, Ji Y, Liu Y, Jiang J, Wang Y, Cui X, Wan Y, Guo B and Yu H (2024) The impact of diabetes mellitus on tendon pathology: a review. Front. Pharmacol. 15:1491633. doi: 10.3389/fphar.2024.1491633
Received: 05 September 2024; Accepted: 10 October 2024;
Published: 05 November 2024.
Edited by:
Yanan Jiang, Harbin Medical University, ChinaReviewed by:
Zhang Yuhan, Capital Medical University, ChinaXiang-Dong Wu, Beijing Jishuitan Hospital, China
Copyright © 2024 Xu, Wang, Ji, Liu, Jiang, Wang, Cui, Wan, Guo and Yu. This is an open-access article distributed under the terms of the Creative Commons Attribution License (CC BY). The use, distribution or reproduction in other forums is permitted, provided the original author(s) and the copyright owner(s) are credited and that the original publication in this journal is cited, in accordance with accepted academic practice. No use, distribution or reproduction is permitted which does not comply with these terms.
*Correspondence: Haiyang Yu, ZnkueWh5QDE2My5jb20=; Biao Guo, aGFwcHlnYkAxMjYuY29t
†These authors have contributed equally to this work