- 1Department of Neurosurgery, West China Hospital, Sichuan University, Chengdu, China
- 2Department of Pharmacy, Sichuan Clinical Research Center for Cancer, Sichuan Cancer Center, Sichuan Cancer Hospital & Institute, Affiliated Cancer Hospital of University of Electronic Science and Technology of China, Chengdu, China
- 3Department of Gynecological Nursing, West China Second Hospital, Sichuan University, Chengdu, China
Under normal physiological conditions, Fyn, a nonreceptor tyrosine kinase, is involved in signal transduction pathways in the nervous system and in the formation and activation of T lymphocytes. Fyn is a member of the Src family of kinases (SFKs) and plays a role in cell morphogenic transformation, motility, proliferation, and death, which in turn influences the development and progression of various cancer types. SFKs are overexpressed or hyperactive in tumours, and they are engaged in several signalling pathways that lead to tumour development. Inhibition of Fyn can enhance patient outcomes and prolong survival. Thus, Fyn is a desirable therapeutic target in a variety of tumour types. To lay the groundwork for further investigation and targeted therapy in tumours, in this article, we review the most recent findings on the function of Fyn in tumours, with an emphasis on its role in gliomas. Understanding the function of Fyn during tumourigenesis and development and in resistance to anticancer therapeutic agents can aid in the development and application of innovative medicines that specifically target this kinase, thus improving the management of cancers.
Introduction
Among the earliest kinases to be identified were the Src family of kinases (SFKs) (Frame, 2002), which contains 11 members, of which 8—c-Src, Fyn, Yes, Lck, Lyn, Hck, Fgr, and Blk—have been extensively researched. While Fyn, Yes, and c-Src are expressed throughout the human body, Blk, Hck, and Fgr are exclusively expressed in certain tissues (Thomas and Brugge, 1997). Among the SFKs, c-Src has been the most extensively researched in terms of cancer biology, as it is a crucial molecule in the genesis, progression, and resistance of tumours to treatment (Larsen et al., 2015). Over the past 10 years, it has become increasingly clear how other members of the SFK family—including Fyn—are involved in different facets of cancer biology.
Myristic and palmitic acids bind and localize Fyn to the inner layer of the cell membrane (Alland et al., 1994). Like other SFKs, Fyn function is controlled by tyrosine phosphorylation- and dephosphorylation-induced intermolecular interactions. Many target proteins, such as focal adhesion kinase (FAK) and breast cancer anti-oestrogen resistance protein 1 (BCAR1), undergo tyrosine phosphorylation as a result of Fyn activation (Yeatman, 2004). Fyn also regulates cell growth, survival, adhesion, cytoskeletal remodelling, motility, axon guidance, synaptic function, myelination in the central nervous system, platelet activation, and T-cell receptor signalling, among other various biochemical processes (Kinsey, 2014). The function of Fyn in the brain is discussed specifically in this review, particularly in relation to several elements of the pathophysiology of brain tumours, such as gliomas.
Gene and protein structure
Chromosome 6q21 contains genetic information for Fyn, a 59-kDa protein with 537 amino acids (p59-FYN, Slk, Syn, MGC45350, Gene ID 2534). Fyn belongs to the Src family and was first discovered in 1986 (reported as Syn or Slk) using probes constructed from v-yes and v-fgr (Semba et al., 1986; Alland et al., 1994). Fyn is mostly found in the cytoplasmic leaflet of the plasma membrane, where it phosphorylates tyrosine residues on important targets connected to a wide range of signalling pathways.
Three distinct transcript isoforms of Fyn have been discovered, and among the three genomic sequences, isoform 1 (isoform a, Fyn [B]) is the longest and was the first to be discovered. Compared with isoform 1, isoform 2 (also known as isoform b, Fyn [T]) is more capable of mobilizing cytoplasmic calcium and is more likely to be expressed in T cells (Thomas and Brugge, 1997). Some of the variations in the regulation of these two isoforms can be attributed to alterations in the linker region between the SH2 and SH1 domains (Alland et al., 1994). In the area close to the start of the kinase domain and the end of the SH2 domain, isoforms 2 and 1 differ by approximately 50 amino acids (Figure 1). While most tissues express a combination of both isoforms (Thomas and Brugge, 1997), the brain expresses high levels of Fyn(B), whereas T cells highly express Fyn(T). Isoform 3 (isoform c) lacks exon 7 (FynD7) and has been reported to be expressed in blood cells; however, no translated protein has been identified (Goldsmith et al., 2002). Other transcript variants have also been identified, but they have not yet been linked to any disease state.
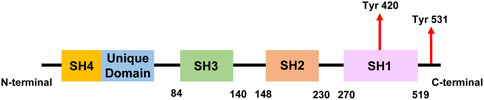
Figure 1. A simplified linear structure of Fyn. The numbers indicate amino acid residues and the locations of tyrosine residues that can be phosphorylated during Fyn activation.
Fyn regulates the phosphorylation of intracellular tyrosine proteins by interacting with numerous cell surface receptors, such as those on mast cells and T cells (Davidson et al., 1994). The development of oligodendrocytes, keratinocytes, and natural killer cells is a result of the physiological function of Fyn in cellular processes such as lymphocyte receptor signalling, and Fyn is known to be involved in adhesion, cell migration, and platelet activation (Cary et al., 1996; Zamoyska et al., 2003; Reddy et al., 2008).
Fyn in the brain
Fyn plays a significant role in both adult brain function and brain development. The biological roles of Fyn in the brain have been thoroughly studied using transgenic animal models. These studies revealed that Fyn is an essential component for the growth and operation of the central nervous system. Mice lacking Fyn exhibit a variety of brain abnormalities, such as distorted hippocampal architecture and aberrant long-term potentiation (Kojima et al., 1997), impaired spatial learning and increased sensitivity to ethanol (Grant et al., 1992), which indicates the physiological importance of Fyn in a variety of brain communication pathways. Furthermore, Fyn has been detected in several brain regions, such as glial cells in white matter tracts and cultured oligodendrocytes, and plays significant roles in CNS myelination, which is the process by which a myelin sheath forms around a nerve fibre. Actually, myelination occurs when Fyn is most active in the brain (Osterhout et al., 1999). Sperber and colleagues reported that Fyn knockout (KO) mice exhibit substantial myelin loss in the forebrain at all ages (from 14 days to 1 year). Their investigation demonstrated that Fyn has a distinct function in myelination according to the number of oligodendrocytes and myelinated fibres, and their findings were supported by the results of experiments in which an inactivated form of Fyn (containing a single amino acid substitution) was tested (Sperber et al., 2001).
Fyn plays a role in the morphological differentiation that causes oligodendrocytes to generate neurites, which are projections that extend from the cell body of a neuron. Fyn interacts with α-tubulin, a tubulin family member that plays a crucial role in the polar orientation and nucleation of microtubules, which are cytoskeletal structures necessary for the generation of neurites. More specifically, membrane-associated α-tubulin combines with Fyn to form a complex that participates in the signalling pathway that initiates the nucleation of membrane-associated microtubules. In one study, pretreatment of P19 embryonal cancer cells with wortmannin or SFK inhibitors consistently inhibited α-tubulin complex nucleation activity (Macurek et al., 2008). Additionally, the association of Fyn with Tau, a cytoskeletal protein that binds to tubulin to stabilize microtubules in the brain, promotes this process (Lee et al., 1998).
Additional research revealed that Tau–Fyn interactions in oligodendrocytes are important for developmental myelination and that some human CNS neurodegenerative diseases, such as multiple sclerosis, may be caused by dysfunctional Tau–Fyn interactions. For example, in multiple sclerosis, axonal degeneration is the primary cause of clinical decline (Belkadi and LoPresti, 2008).
Interestingly, tyrosine-phosphorylation of Tau has also been reported in human prostate cancer cells (Sangrajrang et al., 1998), and it was previously reported that tyrosine within Tau can be phosphorylated in vitro by the oncogene v-fms, a Src family tyrosine kinase expressed in human histiocytic lymphoma cells (Kim et al., 1991). Since cancer and oncogenes are associated with abnormal cell proliferation, these findings in nonneuronal cells may serve to link the tyrosine phosphorylation of Tau to cell signalling pathways that induce cell growth. Preclinical studies in Fyn-deficient mice have shown that Fyn plays a role in development (Grant et al., 1992; Lowell and Soriano, 1996). Fyn also participates in the cell cycle (Yasunaga et al., 1996; Sette et al., 2002). Previous studies have demonstrated the upregulation of Fyn in the AD brain (Shirazi and Wood, 1993) and the presence of a Fyn phosphorylation site in Tau(Lee et al., 2004), which further supports a role for Fyn in the pathogenesis of neurodegenerative disorders.
Some studies have focused on the nonreceptor tyrosine kinase Fyn, which interacts with polyproline helices in Tau through its SH3 domain (Lee et al., 1998), and interestingly, also regulates seizure susceptibility (Cain et al., 1995; Kojima et al., 1998). Tau reduction is protective in a Fyn-dependent model of AD (Roberson et al., 2011). Thus, studies have shown that Tau targets Fyn to dendrites, where Fyn facilitates N-methyl-d-aspartate (NMDA) receptor-mediated dysfunction and aberrant Tau phosphorylation (Ittner et al., 2010; Larson et al., 2012).
The role of Tau or Fyn, or both, as part of a shared mechanism of neuronal hyperexcitability and seizures has been reported (Roberson et al., 2011; Putra et al., 2020). Genetically ablated Fyn or pharmacological inhibition of Fyn/SFK prevents neuronal loss and decreases network hyperexcitability and seizure progression (Kaufman et al., 2015; Sharma et al., 2018). The proconvulsive property of Fyn is also mediated through microglia, independently of Tau, and Fyn/SFK inhibition significantly decreases epileptogenesis (Sharma et al., 2021). These findings suggest the role of Fyn and Tau in promoting seizures and epilepsy. A recent study in an experimental temporal lobe epilepsy (TLE) model demonstrated that amyloidogenic pathways promote Tau pathology during early epileptogenesis (Thom et al., 2011). Similarly, another group reported the deposition of Aβ in the hippocampus of human patients with chronic TLE (Gourmaud et al., 2020), which suggests possible early interactions between Fyn-Tau complexes and amyloid pathways during epileptogenesis that may persist in the chronic stage of epilepsy. Moreover, the degree of Fyn-Tau interactions was shown to be positively correlated with NR2B-PSD95 complexes, Tau phosphorylation and increased Fyn levels in the human epileptic brain. The binding of Fyn-Tau is also correlated with microgliosis, which suggests its contribution to the neuroinflammatory state of the human epileptic brain (Putra et al., 2024). Recently, tat-Tau PxxP5/6, a peptide that targets Fyn-Tau interactions, was shown to prevent Aβ-induced neurotoxicity in vitro, but the efficacy of this peptide has not been tested in vivo (Rush et al., 2020; Roth et al., 2024). Thus, blocking Fyn and Tau interactions with this peptide inhibitor could further validate the outcomes of pharmacological inhibition of Fyn/SFK in an epilepsy model and could identify the Fyn-Tau interaction as a potential therapeutic target in epilepsy.
In the healthy brain, the microglial response is protective and may decrease once damage has occurred; however, under pathological conditions, microglia become activated and release reactive oxygen species (ROS), nitric oxide (NO) and proinflammatory cytokines, such as tumour necrosis factor-α (TNF-α), interleukin (IL)-1β (IL-1β) and interferon gamma (IFN-γ) (Yan et al., 2014). These products further accelerate microglial activation by binding to their microglial cell surface receptors to sustain chronic inflammation (Kim and de Vellis, 2005). Fyn kinase has been demonstrated to be involved in this process through its role in microglial activation via the Fyn‒PKCδ signalling axis and NOD-like receptor protein 3 (NLRP3) inflammasome. Under pathological conditions, such as those observed in neurodegenerative diseases, these proinflammatory cytokines bind to their receptors on the microglial cell surface, which leads to further propagation of aberrant microglial activation (Xu et al., 2021). Studies involving cell culture and transgenic Fyn−/− mice have consistently demonstrated that Fyn is required for cytokine release and activation of iNOS(Ko et al., 2018; Sharma et al., 2018). More recently, Fyn was shown to be critical for the upregulation and posttranslational modification of Kv1.3, a voltage-gated calcium channel, in microglia (Sarkar et al., 2020). Kv1.3 may play a key role in sustaining the chronic neuroinflammatory response observed in PD.
In addition to microglia, Fyn is also expressed to a lesser extent in astrocytes, where it has a key role in astrocytic migration in response to neuronal signals (Dey et al., 2008). Astrocytes are also involved in the regulation of the CNS immune response, and similarly to microglia, they play both beneficial and detrimental roles in the brain’s response to insult or injury (Giovannoni and Quintana, 2020). Fyn kinase, particularly the isoform FynT, plays a role in the astrocyte-mediated production of proinflammatory cytokines (IL-1β and IL-6) via the PKCδ signalling axis, and additionally, Fyn kinase inhibition attenuates this response (Lee et al., 2017). This response is associated specifically with chronic exposure to inflammation, which suggests the involvement of astrocytes in a more chronic insult. Fyn also appears to play a regulatory role in the astrocytic expression of iNOS following inflammatory stimulation, as increased iNOS expression is observed in Fyn-deficient astrocytes (Ko et al., 2018). Interestingly, this seems to contrast with findings in Fyn-deficient microglia, in which iNOS expression is decreased (Panicker et al., 2015). These data suggest that Fyn kinase is not only involved in the upregulation of the microglia-mediated release of iNOS but may also be involved in the downregulation of the astrocyte response.
The postsynaptic density (PSD), the primary cytoskeletal specialization at neuronal excitatory synapses, is where PSD95, NMDAR, and AMPAR, among other proteins, reside. Fyn and other SFK members are involved in synaptic transmission and plasticity at excitatory synapses. PSD95 plays a crucial role in the multiprotein complex formed by NMDARs by directly attaching to the NR2 subunit of NMDARs. Additionally, PSD95 interacts with the SH2 domain of Fyn, and it has been suggested that this interaction helps Fyn phosphorylate tyrosine residues within the NMDAR subunit NR2A (Tezuka et al., 1999; Kalia and Salter, 2003). Thus, Fyn (as well as Src) controls the phosphorylation of the NMDAR complex and increases NMDAR activity, which results in the generation of NMDAR-dependent synaptic potentiation (Salter and Kalia, 2004).
Additionally, Fyn modulates other CNS signalling proteins; Fyn works in tandem with Cas and other FAK family kinases to control the shape of dendritic spines, which are the primary sites of the postsynaptic components of excitatory synapses in the mammalian central nervous system (Bourgin et al., 2007) (Figure 2).
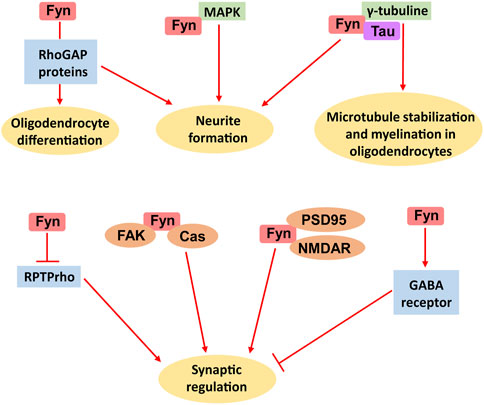
Figure 2. Multiple biological functions of Fyn in the brain. The main roles of Fyn include oligodendrocyte differentiation, neurite formation, microtubule stabilization, myelination by oligodendrocytes and synaptic regulation.
Biological functions of Fyn in cancer
Fyn regulates the tumour cell cycle
As a member of the Src family of kinases, Fyn uses anti-SH2 to halt cytoplasmic division following mitosis, which inhibits cell division (Tominaga et al., 2000; Ng et al., 2005). Fyn controls the stabilization and polymerization of microtubules, which in turn controls the development of mitotic spindles. Through enhanced microtubule aggregation, Fyn stimulates the development of mitotic spindles, which accelerates the progression of M phase (Okamoto et al., 2016). Insufficient Fyn activity results in cytoplasmic division failure and prevents the occurrence of mitosis (Levi et al., 2010). In addition, depending on its N-terminal length, Fyn can be confined to the cortical membrane-bound region during cytoplasmic division (Czech and Vander Zanden, 1991). It is believed that cortical Fyn regulates cytoplasmic division (Levi et al., 2010). According to the findings above, Fyn prevents pericellular growth and suppresses mitotic progression.
Fyn regulates tumour cell adhesion
Dasatinib-induced Fyn inhibition or Fyn silencing has been shown to improve intercellular adhesion (Fenton et al., 2015). T-cell activation is followed by initial T-cell adhesion, which occurs independently of Fyn kinase activity. Nevertheless, noncatalytically functioning Fyn is necessary for late cell attachment (Chapman et al., 2012). In integrin α6-deficient acute lymphoblastic leukaemia (ALL), phosphorylated Fyn (pTyr530) is increased and mediates the development of chemoresistance through adhesion (Gang et al., 2020).
Fyn regulates tumour cell proliferation
Fyn is a proto-oncogene and member of the Src family. Numerous studies have shown that Fyn inhibits apoptosis and promotes cancer cell growth. As an essential mediator and regulator of mitogenic signalling, Fyn controls cell entry into the cell cycle, growth, and proliferation (Gururajan et al., 2015). Fyn is expressed more frequently in thyroid cancer at both the mRNA and protein levels, thus facilitating cell division and preventing apoptosis (Zheng et al., 2017). MicroRNA-125a-3p directly targets Fyn to inhibit both its expression and activity; additionally, this RNA drives the production of Fyn downstream proteins and cell cycle arrest, which further suppresses cell proliferation. This implies that Fyn stimulates tumour cell growth (Ninio-Many et al., 2013). Increased Fyn expression and activity in chronic granulocytic leukaemia facilitates the transition from chronic to acute disease and increases the rate of cell division (Singh et al., 2012). Osteoclast apoptosis is inhibited by Fyn, thus resulting in osteoclast growth (Kim et al., 2010). Samples from patients with acute myeloid leukaemia (AML) exhibit dysregulated Fyn expression, which is linked to both oncogenic FLT3-ITD and wild-type FLT3. The SH2 structural domain of Fyn and the kinase activity of FLT3 are required for this association. FLT3 contains several Fyn binding sites, and Fyn expression increases STAT5 phosphorylation and colony formation but also marginally increases the phosphorylation of AKT, ERK1/2, and p38. Furthermore, a poorer prognosis in AML patients is associated with increased Fyn expression in conjunction with the FLT3-ITD mutation, which is enriched in the STAT5 signalling pathway. These findings show that Fyn preferentially activates the STAT5 pathway in conjunction with the oncogenic FLT3-ITD gene during cell transformation to increase AML cell proliferation (Chougule et al., 2016). By upregulating Fyn expression and downregulating miR-153-3p expression, LINC00152 promotes the growth of oesophageal squamous cell carcinoma (ESCC) (Liu D. et al., 2019). Fyn phosphorylates PIKE-A in glioblastoma, which encourages the binding of this protein to AMPK, reduces the ability of AMPK to prevent tumour growth, and increases the rate at which tumour cells proliferate (Zhang et al., 2016). The proliferation of pancreatic cancer cells is hindered by the inhibition of Fyn activity (Je et al., 2014). Increased Fyn activity in skin squamous cell carcinoma (SCC) cells decreases Notch1/NICD mRNA and protein expression levels and stimulates STAT3 phosphorylation to promote tumourigenesis and proliferation (Zhao et al., 2009). By inhibiting cellular senescence and promoting the formation of malignant gliomas, Fyn phosphorylates STAT3 and increases G6PD expression (Sun et al., 2021). Fyn also interacts with ARHGEF16 to stimulate the growth of colon cancer cells (Yu B. et al., 2020). Moreover, melanoma cell proliferation is inhibited by the Fyn/STAT3 pathway (Tang et al., 2020). Through the activation of GluN2b and the control of the AKT protein kinase signalling pathway, Fyn promotes the growth of pancreatic cancer (Dong et al., 2020).
Fyn and the immune response
Increased expression or activation of Src and its downstream protein PI3K enhances the growth and activation of lymphocytes, macrophages, dendritic cells, and natural killer (NK) cells. Fyn splice variation was originally observed in T lymphocytes (Sugie et al., 2004; Abram and Lowell, 2008). According to one study, Fyn activity is necessary for antigen-specific T-cell activation, as the inhibition of Fyn activity significantly reduces the T-cell response (Sugie et al., 2004). Dasatinib, a Bcr-Abl tyrosine kinase inhibitor that also inhibits SFKs, has been used to treat CML patients in clinical trials. This medication causes transitory immunosuppression, which is characterized by the activation of T lymphocytes and haemophilic cells by T-cell receptors and IgE(Sillaber et al., 2009). The effects of SFK inhibitors on patients treated with dasatinib were described in a previous study. Lipid disturbance and a lack of Fyn binding to intraluminal leaflets reduce NK cell activation (Wu et al., 2021). FasL overexpression increases the death of NK cells and T cells by attracting Fyn via proline-rich domains (Malarkannan, 2020).
Elevated Fyn expression in glioma cells diminishes the immunological response against glioma, whereas Fyn inhibition enhances the effectiveness of antiglioma immunotherapy (Comba et al., 2020). Cytokine production in NK and T cells is selectively regulated by the Fyn-ADAP pathway (Gerbec et al., 2015). ADAP, SKAP55, and SHP-2 are directly bound and phosphorylated by Fyn, while SHP-2 interacts with PD-1 to promote PD-1+ CTLA-4+ CD8+ TILs in malignancies (Li et al., 2015).
Fyn in tumour drug resistance
Numerous studies have revealed that Fyn promotes drug resistance in tumours, which is a significant obstacle to the successful treatment of cancer patients. The susceptibility of TKI-resistant cells to the dual BCR-ABL1/Src inhibitor dasatinib increases when Fyn protein expression levels are knocked down versus when Fyn activity is inhibited (Irwin et al., 2015). In one study, the knockdown of Fyn kinase via pharmacological inhibition or siRNA resensitized a BCR-ABL inhibitor imatinib-resistant chronic granulocytic leukaemia (CML) cell line (IM-R cells) to imatinib (Fenouille et al., 2010). Moreover, the susceptibility of tamoxifen-sensitive cells to tamoxifen therapy decreased after Fyn overexpression. Moreover, tamoxifen sensitivity was restored upon the suppression of Fyn expression, and mechanistic research revealed that Fyn counteracts the antiproliferative effects of tamoxifen by activating crucial cell cycle-related proteins (Elias et al., 2015). Compared with control cells, BC cells are more chemosensitive to DOX when miR-381 downregulates Fyn, which deactivates MAPK signalling (Mi et al., 2018). Drug resistance has been shown to develop in dasatinib-resistant cells through the overactivation of Fyn (Airiau et al., 2017). Tamoxifen resistance in breast cancer (ER+) is caused by Fyn, and the proliferation of tamoxifen-resistant cells and the correlation of tamoxifen-resistant cells with a poor prognosis in breast cancer are markedly decreased by the use of a Fyn inhibitor or by the knockdown of Fyn expression (Joshi et al., 2016). Fyn plays a role in anticancer drug resistance; in K562 cells, increased Fyn expression was linked to imatinib resistance (Grosso et al., 2009). The imatinib resistance observed in prostate cancer patients is modulated by Fyn via its interaction with miR-128/193a-5p/494 (Ergün et al., 2023). Fyn is therefore strongly expressed in numerous types of cancer-resistant cells and contributes to the emergence of treatment resistance in cancer.
Glioma
Gliomas, which account for 40% of all primary brain tumours, are the most common type of brain cancer. The term glioma is used to characterize all primary brain cancers that involve central nervous system (CNS) glial cells (Liang et al., 2020). According to the IARC’s GLOBOCAN report on cancer incidence and mortality, 308,102 new cases of central nervous system malignancies were diagnosed worldwide in 2020, which accounted for 251,329 deaths (Bray et al., 2024). An imbalance between cell proliferation and apoptosis, with reduced apoptosis due to the overexpression of antiapoptotic genes in cells, and increased malignant proliferation resulting in tumour development, is the current theory of glioma development; however, its exact pathogenesis is unknown (Poonan et al., 2021). Fyn tyrosine kinase is overexpressed in human gliomas and is a downstream target of the oncogenic receptor tyrosine kinase pathway (Comba et al., 2020), where abnormal SFK activation results in numerous protumor consequences, such as decreased apoptosis, increased angiogenesis, and enhanced cell invasion, motility, and proliferation (Eskilsson et al., 2016). In patients with GBM, Src and Fyn, two downstream targets of the EGFR oncogenic signalling pathway, are often overexpressed. Since glioblastoma activates the EGFR signalling pathway along with Fyn and Src, blocking Fyn and Src may increase the effectiveness of anti-EGFR-targeted therapy (Ahluwalia et al., 2010). Many malignancies have EGFR mutations. However, EGFR inhibitor-induced clinical responses are rare and fleeting. Fyn and Src were identified as putative EGFR effectors in early studies. Moreover, molecular circuits connecting EGFR/EGFRVIII to Fyn and Src have been shown to increase glioblastoma invasion and tumour growth in a variety of cell lines and mouse models. These findings in tumour tissues validate the clinical significance of the abovementioned results, as glioblastoma patients with activated EGFR signalling also often exhibit activated Fyn and Src. These findings indicate that Fyn and Src are clinically significant targets and that blocking them could improve the effectiveness of treatments that target EGFR (Lu et al., 2009).
Fyn establishes a complex regulatory pathway involving specific molecules during glioma development. T-cell immunoglobulin and mucin domain containing-3 (Tim-3), which is highly expressed in gliomas, is a typical immune checkpoint molecule (Kim et al., 2020). Galectin-9 (Gal-9) is the primary ligand that activates Tim-3. According to one study, Tim-3 interacts with Fyn kinase and binds Gal-9 (Wolf et al., 2020). A member of the PIKE family, PtdIns-3-kinase enhancer-activating Akt (PIKE-A), is an oncogenic factor that is essential for the survival and proliferation of cancer cells (Zhang et al., 2019). Numerous investigations have demonstrated that PIKE-A expression is elevated in a variety of malignancies, including glioblastoma, and that it facilitates the growth, invasion, and survival of glioblastoma cells in situations of cellular energy stress (Jia et al., 2016). Fyn can phosphorylate the GTPase PIKE-A, which prevents its degradation (Zhang et al., 2016). Interestingly, PIKE-A increases the growth of glioblastoma and suppresses cellular senescence by triggering the Fyn-mediated STAT3 signalling pathway, which increases the activation of the pentose phosphate pathway (PPP), promotes G6PD expression, and increases DNA synthesis and ROS detoxification (Sun et al., 2021). G6PD is essential for cancer progression, but its underlying mechanisms are still unknown. Some researchers have demonstrated that Fyn directly phosphorylates and increases G6PD activity in response to EGFR activation, which then activates the PPP. Furthermore, Fyn expression, malignancy, and prognosis are correlated with G6PD pY481 in human glioblastoma. These results demonstrate a critical function for Fyn-dependent G6PD phosphorylation in tumour development stimulated by EGF (Liu et al., 2019b).
A recent article reported that the Fyn gene, together with other genes involved in brain development and neural differentiation, is strongly enriched in astrocytoma, a common and lethal human malignancy (Wu et al., 2010). Moreover, Fyn and c-Src are effectors of oncogenic EGFR signalling in glioblastoma and enhance invasion and tumour cell survival in vivo. In one study, the pan-SFK inhibitor dasatinib consistently inhibited invasion, promoted tumour regression, and induced apoptosis in vivo, which significantly prolonged the survival of mice in an orthotopic glioblastoma model. This study demonstrated a mechanism linking EGFR signalling with Fyn and Src activation to promote tumour progression and invasion and provided a rationale for combined anti-EGFR and anti-SFK targeted therapies (Lu et al., 2009). In addition, a phosphotyrosine proteomic screen identified novel signalling molecules, including JAK1, STAT1, cortactin, FER, p130Cas, c-Src and Fyn, as molecules that undergo tyrosine phosphorylation and activation in human malignant mesothelioma. They also confirmed that known signal transduction pathways previously implicated in mesothelioma, such as EGFR and Met, are coactivated in most human mesothelioma specimens and tested cell lines. Since all these enzymes seem to be hyperactivated in malignant mesothelioma cell lines, dual or multitargeted inhibition of some of these kinases is likely to be more efficacious than inhibition of a single tyrosine kinase to prevent potential antiproliferative activity in glioma treatment (Menges et al., 2010).
Finally, cognitive impairments and recurring seizures affect up to 80% of all patients with diffuse glioma and up to 50% of patients with glioblastoma multiforme (GBM) during the course of the disease (van Breemen et al., 2007; van Kessel et al., 2017). Although no single experimental model recapitulates the full diversity of human gliomas, insight into the emergence of hyperexcitability and the natural history of epileptogenesis in cortical networks, along with the opportunity to link these to specific oncogenic drivers (Yu K. et al., 2020), can provide a precise, mechanism-based approach to individualized medical management of this serious tumour comorbidity. The mechanisms underlying peritumoral hyperexcitability in glioma are likely reciprocal in that greater excitability drives tumour progression, and greater tumour progression promotes further hyperexcitability (Hatcher et al., 2020). These pathological waves involve a massive intracellular calcium influx mediated in part by NMDA receptor activation, which transiently silences neuronal activity and briefly impairs the precise coding of high-frequency synaptic inputs in recovering neurons (Revah et al., 2019). Thus, along with the loss of peritumoral synaptic inhibition, increased extracellular glutamate due to the overexpression of the glial glutamate antiporter system xc-has been proposed to be an important contributor to epileptogenesis in tumour-related epilepsy (Robert et al., 2015; Sørensen et al., 2018). Fyn can regulate neuronal activity, and Tau interacts with Fyn via its amino-terminal projection domain (Lee et al., 1998). Fyn phosphorylates NMDA receptor subunit 2 to facilitate interaction of the NMDA receptor complex with PSD-95 (Nakazawa et al., 2001; Rong et al., 2001), which links NMDA receptors to synaptic excitotoxic downstream signalling (Salter and Kalia, 2004). Disruption of the NMDA receptor/PSD-95 interaction prevents excitotoxic damage in cultured neurons and in a rat model of stroke without affecting synaptic NMDA currents (Aarts et al., 2002), which may decrease neuronal activity and inhibit tumour progression.
Conclusion
Src is a well-known oncogene, but its family members, such as Fyn, have received less attention even though they may be more crucial in some malignancies than c-Src. Since Fyn participates in multiple intracellular signalling pathways to govern processes such as cell proliferation and differentiation, interest in Fyn has increased nearly a century after it was first described. New research has demonstrated that Fyn is aberrantly and extensively expressed in a variety of cell types. Apart from its direct contribution to the control of signalling pathways, Fyn is also linked to certain signalling molecules that are specific to tumour cells. These molecules collectively contribute to the advancement of cancer metastasis and growth. In addition, numerous highly selective Fyn/Src inhibitors have been synthesized and shown to be successful in clinical studies. For example, saracatinib is a highly specific small molecule inhibitor of the SRC family of kinases with an IC50 value of 10 nm against Fyn. In a phase II clinical trial, saracatinib was confirmed to act as a metastasis suppressor in prostate cancer in the initial stages (Posadas et al., 2016). Saracatinib can be used alone or in combination with radiotherapy to treat malignant tumours, such as glioblastoma (Yun et al., 2021). Dasatinib is a novel and effective multitargeted inhibitor of kinases of the SRC family, as well as several other kinases. In a phase II clinical trial in patients with melanoma, dasatinib was not significantly effective because of poor patient tolerance and dosage reductions (Kluger et al., 2011). Ine one study, immunotherapy plus dasatinib treatment in mice with liver metastases from colorectal cancer significantly increased immune cell infiltration into the tumour, thereby enhancing antitumour immunity (Kadota et al., 2022). Chemotherapy combined with dasatinib is also significantly more effective in the treatment of tumours than chemotherapy alone (Ma et al., 2019; Wang et al., 2022). However, Fyn is still a difficult target. However, whether Fyn promotes malignancy in all tumour types is unclear. Due to its strong similarities with other Src family kinases and its widespread expression throughout the body, targeted therapy may have unanticipated and unwanted off-target consequences. To improve the prognosis of cancer patients, more research is necessary to understand the activation and inactivation of Fyn as well as its mode of action in other cancers.
Author contributions
CX: Writing–original draft. YT: Writing–review and editing. XL: Writing–review and editing. RC: Supervision, Writing–review and editing.
Funding
The author(s) declare that financial support was received for the research, authorship, and/or publication of this article. This work was supported by grants from the Sichuan Science and Technology Program (2022NSFSC1315) and the National Natural Science Foundation of China (82301478).
Conflict of interest
The authors declare that the research was conducted in the absence of any commercial or financial relationships that could be construed as a potential conflict of interest.
Publisher’s note
All claims expressed in this article are solely those of the authors and do not necessarily represent those of their affiliated organizations, or those of the publisher, the editors and the reviewers. Any product that may be evaluated in this article, or claim that may be made by its manufacturer, is not guaranteed or endorsed by the publisher.
References
Aarts, M., Liu, Y., Liu, L., Besshoh, S., Arundine, M., Gurd, J. W., et al. (2002). Treatment of ischemic brain damage by perturbing NMDA receptor- PSD-95 protein interactions. Science 298, 846–850. doi:10.1126/science.1072873
Abram, C. L., and Lowell, C. A. (2008). The diverse functions of Src family kinases in macrophages. Front. Biosci. 13, 4426–4450. doi:10.2741/3015
Ahluwalia, M. S., de Groot, J., Liu, W. M., and Gladson, C. L. (2010). Targeting SRC in glioblastoma tumors and brain metastases: rationale and preclinical studies. Cancer Lett. 298, 139–149. doi:10.1016/j.canlet.2010.08.014
Airiau, K., Turcq, B., Mahon, F. X., and Belloc, F. (2017). A new mechanism of resistance to ABL1 tyrosine kinase inhibitors in a BCR-ABL1-positive cell line. Leuk. Res. 61, 44–52. doi:10.1016/j.leukres.2017.08.014
Alland, L., Peseckis, S. M., Atherton, R. E., Berthiaume, L., and Resh, M. D. (1994). Dual myristylation and palmitylation of Src family member p59fyn affects subcellular localization. J. Biol. Chem. 269, 16701–16705. doi:10.1016/s0021-9258(19)89447-4
Belkadi, A., and LoPresti, P. (2008). Truncated Tau with the Fyn-binding domain and without the microtubule-binding domain hinders the myelinating capacity of an oligodendrocyte cell line. J. Neurochem. 107, 351–360. doi:10.1111/j.1471-4159.2008.05600.x
Bourgin, C., Murai, K. K., Richter, M., and Pasquale, E. B. (2007). The EphA4 receptor regulates dendritic spine remodeling by affecting beta1-integrin signaling pathways. J. Cell Biol. 178, 1295–1307. doi:10.1083/jcb.200610139
Bray, F., Laversanne, M., Sung, H., Ferlay, J., Siegel, R. L., Soerjomataram, I., et al. (2024). Global cancer statistics 2022: GLOBOCAN estimates of incidence and mortality worldwide for 36 cancers in 185 countries. CA Cancer J. Clin. 74, 229–263. doi:10.3322/caac.21834
Cain, D. P., Grant, S. G., Saucier, D., Hargreaves, E. L., and Kandel, E. R. (1995). Fyn tyrosine kinase is required for normal amygdala kindling. Epilepsy Res. 22, 107–114. doi:10.1016/0920-1211(95)00029-1
Cary, L. A., Chang, J. F., and Guan, J. L. (1996). Stimulation of cell migration by overexpression of focal adhesion kinase and its association with Src and Fyn. J. Cell Sci. 109 (Pt 7), 1787–1794. doi:10.1242/jcs.109.7.1787
Chapman, N. M., Yoder, A. N., and Houtman, J. C. (2012). Non-catalytic functions of Pyk2 and Fyn regulate late stage adhesion in human T cells. PLoS One 7, e53011. doi:10.1371/journal.pone.0053011
Chougule, R. A., Kazi, J. U., and Rönnstrand, L. (2016). FYN expression potentiates FLT3-ITD induced STAT5 signaling in acute myeloid leukemia. Oncotarget 7, 9964–9974. doi:10.18632/oncotarget.7128
Comba, A., Dunn, P. J., Argento, A. E., Kadiyala, P., Ventosa, M., Patel, P., et al. (2020). Fyn tyrosine kinase, a downstream target of receptor tyrosine kinases, modulates antiglioma immune responses. Neuro Oncol. 22, 806–818. doi:10.1093/neuonc/noaa006
Czech, D. A., and Vander Zanden, J. M. (1991). Drinking behavior in the spiny mouse (Acomys cahirinus) following putative dipsogenic challenges. Pharmacol. Biochem. Behav. 38, 913–916. doi:10.1016/0091-3057(91)90263-2
Davidson, D., Fournel, M., and Veillette, A. (1994). Oncogenic activation of p59fyn tyrosine protein kinase by mutation of its carboxyl-terminal site of tyrosine phosphorylation, tyrosine 528. J. Biol. Chem. 269, 10956–10963. doi:10.1016/s0021-9258(17)34150-9
Dey, N., Crosswell, H. E., De, P., Parsons, R., Peng, Q., Su, J. D., et al. (2008). The protein phosphatase activity of PTEN regulates SRC family kinases and controls glioma migration. Cancer Res. 68, 1862–1871. doi:10.1158/0008-5472.CAN-07-1182
Dong, W., Sun, S. J., Qin, J. J., and Liu, G. M. (2020). Fyn stimulates the progression of pancreatic cancer via Fyn-GluN2b-AKT axis. Eur. Rev. Med. Pharmacol. Sci. 24, 109–121. doi:10.26355/eurrev_202001_19900
Elias, D., Vever, H., Lænkholm, A. V., Gjerstorff, M. F., Yde, C. W., Lykkesfeldt, A. E., et al. (2015). Gene expression profiling identifies FYN as an important molecule in tamoxifen resistance and a predictor of early recurrence in patients treated with endocrine therapy. Oncogene 34, 1919–1927. doi:10.1038/onc.2014.138
Ergün, S., Akgün, O., Hekim, N. T., Aslan, S., Ari, F., Güneş, S., et al. (2023). The interrelationship between FYN and miR-128/193a-5p/494 in imatinib resistance in prostate cancer. Anticancer Agents Med. Chem. 23, 360–365. doi:10.2174/1871520622666220601093452
Eskilsson, E., Rosland, G. V., Talasila, K. M., Knappskog, S., Keunen, O., Sottoriva, A., et al. (2016). EGFRvIII mutations can emerge as late and heterogenous events in glioblastoma development and promote angiogenesis through Src activation. Neuro Oncol. 18, 1644–1655. doi:10.1093/neuonc/now113
Fenouille, N., Puissant, A., Dufies, M., Robert, G., Jacquel, A., Ohanna, M., et al. (2010). Persistent activation of the Fyn/ERK kinase signaling axis mediates imatinib resistance in chronic myelogenous leukemia cells through upregulation of intracellular SPARC. Cancer Res. 70, 9659–9670. doi:10.1158/0008-5472.CAN-10-2034
Fenton, S. E., Hutchens, K. A., and Denning, M. F. (2015). Targeting Fyn in Ras-transformed cells induces F-actin to promote adherens junction-mediated cell‒cell adhesion. Mol. Carcinog. 54, 1181–1193. doi:10.1002/mc.22190
Frame, M. C. (2002). Src in cancer: deregulation and consequences for cell behaviour. Biochim. Biophys. Acta 1602, 114–130. doi:10.1016/s0304-419x(02)00040-9
Gang, E. J., Kim, H. N., Hsieh, Y. T., Ruan, Y., Ogana, H. A., Lee, S., et al. (2020). Integrin α6 mediates the drug resistance of acute lymphoblastic B-cell leukemia. Blood 136, 210–223. doi:10.1182/blood.2019001417
Gerbec, Z. J., Thakar, M. S., and Malarkannan, S. (2015). The fyn-ADAP Axis: cytotoxicity versus cytokine production in killer cells. Front. Immunol. 6, 472. doi:10.3389/fimmu.2015.00472
Giovannoni, F., and Quintana, F. J. (2020). The role of astrocytes in CNS inflammation. Trends Immunol. 41, 805–819. doi:10.1016/j.it.2020.07.007
Goldsmith, J. F., Hall, C. G., and Atkinson, T. P. (2002). Identification of an alternatively spliced isoform of the fyn tyrosine kinase. Biochem. Biophys. Res. Commun. 298, 501–504. doi:10.1016/s0006-291x(02)02510-x
Gourmaud, S., Shou, H., Irwin, D. J., Sansalone, K., Jacobs, L. M., Lucas, T. H., et al. (2020). Alzheimer-like amyloid and tau alterations associated with cognitive deficit in temporal lobe epilepsy. Brain 143, 191–209. doi:10.1093/brain/awz381
Grant, S. G., O'Dell, T. J., Karl, K. A., Stein, P. L., Soriano, P., and Kandel, E. R. (1992). Impaired long-term potentiation, spatial learning, and hippocampal development in fyn mutant mice. Science 258, 1903–1910. doi:10.1126/science.1361685
Grosso, S., Puissant, A., Dufies, M., Colosetti, P., Jacquel, A., Lebrigand, K., et al. (2009). Gene expression profiling of imatinib and PD166326-resistant CML cell lines identifies Fyn as a gene associated with resistance to BCR-ABL inhibitors. Mol. Cancer Ther. 8, 1924–1933. doi:10.1158/1535-7163.MCT-09-0168
Gururajan, M., Cavassani, K. A., Sievert, M., Duan, P., Lichterman, J., Huang, J. M., et al. (2015). SRC family kinase FYN promotes the neuroendocrine phenotype and visceral metastasis in advanced prostate cancer. Oncotarget 6, 44072–44083. doi:10.18632/oncotarget.6398
Hatcher, A., Yu, K., Meyer, J., Aiba, I., Deneen, B., and Noebels, J. L. (2020). Pathogenesis of peritumoral hyperexcitability in an immunocompetent CRISPR-based glioblastoma model. J. Clin. Invest 130, 2286–2300. doi:10.1172/JCI133316
Irwin, M. E., Johnson, B. P., Manshouri, R., Amin, H. M., and Chandra, J. (2015). A NOX2/Egr-1/Fyn pathway delineates new targets for TKI-resistant malignancies. Oncotarget 6, 23631–23646. doi:10.18632/oncotarget.4604
Ittner, L. M., Ke, Y. D., Delerue, F., Bi, M., Gladbach, A., van Eersel, J., et al. (2010). Dendritic function of tau mediates amyloid-beta toxicity in Alzheimer's disease mouse models. Cell 142, 387–397. doi:10.1016/j.cell.2010.06.036
Je, D. W., O, Y. M., Ji, Y. G., Cho, Y., and Lee, D. H. (2014). The inhibition of SRC family kinase suppresses pancreatic cancer cell proliferation, migration, and invasion. Pancreas 43, 768–776. doi:10.1097/MPA.0000000000000103
Jia, W., Feng, Y. I., Sanders, A. J., Davies, E. L., and Jiang, W. G. (2016). Phosphoinositide-3-Kinase enhancers, PIKEs: their biological functions and roles in cancer. Anticancer Res. 36, 1103–1109.
Joshi, T., Elias, D., Stenvang, J., Alves, C. L., Teng, F., Lyng, M. B., et al. (2016). Integrative analysis of miRNA and gene expression reveals regulatory networks in tamoxifen-resistant breast cancer. Oncotarget 7, 57239–57253. doi:10.18632/oncotarget.11136
Kadota, H., Yuge, R., Shimizu, D., Miyamoto, R., Otani, R., Hiyama, Y., et al. (2022). Anti-programmed cell death-1 antibody and dasatinib combination therapy exhibits efficacy in metastatic colorectal cancer mouse models. Cancers (Basel) 14, 6146. doi:10.3390/cancers14246146
Kalia, L. V., and Salter, M. W. (2003). Interactions between Src family protein tyrosine kinases and PSD-95. Neuropharmacology 45, 720–728. doi:10.1016/s0028-3908(03)00313-7
Kaufman, A. C., Salazar, S. V., Haas, L. T., Yang, J., Kostylev, M. A., Jeng, A. T., et al. (2015). Fyn inhibition rescues established memory and synapse loss in Alzheimer mice. Ann. Neurol. 77, 953–971. doi:10.1002/ana.24394
Kim, H., Strong, T. V., and Anderson, S. J. (1991). Evidence for tau expression in cells of monocyte lineage and its in vitro phosphorylation by v-fms kinase. Oncogene 6, 1085–1087.
Kim, H. J., Warren, J. T., Kim, S. Y., Chappel, J. C., DeSelm, C. J., Ross, F. P., et al. (2010). Fyn promotes proliferation, differentiation, survival and function of osteoclast lineage cells. J. Cell Biochem. 111, 1107–1113. doi:10.1002/jcb.22841
Kim, H. S., Chang, C. Y., Yoon, H. J., Kim, K. S., Koh, H. S., Kim, S. S., et al. (2020). Glial TIM-3 modulates immune responses in the brain tumor microenvironment. Cancer Res. 80, 1833–1845. doi:10.1158/0008-5472.CAN-19-2834
Kim, S. U., and de Vellis, J. (2005). Microglia in health and disease. J. Neurosci. Res. 81, 302–313. doi:10.1002/jnr.20562
Kinsey, W. H. (2014). SRC-family tyrosine kinases in oogenesis, oocyte maturation and fertilization: an evolutionary perspective. Adv. Exp. Med. Biol. 759, 33–56. doi:10.1007/978-1-4939-0817-2_3
Kluger, H. M., Dudek, A. Z., McCann, C., Ritacco, J., Southard, N., Jilaveanu, L. B., et al. (2011). A phase 2 trial of dasatinib in advanced melanoma. Cancer 117, 2202–2208. doi:10.1002/cncr.25766
Ko, H. M., Lee, S. H., Bang, M., Kim, K. C., Jeon, S. J., Park, Y. M., et al. (2018). Tyrosine kinase Fyn regulates iNOS expression in LPS-stimulated astrocytes via modulation of ERK phosphorylation. Biochem. Biophys. Res. Commun. 495, 1214–1220. doi:10.1016/j.bbrc.2017.11.143
Kojima, N., Ishibashi, H., Obata, K., and Kandel, E. R. (1998). Higher seizure susceptibility and enhanced tyrosine phosphorylation of N-methyl-D-aspartate receptor subunit 2B in fyn transgenic mice. Learn Mem. 5, 429–445. doi:10.1101/lm.5.6.429
Kojima, N., Wang, J., Mansuy, I. M., Grant, S. G., Mayford, M., and Kandel, E. R. (1997). Rescuing impairment of long-term potentiation in fyn-deficient mice by introducing Fyn transgene. Proc. Natl. Acad. Sci. U. S. A. 94, 4761–4765. doi:10.1073/pnas.94.9.4761
Larsen, S. L., Laenkholm, A. V., Duun-Henriksen, A. K., Bak, M., Lykkesfeldt, A. E., and Kirkegaard, T. (2015). SRC drives growth of antiestrogen resistant breast cancer cell lines and is a marker for reduced benefit of tamoxifen treatment. PLoS One 10, e0118346. doi:10.1371/journal.pone.0118346
Larson, M., Sherman, M. A., Amar, F., Nuvolone, M., Schneider, J. A., Bennett, D. A., et al. (2012). The complex PrP(c)-Fyn couples human oligomeric Aβ with pathological tau changes in Alzheimer's disease. J. Neurosci. 32, 16857–71a. doi:10.1523/JNEUROSCI.1858-12.2012
Lee, C., Low, C. Y., Wong, S. Y., Lai, M. K., and Tan, M. G. (2017). Selective induction of alternatively spliced FynT isoform by TNF facilitates persistent inflammatory responses in astrocytes. Sci. Rep. 7, 43651. doi:10.1038/srep43651
Lee, G., Newman, S. T., Gard, D. L., Band, H., and Panchamoorthy, G. (1998). Tau interacts with src-family non-receptor tyrosine kinases. J. Cell Sci. 111 (Pt 21), 3167–3177. doi:10.1242/jcs.111.21.3167
Lee, G., Thangavel, R., Sharma, V. M., Litersky, J. M., Bhaskar, K., Fang, S. M., et al. (2004). Phosphorylation of tau by fyn: implications for Alzheimer's disease. J. Neurosci. 24, 2304–2312. doi:10.1523/JNEUROSCI.4162-03.2004
Levi, M., Maro, B., and Shalgi, R. (2010). Fyn kinase is involved in cleavage furrow ingression during meiosis and mitosis. Reproduction 140, 827–834. doi:10.1530/REP-10-0312
Li, C., Li, W., Xiao, J., Jiao, S., Teng, F., Xue, S., et al. (2015). ADAP and SKAP55 deficiency suppresses PD-1 expression in CD8+ cytotoxic T lymphocytes for enhanced anti-tumor immunotherapy. EMBO Mol. Med. 7, 754–769. doi:10.15252/emmm.201404578
Liang, J., Lv, X., Lu, C., Ye, X., Chen, X., Fu, J., et al. (2020). Prognostic factors of patients with Gliomas - an analysis on 335 patients with Glioblastoma and other forms of Gliomas. BMC Cancer 20, 35. doi:10.1186/s12885-019-6511-6
Liu, D., Gao, M., Wu, K., Zhu, D., Yang, Y., and Zhao, S. (2019a). LINC00152 facilitates tumorigenesis in esophageal squamous cell carcinoma via miR-153-3p/FYN axis. Biomed. Pharmacother. 112, 108654. doi:10.1016/j.biopha.2019.108654
Liu, R., Li, W., Tao, B., Wang, X., Yang, Z., Zhang, Y., et al. (2019b). Tyrosine phosphorylation activates 6-phosphogluconate dehydrogenase and promotes tumor growth and radiation resistance. Nat. Commun. 10, 991. doi:10.1038/s41467-019-08921-8
Lowell, C. A., and Soriano, P. (1996). Knockouts of Src-family kinases: stiff bones, wimpy T cells, and bad memories. Genes Dev. 10, 1845–1857. doi:10.1101/gad.10.15.1845
Lu, K. V., Zhu, S., Cvrljevic, A., Huang, T. T., Sarkaria, S., Ahkavan, D., et al. (2009). Fyn and SRC are effectors of oncogenic epidermal growth factor receptor signaling in glioblastoma patients. Cancer Res. 69, 6889–6898. doi:10.1158/0008-5472.CAN-09-0347
Ma, L., Wei, J., Su, G. H., and Lin, J. (2019). Dasatinib can enhance paclitaxel and gemcitabine inhibitory activity in human pancreatic cancer cells. Cancer Biol. Ther. 20, 855–865. doi:10.1080/15384047.2019.1579956
Macurek, L., Dráberová, E., Richterová, V., Sulimenko, V., Sulimenko, T., Dráberová, L., et al. (2008). Regulation of microtubule nucleation from membranes by complexes of membrane-bound gamma-tubulin with Fyn kinase and phosphoinositide 3-kinase. Biochem. J. 416, 421–430. doi:10.1042/BJ20080909
Malarkannan, S. (2020). Molecular mechanisms of FasL-mediated 'reverse-signaling. Mol. Immunol. 127, 31–37. doi:10.1016/j.molimm.2020.08.010
Menges, C. W., Chen, Y., Mossman, B. T., Chernoff, J., Yeung, A. T., and Testa, J. R. (2010). A phosphotyrosine proteomic screen identifies multiple tyrosine kinase signaling pathways aberrantly activated in malignant mesothelioma. Genes Cancer 1, 493–505. doi:10.1177/1947601910375273
Mi, H., Wang, X., Wang, F., Li, L., Zhu, M., Wang, N., et al. (2018). miR-381 induces sensitivity of breast cancer cells to doxorubicin by inactivation of MAPK signaling via FYN. Eur. J. Pharmacol. 839, 66–75. doi:10.1016/j.ejphar.2018.09.024
Nakazawa, T., Komai, S., Tezuka, T., Hisatsune, C., Umemori, H., Semba, K., et al. (2001). Characterization of Fyn-mediated tyrosine phosphorylation sites on GluR epsilon 2 (NR2B) subunit of the N-methyl-D-aspartate receptor. J. Biol. Chem. 276, 693–699. doi:10.1074/jbc.M008085200
Ng, M. M., Chang, F., and Burgess, D. R. (2005). Movement of membrane domains and requirement of membrane signaling molecules for cytokinesis. Dev. Cell 9, 781–790. doi:10.1016/j.devcel.2005.11.002
Ninio-Many, L., Grossman, H., Shomron, N., Chuderland, D., and Shalgi, R. (2013). microRNA-125a-3p reduces cell proliferation and migration by targeting Fyn. J. Cell Sci. 126, 2867–2876. doi:10.1242/jcs.123414
Okamoto, M., Nakayama, Y., Kakihana, A., Yuki, R., Yamaguchi, N., and Yamaguchi, N. (2016). Fyn accelerates M phase progression by promoting the assembly of mitotic spindle microtubules. J. Cell Biochem. 117, 894–903. doi:10.1002/jcb.25373
Osterhout, D. J., Wolven, A., Wolf, R. M., Resh, M. D., and Chao, M. V. (1999). Morphological differentiation of oligodendrocytes requires activation of Fyn tyrosine kinase. J. Cell Biol. 145, 1209–1218. doi:10.1083/jcb.145.6.1209
Panicker, N., Saminathan, H., Jin, H., Neal, M., Harischandra, D. S., Gordon, R., et al. (2015). Fyn kinase regulates microglial neuroinflammatory responses in cell culture and animal models of Parkinson's disease. J. Neurosci. 35, 10058–10077. doi:10.1523/JNEUROSCI.0302-15.2015
Poonan, P., Agoni, C., Ibrahim, M. A. A., and Soliman, M. E. S. (2021). Glioma-targeted therapeutics: computer-aided drug design prospective. Protein J. 40, 601–655. doi:10.1007/s10930-021-10021-w
Posadas, E. M., Ahmed, R. S., Karrison, T., Szmulewitz, R. Z., O'Donnell, P. H., Wade, J. L., et al. (2016). Saracatinib as a metastasis inhibitor in metastatic castration-resistant prostate cancer: a university of Chicago phase 2 consortium and dod/PCF prostate cancer clinical trials consortium study. Prostate 76, 286–293. doi:10.1002/pros.23119
Putra, M., Puttachary, S., Liu, G., Lee, G., and Thippeswamy, T. (2020). Fyn-tau ablation modifies PTZ-induced seizures and post-seizure hallmarks of early epileptogenesis. Front. Cell Neurosci. 14, 592374. doi:10.3389/fncel.2020.592374
Putra, M., Rao, N. S., Gardner, C., Liu, G., Trommater, J., Bunney, M., et al. (2024). Enhanced Fyn-tau and NR2B-PSD95 interactions in epileptic foci in experimental models and human epilepsy. Brain Commun. 6, fcae327. doi:10.1093/braincomms/fcae327
Reddy, K. B., Smith, D. M., and Plow, E. F. (2008). Analysis of Fyn function in hemostasis and alphaIIbbeta3-integrin signaling. J. Cell Sci. 121, 1641–1648. doi:10.1242/jcs.014076
Revah, O., Stoler, O., Neef, A., Wolf, F., Fleidervish, I. A., and Gutnick, M. J. (2019). Dynamic gain analysis reveals encoding deficiencies in cortical neurons that recover from hypoxia-induced spreading depolarizations. J. Neurosci. 39, 7790–7800. doi:10.1523/JNEUROSCI.3147-18.2019
Roberson, E. D., Halabisky, B., Yoo, J. W., Yao, J., Chin, J., Yan, F., et al. (2011). Amyloid-β/Fyn-induced synaptic, network, and cognitive impairments depend on tau levels in multiple mouse models of Alzheimer's disease. J. Neurosci. 31, 700–711. doi:10.1523/JNEUROSCI.4152-10.2011
Robert, S. M., Buckingham, S. C., Campbell, S. L., Robel, S., Holt, K. T., Ogunrinu-Babarinde, T., et al. (2015). SLC7A11 expression is associated with seizures and predicts poor survival in patients with malignant glioma. Sci. Transl. Med. 7, 289ra86. doi:10.1126/scitranslmed.aaa8103
Rong, Y., Lu, X., Bernard, A., Khrestchatisky, M., and Baudry, M. (2001). Tyrosine phosphorylation of ionotropic glutamate receptors by Fyn or Src differentially modulates their susceptibility to calpain and enhances their binding to spectrin and PSD-95. J. Neurochem. 79, 382–390. doi:10.1046/j.1471-4159.2001.00565.x
Roth, J. R., Rush, T., Thompson, S. J., Aldaher, A. R., Dunn, T. B., Mesina, J. S., et al. (2024). Development of small-molecule Tau-SH3 interaction inhibitors that prevent amyloid-β toxicity and network hyperexcitability. Neurotherapeutics 21, e00291. doi:10.1016/j.neurot.2023.10.001
Rush, T., Roth, J. R., Thompson, S. J., Aldaher, A. R., Cochran, J. N., and Roberson, E. D. (2020). A peptide inhibitor of Tau-SH3 interactions ameliorates amyloid-β toxicity. Neurobiol. Dis. 134, 104668. doi:10.1016/j.nbd.2019.104668
Salter, M. W., and Kalia, L. V. (2004). Src kinases: a hub for NMDA receptor regulation. Nat. Rev. Neurosci. 5, 317–328. doi:10.1038/nrn1368
Sangrajrang, S., Denoulet, P., Millot, G., Tatoud, R., Podgorniak, M. P., Tew, K. D., et al. (1998). Estramustine resistance correlates with tau over-expression in human prostatic carcinoma cells. Int. J. Cancer 77, 626–631. doi:10.1002/(sici)1097-0215(19980812)77:4<626::aid-ijc24>3.0.co;2-2
Sarkar, S., Nguyen, H. M., Malovic, E., Luo, J., Langley, M., Palanisamy, B. N., et al. (2020). Kv1.3 modulates neuroinflammation and neurodegeneration in Parkinson's disease. J. Clin. Invest 130, 4195–4212. doi:10.1172/JCI136174
Semba, K., Nishizawa, M., Miyajima, N., Yoshida, M. C., Sukegawa, J., Yamanashi, Y., et al. (1986). yes-related protooncogene, syn, belongs to the protein-tyrosine kinase family. Proc. Natl. Acad. Sci. U. S. A. 83, 5459–5463. doi:10.1073/pnas.83.15.5459
Sette, C., Paronetto, M. P., Barchi, M., Bevilacqua, A., Geremia, R., and Rossi, P. (2002). Tr-kit-induced resumption of the cell cycle in mouse eggs requires activation of a Src-like kinase. EMBO J. 21, 5386–5395. doi:10.1093/emboj/cdf553
Sharma, S., Carlson, S., Gregory-Flores, A., Hinojo-Perez, A., Olson, A., and Thippeswamy, T. (2021). Mechanisms of disease-modifying effect of saracatinib (AZD0530), a Src/Fyn tyrosine kinase inhibitor, in the rat kainate model of temporal lobe epilepsy. Neurobiol. Dis. 156, 105410. doi:10.1016/j.nbd.2021.105410
Sharma, S., Carlson, S., Puttachary, S., Sarkar, S., Showman, L., Putra, M., et al. (2018). Role of the Fyn-PKCδ signaling in SE-induced neuroinflammation and epileptogenesis in experimental models of temporal lobe epilepsy. Neurobiol. Dis. 110, 102–121. doi:10.1016/j.nbd.2017.11.008
Shirazi, S. K., and Wood, J. G. (1993). The protein tyrosine kinase, fyn, in Alzheimer's disease pathology. Neuroreport 4, 435–437. doi:10.1097/00001756-199304000-00024
Sillaber, C., Herrmann, H., Bennett, K., Rix, U., Baumgartner, C., Böhm, A., et al. (2009). Immunosuppression and atypical infections in CML patients treated with dasatinib at 140 mg daily. Eur. J. Clin. Invest 39, 1098–1109. doi:10.1111/j.1365-2362.2009.02206.x
Singh, M. M., Howard, A., Irwin, M. E., Gao, Y., Lu, X., Multani, A., et al. (2012). Expression and activity of Fyn mediate proliferation and blastic features of chronic myelogenous leukemia. PLoS One 7, e51611. doi:10.1371/journal.pone.0051611
Sørensen, M. F., Heimisdóttir, S. B., Sørensen, M. D., Mellegaard, C. S., Wohlleben, H., Kristensen, B. W., et al. (2018). High expression of cystine-glutamate antiporter xCT (SLC7A11) is an independent biomarker for epileptic seizures at diagnosis in glioma. J. Neurooncol 138, 49–53. doi:10.1007/s11060-018-2785-9
Sperber, B. R., Boyle-Walsh, E. A., Engleka, M. J., Gadue, P., Peterson, A. C., Stein, P. L., et al. (2001). A unique role for Fyn in CNS myelination. J. Neurosci. 21, 2039–2047. doi:10.1523/JNEUROSCI.21-06-02039.2001
Sugie, K., Jeon, M. S., and Grey, H. M. (2004). Activation of naïve CD4 T cells by anti-CD3 reveals an important role for Fyn in Lck-mediated signaling. Proc. Natl. Acad. Sci. U. S. A. 101, 14859–14864. doi:10.1073/pnas.0406168101
Sun, M., Sheng, H., Wu, T., Song, J., Sun, H., Wang, Y., et al. (2021). PIKE-A promotes glioblastoma growth by driving PPP flux through increasing G6PD expression mediated by phosphorylation of STAT3. Biochem. Pharmacol. 192, 114736. doi:10.1016/j.bcp.2021.114736
Tang, L., Long, J., Li, K., Zhang, X., Chen, X., and Peng, C. (2020). A novel chalcone derivative suppresses melanoma cell growth through targeting Fyn/Stat3 pathway. Cancer Cell Int. 20, 256. doi:10.1186/s12935-020-01336-2
Tezuka, T., Umemori, H., Akiyama, T., Nakanishi, S., and Yamamoto, T. (1999). PSD-95 promotes Fyn-mediated tyrosine phosphorylation of the N-methyl-D-aspartate receptor subunit NR2A. Proc. Natl. Acad. Sci. U. S. A. 96, 435–440. doi:10.1073/pnas.96.2.435
Thom, M., Liu, J. Y., Thompson, P., Phadke, R., Narkiewicz, M., Martinian, L., et al. (2011). Neurofibrillary tangle pathology and Braak staging in chronic epilepsy in relation to traumatic brain injury and hippocampal sclerosis: a post-mortem study. Brain 134, 2969–2981. doi:10.1093/brain/awr209
Thomas, S. M., and Brugge, J. S. (1997). Cellular functions regulated by Src family kinases. Annu. Rev. Cell Dev. Biol. 13, 513–609. doi:10.1146/annurev.cellbio.13.1.513
Tominaga, T., Sahai, E., Chardin, P., McCormick, F., Courtneidge, S. A., and Alberts, A. S. (2000). Diaphanous-related formins bridge Rho GTPase and Src tyrosine kinase signaling. Mol. Cell 5, 13–25. doi:10.1016/s1097-2765(00)80399-8
van Breemen, M. S., Wilms, E. B., and Vecht, C. J. (2007). Epilepsy in patients with brain tumours: epidemiology, mechanisms, and management. Lancet Neurol. 6, 421–430. doi:10.1016/S1474-4422(07)70103-5
van Kessel, E., Baumfalk, A. E., van Zandvoort, M. J. E., Robe, P. A., and Snijders, T. J. (2017). Tumor-related neurocognitive dysfunction in patients with diffuse glioma: a systematic review of neurocognitive functioning prior to anti-tumor treatment. J. Neurooncol 134, 9–18. doi:10.1007/s11060-017-2503-z
Wang, H., Lu, Y., Wang, M., Shen, A., Wu, Y., Xu, X., et al. (2022). Src inhibitor dasatinib sensitized gastric cancer cells to cisplatin. Med. Oncol. 40, 49. doi:10.1007/s12032-022-01879-6
Wolf, Y., Anderson, A. C., and Kuchroo, V. K. (2020). TIM3 comes of age as an inhibitory receptor. Nat. Rev. Immunol. 20, 173–185. doi:10.1038/s41577-019-0224-6
Wu, N., Song, H., and Veillette, A. (2021). Plasma membrane lipid scrambling causing phosphatidylserine exposure negatively regulates NK cell activation. Cell Mol. Immunol. 18, 686–697. doi:10.1038/s41423-020-00600-9
Wu, X., Rauch, T. A., Zhong, X., Bennett, W. P., Latif, F., Krex, D., et al. (2010). CpG island hypermethylation in human astrocytomas. Cancer Res. 70, 2718–2727. doi:10.1158/0008-5472.CAN-09-3631
Xu, Y., Jin, M. Z., Yang, Z. Y., and Jin, W. L. (2021). Microglia in neurodegenerative diseases. Neural Regen. Res. 16, 270–280. doi:10.4103/1673-5374.290881
Yan, J., Fu, Q., Cheng, L., Zhai, M., Wu, W., Huang, L., et al. (2014). Inflammatory response in Parkinson's disease (Review). Mol. Med. Rep. 10, 2223–2233. doi:10.3892/mmr.2014.2563
Yasunaga, M., Yagi, T., Hanzawa, N., Yasuda, M., Yamanashi, Y., Yamamoto, T., et al. (1996). Involvement of Fyn tyrosine kinase in progression of cytokinesis of B lymphocyte progenitor. J. Cell Biol. 132, 91–99. doi:10.1083/jcb.132.1.91
Yu, B., Xu, L., Chen, L., Wang, Y., Jiang, H., Wang, Y., et al. (2020a). FYN is required for ARHGEF16 to promote proliferation and migration in colon cancer cells. Cell Death Dis. 11, 652. doi:10.1038/s41419-020-02830-1
Yu, K., Lin, C. J., Hatcher, A., Lozzi, B., Kong, K., Huang-Hobbs, E., et al. (2020b). PIK3CA variants selectively initiate brain hyperactivity during gliomagenesis. Nature 578, 166–171. doi:10.1038/s41586-020-1952-2
Yun, H. S., Lee, J., Kil, W. J., Kramp, T. R., Tofilon, P. J., and Camphausen, K. (2021). The radiosensitizing effect of AZD0530 in glioblastoma and glioblastoma stem-like cells. Mol. Cancer Ther. 20, 1672–1679. doi:10.1158/1535-7163.MCT-20-0883
Zamoyska, R., Basson, A., Filby, A., Legname, G., Lovatt, M., and Seddon, B. (2003). The influence of the src-family kinases, Lck and Fyn, on T cell differentiation, survival and activation. Immunol. Rev. 191, 107–118. doi:10.1034/j.1600-065x.2003.00015.x
Zhang, S., Qi, Q., Chan, C. B., Zhou, W., Chen, J., Luo, H. R., et al. (2016). Fyn-phosphorylated PIKE-A binds and inhibits AMPK signaling, blocking its tumor suppressive activity. Cell Death Differ. 23, 52–63. doi:10.1038/cdd.2015.66
Zhang, S., Sheng, H., Zhang, X., Qi, Q., Chan, C. B., Li, L., et al. (2019). Cellular energy stress induces AMPK-mediated regulation of glioblastoma cell proliferation by PIKE-A phosphorylation. Cell Death Dis. 10, 222. doi:10.1038/s41419-019-1452-1
Zhao, L., Li, W., Marshall, C., Griffin, T., Hanson, M., Hick, R., et al. (2009). Srcasm inhibits Fyn-induced cutaneous carcinogenesis with modulation of Notch1 and p53. Cancer Res. 69, 9439–9447. doi:10.1158/0008-5472.CAN-09-2976
Keywords: Fyn, brain tumours, Src family of kinases, gliomas, therapeutic target
Citation: Xu C, Tang Y, Lu X and Chen R (2024) Fyn, an important molecule in the brain, is a potential therapeutic target for brain tumours. Front. Pharmacol. 15:1485919. doi: 10.3389/fphar.2024.1485919
Received: 25 August 2024; Accepted: 22 November 2024;
Published: 04 December 2024.
Edited by:
Arturo Mangas, University of Salamanca, SpainReviewed by:
Marson Putra, Iowa State University, United StatesCopyright © 2024 Xu, Tang, Lu and Chen. This is an open-access article distributed under the terms of the Creative Commons Attribution License (CC BY). The use, distribution or reproduction in other forums is permitted, provided the original author(s) and the copyright owner(s) are credited and that the original publication in this journal is cited, in accordance with accepted academic practice. No use, distribution or reproduction is permitted which does not comply with these terms.
*Correspondence: Ruiqi Chen, cnVpcWljaGVuMDFAMTYzLmNvbQ==