- Department of Oral Anatomy and Physiology, Jilin Provincial Key Laboratory of Oral Biomedical Engineering, Hospital of Stomatology, Jilin University, Changchun, China
Artesunate (ART) is a classic antimalarial drug with high efficiency, low toxicity and tolerance. It has been shown to be safe and has good anti-tumor effect. Existing clinical studies have shown that the anti-tumor mechanisms of ART mainly include inducing apoptosis and autophagy of tumor cells, affecting tumor microenvironment, regulating immune response, overcoming drug resistance, as well as inhibiting tumor cell proliferation, migration, invasion, and angiogenesis. ART has been proven to fight against lung cancer, hepatocarcinoma, lymphoma, multiple myeloma, leukemia, colorectal cancer, ovarian cancer, cervical cancer, malignant melanoma, oral squamous cell carcinoma, bladder cancer, prostate cancer and other neoplasms. In this review, we highlight the effects of ART on various tumors with an emphasis on its anti-tumor mechanism, which is helpful to propose the potential research directions of ART and expand its clinical application.
1 Introduction
Chemoresistance is an important cause of tumor treatment failure and recurrence. Therefore, it is of great significance to find effective anti-tumor drugs that can overcome drug resistance. Artesunate (ART), an efficient and relatively safe water-soluble hemisuccinate derivative of dihydroartemisinin (DHA) (an antimalarial drug) (Qian Y. et al., 2021), is derived from artemisia annua. ART is inherently unstable in an aqueous solution, rapidly hydrolyzes after preparation and injection, and can be used for temporary intravenous administration (Gashe et al., 2022). At present, it has been found that two-carbon tied artemisinin-isatin complexes have anti-breast cancer potential and the artemisinin-isatin hybrid with ethylene binding system has anti-lung cancer activity (Wang et al., 2023a; Wang et al., 2023b). ART is inherently unstable in an aqueous solution, rapidly hydrolyzes after preparation and injection, and can be used for temporary intravenous administration. Recent studies have found that in addition to its specific anti-malaria effect, ART also has many other biological activities, such as anti-inflammatory (Ruwizhi et al., 2022), anti-tumor (Eitae and Park, 2020), anti-viral (Hu Y. J. et al., 2021) and hypoglycemic (Alagbonsi et al., 2021). Especially in the field of anti-tumor, several studies have reported the strong anticancer activity of ART in recent years (Ma et al., 2021; Guan et al., 2023; Zeng et al., 2023). The anti-tumor mechanism of ART is largely dependent on the release of DHA from ART as a prodrug. The latest research shows that DHA inhibits tumor progress via blocking ROR1-induced STAT3-activation (Li J.-Y et al., 2022; Li et al., 2024b), inhibits angiogenesis via regulating VEGF and MMP-2/-9 (Rao et al., 2024), remodels tumor micro-environment and improves cancer immunotherapy through inhibiting cyclin-dependent kinases (Zhou et al., 2024), restores the immunogenicity and enhances the anticancer immunosurveillance of cisplatin by activating the PERK/eIF2α pathway (Li G et al., 2022). The other potential anti-tumor mechanisms of ART may be its own mechanism of action. ART inhibits the proliferation of Burkitt lymphoma cells by inhibiting AKT and ERK, so this compound is considered to have the potential to develop novel anti-tumor drugs (Yuan-Ce et al., 2023). Related studies have shown that ART acts on glioblastoma cells by inducing oxidative stress, DNA damage, apoptosis and necrosis (Strik et al., 2024). ART can also induce apoptosis of breast cancer cells, showing an anti-breast cancer effect (Wen et al., 2024). ART could combat choroidal melanoma by promoting apoptosis, inducing cell cycle arrest, and increasing intracellular ROS levels (Ma et al., 2024a). The review aims to exhibit the different mechanisms of ART against tumors, such as inhibiting proliferation and inducing apoptosis, and the latest research progress in different tumors elucidating the anti-tumor mechanism of ART, and lay a theoretical foundation for further development of ART therapy for cancer.
2 Discovery of ART and its clinical application
Artemisia annua is an anti-fever herbal medicine first described in traditional Chinese medicine 1,000 years ago. Artemisinin (ARS), the extract of Artemisia annua, and its derivatives (DHA, artemether, and ART) have been used for the treatment of malaria with substantial efficacy (Zhang T. et al., 2020). As a classic antimalarial drug, ART is widely used to treat malaria caused by the multidrug-resistant strains of Plasmodium falciparum (Adebayo et al., 2020) for its advantages of high potency, low toxicity (Shi et al., 2022), hydrophilic properties, better bioavailability than ARS (Markowitsch et al., 2020), and good tolerance without side effects (Mancuso et al., 2021b), which has broad development prospects. According to the results of relevant experiments, ART inhibits the growth and survival of many types of cancer including leukemia, melanoma cancer, bladder cancer, ovarian cancer, cervical cancer, breast cancer, colon cancer, head and neck tumors, hepatocellular carcinoma, prostate cancer, and multiple myeloma (Hao et al., 2020; Ma and Fei, 2020; Wen et al., 2024), etc. The role of ART against different cancers along with cell proliferation, apoptosis, western and IC50 can be abserved from Table 1. For example, artesunate induces a decrease in Rb and phosphorylated Rb levels and thus promotes Head and neck squamous cell carcinoma (HNSCC) cell apoptosis (Okamoto et al., 2023). ART induces autophagy of tumor cells by activating AMPK-mTOR axis, which plays an anti-glioblastoma multiforme (GBM) role (Ding et al., 2023). Recent studies on the anti-tumor mechanism of ART have shown that ART produces ROS (Zhao P. H. et al., 2020) and causes apoptosis of cancer cells (Yin et al., 2020). Another study reveals that ART elevates the apoptotic rate and suppresses C918 cell (choroidal melanoma cells of the human eye) viability by regulating the MALAT1/YAP signaling pathway (Jiu et al., 2021). In addition, ART inhibits tumor invasion and metastasis by inhibiting angiogenesis and vasculogenic mimicry (VM) formation (Geng et al., 2022). ART has been shown to be safe (Augustin et al., 2020) and effective for clinical use, moreover, it has a wider range of potential clinical applications than originally hypothesized because of its greater biological activity (Yang et al., 2021).
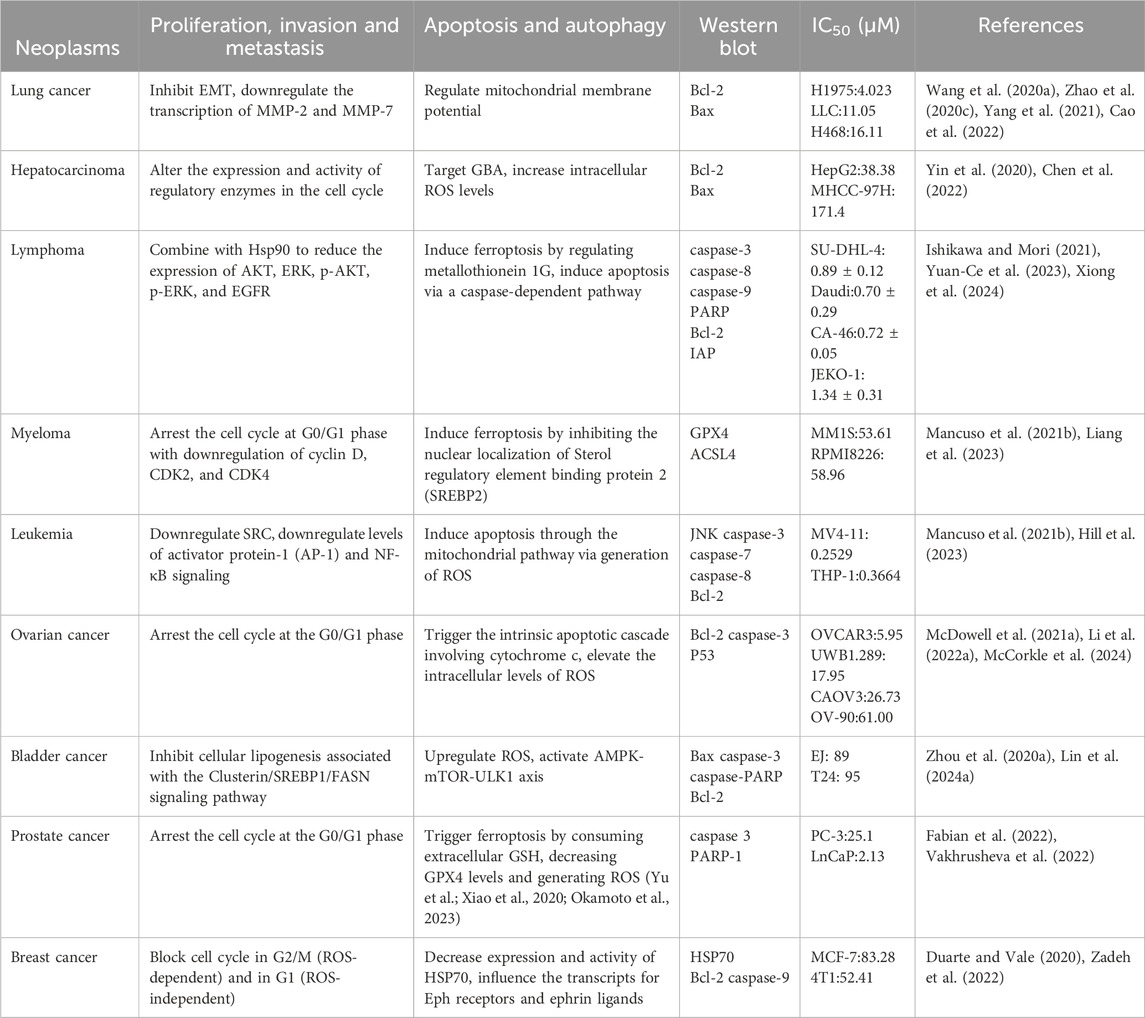
Table 1. The role of ART against different cancer along with other activities (cell proliferation/apoptosis/Western blot/IC50).
3 Antineoplastic mechanism of ART
The main mechanism of ARS and its derivatives (ART, artemether, DHA) toward leukemia, multiple myeloma, and lymphoma cells comprises oxidative stress response, inhibition of proliferation, induction of various types of cell death as apoptosis, autophagy, ferroptosis, inhibition of angiogenesis, and signal transducers, such as NF-κB, MYC, amongst others (Mancuso et al., 2021b). Existing basic and clinical studies have shown that the pharmacological mechanism of ART therapy for cancer is embodied in the inhibition of tumor cell proliferation, migration and invasion (Xu et al., 2022), induction of tumor cell apoptosis and autophagy (Zhao F. G. et al., 2020), regulation of cell signal transduction (Zeng et al., 2022), inhibition of tumor angiogenesis (Lu et al., 2022b) and others (Table 2).
3.1 Inhibition of tumor cell proliferation, invasion and metastasis
Studies have shown that ART can inhibit the proliferation of tumor cells by inhibiting or activating certain signal pathways (Antoszczak et al., 2020). ART causes mitochondrial dysfunction to further activate AMPK and suppress Akt/mTOR (Xiao et al., 2020). ART induces apoptosis in thyroid cancer cells and inhibits their proliferation and migration by inhibiting the PI3K/AKT/FKHR signal pathway (Xu et al., 2022). ART induces apoptosis of leukemia cells and inhibits the growth and stemness of transplanted tumors via the suppression of the MEK/ERK and PI3K/Akt pathways (Chen et al., 2020). ART evidently attenuates the migration, invasion and proliferation of cutaneous squamous cell carcinoma (CSCC) cells, which may be intensely related to PI3K/AKT pathway repression (Huang X. Y. et al., 2022). Another study proves that DHA and ART inhibit the growth of non-small cell lung cancer (NSCLC) via prohibiting cancer cell aerobic glycolysis through ERK/c-Myc pathway (Zhang Y. X. et al., 2022). ART can also inhibit cell proliferation, invasion and metastasis by affecting protein and enzyme expression. For instance, ART induces a significant downregulation of cyclin-dependent kinase-2 (CDK2), CDK4, cyclin D1, and cyclin E1 at various levels and then causes apoptosis, which impairs normal liver cell proliferation by inducing G0/G1 cell cycle arrest and apoptosis (Yin et al., 2020). ART combined with cisplatin (CIS) exerts anticancer effects on A549 cells by influencing the expression of Bcl-2, Box, p-P53, Caspase-3/7, Caspase-9, Cyclin Bl, P34, P21 (Li W. et al., 2021; Liu W. et al., 2023). The antimigration activity of ART is mediated by inhibition of BTBD7 mRNA expression while BTBD7 was found highly expressed in tumor tissues of NSCLC patients (Wang J. S. et al., 2020). ART may suppress the proliferation, migration and invasion of A549 and H1299 cells and induce their apoptosis by decreasing the expressions of human antigen R and matrix metalloproteinase-9 (MMP-9) proteins (Hu et al., 2022). The results obtained from another study demonstrate that the anticancer activity of ART occurs via STAT3 pathway and its target proteins (Berkoz et al., 2021). In addition, ART inhibits intestinal tumorigenesis by inhibiting Wnt signal pathway (Hamoya et al., 2021).
3.2 Induction of tumor cell apoptosis and autophagy
ART evokes ferroptosis, an iron-dependent cell death caused by ROS formation (Olivo et al., 2022; Huang et al., 2023). Accordingly, ferroptotic effects have been demonstrated in ART-induced head and neck tumor cells, pancreatic cancer cells, liver cancer cells (Markowitsch et al., 2020), ovarian serous carcinoma cells (Koike et al., 2022) and glioblastoma cells (Song et al., 2022). ARS derivatives have been shown to have anti-NSCLC activity through induction of ROS-dependent apoptosis/ferroptosis (Zhang Q. T. et al., 2021). ART may induce apoptosis and cell cycle arrest to inhibit cell proliferation, and regulate autophagy and ferroptosis via impairing the STAT3 signaling pathway in diffuse large B cell lymphoma (DLBCL) cells (Chen et al., 2021). ART targets oral tongue squamous cell carcinoma via mitochondrial dysfunction-dependent oxidative damage (Xiao et al., 2020). ART treatment causes significant growth inhibition and apoptosis in A549 cells (Zhang W. W. et al., 2022) and induces apoptosis in breast cancer cells as a HSP70 ATPase activity inhibitor (Pirali et al., 2020). ART has an anti-esophageal cancer effect by inhibiting aerobic glycolysis (Jin et al., 2022). Furthermore, ART induces autophagy dependent apoptosis through upregulating ROS and activating AMPK-mTOR-ULK1 axis in human bladder cancer cells (Zhou X. J. et al., 2020). The researchers demonstrate that high GBA levels over activated autophagic flux, accelerates the rate at which cellular material may be degraded and recycled in balanced, healthy cells. This disturbance enables liver cancer to progress while ART can suppress GBA expression levels and restore normal autophagic flux, boosting the drug’s anticancer activity (Chen et al., 2022).
3.3 Inhibition of tumor angiogenesis
The mechanisms underlying tumor angiogenesis and VM formation involve hypoxia, EMT, and activation of tumor-associated fibroblasts and tumor-associated macrophages. Many molecules participate in one or more of these processes that regulate tumor angiogenesis, such as vascular endothelial growth factor (VEGF), MMPs, VE-cadherin, and non-coding RNAs (Geng et al., 2022) that emerged recently (Figure 1). Further, ART inhibits melanoma vasculogenic mimicry by inhibiting the HIF-1α/VEGF/PDGF pathway (Ma et al., 2024b). ART and other artemisinin derivatives have been identified as anti-cancer agents due to their anti-proliferative, anti-angiogenic, and anti-inflammatory properties (Zhong et al., 2021). ART also inhibits STAT3 and Src activations and STAT3 related protein expressions. The upregulated expressions of STAT3 related protein by STAT3, play positive roles in melanoma metastasis through promoting cell invasion and angiogenesis (Berkoz et al., 2021). Currently, anti-angiogenesis targeting VEGFR-2 has been considered as an important strategy for cancer therapy (Lu et al., 2022c). ART inhibits choroidal melanoma cell growth through the STAT3/AKT signaling pathway (Yao et al., 2024b). Furthermore, the synergistic effect of ART and sorafenib (SOR) can inhibit non-Hodgkin lymphoma (NHL) cell viability and have anti-angiogenic activity. Further studies showed that gene inhibition of STAT3 could promote iron apoptosis and cell apoptosis induced by ART/SOR (Chen Y. et al., 2023).
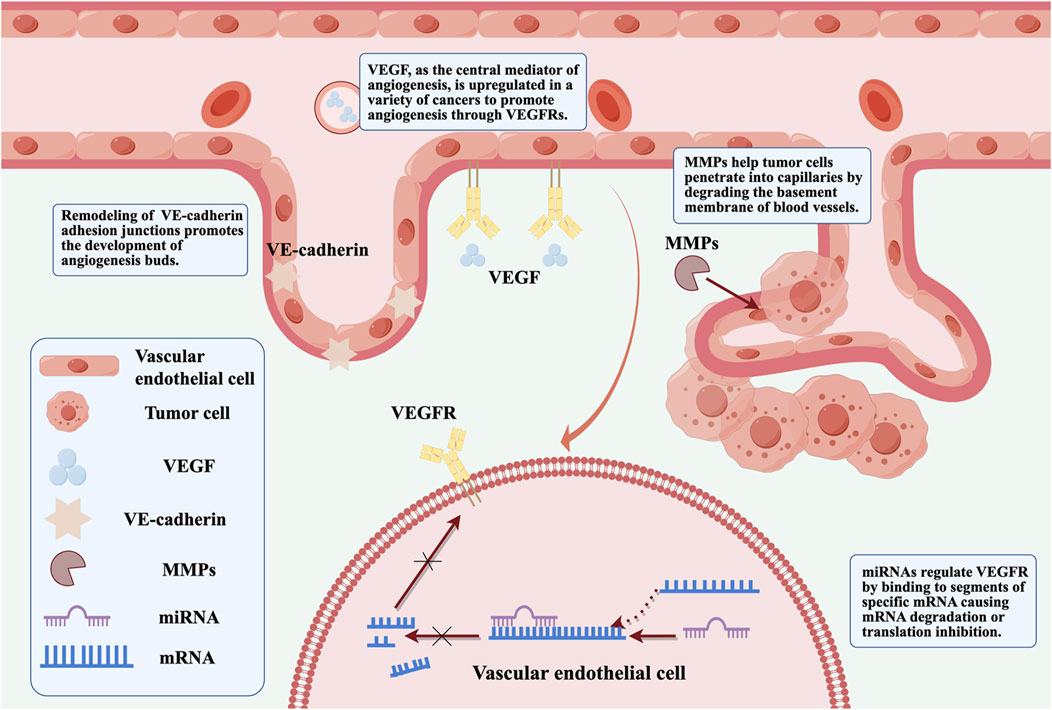
Figure 1. VEGF, MMPs, VE-cadherin and non-coding RNA are involved in regulating the specific mechanisms of tumor angiogenesis (By Figdraw).
3.4 Affecting the tumor microenvironment
The tumor microenvironment refers to the non-cancerous cells and the molecular components produced by the tumor. The interaction between tumor cells and tumor microenvironment plays an important role in tumor genesis, development, metastasis and response to therapy, which has attracted wide attention (Xiao and Yu, 2021). DHA (a metabolite produced in the liver from ART and artemether) regulates the crosstalk between autophagy and IFI16/caspase-1 inflammasome, which inhibits IL-1β production in tumor microenvironment (Shi et al., 2020). Furthermore, nanozyme-like single-atom catalyst combined with ART produces abundant cell cytotoxic radicals in tumor microenvironment (TME) for inhibiting tumor growth (Lv et al., 2023). Monocytes are components of the tumor microenvironment related to cancer progression and immune escape. ART induced changes in the monocyte phenotype are mediated by JAK2/STAT3 downregulation (Mancuso et al., 2021c).
3.5 Modulating immune response
ART has an immunomodulatory effect on various immune cells and cytokines of the immune system, also it shows different regulatory effects in different immune states (Lin et al., 2022). Flagella of tumor-targeting bacteria trigger local hemorrhage to reprogram tumor-associated macrophages for improved anti-tumor therapy (Xu et al., 2023). Other related studies report for the first time about the anti-complement bioactivities of ART and suggest a potential therapeutic benefit of ART in complement-related human diseases (Song et al., 2021). ART protects immunosuppression mice induced by glucocorticoids via enhancing pro-inflammatory cytokines release and bacterial clearance, and does indeed demonstrate to possess immunomodulatory effects (Wang et al., 2021). ART suppresses TAZ/PD-L1–induced T-cell growth inhibition in vitro and enhances anti-tumor immunity by recruiting infiltrating CD8+ T-cells in syngeneic mouse models (Cao et al., 2022). NK cells can eliminate virus-infected cells and tumor cells nonspecifically (Lin et al., 2022) while ART is able to enhance the cytotoxicity of NK92 cells (Zhang J. et al., 2021; Lin et al., 2022).
3.6 Overcoming drug resistance in cancer cells
Overcoming drug resistance and seeking new therapeutic strategies are the main focus of tumor research. Natural products serve as effective substances against drug resistance because of their diverse chemical structures and pharmacological effects. Their main mechanisms for reversing resistance include regulating proteins involved in resistance, targeting non-apoptotic cell death, and inducing other types of non-apoptotic cell death. Signaling pathways associated with tumor resistance include epidermal growth factor receptor (EGFR), renin-angiotensin system (Ras), phosphatidylinositol-3 kinase/protein kinase B (PI3K/Akt), Wnt, Notch, transforming growth factor-β (TGF-β) and their specific natural product signaling pathway inhibitors. This has implications for how to prevent drug resistance to cancer treatment (Yang et al., 2022). Recent evidence shows that lysosomal function is associated with drug resistance of cancer cells. The results suggest that ART or other inhibitors of lysosomal function would be potential in the treatment of cancer cells with drug resistance caused by the enhanced lysosomal function (Li Z. et al., 2021). ART promotes anti-tumor immunity and overcomes EGFR-TKI resistance in non-small-cell lung cancer by enhancing oncogenic TAZ degradation (Cao et al., 2022). In addition, ART is effective against chemoresistant anaplastic thyroid carcinoma by targeting mitochondrial metabolism (Ma and Fei, 2020). Most patients with advanced HCC develop resistance to sorafenib early during treatment. While ART or ginsenoside Rg3 (a main bioactive triterpenoid saponin of red ginseng) in combination with ART can be used to overcome resistance to Sorafenib in hepatocellular carcinoma cells (He et al., 2021; Yao et al., 2022). ART significantly inhibits proliferative and metabolic aspects of cisplatin-sensitive and cisplatin-resistant bladder cancer (BCa) cells, it may hold potential in treating advanced and therapy-resistant BCa (Zhao F. G. et al., 2020). ART reverses the resistance of AML cells to AraC by blocking the JAK/STAT3 signaling pathway, and the combination of ART and cytarabine significantly reduced the proliferation of AML cells and increased its apoptosis rate (Su et al., 2023). ART targeting Noxa/Bim/Mcl-1/p-Chk1 axis improves drug resistance of venetoclax combined with cytarabine in AML, providing a new triple therapy for AML treatment (Zhang J. et al., 2022). ART mitigates sorafenib resistance in hepatocellular carcinoma (HCC) patients by exacerbating AFAP1L2-SRC-FUNDC1 axis-dependent mitochondrial autophagy (Ma Z. et al., 2024) (Figure 2).
4 ART against different types of neoplasm
According to the latest estimates by the International Agency for Research on Cancer (IARC) through 2022, lung cancer was the most common cancer, followed by female breast, colorectal, prostate and stomach cancers. Lung cancer was also the leading cause of cancer death, followed by colorectal, liver, and female breast and stomach cancers. Broken down by sex, breast cancer and lung cancer were the most common cancers in women and men, respectively (Bray et al., 2024). Cancer is a major social, public health and economic problem of the 21st century. In the following, we focus on the role of artesunate in more than a dozen cancers, and present the latest trends and potential prospects for its prevention and control of tumors (Table 3; Figures 3, 4).
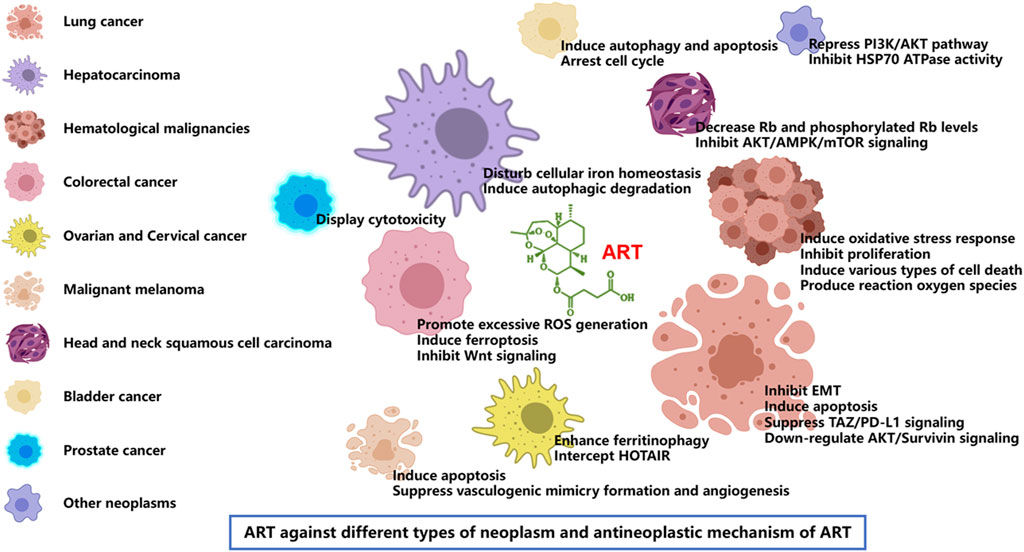
Figure 3. Artesunate inhibits growth and survival of many types of cancer including lung cancer, hepatocarcinoma, hematological malignancies, colorectal cancer, ovarian cancer, cervical cancer, malignant melanoma, head and neck tumor, bladder cancer, and prostate cancer. ART has the same or different mechanisms of action against different tumors, including the inhibition of tumor cell proliferation, migration and invasion [22], induction of tumor cell apoptosis and autophagy [23], regulation of cell signal transduction [24], inhibition of tumor angiogenesis [25] and many other aspects.
4.1 Lung cancer
Lung cancer is the main cause of cancer-related deaths around the world (Sherry, 2022). Recent studies have found that ART has significant therapeutic potential in multiple respiratory diseases (Zhang et al., 2022b). Lung cancer, the most common malignant tumor, is classified into NSCLC and small cell lung cancer (SCLC). ART was involved in inhibiting the epidermal interstitial transformation (EMT) of NSCLC cells by up-regulating the expression of epithelial marker E-cadherin and inhibiting the protein and mRNA levels of mesenchymal markers N-cadherin, vimentin and FN1 in A549 and H1975 cells (Wang J. S. et al., 2020). ART suppresses lung cancer cells growth by regulating mitochondrial membrane potential, inducing apoptosis (Zhao et al., 2020c) and down-regulating the AKT/Survivin Signaling Pathway (Zhang W. W. et al., 2022). In addition, ART enhances anti-tumor immunity and overcomes EGFR-TKI resistance in NSCLC at least in part by suppressing TAZ/PD-L1 signaling (Cao et al., 2022). Results have demonstrated that the ART-loaded PLGA porous microsphere could achieve excellent anti-cancer efficacy, providing a potential approach for NSCLC treatment via the pulmonary administration (Xiong et al., 2021). In addition, spray-dried micelles containing artesunate have the potential to be used as a dry powder formulation for inhalation in the treatment of lung cancer (Somavarapu et al., 2023).
4.2 Hepatocarcinoma
Hepatocellular carcinoma (HCC), one of the most common cancers, causes the fourth cancer-related deaths worldwide (Wang M. Y. et al., 2020). An effective strategy for HCC therapy is the perturbation of iron metabolism. ART regulates the unstable iron pool (LIP) and effectively induces ROS-dependent cell death in a variety of HCC cells, showing anticancer activity (Jiang et al., 2021). Glucosylceramidase (GBA) is required for autophagic degradation, and a preclinical study identified GBA as one of the direct targets of ART, which may have promising potential to inhibit lysosomal autophagy for HCC therapy (Chen et al., 2022). Another research shows that ART alone inhibits the proliferation of five HCC cell lines in a dose-dependent manner, and sorafenib combined with ART exert a synergistic anti-proliferation effect and induced synergistic apoptosis in HCC cell lines (Yao et al., 2020). The synergistic effects include ferroptosis induction (Li Z. J. et al., 2021), apoptosis induction, cell migration inhibition and anti-angiogenesis activity.
4.3 Lymphoma, multiple myeloma and leukemia
ARS and its derivatives (ART, DHA, artemether) act in a multi-specific manner against hematological malignancies, for example, lymphoma, multiple myeloma, and leukemia. The main mechanism of ARTs against different types of hematological malignancies comprises oxidative stress response, inhibition of proliferation, induction of various types of cell death (apoptosis, autophagy, ferroptosis, inhibition of angiogenesis), production of reaction oxygen species, induction of differentiation, and signal transducers, amongst others (Li et al., 2020; Mancuso et al., 2021b). ART is effective against adult T-cell leukemia/lymphoma by blocking G1 and/or G2/M phases, reducing the expression of cyclin-dependent kinases 1/2/4/6, cyclin B1/D2/E, and c-Myc, and increasing the expression of p21 (Ishikawa et al., 2020). ART exhibits cytotoxic effects in primary effusion lymphoma (Ishikawa and Mori, 2021) and induces apoptosis, autophagy and ferroptosis in diffuse large B cell lymphoma cells by impairing STAT3 signaling (Chen et al., 2021; Chen et al., 2023a). Beyond that, ART combined with arsenite inhibits the growth of multiple myeloma cells through the PI3K/AKT signaling pathway (Hu G.-F. et al., 2021). Leukemia is a hematological malignancy originated from the bone marrow (Ng et al., 2021). ART induces apoptosis and inhibits the proliferation, stemness, and tumorigenesis of leukemia (Chen et al., 2020). ART converts monocytes, a component of the tumor microenvironment associated with cancer progression and immune escape, into an inflammatory phenotype with the ability to kill leukemia cells (Mancuso et al., 2021c). Also, artesunate has significant anti-leukemia effects in mice by targeting ROS/Bim and TFRC/Fe2+ pathways (Liu et al., 2023). ART induces endoplasmic reticulum (ER) stress in leukemia cells eventually led to apoptosis (Mancuso et al., 2021a). Venetoclax plus cytarabine therapy is approved for elderly AML patients. Furthermore, ART induces synergistic apoptosis with venetoclax/cytarabine/daunorubicin accompanied (Li J.-Y. et al., 2022; Zhang et al., 2022b). Molecular docking findings reveal that artesunate is critically important in the therapy of AML due to its high affinity for the four primary disease targets, CASP3, EGFR, MAPK1, and STAT3 (Tao et al., 2023). Besides, ART induces cell death in pediatric AML cell lines through cytochrome c (Hill et al., 2023).
4.4 Colorectal cancer
Colorectal cancer has the second highest incidence of malignant tumors and is the fourth leading cause of cancer deaths in China (Cai et al., 2021). The combination of Arte and 5-FU significantly reduced the viability of cancer cells in vitro and synergistically inhibited the growth of CRC xenografts in vivo (Xia et al., 2023). ART has been reported to be a promising candidate for CRC treatment, which induces senescence and autophagy to inhibit cell proliferation in colorectal cancer by promoting excessive ROS generation (Huang Z. Y. et al., 2022). The study proposes that ART induces cytotoxicity in HCT116 colon cancer cells by suppressing the expression of survivin and partially by ferroptosis (Eitae and Park, 2020). Abnormal Wnt signaling pathways are known to lead to unexpected β-catenin nuclear translocation and promote T-cell factor/lymphoid enhancer factor (TCF/LEF) transcriptional activity, resulting in CRC progression. ART inhibits intestinal tumorigenesis through inhibiting Wnt signaling (Hamoya et al., 2021).
4.5 Ovarian cancer and cervical cancer
Ovarian cancer and cervical cancer are life-threatening diseases with a high mortality rate among women (Caird et al., 2022; Yuan et al., 2022). ART has preclinical activity in ovarian cancer that merits further investigation to treat ovarian cancer (McDowell et al., 2021). Enhancement of ferritinophagy is an important step involved in the mechanism of artesunate-induced ferroptosis, and ferritin heavy chain levels may contribute to the regulation of sensitivity in artesunate-induced ferroptosis in ovarian serous carcinoma cells (Koike et al., 2022). Synergetic delivery of ART and isosorbide 5-mononitrate with reduction-sensitive polymer nanoparticles for ovarian cancer chemotherapy (Li et al., 2022b). HOX transcript antisense RNA (HOTAIR), a trans-acting long non-coding RNA (lncRNA), plays an oncogenic role in cervical cancer by promoting cell proliferation, migration, invasion and autophagy, etc. Moreover, the blockade of HOTAIR by ART or propofol shows promise for further development of this lncRNA as a potential therapeutic target in cervical cancer (Zhou et al., 2020a). ART has a cytotoxic effect on squamous cells transformed by HPV. Self-administered vaginal ART inserts are safe and well-tolerated, which can be used at clinically effective doses to treat cervical intraepithelial neoplasia 2/3 (CIN2/3) (Trimble et al., 2020).
4.6 Malignant melanoma
Malignant melanoma is a malignant neoplasm of the skin and mucosal tissues (Zahir et al., 2021) characterized by a potential metastatic tumor of melanocytic origin. ART inhibits cellular proliferation of cancer cells by induction of apoptosis (Wroblewska-Luczka et al., 2023). The treatment shows decreased cellular migration, invasion, and colony formation in melanoma cells (Berkoz et al., 2021). ART suppresses choroidal melanoma (CM) vasculogenic mimicry formation and angiogenesis, while angiogenesis and VM are considered to be the main processes to ensure tumor blood supply during the proliferation and metastasis of CM (Geng et al., 2022). In uveal melanoma, ART elevates the apoptotic rate and suppresses C918 cell viability (Jiu et al., 2021). In addition, ART regulates the AKT/mTOR pathway by reducing the expression of Ang-1 in CM cells, thereby inhibiting the occurrence of CM tumors (Yao et al., 2024a). EphrinA3 (EFNA3) promotes CM cell growth and migration by activating the STAT3/AKT signaling pathway, while ART inhibits this process by down-regulating EFNA3 (Yao et al., 2024a). Meanwhile, ART has played a role in suppressing choroidal melanoma by regulating the HIF-1α/VEGF/PDGF pathway (Ma et al., 2024b).
4.7 Head and neck squamous cell carcinoma
Artesunate and cisplatin synergistically inhibit HNSCC cell growth and promote apoptosis with artesunate-induced decreases in Rb and phosphorylated Rb levels (Okamoto et al., 2023). Oral squamous cell carcinoma, the most common type of oral cancer, affects more than 275,000 people per year worldwide (Pena-Oyarzun et al., 2020). ARS suppresses tumor growth and induces vascular normalization in oral squamous cell carcinoma via inhibition of macrophage migration inhibitory factor (Ding et al., 2019; Yu et al., 2024). Mitochondrial metabolism has recently gained attention as a promising therapeutic strategy in cancer. ART targets oral tongue squamous cell carcinoma via mitochondrial dysfunction-dependent oxidative damage and Akt/AMPK/mTOR inhibition (Xiao et al., 2020).
4.8 Bladder cancer
Urinary bladder cancer is a common urological cancer (Tse et al., 2021) and is the 10th most common cancer type in the world (Bilim et al., 2022). ART can inhibit the viability, proliferation and migration of bladder cancer cells, as well as induce autophagy in a time and dose dependent manner. In addition, ART induced apoptosis of bladder cancer cells by up-regulating ROS and activating AMPK-mTOR-ULK1 pathway (Zhou X. J. et al., 2020). ART impairs growth in cisplatin-resistant bladder cancer cells by cell cycle arrest, apoptosis and autophagy induction, which may hold potential in treating advanced and therapy-resistant bladder cancer (Zhao F. G. et al., 2020). ART induces G2/M cell cycle arrest in HT 1376 and BFTC 909 cell lines, thereby inducing apoptosis and REDOX imbalance, and may be a candidate drug for the treatment of bladder cancer in concert with cisplatin (Chen S. Y. et al., 2023). A new artesunate-metformin dimer triazine derivative AM2 is designed and synthesized by coupling artesunate with metformin. AM2 inhibits the growth of bladder cancer cells T24 by inhibiting cellular adipogenesis associated with the Clusterin/SREBP1/FASN signaling pathway (Lin et al., 2024).
4.9 Prostate cancer
Prostate cancer (PC), a malignant tumor occurring in the male prostate, is the second leading cause of cancer-related deaths in men. The lesions have the characteristics of small size and blurry outline (Qian Y. J. et al., 2021). Combining ART with PTX displays cytotoxicity regardless of the type of prostate cancer cell line. This may offer a promising new therapeutic option for the treatment of metastatic hormone-refractory PC (Adedeji et al., 2020; Fabian et al., 2022). ART induced apoptosis of parent and DX-resistant DU145 cells by increasing ROS, indicating that ART inhibited the growth of docetaxel-resistant PC cells (Vakhrusheva et al., 2022).
4.10 Other neoplasms
ART evidently attenuates the migration, invasion and proliferation, lessened cell numbers at G2/M phase and triggers apoptosis of CSCC cells, which may be intensely related to the PI3K/AKT pathway repression (Huang X. Y. et al., 2022). ART induces the death of breast cancer cell lines 4T1 and MCF-7 by inhibiting the expression of HSP70 and Bcl-2 (Pirali et al., 2020; Svolacchia et al., 2023). ART targets HK1, promotes the degradation of HK1, reduces the expression of HIF-1α and PKM2, which are key glycolytic enzymes, and plays an anti-EC role (Jin et al., 2022). Furthermore, ART can reverse gemcitabine (dFdC) resistance in combination with dFdC in dFdC-resistant Panc-1 cells in vitro (Yao et al., 2022b). ART therapy significantly increases the cytotoxicity of Sunitinib-resistant RCC cells and inhibited proliferation and clonal growth. ART inhibits the growth of KAKI-1, 786-O, and A-498 cell lines through G0/G1 phase arrest and significant regulation of cell cycle regulators, and inhibits the growth of KTCTL-26 through ROS production, ferroptosis, and metabolism (Markowitsch et al., 2020). The inhibition of ART on chemotherapy-sensitive (8505C and KAT-4) and drug-resistant (8505C-R and KAT-4-R) ATC cells is effective against chemotherapy-resistant anaplastic thyroid cancer by inhibiting mitochondrial function, leading to oxidative stress and damage (Ma and Fei, 2020). ART inhibits apoptosis, proliferation and migration of thyroid cancer cells by inhibiting the PI3K/AKT/FKHR signaling pathway (Xu et al., 2022). ART exerts anti-prolactinoma activity by inducing G0/G1 phase arrest and cell apoptosis, thereby inhibiting mitochondrial metabolism and inducing cell apoptosis (Zhang W. Y. et al., 2020). ART influences iron apoptosis by regulating iron homeostasis and p38 and ERK signaling pathways. These findings support the role of ART in inducing ferroptosis through this pathway in glioblastoma (Song et al., 2022). In breast cancer, artesunate also induces apoptosis in cancer cells (Wen et al., 2024).
5 Clinical evidence of ART as an anti-cancer therapy
At present, artesunate has been gradually used in clinical studies to treat tumors, and has become a promising strategy for the treatment of cancer. In glioblastoma cells, artesunate acts as a supplement for cancer treatment. Clinical trials have shown that artesunate itself is cytotoxic and enhances the cytotoxicity of temozolomide, and therefore has the potential to enhance the therapeutic efficacy of glioblastoma (Strik et al., 2024). Artesunate is effective, safe and well tolerated for the treatment of cervical intraepithelial neoplasia 2/3 (CIN2/3) (Trimble et al., 2020). Phase I clinical trials evaluated artesunate suppositories as effective in treating HPV-infected cells with cytotoxic effects while having minimal effects on healthy cells (Fang et al., 2023). Artesunate has shown anticancer activity both in vitro and in vivo against hematological malignancies in a multispecific manner. The main mechanisms of its action on leukemia, multiple myeloma and lymphoma cells include oxidative stress response, inhibition of proliferation and induction of various types of cell death (Li et al., 2020; Mancuso et al., 2021b). Because artesunate drugs are highly effective and well tolerated without side effects, they could be applied in the future as anti-tumor therapy alone or in combination with standard chemotherapy after further clinical trials in various tumors are completed.
6 Conclusion and perspectives
Cancer is one of the most important and common public health problems on Earth endangering human health. And its incidence rates continue to rise. As anti-tumor drugs have always been the most common methods for treating cancers, searching for new anti-tumor agents is of great significance (Sekeroglu and Tuncal, 2021; He et al., 2023). The use of herbal products is booming all over the world because of being believed as safer than conventional drugs and free of side effects (Mwankuna et al., 2023). Chinese herbal medicine (CHM) has long been applied in the clinic due to its advantages of low toxicity and polypharmacology (Li et al., 2022). CHM plays a positive role in regulating patients' immune system, which helps cancer patients to fight against cancer itself and finally improves patients' life quality (Wang S. M. et al., 2020). ART, a sesquiterpene lactone endoperoxide isolated from Chinese herbal medicine, displays excellent anti-tumor and anti-inflammatory activity (Lu W. J. et al., 2022; Wang et al., 2022). It is safe, efficacious and well-tolerated anti-malarial (Savargaonkar et al., 2020). ART possesses profound cytotoxic activity against tumor cells (Khanal, 2021), which brings new hope for the treatment of diseases. The combination of ART and other anti-tumor drugs may provide a new idea for the treatment of tumors in the future (Duarte and Vale, 2020). In this paper, the anti-tumor mechanism of artesunate and its corresponding signaling pathway are reviewed, and the anti-tumor mechanism of artesunate in different types of tumors is analyzed and explained. In addition to this, we also provide a separate explanation of the anti-tumor mechanism of artesunate in different types of tumors. It provides a comprehensive reference for further study of the anti-tumor mechanism of artesunate, and is conducive to expanding its clinical application. In conclusion, the efficacy of ART as an anticancer agent has been demonstrated in multiple tumor types. However, the anti-tumor mechanism of ART is not completely clear, so it needs to be further studied to obtain more theoretical support and experimental basis.
Author contributions
XF: Conceptualization, Methodology, Software, Visualization, Writing–original draft, Writing–review and editing. YY: Visualization, Methodology, Software, Writing–review and editing. YL: Methodology, Software, Writing–review and editing. YS: Conceptualization, Funding acquisition, Writing–review and editing. BL: Conceptualization, Supervision, Validation, Writing–review and editing.
Funding
The author(s) declare that financial support was received for the research, authorship, and/or publication of this article. This research was funded by Natural Science Foundation of Jilin Province (YDZJ202401228ZYTS), Key Research and Development (International Science and Technology Cooperation) Project from Jilin Provincial Department of Science and Technology (2025GH) and Technology Innovation Guidance Program Project from Changchun Municipal Bureau of Science and Technology (No. 21ST01).
Conflict of interest
The authors declare that the research was conducted in the absence of any commercial or financial relationships that could be construed as a potential conflict of interest.
Publisher’s note
All claims expressed in this article are solely those of the authors and do not necessarily represent those of their affiliated organizations, or those of the publisher, the editors and the reviewers. Any product that may be evaluated in this article, or claim that may be made by its manufacturer, is not guaranteed or endorsed by the publisher.
Supplementary material
The Supplementary Material for this article can be found online at: https://www.frontiersin.org/articles/10.3389/fphar.2024.1483049/full#supplementary-material
References
Adebayo, J. O., Tijjani, H., Adegunloye, A. P., Ishola, A. A., Balogun, E. A., and Malomo, S. O. (2020). Enhancing the antimalarial activity of artesunate. Parasitol. Res. 119 (9), 2749–2764. doi:10.1007/s00436-020-06786-1
Adedeji, D., Fabian, J., Adedeji, A., and Payne, G. (2020). Effects of artesunate with paclitaxel on the proliferation and morphology of androgen-sensitive (LNCaP), androgen-insensitive (PC-3) human prostate cancer cell lines and normal epithelial prostate cell line (RWPE-1). Faseb J. 34, 1. doi:10.1096/fasebj.2020.34.s1.09157
Alagbonsi, A. I., Salman, T. M., Sulaiman, S. O., Adedini, K. A., and Kebu, S. (2021). Possible mechanisms of the hypoglycaemic effect of artesunate: gender implication. Metab. open 10, 100087. doi:10.1016/j.metop.2021.100087
Antoszczak, M., Markowska, A., Markowska, J., and Huczynski, A. (2020). Old wine in new bottles: drug repurposing in oncology. Eur. J. Pharmacol. 866, 172784. doi:10.1016/j.ejphar.2019.172784
Augustin, Y., Staines, H. M., and Krishna, S. (2020). Artemisinins as a novel anti-cancer therapy: targeting a global cancer pandemic through drug repurposing. Pharmacol. and Ther. 216, 107706. doi:10.1016/j.pharmthera.2020.107706
Berkoz, M., Ozkan-Yilmaz, F., Ozluer-Hunt, A., Krosniak, M., Turkmen, O., Korkmaz, D., et al. (2021). Artesunate inhibits melanoma progression in vitro via suppressing STAT3 signaling pathway. Pharmacol. Rep. 73 (2), 650–663. doi:10.1007/s43440-021-00230-6
Bilim, V., Kuroki, H., Shirono, Y., Murata, M., Hiruma, K., and Tomita, Y. (2022). Advanced bladder cancer: changing the treatment landscape. J. Personalized Med. 12 (10), 1745. doi:10.3390/jpm12101745
Bray, F., Laversanne, M., Sung, H., Ferlay, J., Siegel, R. L., Soerjomataram, I., et al. (2024). Global cancer statistics 2022: GLOBOCAN estimates of incidence and mortality worldwide for 36 cancers in 185 countries. CA Cancer J. Clin. 74 (3), 229–263. doi:10.3322/caac.21834
Cai, Y. W., Dong, F. F., Shi, Y. H., Lu, L. Y., Chen, C., Lin, P., et al. (2021). Deep learning driven colorectal lesion detection in gastrointestinal endoscopic and pathological imaging. World J. Clin. Cases 9 (31), 9376–9385. doi:10.12998/wjcc.v9.i31.9376
Caird, H., Simkin, J., Smith, L., Van Niekerk, D., and Ogilvie, G. (2022). The path to eliminating cervical cancer in Canada: past, present and future directions. Curr. Oncol. 29 (2), 1117–1122. doi:10.3390/curroncol29020095
Cao, D., Chen, D., Xia, J. N., Wang, W. Y., Zhu, G. Y., Chen, L. W., et al. (2022). Artesunate promoted anti-tumor immunity and overcame EGFR-TKI resistance in non-small-cell lung cancer by enhancing oncogenic TAZ degradation. Biomed. Pharmacother. 155, 113705. doi:10.1016/j.biopha.2022.113705
Cao, W., Chen, H. D., Yu, Y. W., Li, N., and Chen, W. Q. (2021). Changing profiles of cancer burden worldwide and in China: a secondary analysis of the global cancer statistics 2020. Chin. Med. J. Engl. 134 (7), 783–791. doi:10.1097/cm9.0000000000001474
Chen, S. M., Gan, S. L., Han, L. J., Li, X., Xie, X. Q., Zou, D. B., et al. (2020). Artesunate induces apoptosis and inhibits the proliferation, stemness, and tumorigenesis of leukemia. Ann. Transl. Med. 8 (12), 767. doi:10.21037/atm-20-4558
Chen, S. Y., Chao, C. N., Huang, H. Y., Zhao, P. W., and Fang, C. Y. (2023a). Artesunate exhibits synergy with cisplatin and cytotoxicity for upper tract and bladder urothelial carcinoma cells. Anticancer Res. 43 (3), 1175–1184. doi:10.21873/anticanres.16263
Chen, W., Ma, Z., Yu, L., Mao, X., Ma, N., Guo, X., et al. (2022). Preclinical investigation of artesunate as a therapeutic agent for hepatocellular carcinoma via impairment of glucosylceramidase-mediated autophagic degradation. Exp. Mol. Med. 54 (9), 1536–1548. doi:10.1038/s12276-022-00780-6
Chen, Y., Tao, H., Wang, F., Wu, P., Gao, J., Zhang, X., et al. (2023b). Artesunate synergistically promotes sorafenib-induced apoptosis and ferroptosis in non-Hodgkin lymphoma cells through inhibition of the STAT3 pathway. Oncol. Rep. 50 (1), 147. doi:10.3892/or.2023.8584
Chen, Y. Y., Wang, F. J., Wu, P. Q., Gong, S. G., Gao, J., Tao, H., et al. (2021). Artesunate induces apoptosis, autophagy and ferroptosis in diffuse large B cell lymphoma cells by impairing STAT3 signaling. Cell. Signal. 88, 110167. doi:10.1016/j.cellsig.2021.110167
Ding, W., Liao, L., Liu, J., Zhao, J., Tang, Q., and Liao, Y. (2023). Lower dose of metformin combined with artesunate induced autophagy-dependent apoptosis of glioblastoma by activating ROS-AMPK-mTOR axis. Exp. Cell Res. 430 (1), 113691. doi:10.1016/j.yexcr.2023.113691
Ding, X., Yue, W., and Chen, H. (2019). Effect of artesunate on apoptosis and autophagy in tamoxifen resistant breast cancer cells (TAM-R). Transl. Cancer Res. 8 (5), 1863–1872. doi:10.21037/tcr.2019.08.41
Duarte, D., and Vale, N. (2020). New trends for antimalarial drugs: synergism between antineoplastics and antimalarials on breast cancer cells. Biomolecules 10 (12), 1623. doi:10.3390/biom10121623
Eitae, K. I. M., and Park, D.-G. (2020). YM155, specific survivin inhibitor, can enhance artesunate-induced cytotoxicity in HCT116 colon cancer cells. Korean J. Clin. Oncol. 16 (2), 131–137. doi:10.14216/kjco.20020
Fabian, J., Pierce, T., Brown, S., Payne, G., and Adedeji, D. (2022). Preliminary assessment of the combination studies of artesunate with paclitaxel on human prostate cancer cell proliferation. Faseb J. 36. doi:10.1096/fasebj.2022.36.S1.L8040
Fang, S. H., Plesa, M., Carchman, E. H., Cowell, N. A., Staudt, E., Twaroski, K. A., et al. (2023). A phase I study of intra-anal artesunate (suppositories) to treat anal high-grade squamous intraepithelial lesions. PLoS One 18 (12), e0295647. doi:10.1371/journal.pone.0295647
Gashe, F., Wynendaele, E., De Spiegeleer, B., and Suleman, S. (2022). Degradation kinetics of artesunate for the development of an ex-tempore intravenous injection. Malar. J. 21 (1), 256. doi:10.1186/s12936-022-04278-4
Geng, B. C., Zhu, Y. Z., Yuan, Y. Y., Bai, J. Y., Dou, Z. Z., Sui, A. H., et al. (2022). Artesunate suppresses choroidal melanoma vasculogenic mimicry formation and angiogenesis via the wnt/CaMKII signaling Axis. Front. Oncol. 11, 714646. doi:10.3389/fonc.2022.870805
Guan, L., Wang, H. Y., Xu, X. L., and Fan, H. H. (2023). Therapeutical utilization and repurposing of artemisinin and its derivatives: a narrative review. Adv. Biol. 7, e2300086. doi:10.1002/adbi.202300086
Hamoya, T., Fujii, G., Iizumi, Y., Narita, T., Komiya, M., Matsuzawa, Y., et al. (2021). Artesunate inhibits intestinal tumorigenesis through inhibiting wnt signaling. Carcinogenesis 42 (1), 148–158. doi:10.1093/carcin/bgaa084
Hao, D. L., Xie, R., and De, G. J. (2020). Erratum: pH-responsive artesunate polymer prodrugs with enhanced ablation effect on rodent xenograft colon cancer [corrigendum]. Int. J. Nanomedicine 15, 3717–3718. doi:10.2147/ijn.S260275
He, T., Zhou, F. L., Su, A. P., Zhang, Y. J., Xing, Z. C., Mi, L., et al. (2023). Brusatol: a potential sensitizing agent for cancer therapy from Brucea javanica. Biomed. and Pharmacother. 158, 114134. doi:10.1016/j.biopha.2022.114134
He, W. B., Huang, X. X., Berges, B. K., Wang, Y., An, N., Su, R. J., et al. (2021). Artesunate regulates neurite outgrowth inhibitor protein B receptor to overcome resistance to sorafenib in hepatocellular carcinoma cells. Front. Pharmacol. 12, 615889. doi:10.3389/fphar.2021.615889
Hill, K. S., Schuler, E. E., Ellingson, S. R., and Kolesar, J. M. (2023). Artesunate acts through cytochrome c to inhibit growth of pediatric AML cells. Sci. Rep. 13 (1), 22383. doi:10.1038/s41598-023-49928-y
Hu, G.-F., Dong, K., Dong, J.-F., Wang, Y., and Gao, W. (2021a). Effects of artesunate combined with arsenious acid on proliferation and apoptosis of multiple myeloma cells via PI3K/AKT signaling pathway. Zhongguo shi yan xue ye xue za zhi 29 (6), 1819–1824. doi:10.19746/j.cnki.issn.1009-2137.2021.06.022
Hu, P., Ni, C. Y., and Teng, P. (2022). Effects of artesunate on the malignant biological behaviors of non-small cell lung cancer in human and its mechanism. Bioengineered 13 (3), 6590–6599. doi:10.1080/21655979.2022.2042141
Hu, Y. J., Liu, M. Q., Qin, H. B., Lin, H. F., An, X. P., Shi, Z. L., et al. (2021b). Artemether, artesunate, arteannuin B, echinatin, licochalcone B and andrographolide effectively inhibit SARS-CoV-2 and related viruses in vitro. Front. Cell. Infect. Microbiol. 11, 680127. doi:10.3389/fcimb.2021.680127
Huang, Q.-F., Li, Y.-H., Huang, Z.-J., Jun, M., Wang, W., Chen, X.-L., et al. (2023). Artesunate carriers induced ferroptosis to overcome biological barriers for anti-cancer. Eur. J. Pharm. Biopharm. 190, 284–293. official journal of Arbeitsgemeinschaft fur Pharmazeutische Verfahrenstechnik e.V. doi:10.1016/j.ejpb.2023.07.014
Huang, X. Y., Zhang, S. Z., and Wang, W. X. (2022a). Artesunate restrains the malignant progression of human cutaneous squamous cell carcinoma cells via the suppression of the PI3K/AKT pathway. Tissue and Cell 76, 101789. doi:10.1016/j.tice.2022.101789
Huang, Z. Y., Gan, S., Zhuang, X. R., Chen, Y., Lu, L. L., Wang, Y., et al. (2022b). Artesunate inhibits the cell growth in colorectal cancer by promoting ROS-dependent cell senescence and autophagy. Cells 11 (16), 2472. doi:10.3390/cells11162472
Ishikawa, C., and Mori, N. (2021). The anti-malaria agent artesunate exhibits cytotoxic effects in primary effusion lymphoma. Invest New Drugs 39 (1), 111–121. doi:10.1007/s10637-020-00996-1
Ishikawa, C., Senba, M., and Mori, N. (2020). Evaluation of artesunate for the treatment of adult T-cell leukemia/lymphoma. Eur. J. Pharmacol. 872, 172953. doi:10.1016/j.ejphar.2020.172953
Jiang, Z. Y., Wang, Z. W., Chen, L., Zhang, C., Liao, F. Y., Wang, Y. W., et al. (2021). Artesunate induces ER-derived-ROS-mediated cell death by disrupting labile iron pool and iron redistribution in hepatocellular carcinoma cells. Am. J. Cancer Res. 11 (3), 691–+.
Jin, J., Guo, D. L., Wang, Y. Y., Jiao, W. P., Li, D. J., and He, Y. T. (2022). Artesunate inhibits the development of esophageal cancer by targeting HK1 to reduce glycolysis levels in areas with zinc deficiency. Front. Oncol. 12. doi:10.3389/fonc.2022.871483
Jiu, X. D., Liu, Y., and Wen, J. (2021). Artesunate combined with verteporfin inhibits uveal melanoma by regulation of the MALAT1/yes-associated protein signaling pathway. Oncol. Lett. 22 (2), 597. doi:10.3892/ol.2021.12858
Khanal, P. (2021). Antimalarial and anticancer properties of artesunate and other artemisinins: current development. Monatsh. Fur Chem. 152 (4), 387–400. doi:10.1007/s00706-021-02759-x
Koike, T., Takenaka, M., Suzuki, N., Ueda, Y., Mori, M., Hirayama, T., et al. (2022). Intracellular ferritin heavy chain plays the key role in artesunate-induced ferroptosis in ovarian serous carcinoma cells. J. Clin. Biochem. Nutr. 71 (1), 34–40. doi:10.3164/jcbn.21-82
Li G, G., Ling, M., Yu, K., Yang, W., Liu, Q., He, L., et al. (2022). Synergetic delivery of artesunate and isosorbide 5-mononitrate with reduction-sensitive polymer nanoparticles for ovarian cancer chemotherapy. J. Nanobiotechnology 20 (1), 471. doi:10.1186/s12951-022-01676-3
Li J.-Y, J.-Y., Xiong, X., Wang, D.-W., Zhang, X.-Y., Huang, C., Zou, L.-L., et al. (2022). The mechanism of artesunate combined with cytarabine and/or daunorubicin on the apoptosis of MV4-11 MLL-rearranged acute myeloid leukemia cell line. Zhongguo shi yan xue ye xue za zhi 30 (6), 1724–1729. doi:10.19746/j.cnki.issn.1009-2137.2022.06.015
Li, W., Ma, G. Z., Deng, Y. F., Wu, Q., Wang, Z., and Zhou, Q. H. (2021a). Artesunate exhibits synergistic anti-cancer effects with cisplatin on lung cancer A549 cells by inhibiting MAPK pathway. Gene 766, 145134. doi:10.1016/j.gene.2020.145134
Li, Y., Ma, P., Li, J., Wu, F., Guo, M., Zhou, E., et al. (2024a). Dihydroartemisinin restores the immunogenicity and enhances the anticancer immunosurveillance of cisplatin by activating the PERK/eIF2α pathway. Cell Biosci. 14 (1), 100. doi:10.1186/s13578-024-01254-0
Li, Y., Shan, N. N., and Sui, X. H. (2020). Research progress on artemisinin and its derivatives against hematological malignancies. Chin. J. Integr. Med. 26 (12), 947–955. doi:10.1007/s11655-019-3207-3
Li, Y., Sun, H., Bai, C., Hu, Y., Tang, J., Zhang, Y., et al. (2024b). Dihydroartemisinin inhibits tumor progress via blocking ROR1-induced STAT3-activation in non-small cell lung cancer. Int. Immunopharmacol. 133, 112157. doi:10.1016/j.intimp.2024.112157
Li, Z., Zhu, Y. T., Xiang, M., Qiu, J. L., Luo, S. Q., and Lin, F. (2021b). Enhanced lysosomal function is critical for paclitaxel resistance in cancer cells: reversed by artesunate. Acta Pharmacol. Sin. 42 (4), 624–632. doi:10.1038/s41401-020-0445-z
Li, Z. J., Dai, H. Q., Huang, X. W., Feng, J., Deng, J. H., Wang, Z. X., et al. (2021c). Artesunate synergizes with sorafenib to induce ferroptosis in hepatocellular carcinoma. Acta Pharmacol. Sin. 42 (2), 301–310. doi:10.1038/s41401-020-0478-3
Liang, L., Liu, Y., Wu, X., and Chen, Y. (2023). Artesunate induces ferroptosis by inhibiting the nuclear localization of SREBP2 in myeloma cells. Int. J. Med. Sci. 20 (12), 1535–1550. doi:10.7150/ijms.86409
Lin, L. X., Tang, Z. Q., Shi, Z. R., Guo, Q., and Xiong, H. (2022). New insights into artesunate as a pleiotropic regulator of innate and adaptive immune cells. J. Immunol. Res. 2022, 9591544. doi:10.1155/2022/9591544
Lin, P., Yang, X., Wang, L., Zou, X., Mu, L., Xu, C., et al. (2024. Novel artesunate-metformin conjugate inhibits bladder cancer cell growth associated with Clusterin/SREBP1/FASN signaling pathway. Korean J. Physiol. Pharmacol. 28 (3), 219–227. doi:10.4196/kjpp.2024.28.3.219
Liu, W., Yu, C. Y., Wang, M. M., He, Y. Y., Guo, Z. J., He, J., et al. (2023a). Discovery of platinum(IV)-Artesunate multiaction prodrugs as potent antitumor and antimalarial agents. J. Med. Chem. 66, 8066–8085. doi:10.1021/acs.jmedchem.3c00396
Liu, Y., Li, H., Luo, Z. H., Yu, Y., Yang, J. Z., Zhang, M., et al. (2023). Artesunate, a new antimalarial clinical drug, exhibits potent anti-AML activity by targeting the ROS/Bim and TFRC/Fe2+ pathways. Br. J. Pharmacol. 180, 701–720. doi:10.1111/bph.15986
Lu, W. J., Tsai, C. H., Chen, R. J., Huang, L. T., Chen, T. Y., Chen, L. C., et al. (2022a). Artesunate as a glycoprotein VI antagonist for preventing platelet activation and thrombus formation. Biomed. and Pharmacother. 153, 113531. doi:10.1016/j.biopha.2022.113531
Lu, X. H., Blatt, S., Dawood, M., Klauck, S. M., Fleischer, E., Kammerer, P. W., et al. (2022b). Novel artemisinin derivative FO8643 with anti-angiogenic activity inhibits growth and migration of cancer cells via VEGFR2 signaling. Eur. J. Pharmacol. 930, 175158. doi:10.1016/j.ejphar.2022.175158
Lu, X. H., Elbadawi, M., Blatt, S., Saeed, M. E. M., Xiao, X. L., Ma, X., et al. (2022c). Artemisinin derivative FO-ARS-123 as a novel VEGFR2 inhibitor suppresses angiogenesis, cell migration, and invasion. Chemico-Biological Interact. 365, 110062. doi:10.1016/j.cbi.2022.110062
Lv, Q., Chi, K., Shi, X., Liu, M., Li, X., Zhou, C., et al. (2023). Nanozyme-like single-atom catalyst combined with artesunate achieves photothermal-enhanced nanocatalytic therapy in the near-infrared biowindow. Acta biomater. 158, 686–697. doi:10.1016/j.actbio.2022.12.071
Ma, L., and Fei, H. H. (2020). Antimalarial drug artesunate is effective against chemoresistant anaplastic thyroid carcinoma via targeting mitochondrial metabolism. J. Bioenergetics Biomembr. 52 (2), 123–130. doi:10.1007/s10863-020-09824-w
Ma, Q. Y., Liu, Y. C., Zhang, Q., Yi, W. D., Sun, Y., Gao, X. D., et al. (2024a). Integrating network pharmacology, molecular docking and experimental verification to reveal the mechanism of artesunate in inhibiting choroidal melanoma. Front. Pharmacol. 15, 1448381. doi:10.3389/fphar.2024.1448381
Ma, Q. Y., Xu, X. Y., Zhu, Y. Z., Yao, N. N., Liu, Y. C., Gao, X. D., et al. (2024b). Artesunate inhibits vasculogenic mimicry in choroidal melanoma through HIF-1 α/VEGF/PDGF pathway. Acta histochem. 126 (5-7), 152174. doi:10.1016/j.acthis.2024.152174
Ma, Z., Chen, W., Liu, Y., Yu, L., Mao, X., Guo, X., et al. (2024c). Artesunate Sensitizes human hepatocellular carcinoma to sorafenib via exacerbating AFAP1L2-SRC-FUNDC1 axis-dependent mitophagy. Autophagy 20 (3), 541–556. doi:10.1080/15548627.2023.2261758
Ma, Z. W., Woon, C. Y. N., Liu, C. G., Cheng, J. T., You, M. L., Sethi, G., et al. (2021). Repurposing artemisinin and its derivatives as anticancer drugs: a chance or challenge? Front. Pharmacol. 12, 828856. doi:10.3389/fphar.2021.828856
Mancuso, R. I., Azambuja, J. H., Niemann, F. S., Congrains, A., Foglio, M. A., Rego, E. M., et al. (2021a). Artemisinins induce endoplasmic reticulum stress in acute leukaemia cells in vitro and in vivo. EJHaem 2 (4), 818–822. doi:10.1002/jha2.314
Mancuso, R. I., Foglio, M. A., and Olalla Saad, S. T. (2021b). Artemisinin-type drugs for the treatment of hematological malignancies. Cancer Chemother. Pharmacol. 87 (1), 1–22. doi:10.1007/s00280-020-04170-5
Mancuso, R. I., Saad, S. T. O., and Azambuja, J. H. (2021c). Artesunate switches monocytes to an inflammatory phenotype with the ability to kill leukemic cells. Int. J. Mol. Sci. 22 (2), 608. doi:10.3390/ijms22020608
Markowitsch, S. D., Schupp, P., Lauckner, J., Vakhrusheva, O., Slade, K. S., Mager, R., et al. (2020). Artesunate inhibits growth of sunitinib-resistant renal cell carcinoma cells through cell cycle arrest and induction of ferroptosis. Cancers 12 (11), 3150. doi:10.3390/cancers12113150
McCorkle, J. R., Ahn, R., Cao, C. D., Hill, K. S., Dietrich, C. S., and Kolesar, J. M. (2024). Antineoplastic drug synergy of artesunate with navitoclax in models of high-grade serous ovarian cancer. Cancers (Basel) 16 (7), 1321. doi:10.3390/cancers16071321
McDowell, A., Hill, K. S., McCorkle, J. R., Gorski, J., Zhang, Y. L., Salahudeen, A. A., et al. (2021). Preclinical evaluation of artesunate as an antineoplastic agent in ovarian cancer treatment. Diagnostics 11 (3), 395. doi:10.3390/diagnostics11030395
Mwankuna, C. J., Kiros, F., Mariki, E. E., Mabiki, F. P., Malebo, H. M., Mdegela, R. H., et al. (2023). Optimization of HPLC-MS/MS method for determination of antimalarial adulterants in herbal products. Anal. Sci. Int. J. Jpn. Soc. Anal. Chem. doi:10.1007/s44211-022-00255-8
Ng, M. G., Ng, K. Y., Koh, R. Y., and Chye, S. M. (2021). Potential role of melatonin in prevention and treatment of leukaemia. Hormone Mol. Biol. Clin. Investigation 42 (4), 445–461. doi:10.1515/hmbci-2021-0009
Okamoto, H., Yoshikawa, K., Shimada, A., Sano, R., Inukai, D., Yamanaka, S., et al. (2023). Artesunate and cisplatin synergistically inhibit HNSCC cell growth and promote apoptosis with artesunate-induced decreases in Rb and phosphorylated Rb levels. Oncol. Rep. 50 (2), 154. doi:10.3892/or.2023.8591
Olivo, E., La Chimia, M., Ceramella, J., Catalano, A., Chiaradonna, F., Sinicropi, M. S., et al. (2022). Moving beyond the tip of the iceberg: DJ-1 implications in cancer metabolism. Cells 11 (9), 1432. doi:10.3390/cells11091432
Pena-Oyarzun, D., Reyes, M., Hernandez-Caceres, M. P., Kretschmar, C., Morselli, E., Ramirez-Sarmiento, C. A., et al. (2020). Role of autophagy in the microenvironment of oral squamous cell carcinoma. Front. Oncol. 10, 602661. doi:10.3389/fonc.2020.602661
Pirali, M., Taheri, M., Zarei, S., Majidi, M., and Ghafouri, H. (2020). Artesunate, as a HSP70 ATPase activity inhibitor, induces apoptosis in breast cancer cells. Int. J. Biol. Macromol. 164, 3369–3375. doi:10.1016/j.ijbiomac.2020.08.198
Qian, Y., Xia, L., Wei, L., Li, D., and Jiang, W. W. (2021a). Artesunate inhibits Staphylococcus aureus biofilm formation by reducing alpha-toxin synthesis. Archives Microbiol. 203 (2), 707–717. doi:10.1007/s00203-020-02077-6
Qian, Y. J., Zhang, Z. Y., and Wang, B. (2021b). ProCDet: a new method for prostate cancer detection based on mr images. Ieee Access 9, 143495–143505. doi:10.1109/access.2021.3114733
Rao, Q., Yu, H., Li, R., He, B., Wang, Y., Guo, X., et al. (2024). Dihydroartemisinin inhibits angiogenesis in breast cancer via regulating VEGF and MMP-2/-9. Fundam. Clin. Pharmacol. 38 (1), 113–125. doi:10.1111/fcp.12941
Ruwizhi, N., Maseko, R. B., and Aderibigbe, B. A. (2022). Recent advances in the therapeutic efficacy of artesunate. Pharmaceutics 14 (3), 504. doi:10.3390/pharmaceutics14030504
Savargaonkar, D., Das, M. K., Verma, A., Mitra, J. K., Yadav, C. P., Srivastava, B., et al. (2020). Delayed haemolysis after treatment with intravenous artesunate in patients with severe malaria in India. Malar. J. 19 (1), 39. doi:10.1186/s12936-020-3120-4
Sekeroglu, B., and Tuncal, K. (2021). Prediction of cancer incidence rates for the European continent using machine learning models. Health Inf. J. 27 (1), 1460458220983878. doi:10.1177/1460458220983878
Sherry, V. (2022). Lung cancer: prevention and early identification are key. Nurse Pract. 47 (7), 42–47. doi:10.1097/01.NPR.0000832548.88417.be
Shi, Q. L., Xia, F., Wang, Q. X., Liao, F. L., Guo, Q. Y., Xu, C. C., et al. (2022). Discovery and repurposing of artemisinin. Front. Med. 16 (1), 1–9. doi:10.1007/s11684-021-0898-6
Shi, X. L., Li, S. H., Wang, L., Li, H., Li, Z., Wang, W. Y., et al. (2020). RalB degradation by dihydroartemisinin induces autophagy and IFI16/caspase-1 inflammasome depression in the human laryngeal squamous cell carcinoma. Chin. Med. 15 (1), 64. doi:10.1186/s13020-020-00340-y
Somavarapu, S., Sornsute, A., Trill, J., Abdelhakim, H. E., Goonatilaka, D., Pannala, A. S., et al. (2023). Engineering artesunate-loaded micelles using spray drying for pulmonary drug delivery. J. Drug Deliv. Sci. Technol. 86, 104641. doi:10.1016/j.jddst.2023.104641
Song, L. H., Ge, T. Q., Li, Z. Q., Sun, J. F., Li, G., Sun, Y., et al. (2021). Artesunate: a natural product-based immunomodulator involved in human complement. Biomed. and Pharmacother. 136, 111234. doi:10.1016/j.biopha.2021.111234
Song, Q. X., Peng, S. X., Che, F. Y., and Zhu, X. S. (2022). Artesunate induces ferroptosis via modulation of p38 and ERK signaling pathway in glioblastoma cells. J. Pharmacol. Sci. 148 (3), 300–306. doi:10.1016/j.jphs.2022.01.007
Strik, H., Efferth, T., and Kaina, B. (2024). Artesunate in glioblastoma therapy: case reports and review of clinical studies. Phytomedicine 123, 155274. doi:10.1016/j.phymed.2023.155274
Su, Q., Huang, P., Luo, X., Zhang, P., Li, H., and Chen, Y. (2023). Artesunate reverses cytarabine resistance in acute myeloid leukemia by blocking the JAK/STAT3 signaling. Hematology 28 (1), 2255802. doi:10.1080/16078454.2023.2255802
Svolacchia, F., Brongo, S., Catalano, A., Ceccarini, A., Svolacchia, L., Santarsiere, A., et al. (2023). Natural products for the prevention, treatment and progression of breast cancer. Cancers 15 (11), 2981. doi:10.3390/cancers15112981
Tao, Y. C., Li, W. H., Yang, J. Y., Xue, T. T., Wang, Y. L., Dong, X. J., et al. (2023). Exploring underlying mechanism of artesunate in treatment of acute myeloid leukemia using network pharmacology and molecular docking. Clin. and Transl. Oncol. 25 (8), 2427–2437. doi:10.1007/s12094-023-03125-5
Trimble, C. L., Levinson, K., Maldonado, L., Donovan, M. J., Clark, K. T., Fu, J., et al. (2020). A first-in-human proof-of-concept trial of intravaginal artesunate to treat cervical intraepithelial neoplasia 2/3 (CIN2/3). Gynecol. Oncol. 157 (1), 188–194. doi:10.1016/j.ygyno.2019.12.035
Tse, R. T. H., Zhao, H. D., Wong, C. Y. P., Ka-Lo Cheng, C., Kong, A. W. Y., Peng, Q., et al. (2021). Urinary cell-free DNA in bladder cancer detection. Diagnostics 11 (2), 306. doi:10.3390/diagnostics11020306
Vakhrusheva, O., Erb, H. H. H., Braeunig, V., Markowitsch, S. D., Schupp, P., Baer, P. C., et al. (2022). Artesunate inhibits the growth behavior of docetaxel-resistant prostate cancer cells. Front. Oncol. 12, 789284. doi:10.3389/fonc.2022.789284
Wang, J. S., Wang, M. J., Lu, X., Zhang, J., Liu, Q. X., Zhou, D., et al. (2020a). Artesunate inhibits epithelial-mesenchymal transition in non-small-cell lung cancer (NSCLC) cells by down-regulating the expression of BTBD7. Bioengineered 11 (1), 1197–1207. doi:10.1080/21655979.2020.1834727
Wang, M. Y., Yang, Y. L., Yang, J., Yang, J. J., and Han, S. M. (2020b). circ_KIAA1429 accelerates hepatocellular carcinoma advancement through the mechanism of m(6)A-YTHDF3-Zeb1. Life Sci. 257, 118082. doi:10.1016/j.lfs.2020.118082
Wang, R., Huang, R., Yuan, Y., Wang, Z., and Shen, K. (2023a). Two-carbon tethered artemisinin-isatin hybrids: design, synthesis, anti-breast cancer potential, and in silico study. Front. Mol. Biosci. 10. doi:10.3389/fmolb.2023.1293763
Wang, R., Zhang, Q., and Chen, M. (2023b). Artemisinin-isatin hybrids tethered via ethylene linker and their anti-lung cancer activity. Arch. Der Pharm. 356 (4), e2200563. doi:10.1002/ardp.202200563
Wang, S. M., Long, S. Q., Deng, Z. Y., and Wu, W. Y. (2020c). Positive role of Chinese herbal medicine in cancer immune regulation. Am. J. Chin. Med. 48 (7), 1577–1592. doi:10.1142/s0192415x20500780
Wang, X. X., Du, H. J., and Li, X. D. (2022). Artesunate attenuates atherosclerosis by inhibiting macrophage M1-like polarization and improving metabolism. Int. Immunopharmacol. 102, 108413. doi:10.1016/j.intimp.2021.108413
Wang, Y., Liao, M. L., Zhang, Y., Deng, F., Luo, J., Wang, N. Y., et al. (2021). Artesunate protects immunosuppression mice induced by glucocorticoids via enhancing pro-inflammatory cytokines release and bacterial clearance. Eur. J. Pharmacol. 890, 173630. doi:10.1016/j.ejphar.2020.173630
Wen, L., Chan, B. C., Qiu, M. H., Leung, P. C., and Wong, C. K. (2024). Artemisinin and its derivatives as potential anticancer agents. Molecules 29 (16), 3886. doi:10.3390/molecules29163886
Wroblewska-Luczka, P., Cabaj, J., Bargiel, J., and Luszczki, J. J. (2023). Anticancer effect of terpenes: focus on malignant melanoma. Pharmacol. Rep. 75, 1115–1125. doi:10.1007/s43440-023-00512-1
Xia, J., Dai, Q. L., He, S. Y., Jia, H. J., Liu, X. G., Hua, H., et al. (2023). Artesunate alleviates 5-fluorouracil-induced intestinal damage by suppressing cellular senescence and enhances its antitumor activity. Discov. Oncol. 14 (1), 139. doi:10.1007/s12672-023-00747-7
Xiao, Q. F., Yang, L., Hu, H., and Ke, Y. (2020). Artesunate targets oral tongue squamous cell carcinoma via mitochondrial dysfunction-dependent oxidative damage and Akt/AMPK/mTOR inhibition. J. Bioenergetics Biomembr. 52 (2), 113–121. doi:10.1007/s10863-020-09823-x
Xiao, Y., and Yu, D. H. (2021). Tumor microenvironment as a therapeutic target in cancer. Pharmacol. and Ther. 221, 107753. doi:10.1016/j.pharmthera.2020.107753
Xiong, B. Y., Chen, Y. X., Liu, Y., Hu, X. L., Han, H. B., and Li, Q. S. (2021). Artesunate-loaded porous PLGA microsphere as a pulmonary delivery system for the treatment of non-small cell lung cancer. Colloids Surfaces B-Biointerfaces 206, 111937. doi:10.1016/j.colsurfb.2021.111937
Xiong, D., Geng, C., Zeng, L., Yao, H., Tan, J., Zhang, L., et al. (2024). Artesunate induces ferroptosis by regulating MT1G and has an additive effect with doxorubicin in diffuse large B-cell lymphoma cells. Heliyon 10 (7), e28584. doi:10.1016/j.heliyon.2024.e28584
Xu, H. H., Xiong, S. Q., Chen, Y. Y., Ye, Q. S., Guan, N., Hu, Y. Q., et al. (2023). Flagella of tumor-targeting bacteria trigger local hemorrhage to reprogram tumor-associated macrophages for improved antitumor therapy. Adv. Mater. 35, e2303357. doi:10.1002/adma.202303357
Xu, Z. W., Liu, X. J., and Zhuang, D. P. (2022). Artesunate inhibits proliferation, migration, and invasion of thyroid cancer cells by regulating the PI3K/AKT/FKHR pathway. Biochem. Cell Biol. 100 (1), 85–92. doi:10.1139/bcb-2021-0275
Yang, C. S., Mai, Z. K., Liu, C., Yin, S. H., Cai, Y. T., and Xia, C. L. (2022). Natural products in preventing tumor drug resistance and related signaling pathways. Molecules 27 (11), 3513. doi:10.3390/molecules27113513
Yang, X., Zheng, Y., Liu, L., Huang, J., Wang, F., and Zhang, J. (2021). Progress on the study of the anticancer effects of artesunate. Oncol. Lett. 22 (5), 750. doi:10.3892/ol.2021.13011
Yao, J., Huang, M., Shen, Q., Ding, M., Yu, S., Guo, Y., et al. (2022). c-Myc-PD-L1 Axis sustained gemcitabine-resistance in pancreatic cancer. Front. Pharmacol. 13, 851512. doi:10.3389/fphar.2022.851512
Yao, N., Ma, Q., Yi, W., Liu, Y., Zhang, Q., Gao, X., et al. (2024a). Ang-1 promotes tumorigenesis and mediates the anti-cancer effects of Artesunate on Choroidal melanoma via the regulation of Akt/mTOR signaling pathway. Cytokine 184, 156771. doi:10.1016/j.cyto.2024.156771
Yao, N., Ma, Q., Yi, W., Zhu, Y., Liu, Y., Gao, X., et al. (2024b). Artesunate attenuates the tumorigenesis of choroidal melanoma via inhibiting EFNA3 through Stat3/Akt signaling pathway. J. Cancer Res. Clin. Oncol. 150 (4), 202. doi:10.1007/s00432-024-05711-8
Yao, X., Zhao, C. R., Yin, H., Wang, K. W., and Gao, J. J. (2020). Synergistic antitumor activity of sorafenib and artesunate in hepatocellular carcinoma cells. Acta Pharmacol. Sin. 41 (12), 1609–1620. doi:10.1038/s41401-020-0395-5
Yin, S., Yang, H., Zhao, X., Wei, S., Tao, Y., Liu, M., et al. (2020). Antimalarial agent artesunate induces G0/G1 cell cycle arrest and apoptosis via increasing intracellular ROS levels in normal liver cells. Hum. and Exp. Toxicol. 39 (12), 1681–1689. doi:10.1177/0960327120937331
Yu, X. H., Wu, J. B., Fan, H. Y., Dai, L., Xian, H. C., Chen, B. J., et al. (2024). Artemisinin suppressed tumour growth and induced vascular normalisation in oral squamous cell carcinoma via inhibition of macrophage migration inhibitory factor. Oral Dis. 30, 363–375. doi:10.1111/odi.14418
Yuan, D. L., Guo, T., Zhu, D. D., Ge, H. S., Zhao, Y. L., Huang, A. H., et al. (2022). Exosomal lncRNA ATB derived from ovarian cancer cells promotes angiogenesis via regulating miR-204-3p/tgfβr2 Axis. Cancer Manag. Res. 14, 327–337. doi:10.2147/cmar.S330368
Yuan-Ce, L., Qi, Z., Hong-Yang, Z., Yan-Wen, W., Yu-Mei, S., Bi-Juan, Y., et al. (2023). Artesunate, as an Hsp90 inhibitor, inhibits the proliferation of Burkitt's lymphoma cells by inhibiting AKT and ERK. Front. Pharmacol. 14, 1218467. doi:10.3389/fphar.2023.1218467
Zadeh, T., Lucero, M., and Kandpal, R. P. (2022). Artesunate-induced cellular effects are mediated by specific EPH receptors and ephrin ligands in breast carcinoma cells. Cancer Genomics Proteomics 19 (1), 19–26. doi:10.21873/cgp.20300
Zahir, S. T., Rahmani, K., and Hosseini, S. A. (2021). Malignant melanoma metastasis to the appendix as the first presentation of lentigo maligna melanoma. Archives Iran. Med. 24 (4), 330–332. doi:10.34172/aim.2021.46
Zeng, G., Wu, R. L., and Wan, C. T. (2022). ARTESUNATE IMPROVES OSTEOARTHRITIS PAIN AND CHONDROCYTE INFLAMMATION IN MICE BY REGULATING THE JAK/STAT PATHWAY. Acta Medica Mediterr. 38 (3), 1611–1616. doi:10.19193/0393-6384_2022_3_244
Zeng, Z. W., Chen, D., Chen, L., He, B., and Li, Y. (2023). A comprehensive overview of Artemisinin and its derivatives as anticancer agents. Eur. J. Med. Chem. 247, 115000. doi:10.1016/j.ejmech.2022.115000
Zhang, J., Wang, Y., Yin, C., Gong, P., Zhang, Z., Zhao, L., et al. (2022a). Artesunate improves venetoclax plus cytarabine AML cell targeting by regulating the Noxa/Bim/Mcl-1/p-Chk1 axis. Cell Death Dis. 13 (4), 379. doi:10.1038/s41419-022-04810-z
Zhang, J., Zhou, L., Xiang, J. D., Jin, C. S., Li, M. Q., and He, Y. Y. (2021a). Artesunate-induced ATG5-related autophagy enhances the cytotoxicity of NK92 cells on endometrial cancer cells via interactions between CD155 and CD226/TIGIT. Int. Immunopharmacol. 97, 107705. doi:10.1016/j.intimp.2021.107705
Zhang, J. Y., Li, Y., Wan, J. X., Zhang, M. Y., Li, C. X., and Lin, J. T. (2022b). Artesunate: a review of its therapeutic insights in respiratory diseases. Phytomedicine 104, 154259. doi:10.1016/j.phymed.2022.154259
Zhang, Q. T., Yi, H. M., Yao, H., Lu, L., He, G. C., Wu, M., et al. (2021b). Artemisinin derivatives inhibit non-small cell lung cancer cells through induction of ROS-dependent apoptosis/ferroptosis. J. Cancer 12 (13), 4075–4085. doi:10.7150/jca.57054
Zhang, T., Zhang, Y. W., Jiang, N., Zhao, X., Sang, X. Y., Yang, N., et al. (2020a). Dihydroartemisinin regulates the immune system by promotion of CD8(+) T lymphocytes and suppression of B cell responses. Sci. China-Life Sci. 63 (5), 737–749. doi:10.1007/s11427-019-9550-4
Zhang, W. W., Ning, N., and Huang, J. (2022d). Artesunate suppresses the growth of lung cancer cells by downregulating the AKT/survivin signaling pathway. Biomed Res. Int. 2022, 1–10. doi:10.1155/2022/9170053
Zhang, W. Y., Du, Q., Bian, P. P., Xiao, Z., Wang, X., Feng, Y. J., et al. (2020b). Artesunate exerts anti-prolactinoma activity by inhibiting mitochondrial metabolism and inducing apoptosis. Ann. Transl. Med. 8 (14), 858. doi:10.21037/atm-20-1113
Zhang, Y. X., Wang, Y., Li, Y. P., Huang, C., Xiao, X. Q., Zhong, Z. Q., et al. (2022e). Dihydroartemisinin and artesunate inhibit aerobic glycolysis via suppressing c-Myc signaling in non-small cell lung cancer. Biochem. Pharmacol. 198, 114941. doi:10.1016/j.bcp.2022.114941
Zhao, F. G., Vakhrusheva, O., Markowitsch, S. D., Slade, K. S., Tsaur, I., Cinatl, J., et al. (2020a). Artesunate impairs growth in cisplatin-resistant bladder cancer cells by cell cycle arrest, apoptosis and autophagy induction. Cells 9 (12), 2643. doi:10.3390/cells9122643
Zhao, P. H., Ma, S. T., Hu, J. Q., Zheng, B. Y., Ke, M. R., and Huang, J. D. (2020b). Artesunate-Based multifunctional nanoplatform for photothermal/photoinduced thermodynamic synergistic anticancer therapy. Acs Appl. Bio Mater. 3 (11), 7876–7885. doi:10.1021/acsabm.0c01026
Zhao, Y., Liu, J., and Liu, L. (2020c). Artesunate inhibits lung cancer cells via regulation of mitochondrial membrane potential and induction of apoptosis. Mol. Med. Rep. 22 (4), 3017–3022. doi:10.3892/mmr.2020.11341
Zhong, S. W., Huang, C. H., Chen, Z. K., Chen, Z. H., and Luo, J. L. (2021). Targeting inflammatory signaling in prostate cancer castration resistance. J. Clin. Med. 10 (21), 5000. doi:10.3390/jcm10215000
Zhou, X., Chen, Y., Wang, F., Wu, H., Zhang, Y., Liu, J., et al. (2020a). Artesunate induces autophagy dependent apoptosis through upregulating ROS and activating AMPK-mTOR-ULK1 axis in human bladder cancer cells. Chem. Biol. Interact. 331, 109273. doi:10.1016/j.cbi.2020.109273
Zhou, Y. H., Cui, Y. H., Wang, T., and Luo, Y. (2020b). Long non-coding RNA HOTAIR in cervical cancer: molecular marker, mechanistic insight, and therapeutic target. Adv. Clin. Chem. 97, 117–140. doi:10.1016/bs.acc.2019.12.004
Keywords: artesunate, anti-tumor, mechanism, cancer, drug
Citation: Fan X, Yan Y, Li Y, Song Y and Li B (2024) Anti-tumor mechanism of artesunate. Front. Pharmacol. 15:1483049. doi: 10.3389/fphar.2024.1483049
Received: 19 August 2024; Accepted: 14 October 2024;
Published: 25 October 2024.
Edited by:
Santanu Kar Mahapatra, Midnapore City College, IndiaReviewed by:
Ruo Wang, Shanghai Jiao Tong University, ChinaSimran Sinsinwar, Indian Statistical Institute, India
Copyright © 2024 Fan, Yan, Li, Song and Li. This is an open-access article distributed under the terms of the Creative Commons Attribution License (CC BY). The use, distribution or reproduction in other forums is permitted, provided the original author(s) and the copyright owner(s) are credited and that the original publication in this journal is cited, in accordance with accepted academic practice. No use, distribution or reproduction is permitted which does not comply with these terms.
*Correspondence: Bo Li, bGJvQGpsdS5lZHUuY24=