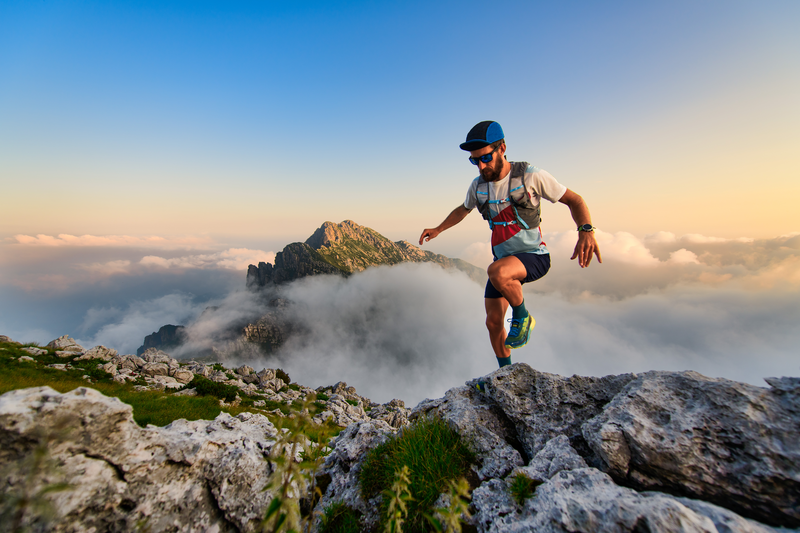
94% of researchers rate our articles as excellent or good
Learn more about the work of our research integrity team to safeguard the quality of each article we publish.
Find out more
REVIEW article
Front. Pharmacol. , 12 September 2024
Sec. Inflammation Pharmacology
Volume 15 - 2024 | https://doi.org/10.3389/fphar.2024.1472771
This article is part of the Research Topic Pharmacological Advances to Treat Pathological Pain View all 5 articles
Ion channels play an important role in mediating pain through signal transduction, regulation, and control of responses, particularly in neuropathic pain. Transient receptor potential channel superfamily plays an important role in cation permeability and cellular signaling. Transient receptor potential channel Melastatin 2 (TRPM2) subfamily regulates Ca2+ concentration in response to various chemicals and signals from the surrounding environment. TRPM2 has a role in several physiological functions such as cellular osmosis, temperature sensing, cellular proliferation, as well as the manifestation of many disease processes such as pain process, cancer, apoptosis, endothelial dysfunction, angiogenesis, renal and lung fibrosis, and cerebral ischemic stroke. Toll-like Receptor 4 (TLR4) is a critical initiator of the immune response to inflammatory stimuli, particularly those triggered by Lipopolysaccharide (LPS). It activates downstream pathways leading to the production of oxidative molecules and inflammatory cytokines, which are modulated by basal and store-operated calcium ion signaling. The cytokine production and release cause an imbalance of antioxidant enzymes and redox potential in the Endoplasmic Reticulum and mitochondria due to oxidative stress, which results from TLR-4 activation and consequently induces the production of inflammatory cytokines in neuronal cells, exacerbating the pain process. Very few studies have reported the role of TRPM2 and its association with Toll-like receptors in the context of neuropathic pain. However, the molecular mechanism underlying the interaction between TRPM2 and TLR-4 and the quantum of impact in acute and chronic neuropathic pain remains unclear. Understanding the link between TLR-4 and TRPM2 will provide more insights into pain regulation mechanisms for the development of new therapeutic molecules to address neuropathic pain.
Pain is defined as a characteristic phenomenon caused by noxious stimuli from pathogenic microorganisms such as bacteria, viruses, and fungi and from injury caused by various forms of mechanical and thermal stimuli. The physiological response to these conditions results in pain. Relief from pain is crucial for an organism’s survival and protection because pain serves as a warning signal for potential harm or injury. It triggers reflexes and behaviors that help avoid or minimize damage, promoting healing and preventing further injury. Without effective pain relief mechanisms, an organism could suffer prolonged stress, which can lead to immune suppression, impaired function, and reduced chances of survival. Therefore, managing pain is not only important for comfort but also vital for the overall wellbeing and preservation of life. Normal pain symptoms involve allodynia, which is non-painful stimuli, such as a light touch or mild temperature change, whereas hyperalgesia refers to an increased sensitivity to painful stimuli generated during minor injury. The symptoms of hypersensitivity involve both allodynia and hyperalgesia, which are intense responses to sensory stimuli, resulting in enhanced sensitivity and discomfort. Persistent and extreme sensitivity are also referred to as chronic pain (Loeser and Melzack, 1999; Świeboda et al., 2013). Acute pain condition is a protective mechanism in which tissue stimulates the healing process. Beyond any alteration in the pain pathway and duration leads to hypersensitivity and intolerable or chronic pain.
Depending on the duration of symptoms, pain is classified as acute pain (<3 months) and chronic pain (>3 months). Pain is also classified into many subtypes such as anatomical pain, physiological pain, pathological pain, deep pain, vascular pain, bone and joint pain, myalgia, organ pain, wired pain, neuralgia, causalgia, convolutional pain, and phantom pain, depending upon the stimuli, location, physiological and social factors (Świeboda et al., 2013; Sela et al., 2002).
According to the International Association for the Study of Pain defines, neuropathic pain is a pain caused by a lesion or disease affecting the somatosensory nervous system. As per the International classification of diseases, conditions for peripheral neuropathic pain include trigeminal neuralgia, polyneuropathy, peripheral nerve injury, and painful radiculopathy. Conditions for central neuropathy include pain caused by brain or spinal cord injury, post-stroke pain, and pain associated with multiple sclerosis (Scholz et al., 2019). Neuropathic pain is associated with damage to the nerves, including central, peripheral, and somatosensory nervous systems. It involves both positive and negative symptoms. Positive symptoms include paresthesia, dysesthesia, superficial pain, allodynia, and hyperalgesia. Negative symptoms include tactile hypoesthesia, thermal hypoesthesia, and pinprick hypoalgesia (Baron, 2006). Neuropathic pain is considered chronic pain that affects the quality of normal life of an individual. Trigeminal neuralgia, diabetic neuropathic pain, cancer pain, and sciatica are some examples of chronic pain. Many of the neuronal complex structures are involved in the activation, transmission, regulation, and healing processes (Backonja, 2003). Nerve endings, dorsal root ganglia, microglia, Schwann cells, and thalamus are the important structures that are involved in chronic pain. Structural changes, numerous cellular interactions, and signaling processes contribute to the nociceptive pathway, including ion channels, receptors, immune cells, and microglia (Finnerup et al., 2020; Kerstman et al., 2013). The transition from acute neuropathic pain to chronic pain is a complex process and involves multiple signaling pathways, central and peripheral processes, and inherited and environmental risks.
Neuroinflammation is an immune-mediated inflammatory response elicited by various stimuli. Cytokines released in response to the inflammation regulate oxidative stress and mediate the injury and/or repair process (Myers et al., 2006). Sensitization of nerve endings and other inflammatory molecules mediate the pain process in which ion channels play an important role in mediating pain, inflammation, and repair depending on Ca2+ ion influx (Kuffler, 2020). Many of the ion channels are regulated either by a change in the voltage (cation/anion exchange) or receptor-mediated responses. These ion channels respond to changes in the cellular external and internal environments, thereby eliciting a physiological response (Bouali-Benazzouz et al., 2021; Vicario et al., 2020). Ion channels exhibit regulatory properties of immune cells, metabolism, and inflammation pathways (Ruck et al., 2022; Bittner et al., 2014). Apart from the homeostatic functions, ion channels may also be involved in the pathological mechanisms of neuronal inflammation that leads to pain. Membrane ion channels that govern sodium, potassium, and calcium ions and their modulation by various inflammatory mediators are involved in neuropathic pain.
Inflammatory responses and oxidative stress are regulated by molecular inhibitors and activators, Toll-like-receptor (TLR-4) is a transmembrane pattern recognition receptor (Fehder et al., 2007), Nicotinamide Adenine Dinucleotide phosphate (NADPH) oxidase (NOX), and Transient receptor potential (TRP), an ion channel and a well-known receptor for immune responses. TLR-4 is associated with many pathological conditions with chronic neuropathic pain. TRPM2 is a calcium-permeable cation channel in sensory neurons. NOX is part of a family of enzymes that includes NOX1, NOX2, NOX3, NOX4, NOX5, DUOX1, and DUOX2. NOX enzymes are known for immune defenses in which Reactive Oxygen Species (ROS) production by phagocytic cells to kill pathogens (Vermot et al., 2021). TRPM2 channels are activated by ROS produced by NOX, which leads to calcium influx. This calcium signaling boosts NOX activity, which amplifies ROS production and oxidative stress. This pathway is associated with several diseases, including neurodegenerative diseases and chronic pain (Görlach et al., 2015).
The present review aims to address pathways in which oxidative stress and inflammatory reactions by TLR-4, NOX, and TRPM2 implicate pain (Figure 1). These findings highlight the biological importance of TRPM2 and TLR-4 for elucidating pain pathways due to producing ROS and inflammatory cytokines, which are important in regulating oxidative stress and inflammation in cells. TRPM2 inhibitors could decrease oxidative stress-induced injuries and neuropathic pain in a wide variety of ways. TLR-4 antagonists may decrease inflammatory responses and alleviate chronic inflammation. The discovery of the mechanisms by which TRPM2 activation drives nerve damage and inflammation, and its association with TLR-4 can show new pathways; such understanding may become apparent as an innovative therapy for disorders assumed to cause or intensify neuropathic pain.
The process of recognizing pain involves the recognition of pain signals by sensory neurons, initiation of nerve impulses, and transforming the noxious stimuli to pain response. These nociceptive neurons are specialized in the recognition of noxious stimuli, of which ion channels on cell membrane play an important role in intermembrane transporters of various ions, thereby regulating various physiological functions ranging from excitation of tissue transportation, cell migration tumorigenesis, atherosclerosis, and apoptosis (Kew and Davies, 2010; Schwab et al., 2012; Jentsch, 2016). Voltage-gated ion channels are widely expressed in all tissues, including neurons, such as sodium Nav1.8 and Nav1.9 channels (Giniatullin, 2020). Other ion channels such as purinergic receptor, TRPV1(vanilloid)/A1 (ankyrin), TRPC (canonical), TRPM2, and voltage-gated (sodium, calcium, and potassium) channels are also involved in nociception. Many of the neuronal diseases, including neuropathic pain, are linked to ion channel dysfunction and neuroinflammation (Eren-Koçak and Dalkara, 2021).
Transient receptor potential (TRP) channels are widely distributed throughout the tissues. In 1969, TRP was discovered in drosophila and subsequently in humans (Montell and Rubin, 1989; Samanta et al., 2018). The TRP channels superfamily were extended up to 48 members and their major role was found in cation permeability and cellular sensory signaling. These channel proteins span the transmembrane with a C-terminal intracellular domain and N-terminal intracellular domain (Kassmann et al., 2013), which are non-selective ion channels that regulate Ca2+, Mg2+ influx, and other monovalent cations. These TRP channels can be activated in cellular organelles, such as endoplasmic reticulum, lysosomes, Golgi apparatus, and synaptic vesicles by Ca2+ influx. TRP channels are also involved in the regulation of osmotic pressure, temperature, pH oxidation-reduction vascular tone, proliferation, mechanosensing, proliferation, apoptosis, and angiogenesis (Gees et al., 2010). Therefore, TRP channels respond to various stimuli such as stress, mediators, hormones, chemicals, and environmental factors (Lolignier et al., 2016; Kaneko and Szallasi, 2014). Changes in the channel function result in many disorders, such as retinal, skin, cardiac, neuronal, and vascular, forming the basis of mutation in the genes responsible for coding for TRP channel (Tsagareli and Nozadze, 2020). TRP Channels have been implicated in various neurodegenerative diseases such as Alzheimer’s, Parkinson’s, and Huntington’s diseases (Rather et al., 2023). TRP channels are proven to play as central elements of nociception, thereby acting as potential targets for alleviating neuropathic pain. Modulating the TRP channel function offers a wide scope for altering cellular functions, thereby increasing the research interest. Modulation of TRP channels has emerged as a novel field of therapeutic strategy for the treatment of pain, especially neuropathic pain. Many small molecule compounds that modulate TRP channels have entered clinical trials aiming to treat a variety of neuronal diseases (Table 1).
TRPM channels are ubiquitous in nature and widely expressed and distributed in tissues and contribute to health and disease. The three domains of these ion channels are N, C, and channel domain, where the N-terminal is associated with four similar melastatin subunits and a pre-S1 domain to play a role in sensing and channel assembly. The channel domain S4 is specific to the TRPM family and participates in a voltage-sensing-like domain, whereas S5-S6 forms the P-loop, which mainly acts as an ion-conducting pore. The C-Domain is composed of a TRP box, which is highly conserved and maintains channel stability, whereas the coiled-coil domain contains motifs that modulate pore gating. The differences in the homology sequence of the C-terminus divide the TRPM subfamily into four groups: TRPM3/TRPM6/TRPM7, highly permeable to Ca2+, TRPM2/TRPM8 nonselective for monovalent and divalent cations, whereas TRPM4/TRPM5 is permeable to monovalent cations. TRPM2/TRPM6/TRPM7 present enzymatic properties at the C terminus, and TRPM2 has an additional domain for nucleoside diphosphate pyrophosphatase, which shares a similar homology with Nudix hydrolase NUDT (-H domain), which exhibit a wide range of functions such as metabolism of nicotinamide adenine dinucleotide (NAD), ADP-ribose, and their derivatives and is characterized by different substrate specificity and intracellular localization. (Samanta et al., 2018; McLennan, 2006). TRPM8 is temperature sensitive and activated by innocuous cold to noxious cold. It is also activated by menthol and icilin, synthetic super-agonist. Both TRPM8 agonists and antagonists, e.g., AMTB, proved to be beneficial in pain pathways (Fernández-Peña and Viana, 2013). TRPM3 is a polymodal nociceptor thought to be involved in the detection of noxious heat (Vriens et al., 2011). TRPM3, together with TRPV1 and TRPA1, mediate acute noxious heat detection in mice (Vandewauw et al., 2018). Given the importance of TRPM channels, there is an increase in research interest in TRPM channels and their role in triggering various diseases, including pain process, cellular osmosis, temperature sensing, cellular proliferation, cancer, apoptosis, endothelial dysfunction, angioparagraphgenesis, renal, and lung fibrosis (Duitama et al., 2020).
TRPM2 is particularly gaining importance as it is permeable to both monovalent and divalent cations, as well as its activation/inhibition in response to various stimuli from both exogenous and endogenous sources, leading to changes in cellular responses that vary from picoseconds to several seconds. This is the only ion channel of the TRPM family associated with enzyme activity at NUDT9-H domain of the C-terminus sensitive to adenosine diphosphate ribose (ADPR) (Sumoza-Toledo and Penner, 2011; Perraud et al., 2001) thereby involving multiple functional roles like Ca2+ homeostasis (Hasan and Zhang, 2018), redox potential (Song et al., 2016), osmotic regulation, temperature sensitive gating (Vilar et al., 2020; Tan and McNaughton, 2018), production of inflammatory mediators (Yamamoto and Shimizu, 2016; Akyuva and Nazıroğlu, 2020), pain modulation (Di et al., 2012; Yüksel et al., 2017), cellular migration, cytoskeleton remodeling (Schwab et al., 2012), and immunity (Knowles et al., 2013; Syed Mortadza et al., 2015). Functional role of TRPM2 have been reported in many diseases such as Alzheimer’s and Parkinson’s disease, ischemic stroke, neuronal cell death, neurovascular functional injury, myocardial ischemia/reperfusion injury, vascular dysfunction, pancreatic β-cell death associated with pancreatitis, acute and chronic diseases, and liver toxicity (Malko and Jiang, 2020). TRPM2 channel is widely expressed in immune cells, microglia, neutrophils, T-lymphocytes. It also acts as a sensor for ROS and plays a key role in the inflammatory response in both normal and pathological states. Since TRPM2 has multifunctional role in oxidative stress and inflammation it would be worth exploring its mechanisms in attenuating pain-related diseases.
TRPM2 is widely expressed in neurons and its role in ischemic stroke was evaluated in several in vitro and in vivo studies. TRPM2 have shown to increase the cell survival and inhibit apoptosis by various mechanisms (Ali et al., 2023; Shi et al., 2021). The following few studies represented the role of TRPM2 in ischemic stroke models.TRPM2 in primary cortical cultures of rat subjected to H2O2 induces apoptosis, and this is significantly reversed by using TRPM2 siRNA by inhibiting Ca2+ influx and cell death (Kaneko et al., 2006). The role of TRPM2 in oxidative stress induced by hypoxia has been studied in different animal models. TRPM2 KO mice, when subjected to transient ischemia by carotid artery occlusion, showed a 40% reduction in infarct volume. Some of the molecular pathways that contribute to cell survival are activation of protein kinase B (Akt) pathway and inhibition of glycogen synthase kinase-3β (GSK3β) pathway in TRPM2 KO mice (Alim et al., 2013). Genetic deletion of TRPM2 shows neuroprotective effects and improved sensory and motor outcomes in neonatal mice when compared to the wild type. TRPM2 KO mice showed an increased pro-survival signaling pathway and produced neuroprotective effects through Akt/GSK3β pathway (Huang et al., 2017). The use of TRPM2 inhibitors such as flufenamic acid (Nazıroğlu et al., 2007), clotrimazole (Hill et al., 2004), aminoethoxy diphenyl borate (APB) (Togashi et al., 2008), N-(p-amylcinnamoyl)anthranilic acid (ACA) (Kraft et al., 2006), and TRPM2 shRNA significantly reduced neuronal cell death following oxygen-glucose deprivation in males (Jia et al., 2011). Pharmacological inhibition of TRPM2 inhibits the adhesion of neutrophils in ischemic conditions (Gelderblom et al., 2014). There is a strong correlation between TRPM2 oxidative stress, inflammation, and ischemia followed by reperfusion injury (Huang et al., 2024).
Apart from neuronal cells, TRPM2 is widely expressed in non-neuronal cells such as microglia and astrocytes (Turlova et al., 2018). Hypoxia-induced generation of H2O2 activated microglia increased TRPM2 mediated Ca2+ conductance in middle cerebral artery occlusion model injury (Patel et al., 2013). H2O2-induced oxidative stress revealed upregulation of TRPM2-like conductance and was reversed by flufenamic acid (Fonfria et al., 2006) in rat microglia. An increase in TRPM2 activity is associated with the generation of ROS, leading to the activation of Poly adenosine-diphosphate ribose polymerase 1 and TRPM2 activity were suppressed by inhibiting PKC and Nicotinamide Adenine Dinucleotide Phosphate (NADPH) oxidase (NOX) and downstream Mitogen-Activated Protein Kinase (MAPK)/extracellular signal-regulated kinase (MEK/ERK) pathway (Alawieyah Syed Mortadza et al., 2018). Release of cytokine interleukin-1 beta (IL-1β) from microglia is also mediated through TRPM2-dependent activation of Nucleotide-binding Oligomerization Domain-like receptor pyrin domain 3 inflammasome (Aminzadeh et al., 2018). Selenium, an important essential element through the GSH (Glutathione) peroxidase pathway, prevents interferon-gamma (IFNγ) induced activation of TRPM2 channel-mediated apoptosis in microglia (Akyuva et al., 2021). TRPM2 (knock out) KO in microglia suppressed the kainic acid-induced glial activation, cytokine production, and hippocampus paroxysmal discharges, affirming the role of TRPM2 via AMPK and mTOR pathway in epileptogenesis (Yıldızhan and Nazıroğlu, 2020). When stimulated with interferon-γ, microglia in the brain of C57bl/6 mice displayed excessive currents of TRPM2 (Akyuva et al., 2021). Macrophage exposure to LPS triggers the activation of TRPM2, thereby promoting NO production by regulating MAPK and JNK signaling pathways (Miyake et al., 2014).
Reactive oxygen species formed by renal ischemia-induced hypoxia and reperfusion activate TRPM2 ion channels. TRPM2-KO mice have shown resistance to renal injury (Khanahmad et al., 2022). Administration of TRPM2 antagonist, 8-bromo cADPR in Wistar rats shown to inhibit renal ischemia-reperfusion injury via caspase 3 inhibition (Eraslan et al., 2019). Cisplatin induced nephrotoxicity is also reduced by the activation of TRPM2 mediated autophagy (Yu et al., 2023). TRPM2-KO mice markedly improved renal dysfunction and its ablation remarkedly suppressed TGFβ mediated JNK activation renal fibrosis (Wang Y. et al., 2019). Thus, TRPM2 highlights its role in renal ischemic injury and fibrosis which is elevated during renal ischemic/reperfusion injury (Gao et al., 2014). Ischemia reperfusion-activated NOX and RAC1 were activated in WT mice but not in TRPM2-KO mice (Gao et al., 2014). Curcumin also reduces the albumin-evoked Ca2+-induced oxidative stress through the TRPM2 channel in renal tubules (Nazıroğlu et al., 2019).
ROS-dependent neuronal inflammation and death involve multiple mechanisms, including pyro-apoptosis, autophagy, and apoptosis. ROS-mediated TRPM2 inhibits autophagy through downregulation of AMPK-induced Mammalian rapamycin target protein (mTOR), leading to ischemic/reperfusion-induced neuronal cell death (Hu et al., 2021). TRPM2 channels regulate cation permeability across the cell membrane that includes Zn2+ and the accumulation of the Zn2+ intracellularly induces ROS production, triggering lysosomal dysfunction and an increase in neuronal cell death (Ye et al., 2014). In addition, TRPM2 activates Calmodulin-dependent kinase II -mediated phosphorylation of Beclin1 that inhibits autophagy and induces neuronal death (Wang et al., 2016). TRPM2 mediated Ca2+ induced neuronal death was modulated by duloxetine preventing from apoptosis in hippocampus and dorsal root ganglion (DRG) of rats (Demirdaş et al., 2017).
The role of TRPM2 in inflammation has been extensively evaluated for Ca2+ mediated oxidative stress (ROS) that, in turn, activates innate immunity, thus delineating its role in autoimmune disorders such as rheumatoid arthritis, type 1 diabetes, multiple sclerosis, inflammatory bowel disease through multiple mechanisms. In an animal model of multiple sclerosis, TRPM2-KO or pharmacological inhibition of TRPM2 inhibits the progression of the disease. Moreover, decreased neutrophil infiltration in the central nervous system was observed in KO mice than in WT (Tsutsui et al., 2018). A decrease in neuronal antioxidant glutathione triggers the increased current of the TRPM2 channel (Belrose et al., 2012). Additional mechanisms such as ROS-ADPR- Poly adenosine diphosphate ribose polymerase mediated TRPM2 current in microglia thereby increase intracellular Ca2+ and thereby induce apoptosis of hippocampal pyramidal neurons in Alzheimer’s disease (Övey and Naziroğlu, 2015). TRPM2 also plays a key role in Amyloid beta (Aβ)/ROS-induced microglial-mediated neuroinflammation and neuronal death (Jiang et al., 2018). Glutathione depletion linked to oxidative stress induces apoptosis mediated through TRPM2 channels in microglial cells with Alzheimer’s disease model.
The liver is known as an important metabolic organ that regulates various functions. Acetaminophen is the commonly prescribed drug for pyrexia and is known to cause unwanted effects such as hepatotoxicity. ROS is the key mediator of acetaminophen-induced toxicity. Acetaminophen induced hepatocyte death by increasing Ca2+ influx in cultured rat and mouse hepatocytes which were blocked by ACA, clotrimazole or TRPM2-siRNA, and TRPM2-KO (Wang et al., 2016; Kheradpezhouh et al., 2014). TRPM2-mediated Ca2+ signalling activates Ca2+/CaMKII to inhibit autophagy (Ni et al., 2012). Also, acetaminophen-induced liver injury in WT mice was mitigated by treatment with CaMKII inhibitor KN-93 (Wang et al., 2016). In the hepatic ischemia-reperfusion injury model, TRPM2-mediated Ca2+ influx causes mitochondrial lipid peroxidation due to increasing arachidonate 12-lipoxygenase (Zhong et al., 2023). In addition, pretreatment with antioxidants such as N-acetyl cysteine and thymoquinone inhibits the Ca2+ entry by reducing the TRPM2 gene expression in the hepatocyte model of ischemia-reperfusion injury (Atilgan et al., 2022; Caglar et al., 2024).
TRPM2 ion channels play an important role in generating, transmitting, and transforming nerve signals. Calcium ions play an important role in interneuronal communication, triggering an action potential and the release of certain neurotransmitters and mediators (Malko et al., 2019; Wang H. et al., 2019). Primary afferent neurons such as DRG and trigeminal ganglia also express TRPM2 channels (Vilar et al., 2020). The C-fibers of afferent neurons are mainly responsible for pain perception leading to depolarization. Substance P and calcitonin gene-related peptide (CGRP) of the peptidergic neurons, upon sustained stimulation, exacerbate tissue inflammation by infiltrating immune cells. TRPM2 is known to express abundantly in both peptidergic and non-peptidergic C-fibres (Matsumoto et al., 2016). TRPM2 is known to play a crucial role in afferent ganglia, where it responds to oxidative stress, particularly that caused by inflammatory injuries. This channel contributes to the sensory signaling processes by detecting and reacting to oxidative damage, which can occur during inflammation. The H2O2-induced inward currents in a whole cell patch clamp experiment with DRG neurons H2O2-induced inward currents were reversibly abolished by TRPM2 inhibitors 2-APB and ACA (Nazıroğlu et al., 2011; Naziroğlu et al., 2013). In addition, H2O2-mediated TRPM2 currents were inhibited by NADPH oxidase inhibitors such as apocynin and N-acetyl cysteine, which regulate the H2O2-ADPR-TRPM2 axis (Nazıroğlu, 2017). In cultured trigeminal ganglia upon H2O2 exposure, there is a significant increase in cytokine and chemokine levels which were prevented by treatment with TRPM2 inhibitors 2-APB (Chung et al., 2015). Therefore, TRPM2 plays an important role in the mechanisms of neuronal excitability and in the proinflammatory conditions under oxidative stress.
TRPM2 is widely expressed in immune cells. LPS stimulation of immune cells increased the expression of TRPM2, contributing to the production of cytokines (Wehrhahn et al., 2010). Also, neutrophil infiltration is linked with TRPM2 deficiency in carrageenan-induced inflammation (Haraguchi et al., 2012). IL- 1β secretion is also increased in macrophages by ROS-mediated TRPM2 activation (Zhong et al., 2013). The factors that regulate the calcium gating through TRPM2 include adenosine dinucleotide, cytokines, ROS, and intracellular calcium ions (Wang H. et al., 2019). Increased expression of TRPM2 in macrophages is associated with acceleration of inflammatory signals that regulate the pathophysiology of pain (Jang et al., 2018). Neuronal cell response to LPS is dependent on Ca2+, but the mechanisms involved are poorly elucidated. LPS is known to activate TLR-4, which initiates to activate of the downstream pathways of inflammation, such as phosphorylation of MAPKs, NF-kB translocation to the nucleus, and upregulation of inflammatory genes for cytokines such as TNFα, IL-1β, and IL-6 as shown in Figure 1. There is also evidence that LPS-mediated TLR-4 activates PLC, thereby mobilizing intracellular Ca2+. Thus, the role of Ca2+ and the production of cytokines are interlinked. Then, it comes to the question that controlling Ca2+ entry into the cell decreases inflammation. To understand this, the cytokine production generates ROS from mitochondria via modulating NADPH redox system is to be studied. The cytokine production generates ROS by activating NADPH oxidase, disrupting mitochondrial function, and activating MAPK pathways, including inducible nitric acid synthase and activating inflammasomes, contributing to the inflammatory response and cellular signaling (Bordt and Polster, 2014). The generation of free radicals takes place by intracellular production of H2O2 (Checa and Aran, 2020). Cellular activity sensors such as ion channels, particularly TRPM2, regulate the cation influx, including Ca2+, which further increases the inflammation process. Since regulation of inflammation is Ca2+ dependent which is also regulated by TRPM2 ion channel. Given the importance of TRPM2 ion channel in current scenario, modulating the function of TRPM2 is warranted. TRPM2 modulation with antioxidant compounds will alleviate the inflammation, thereby reducing nociception. Table 2 provides a summary of some research studies that use oxidative stress and inflammation as a model to modulate neuronal pain via TRPM2.
Table 2. Evidence of TRPM2 mediated neuropathic pain in response to inflammation and oxidative stress.
The role of TRPM2 in acute inflammatory mechanisms is acknowledged not only in neuronal cells but also in nonneuronal cells. TRPM2 channels are widely expressed in various cell types, including microglia (Mortadza et al., 2017; Kraft et al., 2004), macrophages (Zou et al., 2013), neurons (Kaneko et al., 2006; Alim et al., 2013), endothelial cells (Hecquet and Malik, 2009; Mittal et al., 2017; Yang et al., 2006), cardiomyocytes (Yang et al., 2006), dendritic cells (Sumoza-Toledo et al., 2011), and pancreatic beta cells (Ishii et al., 2006; Togashi et al., 2006). This indicates that TRPM2 plays multiple functional roles in many physiological and pathological processes. TRPM2 has been shown to regulate intracellular calcium levels through lysosomal, endoplasmic reticulum, and mitochondrial compartments through various pathways that mediate through both exogenous and endogenous mediators (Zhang et al., 2018).
TRPM2 is known to be regulated by other receptor mechanisms in inflammation and pain, such as the N-methyl-D-aspartate (NMDA) receptor (NMDAR), which activates to promote TRPM2-mediated Ca2+ influx via ERK1/2-dependent PARP-1 recruitment of microglia-dependent neuroinflammation (Raghunatha et al., 2020). TRPM2 channels can also be activated through intracellular signaling cascades initiated by pruritogen receptors and, thereby, neuronal activation. TRPM2 via Protein Kinase C gamma (PKC-γ), a neuro-specific PKC phosphorylates serine/threonine residues, activates and increases the expression of NMDAR, thereby increasing the excitotoxicity of NMDARS, and the interaction between them is thought to be mediated by oxidative stress (Sun and Alkon, 2014). Antioxidants such as Selenium reduced the fibromyalgia induced increase in TRPM2 and TRPV1 currents, pain intensity, ROS, and intracellular free Ca2+ in sciatic and DRG neurons (Yüksel et al., 2017).
Toll-like receptors (TLRs) are pattern recognition receptors embedded in the phospholipid membrane as well as endosomes and lysosomes (Sahoo, 2020). In humans, there are ten TLRs (TLR1-TLR10), but overall, there are 12 TLRs. Different TLRs recognize and activate different pathogen-associated molecular patterns (PAMPs) (Kawai and Akira, 2007). TLR-1 and TLR-2 link together and recognize bacteria lipoproteins which activate immune responses. TLR-2 not only forms a heterodimer with TLR-1 but also with TLR-6 to recognize microbial patterns. TLR-3 is found embedded in endosomes, senses viral infections, and activates the production of type-I interferons. TLR-4 is activated by bacterial LPS, which is the main cell wall component of Gram-negative bacteria (Kuzmich et al., 2017). This activation can contribute to inflammation and pain pathways, leading to the release of pro-inflammatory cytokines and other mediators that contribute to the development or exacerbation of neuropathic pain. Some Gram-negative bacteria associated with this process include Escherichia coli (E. coli), Pseudomonas aeruginosa, Klebsiella pneumoniae, Salmonella spp., Neisseria meningitidis, Haemophilus influenzae, Helicobacter pylori (Deng and Chiu, 2021; Staurengo-Ferrari et al., 2022). TLR4 can also be activated by a variety of non-LPS ligands. These ligands include endogenous danger-associated molecular patterns (DAMPs) and exogenous pathogen-associated molecular patterns (PAMPs) other than LPS (Facchini, 2018; Alexzander Asea et al., 2002; Erridge, 2010). TLR-7, TLR-8, and TLR-9 are located in endosomes and lysosomes, while TLR-5, found on the cell surface, recognizes bacterial flagellins involved in motility, leading to the activation of inflammatory cytokines (Tegtmeyer et al., 2020). They are mainly expressed in various immune cells and initiate inflammatory responses. TLR-10, although found on both the cell surface and endosomes, the actual functions are not well-defined (Noreen and Arshad, 2015).
However, Toll-like receptors are a group of receptors that recognize and initiate innate and adaptive immune responses. Like other receptors, they span cellular transmembrane with an external domain of leucine repeats and cytosolic Toll interleukin-1 receptor (TIR) domains to activate downstream pathways. The external domain tends to recognize endotoxins, heat shock proteins, cellular damage products, and High mobility group box 1 (HMGB-1) proteins (Bettoni et al., 2008). The receptors are widely distributed not only on antigen-presenting cells but also on myocytes, adipocytes, thyroid cells, mesangial cells, and fibroblasts. The receptors are also expressed in sensory neurons, microglial cells, and astrocytes (Vaure and Liu, 2014). TLR-4 receptor undergoes dimerization with myeloid differentiation protein-2 upon ligand binding to microdomain lipid rafts and initiates TIR adoption molecules, thereby initiating a signaling cascade.
TLR-4, through the TIR domain-containing adapter-inducing Inhibitor of the kappa B (IkB) pathway, induces NF-kB and expression of proinflammatory cytokines such as IL-1, IL-6, and TNF-α. Activation and inhibition of these pathways create a balance between the production of these cytokines and Interferon Type-1 (IFN-1) (Roy et al., 2016; Kawasaki and Kawai, 2014).
TLR4 plays an important role in adaptive immune response, induction, and maintenance of acute and chronic pain states. Recognition of pathogens and products from damaged neurons by microglia leads to activation of TLR-4 and increased expression of proinflammatory mediators. Sustained activation or dysregulation of TLR-4 contributes to inflammatory and autoimmune diseases such as Crohn’s Disease, atherosclerosis, rheumatoid arthritis, type 1 diabetes, and other neurodegenerative diseases (Takeda et al., 2003; Booth et al., 2011; Hosseini et al., 2015).
Microglial activation of TLR-4 after spinal cord injury is pivotal for the induction of pain by modulating proinflammatory cascade and expression of TNFα, IFN-γ, IL-6, IL-1β, and NF-kB (Kuang et al., 2012; Stokes et al., 2013). In rat animal model of chronic constriction injury TLR-4 antagonists and siRNA-mediated suppression of spinal cord TLR-4 signaling prevent the activation of the NF-kB pathway and production of proinflammatory cytokines, thereby attenuating allodynia and thermal hyperalgesia, emphasizing its preventive role in neuropathic pain (Eidson and Murphy, 2013; Wu et al., 2010). TLR-4 also mediates the conversion of acute to chronic pain. Intrathecal administration of TLR-4 antagonists reversed the chronic constriction injury-induced thermal hyperalgesia and mechanical allodynia in wild-type mice, whereas in knockout and point mutant mice, attenuation of thermal hypersensitivity along with spinal microglial activation and lower proinflammatory cytokines suggesting its role in the maintenance of chronic pain (Hutchinson et al., 2008; Christianson et al., 2011; Nicotra et al., 2012; Woller et al., 2017). Administration of TLR-4 antisense oligonucleotide prevents thermal hypersensitivity with reduced expression of mRNA for microglial markers and spinal proinflammatory cytokines (Tanga et al., 2005).
Hydrogen peroxide, a ROS, oxidatively modifies TLR-4 proteins, and it mimics LPS binding (Powers et al., 2006). It activates the release of damaged-associated molecular proteins (DAMPs) from dying cells, which trigger TLR-4 proteins (Shim et al., 2017). H2O2 also modulates the activity of kinases and transcription factors such as NF-κB and MAPKs, which are critical for TLR-4 signaling, which can amplify inflammatory responses (Burgueno et al., 2021). When H2O2 production is increased, it acts as a secondary signal to aid TLR-4-mediated defenses guaranteeing an immune response (Zhou et al., 2013). The interaction between ROS and TLR-4 can intensify inflammation indicating tissue damage and disease development (Gunawardena et al., 2019).
The generation of neuropathic pain involves complex pathways within the nervous system, both peripheral and central. The process is initiated by damage or dysfunction in the somatosensory nervous system, which leads to abnormal pain signaling. Neuropathic pain often involves nerve injury or chronic inflammation, leading to increased oxidative stress and inflammatory mediators such as proinflammatory cytokines, chemokine, and ROS, which can activate TRPM2 channels. Understanding the mechanisms by which TRPM2 contributes to neuropathic pain can provide insights into potential therapeutic targets for managing chronic pain conditions.
On the other hand, TLR-4 is a key player in the immune system, primarily recognized for its role in detecting pathogens and initiating inflammatory responses. However, TLR-4 plays a critical role in the pathogenesis of neuropathic pain by driving neuroinflammation, central sensitization, and the maintenance of a chronic pain state. Targeting TLR-4 or its downstream signaling pathways is a potential therapeutic strategy for managing neuropathic pain.
TLR-4 is expressed on the cell membrane and senses pathogen-associated molecular patterns such as LPS, peptidoglycans, chitin, and glucans (Brown et al., 2011). Upon recognition and activation, TLR-4 recruits adaptor molecules such as MyD88 and intracellular Toll-interleukin (IL)-1 receptor domains and promotes downstream pathways leading to the secretion of proinflammatory mediators, including cytokines (Blasius and Beutler, 2010; Santoni et al., 2015). These proinflammatory responses by the immunocompetent cells in the brain, such as microglia, contribute to neuroinflammation leading to pain. In chronic states such as neuropathic pain, microglial activation results in deleterious consequences (Block et al., 2007). Microglia express a wide variety of ion channels, including TRP channels, that are necessary for cytokine production, proliferation, and migration of microglia (Echeverry et al., 2016). Studies indicate that H2O2-induced TRPM2 activation mediates Ca2+ influx, which modulates physiological and pathological cellular functions (Shirakawa and Kaneko, 2018). TRPM2 is expressed in both neurons and glia, and oxidative stress-induced TRPM2 activation is implicated in neuronal diseases (So et al., 2015). Miyake et al. (2014) demonstrated that LPS and IFN-γ can stimulate TRPM2 mediated Ca2+ in microglia. They also showed that activation of TRPM2 results in increased NO production. Interaction between nociceptive neurons and glial cells plays an important role in neuropathic pain (Ren and Dubner, 2010).
TRPM2, a calcium-permeable cation channel present in sensory neurons, is capable of recognizing mechanical, chemical, and thermal stimuli (Santoni et al., 2015). TRPM2 activation leads to increased calcium levels, which also results in a pain-signaling response in the sensory neurons. TLR-4 has been linked to neuroinflammation (Table 3) and the sensitization of nociceptors (Malko et al., 2019). When TLR-4 interacts with ROS, TLR-4 proteins can intensify inflammation and hypersensitivity to pain through the opening of TRPM2 channels. Thus, the pathways of both TRPM2 and TLR4 converge on neuroinflammation (Yamamoto and Shimizu, 2016). Neuropathic Pain is a chronic pain caused by a disease of the somatosensory nervous system (Finnerup et al., 2021). TRPM2 contributes to the release of pro-inflammatory cytokines (Haraguchi et al., 2012), and the production of cytokines, in turn, activates TLR-4, which plays an important role in neuropathic pain and induces inflammatory and immune responses (Kuzmich et al., 2017).
TRPM2 channel is a newly cited player for neuroinflammation mediated in response to LPS, a classical target for TLR-4 activation where the interaction between TLR-4 and TRPM2 in neuronal cells was least explored. TLR-4-mediated responses required Ca2+ influx through TRPM2 (Schappe et al., 2018; Tauseef et al., 2012), and the binding of LPS to TLR-4 activation increases the production of diacylglycerol (DAG) and the subsequent increase in Ca2+ concentration, resulting in MyD88-NF-kB activation (Mohammadi, 2019). TRPM2-mediated Ca2+ entry is implicated in inflammatory responses in neuronal and non-neuronal cells (Tauseef et al., 2012; Mehta et al., 2003). LPS-TLR-4 activation triggers NF-kB-mediated cytokine production resulting in TRP-mediated Ca2+ influx. Cytokine increases intracellular Ca2+ concentration, thereby inducing early ROS production preceded by TRPM2-mediated Ca2+ influx (Simon et al., 2017; López-Requena et al., 2017). Consequently, TRPM2 regulates total ROS production and Ca2+ influx. The existing studies suggest TRPM2 is not only involved in physiological nociceptive pain, but also in inflammatory and neuropathic pain (So et al., 2015).
The role of TLR-4-induced inflammation via calcium channels has been reported. Activation of TLR-4 through LPS-induced cytokine production results in an imbalance of basal calcium and store-operated calcium channels, particularly TRPM2, in microglia through Orai-1, a downstream protein of TLR4-MAPK (Birla et al., 2022). Various proinflammatory cytokines are produced during the immune response to an infection. The absence of TRPM2 channels improved neuroethology and pathological changes and decreased inflammatory cytokines and apoptosis proteins in TRPM2 knock-out mice in the LPS-induced sepsis model associated with encephalopathy infection (Zhu et al., 2019). In a study, where the LPS-induced production of IL-6, IL-8, and TNF-α in THP1 monocytic cells was significantly attenuated by using shRNA to reduce TRPM2 expression (Wehrhahn et al., 2010). Also, increased cell-free heme activates TLR4-mediated ER stress and ROS leading to spinal microglial activation and neuroinflammation, contributing to acute and chronic pain (Lei et al., 2021). Ketamine, an anesthetic agent, attenuated hypoxia-induced TRPM2-mediated Ca2+ influx, ROS, NMDAR, and ROS in neuronal cell (SH-SY5Y) death (Osmanlioğlu, 2022).
There is a reciprocal relationship between neuroinflammation and oxidative stress. These two processes are not independent but rather complex reciprocal interactions (Teixeira-Santos et al., 2020). NOX activity upregulates the expression of proinflammatory cytokines and proinflammatory cytokines increase ROS through NOX (Kallenborn-Gerhardt et al., 2013). Initiation of TLR4 downstream pathway requires NOX activity (Haslund-Vinding et al., 2017) and TLR-4 receptors may be required for NOX expression (Lim et al., 2013), thus both are dependent on each other. These interdependent complex mechanisms that regulate neuropathic pain require Ca2+ ion, which is well controlled by the TRPM2 ion channel sensitive to cellular redox potential (Ogawa et al., 2016), and hence modulated TRPM2 can alleviate neuropathic pain.
NOX 2 and NOX 4 have been implicated in chronic pain mechanisms such as neuropathic pain (Grace et al., 2016). Inhibition of NOX and its isoforms 2 and 4 have shown to be beneficial in neuropathic pain models (Kallenborn-Gerhardt et al., 2013). Macrophages produce ROS during immune response, and it is well regulated by NOX. This enzyme activity can be regulated by Ca2+, which is controlled by protein kinase C α and membrane potential (Bedard and Krause, 2007). However, TRPM2KO mice showed greater mortality than WT in LPS-induced inflammation (Di et al., 2012). In addition, TRPM2 deficiency reduces oxidized low-density lipoprotein uptake by macrophages, thereby minimizing macrophage infiltration and inflammatory response by modulation of NOX (Zong et al., 2022).
However, the molecular mechanism underlying the interaction between TRPM2 and TLR4s and the quantum of impact in acute and chronic neuropathic pain remains unclear. It would be worth focusing on TRPM2 interaction with TLR4 in the context of neuropathic pain. Mechanistic studies that focus on TLR4-TRPM2 interaction will open new pathway interactions for treating inflammation-associated diseases such as neuropathic pain.
This review summarizes the functional role and mechanisms of TRPM2 that mediate Ca2+ influx under oxidative stress and inflammation linked with TLR-4. The reported literature in this review demonstrates the role of the TRPM2 ion channel in various pathological states and, more importantly, neuropathic pain. Many research findings support the evidence that oxidative stress and LPS-induced TLR4-mediated inflammation exert their effects mediated by the TRPM2 channel in neuronal and non-neuronal tissues. The existing and growing scientific evidence of TRPM2 in various pain pathologies has made researchers and the pharmaceutical industry focus on the development of novel targets for diagnostic and therapeutic approaches for pain treatment. This review also demonstrates the role of various inhibitors/drugs that modulate neuropathic pain in various disease states. However, the role of TRPM2 ion channels and their association with Toll-like receptors in pain management needs to be studied in more detail for the development of effective strategies to treat neuropathic pain.
VM: Writing–original draft, Investigation. AR: Writing–review and editing, Investigation. ZK: Investigation, Writing–review and editing. SQ: Data curation, Formal Analysis, Writing–review and editing, Investigation, Visualization. FD: Conceptualization, Data curation, Formal Analysis, Funding acquisition, Investigation, Methodology, Resources, Software, Supervision, Validation, Visualization, Writing–review and editing.
The author(s) declare that financial support was received for the research, authorship, and/or publication of this article.
The authors of this review were in part supported by the Fisch College of Pharmacy, at UT-Tyler, Endowment Fellowship funds, and UT-Tyler Office of Research and Scholarship funds.
The authors declare that the research was conducted in the absence of any commercial or financial relationships that could be construed as a potential conflict of interest.
All claims expressed in this article are solely those of the authors and do not necessarily represent those of their affiliated organizations, or those of the publisher, the editors and the reviewers. Any product that may be evaluated in this article, or claim that may be made by its manufacturer, is not guaranteed or endorsed by the publisher.
Aizawa, N., Fujimori, Y., Kobayashi, J. I., Nakanishi, O., Hirasawa, H., Kume, H., et al. (2018). KPR-2579, a novel TRPM8 antagonist, inhibits acetic acid-induced bladder afferent hyperactivity in rats. Neurourol. Urodyn. 37 (5), 1633–1640. doi:10.1002/nau.23532
Aizawa, N., and Fujita, T. (2022). The TRPM8 channel as a potential therapeutic target for bladder hypersensitive disorders. J. Smooth Muscle Res. 58 (0), 11–21. doi:10.1540/jsmr.58.11
Akpınar, H., Nazıroğlu, M., Övey, İ. S., Çiğ, B., and Akpınar, O. (2016). The neuroprotective action of dexmedetomidine on apoptosis, calcium entry and oxidative stress in cerebral ischemia-induced rats: contribution of TRPM2 and TRPV1 channels. Sci. Rep. 6 (1), 37196. doi:10.1038/srep37196
Akyuva, Y., and Nazıroğlu, M. (2020). Resveratrol attenuates hypoxia-induced neuronal cell death, inflammation and mitochondrial oxidative stress by modulation of TRPM2 channel. Sci. Rep. 10 (1), 6449. doi:10.1038/s41598-020-63577-5
Akyuva, Y., Nazıroğlu, M., and Yıldızhan, K. (2021). Selenium prevents interferon-gamma induced activation of TRPM2 channel and inhibits inflammation, mitochondrial oxidative stress, and apoptosis in microglia. Metab. Brain Dis. 36, 285–298. doi:10.1007/s11011-020-00624-0
Alawieyah Syed Mortadza, S., Sim, J. A., Neubrand, V. E., and Jiang, L. H. (2018). A critical role of TRPM2 channel in Aβ42 -induced microglial activation and generation of tumor necrosis factor-α. Glia 66 (3), 562–575. doi:10.1002/glia.23265
Alexzander Asea, M. R., Kabingu, E., Boch, J. A., Baré, O., Auron, P. E., Stevenson, M. A., et al. (2002). Novel signal transduction pathway utilized by extracellular HSP70: ROLE of toll-LIKE receptor (TLR) 2 and TLR4. J. Biol. Chem. 277 (17), 15028–15034. doi:10.1074/jbc.M200497200
Ali, E. S., Chakrabarty, B., Ramproshad, S., Mondal, B., Kundu, N., Sarkar, C., et al. (2023). TRPM2-mediated Ca2+ signaling as a potential therapeutic target in cancer treatment: an updated review of its role in survival and proliferation of cancer cells. Cell Commun. Signal. 21 (1), 145. doi:10.1186/s12964-023-01149-6
Alim, I., Teves, L., Li, R., Mori, Y., and Tymianski, M. (2013). Modulation of NMDAR subunit expression by TRPM2 channels regulates neuronal vulnerability to ischemic cell death. J. Neurosci. 33 (44), 17264–17277. doi:10.1523/JNEUROSCI.1729-13.2013
Aminzadeh, M., Roghani, M., Sarfallah, A., and Riazi, G. H. (2018). TRPM2 dependence of ROS-induced NLRP3 activation in Alzheimer's disease. Int. Immunopharmacol. 54, 78–85. doi:10.1016/j.intimp.2017.10.024
Asiri, Y. I., and Hassan, M. Z. (2023). An overview of ion channels therapeutics in the treatment of pain. Arabian J. Chem. 16 (10), 105180. doi:10.1016/j.arabjc.2023.105180
Atilgan, F. A., Atescelik, M., Yilmaz, M., Turk, A., Gurger, M., Goktekin, M. C., et al. (2022). Effects of N-acetyl cysteine on TRPM2 expression in kidney and liver tissues following malathion intoxication. Biotech. and Histochem. 97 (5), 340–346. doi:10.1080/10520295.2021.1986639
Backonja, M.-M. (2003). Defining neuropathic pain. Anesth. and Analgesia 97 (3), 785–790. doi:10.1213/01.ANE.0000062826.70846.8D
Bamps, D., Blockeel, A. J., Dreesen, E., Marynissen, H., Laenen, J., Van Hecken, A., et al. (2023). TRPA1 antagonist LY3526318 inhibits the cinnamaldehyde-evoked dermal blood flow increase: translational proof of pharmacology. Clin. Pharmacol. Ther. 114 (5), 1093–1103. doi:10.1002/cpt.3024
Baron, R. (2006). Mechanisms of disease: neuropathic pain—a clinical perspective. Nat. Clin. Pract. Neurol. 2 (2), 95–106. doi:10.1038/ncpneuro0113
Bayir, M. H., Yıldızhan, K., and Altındağ, F. (2023). Effect of hesperidin on sciatic nerve damage in STZ-induced diabetic neuropathy: modulation of TRPM2 channel. Neurotox. Res. 41 (6), 638–647. doi:10.1007/s12640-023-00657-0
Bedard, K., and Krause, K.-H. (2007). The NOX family of ROS-generating NADPH oxidases: physiology and pathophysiology. Physiol. Rev. 87 (1), 245–313. doi:10.1152/physrev.00044.2005
Belrose, J. C., Xie, Y. F., Gierszewski, L. J., MacDonald, J. F., and Jackson, M. F. (2012). Loss of glutathione homeostasis associated with neuronal senescence facilitates TRPM2 channel activation in cultured hippocampal pyramidal neurons. Mol. Brain 5 (1), 11. doi:10.1186/1756-6606-5-11
Bettoni, I., Comelli, F., Rossini, C., Granucci, F., Giagnoni, G., Peri, F., et al. (2008). Glial TLR4 receptor as new target to treat neuropathic pain: efficacy of a new receptor antagonist in a model of peripheral nerve injury in mice. Glia 56 (12), 1312–1319. doi:10.1002/glia.20699
Biggs, J. E., Stemkowski, P. L., Knaus, E. E., Chowdhury, M. A., Ballanyi, K., and Smith, P. A. (2015). Suppression of network activity in dorsal horn by gabapentin permeation of TRPV1 channels: implications for drug access to cytoplasmic targets. Neurosci. Lett. 584, 397–402. doi:10.1016/j.neulet.2014.07.033
Birla, H., Xia, J., Gao, X., Zhao, H., Wang, F., Patel, S., et al. (2022). Toll-like receptor 4 activation enhances Orai1-mediated calcium signal promoting cytokine production in spinal astrocytes. Cell calcium 105, 102619. doi:10.1016/j.ceca.2022.102619
Bittner, S., Ruck, T., Fernández-Orth, J., and Meuth, S. G. (2014). TREK-king the blood–brain-barrier. J. Neuroimmune Pharmacol. 9, 293–301. doi:10.1007/s11481-014-9530-8
Blasius, A. L., and Beutler, B. (2010). Intracellular toll-like receptors. Immunity 32 (3), 305–315. doi:10.1016/j.immuni.2010.03.012
Block, M. L., Zecca, L., and Hong, J.-S. (2007). Microglia-mediated neurotoxicity: uncovering the molecular mechanisms. Nat. Rev. Neurosci. 8 (1), 57–69. doi:10.1038/nrn2038
Booth, J., Wilson, H., Jimbo, S., and Mutwiri, G. (2011). Modulation of B cell responses by Toll-like receptors. Cell tissue Res. 343, 131–140. doi:10.1007/s00441-010-1031-3
Bordt, E. A., and Polster, B. M. (2014). NADPH oxidase-and mitochondria-derived reactive oxygen species in proinflammatory microglial activation: a bipartisan affair? Free Radic. Biol. Med. 76, 34–46. doi:10.1016/j.freeradbiomed.2014.07.033
Bouali-Benazzouz, R., Landry, M., Benazzouz, A., and Fossat, P. (2021). Neuropathic pain modeling: focus on synaptic and ion channel mechanisms. Prog. Neurobiol. 201, 102030. doi:10.1016/j.pneurobio.2021.102030
Brown, J., Wang, H., Hajishengallis, G. N., and Martin, M. (2011). TLR-signaling networks: an integration of adaptor molecules, kinases, and cross-talk. J. Dent. Res. 90 (4), 417–427. doi:10.1177/0022034510381264
Burgueno, J. F., Fritsch, J., González, E. E., Landau, K. S., Santander, A. M., Fernández, I., et al. (2021). Epithelial TLR4 signaling activates DUOX2 to induce microbiota-driven tumorigenesis. Gastroenterology 160 (3), 797–808 e6. doi:10.1053/j.gastro.2020.10.031
Caglar, K., Dokuyucu, R., Agturk, G., Tumer, C., Tutuk, O., Gocmen, H. D., et al. (2024). Effect of thymoquinone on transient receptor potential melastatin (TRPM) channels in rats with liver ischemia reperfusion model in rats. Iran. J. Basic Med. Sci. 27, 319–325. doi:10.22038/IJBMS.2023.71990
Checa, J., and Aran, J. M. (2020). Reactive oxygen species: drivers of physiological and pathological processes. J. Inflamm. Res. 13, 1057–1073. doi:10.2147/JIR.S275595
Christianson, C. A., Dumlao, D. S., Stokes, J. A., Dennis, E. A., Svensson, C. I., Corr, M., et al. (2011). Spinal TLR4 mediates the transition to a persistent mechanical hypersensitivity after the resolution of inflammation in serum-transferred arthritis. Pain 152 (12), 2881–2891. doi:10.1016/j.pain.2011.09.020
Chung, M.-K., Asgar, J., Lee, J., Shim, M. S., Dumler, C., and Ro, J. Y. (2015). The role of TRPM2 in hydrogen peroxide-induced expression of inflammatory cytokine and chemokine in rat trigeminal ganglia. Neuroscience 297, 160–169. doi:10.1016/j.neuroscience.2015.03.067
de Almeida, A. S., Pereira, G. C., Brum, E. d. S., Silva, C. R., Antoniazzi, C. T. d. D., Ardisson-Araújo, D., et al. (2021). Role of TRPA1 expressed in bone tissue and the antinociceptive effect of the TRPA1 antagonist repeated administration in a breast cancer pain model. Life Sci. 276, 119469. doi:10.1016/j.lfs.2021.119469
Demirdaş, A., Nazıroğlu, M., and Övey, İ. S. (2017). Duloxetine reduces oxidative stress, apoptosis, and Ca 2+ entry through modulation of TRPM2 and TRPV1 channels in the hippocampus and dorsal root ganglion of rats. Mol. Neurobiol. 54, 4683–4695. doi:10.1007/s12035-016-9992-1
Deng, L., and Chiu, I. M. (2021). Microbes and pain. PLoS Pathog. 17 (4), e1009398. doi:10.1371/journal.ppat.1009398
Di, A., Gao, X. P., Qian, F., Kawamura, T., Han, J., Hecquet, C., et al. (2012). The redox-sensitive cation channel TRPM2 modulates phagocyte ROS production and inflammation. Nat. Immunol. 13 (1), 29–34. doi:10.1038/ni.2171
Duitama, M., Vargas-López, V., Casas, Z., Albarracin, S. L., Sutachan, J. J., and Torres, Y. P. (2020). TRP channels role in pain associated with neurodegenerative diseases. Front. Neurosci. 14, 782. doi:10.3389/fnins.2020.00782
Echeverry, S., Rodriguez, M. J., and Torres, Y. P. (2016). Transient receptor potential channels in microglia: roles in physiology and disease. Neurotox. Res. 30, 467–478. doi:10.1007/s12640-016-9632-6
Eidson, L. N., and Murphy, A. Z. (2013). Blockade of Toll-like receptor 4 attenuates morphine tolerance and facilitates the pain relieving properties of morphine. J. Neurosci. 33 (40), 15952–15963. doi:10.1523/JNEUROSCI.1609-13.2013
Eraslan, E., Tanyeli, A., Polat, E., and Polat, E. (2019). 8-Br-cADPR, a TRPM2 ion channel antagonist, inhibits renal ischemia–reperfusion injury. J. Cell. physiology 234 (4), 4572–4581. doi:10.1002/jcp.27236
Eren-Koçak, E., and Dalkara, T. (2021). Ion channel dysfunction and neuroinflammation in migraine and depression. Front. Pharmacol. 12, 777607. doi:10.3389/fphar.2021.777607
Erridge, C. (2010). Endogenous ligands of TLR2 and TLR4: agonists or assistants? J. Leukoc. Biol. 87 (6), 989–999. doi:10.1189/jlb.1209775
Facchini, F. (2018). Toll-like Receptor 4 (TLR4) therapeutic modulation: a chemical biology approach.
Fan, P. C., Lai, T. H., Hor, C. C., Lee, M. T., Huang, P., Sieghart, W., et al. (2018). The α6 subunit-containing GABA(A) receptor: a novel drug target for inhibition of trigeminal activation. Neuropharmacology 140, 1–13. doi:10.1016/j.neuropharm.2018.07.017
Fehder, W. P., Tuluc, F., Ho, W.-Z., and Douglas, S. D. (2007). “Macrophages,” in Encyclopedia of stress. Editor G. Fink Second Edition (New York: Academic Press), 634–639.
Finnerup, N. B., Kuner, R., and Jensen, T. S. (2020). Neuropathic pain: from mechanisms to treatment. Physiol. Rev. 101, 259–301. doi:10.1152/physrev.00045.2019
Finnerup, N. B., Kuner, R., and Jensen, T. S. (2021). Neuropathic pain: from mechanisms to treatment. Physiol. Rev. 101 (1), 259–301. doi:10.1152/physrev.00045.2019
Fonfria, E., Mattei, C., Hill, K., Brown, J. T., Randall, A., Benham, C. D., et al. (2006). TRPM2 is elevated in the tMCAO stroke model, transcriptionally regulated, and functionally expressed in C13 microglia. J. Recept. Signal Transduct. 26 (3), 179–198. doi:10.1080/10799890600637522
Gao, G., Wang, W., Tadagavadi, R. K., Briley, N. E., Love, M. I., Miller, B. A., et al. (2014). TRPM2 mediates ischemic kidney injury and oxidant stress through RAC1. J. Clin. investigation 124 (11), 4989–5001. doi:10.1172/JCI76042
Gees, M., Colsoul, B., and Nilius, B. (2010). The role of transient receptor potential cation channels in Ca2+ signaling. Cold Spring Harb. Perspect. Biol. 2 (10), a003962. doi:10.1101/cshperspect.a003962
Gelderblom, M., Melzer, N., Schattling, B., Göb, E., Hicking, G., Arunachalam, P., et al. (2014). Transient receptor potential melastatin subfamily member 2 cation channel regulates detrimental immune cell invasion in ischemic stroke. Stroke 45 (11), 3395–3402. doi:10.1161/STROKEAHA.114.005836
Görlach, A., Bertram, K., Hudecova, S., and Krizanova, O. (2015). Calcium and ROS: a mutual interplay. Redox Biol. 6, 260–271. doi:10.1016/j.redox.2015.08.010
Gosset, J. R., Beaumont, K., Matsuura, T., Winchester, W., Attkins, N., Glatt, S., et al. (2017). A cross-species translational pharmacokinetic-pharmacodynamic evaluation of core body temperature reduction by the TRPM8 blocker PF-05105679. Eur. J. Pharm. Sci. 109s, S161–s167. doi:10.1016/j.ejps.2017.06.009
Grace, P. M., Gaudet, A. D., Staikopoulos, V., Maier, S. F., Hutchinson, M. R., Salvemini, D., et al. (2016). Nitroxidative signaling mechanisms in pathological pain. Trends Neurosci. 39 (12), 862–879. doi:10.1016/j.tins.2016.10.003
Gunawardena, D., Raju, R., and Münch, G. (2019). Hydrogen peroxide mediates pro-inflammatory cell-to-cell signaling: a new therapeutic target for inflammation? Neural Regen. Res. 14 (8), 1430–1437. doi:10.4103/1673-5374.253529
Haraguchi, K., Kawamoto, A., Isami, K., Maeda, S., Kusano, A., Asakura, K., et al. (2012). TRPM2 contributes to inflammatory and neuropathic pain through the aggravation of pronociceptive inflammatory responses in mice. J. Neurosci. 32 (11), 3931–3941. doi:10.1523/JNEUROSCI.4703-11.2012
Hasan, R., and Zhang, X. (2018). Ca2+ regulation of TRP ion channels. Int. J. Mol. Sci. 19 (4), 1256. doi:10.3390/ijms19041256
Haslund-Vinding, J., McBean, G., Jaquet, V., and Vilhardt, F. (2017). NADPH oxidases in oxidant production by microglia: activating receptors, pharmacology and association with disease. Br. J. Pharmacol. 174 (12), 1733–1749. doi:10.1111/bph.13425
Hecquet, C. M., and Malik, A. B. (2009). Role of H2O2-activated TRPM2 calcium channel in oxidant-induced endothelial injury. Thrombosis haemostasis 101 (04), 619–625. doi:10.1160/th08-10-0641
Hill, K., McNulty, S., and Randall, A. (2004). Inhibition of TRPM2 channels by the antifungal agents clotrimazole and econazole. Naunyn-Schmiedeberg's archives Pharmacol. 370, 227–237. doi:10.1007/s00210-004-0981-y
Horne, D. B., Biswas, K., Brown, J., Bartberger, M. D., Clarine, J., Davis, C. D., et al. (2018). Discovery of TRPM8 antagonist (S)-6-(((3-Fluoro-4-(trifluoromethoxy)phenyl)(3-fluoropyridin-2-yl)methyl)carbamoyl)nicotinic acid (AMG 333), a clinical candidate for the treatment of migraine. J. Med. Chem. 61 (18), 8186–8201. doi:10.1021/acs.jmedchem.8b00518
Hosseini, A. M., Majidi, J., Baradaran, B., and Yousefi, M. (2015). Toll-like receptors in the pathogenesis of autoimmune diseases. Adv. Pharm. Bull. 5 (Suppl. 1), 605–614. doi:10.15171/apb.2015.082
Hu, X., Wu, L., Liu, X., Zhang, Y., Xu, M., Fang, Q., et al. (2021). Deficiency of ROS-activated TRPM2 channel protects neurons from cerebral ischemia-reperfusion injury through upregulating autophagy. Oxidative Med. Cell. Longev. 2021, 7356266–7356312. doi:10.1155/2021/7356266
Huang, P., Qu, C., Rao, Z., Wu, D., and Zhao, J. (2024). Bidirectional regulation mechanism of TRPM2 channel: role in oxidative stress, inflammation and ischemia-reperfusion injury. Front. Immunol. 15, 1391355. doi:10.3389/fimmu.2024.1391355
Huang, S., Wang, H., Turlova, E., Abussaud, A., Ji, X., Britto, L. R., et al. (2017). GSK-3β inhibitor TDZD-8 reduces neonatal hypoxic-ischemic brain injury in mice. CNS Neurosci. and Ther. 23 (5), 405–415. doi:10.1111/cns.12683
Hutchinson, M. R., Zhang, Y., Brown, K., Coats, B. D., Shridhar, M., Sholar, P. W., et al. (2008). Non-stereoselective reversal of neuropathic pain by naloxone and naltrexone: involvement of toll-like receptor 4 (TLR4). Eur. J. Neurosci. 28 (1), 20–29. doi:10.1111/j.1460-9568.2008.06321.x
Ishii, M., Shimizu, S., Hara, Y., Hagiwara, T., Miyazaki, A., Mori, Y., et al. (2006). Intracellular-produced hydroxyl radical mediates H2O2-induced Ca2+ influx and cell death in rat beta-cell line RIN-5F. Cell calcium 39 (6), 487–494. doi:10.1016/j.ceca.2006.01.013
Jang, Y., Cho, P. S., Yang, Y. D., and Hwang, S. W. (2018). Nociceptive roles of TRPM2 ion channel in pathologic pain. Mol. Neurobiol. 55, 6589–6600. doi:10.1007/s12035-017-0862-2
Jentsch, T. J. (2016). VRACs and other ion channels and transporters in the regulation of cell volume and beyond. Nat. Rev. Mol. Cell Biol. 17 (5), 293–307. doi:10.1038/nrm.2016.29
Jia, J., Verma, S., Nakayama, S., Quillinan, N., Grafe, M. R., Hurn, P. D., et al. (2011). Sex differences in neuroprotection provided by inhibition of TRPM2 channels following experimental stroke. J. Cereb. Blood Flow and Metabolism 31 (11), 2160–2168. doi:10.1038/jcbfm.2011.77
Jiang, L.-H., Li, X., Syed Mortadza, S. A., Lovatt, M., and Yang, W. (2018). The TRPM2 channel nexus from oxidative damage to Alzheimer’s pathologies: an emerging novel intervention target for age-related dementia. Ageing Res. Rev. 47, 67–79. doi:10.1016/j.arr.2018.07.002
Kallenborn-Gerhardt, W., Schröder, K., Geisslinger, G., and Schmidtko, A. (2013). NOXious signaling in pain processing. Pharmacol. and Ther. 137 (3), 309–317. doi:10.1016/j.pharmthera.2012.11.001
Kamei, J., Aizawa, N., Nakagawa, T., Kaneko, S., Fujimura, T., Homma, Y., et al. (2021). Lacking transient receptor potential melastatin 2 attenuates lipopolysaccharide-induced bladder inflammation and its associated hypersensitivity in mice. Int. J. Urology 28 (1), 107–114. doi:10.1111/iju.14389
Kaneko, S., Kawakami, S., Hara, Y., Wakamori, M., Itoh, E., Minami, T., et al. (2006). A critical role of TRPM2 in neuronal cell death by hydrogen peroxide. J. Pharmacol. Sci. 101 (1), 66–76. doi:10.1254/jphs.fp0060128
Kaneko, Y., and Szallasi, A. (2014). Transient receptor potential (TRP) channels: a clinical perspective. Br. J. Pharmacol. 171 (10), 2474–2507. doi:10.1111/bph.12414
Kassmann, M., Harteneck, C., Zhu, Z., Nürnberg, B., Tepel, M., and Gollasch, M. (2013). Transient receptor potential vanilloid 1 (TRPV 1), TRPV 4, and the kidney. Acta physiol. 207 (3), 546–564. doi:10.1111/apha.12051
Kawai, T., and Akira, S. (2007). TLR signaling. Semin. Immunol. 19 (1), 24–32. doi:10.1016/j.smim.2006.12.004
Kawasaki, T., and Kawai, T. (2014). Toll-like receptor signaling pathways. Front. Immunol. 5, 461. doi:10.3389/fimmu.2014.00461
Kerstman, E., Ahn, S., Battu, S., Tariq, S., and Grabois, M. (2013). Neuropathic pain. Handb. Clin. neurology 110, 175–187. doi:10.1016/B978-0-444-52901-5.00015-0
Kew, J. N., and Davies, C. H. (2010). Ion channels: from structure to function. Oxford University Press.
Khanahmad, H., Mirbod, S. M., Karimi, F., Kharazinejad, E., Owjfard, M., Najaflu, M., et al. (2022). Pathological mechanisms induced by TRPM2 ion channels activation in renal ischemia-reperfusion injury. Mol. Biol. Rep. 49 (11), 11071–11079. doi:10.1007/s11033-022-07836-w
Kheradpezhouh, E., Ma, L., Morphett, A., Barritt, G. J., and Rychkov, G. Y. (2014). TRPM2 channels mediate acetaminophen-induced liver damage. Proc. Natl. Acad. Sci. 111 (8), 3176–3181. doi:10.1073/pnas.1322657111
Klionsky, L., Tamir, R., Gao, B., Wang, W., Immke, D. C., Nishimura, N., et al. (2007). Species-specific pharmacology of Trichloro(sulfanyl)ethyl benzamides as transient receptor potential ankyrin 1 (TRPA1) antagonists. Mol. Pain 3, 39. doi:10.1186/1744-8069-3-39
Knowles, H., Li, Y., and Perraud, A.-L. (2013). The TRPM2 ion channel, an oxidative stress and metabolic sensor regulating innate immunity and inflammation. Immunol. Res. 55, 241–248. doi:10.1007/s12026-012-8373-8
Kraft, R., Grimm, C., Frenzel, H., and Harteneck, C. (2006). Inhibition of TRPM2 cation channels by N-(p-amylcinnamoyl) anthranilic acid. Br. J. Pharmacol. 148 (3), 264–273. doi:10.1038/sj.bjp.0706739
Kraft, R., Grimm, C., Grosse, K., Hoffmann, A., Sauerbruch, S., Kettenmann, H., et al. (2004). Hydrogen peroxide and ADP-ribose induce TRPM2-mediated calcium influx and cation currents in microglia. Am. J. Physiology-Cell Physiology 286 (1), C129–C137. doi:10.1152/ajpcell.00331.2003
Kuang, X., Huang, Y., Gu, H. F., Zu, X. Y., Zou, W. Y., Song, Z. B., et al. (2012). Effects of intrathecal epigallocatechin gallate, an inhibitor of Toll-like receptor 4, on chronic neuropathic pain in rats. Eur. J. Pharmacol. 676 (1-3), 51–56. doi:10.1016/j.ejphar.2011.11.037
Kuffler, D. P. (2020). Mechanisms for reducing neuropathic pain. Mol. Neurobiol. 57 (1), 67–87. doi:10.1007/s12035-019-01757-9
Kuzmich, N. N., Sivak, K. V., Chubarev, V. N., Porozov, Y. B., Savateeva-Lyubimova, T. N., and Peri, F. (2017). TLR4 signaling pathway modulators as potential therapeutics in inflammation and sepsis. Vaccines (Basel) 5 (4), 34. doi:10.3390/vaccines5040034
Kwilasz, A. J., Clements, M. A., Larson, T. A., Harris, K. M., Litwiler, S. T., Woodall, B. J., et al. (2022). Involvement of TLR2-TLR4, NLRP3, and IL-17 in pain induced by a novel Sprague-Dawley rat model of experimental autoimmune encephalomyelitis. Front. Pain Res. (Lausanne) 3, 932530. doi:10.3389/fpain.2022.932530
Lambert, G. A., Davis, J. B., Appleby, J. M., Chizh, B. A., Hoskin, K. L., and Zagami, A. S. (2009). The effects of the TRPV1 receptor antagonist SB-705498 on trigeminovascular sensitisation and neurotransmission. Naunyn Schmiedeb. Arch. Pharmacol. 380 (4), 311–325. doi:10.1007/s00210-009-0437-5
Lei, J., Paul, J., Wang, Y., Gupta, M., Vang, D., Thompson, S., et al. (2021). Heme causes pain in sickle mice via toll-like receptor 4-mediated reactive oxygen species-and endoplasmic reticulum stress-induced glial activation. Antioxidants and Redox Signal. 34 (4), 279–293. doi:10.1089/ars.2019.7913
Li, W., Wang, K., Liu, Y., Wu, H., He, Y., Li, C., et al. (2022). A novel drug combination of mangiferin and cinnamic acid alleviates rheumatoid arthritis by inhibiting TLR4/nfκb/NLRP3 activation-induced pyroptosis. Front. Immunol. 13, 912933. doi:10.3389/fimmu.2022.912933
Li, Y., Yin, C., Li, X., Liu, B., Wang, J., Zheng, X., et al. (2019). Electroacupuncture alleviates paclitaxel-induced peripheral neuropathic pain in rats via suppressing TLR4 signaling and TRPV1 upregulation in sensory neurons. Int. J. Mol. Sci. 20 (23), 5917. doi:10.3390/ijms20235917
Lim, H., Kim, D., and Lee, S. J. (2013). Toll-like receptor 2 mediates peripheral nerve injury-induced NADPH oxidase 2 expression in spinal cord microglia. J. Biol. Chem. 288 (11), 7572–7579. doi:10.1074/jbc.M112.414904
Liu, Y., Liu, W., Wang, X., Wan, Z., and Leng, Y. (2018). Dexmedetomidine relieves acute inflammatory visceral pain in rats through the ERK pathway, toll-like receptor signaling, and TRPV1 channel. J. Mol. Neurosci. 66 (2), 279–290. doi:10.1007/s12031-018-1172-5
Loeser, J. D., and Melzack, R. (1999). Pain: an overview. lancet 353 (9164), 1607–1609. doi:10.1016/S0140-6736(99)01311-2
Lolignier, S., Gkika, D., Andersson, D., Leipold, E., Vetter, I., Viana, F., et al. (2016). New insight in cold pain: role of ion channels, modulation, and clinical perspectives. J. Neurosci. 36 (45), 11435–11439. doi:10.1523/JNEUROSCI.2327-16.2016
López-Requena, A., Boonen, B., Van Gerven, L., Hellings, P. W., Alpizar, Y. A., and Talavera, K. (2017). “Roles of Neuronal TRP Channels in Neuroimmune Interactions,” in Neurobiology of TRP Channels. Editor T. L. R. Emir (CRC Press/Taylor & Francis), 277–294. doi:10.4324/9781315152837-15
Malko, P., and Jiang, L.-H. (2020). TRPM2 channel-mediated cell death: an important mechanism linking oxidative stress-inducing pathological factors to associated pathological conditions. Redox Biol. 37, 101755. doi:10.1016/j.redox.2020.101755
Malko, P., Syed Mortadza, S. A., McWilliam, J., and Jiang, L. H. (2019). TRPM2 channel in microglia as a new player in neuroinflammation associated with a spectrum of central nervous system pathologies. Front. Pharmacol. 10, 239. doi:10.3389/fphar.2019.00239
Maqboul, A., and Elsadek, B. (2018). Expression profiles of TRPV1, TRPV4, TLR4 and ERK1/2 in the dorsal root ganglionic neurons of a cancer-induced neuropathy rat model. PeerJ 6, e4622. doi:10.7717/peerj.4622
Matsumoto, K., Takagi, K., Kato, A., Ishibashi, T., Mori, Y., Tashima, K., et al. (2016). Role of transient receptor potential melastatin 2 (TRPM2) channels in visceral nociception and hypersensitivity. Exp. Neurol. 285, 41–50. doi:10.1016/j.expneurol.2016.09.001
McLennan, A. (2006). The Nudix hydrolase superfamily. Cell. Mol. Life Sci. CMLS 63, 123–143. doi:10.1007/s00018-005-5386-7
Mehta, D., Ahmmed, G. U., Paria, B. C., Holinstat, M., Voyno-Yasenetskaya, T., Tiruppathi, C., et al. (2003). RhoA interaction with inositol 1, 4, 5-trisphosphate receptor and transient receptor potential channel-1 regulates Ca2+ entry: role in signaling increased endothelial permeability. J. Biol. Chem. 278 (35), 33492–33500. doi:10.1074/jbc.M302401200
Mittal, M., Nepal, S., Tsukasaki, Y., Hecquet, C. M., Soni, D., Rehman, J., et al. (2017). Neutrophil activation of endothelial cell-expressed TRPM2 mediates transendothelial neutrophil migration and vascular injury. Circulation Res. 121 (9), 1081–1091. doi:10.1161/CIRCRESAHA.117.311747
Miyake, T., Shirakawa, H., Kusano, A., Sakimoto, S., Konno, M., Nakagawa, T., et al. (2014). TRPM2 contributes to LPS/IFNγ-induced production of nitric oxide via the p38/JNK pathway in microglia. Biochem. biophysical Res. Commun. 444 (2), 212–217. doi:10.1016/j.bbrc.2014.01.022
Mohammadi, M. (2019). Role of oxidized phospholipids in inflammatory pain. Universität Würzburg [Doctoral dissertation].
Montell, C., and Rubin, G. M. (1989). Molecular characterization of the Drosophila trp locus: a putative integral membrane protein required for phototransduction. Neuron 2 (4), 1313–1323. doi:10.1016/0896-6273(89)90069-x
Mortadza, S. S., Sim, J. A., Stacey, M., and Jiang, L. H. (2017). Signalling mechanisms mediating Zn2+-induced TRPM2 channel activation and cell death in microglial cells. Sci. Rep. 7 (1), 45032. doi:10.1038/srep45032
Myers, R. R., Campana, W. M., and Shubayev, V. I. (2006). The role of neuroinflammation in neuropathic pain: mechanisms and therapeutic targets. Drug Discov. today 11 (1-2), 8–20. doi:10.1016/S1359-6446(05)03637-8
Nazıroğlu, M. (2017). Activation of TRPM2 and TRPV1 channels in dorsal root ganglion by NADPH oxidase and protein kinase C molecular pathways: a patch clamp study. J. Mol. Neurosci. 61, 425–435. doi:10.1007/s12031-017-0882-4
Nazıroğlu, M., and Braidy, N. (2017). Thermo-sensitive TRP channels: novel targets for treating chemotherapy-induced peripheral pain. Front. Physiology 8, 1040. doi:10.3389/fphys.2017.01040
Nazıroğlu, M., Çiğ, B., Yazğan, Y., Schwaerzer, G. K., Theilig, F., and Pecze, L. (2019). Albumin evokes Ca2+-induced cell oxidative stress and apoptosis through TRPM2 channel in renal collecting duct cells reduced by curcumin. Sci. Rep. 9 (1), 12403. doi:10.1038/s41598-019-48716-x
Nazıroğlu, M., Lückhoff, A., and Jüngling, E. (2007). Antagonist effect of flufenamic acid on TRPM2 cation channels activated by hydrogen peroxide. Cell Biochem. Funct. 25 (4), 383–387. doi:10.1002/cbf.1310
Nazıroğlu, M., Özgül, C., Çiğ, B., Doğan, S., and Uğuz, A. C. (2011). Glutathione modulates Ca 2+ influx and oxidative toxicity through TRPM2 channel in rat dorsal root ganglion neurons. J. Membr. Biol. 242, 109–118. doi:10.1007/s00232-011-9382-6
Naziroğlu, M., Uğuz, A. C., Ismailoğlu, Ö., Çiğ, B., Özgül, C., and Borcak, M. (2013). Role of TRPM2 cation channels in dorsal root ganglion of rats after experimental spinal cord injury. Muscle and Nerve 48 (6), 945–950. doi:10.1002/mus.23844
Ni, H. M., Bockus, A., Boggess, N., Jaeschke, H., and Ding, W. X. (2012). Activation of autophagy protects against acetaminophen-induced hepatotoxicity. Hepatology 55 (1), 222–232. doi:10.1002/hep.24690
Nicotra, L., Loram, L. C., Watkins, L. R., and Hutchinson, M. R. (2012). Toll-like receptors in chronic pain. Exp. Neurol. 234 (2), 316–329. doi:10.1016/j.expneurol.2011.09.038
Noreen, M., and Arshad, M. (2015). Association of TLR1, TLR2, TLR4, TLR6, and TIRAP polymorphisms with disease susceptibility. Immunol. Res. 62 (2), 234–252. doi:10.1007/s12026-015-8640-6
Ogawa, N., Kurokawa, T., and Mori, Y. (2016). Sensing of redox status by TRP channels. Cell calcium 60 (2), 115–122. doi:10.1016/j.ceca.2016.02.009
Onakpoya, I. J., Thomas, E. T., Lee, J. J., Goldacre, B., and Heneghan, C. J. (2019). Benefits and harms of pregabalin in the management of neuropathic pain: a rapid review and meta-analysis of randomised clinical trials. BMJ Open 9 (1), e023600. doi:10.1136/bmjopen-2018-023600
Osmanlioğlu, H. Ö. (2022). Ketamine attenuates hypoxia-induced cell death and oxidative toxicity via inhibition of the TRPM2 channel in neuronal cells. J. Cell. Neurosci. Oxidative Stress 14 (3), 1095–1104. doi:10.37212/jcnos.1325007
Osmanlıoğlu, H. Ö., Yıldırım, M. K., Akyuva, Y., Yıldızhan, K., and Nazıroğlu, M. (2020). Morphine induces apoptosis, inflammation, and mitochondrial oxidative stress via activation of TRPM2 channel and nitric oxide signaling pathways in the hippocampus. Mol. Neurobiol. 57 (8), 3376–3389. doi:10.1007/s12035-020-01975-6
Övey, I., and Naziroğlu, M. (2015). Homocysteine and cytosolic GSH depletion induce apoptosis and oxidative toxicity through cytosolic calcium overload in the hippocampus of aged mice: involvement of TRPM2 and TRPV1 channels. Neuroscience 284, 225–233. doi:10.1016/j.neuroscience.2014.09.078
Özdemir, Ü. S., Nazıroğlu, M., Şenol, N., and Ghazizadeh, V. (2016). Hypericum perforatum attenuates spinal cord injury-induced oxidative stress and apoptosis in the dorsal root ganglion of rats: involvement of TRPM2 and TRPV1 channels. Mol. Neurobiol. 53, 3540–3551. doi:10.1007/s12035-015-9292-1
Pasierski, M., and Szulczyk, B. (2022). Beneficial effects of capsaicin in disorders of the central nervous system. Molecules 27 (8), 2484. doi:10.3390/molecules27082484
Patel, A. R., Ritzel, R., McCullough, L. D., and Liu, F. (2013). Microglia and ischemic stroke: a double-edged sword. Int. J. physiology 5 (2), 73–90. pathophysiology and pharmacology.
Perraud, A.-L., Fleig, A., Dunn, C. A., Bagley, L. A., Launay, P., Schmitz, C., et al. (2001). ADP-ribose gating of the calcium-permeable LTRPC2 channel revealed by Nudix motif homology. Nature 411 (6837), 595–599. doi:10.1038/35079100
Powers, K. A., Szászi, K., Khadaroo, R. G., Tawadros, P. S., Marshall, J. C., Kapus, A., et al. (2006). Oxidative stress generated by hemorrhagic shock recruits Toll-like receptor 4 to the plasma membrane in macrophages. J. Exp. Med. 203 (8), 1951–1961. doi:10.1084/jem.20060943
Quan, J., Lee, J. Y., Choi, H., Kim, Y. C., Yang, S., Jeong, J., et al. (2022). Effect of pregabalin combined with duloxetine and tramadol on allodynia in chronic postischemic pain and spinal nerve ligation mouse models. Pharmaceutics 14 (3), 670. doi:10.3390/pharmaceutics14030670
Raghunatha, P., Vosoughi, A., Kauppinen, T. M., and Jackson, M. F. (2020). Microglial NMDA receptors drive pro-inflammatory responses via PARP-1/TRMP2 signaling. Glia 68 (7), 1421–1434. doi:10.1002/glia.23790
Ramachandran, R., Wang, Z., Saavedra, C., DiNardo, A., Corr, M., Powell, S. B., et al. (2019). Role of Toll-like receptor 4 signaling in mast cell-mediated migraine pain pathway. Mol. Pain 15, 1744806919867842. doi:10.1177/1744806919867842
Rather, M. A., Khan, A., Wang, L., Jahan, S., Rehman, M. U., Makeen, H. A., et al. (2023). TRP channels: role in neurodegenerative diseases and therapeutic targets. Heliyon 9 (6), e16910. doi:10.1016/j.heliyon.2023.e16910
Ren, K., and Dubner, R. (2010). Interactions between the immune and nervous systems in pain. Nat. Med. 16 (11), 1267–1276. doi:10.1038/nm.2234
Round, P., Priestley, A., and Robinson, J. (2011). An investigation of the safety and pharmacokinetics of the novel TRPV1 antagonist XEN-D0501 in healthy subjects. Br. J. Clin. Pharmacol. 72 (6), 921–931. doi:10.1111/j.1365-2125.2011.04040.x
Roy, A., Srivastava, M., Saqib, U., Liu, D., Faisal, S. M., Sugathan, S., et al. (2016). Potential therapeutic targets for inflammation in toll-like receptor 4 (TLR4)-mediated signaling pathways. Int. Immunopharmacol. 40, 79–89. doi:10.1016/j.intimp.2016.08.026
Ruck, T., Bock, S., Pfeuffer, S., Schroeter, C. B., Cengiz, D., Marciniak, P., et al. (2022). K2P18. 1 translates T cell receptor signals into thymic regulatory T cell development. Cell Res. 32 (1), 72–88. doi:10.1038/s41422-021-00580-z
Sahoo, B. R. (2020). Structure of fish Toll-like receptors (TLR) and NOD-like receptors (NLR). Int. J. Biol. Macromol. 161, 1602–1617. doi:10.1016/j.ijbiomac.2020.07.293
Samanta, A., Hughes, T. E., and Moiseenkova-Bell, V. Y. (2018). Transient receptor potential (TRP) channels. Membr. protein complexes Struct. Funct. 87, 141–165. doi:10.1007/978-981-10-7757-9_6
Santoni, G., Cardinali, C., Morelli, M. B., Santoni, M., Nabissi, M., and Amantini, C. (2015). Danger-and pathogen-associated molecular patterns recognition by pattern-recognition receptors and ion channels of the transient receptor potential family triggers the inflammasome activation in immune cells and sensory neurons. J. neuroinflammation 12, 21–10. doi:10.1186/s12974-015-0239-2
Santos-Silva, A., Charrua, A., Cruz, C. D., Gharat, L., Avelino, A., and Cruz, F. (2012). Rat detrusor overactivity induced by chronic spinalization can be abolished by a transient receptor potential vanilloid 1 (TRPV1) antagonist. Auton. Neurosci. 166 (1-2), 35–38. doi:10.1016/j.autneu.2011.09.005
Schappe, M. S., Szteyn, K., Stremska, M. E., Mendu, S. K., Downs, T. K., Seegren, P. V., et al. (2018). Chanzyme TRPM7 mediates the Ca2+ influx essential for lipopolysaccharide-induced toll-like receptor 4 endocytosis and macrophage activation. Immunity 48 (1), 59–74. doi:10.1016/j.immuni.2017.11.026
Scholz, J., Finnerup, N. B., Attal, N., Aziz, Q., Baron, R., Bennett, M. I., et al. (2019). The IASP classification of chronic pain for ICD-11: chronic neuropathic pain. Pain 160 (1), 53–59. doi:10.1097/j.pain.0000000000001365
Schwab, A., Fabian, A., Hanley, P. J., and Stock, C. (2012). Role of ion channels and transporters in cell migration. Physiol. Rev. 92, 1865–1913. doi:10.1152/physrev.00018.2011
Sela, R. A., Bruera, E., Conner-spady, B., Cumming, C., and Walker, C. (2002). Sensory and affective dimensions of advanced cancer pain. Psycho-Oncology J. Psychol. Soc. Behav. Dimensions Cancer 11 (1), 23–34. doi:10.1002/pon.551
Shi, R., Fu, Y., Zhao, D., Boczek, T., Wang, W., and Guo, F. (2021). Cell death modulation by transient receptor potential melastatin channels TRPM2 and TRPM7 and their underlying molecular mechanisms. Biochem. Pharmacol. 190, 114664. doi:10.1016/j.bcp.2021.114664
Shim, Y. J., Tae, Y. K., Kang, B. H., Park, J. S., Jeon, S. Y., and Min, B. H. (2017). Toll-like receptor 4 signaling is required for clusterin-induced tumor necrosis factor-α secretion in macrophage. Biochem. Biophys. Res. Commun. 482 (4), 1407–1412. doi:10.1016/j.bbrc.2016.12.049
Shirakawa, H., and Kaneko, S. (2018). Physiological and pathophysiological roles of transient receptor potential channels in microglia-related CNS inflammatory diseases. Biol. Pharm. Bull. 41 (8), 1152–1157. doi:10.1248/bpb.b18-00319
Simon, F., Tapia, P., Armisen, R., Echeverria, C., Gatica, S., Vallejos, A., et al. (2017). Human peritoneal mesothelial cell death induced by high-glucose hypertonic solution involves Ca2+ and Na+ ions and oxidative stress with the participation of PKC/NOX2 and PI3K/Akt pathways. Front. physiology 8, 379. doi:10.3389/fphys.2017.00379
So, K., Haraguchi, K., Asakura, K., Isami, K., Sakimoto, S., Shirakawa, H., et al. (2015). Involvement of TRPM2 in a wide range of inflammatory and neuropathic pain mouse models. J. Pharmacol. Sci. 127 (3), 237–243. doi:10.1016/j.jphs.2014.10.003
Song, K., Wang, H., Kamm, G. B., Pohle, J., Reis, F. d. C., Heppenstall, P., et al. (2016). The TRPM2 channel is a hypothalamic heat sensor that limits fever and can drive hypothermia. Science 353 (6306), 1393–1398. doi:10.1126/science.aaf7537
Song, Z., Wu, T., Sun, J., Wang, H., Hua, F., Nicolas, Y. S. M., et al. (2021). Metformin attenuates post-epidural fibrosis by inhibiting the TGF-β1/Smad3 and HMGB1/TLR4 signaling pathways. J. Cell Mol. Med. 25 (7), 3272–3283. doi:10.1111/jcmm.16398
Staurengo-Ferrari, L., Deng, L., and Chiu, I. M. (2022). Interactions between nociceptor sensory neurons and microbial pathogens in pain. Pain 163 (Suppl. 1), S57–s68. doi:10.1097/j.pain.0000000000002721
Stokes, J. A., Corr, M., and Yaksh, T. L. (2013). Spinal toll-like receptor signaling and nociceptive processing: regulatory balance between TIRAP and TRIF cascades mediated by TNF and IFNβ. PAIN® 154 (5), 733–742. doi:10.1016/j.pain.2013.01.012
Sumoza-Toledo, A., Lange, I., Cortado, H., Bhagat, H., Mori, Y., Fleig, A., et al. (2011). Dendritic cell maturation and chemotaxis is regulated by TRPM2-mediated lysosomal Ca2+ release. FASEB J. 25 (10), 3529–3542. doi:10.1096/fj.10-178483
Sumoza-Toledo, A., and Penner, R. (2011). TRPM2: a multifunctional ion channel for calcium signalling. J. physiology 589 (7), 1515–1525. doi:10.1113/jphysiol.2010.201855
Sun, M.-K., and Alkon, D. L. (2014). The “memory kinases”: roles of PKC isoforms in signal processing and memory formation. Prog. Mol. Biol. Transl. Sci. 122, 31–59. doi:10.1016/B978-0-12-420170-5.00002-7
Świeboda, P., Filip, R., Prystupa, A., and Drozd, M. (2013). Assessment of pain: types, mechanism and treatment. Pain 2 (7), 2–7.
Syed Mortadza, S. A., Wang, L., Li, D., and Jiang, L. H. (2015). TRPM2 channel-mediated ROS-sensitive Ca2+ signaling mechanisms in immune cells. Front. Immunol. 6, 407. doi:10.3389/fimmu.2015.00407
Szallasi, A. (2023). Resiniferatoxin: nature's precision medicine to silence TRPV1-positive afferents. Int. J. Mol. Sci. 24 (20), 15042. doi:10.3390/ijms242015042
Takeda, K., Kaisho, T., and Akira, S. (2003). Toll-like receptors. Annu. Rev. Immunol. 21 (1), 335–376. doi:10.1146/annurev.immunol.21.120601.141126
Tan, C.-H., and McNaughton, P. A. (2018). TRPM2 and warmth sensation. Pflügers Archiv-European J. Physiology 470 (5), 787–798. doi:10.1007/s00424-018-2139-7
Tanga, F. Y., Nutile-McMenemy, N., and DeLeo, J. A. (2005). The CNS role of Toll-like receptor 4 in innate neuroimmunity and painful neuropathy. Proc. Natl. Acad. Sci. 102 (16), 5856–5861. doi:10.1073/pnas.0501634102
Tauseef, M., Knezevic, N., Chava, K. R., Smith, M., Sukriti, S., Gianaris, N., et al. (2012). TLR4 activation of TRPC6-dependent calcium signaling mediates endotoxin-induced lung vascular permeability and inflammation. J. Exp. Med. 209 (11), 1953–1968. doi:10.1084/jem.20111355
Tegtmeyer, N., Neddermann, M., Lind, J., Pachathundikandi, S. K., Sharafutdinov, I., Gutiérrez-Escobar, A. J., et al. (2020). Toll-like receptor 5 activation by the CagY repeat domains of Helicobacter pylori. Cell Rep. 32 (11), 108159. doi:10.1016/j.celrep.2020.108159
Teixeira-Santos, L., Albino-Teixeira, A., and Pinho, D. (2020). Neuroinflammation, oxidative stress and their interplay in neuropathic pain: focus on specialized pro-resolving mediators and NADPH oxidase inhibitors as potential therapeutic strategies. Pharmacol. Res. 162, 105280. doi:10.1016/j.phrs.2020.105280
Togashi, K., Hara, Y., Tominaga, T., Higashi, T., Konishi, Y., Mori, Y., et al. (2006). TRPM2 activation by cyclic ADP-ribose at body temperature is involved in insulin secretion. EMBO J. 25 (9), 1804–1815. doi:10.1038/sj.emboj.7601083
Togashi, K., Inada, H., and Tominaga, M. (2008). Inhibition of the transient receptor potential cation channel TRPM2 by 2-aminoethoxydiphenyl borate (2-APB). Br. J. Pharmacol. 153 (6), 1324–1330. doi:10.1038/sj.bjp.0707675
Tsagareli, M. G., and Nozadze, I. (2020). An overview on transient receptor potential channels superfamily. Behav. Pharmacol. 31 (5), 413–434. doi:10.1097/FBP.0000000000000524
Tsutsui, M., Hirase, R., Miyamura, S., Nagayasu, K., Nakagawa, T., Mori, Y., et al. (2018). TRPM2 exacerbates central nervous system inflammation in experimental autoimmune encephalomyelitis by increasing production of CXCL2 chemokines. J. Neurosci. 38 (39), 8484–8495. doi:10.1523/JNEUROSCI.2203-17.2018
Turlova, E., Feng, Z.-p., and Sun, H.-s. (2018). The role of TRPM2 channels in neurons, glial cells and the blood-brain barrier in cerebral ischemia and hypoxia. Acta Pharmacol. Sin. 39 (5), 713–721. doi:10.1038/aps.2017.194
Vandewauw, I., De Clercq, K., Mulier, M., Held, K., Pinto, S., Van Ranst, N., et al. (2018). A TRP channel trio mediates acute noxious heat sensing. Nature 555 (7698), 662–666. doi:10.1038/nature26137
Vaure, C., and Liu, Y. (2014). A comparative review of toll-like receptor 4 expression and functionality in different animal species. Front. Immunol. 5, 316. doi:10.3389/fimmu.2014.00316
Vermot, A., Petit-Härtlein, I., Smith, S. M. E., and Fieschi, F. (2021). NADPH oxidases (NOX): an overview from discovery, molecular mechanisms to physiology and pathology. Antioxidants (Basel) 10 (6), 890. doi:10.3390/antiox10060890
Vicario, N., Turnaturi, R., Spitale, F. M., Torrisi, F., Zappalà, A., Gulino, R., et al. (2020). Intercellular communication and ion channels in neuropathic pain chronicization. Inflamm. Res. 69, 841–850. doi:10.1007/s00011-020-01363-9
Vilar, B., Tan, C.-H., and McNaughton, P. A. (2020). Heat detection by the TRPM2 ion channel. Nature 584 (7820), E5–E12. doi:10.1038/s41586-020-2510-7
Vriens, J., Owsianik, G., Hofmann, T., Philipp, S. E., Stab, J., Chen, X., et al. (2011). TRPM3 is a nociceptor channel involved in the detection of noxious heat. Neuron 70 (3), 482–494. doi:10.1016/j.neuron.2011.02.051
Wang, H., Song, T., Wang, W., and Zhang, Z. (2019b). TRPM2 participates the transformation of acute pain to chronic pain during injury-induced neuropathic pain. Synapse 73 (10), e22117. doi:10.1002/syn.22117
Wang, Q., Guo, W., Hao, B., Shi, X., Lu, Y., Wong, C. W. M., et al. (2016). Mechanistic study of TRPM2-Ca2+-CAMK2-BECN1 signaling in oxidative stress-induced autophagy inhibition. Autophagy 12 (8), 1340–1354. doi:10.1080/15548627.2016.1187365
Wang, Y., Chen, L., Wang, K., Da, Y., Zhou, M., Yan, H., et al. (2019a). Suppression of TRPM2 reduces renal fibrosis and inflammation through blocking TGF-β1-regulated JNK activation. Biomed. and Pharmacother. 120, 109556. doi:10.1016/j.biopha.2019.109556
Wehrhahn, J., Kraft, R., Harteneck, C., and Hauschildt, S. (2010). Transient receptor potential melastatin 2 is required for lipopolysaccharide-induced cytokine production in human monocytes. J. Immunol. 184 (5), 2386–2393. doi:10.4049/jimmunol.0902474
Woller, S. A., Eddinger, K. A., Corr, M., and Yaksh, T. L. (2017). An overview of pathways encoding nociception. Clin. Exp. rheumatology 35 (Suppl. 107), 40–46.
Wu, F.-x., Bian, J. j., Miao, X. r., Huang, S. d., Xu, X. w., Gong, D. j., et al. (2010). Intrathecal siRNA against Toll-like receptor 4 reduces nociception in a rat model of neuropathic pain. Int. J. Med. Sci. 7 (5), 251–259. doi:10.7150/ijms.7.251
Yamamoto, S., and Shimizu, S. (2016). Targeting TRPM2 in ROS-coupled diseases. Pharmaceuticals 9 (3), 57. doi:10.3390/ph9030057
Yang, K., Chang, W. L., Yang, P. C., Chien, C. L., Lai, M. S., Su, M. J., et al. (2006). Activation of the transient receptor potential M2 channel and poly (ADP-ribose) polymerase is involved in oxidative stress-induced cardiomyocyte death. Cell Death and Differ. 13 (10), 1815–1826. doi:10.1038/sj.cdd.4401813
Yang, Y., Yao, Z., Wang, H., Jia, S., Wang, M., Wang, S., et al. (2024). Severe inflammation in C57/BL6 mice leads to prolonged cognitive impairment by initiating the IL-1β/TRPM2 pathway. Int. Immunopharmacol. 128, 111380. doi:10.1016/j.intimp.2023.111380
Ye, M., Yang, W., Ainscough, J. F., Hu, X. P., Li, X., Sedo, A., et al. (2014). TRPM2 channel deficiency prevents delayed cytosolic Zn2+ accumulation and CA1 pyramidal neuronal death after transient global ischemia. Cell death and Dis. 5 (11), e1541. doi:10.1038/cddis.2014.494
Yıldızhan, K., and Nazıroğlu, M. (2020). Glutathione depletion and parkinsonian neurotoxin MPP+-induced TRPM2 channel activation play central roles in oxidative cytotoxicity and inflammation in microglia. Mol. Neurobiol. 57 (8), 3508–3525. doi:10.1007/s12035-020-01974-7
Yu, B., Jin, L., Yao, X., Zhang, Y., Zhang, G., Wang, F., et al. (2023). TRPM2 protects against cisplatin-induced acute kidney injury and mitochondrial dysfunction via modulating autophagy. Theranostics 13 (13), 4356–4375. doi:10.7150/thno.84655
Yüksel, E., Nazıroğlu, M., Şahin, M., and Çiğ, B. (2017). Involvement of TRPM2 and TRPV1 channels on hyperalgesia, apoptosis and oxidative stress in rat fibromyalgia model: protective role of selenium. Sci. Rep. 7 (1), 17543. doi:10.1038/s41598-017-17715-1
Zhang, C., Huang, Y., Ouyang, F., Su, M., Li, W., Chen, J., et al. (2022). Extracellular vesicles derived from mesenchymal stem cells alleviate neuroinflammation and mechanical allodynia in interstitial cystitis rats by inhibiting NLRP3 inflammasome activation. J. Neuroinflammation 19 (1), 80. doi:10.1186/s12974-022-02445-7
Zhang, D., Li, X., Jing, B., Chen, Z., Shi, H., Zheng, Y., et al. (2023). α-Asarone attenuates chronic sciatica by inhibiting peripheral sensitization and promoting neural repair. Phytother. Res. 37 (1), 151–162. doi:10.1002/ptr.7603
Zhang, X., Hu, M., Yang, Y., and Xu, H. (2018). Organellar TRP channels. Nat. Struct. and Mol. Biol. 25 (11), 1009–1018. doi:10.1038/s41594-018-0148-z
Zheng, Y., Zhao, J., Chang, S., Zhuang, Z., Waimei, S., Li, X., et al. (2023). β-Sitosterol alleviates neuropathic pain by affect microglia polarization through inhibiting TLR4/NF-κB signaling pathway. J. Neuroimmune Pharmacol. 18 (4), 690–703. doi:10.1007/s11481-023-10091-w
Zhong, C., Yang, J., Zhang, Y., Fan, X., Fan, Y., Hua, N., et al. (2023). TRPM2 mediates hepatic ischemia–reperfusion injury via Ca2+-induced mitochondrial lipid peroxidation through increasing ALOX12 expression. Research 6, 0159. doi:10.34133/research.0159
Zhong, Z., Zhai, Y., Liang, S., Mori, Y., Han, R., Sutterwala, F. S., et al. (2013). TRPM2 links oxidative stress to NLRP3 inflammasome activation. Nat. Commun. 4 (1), 1611. doi:10.1038/ncomms2608
Zhou, Y. H., Liao, S. J., Li, D., Luo, J., Wei, J. J., Yan, B., et al. (2013). TLR4 ligand/H₂O₂ enhances TGF-β1 signaling to induce metastatic potential of non-invasive breast cancer cells by activating non-Smad pathways. PLoS One 8 (5), e65906. doi:10.1371/journal.pone.0065906
Zhu, T., Zhao, Y., Hu, H., Zheng, Q., Luo, X., Ling, Y., et al. (2019). TRPM2 channel regulates cytokines production in astrocytes and aggravates brain disorder during lipopolysaccharide-induced endotoxin sepsis. Int. Immunopharmacol. 75, 105836. doi:10.1016/j.intimp.2019.105836
Zong, P., Feng, J., Yue, Z., Yu, A. S., Vacher, J., Jellison, E. R., et al. (2022). TRPM2 deficiency in mice protects against atherosclerosis by inhibiting TRPM2–CD36 inflammatory axis in macrophages. Nat. Cardiovasc. Res. 1 (4), 344–360. doi:10.1038/s44161-022-00027-7
Zou, J., Ainscough, J. F., Yang, W., Sedo, A., Yu, S. P., Mei, Z. Z., et al. (2013). A differential role of macrophage TRPM2 channels in Ca²⁺ signaling and cell death in early responses to H₂O₂. Am. J. Physiology-Cell Physiology 305 (1), C61–C69. doi:10.1152/ajpcell.00390.2012
Keywords: inflammation, neuropathic pain, lipopolysaccharide reactive oxygen species (ROS), nicotinamide adenine dinucleotide phosphate (NADPH) oxidase (NOX), TRPM2 and TLR4
Citation: Mandlem VKK, Rivera A, Khan Z, Quazi SH and Deba F (2024) TLR4 induced TRPM2 mediated neuropathic pain. Front. Pharmacol. 15:1472771. doi: 10.3389/fphar.2024.1472771
Received: 30 July 2024; Accepted: 02 September 2024;
Published: 12 September 2024.
Edited by:
Sara Marchesan Oliveira, Federal University of Santa Maria, BrazilReviewed by:
Cassia Regina Silva, Federal University of Uberlandia, BrazilCopyright © 2024 Mandlem, Rivera, Khan, Quazi and Deba. This is an open-access article distributed under the terms of the Creative Commons Attribution License (CC BY). The use, distribution or reproduction in other forums is permitted, provided the original author(s) and the copyright owner(s) are credited and that the original publication in this journal is cited, in accordance with accepted academic practice. No use, distribution or reproduction is permitted which does not comply with these terms.
*Correspondence: Farah Deba, ZmRlYmFAdXR0eWxlci5lZHU=
Disclaimer: All claims expressed in this article are solely those of the authors and do not necessarily represent those of their affiliated organizations, or those of the publisher, the editors and the reviewers. Any product that may be evaluated in this article or claim that may be made by its manufacturer is not guaranteed or endorsed by the publisher.
Research integrity at Frontiers
Learn more about the work of our research integrity team to safeguard the quality of each article we publish.