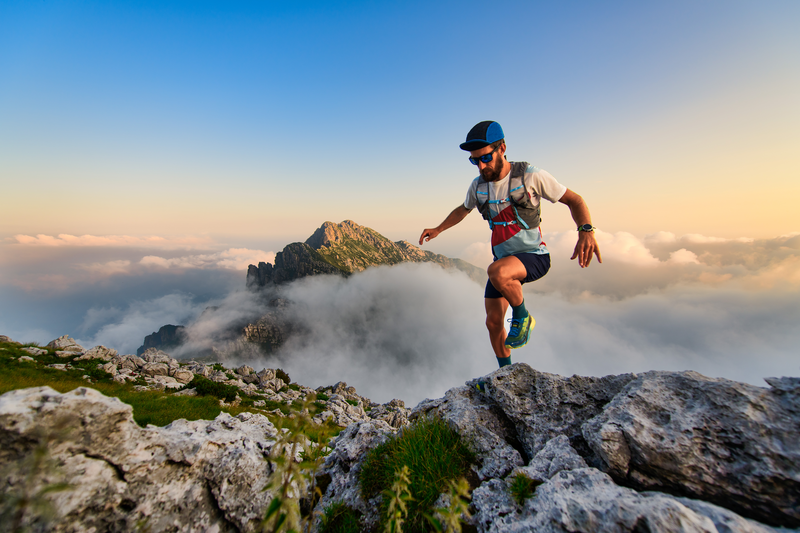
94% of researchers rate our articles as excellent or good
Learn more about the work of our research integrity team to safeguard the quality of each article we publish.
Find out more
ORIGINAL RESEARCH article
Front. Pharmacol. , 20 November 2024
Sec. Drugs Outcomes Research and Policies
Volume 15 - 2024 | https://doi.org/10.3389/fphar.2024.1485190
Objective: Hypertension is a leading global risk factor for disability and death. Irbesartan, a potent angiotensin II receptor blocker, requires continuous safety monitoring. We conducted a disproportionality analysis of irbesartan-related adverse drug events (ADEs) using the FDA’s FAERS and Japan’s JADER databases.
Methods: We extracted irbesartan-related ADE reports from FAERS (Q1 2004 to Q1 2024) and JADER (Q2 2008 to Q4 2023). We used Reporting Odds Ratio (ROR), Proportional Reporting Ratio (PRR), Bayesian Confidence Propagation Neural Network (BCPNN), and Empirical Bayesian Geometric Mean (EBGM) for signal detection. Sensitivity analyses were conducted to exclude comorbid medications, and subgroup analyses by age and gender were performed to explore ADE occurrence in specific populations. Th time to onset (TTO) of ADEs was assessed using Weibull distribution test and Kaplan-Meier curves.
Results: A total of 5,816 (FAERS) and 366 (JADER) reports were analyzed, with irbesartan-related preferred terms (PTs) involving 27 System Organ Classes (SOCs) in FAERS and 22 in JADER. Three SOCs met detection thresholds in both databases: “metabolism and nutrition disorders,” “cardiac disorders,” and “renal and urinary disorders.” We identified 219 positive signals in FAERS and 20 in JADER, including known signals like hyperkalemia, hypotension, and acute kidney injury. Notably, newly identified signals such as acute pancreatitis (n = 50, ROR: 7.76 [5.88–10.25]) and rhabdomyolysis (n = 50, ROR: 7.76 [5.88–10.25]) in FAERS and respiratory failure (n = 7, ROR: 6.76 [3.20–14.26]) in JADER could have significant clinical implications, as they may lead to severe outcomes if not recognized and managed promptly. Subgroup analyses revealed both similarities and differences in signal detection across gender and age groups. Sensitivity analyses, excluding concomitant medications, confirmed the persistence of key positive signals, including hyperkalemia, angioedema, acute pancreatitis, and agranulocytosis. ADEs mainly occurred within 1 month (34.14%) and after 1 year (32.32%) after dosing, with a median onset of 107 days.
Conclusion: This study provides valuable real-world evidence on the safety profile of irbesartan. The identification of new safety signals underscores the necessity of updating drug labels, particularly for assessing and managing high-risk patients. Additionally, the TTO analysis emphasizes the importance of sustained vigilance for adverse events over time. In conclusion, our findings contribute to a more comprehensive understanding of irbesartan’s safety, aiding healthcare professionals in optimizing its use in clinical practice.
Hypertension, characterized by a sustained systolic blood pressure (SBP) of at least 130 mm Hg or diastolic blood pressure (DBP) of at least 80 mm Hg, significantly elevates the risk of cardiovascular disease events, including coronary heart disease, heart failure, stroke (Cai and Li, 2022), myocardial infarction, and atrial fibrillation, leading to increased mortality and disability worldwide (Carey et al., 2022). Over the past 50 years, advances in pharmacological therapy have significantly improved the safety and efficacy of antihypertensive drugs, particularly those targeting the renin-angiotensin system (RAS), such as angiotensin-converting enzyme inhibitors (ACEIs), angiotensin II receptor blockers (ARBs), and aldosterone antagonists like spironolactone (Cai and Li, 2022; Warwick et al., 2015; Zhou et al., 2021; Robles et al., 2014; Song et al., 2024). Irbesartan, a long-acting angiotensin II type 1 receptor antagonist, is widely approved for hypertension treatment (Morales-Olivas et al., 2004). Irbesartan selectively antagonizes the angiotensin II type 1 (AT1) receptor, effectively inhibiting angiotensin II-induced vasoconstriction and aldosterone secretion. This mechanism promotes vasodilation, reduces sodium and water retention, and consequently lowers blood pressure. Irbesartan is rapidly absorbed orally, with 60%–80% bioavailability unaffected by food, reaching peak blood concentration within 1.5–2 h, and has a plasma protein binding rate of over 90%, primarily metabolized by the liver (Borghi and Cicero, 2012). Compared to enalapril, atenolol, and amlodipine, irbesartan demonstrates superior efficacy in absolute blood pressure reduction and remission rates (Mazzolai et al., 1999). Beyond blood pressure reduction, irbesartan also induces regression of left ventricular hypertrophy, slows the progression of kidney disease in hypertensive patients with type 2 diabetes mellitus, and improves diabetes-related atherosclerosis (Derosa et al., 2009; Chen C. et al., 2018).
Despite irbesartan’s pharmacological benefits, potential safety risks persist in its practical application. Major adverse events reported in epidemiological studies and premarket randomized clinical trials include headache, dizziness, gastric discomfort, skeletal muscle pain, and influenza, with incidence rates varying between 2% and 55% (Morales-Olivas et al., 2004; Kawano et al., 2008; Larochelle et al., 1997; Stumpe et al., 1998; Fogari et al., 1997; Kassler-Taub et al., 1998). In 1,779 patients treated with irbesartan in nine clinical trials, 7.7% reported adverse events within 24 h of the first dose, mainly headache, dizziness and fatigue, with elevated serum creatinine being the most common laboratory abnormality (Simon et al., 1998). A multicenter, randomized, double-blind study of 65-year-old patients with mild-to-moderate hypertension receiving irbesartan therapy showed headache (12.9%), dizziness (4.3%), and arthralgia (2.9%) as the most common non-serious adverse events (Lacourcière, 2000). Although rare, serious adverse effects include hypotension, hyperkalemia, and renal impairment (Hsu et al., 2017). Poor patient compliance due to adverse events remains a major barrier to successful hypertension treatment (Paz Ocaranza et al., 2020). As irbesartan use becomes more widespread, heightened awareness of its safety is essential, particularly for adverse events not explicitly mentioned in drug inserts.
Spontaneous reporting systems are critical for detecting adverse drug events (ADEs) not identified in clinical trials and for conducting safety assessments in specific populations and clinical settings (Noguchi et al., 2021). Recently, the U.S. Food and Drug Administration (FDA) Adverse Event Reporting System (FAERS) and the Japanese Adverse Drug Event Report (JADER) have collected numerous adverse event reports from diverse cohorts (Noguchi et al., 2021; Nomura et al., 2015). These databases are valuable for early detection and identification of potential ADEs, promoting ongoing monitoring and tracking through pharmacovigilance research (Edwards and Biriell, 1994). A study utilizing the WHO pharmacovigilance database (VigiBase), found that patients treated with ARBs reported diarrhea more frequently than those on ACEIs (Guion-Firmin et al., 2022). Additionally, an analysis from the EudraVigilance Data Analysis System (EVDAS) identified a potential association between ARBs, specifically valsartan, and a potential risk of neoplasms (Sharma et al., 2024). Although irbesartan’s safety has been evaluated in clinical trials, stringent inclusion criteria, limited sample sizes, and low incidence of serious ADEs may not capture the full range observed in widespread clinical use (Andersen and Jess, 2014; Luna et al., 2020). Moreover, significant gaps remain in post-marketing pharmacovigilance studies concerning irbesartan’s safety profile in real-world clinical practice. This study aims to address these gaps by providing critical insights into the drug’s safety, focusing on adverse events that may not have been captured in pre-approval trials. This pharmacovigilance study is the first to comprehensively quantify and visualize the safety profile of irbesartan using data from the FAERS and JADER databases, identify new safety signals not previously listed on the drug label, and estimate the timing of ADE occurrence. Our analysis provides valuable information for irbesartan’s clinical practice, guide treatment decisions, and ultimately protect patient health.
FAERS is a publicly accessible database that aggregates and summarizes adverse drug reaction reports from across the globe, providing a comprehensive view of ADE occurrences and facilitating post-marketing safety monitoring. It houses over 9 million individual drug-related adverse event reports submitted by industry professionals, physicians, pharmacists, healthcare professionals, consumers, and others, making it the largest spontaneous reporting system database worldwide (Okada et al., 2019; van Hasselt et al., 2020). The FAERS data is categorized into seven datasets: demographic and administrative information (DEMO), drug information (DRUG), adverse drug reaction information (REAC), patient outcomes information (OUCT), reported sources (RPSR), drug therapy start dates and end dates (THER), and indications for drug administration (INDI). A relationship is established within the FAERS database architecture that connects each data file through a unique identification number (Zhou et al., 2024). Detailed data from FAERS can be downloaded from the FDA website (http://www.fda.gov/).
The JADER database contains information on cases reported by pharmaceutical companies and medical institutions since 2004. The JADER database comprises four files: DEMO, DRUG, REAC, and HIST (Imai et al., 2022). The “DEMO” file includes basic patient information such as sex, age, and weight. The “DRUG” file contains the generic name of the drug, route of administration, and the start and end dates of administration. The “REAC” file records the name of the adverse event, outcome, and the date of occurrence. The “HIST” file contains information about the patient’s underlying condition (Inaba et al., 2019). Data from JADER were downloaded from the Pharmaceutical and Medical Devices Agency website (https://www.pmda.go.jp/index.html).
In our study, we utilized two spontaneous reporting system databases, FAERS and JADER, to mitigate reporting bias and enhance the reliability of the results. Considering the differing marketing times of irbesartan, the analysis period for this study spans from April 2004 to March 2024 in FAERS and from April 2008 to December 2023 in JADER. In FAERS, searches were conducted using generic and brand names such as “IRBESARTAN,” “APROVEL,” and “KARVEA.” In JADER, “イルベサルタン” was used for retrieval. Since FAERS updates quarterly, which may encompass duplicate reports or those that have been withdrawn or deleted, we performed deduplication following FDA recommendations to address duplicate reports submitted by different sources: In the DEMO file, we selected the PRIMARYIDs, CASEIDs, and FDA_DTs, subsequently sorting them by CASEIDs, FDA_DTs, and PRIMARYIDs. (1) If CASEIDs are the same, the latest FDA_DTs is selected; (2) If CASEIDs and FDA_DTs are the same, the higher PRIMARYIDs is selected (Wan et al., 2022). ADE reports associated with irbesartan use were extracted, standardized, and mapped to Medical Dictionary for Regulatory Activities (MedDRA 26.0) (Singh, 2015). The role code for ADEs was retained as the primary suspect (PS) by mitigating drug interactions (I), concomitant drugs (C), secondary suspect drugs, and other unknown drugs that may cause ADEs (Ji et al., 2022; Zhang et al., 2023). Based on MedDRA’s structural hierarchy, the screened ADEs were mapped into preferred terms (PTs) and system organ classes (SOCs). In FAERS, we conducted a statistical analysis of ADE reports, focusing on variables such as sex, age, weight, indication, reporting countries, outcome, and the reported person. During subgroup analyses, we excluded reports with missing data for sex and age. In the JADER database, we removed duplicate data from the DRUG and REAC files and linked the DEMO table to these tables based on the identified conditions (Kato et al., 2022). The drugs were classified into three categories—“PS,” “C,” and “I”—according to their impact on adverse events. Consistent with our approach in FAERS, we designated the role code as PS. Adverse events were coded following the terminology recommended in MedDRA, Japanese version 26.0 (Yamaoka et al., 2022). It is important to note that the DEMO file includes demographic information organized in 10-year age intervals (e.g., 60–69 years). We excluded missing sex data and non-numeric age categories, such as early, mid, late gestation, neonatal, infant, pediatric, young adult, adult, elderly, and unknown, from the subgroup analyses (Kose et al., 2023).
Considering the differences in reporting formats and data structures between FAERS and JADER, we employed the following methods to ensure the comparability of the analysis results:1: Uniform coding system: We utilized a standardized coding system for medical terminology (MedDRA 26.0) to mitigate bias in data analysis stemming from the differing database formats and to ensure the comparability of terms used for adverse reactions across both databases (Zou et al., 2024). 2: Removal of duplicate and erroneous records: We eliminated spontaneous reporting of duplicate or erroneous records within the databases to reduce inconsistencies and to enhance the completeness and credibility of the data analyses. 3: Bayesian algorithms: We employed Bayesian-based algorithms and empirical Bayesian geometric averaging, which effectively address variations in data by adapting to different distributions and structures, thereby generating consistent and reliable signals to ensure comparability of results (Ewers et al., 2012; Agrawal et al., 2019). 4: Timeframe selection: We selected the period following the FDA approval of Irbesartan (September 1997) and its approval in Japan (April 2008) for analysis to minimize bias associated with reporting during the clinical trial phase.
For pharmacovigilance, disproportionality analysis is commonly employed with post-marketing surveillance databases to assess associations between drugs and adverse events (Shen et al., 2019). This analysis compares the observed and expected number of reports for any given combination of drug and adverse event, generating hypotheses about possible causal relationships. In our study, we applied both Bayesian and frequency (non-Bayesian) methods to evaluate the relationship between irbesartan and ADEs. Frequency methods include Reporting Odds Ratio (ROR) (van Puijenbroek et al., 2002) and Proportional Reporting Ratio (PRR) (Evans et al., 2001). Bayesian methods encompass the Bayesian Confidence Propagation Neural Network (BCPNN) (Bate et al., 1998) and the Multi-Item Gamma Poisson Shrinker (MGPS) (Szarfman et al., 2002). While frequency methods are computationally simple and highly sensitive, they are prone to false positives when the number of adverse events is small (Wu et al., 2023). In contrast, Bayesian methods account for uncertainty in the disproportionality rate when reported cases are limited (Almenoff et al., 2007). In particular, the ROR is more suitable for high-frequency adverse event reporting, offering the advantage of correcting for bias due to a low number of reports for certain events (Fan et al., 2024). In contrast, the PRR is recognized for its higher specificity compared to ROR (Jiang et al., 2024). The BCPNN excels in integrating data from multiple sources and demonstrates robust performance in cross-validation, which helps to reduce the occurrence of false-positive signals. However, it tends to be more conservative, potentially missing signals for very rare events (Lin et al., 2024). In this regard, the MGPS algorithm offers a more comprehensive approach, particularly effective in detecting signals associated with rare events (Li Y. et al., 2024). Each method contributes uniquely to the detection of drug-associated safety signals, and their combined use provides a more balanced and comprehensive understanding of potential drug-adverse event associations (Zou et al., 2023) (Table 1). We define a PT as a positive signal if it simultaneously meets the thresholds of all four methods (Cui et al., 2023). To mitigate the risk of false-positive results (type I errors), we employed the Bonferroni method to adjust for multiple comparisons of P-values (Curtin and Schulz, 1998). The adjusted threshold is computed as: Bonferroni-corrected P-value = P/n, where P is the original significance threshold and n represents the total number of tests conducted. R software (version 4.2.1) was used for data processing and statistical analysis.
Table 1. Methods and thresholds for ROR, PRR, BCPNN, and EBGM (Wang et al., 2024b; Li R. et al., 2024).
The time to onset (TTO) of irbesartan-related ADEs was defined as the interval between EVENT_DT (date of ADE onset in the DEMO file) and START_DT (date of medication initiation in the THER file). Cases with missing dates (either the initiation of medication or the onset of ADEs) or inaccuracies (not specified to a particular day, month, or year) were excluded. Additionally, cases in which the onset date of ADE occurred before the initiation date of irbesartan therapy were also excluded, as this would result in a negative time-to-onset calculation (Zou et al., 2024). We employed the median, quartile, minimum, maximum, and Weibull shape parameter to comprehensively assess TTO characteristics in our study (Kinoshita et al., 2020). The Kaplan-Meier method was employed to illustrate the cumulative incidence of ADEs associated with irbesartan (Wang et al., 2024). The frequency of adverse events following the initiation of treatment is contingent upon the drug’s mechanism of action and may vary over time. In contrast, the incidence of adverse events not associated with drug therapy tends to be more stable (Cornelius et al., 2012). Changes in the risk incidence of ADEs over time can be identified and predicted by the Weibull distribution test, with scale (α) and shape (β) parameters characterizing the Weibull distribution’s shape (Mazhar et al., 2021). In this study, we focus exclusively on the parameter β. When the shape parameter β is less than 1 and its 95% confidence interval (CI) is also below 1, the risk of adverse effects is considered to decrease over time, indicative of an early failure-type curve. Conversely, if β is approximately equal to 1, with its 95% CI containing the value of 1, the risk is estimated to remain persistent over time, representing a random failure-type curve. Finally, if β is greater than 1 and its 95% CI does not encompass the value of 1, the hazard is interpreted as increasing over time, characteristic of a wear-out failure-type curve (Mazhar et al., 2021; Sauzet et al., 2013).
A total of 5,816 and 366 ADE reports were obtained from the FAERS (January 2004-January 2024) and JADER (April 2008-December 2023) databases, respectively (Figure 1). The number of ADE reports in the FAERS database remained above 400 annually from 2018 through 2023 (Figure 2A), whereas in JADER, the number of reports did not exceed 50 per year (Figure 2B).
Figure 1. Flowchart of the research. The study comprises data collection and cleaning (Section 1), disproportionality analysis methods to calculate signal strength (Section 2), and presentation of results (Section 3). FAERS: FDA Adverse Event Reporting System, JADER: Japanese Adverse Drug Event Report, Q1: first quarter, Q4: fourth quarter, PT: preferred term, PS: primary suspect.
Figure 2. Signal detection at the SOC level. Annual ADE reports in the FAERS (A) and JADER (B) databases are shown as bar charts. The number of irbesartan-induced ADEs at the SOC level in FAERS (C) and JADER (D). Signal detection at the SOC level in FAERS (E) and JADER (F). ROR values and their 95% confidence intervals (95% CI) are visualized. SOC: System Organ Class, ADE: adverse drug event, FAERS: FDA Adverse Event Reporting System, JADER: Japanese Adverse Drug Event Report, ROR: reporting odds ratio.
The characteristics of ADEs reported in both databases, including age, weight, sex, outcome, indication, and reported country, are detailed in Tables 2, 3. In FAERS, ADEs were more frequently reported in female patients (n = 2,916, 50.1%) than male patients (n = 2,095, 36.0%). Among the known age reports, patients aged 65 years or older constituted a major portion (n = 2,878, 49.5%). Body weight, a critical indicator for evaluating adverse reactions to chemical drugs (Yuan et al., 2020), was missing for most patients (n = 4,075, 70.1%). Most reports were submitted by health professionals (n = 3,707, 63.8%), with serious adverse outcomes mainly being other-serious medical events (n = 2,797, 38.2%) and hospitalization-initial or prolonged (n = 2,682, 36.6%). Notably, the proportion of deaths and life-threatening events reported was 3.6% and 5.5%, respectively. The primary indication for irbesartan use was hypertension (n = 2,997, 51.5%), with other indications including essential hypertension (n = 74, 1.2%) and cardiac failure (n = 57, 0.9%) (Table 2).
Table 2. Demographic characteristics of ADEs reported in the FAERS database with irbesartan as the primary suspect drug.
Table 3. Demographic characteristics of ADEs reported in the JADER database with irbesartan as the primary suspect drug.
Conversely, in JADER, higher proportions of male patients (n = 204, 55.7%) submitted more ADE reports compared to female patients (n = 152, 41.5%). The number of reported cases for individuals under 65 years of age is 135, accounting for 36.9% of the total reports. In contrast, the number of reports for individuals aged 65 years or older is 212, representing 57.9% of the total. Among those who provided specific body weight information, the largest number of reports were in the 50–100 kg weight range (n = 158, 43.2%). Sixty nine percent of the submitters experienced varying degrees of recovery or rehabilitation, but 5.2% of the submitters died. Consistent with FAERS reports, the primary indication for irbesartan was hypertension (n = 291, 72.6%) (Table 3).
Mapping the PTs in ADE reports to the corresponding SOC level, we counted the number of reports in both databases. We ranked the case number of PTs in descending order for each SOC and found that irbesartan-associated PTs mainly involved 27 SOCs in FAERS and 22 SOCs in JADER. The top 3 SOCs in terms of reported cases differed between the databases. In FAERS, they were general disorders and administration site conditions (n = 2,186), nervous system disorders (n = 1,881), and gastrointestinal disorders (n = 1,559) (Figure 2C). In JADER, they were metabolism and nutrition disorders (n = 94), cardiac disorders (n = 61), and investigations (n = 58) (Figure 2D). However, among the top 5 SOCs, three overlapped: nervous system disorders, metabolism and nutrition disorders, and investigations.
The signal strength of ADE reports in both databases at the SOC level was calculated using disproportionality analysis. Based on the ROR method, we plotted the forest of signal strength. In FAERS, ten SOCs met the ROR positive threshold: nervous system disorders (SOC code: 10029205), investigations (SOC code: 10022891), metabolism and nutrition disorders (SOC code: 10027433), renal and urinary disorders (SOC code: 10038359), respiratory, thoracic and mediastinal disorders (SOC code: 10038738), vascular disorders (SOC code: 10047065), cardiac disorders (SOC code: 10007541), hepatobiliary disorders (SOC code: 10019805), ear and labyrinth disorders (SOC code: 10013993), and endocrine disorders (SOC code: 10014698) (Figure 2E). In JADER, four SOCs met the positive threshold: metabolism and nutrition disorders, cardiac disorders, renal and urinary disorders, and musculoskeletal and connective tissue disorders (SOC code: 10028395) (Figure 2F). The three SOCs positive in both databases were metabolism and nutrition disorders, cardiac disorders, and renal and urinary disorders.
Notably, in FAERS, the signal strength of renal and urinary disorders (ROR: 3.33 [3.13–3.53], PRR: 3.19, EBGM05: 3.00, IC025: 0.01) met the positive threshold for all four disproportionality methods (Table 4). In JADER, metabolism and nutrition disorders (ROR: 5.17 [4.14–6.46], PRR: 4.45, EBGM05: 3.55, IC025: 0.48) was the only SOC that simultaneously met the positivity threshold for all four methods (Table 5). The complete results are presented in Tables 4, 5.
Subsequently, considering only the frequency of reports, we identified the top 20 PTs in both cohorts. In FAERS, the PT with the highest number of reported cases was acute kidney injury (n = 586, 3.18%), followed by hyponatremia (n = 343, 1.86%), hypotension (n = 326, 1.77%), drug ineffective (n = 321, 1.74%), and dizziness (n = 272, 1.48%) (Figure 3A). In contrast, the top 5 PTs in the JADER database were hyperkalemia (n = 52, 9.65%), rhabdomyolysis (n = 22, 4.08%), renal impairment (n = 19, 3.53%), hepatic function abnormal (n = 18, 3.34%), and interstitial lung disease (n = 14, 2.60%) (Figure 3B). Additionally, we identified six overlapping signals: acute kidney injury, hyponatremia, hypotension, hyperkalemia, diarrhea, and bradycardia.
Figure 3. Bar plot illustrating the top 20 PT statistics in FAERS (A) and JADER (B) databases. The color indicates the SOC of the corresponding PT. Percentage values represent the proportion of cases with such ADEs out of the total reported ADEs.
Using disproportionality methods, we calculated the signal strength of each PT and filtered out all signals that simultaneously met the positivity thresholds. In FAERS, 219 signals met the criteria, while in JADER, 20 signals were identified. Based on the descending order of filtered signals from the reported cases and grouped according to SOC, we presented the ROR values and their 95% CIs for the top 20 signals in each cohort using a forest plot. Generally, higher ROR values indicate a stronger association of these signals with irbesartan. In FAERS, several PTs had a high number of reports along with relatively strong signal strength, including hyponatremia (n = 343, ROR: 20.68 [18.58–23.02], PRR: 20.31, EBGM05: 18.45, IC025: 2.67), hyperkalemia (n = 234, ROR: 23.16 [20.35–26.36], PRR: 22.88, EBGM05: 20.38, IC025: 2.84), and orthostatic hypotension (n = 74, ROR: 14.04 [11.17–17.65], PRR: 13.99, EBGM05: 11.50, IC025: 2.13). Despite the small number of cases, some signals demonstrated strong signal strength, including amyloid arthropathy (n = 11, ROR: 4361.81 [728.77–1602.36], PRR: 820.04, EBGM05: 365.48, IC025: 7.61), neurologic neglect syndrome (n = 22, ROR: 156.19 [101.69–239.91], PRR: 156.01, EBGM05: 148.11, IC025: 5.54), carotid artery thrombosis (n = 24, ROR: 108.66 [72.27–163.36], PRR: 108.52, EBGM05: 74.40, IC025: 5.04), and personality disorder (n = 24, ROR: 30.10 [20.13–45.01], PRR: 30.06, EBGM05: 21.26, IC025: 3.23) (Supplementary Table S1). Notably, we identified several new signals not listed in the drug label, including hyponatremia, syncope, lactic acidosis, arrhythmia, acute pancreatitis, rhabdomyolysis, and cholestasis (Figure 4A). Supplementary Table S1 provides the full results of the analysis in FAERS.
Figure 4. Signal detection at the PT level. The forest plot presents the ROR values along with their confidence intervals for the top 20 positive signals (ranked by case number) associated with irbesartan at the PT level in FAERS (A) and JADER (B) databases. These PTs are categorized by SOC. Adjacent to this, the heatmap illustrates the PRR, EBGM05, and IC025 values for these signals, offering a comprehensive visual of signal strength across different metrics. Arrows in the forest plot indicate instances where the lower limit of the ROR exceeds 30, signifying a notably strong association between irbesartan and the specific adverse event signals at the PT level. Signals of interest have been highlighted in red for emphasis. Venn diagram showing the overlap of 219 positive signals in FAERS and 20 positive signals in JADER (C). ROR: Reporting Odds Ratio; PRR: Proportional Reporting Ratio, EBGM05: the lower limit of the 95% CI of EBGM, IC025: the lower limit of the 95% CI of the IC, SOC: System Organ Class, PT: preferred term, FAERS: FDA Adverse Event Reporting System, JADER: Japanese Adverse Drug Event Report.
In the JADER database, the filtered 20 signals are listed in Figure 4B. PTs with a high number of reported cases included hyperkalemia (n = 52, ROR: 39.26 [29.44–52.34], PRR: 35.57, EBGM05: 27.60, IC025: 3.46), rhabdomyolysis (n = 22, ROR: 8.02 [5.23–12.29], PRR: 7.73, EBGM05: 5.39, IC025: 1.28), and renal impairment (n = 19, ROR: 3.46 [2.19–5.47], PRR: 3.38, EBGM05: 2.30, IC025: 0.08). PTs with strong signal strengths included hyperkalemia, increased blood potassium (n = 9, ROR: 33.70 [17.37–65.39], PRR: 33.15, EBGM05: 18.81, IC025: 3.36), procedural hypotension (n = 4, ROR: 125.32 [45.82–342.73], PRR: 124.39, EBGM05: 51.24, IC025: 5.20), and hypochloremia (n = 4, ROR: 131.43 [48.00–359.84], PRR: 130.46, EBGM05: 53.57, IC025: 5.26). New signals included cardiac failure (n = 12, ROR: 3.75 [2.12–6.65], PRR: 3.69, EBGM05: 2.28, IC025: 0.21), hyponatremia (n = 11, ROR: 7.53 [4.14–13.69], PRR: 7.40, EBGM05: 4.47, IC025: 1.21), respiratory failure (n = 7, ROR: 6.76 [3.20–14.26], PRR: 6.68, EBGM05: 3.57, IC025: 1.07), tubulointerstitial nephritis (n = 6, ROR: 5.77 [2.58–12.92], PRR: 5.72, EBGM05: 2.91, IC025: 0.84), and blood urea increased (n = 5, ROR: 13.34 [5.52–32.25], PRR: 13.23, EBGM05: 6.29, IC025: 2.05) (Figure 4B). Supplementary Table S2 provides the full results of the analysis in JADER.
Combining the results from both databases, we identified eight positive new signals screened in both databases: hyponatremia, hypotension, hyperkalemia, bradycardia, angioedema, rhabdomyolysis, tubulointerstitial nephritis, and hypochloremia (Figure 4C). To visually represent the most significant ADE signals, volcano plots were generated for the analysis results of both FAERS (Supplementary Figure S1A) and JADER (Supplementary Figure S1B) databases. The plots display 219 and 20 positive signals, respectively. In these plots, the horizontal axis represents the log2-transformed ROR values, while the vertical axis shows the -log10-transformed corrected P-values (adjusted using the Bonferroni method). Signals on the right side of the plot, corresponding to higher log2-transformed ROR values, indicate a stronger association with irbesartan compared to those on the left. Strong positive signals identified in FAERS include rare events such as atrial standstill and amyloid arthropathy. In the JADER database, notable signals include hypochloremia and procedural hypotension. These signals represent adverse drug events that show a strong association with irbesartan based on the data from these pharmacovigilance databases, further highlighting the importance of monitoring specific reactions in different populations.
To minimize the influence of confounding factors, we conducted subgroup analyses of adverse events associated with irbesartan using data from both the FAERS and JADER databases. The top 15 most common adverse events for each subgroup were identified based on positive signal criteria (Supplementary Figures S2A, C, E, G). In FAERS, rhabdomyolysis and acute pancreatitis were male-specific, while aphasia and arrhythmia were female-specific (Supplementary Figure S2B). Signals such as muscle spasticity, urinary retention, and dysphagia were predominant in patients under 65, while bradycardia, eczema, and orthostatic hypotension were observed in those 65 and older (Supplementary Figure S2D). In JADER, four overlapping signals—hyperkalemia, rhabdomyolysis, blood potassium increased, and blood creatinine increased were found in both male and female subgroups (Supplementary Figure S2F). Age-specific signals included renal impairment and hepatic function abnormal in the age less than 65 subgroup, and agranulocytosis and respiratory failure in those aged 65 and older (Supplementary Figure S2H).
In clinical practice, irbesartan is often co-administered with other antihypertensive agents such as hydrochlorothiazide and amlodipine to enhance blood pressure control. To eliminate the potential effects of concomitant medications on our results, we conducted a sensitivity analysis. After excluding cases with co-administration of other drugs, we identified 1,479 reports. Persistent adverse reactions included hyponatraemia, hyperkalemia, angioedema, acute pancreatitis, arrhythmia, increased blood creatine phosphokinase, presyncope, swollen tongue, and agranulocytosis, among others (Supplementary Table S3).
Due to the limited number of effective TTO reports in the JADER database, we only statistically analyzed the TTO reports in the FAERS database. In FAERS, there were 987 (17.0%) total effective TTO reports. The median onset time of all ADEs was 107 days, with an interquartile range (IQR) of 15–469 days (Figure 5B).
Figure 5. Time to onset (TTO) analysis (in days) of irbesartan-related ADEs. (A). Bar graphs depict the number and proportion of ADE reports at different time intervals. (B). Overall description and Weibull distribution test analysis of effective TTO reports. The overall analysis highlights the median occurrence of ADEs along with the maximum and minimum values across all TTO reports. The results from the Weibull distribution analysis are presented in terms of scale and shape parameters, which describe the time-dependent risk patterns of ADEs. The scale parameter provides insight into the timeframe of event occurrence, while the shape parameter indicates whether the risk of ADE increases, decreases, or remains constant over time. (C). Box plot of the TTO at the SOC level. The bold bar within the box represents the median TTO, while the lower and upper ends of the box denote the 1st and 3rd quartiles, respectively, indicating the interquartile range. (D). The Kaplan-Meier curve depicts the cumulative incidence of TTO occurrence over time, providing a visual representation of the probability of event occurrence across different time intervals. ADE: adverse drug event; IQR: interquartile range.
ADEs following irbesartan administration occurred primarily within 1 month of administration (n = 337, 34.14%) and after 1 year of administration (n = 319, 32.32%). Within 180 days, the number of TTO reports tended to decrease over time, but reports beyond 180 days still accounted for about 40% of cases (Figure 5A). The Weibull distribution test for TTO indicated that the upper limit of the 95% confidence interval (CI) for the shape parameter (β) was less than 1 in FAERS (0.55), indicating an early failure type, suggesting that the probability of an ADE gradually decreased over time (Figure 5B).
Additionally, we analyzed the TTO reports at the SOC level. Among the 23 SOC levels with at least 10 valid TTO reports, there was a significant difference in TTOs (P < 0.0001, Figure 5C). SOCs with the shortest median onset times included “product issues” [median onset time (MOT): 10 days], “immune system disorders” (MOT: 17 days), and “eye disorders” (MOT: 31 days). SOCs with the longest median TTOs included “surgical and medical procedures” (MOT: 3,667 days), “neoplasms benign, malignant, and unspecified (incl. cysts and polyps)” (MOT: 911 days), and “injury, poisoning, and procedural complications” (MOT: 730 days) (Supplementary Table S4). The cumulative incidence of ADEs over time is depicted in a Kaplan-Meier plot (Figure 5D).
Our analysis of baseline information revealed notable differences in the demographic data concerning irbesartan ADEs between the FAERS and JADER databases. In FAERS, the majority of ADE reports were submitted by females (50.1%) compared to males (36.0%), with 49.5% of submitters aged over 65 years. Despite a significant portion of reports lacking specific weight information, weights over 100 kg represented a major segment of the known weights. The primary reporting regions were the Americas (United States, Canada) and Europe (France, United Kingdom, and Italy), constituting 61.7% of the reports.
Conversely, in JADER, the proportion of male submitters (55.7%) exceeded that of females (41.5%). While 57.9% of the submitters were aged 65 years or older, 36.9% were under 65 years of age, and weights fell primarily within the 50–100 kg range (43.2%), with the vast majority of reports originating from Japan. These demographic variations can be partially attributed to the epidemiological characteristics of hypertension. In 2016, 34.6% of Japanese men and 24.8% of Japanese women were reported to suffer from hypertension (Asakura et al., 2021). By 2017, the prevalence of hypertension among the Japanese working-age population (20–64 years) was 37.5%. Notably, mean systolic and DBP levels have decreased significantly in middle-aged and older Japanese adults due to advancements in hypertension treatment (Hisamatsu et al., 2020). Examining trends over a 55-year period from 1961 to 2016, DBP levels in women decreased by 4–8 mm Hg across all age groups. However, in men aged 50–59, DBP levels remained unchanged, and in men aged 30–49, they increased, potentially due to rising obesity rates, reduced physical activity, and inadequate diastolic hypertension treatment (Nagai et al., 2015; Hisamatsu and Miura, 2024). Similarly, a report from the American Heart Association indicates that in the United States, the prevalence of hypertension is increasing twice as fast in women compared to men, with older women (≥65 years) showing higher prevalence rates than men (Mozaffarian et al., 2015). This trend may be related to post-menopausal hormonal changes, activation of the RAS system, sympathetic nervous system, and increased anxiety and depression levels (Yanes and Reckelhoff, 2011; Maric-Bilkan and Galis, 2016).
The epidemiological studies mentioned above support our analysis. It is noteworthy that differences reported by ADEs in the two databases could be attributed to lifestyle and genetic (ethnic) differences between Japan and Western countries. Hypertension, a multifactorial disease influenced by environmental and genetic factors, shows differing prevalence and control rates across regions (Takeuchi et al., 2018). Japan has seen relatively lower improvements in hypertension awareness, treatment, and control compared to the United States and Europe (Sekikawa and Hayakawa, 2004). Despite higher obesity rates in European and American populations, East Asians are genetically more sensitive to salt and consume higher amounts of it (Bailly et al., 2020; Alfaras et al., 2016). Additionally, Japanese men’s lifestyle choices, such as excessive alcohol consumption and smoking, exacerbate hypertension (Kokubo, 2014). Interestingly, the indications for irbesartan were highly consistent across the two databases. Thorough analysis of these baseline data differences is crucial, as these demographic characteristics may introduce bias into ADE outcomes. Understanding these variations can enhance the interpretation of cohort differences and improve the accuracy of our findings.
Pharmacological hyperkalemia is a predominant cause of elevated potassium levels in clinical practice (Kostis et al., 1996; Mahoney et al., 2005; Park et al., 2012; Antrobus et al., 1993). Although it may be asymptomatic, it can also pose life-threatening risks. Statistics reveal that approximately 1% of emergency department patients and 2% of hospitalized patients presenting with hyperkalemia succumb to the condition (Steiner et al., 2002). Multiple drugs induce hyperkalemia through various mechanisms, such as promoting transcellular potassium transfer or impairing renal potassium excretion. The inhibition of the RAS system, which reduces renal potassium excretion, is the primary mechanism by which drugs cause hyperkalemia (Ben Salem et al., 2014). Several clinical trials have shown that ARB therapy is associated with hyperkalemia, ranging from mildly asymptomatic to clinically significant and life-threatening levels (Mahoney et al., 2005; Palmer, 2003; Ramadan et al., 2005; Piner and Spangler, 2023).
A prospective, randomized controlled trial involving 244 elderly patients with mild-to-moderate essential hypertension demonstrated that low-dose irbesartan (150 mg/day) combined with spironolactone achieved better therapeutic efficacy with a lower risk of hyperkalemia compared to high-dose irbesartan (300 mg/day) (Chen Y. et al., 2018). A randomized controlled clinical trial demonstrated that the incidence of hyperkalemia in the irbesartan treatment group was 1.9% (Lewis et al., 2001). Another retrospective cohort study indicated that patients treated with ARBs alone had a poorer renal prognosis [HR (hazard ratio) 1.31] and a higher risk of hyperkalemia (HR 1.17) compared to those treated with Angiotensin converting enzyme inhibitors (ACEIs) alone. Irbesartan, in particular, showed inferior renoprotective effects (HR 1.35) (Hsu et al., 2017). In addition to irbesartan, other ARBs have been clinically reported to cause hyperkalemia (Desai et al., 2007; Konstam et al., 2009). ARBs may impair renal potassium excretion by blocking angiotensin II binding to adrenoceptors, thereby interfering with adrenal aldosterone secretion (Shier et al., 1989; Raebel, 2012). Age, medications, and reduced renal function are additional factors that increase hyperkalemia risk in most patients. Clinical trials have reported hyperkalemia in up to 6% of patients treated with ARBs, and this percentage rises to 30% in high-risk patients (e.g., those with renal insufficiency or diabetes mellitus) (Park et al., 2012; Palmer, 2004; de Denus et al., 2006).
Hyperkalemia exhibited significant case numbers and strong signal values in both FAERS (n = 234, EBGM05 = 20.38) and JADER (n = 52, EBGM05 = 27.60). This ADE is listed in both Japanese and FDA drug labels. Given the potential for life-threatening consequences due to fatal arrhythmias, timely diagnosis and management of hyperkalemia are crucial (Ideguchi et al., 2016). Prevention is preferable to treatment; thus, medical practitioners should inquire about concomitant medications, diets, supplements, and salt substitutes that may cause hyperkalemia before prescribing irbesartan (Roscioni et al., 2012). Important considerations when initiating irbesartan therapy include obtaining estimates of glomerular filtration rate and baseline serum potassium concentration. Timely monitoring of serum potassium after therapy initiation can help prevent hyperkalemia. If hyperkalemia occurs, prompt recognition and effective treatment are essential to counteract the effects of potassium on the heart, redistribute potassium into cells, and eliminate excess potassium from the body (Raebel, 2012).
Hyponatremia is the most common electrolyte disorder in clinical practice, occurring in 15%–30% of hospitalized patients. Although often mild and asymptomatic, it remains clinically significant due to its potential to cause substantial morbidity and mortality from osmotic cerebral edema and osmotic demyelination if improperly treated (Verbalis et al., 2013). Certain medications (e.g., diuretics, antidepressants, and antiepileptics) are known causes of asymptomatic or symptomatic hyponatremia. However, hyponatremia can also occur with medications used in daily practice, including newer antihypertensive drugs (Liamis et al., 2008). For instance, a middle-aged woman with heart failure and reduced ejection fraction developed severe hyponatremia while on valsartan, with serum sodium levels dropping to 117 mmol/L, which normalized to 140 mmol/L 2 weeks after discontinuation (Mohammed et al., 2022). Similar cases have been reported with telmisartan and in elderly Japanese patients treated with an ARB and a thiazide (Takayama et al., 2019; Yamada et al., 2014).
A previous study showed that angiotensin II type 2 receptor blockers increase the risk of hyponatremia by 4.097-fold (Correia et al., 2014). In animal experiments, AT1 receptor blockers reduced glomerular filtration rate by more than 50% and increased urinary sodium excretion tenfold in neonatal rats (Chevalier, 2012). The ARBs’ inhibition of AT1 receptors, which reduces tubular sodium reabsorption and aldosterone secretion, may explain the induction of hyponatremia (Yamada et al., 2014; Fuzaylova et al., 2020). More recently, a pharmacovigilance analysis of the Spanish Pharmacovigilance database revealed that hyponatremia [ROR: 18.6 (9.6–35.9)] occurred with disproportionality in association with irbesartan use (Estévez Asensio et al., 2024). Our study also identified hyponatremia as a positive signal in FAERS (n = 343, EBGM05 = 18.45) and JADER (n = 11, EBGM05 = 4.47), although it is not listed in the drug labels. While no cases of irbesartan-associated hyponatremia have been reported, vigilance is necessary for the potential risk of hyponatremia with irbesartan use.
In both FAERS and JADER, hyperuricemia and acidosis have emerged as new and significant signals. Hyperuricemia is an evolving metabolic disorder associated with conditions such as hypertension, myocardial infarction, metabolic syndrome, and heart failure (Borghi et al., 2020). The relationship between ARBs and blood uric acid levels is currently debated. For instance, losartan has been shown to have a uric acid-lowering effect by acting on the uric acid transporter protein 1 (URAT1) in the renal proximal tubule, inhibiting URAT1-mediated tubular reabsorption of uric acid, thereby increasing uric acid excretion (Enomoto et al., 2002; Iwanaga et al., 2007). Conversely, azilsartan and olmesartan are associated with elevated blood uric acid levels (Iwanaga et al., 2007; Shiga et al., 2017), suggesting that the uric acid-lowering effect of ARBs is drug-specific rather than class-specific (Smink et al., 2012; Chida et al., 2015). Studies on irbesartan’s impact on serum uric acid levels have produced mixed results, with some reporting beneficial effects and others finding no significant impact (Chida et al., 2015; Dang et al., 2006; Würzner et al., 2001). This inconsistency may stem from differences in study design and baseline characteristics of the populations studied. Given that serum uric acid levels can influence cardiometabolic risk factors, it is crucial to elucidate the relationship between irbesartan and uric acid.
Additionally, reports have linked the use of sartans to acidosis by suppressing the acidification of distal renal units, leading to metabolic acidosis (Sakallı et al., 2014; Wesson et al., 2012). Recognizing the potential risk of acid-base disturbances during irbesartan administration is essential for better patient management.
Our findings indicate that “cardiac disorders” was a significant SOC in both databases. Among these, bradycardia and various arrhythmias (e.g., cardiogenic shock, first-degree atrioventricular block) had higher case report numbers. Previous studies have demonstrated that endogenous angiotensin II exerts a tonic inhibitory effect on cardiac vagal neurotransmission through presynaptic AT1 receptor stimulation. AT1 receptor blockers inhibit this mechanism, promoting acetylcholine release from vagal endings, which may explain the reports of bradycardia with irbesartan (Yamaki et al., 2013; Potter, 1982; Kawada et al., 2007; Chiu et al., 1989; Wong et al., 1991). Although arrhythmias are infrequently reported with irbesartan dosing, it is noteworthy that many arrhythmias (e.g., atrial arrest) are secondary to hyperkalemia, a common adverse drug reaction following irbesartan use (Ideguchi et al., 2016; Kovesdy, 2014). Additionally, some studies involving populations undergoing renal dialysis indicate that irbesartan may have limited efficacy in preventing specific cardiovascular events, such as atrial fibrillation and heart failure, with reports of symptomatic hypotension and renal dysfunction occurring more frequently (Yusuf et al., 2011; Disertori et al., 2009; Peters et al., 2014). Thus, it is essential to remain vigilant for potential cardiac issues following irbesartan administration and to manage them promptly.
Although irbesartan has potential renoprotective effects, such as reducing inflammation and lowering urinary protein (Lewis et al., 2001; Zhong et al., 2020; Parving et al., 2001; Deferrari et al., 2002), ARB use may also increase the risk of acute kidney injury (Wynckel et al., 1998; Toto et al., 1991). Our study identified signals such as acute kidney injury (listed in the drug label) and tubulointerstitial nephritis (new signal). While angiotensin II is believed to cause local ischemia and inflammation in the kidneys, it may also have renoprotective and beneficial effects by enhancing the myogenic response to changes in stress (Benndorf et al., 2009; Griffin and Bidani, 2009). Long-term ARB treatment can lead to an increase in plasma aldosterone concentrations to pre-treatment levels, resulting in “aldosterone escape,” which can cause glomerular and tubular fibrosis (Hubers and Brown, 2016). Angiotensin II receptor antagonist-induced acute renal failure may occur in patients sensitive to reduced renal blood flow (Lee and Kim, 2001). A significantly increased risk of end-stage renal disease following losartan treatment and acute interstitial nephritis after valsartan treatment has been reported, potentially counteracting the renoprotective effects of ARBs (Chen et al., 2019; Miao et al., 2011).
Irbesartan treatment can also cause potential renal injury. In a trial of heart failure treatment with preserved ejection fraction, patients receiving irbesartan experienced a greater decrease in estimated glomerular filtration rate and a higher likelihood of worsening renal function compared to those receiving a placebo (8% versus 4%) (Metra and Lombardi, 2014). Similar renal injuries have been reported in studies by Damman et al. (2016), Veelken et al. (1998), and Metra and Lombardi (2014) The results of a prospective, double-blind, multicenter trial investigating the treatment of severe hypertension indicated that the incidence of elevated blood creatinine levels was 3.0% in patients treated with irbesartan/hydrochlorothiazide and 1.8% in those receiving irbesartan alone (Neutel et al., 2009). These findings align with our observation of a potential association between irbesartan and renal injury. Therefore, we advocate that all patients treated with irbesartan undergo a complete renal and renal vascular ultrasound (to rule out vascular stenosis), and that renal function be dynamically monitored during the course of medication, especially in patients with renal hypoperfusion (Descombes and Fellay, 2000).
Syncope has the highest number of reported cases (n = 151, EBGM05 = 4.30) under “nervous system disorders” in FAERS and is a new signal. Previous studies have shown that vagal excitatory events, including syncope, are associated with AT1 receptor blockers during the treatment of essential hypertension (Sever and Hughes, 2001; Lovelace et al., 2023). This blockade eliminates the modulatory effect of angiotensin II on the Bezold-Jarisch reflex (Lovelace et al., 2023). Additionally, a large double-blind randomized clinical trial demonstrated an increased risk of syncope with the combination of telmisartan and ramipril compared to ramipril alone (Yusuf et al., 2008). It is noteworthy that baroreflex sensitivity decreases with age and systemic hypertension, making older hypertensive patients more susceptible to syncope, which aligns with the age profile in FAERS (nearly half of the reports were from patients ≥65 years of age) (Albasri et al., 2021). In a prospective, observational, descriptive, multicenter clinical study, the highest rate of neurological adverse events was reported following irbesartan treatment, at 1.62% (50 adverse drug events reported), which supports our findings (Ihm et al., 2019).
All antihypertensive medications may predispose older patients to symptomatic orthostatic hypotension (Aronow, 2009). Orthostatic hypotension is characterized by an abnormally large drop in blood pressure upon standing, increasing the risk of adverse outcomes (Wieling et al., 2022). With age, left ventricular compliance decreases, ventricular wall thickness increases, left ventricular diastolic filling decreases, and diastolic function is impaired. Therefore, older hypertensive patients are more likely to develop orthostatic hypotension, consistent with our baseline information analysis (Albasri et al., 2021). Other potential mechanisms include inhibition of bradykinin catabolism by ARBs, anaphylaxis induced by acute mast cell degranulation, or overtreatment (Nielsen, 2005). A clinical study involving 9016 patients with a mean follow-up of 4.1 years found that more patients in the irbesartan group than in the placebo group developed symptomatic hypotension (127 versus 64 patients) (Yusuf et al., 2011). Additionally, a meta-analysis showed that perioperative continuation of ARBs was associated with an approximately 30% increase in intraoperative hypotension incidence (Hollmann et al., 2018). Considering that orthostatic hypotension greatly reduces quality of life and may cause disability, syncope, and traumatic injuries, patients on irbesartan should be adequately informed of the potential risk of hypotension and be encouraged to adopt simple lifestyle measures (moderate, non-strenuous activity, slow changes in posture, etc.) (Ricci et al., 2015).
Pharmacological liver injury is a potential complication of almost all prescribed medications, including irbesartan (Guo et al., 2005). Initially, manufacturers of irbesartan did not recognize any association between the drug and severe liver dysfunction (Hariraj et al., 2000). However, case reports have emerged indicating otherwise. One such case involved a 56-year-old man treated with irbesartan 300 mg/day for 8 days, who was subsequently admitted with jaundice. After excluding other etiologies, cholestatic hepatitis due to irbesartan was diagnosed (Andrade et al., 2002). Another case involved a 62-year-old woman admitted with jaundice after 1 week, having been treated with irbesartan 300 mg/day for a month. Examination revealed jaundice, hepatomegaly, and a liver biopsy showing bile duct dilatation and cholestasis (Hariraj et al., 2000). These findings align with our study.
Current understanding suggests a combination of drug-induced and immune-mediated hepatic injury associated with irbesartan (Annicchiarico and Siciliano, 2005). The hepatotoxicity mechanism likely involves metabolic mediation, with genetic variants in AT1RA metabolism producing reactive metabolites via hepatic cytochrome P450, predisposing patients to drug hepatotoxicity (Andrade et al., 2002). Similar hepatotoxicity has been reported with other sartan drugs (Zahedi et al., 2023; Bosch, 1997a; Vallejo et al., 2000; González-Jiménez et al., 2000; Odak et al., 2021; Basile et al., 2003). In the United States, drug therapy has become a leading cause of acute liver failure (Lee, 2003). Therefore, patients with pre-existing liver disease should be informed of these risks, and immediate discontinuation of the drug with clinical follow-up may be necessary to prevent serious liver damage.
In the JADER database, the SOC “musculoskeletal and connective tissue disorders” met all the algorithmic signal values positively. The number of reported cases of rhabdomyolysis (n = 22, EBGM05 = 5.39) was second only to hyperkalemia. Interestingly, rhabdomyolysis is listed in the Japanese drug label but not in the FDA drug label. Rhabdomyolysis involves catabolic necrosis of muscle tissue and the release of intracellular contents into the bloodstream, caused by various mechanisms, including drugs and toxins, and can be life-threatening in severe cases (Cabral et al., 2020). A common feature of rhabdomyolysis is a decrease in myoplasmic ATP paralleled by a sustained increase in cytoplasmic Ca2+ concentration (Hohenegger, 2012). Irbesartan is primarily metabolized by the liver, and some of its metabolites may exert toxic effects on skeletal muscle cells. By interfering with oxidative phosphorylation in myocytes, the drug or its metabolites can cause mitochondrial dysfunction, increase the production of reactive oxygen species, damage cell membranes and organelles, and ultimately lead to myocyte necrosis and lysis (Bonora et al., 2019). Furthermore, irbesartan controls blood pressure mainly through the RAAS; an imbalance in RAAS regulation may result in inadequate muscle perfusion, particularly in patients with renal insufficiency or other underlying conditions, predisposing myocytes to ischemic necrosis (Sunaga and Ryo, 2022). Irbesartan may also elevate the incidence of rhabdomyolysis by interfering with the metabolic pathways of statins, leading to statin accumulation in the body (Kiaie et al., 2021). Lastly, irbesartan may indirectly increase the risk of rhabdomyolysis by inducing electrolyte disturbances, such as imbalances in potassium ion concentration.
A case-control study based on a Japanese population identified ARB as a risk factor for rhabdomyolysis associated with statin administration (Hashiguchi et al., 2018). Although there is no direct evidence of irbesartan-induced rhabdomyolysis, it is important to note that rhabdomyolysis can exacerbate renal injury through hypovolemia, myoglobinuria, and metabolic acidosis, potentially amplifying the renal injury effects of irbesartan (Chavez et al., 2016). In the event of rhabdomyolysis, it is crucial to discontinue the medication, perform prompt fluid replacement, correct electrolyte abnormalities, and, if necessary, initiate continuous renal replacement therapy (Chavez et al., 2016).
Acute pancreatitis is a leading cause of gastrointestinal-related hospitalizations, with a mortality rate of approximately 30% in critically ill patients (Tu et al., 2012). RAS inhibitors have been associated with an increased risk of acute pancreatitis (Eland et al., 2006; Birck et al., 1998; Bosch, 1997b). The pancreas contains a localized RAS with angiotensin II receptor subtypes AT1 and AT2 found in pancreatic ducts, blood vessels, and adenohypophysial cells, involved in the physiological regulation of digestive enzyme secretion (Famularo et al., 2005). It is hypothesized that bradykinin may contribute to the pathogenesis of acute pancreatitis through its role in the RAS and the bradykinin-kinin system (Bas et al., 2007; Hirata et al., 2002). Two cases of acute pancreatitis have been reported in patients exposed to irbesartan (Famularo et al., 2005; Fisher and Bassett, 2002). Irbesartan may contribute to the development of pancreatitis by triggering pancreatic cell dysfunction through alterations in local hemodynamics or by directly impacting metabolic pathways within pancreatic cells (Mayerle et al., 2019). Furthermore, angiotensin II receptor blockers, including irbesartan, have the potential to induce metabolic disturbances that affect lipid metabolism, insulin sensitivity, and glucose metabolism. Given that metabolic syndrome is a recognized risk factor for pancreatitis, irbesartan may indirectly elevate the risk of pancreatitis by influencing insulin metabolic pathways (Badalov et al., 2007). Additionally, there is a hypothesis suggesting that irbesartan may induce cholestasis by affecting the biliary system (this signal is also indicated in Figure 4A) (Maleszka et al., 2017). This condition may further contribute to biliary pancreatitis, particularly in patients with a history of gallstones or biliary tract disease. Given the limited number of studies examining the association between acute pancreatitis and irbesartan, the new signals of acute pancreatitis that we observed should be interpreted with caution and warrant further investigation in future studies. Nonetheless, clinicians should consider pharmacological pancreatitis in irbesartan-treated patients presenting with severe, unexplained abdominal pain (Famularo et al., 2005).
Our analyses identified several irbesartan-associated ADEs affecting the skin, including “rash maculopapular” (n = 25, EBGM05 = 2.80), “toxic skin eruption” (n = 25, EBGM05 = 6.00), and “pemphigoid” (n = 23, EBGM05 = 8.14). Although cutaneous adverse reactions to ARBs are uncommon (Steckelings et al., 2001; Palleria et al., 2019), the number of cases in our analysis is significant. Of the seven case reports of irbesartan-induced rash, there was one case each of erythema multiforme (Constable et al., 2006), lichenoid rash (Pfab et al., 2006), and pruritic erythematous papules (Cardoso et al., 2019), and four cases of purpuric rash (Foti et al., 2014). Gambini et al. reported a case of irbesartan-induced maculopapular rash in a patient who developed an acute febrile reaction 5 days after starting irbesartan (Gambini et al., 2003). Vena et al. described eczema-like reactions to irbesartan, suggesting that these reactions were related to interference with the kallikrein-kinin system and elevation of circulating and cutaneous pro-inflammatory bradykinin peptides, as ARBs can increase bradykinin levels in hypertensive patients (Campbell et al., 2005; Vena et al., 2013). Additionally, a positive lymphocyte transformation test in patients with rashes suggests an immune mechanism, classifying it as an allergic reaction (Cardoso et al., 2019). In this case, irbesartan may induce a hypersensitivity reaction by stimulating the production of specific antibodies in the body. Upon re-exposure to irbesartan, the drug can bind to these antibodies, activating mast cells and basophils, which results in the release of histamine and other inflammatory mediators. The release of histamine can cause vasodilation and increased vascular permeability, potentially leading to the development of a rash. Additionally, there is a possibility that irbesartan may directly induce mast cell degranulation, resulting in the release of histamine independent of the immune pathway (Nielsen, 2005). This mechanism is typically associated with individual susceptibility and may lead to nonspecific papular urticaria. Recent investigations into cutaneous adverse reactions associated with irbesartan have employed spontaneous reporting databases. A study by Sridharan K et al. identified a potentially increased risk of angioedema with losartan (ROR 3.6 [3.3, 3.8]) and irbesartan (ROR 2.4 [2.1, 2.7]) in comparison to other ARBs, as demonstrated in a pharmacovigilance analysis utilizing the FAERS. Furthermore, Viola E et al. reported a significant disproportionate signal for irbesartan-associated photosensitivity (IC025: 0.62) based on data from VigiBase (Sridharan and Sivaramakrishnan, 2024). Our analysis of FAERS data corroborates these findings, revealing that angioedema and skin photosensitivity were reported in asymmetric proportions among the ADEs related to irbesartan, thereby reinforcing the conclusions of the aforementioned pharmacovigilance studies (Viola et al., 2015).
Our study suggests that several hematological adverse reactions not listed in the specifications may be associated with irbesartan use, including “agranulocytosis” (n = 34, EBGM05 = 4.93) and “bicytopenia” (n = 5, EBGM05 = 4.65). Hematological toxicity has been reported in 0.3% of the treated population due to irbesartan use. This rare but potentially serious adverse reaction is particularly concerning in older adults receiving multiple medications (Gómez-Sayago et al., 2012). An 85-year-old woman developed leukopenia after treatment with irbesartan 150 mg/day, with the Karch-Lasagna algorithm used to demonstrate this causality (Gómez-Sayago et al., 2012). Another retrospective investigation of 184 dialysis patients found that 4–6 weeks after starting treatment with losartan (50 mg/day), the mean hemoglobin concentration decreased from 118 g/L to 101 g/L (Schwarzbeck et al., 1998). Similarly, there were “susceptible” patients whose hemoglobin levels decreased significantly after irbesartan treatment (Simonetti et al., 2007). Irbesartan may contribute to anemia through direct inhibition of erythropoietin or insulin-like growth factor-1 production, or indirectly by improving renal perfusion and subsequently reducing oxygen consumption (Afzali et al., 2006). A negative effect on hematopoiesis at the bone marrow level has also been proposed due to the discovery of AT1 on progenitor cells (Ersoy et al., 2005). These findings may partially explain the new signals we identified. Therefore, close monitoring of hemoglobin and white blood cell counts is recommended when treating patients with severe renal insufficiency with sartans (Schwarzbeck et al., 1998).
In summary, the identification of these new signals necessitates a reassessment of irbesartan prescribing practices, particularly for specific patient populations such as those with pre-existing renal impairment and the elderly. Careful consideration is required before initiating therapy, including regular assessments of renal function and vigilant monitoring for signs of acute pancreatitis or agranulocytosis. Furthermore, the study’s findings support a recommendation to update the drug labeling for irbesartan, informing prescribers of these newly identified risks and emphasizing the importance of monitoring at-risk populations to enhance patient safety. Lastly, our results contribute to the development of updated clinical guidelines for irbesartan, incorporating recommendations on risk stratification, monitoring strategies, and patient counseling to ensure awareness of potential risks among healthcare professionals and patients alike.
In drug safety evaluation, it is crucial to assess the interval between drug administration and the onset of ADEs. This assessment can elucidate the underlying mechanisms of ADEs, identify specific time windows of risk during treatment, and facilitate earlier prevention or diagnosis of adverse reactions (Leroy et al., 2014). The timing of ADEs following RAS inhibitor use has been previously reported. For instance, angioedema after ACEI use typically occurs within the first week of treatment, although a significant proportion can manifest after months or even years (Howes and Tran, 2002). Diseases such as pemphigus associated with ARB therapy often appear months or years after treatment initiation (Steckelings et al., 2001).
A study by Zahedi I et al. suggests that liver function enzymes should be monitored in high-risk patients from the initiation of losartan, from a few days to several months (Zahedi et al., 2023). In our study, the median TTO of “hepatobiliary disorders” and “skin and subcutaneous tissue disorders” was 195 and 46 days, respectively, aligning with the clinical studies mentioned. Additionally, we found that the adverse effects of irbesartan predominantly occurred at 1 month (34.14%) and 1 year (32.32%) after administration. Acute hypersensitivity or immune-mediated reactions, such as angioedema or anaphylactic rash, may occur early in irbesartan therapy, supported by median onset times of 47 days for “skin and subcutaneous tissue disorders” and 17 days for “immune system disorders” in our TTO analysis. Early drug interactions, especially with concurrent medications, may lead to increased irbesartan concentrations, causing adverse reactions like hypotension and dizziness (Nunnery and Mayer, 2019). Long-term use can gradually impair renal function, particularly in patients with chronic kidney disease, as indicated by a median onset of 161 days for “renal and urinary disorders”. Furthermore, long-term therapy may result in drug tolerance or adaptation, leading to an increased frequency of adverse reactions over time (Brass, 1984). These findings highlight the need for clinicians to closely monitor patients in the early stages of treatment to promptly detect immune reactions or organ damage. Additionally, regular assessments of renal and hepatic function, as well as electrolyte levels, are essential during long-term therapy, especially beyond 1 year, to prevent the progression of chronic adverse reactions. In summary, our findings underscore the necessity for continuous vigilance in clinical practice (Maignen et al., 2010).
It is important to acknowledge the limitations of our study:
1. Differences in case reports: The total number of cases in the two databases varied significantly; JADER was limited to case reports, whereas FAERS included periodic reports that encompassed non-serious cases over an extended period. This discrepancy may have influenced the results of our analyses (Zou et al., 2024).
2. Missing key variables: Despite conducting subgroup analyses, some critical variables, such as age and sex, were missing from the data. This absence could have impacted the results, alongside issues related to underreporting and overreporting, which may introduce bias into the findings (Zhang Y. et al., 2024).
3. Lack of comorbidity data: We were unable to obtain information on patients’ comorbidities, preventing us from excluding reports that may represent high-risk factors for certain ADEs (Zhang X. et al., 2024).
4. Signal assessment limitations: Our differential analyses were restricted to assessing the strength of signals related to ADEs, which did not permit quantification of risk or identification of drug-related causality. Consequently, prospective studies are necessary to validate the signals associated with newly identified ADEs (Wu et al., 2024).
5. Confounding variables: Although we employed sensitivity analyses, the presence of confounding variables—such as dosage administered, duration of use, comorbidities, and polypharmacy—may affect the accuracy of our results (Wang et al., 2024b; Wei et al., 2024).
6. Generalizability of findings: This study primarily focused on data from two databases representing the United States and Japan, which may limit the generalizability of the findings to other populations with differing demographic characteristics, health practices, and prescribing patterns.
Despite these limitations, the combined FAERS and JADER databases provide valuable resources for post-marketing safety monitoring of irbesartan.
This study represents the first comprehensive and systematic analysis of ADEs associated with irbesartan, utilizing data from the FAERS and JADER databases. Signal detection at the SOC level identified three SOCs with significant signal strength in both databases: “metabolism and nutrition disorders,” “cardiac disorders,” and “renal and urinary disorders.” Common adverse events such as “acute kidney injury,” “hyperkalemia,” “bradycardia,” and “hypotension” were consistent with the drug label. Additionally, we identified new signals, including “acute pancreatitis,” “rhabdomyolysis,” “maculopapular rash,” “pemphigoid,” and “agranulocytosis.” Moreover, we provide a detailed timeline for the onset of ADEs. This pharmacovigilance assessment not only enhances our understanding of irbesartan’s safety profile but also offers valuable insights for future research and informs clinical practice.
The original contributions presented in the study are included in the article/Supplementary Material, further inquiries can be directed to the corresponding author.
QL: Conceptualization, Visualization, Writing–original draft, Writing–review and editing. ZC: Formal Analysis, Writing–original draft, Writing–review and editing. CD: Formal Analysis, Writing–original draft. CY: Visualization, Writing–original draft. TS: Funding acquisition, Writing–original draft.
The author(s) declare that financial support was received for the research, authorship, and/or publication of this article. This study was supported by the Key Research and Development Program of Shaanxi Province (no. 2022SF-019) and the Key Research and Development Program of Shaanxi Province (no. 2023-YBSF-626).
We are very grateful to the developers and maintainers of the databases mentioned in the manuscript.
The authors declare that the research was conducted in the absence of any commercial or financial relationships that could be construed as a potential conflict of interest.
All claims expressed in this article are solely those of the authors and do not necessarily represent those of their affiliated organizations, or those of the publisher, the editors and the reviewers. Any product that may be evaluated in this article, or claim that may be made by its manufacturer, is not guaranteed or endorsed by the publisher.
The Supplementary Material for this article can be found online at: https://www.frontiersin.org/articles/10.3389/fphar.2024.1485190/full#supplementary-material
SUPPLEMENTARY FIGURE S1 | Volcano plots were generated to display positive risk signals for irbesartan in FAERS (A) and JADER (B) databases, with the horizontal axis representing log2-transformed ROR values and the vertical axis displaying −log10 of Bonferroni-adjusted P-values. Statistically significant signals are highlighted in color. In the FAERS and JADER databases, the top 20 and top 10 signals were labelled ranking by log2 ROR values, respectively. The P-values were adjusted using the Bonferroni method.
SUPPLEMENTARY FIGURE S2 | Subgroup analysis was performed to identify the top 15 signals at the preferred term level, stratified by sex and age groups in the FAERS and JADER databases. Sex-based subgroup analyses were conducted for FAERS (A) and JADER (E), with Venn diagrams (B, F) illustrating the intersection of signals between male and female subgroups. Age-based subgroup analyses were conducted for FAERS (C) and JADER (G), with Venn diagrams (D, H) showing the intersection of signals between the <65 years and ≥65 years age groups. Arrows indicate instances where the lower bound of the 95% confidence interval for the ROR exceeds 30. Due to the limited number of positive signals in the JADER database, E and G displayed fewer than 15 positive signals.
SUPPLEMENTARY TABLE S1 | The 219 PT entries simultaneously satisfying the four methods of disproportionality analysis with positive signal values in FAERS, displayed in descending order of case number. Asterisks (*) indicate new signals not listed in the drug label. The P-value is adjusted with Bonferroni correction method.
SUPPLEMENTARY TABLE S2 | The 20 PT entries simultaneously satisfying the four methods of disproportionality analysis with positive signal values in JADER, displayed in descending order of case number. Asterisks (*) indicate new signals not listed in the drug label. The P-value is adjusted with Bonferroni correction method.
SUPPLEMENTARY TABLE S3 | The results of the sensitivity analysis identified positive ADEs for irbesartan using the FAERS data, specifically excluding cases involving common co-administered drugs at the PT level. This approach ensured that the signals detected were directly attributable to irbesartan, thereby improving the reliability of the findings related to its safety profile. ADEs: adverse drug events; PT, preferred term.
SUPPLEMENTARY TABLE S4 | Detailed TTO analysis results at the SOC level. min: minimum; max: maximum; IQR: interquartile range; q1: quartile 1/4; q3: quartile 3/4; SD: standard deviation; SE: standard error.
Afzali, B., Al-Khoury, S., Shah, N., Mikhail, A., Covic, A., and Goldsmith, D. (2006). Anemia after renal transplantation. Am. J. Kidney Dis. 48 (4), 519–536. doi:10.1053/j.ajkd.2006.07.006
Agrawal, S., Vamadevan, P., Mazibuko, N., Bannister, R., Swery, R., Wilson, S., et al. (2019). A new method for ethical and efficient evidence generation for off-label medication use in oncology (A case study in glioblastoma). Front. Pharmacol. 10, 681. doi:10.3389/fphar.2019.00681
Albasri, A., Hattle, M., Koshiaris, C., Dunnigan, A., Paxton, B., Fox, S. E., et al. (2021). Association between antihypertensive treatment and adverse events: systematic review and meta-analysis. BMJ 372, n189. doi:10.1136/bmj.n189
Alfaras, I., Di Germanio, C., Bernier, M., Csiszar, A., Ungvari, Z., Lakatta, E. G., et al. (2016). Pharmacological strategies to retard cardiovascular aging. Circ. Res. 118 (10), 1626–1642. doi:10.1161/CIRCRESAHA.116.307475
Almenoff, J. S., Pattishall, E. N., Gibbs, T. G., DuMouchel, W., Evans, S. J. W., and Yuen, N. (2007). Novel statistical tools for monitoring the safety of marketed drugs. Clin. Pharmacol. Ther. 82 (2), 157–166. doi:10.1038/sj.clpt.6100258
Andersen, N. N., and Jess, T. (2014). Risk of infections associated with biological treatment in inflammatory bowel disease. World J. Gastroenterol. 20 (43), 16014–16019. doi:10.3748/wjg.v20.i43.16014
Andrade, R. J., Lucena, M. I., Fernández, M. C., Vega, J. L., García-Cortés, M., Casado, M., et al. (2002). Cholestatic hepatitis related to use of irbesartan: a case report and a literature review of angiotensin II antagonist-associated hepatotoxicity. Eur. J. Gastroenterol. Hepatol. 14 (8), 887–890. doi:10.1097/00042737-200208000-00014
Annicchiarico, B. E., and Siciliano, M. (2005). Could irbesartan trigger autoimmune cholestatic hepatitis? Eur. J. Gastroenterol. Hepatol. 17 (2), 247–248. doi:10.1097/00042737-200502000-00019
Antrobus, J. H., Doolan, L. A., and Bethune, D. W. (1993). Hyperkalemia and myocardial atonia following cardioselective beta-blockade. J. Cardiothorac. Vasc. Anesth. 7 (1), 76–78. doi:10.1016/1053-0770(93)90124-4
Aronow, W. S. (2009). Treating hypertension in older adults: safety considerations. Drug Saf. 32 (2), 111–118. doi:10.2165/00002018-200932020-00004
Asakura, E., Ademi, Z., Liew, D., and Zomer, E. (2021). Productivity burden of hypertension in Japan. Hypertens. Res. 44 (11), 1524–1533. doi:10.1038/s41440-021-00731-0
Badalov, N., Baradarian, R., Iswara, K., Li, J., Steinberg, W., and Tenner, S. (2007). Drug-induced acute pancreatitis: an evidence-based review. Clin. Gastroenterol. Hepatol. 5 (6), 648–661. doi:10.1016/j.cgh.2006.11.023
Bailly, L., Fabre, R., Pradier, C., and Iannelli, A. (2020). Colorectal cancer risk following bariatric surgery in a nationwide study of French individuals with obesity. JAMA Surg. 155 (5), 395–402. doi:10.1001/jamasurg.2020.0089
Bas, M., Adams, V., Suvorava, T., Niehues, T., Hoffmann, T. K., and Kojda, G. (2007). Nonallergic angioedema: role of bradykinin. Allergy 62 (8), 842–856. doi:10.1111/j.1398-9995.2007.01427.x
Basile, G., Villari, D., Gangemi, S., Ferrara, T., Accetta, M. G., and Nicita-Mauro, V. (2003). Candesartan cilexetil-induced severe hepatotoxicity. J. Clin. Gastroenterol. 36 (3), 273–275. doi:10.1097/00004836-200303000-00017
Bate, A., Lindquist, M., Edwards, I. R., Olsson, S., Orre, R., Lansner, A., et al. (1998). A Bayesian neural network method for adverse drug reaction signal generation. Eur. J. Clin. Pharmacol. 54 (4), 315–321. doi:10.1007/s002280050466
Benndorf, R. A., Krebs, C., Hirsch-Hoffmann, B., Schwedhelm, E., Cieslar, G., Schmidt-Haupt, R., et al. (2009). Angiotensin II type 2 receptor deficiency aggravates renal injury and reduces survival in chronic kidney disease in mice. Kidney Int. 75 (10), 1039–1049. doi:10.1038/ki.2009.2
Ben Salem, C., Badreddine, A., Fathallah, N., Slim, R., and Hmouda, H. (2014). Drug-induced hyperkalemia. Drug Saf. 37 (9), 677–692. doi:10.1007/s40264-014-0196-1
Birck, R., Keim, V., Fiedler, F., van der Woude, F. J., and Rohmeiss, P. (1998). Pancreatitis after losartan. Lancet 351 (9110), 1178. doi:10.1016/s0140-6736(05)79122-4
Bonora, M., Wieckowski, M. R., Sinclair, D. A., Kroemer, G., Pinton, P., and Galluzzi, L. (2019). Targeting mitochondria for cardiovascular disorders: therapeutic potential and obstacles. Nat. Rev. Cardiol. 16 (1), 33–55. doi:10.1038/s41569-018-0074-0
Borghi, C., and Cicero, A. F. G. (2012). The role of irbesartan in the treatment of patients with hypertension: a comprehensive and practical review. High. Blood Press Cardiovasc Prev. 19 (1), 19–31. doi:10.2165/11632100-000000000-00000
Borghi, C., Palazzuoli, A., Landolfo, M., and Cosentino, E. (2020). Hyperuricemia: a novel old disorder-relationship and potential mechanisms in heart failure. Heart Fail Rev. 25 (1), 43–51. doi:10.1007/s10741-019-09869-z
Bosch, X. (1997a). Losartan-induced hepatotoxicity. JAMA 278 (19), 1572. doi:10.1001/jama.278.19.1572
Bosch, X. (1997b). Losartan-induced acute pancreatitis. Ann. Intern Med. 127 (11), 1043–1044. doi:10.7326/0003-4819-127-11-199712010-00031
Brass, E. P. (1984). Effects of antihypertensive drugs on endocrine function. Drugs 27 (5), 447–458. doi:10.2165/00003495-198427050-00004
Cabral, B. M. I., Edding, S. N., Portocarrero, J. P., and Lerma, E. V. (2020). Rhabdomyolysis. Dis. Mon. 66 (8), 101015. doi:10.1016/j.disamonth.2020.101015
Cai, X., and Li, N. (2022). Association between use of spironolactone and risk of stroke in hypertensive patients: a cohort study. Pharm. (Basel) 16 (1), 57. doi:10.3390/ph16010057
Campbell, D. J., Krum, H., and Esler, M. D. (2005). Losartan increases bradykinin levels in hypertensive humans. Circulation 111 (3), 315–320. doi:10.1161/01.CIR.0000153269.07762.3B
Cardoso, B. K., Martins, M., Farinha, S. M., Viseu, R., Tomaz, E., and Inácio, F. (2019). Late-onset rash from irbesartan: an immunological reaction. Eur. J. Case Rep. Intern Med. 6 (6), 001128. doi:10.12890/2019_001128
Carey, R. M., Moran, A. E., and Whelton, P. K. (2022). Treatment of hypertension: a review. JAMA 328 (18), 1849–1861. doi:10.1001/jama.2022.19590
Chavez, L. O., Leon, M., Einav, S., and Varon, J. (2016). Beyond muscle destruction: a systematic review of rhabdomyolysis for clinical practice. Crit. Care 20 (1), 135. doi:10.1186/s13054-016-1314-5
Chen, C., Li, L., Qin, H., Huang, Z., Xian, J., Cai, J., et al. (2018a). Effects of irbesartan pretreatment on pancreatic β-cell apoptosis in STZ-induced acute prediabetic mice. Oxid. Med. Cell Longev. 2018, 8616194. doi:10.1155/2018/8616194
Chen, T., Xu, P.-C., Hu, S.-Y., Yan, T.-K., Jiang, J.-Q., Jia, J.-Y., et al. (2019). Severe acute interstitial nephritis induced by valsartan: a case report. Med. Baltim. 98 (6), e14428. doi:10.1097/MD.0000000000014428
Chen, Y., Liu, P., Chen, X., Li, Y., Zhang, F., and Wang, Y. (2018b). Effects of different doses of irbesartan combined with spironolactone on urinary albumin excretion rate in elderly patients with early type 2 diabetic nephropathy. Am. J. Med. Sci. 355 (5), 418–424. doi:10.1016/j.amjms.2018.01.017
Chevalier, R. L. (2012). Mechanisms of fetal and neonatal renal impairment by pharmacologic inhibition of angiotensin. Curr. Med. Chem. 19 (27), 4572–4580. doi:10.2174/092986712803306411
Chida, R., Hisauchi, I., Toyoda, S., Kikuchi, M., Komatsu, T., Hori, Y., et al. (2015). Impact of irbesartan, an angiotensin receptor blocker, on uric acid level and oxidative stress in high-risk hypertension patients. Hypertens. Res. 38 (11), 765–769. doi:10.1038/hr.2015.82
Chiu, A. T., Herblin, W. F., McCall, D. E., Ardecky, R. J., Carini, D. J., Duncia, J. V., et al. (1989). Identification of angiotensin II receptor subtypes. Biochem. Biophys. Res. Commun. 165 (1), 196–203. doi:10.1016/0006-291x(89)91054-1
Constable, S., Farrell, J., Naisbitt, D., King, C., Leonard, N., and Pirmohamed, M. (2006). Systemic illness with skin eruption, fever and positive lymphocyte transformation test in a patient on irbesartan. Br. J. Dermatol 155 (2), 491–493. doi:10.1111/j.1365-2133.2006.07338.x
Cornelius, V. R., Sauzet, O., and Evans, S. J. W. (2012). A signal detection method to detect adverse drug reactions using a parametric time-to-event model in simulated cohort data. Drug Saf. 35 (7), 599–610. doi:10.2165/11599740-000000000-00000
Correia, L., Ferreira, R., Correia, I., Lebre, A., Carda, J., Monteiro, R., et al. (2014). Severe hyponatremia in older patients at admission in an internal medicine department. Arch. Gerontol. Geriatr. 59 (3), 642–647. doi:10.1016/j.archger.2014.08.002
Cui, Z., Cheng, F., Wang, L., Zou, F., Pan, R., Tian, Y., et al. (2023). A pharmacovigilance study of etoposide in the FDA adverse event reporting system (FAERS) database, what does the real world say? Front. Pharmacol. 14, 1259908. doi:10.3389/fphar.2023.1259908
Curtin, F., and Schulz, P. (1998). Multiple correlations and Bonferroni's correction. Biol. Psychiatry 44 (8), 775–777. doi:10.1016/s0006-3223(98)00043-2
Damman, K., Solomon, S. D., Pfeffer, M. A., Swedberg, K., Yusuf, S., Young, J. B., et al. (2016). Worsening renal function and outcome in heart failure patients with reduced and preserved ejection fraction and the impact of angiotensin receptor blocker treatment: data from the CHARM-study programme. Eur. J. Heart Fail 18 (12), 1508–1517. doi:10.1002/ejhf.609
Dang, A., Zhang, Y., Liu, G., Chen, G., Song, W., and Wang, B. (2006). Effects of losartan and irbesartan on serum uric acid in hypertensive patients with hyperuricaemia in Chinese population. J. Hum. Hypertens. 20 (1), 45–50. doi:10.1038/sj.jhh.1001941
de Denus, S., Tardif, J.-C., White, M., Bourassa, M. G., Racine, N., Levesque, S., et al. (2006). Quantification of the risk and predictors of hyperkalemia in patients with left ventricular dysfunction: a retrospective analysis of the Studies of Left Ventricular Dysfunction (SOLVD) trials. Am. Heart J. 152 (4), 705–712. doi:10.1016/j.ahj.2006.05.030
Deferrari, G., Ravera, M., Deferrari, L., Vettoretti, S., Ratto, E., and Parodi, D. (2002). Renal and cardiovascular protection in type 2 diabetes mellitus: angiotensin II receptor blockers. J. Am. Soc. Nephrol. 13 (Suppl. 3), S224–S229. doi:10.1097/01.asn.0000032544.37147.ae
Derosa, G., Ferrari, I., and Cicero, A. F. G. (2009). Irbesartan and hydrochlorothiazide association in the treatment of hypertension. Curr. Vasc. Pharmacol. 7 (2), 120–136. doi:10.2174/157016109787455644
Desai, A. S., Swedberg, K., McMurray, J. J. V., Granger, C. B., Yusuf, S., Young, J. B., et al. (2007). Incidence and predictors of hyperkalemia in patients with heart failure: an analysis of the CHARM Program. J. Am. Coll. Cardiol. 50 (20), 1959–1966. doi:10.1016/j.jacc.2007.07.067
Descombes, E., and Fellay, G. (2000). End-stage renal failure after irbesartan prescription in a diabetic patient with previously stable chronic renal insufficiency. Ren. Fail 22 (6), 815–821. doi:10.1081/jdi-100101967
Disertori, M., Latini, R., Barlera, S., Franzosi, M. G., Staszewsky, L., Maggioni, A. P., et al. (2009). Valsartan for prevention of recurrent atrial fibrillation. N. Engl. J. Med. 360 (16), 1606–1617. doi:10.1056/NEJMoa0805710
Edwards, I. R., and Biriell, C. (1994). Harmonisation in pharmacovigilance. Drug Saf. 10 (2), 93–102. doi:10.2165/00002018-199410020-00001
Eland, I. A., Sundström, A., Velo, G. P., Andersen, M., Sturkenboom, M. C. J. M., Langman, M. J. S., et al. (2006). Antihypertensive medication and the risk of acute pancreatitis: the European case-control study on drug-induced acute pancreatitis (EDIP). Scand. J. Gastroenterol. 41 (12), 1484–1490. doi:10.1080/00365520600761676
Enomoto, A., Kimura, H., Chairoungdua, A., Shigeta, Y., Jutabha, P., Cha, S. H., et al. (2002). Molecular identification of a renal urate anion exchanger that regulates blood urate levels. Nature 417 (6887), 447–452. doi:10.1038/nature742
Ersoy, A., Kahvecioglu, S., Ersoy, C., Cift, A., and Dilek, K. (2005). Anemia due to losartan in hypertensive renal transplant recipients without posttransplant erythrocytosis. Transpl. Proc. 37 (5), 2148–2150. doi:10.1016/j.transproceed.2005.03.085
Estévez Asensio, L., García, M., Verde Rello, Z., Velasco-González, V., Fernández-Araque, A. M., and Sainz-Gil, M. (2024). Drug-induced hyponatraemia and possible related signals: analysis of 659 cases reported to the Spanish Pharmacovigilance System and disproportionality analysis. Med. Clin. Barc. S0025-7753 (24), 00553 doi:10.1016/j.medcli.2024.07.021
Evans, S. J., Waller, P. C., and Davis, S. (2001). Use of proportional reporting ratios (PRRs) for signal generation from spontaneous adverse drug reaction reports. Pharmacoepidemiol Drug Saf. 10 (6), 483–486. doi:10.1002/pds.677
Ewers, M., Schmitz, S., Hansson, O., Walsh, C., Fitzpatrick, A., Bennett, D., et al. (2012). Body mass index is associated with biological CSF markers of core brain pathology of Alzheimer's disease. Neurobiol. Aging 33 (8), 1599–1608. doi:10.1016/j.neurobiolaging.2011.05.005
Famularo, G., Minisola, G., Nicotra, G. C., and De Simone, C. (2005). Acute pancreatitis associated with irbesartan therapy. Pancreas 31 (3), 294–295. doi:10.1097/01.mpa.0000178281.58158.64
Fan, Y., Wu, T., Xu, P., Yang, C., An, J., Zhang, H., et al. (2024). Neratinib safety evaluation: real-world adverse event analysis from the FAERS database. Front. Pharmacol. 15, 1425171. doi:10.3389/fphar.2024.1425171
Fisher, A. A., and Bassett, M. L. (2002). Acute pancreatitis associated with angiotensin II receptor antagonists. Ann. Pharmacother. 36 (12), 1883–1886. doi:10.1345/aph.1C099
Fogari, R., Ambrosoli, S., Corradi, L., Degli Esposti, E., Mos, L., Nami, R., et al. (1997). 24-hour blood pressure control by once-daily administration of irbesartan assessed by ambulatory blood pressure monitoring. Irbesartan Multicenter Investigators' Group. J. Hypertens. 15 (12 Pt 1), 1511–1518. doi:10.1097/00004872-199715120-00020
Foti, C., Carbonara, A. M., Guida, S., Antelmi, A., Mazzocca, A., Romita, P., et al. (2014). Frictional purpuric eruption associated with angiotensin II receptor blockers. Dermatol Ther. 27 (2), 97–100. doi:10.1111/dth.12063
Fuzaylova, I., Lam, C., Talreja, O., Makaryus, A. N., Ahern, D., and Cassagnol, M. (2020). Sacubitril/valsartan (Entresto®)-Induced hyponatremia. J. Pharm. Pract. 33 (5), 696–699. doi:10.1177/0897190019828915
Gambini, D., Sala, F., Gianotti, R., and Cusini, M. (2003). Exanthematous reaction to irbesartan. J. Eur. Acad. Dermatol Venereol. 17 (4), 472–473. doi:10.1046/j.1468-3083.2003.00614_3.x
Gómez-Sayago, L., Queizán Hernández, J. A., García-López, L., and Criado Illana, M. T. (2012). Leukoneutropenia associated with irbesartan. Farm Hosp. 36 (4), 302–303. doi:10.1016/j.farma.2011.05.005
González-Jiménez, D., Varela, J. M., Calderón, E., Galindo, J., and González de la Puente, M. A. (2000). Candesartan and acute liver injury. Eur. J. Clin. Pharmacol. 56 (9-10), 769–770. doi:10.1007/s002280000220
Griffin, K. A., and Bidani, A. K. (2009). Angiotensin II type 2 receptor in chronic kidney disease: the good side of angiotensin II? Kidney Int. 75 (10), 1006–1008. doi:10.1038/ki.2009.59
Guion-Firmin, J., Tessier, S., and Montastruc, J.-L. (2022). Diarrhea and angiotensin II receptor blockers: is there any difference between the different drugs? Fundam. Clin. Pharmacol. 36 (2), 443–447. doi:10.1111/fcp.12741
Guo, J. J., Wigle, P. R., Lammers, K., and Vu, O. (2005). Comparison of potentially hepatotoxic drugs among major US drug compendia. Res. Soc. Adm. Pharm. 1 (3), 460–479. doi:10.1016/j.sapharm.2005.06.005
Hariraj, R., Stoner, E., Jader, S., and Preston, D. M. (2000). Drug points: prolonged cholestasis associated with irbesartan. BMJ 321 (7260), 547. doi:10.1136/bmj.321.7260.547
Hashiguchi, M., Hakamata, J., Shimizu, M., Maruyama, J., Shiga, T., and Mochizuki, M. (2018). Risk factors for rhabdomyolysis with HMG-CoA reductase inhibitors identified using a postmarketing surveillance database in Japan. Int. J. Clin. Pharmacol. Ther. 56 (7), 310–320. doi:10.5414/CP203237
Hirata, M., Hayashi, I., Yoshimura, K., Ishii, K.-I., Soma, K., Ohwada, T., et al. (2002). Blockade of bradykinin B(2) receptor suppresses acute pancreatitis induced by obstruction of the pancreaticobiliary duct in rats. Br. J. Pharmacol. 135 (1), 29–36. doi:10.1038/sj.bjp.0704462
Hisamatsu, T., and Miura, K. (2024). Epidemiology and control of hypertension in Japan: a comparison with Western countries. J. Hum. Hypertens. 38 (6), 469–476. doi:10.1038/s41371-021-00534-3
Hisamatsu, T., Segawa, H., Kadota, A., Ohkubo, T., Arima, H., and Miura, K. (2020). Epidemiology of hypertension in Japan: beyond the new 2019 Japanese guidelines. Hypertens. Res. 43 (12), 1344–1351. doi:10.1038/s41440-020-0508-z
Hohenegger, M. (2012). Drug induced rhabdomyolysis. Curr. Opin. Pharmacol. 12 (3), 335–339. doi:10.1016/j.coph.2012.04.002
Hollmann, C., Fernandes, N. L., and Biccard, B. M. (2018). A systematic review of outcomes associated with withholding or continuing angiotensin-converting enzyme inhibitors and angiotensin receptor blockers before noncardiac surgery. Anesth. Analg. 127 (3), 678–687. doi:10.1213/ANE.0000000000002837
Howes, L. G., and Tran, D. (2002). Can angiotensin receptor antagonists be used safely in patients with previous ACE inhibitor-induced angioedema? Drug Saf. 25 (2), 73–76. doi:10.2165/00002018-200225020-00001
Hsu, F.-Y., Lin, F.-J., Ou, H.-T., Huang, S.-H., and Wang, C.-C. (2017). Renoprotective effect of angiotensin-converting enzyme inhibitors and angiotensin II receptor blockers in diabetic patients with proteinuria. Kidney Blood Press Res. 42 (2), 358–368. doi:10.1159/000477946
Hubers, S. A., and Brown, N. J. (2016). Combined angiotensin receptor antagonism and neprilysin inhibition. Circulation 133 (11), 1115–1124. doi:10.1161/CIRCULATIONAHA.115.018622
Ideguchi, T., Tsuruda, T., Sato, Y., and Kitamura, K. (2016). Coexisting hyponatremia and decline in diastolic blood pressure predispose to atrial standstill in hyperkalemic patients. Circ. J. 80 (8), 1781–1786. doi:10.1253/circj.CJ-16-0283
Ihm, S.-H., Shin, J., Park, C.-G., and Kim, C.-H. (2019). Efficacy of a fixed dose combination of irbesartan and atorvastatin (Rovelito®) in Korean adults with hypertension and hypercholesterolemia. Drug Des. Devel Ther. 13, 633–645. doi:10.2147/DDDT.S191973
Imai, T., Hazama, K., Kosuge, Y., Suzuki, S., and Ootsuka, S. (2022). Preventive effect of rebamipide on NSAID-induced lower gastrointestinal tract injury using FAERS and JADER. Sci. Rep. 12 (1), 2631. doi:10.1038/s41598-022-06611-y
Inaba, I., Kondo, Y., Iwasaki, S., Tsuruhashi, S., Akaishi, A., Morita, K., et al. (2019). Risk evaluation for acute kidney injury induced by the concomitant use of valacyclovir, analgesics, and renin-angiotensin system inhibitors: the detection of signals of drug-drug interactions. Front. Pharmacol. 10, 874. doi:10.3389/fphar.2019.00874
Iwanaga, T., Sato, M., Maeda, T., Ogihara, T., and Tamai, I. (2007). Concentration-dependent mode of interaction of angiotensin II receptor blockers with uric acid transporter. J. Pharmacol. Exp. Ther. 320 (1), 211–217. doi:10.1124/jpet.106.112755
Ji, C., Bai, J., Zhou, J., Zou, Y., and Yu, M. (2022). Adverse event profiles of PCSK9 inhibitors alirocumab and evolocumab: data mining of the FDA adverse event reporting system. Br. J. Clin. Pharmacol. 88 (12), 5317–5325. doi:10.1111/bcp.15460
Jiang, Y., Qu, Y., Du, Z., Ou, M., Shen, Y., Zhou, Q., et al. (2024). Exploring adverse events of Vilazodone: evidence from the FAERS database. BMC Psychiatry 24 (1), 371. doi:10.1186/s12888-024-05813-0
Kassler-Taub, K., Littlejohn, T., Elliott, W., Ruddy, T., and Adler, E. (1998). Comparative efficacy of two angiotensin II receptor antagonists, irbesartan and losartan in mild-to-moderate hypertension. Irbesartan/Losartan Study Investigators. Am. J. Hypertens. 11 (4 Pt 1), 445–453. doi:10.1016/s0895-7061(97)00491-3
Kato, K., Mizuno, T., Koseki, T., Ito, Y., Takahashi, K., Tsuboi, N., et al. (2022). Frequency of immune checkpoint inhibitor-induced vasculitides: an observational study using data from the Japanese adverse drug event report database. Front. Pharmacol. 13, 803706. doi:10.3389/fphar.2022.803706
Kawada, T., Yamazaki, T., Akiyama, T., Li, M., Zheng, C., Shishido, T., et al. (2007). Angiotensin II attenuates myocardial interstitial acetylcholine release in response to vagal stimulation. Am. J. Physiol. Heart Circ. Physiol. 293 (4), H2516–H2522. doi:10.1152/ajpheart.00424.2007
Kawano, Y., Sato, Y., and Yoshinaga, K. (2008). A randomized trial of the effect of an angiotensin II receptor blocker SR47436 (irbesartan) on 24-hour blood pressure in patients with essential hypertension. Hypertens. Res. 31 (9), 1753–1763. doi:10.1291/hypres.31.1753
Kiaie, N., Gorabi, A. M., Reiner, Ž., Jamialahmadi, T., Ruscica, M., and Sahebkar, A. (2021). Effects of statins on renin-angiotensin system. J. Cardiovasc Dev. Dis. 8 (7), 80. doi:10.3390/jcdd8070080
Kinoshita, S., Hosomi, K., Yokoyama, S., and Takada, M. (2020). Time-to-onset analysis of amiodarone-associated thyroid dysfunction. J. Clin. Pharm. Ther. 45 (1), 65–71. doi:10.1111/jcpt.13024
Kokubo, Y. (2014). Prevention of hypertension and cardiovascular diseases: a comparison of lifestyle factors in Westerners and East Asians. Hypertension 63 (4), 655–660. doi:10.1161/HYPERTENSIONAHA.113.00543
Konstam, M. A., Neaton, J. D., Dickstein, K., Drexler, H., Komajda, M., Martinez, F. A., et al. (2009). Effects of high-dose versus low-dose losartan on clinical outcomes in patients with heart failure (HEAAL study): a randomised, double-blind trial. Lancet 374 (9704), 1840–1848. doi:10.1016/S0140-6736(09)61913-9
Kose, E., Yamamoto, T., Tate, N., Ando, A., Enomoto, H., and Yasuno, N. (2023). Adverse drug event profile associated with anti-dementia drugs: analysis of a spontaneous reporting database. Pharmazie 78 (5), 42–46. doi:10.1691/ph.2023.2584
Kostis, J. B., Shelton, B., Gosselin, G., Goulet, C., Hood, W. B., Kohn, R. M., et al. (1996). Adverse effects of enalapril in the studies of left ventricular dysfunction (SOLVD). SOLVD investigators. Am. Heart J. 131 (2), 350–355. doi:10.1016/s0002-8703(96)90365-8
Kovesdy, C. P. (2014). Management of hyperkalaemia in chronic kidney disease. Nat. Rev. Nephrol. 10 (11), 653–662. doi:10.1038/nrneph.2014.168
Lacourcière, Y. (2000). A multicenter, randomized, double-blind study of the antihypertensive efficacy and tolerability of irbesartan in patients aged > or = 65 years with mild to moderate hypertension. Clin. Ther. 22 (10), 1213–1224. doi:10.1016/s0149-2918(00)83064-7
Larochelle, P., Flack, J. M., Marbury, T. C., Sareli, P., Krieger, E. M., and Reeves, R. A. (1997). Effects and tolerability of irbesartan versus enalapril in patients with severe hypertension. Irbesartan Multicenter Investigators. Am. J. Cardiol. 80 (12), 1613–1615. doi:10.1016/s0002-9149(97)00784-4
Lee, H. Y., and Kim, C. H. (2001). Acute oliguric renal failure associated with angiotensin II receptor antagonists. Am. J. Med. 111 (2), 162–163. doi:10.1016/s0002-9343(01)00784-7
Lee, W. M. (2003). Drug-induced hepatotoxicity. N. Engl. J. Med. 349 (5), 474–485. doi:10.1056/NEJMra021844
Leroy, F., Dauxois, J.-Y., Théophile, H., Haramburu, F., and Tubert-Bitter, P. (2014). Estimating time-to-onset of adverse drug reactions from spontaneous reporting databases. BMC Med. Res. Methodol. 14, 17. doi:10.1186/1471-2288-14-17
Lewis, E. J., Hunsicker, L. G., Clarke, W. R., Berl, T., Pohl, M. A., Lewis, J. B., et al. (2001). Renoprotective effect of the angiotensin-receptor antagonist irbesartan in patients with nephropathy due to type 2 diabetes. N. Engl. J. Med. 345 (12), 851–860. doi:10.1056/NEJMoa011303
Li, R., Yuan, X., Chen, X., Ou, Y., and Chen, J. (2024b). Analysis of adverse drug reactions of Denosumab (Prolia) in osteoporosis based on FDA adverse event reporting system (FAERS). Front. Pharmacol. 15, 1358592. doi:10.3389/fphar.2024.1358592
Li, Y., Sun, S., Wu, H., Zhao, L., and Peng, W. (2024a). Safety assessment of Tafamidis: a real-world pharmacovigilance study of FDA adverse event reporting system (FAERS) events. BMC Pharmacol. Toxicol. 25 (1), 71. doi:10.1186/s40360-024-00790-2
Liamis, G., Milionis, H., and Elisaf, M. (2008). A review of drug-induced hyponatremia. Am. J. Kidney Dis. 52 (1), 144–153. doi:10.1053/j.ajkd.2008.03.004
Lin, J., Chen, X., Luo, M., Zhuo, Q., Zhang, H., Chen, N., et al. (2024). Safety of tildrakizumab: a disproportionality analysis based on the FDA adverse event reporting system (FAERS) database from 2018-2023. Front. Pharmacol. 15, 1420478. doi:10.3389/fphar.2024.1420478
Lovelace, J. W., Ma, J., Yadav, S., Chhabria, K., Shen, H., Pang, Z., et al. (2023). Vagal sensory neurons mediate the Bezold-Jarisch reflex and induce syncope. Nature 623 (7986), 387–396. doi:10.1038/s41586-023-06680-7
Luna, G., Alping, P., Burman, J., Fink, K., Fogdell-Hahn, A., Gunnarsson, M., et al. (2020). Infection risks among patients with multiple sclerosis treated with fingolimod, natalizumab, rituximab, and injectable therapies. JAMA Neurol. 77 (2), 184–191. doi:10.1001/jamaneurol.2019.3365
Mahoney, B. A., Smith, W. A. D., Lo, D. S., Tsoi, K., Tonelli, M., and Clase, C. M. (2005). Emergency interventions for hyperkalaemia. Cochrane Database Syst. Rev. 2005 (2), CD003235. doi:10.1002/14651858.CD003235.pub2
Maignen, F., Hauben, M., and Tsintis, P. (2010). Modelling the time to onset of adverse reactions with parametric survival distributions: a potential approach to signal detection and evaluation. Drug Saf. 33 (5), 417–434. doi:10.2165/11532850-000000000-00000
Maleszka, A., Dumnicka, P., Matuszyk, A., Pędziwiatr, M., Mazur-Laskowska, M., Sporek, M., et al. (2017). The diagnostic usefulness of serum total bile acid concentrations in the early phase of acute pancreatitis of varied etiologies. Int. J. Mol. Sci. 18 (1), 106. doi:10.3390/ijms18010106
Maric-Bilkan, C., and Galis, Z. S. (2016). Trends in NHLBI-funded research on sex differences in hypertension. Circ. Res. 119 (5), 591–595. doi:10.1161/CIRCRESAHA.116.308963
Mayerle, J., Sendler, M., Hegyi, E., Beyer, G., Lerch, M. M., and Sahin-Tóth, M. (2019). Genetics, cell biology, and pathophysiology of pancreatitis. Gastroenterology 156 (7), 1951–1968. doi:10.1053/j.gastro.2018.11.081
Mazhar, F., Battini, V., Gringeri, M., Pozzi, M., Mosini, G., Marran, A. M. N., et al. (2021). The impact of anti-TNFα agents on weight-related changes: new insights from a real-world pharmacovigilance study using the FDA adverse event reporting system (FAERS) database. Expert Opin. Biol. Ther. 21 (9), 1281–1290. doi:10.1080/14712598.2021.1948529
Mazzolai, L., Maillard, M., Rossat, J., Nussberger, J., Brunner, H. R., and Burnier, M. (1999). Angiotensin II receptor blockade in normotensive subjects: a direct comparison of three AT1 receptor antagonists. Hypertension 33 (3), 850–855. doi:10.1161/01.hyp.33.3.850
Metra, M., and Lombardi, C. (2014). Renin-Angiotensin system blockade and worsening renal function in heart failure: an unfinished story. J. Am. Coll. Cardiol. 64 (11), 1114–1116. doi:10.1016/j.jacc.2014.04.080
Miao, Y., Dobre, D., Heerspink, H. J. L., Brenner, B. M., Cooper, M. E., Parving, H. H., et al. (2011). Increased serum potassium affects renal outcomes: a post hoc analysis of the Reduction of Endpoints in NIDDM with the Angiotensin II Antagonist Losartan (RENAAL) trial. Diabetologia 54 (1), 44–50. doi:10.1007/s00125-010-1922-6
Mohammed, S. R., Pinto Pereira, L. M., Hanoman, H., and Teelucksingh, S. (2022). Chronic asymptomatic hyponatraemia following angiotensin receptor blocker-neprilysin inhibitor (ARNi) therapy: a case report. Postgrad. Med. J. 98 (1157), 165. doi:10.1136/postgradmedj-2020-139284
Morales-Olivas, F. J., Arístegui, I., Estañ, L., Rodicio, J. L., Moreno, A., Gil, V., et al. (2004). The KARTAN study: a postmarketing assessment of Irbesartan in patients with hypertension. Clin. Ther. 26 (2), 232–244. doi:10.1016/s0149-2918(04)90022-7
Mozaffarian, D., Benjamin, E. J., Go, A. S., Arnett, D. K., Blaha, M. J., Cushman, M., et al. (2015). Heart disease and stroke statistics--2015 update: a report from the American Heart Association. Circulation 131 (4), e29–e322. doi:10.1161/CIR.0000000000000152
Nagai, M., Ohkubo, T., Murakami, Y., Takashima, N., Kadota, A., Miyagawa, N., et al. (2015). Secular trends of the impact of overweight and obesity on hypertension in Japan, 1980-2010. Hypertens. Res. 38 (11), 798–795. doi:10.1038/hr.2015.94
Neutel, J. M., Franklin, S. S., Bhaumik, A., Lapuerta, P., and Oparil, S. (2009). Safety and tolerability of fixed-dose irbesartan/hydrochlorothiazide for rapid control of severe hypertension. Clin. Exp. Hypertens. 31 (7), 572–584. doi:10.3109/10641960902929420
Nielsen, E. W. (2005). Hypotensive shock and angio-oedema from angiotensin II receptor blocker: a class effect in spite of tripled tryptase values. J. Intern Med. 258 (4), 385–387. doi:10.1111/j.1365-2796.2005.01547.x
Noguchi, Y., Tachi, T., and Teramachi, H. (2021). Detection algorithms and attentive points of safety signal using spontaneous reporting systems as a clinical data source. Brief. Bioinform 22 (6), bbab347. doi:10.1093/bib/bbab347
Nomura, K., Takahashi, K., Hinomura, Y., Kawaguchi, G., Matsushita, Y., Marui, H., et al. (2015). Effect of database profile variation on drug safety assessment: an analysis of spontaneous adverse event reports of Japanese cases. Drug Des. Devel Ther. 9, 3031–3041. doi:10.2147/DDDT.S81998
Nunnery, S. E., and Mayer, I. A. (2019). Management of toxicity to isoform α-specific PI3K inhibitors. Ann. Oncol. 30 (Suppl. l_10), x21–x26. doi:10.1093/annonc/mdz440
Odak, M., Udongwo, N., Alfraji, N., Zheng, M., and Zaidi, S. (2021). Sprue-like enteropathy and liver injury: a rare emerging association with olmesartan. J. Investig. Med. High. Impact Case Rep. 9, 23247096211037463. doi:10.1177/23247096211037463
Okada, N., Niimura, T., Zamami, Y., Hamano, H., Ishida, S., Goda, M., et al. (2019). Pharmacovigilance evaluation of the relationship between impaired glucose metabolism and BCR-ABL inhibitor use by using an adverse drug event reporting database. Cancer Med. 8 (1), 174–181. doi:10.1002/cam4.1920
Palleria, C., Bennardo, L., Dastoli, S., Iannone, L. F., Silvestri, M., Manti, A., et al. (2019). Angiotensin-converting-enzyme inhibitors and angiotensin II receptor blockers induced pemphigus: a case series and literature review. Dermatol Ther. 32 (1), e12748. doi:10.1111/dth.12748
Palmer, B. F. (2003). Angiotensin-converting enzyme inhibitors and angiotensin receptor blockers: what to do if the serum creatinine and/or serum potassium concentration rises. Nephrol. Dial. Transpl. 18 (10), 1973–1975. doi:10.1093/ndt/gfg282
Palmer, B. F. (2004). Managing hyperkalemia caused by inhibitors of the renin-angiotensin-aldosterone system. N. Engl. J. Med. 351 (6), 585–592. doi:10.1056/NEJMra035279
Park, I., Sheen, S. S., Lim, H.-S., Yoon, D., Park, M. Y., Lee, S.-H., et al. (2012). Comparison of hyperkalemic risk in hospitalized patients treated with different angiotensin receptor blockers: a retrospective cohort study using a Korean clinical research database. Am. J. Cardiovasc Drugs 12 (4), 255–262. doi:10.1007/BF03261834
Parving, H. H., Lehnert, H., Bröchner-Mortensen, J., Gomis, R., Andersen, S., Arner, P., et al. (2001). The effect of irbesartan on the development of diabetic nephropathy in patients with type 2 diabetes. N. Engl. J. Med. 345 (12), 870–878. doi:10.1056/NEJMoa011489
Paz Ocaranza, M., Riquelme, J. A., García, L., Jalil, J. E., Chiong, M., Santos, R. A. S., et al. (2020). Counter-regulatory renin-angiotensin system in cardiovascular disease. Nat. Rev. Cardiol. 17 (2), 116–129. doi:10.1038/s41569-019-0244-8
Peters, C. D., Kjaergaard, K. D., Jensen, J. D., Christensen, K. L., Strandhave, C., Tietze, I. N., et al. (2014). No significant effect of angiotensin II receptor blockade on intermediate cardiovascular end points in hemodialysis patients. Kidney Int. 86 (3), 625–637. doi:10.1038/ki.2014.69
Pfab, F., Athanasiadis, G. I., Kollmar, A., Ring, J., and Ollert, M. (2006). Lichenoid drug eruption due to an antihypertonic drug containing irbesartan and hydrochlorothiazide. Allergy 61 (6), 786–787. doi:10.1111/j.1398-9995.2006.01052.x
Piner, A., and Spangler, R. (2023). Disorders of potassium. Emerg. Med. Clin. North Am. 41 (4), 711–728. doi:10.1016/j.emc.2023.07.005
Potter, E. K. (1982). Angiotensin inhibits action of vagus nerve at the heart. Br. J. Pharmacol. 75 (1), 9–11. doi:10.1111/j.1476-5381.1982.tb08752.x
Raebel, M. A. (2012). Hyperkalemia associated with use of angiotensin-converting enzyme inhibitors and angiotensin receptor blockers. Cardiovasc Ther. 30 (3), e156–e166. doi:10.1111/j.1755-5922.2010.00258.x
Ramadan, F. H., Masoodi, N., and El-Solh, A. A. (2005). Clinical factors associated with hyperkalemia in patients with congestive heart failure. J. Clin. Pharm. Ther. 30 (3), 233–239. doi:10.1111/j.1365-2710.2005.00638.x
Ricci, F., De Caterina, R., and Fedorowski, A. (2015). Orthostatic hypotension: epidemiology, prognosis, and treatment. J. Am. Coll. Cardiol. 66 (7), 848–860. doi:10.1016/j.jacc.2015.06.1084
Robles, N. R., Cerezo, I., and Hernandez-Gallego, R. (2014). Renin-angiotensin system blocking drugs. J. Cardiovasc Pharmacol. Ther. 19 (1), 14–33. doi:10.1177/1074248413501018
Roscioni, S. S., de Zeeuw, D., Bakker, S. J. L., and Lambers Heerspink, H. J. (2012). Management of hyperkalaemia consequent to mineralocorticoid-receptor antagonist therapy. Nat. Rev. Nephrol. 8 (12), 691–699. doi:10.1038/nrneph.2012.217
Sakallı, H., Baskın, E., Bayrakcı, U. S., Moray, G., and Haberal, M. (2014). Acidosis and hyperkalemia caused by losartan and enalapril in pediatric kidney transplant recipients. Exp. Clin. Transpl. 12 (4), 310–313. doi:10.6002/ect.2013.0172
Sauzet, O., Carvajal, A., Escudero, A., Molokhia, M., and Cornelius, V. R. (2013). Illustration of the weibull shape parameter signal detection tool using electronic healthcare record data. Drug Saf. 36 (10), 995–1006. doi:10.1007/s40264-013-0061-7
Schwarzbeck, A., Wittenmeier, K. W., and Hällfritzsch, U. (1998). Anaemia in dialysis patients as a side-effect of sartanes. Lancet 352 (9124), 286. doi:10.1016/s0140-6736(05)60259-0
Sekikawa, A., and Hayakawa, T. (2004). Prevalence of hypertension, its awareness and control in adult population in Japan. J. Hum. Hypertens. 18 (12), 911–912. doi:10.1038/sj.jhh.1001765
Sever, P. S., and Hughes, A. (2001). Angiotensin receptor antagonists and vaso-vagal attacks due to sensitisation of the Bezold-Jarisch reflex? J. Hum. Hypertens. 15 (6), 437–438. doi:10.1038/sj.jhh.1001190
Sharma, A. K., Rastogi, S., and Goyal, R. K. (2024). Retrospective analysis of neoplasms in patients using angiotensin receptor blockers. Sci. Rep. 14 (1), 15774. doi:10.1038/s41598-024-64867-y
Shen, J., Yang, J., and Zhao, B. (2019). A survey of the FDA's adverse event reporting system database concerning urogenital tract infections and sodium glucose cotransporter-2 inhibitor use. Diabetes Ther. 10 (3), 1043–1050. doi:10.1007/s13300-019-0611-9
Shier, D. N., Kusano, E., Stoner, G. D., Franco-Saenz, R., and Mulrow, P. J. (1989). Production of renin, angiotensin II, and aldosterone by adrenal explant cultures: response to potassium and converting enzyme inhibition. Endocrinology 125 (1), 486–491. doi:10.1210/endo-125-1-486
Shiga, Y., Miura, S.-I., Motozato, K., Norimatsu, K., Yano, M., Hitaka, Y., et al. (2017). Comparison of efficacy and safety of azilsartan and olmesartan in patients with essential hypertension. Int. Heart J. 58 (3), 416–421. doi:10.1536/ihj.16-285
Simon, T. A., Gelarden, R. T., Freitag, S. A., Kassler-Taub, K. B., and Davies, R. (1998). Safety of irbesartan in the treatment of mild to moderate systemic hypertension. Am. J. Cardiol. 82 (2), 179–182. doi:10.1016/s0002-9149(98)00313-0
Simonetti, G. D., Bianchetti, M. G., Konrad, M., and von Vigier, R. O. (2007). Severe anemia caused by the angiotensin receptor blocker irbesartan after renal transplantation. Pediatr. Nephrol. 22 (5), 756–757. doi:10.1007/s00467-006-0400-3
Singh, J. (2015). International conference on harmonization of technical requirements for registration of pharmaceuticals for human use. J. Pharmacol. Pharmacother. 6 (3), 185–187. doi:10.4103/0976-500X.162004
Smink, P. A., Bakker, S. J. L., Laverman, G. D., Berl, T., Cooper, M. E., de Zeeuw, D., et al. (2012). An initial reduction in serum uric acid during angiotensin receptor blocker treatment is associated with cardiovascular protection: a post-hoc analysis of the RENAAL and IDNT trials. J. Hypertens. 30 (5), 1022–1028. doi:10.1097/HJH.0b013e32835200f9
Song, S., Cai, X., Hu, J., Zhu, Q., Shen, D., Ma, H., et al. (2024). Effectiveness of spironolactone in reducing osteoporosis and future fracture risk in middle-aged and elderly hypertensive patients. Drug Des. Devel Ther. 18, 2215–2225. doi:10.2147/DDDT.S466904
Sridharan, K., and Sivaramakrishnan, G. (2024). A pharmacovigilance study assessing risk of angioedema with angiotensin receptor blockers using the US FDA Adverse Event Reporting System. Expert Opin. Drug Saf., 1–8. doi:10.1080/14740338.2024.2393279
Steckelings, U. M., Artuc, M., Wollschläger, T., Wiehstutz, S., and Henz, B. M. (2001). Angiotensin-converting enzyme inhibitors as inducers of adverse cutaneous reactions. Acta Derm. Venereol. 81 (5), 321–325. doi:10.1080/000155501317140007
Steiner, C., Elixhauser, A., and Schnaier, J. (2002). The healthcare cost and utilization project: an overview. Eff. Clin. Pract. 5 (3), 143–151.
Stumpe, K. O., Haworth, D., Hoglund, C., Kerwin, L., Martin, A., Simon, T., et al. (1998). Comparison of the angiotensin II receptor antagonist irbesartan with atenolol for treatment of hypertension. Blood Press 7 (1), 31–37. doi:10.1080/080370598437547
Sunaga, T., and Ryo, Y. (2022). Potential safety signals for rhabdomyolysis associated with high-potency statin use with or without sacubitril/valsartan. Am. J. Cardiol. 166, 127–130. doi:10.1016/j.amjcard.2021.11.026
Szarfman, A., Machado, S. G., and O'Neill, R. T. (2002). Use of screening algorithms and computer systems to efficiently signal higher-than-expected combinations of drugs and events in the US FDA's spontaneous reports database. Drug Saf. 25 (6), 381–392. doi:10.2165/00002018-200225060-00001
Takayama, A., Nagamine, T., Matsumoto, Y., and Nakamura, M. (2019). Duloxetine and angiotensin II receptor blocker combination potentially induce severe hyponatremia in an elderly woman. Intern Med. 58 (12), 1791–1794. doi:10.2169/internalmedicine.2059-18
Takeuchi, F., Akiyama, M., Matoba, N., Katsuya, T., Nakatochi, M., Tabara, Y., et al. (2018). Interethnic analyses of blood pressure loci in populations of East Asian and European descent. Nat. Commun. 9 (1), 5052. doi:10.1038/s41467-018-07345-0
Toto, R. D., Mitchell, H. C., Lee, H. C., Milam, C., and Pettinger, W. A. (1991). Reversible renal insufficiency due to angiotensin converting enzyme inhibitors in hypertensive nephrosclerosis. Ann. Intern Med. 115 (7), 513–519. doi:10.7326/0003-4819-115-7-513
Tu, X.-H., Song, J.-X., Xue, X.-J., Guo, X.-W., Ma, Y.-X., Chen, Z.-Y., et al. (2012). Role of bone marrow-derived mesenchymal stem cells in a rat model of severe acute pancreatitis. World J. Gastroenterol. 18 (18), 2270–2279. doi:10.3748/wjg.v18.i18.2270
Vallejo, I., García Morillo, S., and Pamies, E. (2000). Acute hepatitis induced by candesartan. Med. Clin. Barc. 115 (18), 719. doi:10.1016/s0025-7753(00)71672-1
van Hasselt, J. G. C., Rahman, R., Hansen, J., Stern, A., Shim, J. V., Xiong, Y., et al. (2020). Transcriptomic profiling of human cardiac cells predicts protein kinase inhibitor-associated cardiotoxicity. Nat. Commun. 11 (1), 4809. doi:10.1038/s41467-020-18396-7
van Puijenbroek, E. P., Bate, A., Leufkens, H. G. M., Lindquist, M., Orre, R., and Egberts, A. C. G. (2002). A comparison of measures of disproportionality for signal detection in spontaneous reporting systems for adverse drug reactions. Pharmacoepidemiol Drug Saf. 11 (1), 3–10. doi:10.1002/pds.668
Veelken, R., Hilgers, K. F., and Mann, J. F. (1998). The acute renal effects of angiotensin II receptor blockers. Nephrol. Dial. Transpl. 13 (8), 1928–1929. doi:10.1093/ndt/13.8.1928
Vena, G. A., Cassano, N., Coco, V., and De Simone, C. (2013). Eczematous reactions due to angiotensin-converting enzyme inhibitors or angiotensin II receptor blockers. Immunopharmacol. Immunotoxicol. 35 (3), 447–450. doi:10.3109/08923973.2013.797992
Verbalis, J. G., Goldsmith, S. R., Greenberg, A., Korzelius, C., Schrier, R. W., Sterns, R. H., et al. (2013). Diagnosis, evaluation, and treatment of hyponatremia: expert panel recommendations. Am. J. Med. 126 (10 Suppl. 1), S1–S42. doi:10.1016/j.amjmed.2013.07.006
Viola, E., Coggiola Pittoni, A., Drahos, A., Moretti, U., and Conforti, A. (2015). Photosensitivity with angiotensin II receptor blockers: a retrospective study using data from VigiBase(®). Drug Saf. 38 (10), 889–894. doi:10.1007/s40264-015-0323-7
Wan, Q., Li, Q., Lai, X., Xu, T., Hu, J., and Peng, H. (2022). Data mining and safety analysis of BTK inhibitors: a pharmacovigilance investigation based on the FAERS database. Front. Pharmacol. 13, 995522. doi:10.3389/fphar.2022.995522
Wang, J., Zhang, Z., Liu, X., Shi, S., Lv, J., Zhang, Y., et al. (2024). Exploring novel adverse events of nefecon. Kidney Int. Rep. 9 (9), 2705–2717. doi:10.1016/j.ekir.2024.07.006
Wang, Q., Zhou, Q., Du, Z., Lu, R., Jiang, Y., and Zhu, H. (2024b). Clinical safety of daridorexant in insomnia treatment: analysis of FDA adverse event reports. J. Affect Disord. 362, 552–559. doi:10.1016/j.jad.2024.07.034
Warwick, J., Falaschetti, E., Rockwood, K., Mitnitski, A., Thijs, L., Beckett, N., et al. (2015). No evidence that frailty modifies the positive impact of antihypertensive treatment in very elderly people: an investigation of the impact of frailty upon treatment effect in the HYpertension in the Very Elderly Trial (HYVET) study, a double-blind, placebo-controlled study of antihypertensives in people with hypertension aged 80 and over. BMC Med. 13, 78. doi:10.1186/s12916-015-0328-1
Wei, W., Bai, Y.-T., Chang, E., and Liu, J.-F. (2024). Post-marketing safety surveillance of fostamatinib: an observational, pharmacovigilance study leveraging FAERS database. Expert Opin. Drug Saf., 1–9. doi:10.1080/14740338.2024.2387315
Wesson, D. E., Jo, C.-H., and Simoni, J. (2012). Angiotensin II receptors mediate increased distal nephron acidification caused by acid retention. Kidney Int. 82 (11), 1184–1194. doi:10.1038/ki.2012.267
Wieling, W., Kaufmann, H., Claydon, V. E., van Wijnen, V. K., Harms, M. P. M., Juraschek, S. P., et al. (2022). Diagnosis and treatment of orthostatic hypotension. Lancet Neurol. 21 (8), 735–746. doi:10.1016/S1474-4422(22)00169-7
Wong, P. C., Price, W. A., Chiu, A. T., Duncia, J. V., Carini, D. J., Wexler, R. R., et al. (1991). In vivo pharmacology of DuP 753. Am. J. Hypertens. 4 (4 Pt 2), 288S–298S. doi:10.1093/ajh/4.4.288s
Wu, J., Wu, H., Chen, L., Liang, H., Huang, G., Yang, S., et al. (2024). Safety of daratumumab in the real-world: a pharmacovigilance study based on FAERS database. Expert Opin. Drug Saf. 23 (7), 905–916. doi:10.1080/14740338.2023.2296966
Wu, Y., Wei, M., and Zhang, J. (2023). A real-world pharmacovigilance analysis of FDA adverse event reporting system database for upadacitinib. Front. Pharmacol. 14, 1200254. doi:10.3389/fphar.2023.1200254
Würzner, G., Gerster, J. C., Chiolero, A., Maillard, M., Fallab-Stubi, C. L., Brunner, H. R., et al. (2001). Comparative effects of losartan and irbesartan on serum uric acid in hypertensive patients with hyperuricaemia and gout. J. Hypertens. 19 (10), 1855–1860. doi:10.1097/00004872-200110000-00021
Wynckel, A., Ebikili, B., Melin, J. P., Randoux, C., Lavaud, S., and Chanard, J. (1998). Long-term follow-up of acute renal failure caused by angiotensin converting enzyme inhibitors. Am. J. Hypertens. 11 (9), 1080–1086. doi:10.1016/s0895-7061(98)00117-4
Yamada, H., Asano, T., Aoki, A., Ikoma, A., Yoshida, M., Kusaka, I., et al. (2014). Combination therapy of angiotensin II receptor blocker and thiazide produces severe hyponatremia in elderly hypertensive subjects. Intern Med. 53 (7), 749–752. doi:10.2169/internalmedicine.53.1738
Yamaki, F., Arai, T., Aoyama, M., Watanabe, A., and Takata, Y. (2013). Angiotensin AT(1)-receptor blockers enhance cardiac responses to parasympathetic nerve stimulation via presynaptic AT(1) receptors in pithed rats. J. Pharmacol. Sci. 122 (1), 28–33. doi:10.1254/jphs.12283fp
Yamaoka, K., Fujiwara, M., Uchida, M., Uesawa, Y., Muroi, N., and Shimizu, T. (2022). Comprehensive analysis of ixazomib-induced adverse events using the Japanese pharmacovigilance database. Oncology 100 (7), 413–418. doi:10.1159/000524806
Yanes, L. L., and Reckelhoff, J. F. (2011). Postmenopausal hypertension. Am. J. Hypertens. 24 (7), 740–749. doi:10.1038/ajh.2011.71
Yuan, L., Li, Q., Bai, D., Shang, X., Hu, F., Chen, Z., et al. (2020). La2O3 nanoparticles induce reproductive toxicity mediated by the nrf-2/ARE signaling pathway in kunming mice. Int. J. Nanomedicine 15, 3415–3431. doi:10.2147/IJN.S230949
Yusuf, S., Healey, J. S., Pogue, J., Chrolavicius, S., Flather, M., Hart, R. G., et al. (2011). Irbesartan in patients with atrial fibrillation. N. Engl. J. Med. 364 (10), 928–938. doi:10.1056/NEJMoa1008816
Yusuf, S., Teo, K. K., Pogue, J., Dyal, L., Copland, I., Schumacher, H., et al. (2008). Telmisartan, ramipril, or both in patients at high risk for vascular events. N. Engl. J. Med. 358 (15), 1547–1559. doi:10.1056/NEJMoa0801317
Zahedi, I., Sami, M., Ijishakin, O., Onyemarim, H., Cutliff, R. T., and Ezeamii, V. C. (2023). Marked hepatotoxicity associated with losartan treatment: a case report. Cureus 15 (12), e49846. doi:10.7759/cureus.49846
Zhang, X., Pan, J., Zhang, X., Yang, Q., Li, Z., and Liu, F. (2024b). Safety profiles of tetracycline-class drugs: a pharmacovigilance analysis of the FAERS database. Expert Opin. Drug Saf., 1–10. doi:10.1080/14740338.2024.2393276
Zhang, Y., Ran, L., Liang, Y., Zhang, Y., and An, Z. (2023). Safety analysis of pemigatinib leveraging the US Food and Drug administration adverse event reporting system. Front. Pharmacol. 14, 1194545. doi:10.3389/fphar.2023.1194545
Zhang, Y., Sun, S., and Wang, Y. (2024a). Adverse events associated with Atogepant: a FAERS-based pharmacovigilance analysis. Expert Opin. Drug Saf., 1–7. doi:10.1080/14740338.2024.2393268
Zhong, Y., Tang, R., Lu, Y., Wang, W., Xiao, C., Meng, T., et al. (2020). Irbesartan may relieve renal injury by suppressing Th22 cells chemotaxis and infiltration in Ang II-induced hypertension. Int. Immunopharmacol. 87, 106789. doi:10.1016/j.intimp.2020.106789
Zhou, B., Perel, P., Mensah, G. A., and Ezzati, M. (2021). Global epidemiology, health burden and effective interventions for elevated blood pressure and hypertension. Nat. Rev. Cardiol. 18 (11), 785–802. doi:10.1038/s41569-021-00559-8
Zhou, J., Ye, J., Chen, M., and Zheng, X. (2024). A real-world disproportionality analysis of baloxavir marboxil: post-marketing pharmacovigilance data. Expert Opin. Drug Saf., 1–9. doi:10.1080/14740338.2024.2393269
Zou, F., Zhu, C., Lou, S., Cui, Z., Wang, D., Ou, Y., et al. (2023). A real-world pharmacovigilance study of mepolizumab in the FDA adverse event reporting system (FAERS) database. Front. Pharmacol. 14, 1320458. doi:10.3389/fphar.2023.1320458
Keywords: pharmacovigilance, disproportionality analysis, FAERS, JADER, irbesartan, adverse drug events
Citation: Liu Q, Cui Z, Deng C, Yang C and Shi T (2024) A real-world pharmacovigilance analysis of adverse events associated with irbesartan using the FAERS and JADER databases. Front. Pharmacol. 15:1485190. doi: 10.3389/fphar.2024.1485190
Received: 23 August 2024; Accepted: 28 October 2024;
Published: 20 November 2024.
Edited by:
Xintian Cai, People’s Hospital of Xinjiang Uygur Autonomous Region, ChinaReviewed by:
Di Shen, People’s Hospital of Xinjiang Uygur Autonomous Region, ChinaCopyright © 2024 Liu, Cui, Deng, Yang and Shi. This is an open-access article distributed under the terms of the Creative Commons Attribution License (CC BY). The use, distribution or reproduction in other forums is permitted, provided the original author(s) and the copyright owner(s) are credited and that the original publication in this journal is cited, in accordance with accepted academic practice. No use, distribution or reproduction is permitted which does not comply with these terms.
*Correspondence: Tao Shi, c2hpMjAwOXRhb0AxNjMuY29t
Disclaimer: All claims expressed in this article are solely those of the authors and do not necessarily represent those of their affiliated organizations, or those of the publisher, the editors and the reviewers. Any product that may be evaluated in this article or claim that may be made by its manufacturer is not guaranteed or endorsed by the publisher.
Research integrity at Frontiers
Learn more about the work of our research integrity team to safeguard the quality of each article we publish.