- 1Department of Breast and Thyroid Surgery, First Affiliated Hospital of Huzhou University, Huzhou, China
- 2Department of Cardiovascular Center, First Affiliated Hospital of Huzhou University, Huzhou, China
- 3School of Medicine, Huzhou University, Huzhou, China
The emergence of targeted anti-tumor drugs has significantly prolonged the lifespan and improved the prognosis of cancer patients. Among these drugs, vascular endothelial growth factor (VEGF) inhibitors, particularly novel small molecule tyrosine kinase inhibitors (TKIs), are extensively employed as VEGF inhibitors; however, they are also associated with a higher incidence of complications, with hypertension being the most prevalent cardiovascular toxic side effect. Currently, it is widely accepted that TKIs-induced hypertension involves multiple mechanisms including dysregulation of the endothelin (ET) axis, reduced bioavailability of nitric oxide (NO), imbalance in NO-ROS equilibrium system, vascular rarefaction, and activation of epithelial sodium calcium channels; nevertheless, excessive activation of ET system appears to be predominantly responsible for this condition. Moreover, studies have demonstrated that ET plays a pivotal role in driving TKIs-induced hypertension. Therefore, this review aims to explore the significance of ET in the pathogenesis of hypertension induced by targeted anti-tumor drugs and investigate the potential therapeutic value of endothelin antagonists in managing hypertension caused by targeted anti-tumor drugs.
1 The current status and research significance of hypertension caused by targeted anti-cancer drugs
With the advancement of targeted therapy for tumors, the survival period of tumor patients has been continuously extended, and cardiovascular events have gradually emerged as one of the significant causes of mortality in tumor patients. The primary anti-tumor drugs encompass vascular endothelial growth factor (VEGF) inhibitors (Shaw et al., 2024; Tavakolian et al., 2024). Particularly small molecule tyrosine kinase inhibitors (TKIs) that effectively inhibit signal cascades (Liu et al., 2023a). By targeting vascular endothelial growth factor receptor (VEGFR) to impede tumor angiogenesis, they have become a predominant treatment modality for numerous solid malignant tumors (Ciccarese et al., 2021; Du et al., 2022). Nevertheless, approximately one-fourth of patients using VEGF inhibitors will experience hypertension, while nearly every patient will exhibit significantly elevated blood pressure levels (Narayan et al., 2023; Pannucci et al., 2023). Cardiovascular toxic side effects including hypertension not only impact patient prognosis but also influence the efficacy of anti-tumor drugs. Therefore, the objective of anti-tumor treatment is to maximize its therapeutic effect while minimizing treatment-related cardiovascular events. It is imperative to explore novel specific therapeutic agents to facilitate seamless application of anti-tumor treatments and prevent associated cardiovascular events while improving patient prognosis.
2 The primary mechanism of TKIs in anti-tumor therapy
The vascular endothelial growth factor (VEGF) is the most potent factor in inducing vascular permeability and a specific mitogen for endothelial cells, playing a crucial role in promoting proliferation, migration, and angiogenesis of these cells (Liu et al., 2023b). VEGF exerts its biological effects through three main receptor subtypes: VEGFR 1–3. Among them, VEGFR2 serves as the primary mediator of VEGF’s actions and is closely associated with cell chemotaxis, division, and recombination (Sun et al., 2014). It plays a pivotal role in stimulating endothelial cell proliferation and migration while also regulating vascular permeability. VEGF signaling pathway inhibitors encompass monoclonal antibodies targeting VEGFA factor, VEGF trap, monoclonal antibodies against VEGF receptors, and TKIs. Among these options, TKIs competitively bind to the ATP site of tyrosine kinases, thereby impeding phosphorylation levels of tyrosine kinases. Consequently, tumor cell DNA repair is inhibited, G1 phase cell division is blocked, and angiogenesis suppression is achieved to exert anti-tumor effects. As a result of their efficacy in cancer treatment, TKIs have emerged as extensively utilized anticancer drugs with favorable therapeutic outcomes. However, it should be noted that cardiovascular toxic side effects such as hypertension are commonly associated with their use.
The classification of TKIs can be based on their main target of action, which includes inhibitors targeting epidermal growth factor receptor (EGFR), anaplastic lymphoma kinase (ALK), human epidermal growth factor receptor 2 (HER-2), VEGFR, Abelson murine leukemia viral oncogene (ABL), and breakpoint cluster region-fusion gene (BCR-ABL) (Réa and Hughes, 2022; Le et al., 2021). Among them, certain TKIs specifically target a single receptor, such as the widely used EGFR-TKIs osimertinib and erlotinib in lung cancer (Soria et al., 2018; Ramalingam et al., 2020). Apatinib is an innovative TKI that effectively inhibits VEGFR-2 among various tumor-related kinases (TRKs) and induces apoptosis of VEGFR-2, thereby effectively suppressing the proliferation of multiple tumor cells (Xie et al., 2021; Zhao et al., 2023). Currently, apatinib has demonstrated efficacy and safety in gastric cancer, lung cancer, and breast cancer; moreover, the combination therapy of apatinib with camrelizumab has been recommended as first-line treatment for advanced liver cancer (Xia et al., 2022; Mao et al., 2023). Meanwhile, some TKIs exhibit multi-target activity. The kinase inhibitor sunetinib specifically targets VEGFR, platelet-derived growth factor receptor (PDGFR), PDGFR-a, PDGFR-b, and other receptors (Wang et al., 2023). It is indicated for use in patients with gastrointestinal stromal tumors (GIST) who have experienced treatment failure or intolerance to imatinib therapy, as well as in patients with advanced renal cell carcinoma (RCC) who have shown no response to cytokine therapy (Jin et al., 2023; Plimack et al., 2023). The multi-targeted kinase inhibitor Anlotinib demonstrates simultaneous inhibition of VEGFR, PDGFR, and fibroblast growth factor receptor (FGFR), making it a viable third-line treatment option for patients with advanced non-small cell lung cancer (Shen et al., 2018; Lei et al., 2023). Additionally, this drug has gained approval for its efficacy in treating soft tissue sarcoma, small cell lung cancer, medullary thyroid carcinoma, Metastatic Cervical Cancer and differentiated thyroid carcinoma (Lv et al., 2022; Li, 2021; Wu et al., 2023; Xu et al., 2022). The first anti-tumor drug, sorafenib, exerts dual inhibition on Raf protein kinase (RAF) and VEGFR kinases. By suppressing the activity of VGFR-2, VGFR-3, and RAF-1, it effectively hampers tumor cell proliferation through direct blockade of the RAF/MEK/ERK-mediated signaling pathway (Wilhelm et al., 2008; Gentile et al., 2016; Kim et al., 2018). Moreover, its impact on VEGFR and PDGFR enables angiogenesis inhibition and disruption of nutrient supply to restrict tumor cell growth (Mangana et al., 2012; Fallahi et al., 2022). Sorafenib is widely recognized as a standard first-line treatment for advanced renal cell carcinoma (Hsieh et al., 2017; Sun et al., 2022).
2.1 Possible mechanisms of ET-1 in TKIs-induced hypertension
ET-1 exerts its influence on blood pressure through multiple mechanisms, rendering it an appealing therapeutic target for hypertension and other related conditions. Moreover, ET-1 plays a pivotal role in the pathogenesis of antineoplastic drug-induced hypertension, as well as being a crucial pathway involved in VEGF inhibitor-induced hypertension and renal damage (Facemire et al., 2009; Kappers et al., 2012). Studies have demonstrated that patients and animals treated with VEGF inhibitors exhibit a two-to three-fold increase in plasma levels of cleared receptors (Kappers et al., 2010). Consistent with the dose-dependent nature of elevated blood pressure, the increase in circulating ET-1 exhibited a corresponding dose-dependence during VEGF inhibition (Lankhorst et al., 2015). The mechanism by which VEGF deactivation leads to an elevation in ET-1 remains unclear. One hypothesis suggests that VEGF inactivation results in the loss of vasodilatory endothelial ETB receptors, thereby reducing ET-1 clearance and increasing its circulation (Versmissen et al., 2019). Additionally, dual ETA/B receptor antagonism or selective ETA receptor blockade has shown to prevent VEGF-inhibitor-induced hypertension, indicating that stimulation of ETA receptors by ET-1 is responsible for this condition (Carneiro et al., 2008). The utilization of sunitinib in a clinical pilot study resulted in an elevation of circulating levels of ET-1 in vivo (Sourdon et al., 2017). Indicating the crucial role played by endothelin in hypertension induced by antineoplastic drug therapy that should not be disregarded. (Figure 1).
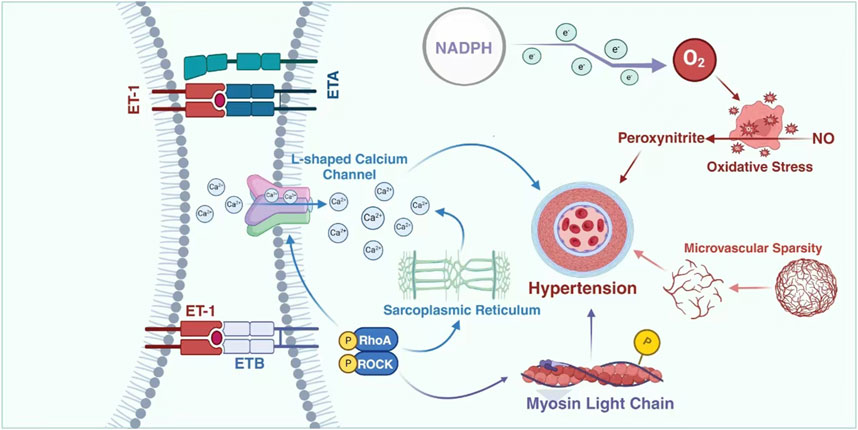
Figure 1. Possible mechanisms of ET-1 in TKIs-induced hypertension. In the hypertension conditions induced by TKIs, microvascular damage is highly likely to be one of the most crucial and leading factors. The substance ET can inflict damage on endothelial cells via L-type calcium channels, further intensifying this condition. This will lead to a marked reduction in the density of the microvascular network, making it increasingly rarefied. Such changes in the density and structure of the microvascular network gradually break the originally normal physiological balance and interfere with the normal functions of blood vessels, thereby gradually triggering the occurrence of hypertension. ETA: endothelin A; ETB: endothelin B; ET1: endothelin 1; ET2: endothelin 2; ET3: endothelin 3; NO: endothelial nitric; NAPDH: Nicotinamide Adenine Dinucleotide Phosphate (reduced form).
3 The mechanisms of TKI-induced hypertension
VEGF inhibitors can increase the risk of heart failure, coronary artery disease, hypertension, and thromboembolic diseases through mechanisms such as endothelial injury, vasoconstriction and remodeling, inflammatory response, and platelet activation (Porta and Striglia, 2020; Chen et al., 2018). The potential mechanisms underlying the induction of hypertension by this class of drugs are currently believed to include (Wang et al., 2021; Wang et al., 2022a; Simonetti et al., 2009; Pérez-Gutiérrez and Ferrara, 2023; Wang et al., 2024): (i) Inhibiting nitric oxide synthase (NOS) reduces the synthesis of NO, thereby blocking its vasodilatory effects; (ii) Increased production of endothelin (ET) enhances vasoconstriction; (iii) Endothelial cell apoptosis and necrosis lead to a decrease in capillary bed density (rarefaction); (iv) Impaired renal function, increased salt sensitivity, and water-sodium retention. Among these mechanisms, the relationship between endothelin and hypertension is worth further investigation due to the varying degrees of interaction between them (Figure 2).
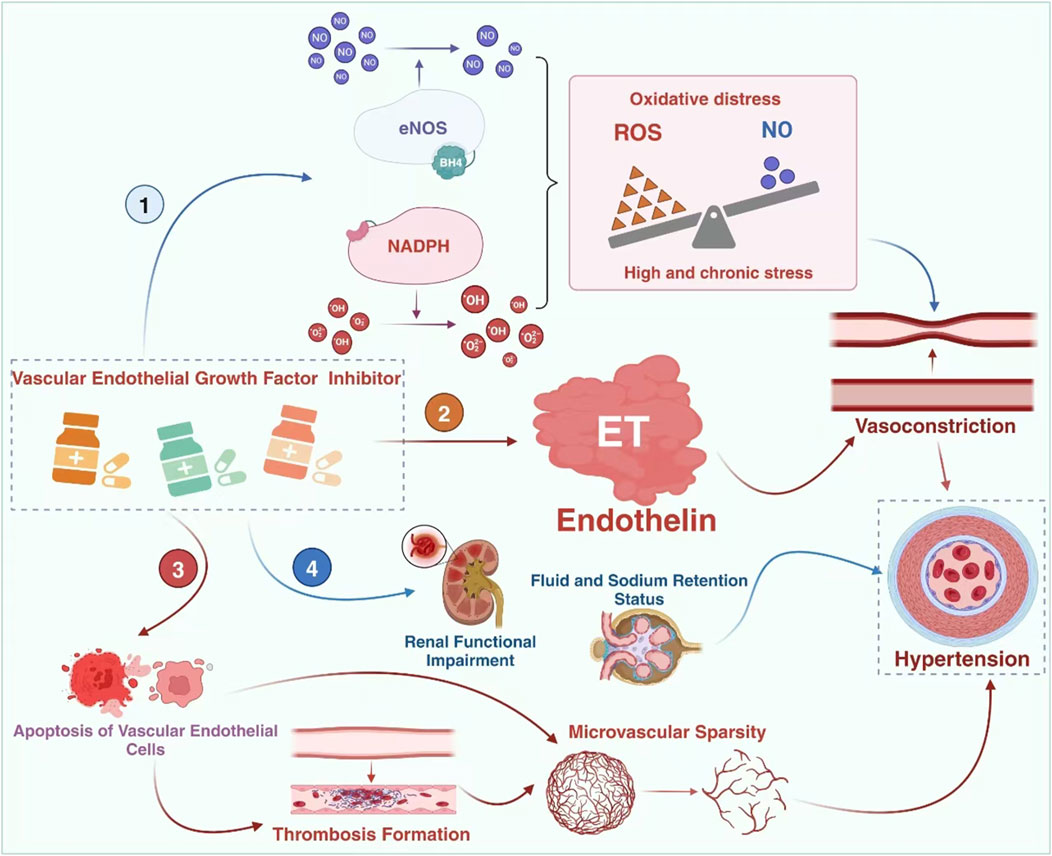
Figure 2. The mechanisms of TKI-induced hypertension. The mechanism of hypertension caused by vascular endothelial growth factor inhibitors may involve 4 pathways, namely the imbalance of the NO-ROS system, the impairment of the endothelin system, microvascular injury and the occurrence of renal damage. However, ET-1 might be the central link. eNOS: endothelial nitric oxide synthase; BH4: tetrahydrobiopterin.
4 The upregulation of ET-1 may contribute to the development of hypertension induced by TKIs
ET-1, NO, and vascular pseudohaemophilic factor (VWF) are bioactive substances that reflect the function of vascular endothelium and play a crucial role in the occurrence and progression of cardiovascular disease and essential hypertension (Taneja et al., 2019; Yu et al., 2020). NO is a pivotal factor in endothelial nitric oxide synthase (eNOS)-mediated physiological changes in smooth muscle relaxation, which is critical for angiogenesis (Chen et al., 2024). Normal endothelial cells constitutively express eNOS; however, under pathological conditions, its inhibition leads to reduced bioavailability of NO that inhibits eNOS expression (Leo et al., 2021). Meta-analyses have demonstrated that VEGF inhibitors can increase ET-1 levels while decreasing NO expression, ultimately leading to severe cardiovascular complications such as hypertension (Xu et al., 2021).
4.1 Relationship between ET-1 and vasodilation and contraction
ET was initially identified as a potent vasoconstrictive peptide for porcine aortic endothelial cells (ECs) (Ma et al., 2023). It consists of 21 amino acid residues with a hydrophobic C-terminus connected by two sets of intrastrand disulphide bonds, exhibiting vasopressor effects (Laudette et al., 2021). Three isomers of human ET exist, namely ET-1, ET-2, and ET-3 (Davenport and Maguire, 2006). Although they differ in structure and activity, all three are antihypertensive in vivo and exhibit strong contractile effects on vascular smooth muscle cells in vitro. Among these isomers, ET-1 plays a major role in regulating the cardiovascular system and vascular endothelial cells serve as the primary source of this isopeptide (Harrison et al., 2024; Finch and Conklin, 2016). In addition to ECs, various cell types including vascular smooth muscle cells (VSMCs), cardiomyocytes, fibroblasts, macrophages, epithelial cells of the lungs and kidneys as well as neurons and glial cells express ET-1 (Chen et al., 2020; Davenport et al., 2016). The degree of vasoconstrictive activity induced by these peptides follows the order: ET-2 > ET-1 > ET-3 (Inoue et al., 1989). However, ET-1 is currently recognized as the most potent vasoconstrictor known to induce constriction or relaxation in the vasculature by binding to endothelin A (ETA)/endothelin B (ETB) receptors on smooth muscle cells (SMCs) (Maguire and Davenport, 2015; Kumar et al., 2013). The interaction between ET-1 and ETA receptors on vascular smooth muscle cells leads to an increase in intracellular calcium levels, while the interaction between ETA receptors further elevates intracellular calcium levels (Ortega Mateo and de Artiñano, 1997; Hopfner et al., 1998). This rise in intracellular calcium triggers phosphorylation and activation of myosin light chains, ultimately resulting in vasoconstriction. Similarly, binding of ET-1 to ETB receptors on endothelial cells activates both eNOS and prostaglandin pathways, leading to vasodilation of the vasculature (Wolf et al., 2014; Leite et al., 2013; Neves et al., 2019) (Figure 3).
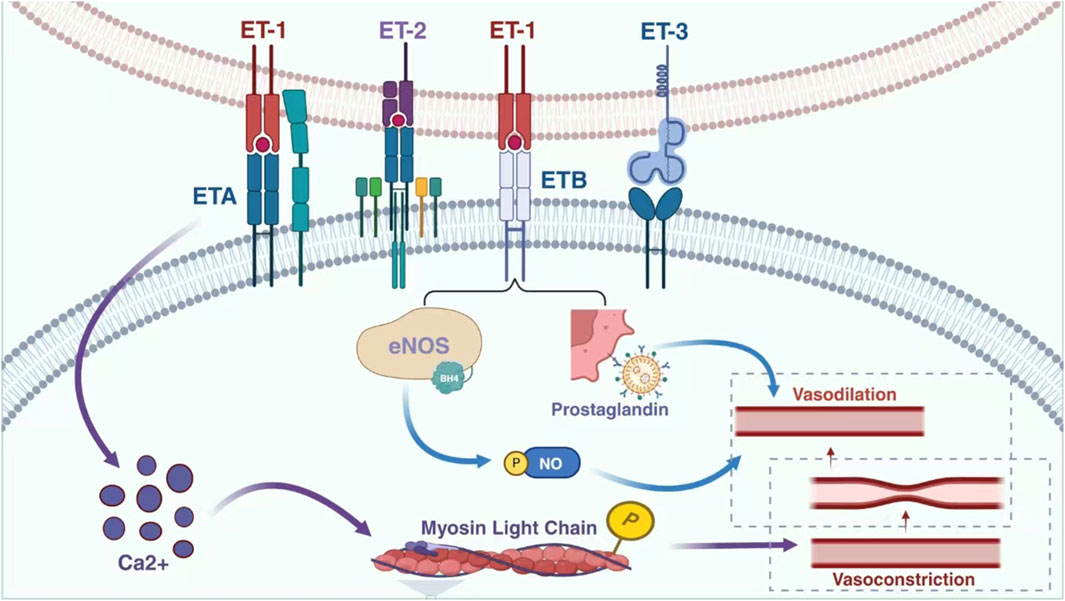
Figure 3. Relationship between ET-1 and vasodilation and contraction. Endothelin, a significant biomolecule, encompasses endothelin receptor A and endothelin receptor B. These receptors respectively combine with three distinct subtypes of endothelin, namely endothelin 1, endothelin 2, and endothelin 3. Through this specific interaction and combination, a physiological process is initiated that ultimately leads to vasoconstriction. This mechanism plays a crucial role in regulating vascular tone and blood flow within the complex biological systems of the body. eNOS: endothelial nitric oxide synthase; NO: endothelial nitric; ETA: endothelin A; ETB: endothelin B; ET1: endothelin 1; ET2: endothelin 2; ET3: endothelin 3.
4.2 Key factors involved in the ET-1 induced hypertension
4.2.1 NO-ROS imbalance
The exploration of the mechanism underlying hypertension induced by antineoplastic drugs has suggested a correlation with ROS oxidative stress triggered by ET-1, which enhances NADPH oxidase activity and consequently elevates ROS production. This, in turn, may contribute to hypertension by further diminishing available NO, thereby challenging the disassociation of ET-1from the NO-ROS equilibrium (Camarda et al., 2022; Wilcox et al., 2019; Muhammad et al., 2020).
Oxidative stress resulting from excessive production of ROS is a crucial mechanism underlying endothelial cell death (Zheng et al., 2022; Fan et al., 2024; Lin et al., 2022). ROS, such as superoxide and hydrogen peroxide, have been identified as molecules involved in angiogenesis. While excessive ROS levels induce senescence and apoptosis in endothelial cells and stem/progenitor cells, low concentrations of ROS play a pivotal role in redox signaling pathways that mediate angiogenesis (Ushio-Fukai and Alexander, 2004; Yingze et al., 2022). During antiangiogenic therapy, oxidative stress may contribute to the development of hypertension by oxidizing NO to peroxynitrite, thereby reducing NO-mediated vasodilator tone (Sedeek et al., 2003). The literature has extensively investigated whether the elevation of MAP induced by sunitinib is accompanied by an increase in oxidative stress markers and if antioxidants can prevent or reverse this potential rise in MAP (Kappers et al., 2011; Lankhorst et al., 2014). Through studying the role of oxidative stress in hypertension, it has been determined that upregulation of ROS production is more likely to be a consequence of VEGF inhibitor-induced hypertension (Versmissen et al., 2019). The reduced availability of NO plays a crucial role in the development of hypertension induced by VEGF inhibitors, while it has been demonstrated that ET-1 enhances NADPH oxidase activity, leading to increased production of ROS and potentially contributing to hypertension by further diminishing the levels of available NO (Kappers et al., 2011; Sarkar et al., 2019). ETA receptors are implicated in blood pressure regulation as well as chemotaxis, release of inflammatory mediators, generation of ROS, and neointimal formation associated with vascular remodeling processes (Eid, 2024; Sobrano Fais et al., 2023).
4.2.2 Microvascular damage
Microvessel thinning (reduced microvessel density), leading to impaired microcirculation and increased vascular resistance. This is one of the mechanisms of VEGF inhibitor-induced hypertension (Touyz and Herrmann, 2018). Microvessel thinning was initially speculated to be possibly functional, and with intense vasoconstriction, and later structural, then the relationship between endothelin and microvessel thinning had to be revisited. There are experimental and clinical data suggesting that ET-1 is responsible for maintaining arterial stiffness (Amiri et al., 2004). Increased ET-1 activity may lead to atherosclerosis and atherosclerotic arterial stiffness (Sedeek et al., 2003; Kostov, 2021; Gu et al., 2015). These pathological processes significantly reduce the elastic properties of central conduit arteries, leading to the manifestation of isolated systolic HTN. Increases in systolic and central pulse pressure may lead to eutrophication or hypertrophic remodelling of small arteries. In particular, hypertrophic remodelling of resistant arteries is a hallmark of ET-1 involvement in the hypertensive process.
VEGF inhibition resulted in a decrease in the number of perfused capillary networks, leading to an elevation in total peripheral resistance (TPR) and blood pressure. Capillary atrophy is attributed to endothelial cell apoptosis and chronic remodeling of the microvasculature observed during VEGF occlusion (Iwagawa et al., 2023). In one study, sunitinib-treated patients were examined for capillary density using microscopy (Gu et al., 2015). The researchers discovered that a reduction in microvascular network density during tki treatment was correlated with an increase in blood pressure. The reduced density of microvessels also results in obstructed microcirculation and increased vascular resistance. In a study involving patients with colorectal cancer, bevacizumab was administered and after 6 months of treatment, the patients’ blood vessels were evaluated. Moderate vasodilution was observed, suggesting that vasodilution may be one of the mechanisms by which VEGFI induces high blood pressure (Mourad et al., 2008). Furthermore, it is likely that vascular thinning is functional rather than structural (Chuang et al., 2018). Additionally, endothelial cell dysfunction may contribute to thrombosis, leading to further reduction in vascular perfusion, increased apoptosis, and loss of microvasculature.
4.2.3 Activation of related signalling pathways
Previously, our team’s study has demonstrated that apatinib can induce hypertension in WKY rat models through the activation of the RhoA/ROCK pathway, specifically via Rho protein kinase (ROCK) (Li et al., 2022). Furthermore, we simulated the tumor microenvironment for in vivo experiments and discovered that the RhoA/ROCK signaling pathway is also implicated in apatinib-induced hypertension and vascular remodeling mechanisms in mice with gastric cancer (Wang et al., 2022b; Wang et al., 2022c).
In recent years, it has been proposed that ET-1 acts as an upstream effector, stimulating the phosphorylation of myosin light chain (MLC) in vascular smooth muscle through the RhoA/ROCK pathway (Hwang et al., 2024). Simultaneously, it enhances oxidative stress in vascular endothelial cells via this pathway, leading to increased peroxide production and subsequent reduction in NO production (Choraghe et al., 2020). These effects ultimately contribute to vasoconstriction and the development of hypertension. Consequently, high blood pressure ensues. Prolonged (3 months) endothelium-specific overexpression of ET-1 not only results in persistent elevation of blood pressure, but also induces endothelial and renal damage (Coelho et al., 2018; Zou et al., 2019). Furthermore, the vasodilatory effects of NO are mediated through the RhoA/ROCK pathway and NO can exert its vasodilatory effect via the RhoA/ROCK pathway (Kai et al., 2019). Moreover, several studies have confirmed that the RhoA/ROCK pathway plays a crucial role in the development of salt-sensitive hypertension and hypertension-induced cardiac hypertrophy (Cao et al., 2016; Kobayashi et al., 2009). The vasoconstrictive effect of ET-1 can be potentiated by the activation of the RhoA/ROCK pathway, which is implicated in reducing vasodilator function by enhancing oxidative stress, promoting peroxide production, and diminishing endothelial NO production (Zhuang et al., 2018; Tsai et al., 2017). Notably, ET-1 plays a pivotal role in mediating TKIs-induced hypertension and serves as a key driver for this condition.
4.2.4 Regulation of calcium ions
The study demonstrated that ET-1-mediated vasoconstriction is associated with calcium ions, and experimental evidence also confirmed the coupling of receptor-specific calcium signaling cascades to endothelin ETA and ETB receptors in drug-resistant arteries (Abdel-Samad et al., 2016). Endothelin ETA primarily mediates ET-1-induced vasoconstriction, with a minor contribution from VSM endothelin ETB receptors. This process involves mobilization of Ca2+ from intracellular stores, activation of nonselective cationic TRPC3 channels, entry of extracellular Ca2+ through dihydropyridine-sensitive L-type channels, and mechanisms enhancing Ca2+ sensitivity (Peppiatt-Wildman et al., 2007; Adebiyi et al., 2012). Additionally, protein kinase C (pKC) plays a crucial role in regulating Ca2+ handling by augmenting voltage-dependent Ca2+ influx (Ziemba and Falke, 2018). Furthermore, regulation of Ca2+ handling serves a dual purpose through a feedback loop that inhibits release of intracellular SR stores’ stored Ca2+. ROCK not only regulates intracellular Ca2+ mobilization and entry but also acts as a major determinant for increased myofilament sensitivity to vasoconstriction induced by activation of ET receptors in drug-resistant arteries (Rattan, 2017; Zhao et al., 2021). Enhanced ROCK activity contributes to increased Ca2+ sensitization, vasoconstriction, and vascular remodeling in hypertension. Additionally, PKC-mediated increases in L-type Ca2+ entry are associated with abnormal vasoconstriction in insulin-resistant states (Zhang et al., 2003). Therefore, these kinases represent potential pharmacological targets for vascular diseases that involve impaired ET pathways.
5 Traditional antihypertensive treatment regimens
The position paper on tumor treatment and its impact on cancer and cardiovascular toxicity suggests that medication recommendations for high blood pressure can enhance the prognosis of patients in the long term, particularly through the use of angiotensin converting enzyme inhibitors (ACEIs), angiotensin receptor blockers (ARBs), β-receptor blockers (Zamorano et al., 2016). Similarly, both cardio-oncology guidelines in the United States and Europe continue to advocate ACEIs/ARBs as the primary choice for hypertension management (Alexandre et al., 2020). However, non-dihydropyridine calcium channel blockers (CCB) are generally not recommended due to their potential interaction with VEGF-targeted therapy. Diuretics are used carefully, because diarrhea or using diuretics cause electrolyte can increase the risk of QT extension. If cause liver toxicity, VEGF inhibitors should be careful to use the CCB. When TKIs cause bradycardia should be careful to use beta-blockers drugs. The different classes of antihypertensive medications commonly interact with antitumor drugs see in Table 1.
6 Potential therapeutic targets of TKIs for enhancing blood pressure through modulation of the endothelin system
The pathophysiological effects of ET-1 are primarily mediated through ETA isoforms. In preclinical and acute experimental studies, highly selective peptide antagonists targeting ETA (including BQ123 and TAK-044) as well as ETB (BQ788), along with three nonpeptide antagonists (bosentan, macitentan, and ambrisentan), which either exhibit hybrid ETA/ETB antagonist properties or demonstrate ETA selectivity, have been clinically approved for use primarily in the treatment of pulmonary hypertension (Maguire and Davenport, 2015; Bonvallet et al., 1993; Watanabe et al., 1995; Mazzotta et al., 2023). With further research, endothelin receptor antagonists may also find application in the management of refractory hypertension. A variety of ETRAs have been developed and are categorized into three groups based on their functions: selective ETAR antagonists (ETARA), such as darusentan and ambrisentan (Enseleit et al., 2008; Croxtall and Keam, 2008). Selective ETBR antagonists (ETBRA) like bosentan and non-selective ETRAs including macitentan (Hosseinbalam et al., 2023; Grünig et al., 2024). Additionally, there are non-selective ERA aprocitentan (Dhillon, 2024).
6.1 The progression of antihypertensive effects exerted by endothelin and its antagonists
Endothelin antagonists can be utilized for the treatment of essential and refractory hypertension, with bosentan being the first ETRA employed in clinical trials for hypertension management. The findings from this study demonstrated that bosentan alone exhibited a statistically significant reduction in blood pressure after 4 weeks among patients with essential hypertension, comparable to the antihypertensive effect of enalapril (Karam et al., 1996). Another study revealed that darusentan could potentially exert an evident antihypertensive effect in treating essential hypertension (Black et al., 2007). However, its efficacy was not superior to ACE inhibitors. Moreover, serious hepatic damage, pulmonary arterial dilatation, and other adverse effects associated with darusentan use, thereby limiting further exploration into monotherapy for essential hypertension treatment. In terms of the antihypertensive effect of different drugs in ETRA, it has been newly reported that in animal experiments, the new drug macitentan has a stronger antihypertensive effect than bosentan, and it can also be clinically studied (Iglarz et al., 2014). Aprocitentan helps to dilate blood vessels and lower blood pressure by antagonising ETA receptors. Aprocitentan is commonly used in the treatment of refractory hypertension, patients with hypertension that has failed to respond to other medications, either as monotherapy or in combination with other antihypertensive medications (Georgianos and Agarwal, 2023). Clinical trials have shown that Aprocitentan provides clinically meaningful reductions in systolic blood pressure (SBP) and diastolic blood pressure (DBP) in the treatment of patients with refractory hypertension, that such reductions in blood pressure can be sustained for up to 48 weeks, and that its adverse effects can be controlled and the adverse effects are controllable (Schlaich et al., 2022).
6.2 Potential targets for endothelin receptor antagonists in hypertension caused by antitumor therapy
Endothelin is not only associated with cancer, but also implicated in the adverse effects induced by antineoplastic drugs, particularly hypertension. Studies have demonstrated a significant elevation of ET-1 levels during the treatment of patients across various types of cancers (Aliabadi et al., 2022; Tapia and Niechi, 2019; Rosanò and Bagnato, 2016). Targeting the ET axis and inhibiting it through specific, selective, and dual-competitive ET receptor antagonists represents an appealing approach for cancer therapy (Tocci et al., 2023). Currently, ETA and/or ETB antagonists are undergoing clinical trials to evaluate their efficacy in diverse indications such as cardiovascular disease and cancer.
It has been demonstrated that the targeting mechanism of anti-tumour drugs is intricately associated with ET-1, while cancer metastasis relies on neovasculogenesis. Tumour cells secrete factors that stimulate angiogenic pathways to facilitate rapid growth and formation of new microvessels in a state of uncontrolled cell proliferation (Farhan et al., 2020). One approach employed by targeted anti-tumour drugs involves activating the endothelin system to induce vasoconstriction and thinning.
Activation of the ET system is a crucial factor contributing to the adverse effects caused by VEGF inhibitors, thereby favoring the utilization of ET receptor antagonists as a means to mitigate these undesired side effects. Selective ETA receptor antagonists exhibit promising potential in this regard. In a study investigating the cardioprotective effects of macitentan on animals treated with sunitinib, it was determined that concurrent administration of macitentan effectively prevented sunitinib-induced hypertension while also improving ejection fraction and reducing cardiac fibrosis (Sourdon et al., 2021).
However, ET receptor antagonists are currently not approved for the treatment of systemic hypertension or renal injury, and there is also a potential risk of adverse effects associated with selective ETA receptor blockade, particularly edema (Wykoff et al., 2022). Additionally, during VEGF inactivation, ETB receptors may undergo a phenotypic switch from vasodilator to vasoconstrictor, necessitating the use of dual ET receptor antagonists (Koyama et al., 2014). Another potentially superior approach could be targeting downstream ET-1 signaling to prevent VEGF inhibitor-induced hypertension and renal injury or interfering with ET-1 upregulation.
Endothelin receptor antagonists possess potential therapeutic value not only in the management of hypertension caused by targeted antineoplastic agents, but also in directly targeting cancer itself. Macitentan can disrupt the β-arr1 signaling network by obstructing the ET-1 receptor, thereby impeding ET-1 signaling and enhancing cancer cell response to platinum-based chemotherapy (Tocci et al., 2021). Furthermore, it downregulates angiogenic and metastatic effects across various types of cancer. In a porcine model of sunitinib-induced hypertension discovered that treatment with tizosentan (a non-selective endothelin receptor antagonist) completely mitigated sunitinib-induced elevation in blood pressure effects (Kappers et al., 2012). Using a selective endothelin receptor antagonist, further elucidated that sunitinib-induced hypertension and proteinuria are mediated via ETA receptors rather than ETB receptors. This finding aligns with known ETA-mediated effects on smooth muscle cell contraction. Aprocitentan is an endogenous antagonist of the angiotensin ETA receptor, which plays a crucial role in regulating vascular tone and water-salt homeostasis (Danaietash et al., 2022). In the field of oncology and the management of tumor-induced hypertension, Aprocitentan emerges as a promising therapeutic target due to its novel mechanism, remarkable efficacy, and excellent tolerability.
7 The summary and future prospects
The cardiovascular toxicity and other side effects caused by VEGF inhibitors in anti-tumor therapy have become one of the main reasons limiting the anti-tumor therapy process with VEGF inhibitors. Traditional anti-hypertensive drugs are not effective for treating such hypertension, and there is a close relationship between traditional anti-hypertensive drugs and the occurrence and development of some tumors. Therefore, it is of great importance and urgent need to explore the relevant mechanisms of cardiovascular complications caused by VEGF inhibitors in anti-tumor therapy for clinical smooth application of VEGF inhibitors.
Vasoactive molecules, such as VEGF and ET, exhibit cytokine-like activity and regulate endothelial cell growth, migration, and inflammation. Several endothelial mediators and their receptors are targeted by currently approved angiogenesis inhibitors, including monoclonal antibodies against VEGF or inhibitors of vascular receptor protein kinases and signaling pathways. Pharmacological intervention that disrupts the protective function of endothelial cells can lead to similar adverse effects. Clinically, hypertension is the most common side effect associated with inhibition of the VEGF signaling pathway. Hypertension also poses a significant risk for cancer patients, as it increases mortality and morbidity related to cardiovascular disease. When hypertension reaches a certain level, cancer patients may need to discontinue antineoplastic drugs in order to prevent further elevation of blood pressure. Therefore, hypertension is not only an adverse reaction to the use of antineoplastic drugs in cancer patients but also a significant risk factor for increased mortality in this population. The management of oncological hypertension is a critical aspect that requires attention. Commonly used antihypertensive medications include diuretics, β-blockers, calcium antagonists, angiotensin-converting enzyme inhibitors, and angiotensin II receptor antagonists. However, there is a need to explore new drugs for refractory hypertension associated with cancer treatment. One promising option worth investigating is endothelin antagonists. Endothelin plays a crucial role in the pathophysiology of hypertensive complications related to cancer therapy, and inhibiting its axis can effectively reduce blood pressure levels. This research direction represents our current focus and exploration.
Author contributions
QH: Conceptualization, Data curation, Methodology, Project administration, Writing–original draft. JL: Software, Writing–review and editing. CM: Investigation, Writing–review and editing. GL: Data curation, Validation, Writing–review and editing. JL: Methodology, Writing–review and editing. QS: Conceptualization, Formal Analysis, Writing–review and editing. LC: Formal Analysis, Writing–review and editing. HG: Software, Writing–review and editing. QS: Software, Writing–review and editing. JY: Conceptualization, Visualization, Writing–review and editing. SL: Software, Writing–review and editing. WW: Conceptualization, Data curation, Formal Analysis, Investigation, Project administration, Writing–original draft, Writing–review and editing.
Funding
The author(s) declare that financial support was received for the research, authorship, and/or publication of this article. Funded by the Medicine and health science and technology plan projects in zhejiang province (2024KY1646) and Huzhou City Natural Science Foundation (2024YZ60).
Acknowledgments
We thank each editor and reviewer of this article, which has undergone a very careful and thorough review that greatly improved the original paper. We also thank all the participants at the First People’s Hospital of Huzhou and Huzhou Key Laboratory of Translational Medicine.
Conflict of interest
The authors declare that the research was conducted in the absence of any commercial or financial relationships that could be construed as a potential conflict of interest.
Publisher’s note
All claims expressed in this article are solely those of the authors and do not necessarily represent those of their affiliated organizations, or those of the publisher, the editors and the reviewers. Any product that may be evaluated in this article, or claim that may be made by its manufacturer, is not guaranteed or endorsed by the publisher.
Abbreviations
eNOS, Endothelial Nitric Oxide Synthase; ET-1, Endothelin 1; ETA, Endothelin A; ETB, Endothelin B; NO, Nitric Oxid; ET3, Endothelin 3; VEGF, Vascular Endothelial Growth Factor; VEGFR, Vascular Endothelial Growth Factor Receptor; TKI, Tyrosine Kinase Inhibitors; BH4, Tetrahydrobiopterin; FOXO3a, Forkhead Box O3a; AXL, AXL Receptor Tyrosine Kinase; c-MET, Hepatocyte Growth Factor Receptor; NAPDH, Nicotinamide Adenine Dinucleotide Phosphate (reduced form).
References
Abdel-Samad, D., Bkaily, G., Magder, S., and Jacques, D. (2016). ETA and ETB receptors contribute to neuropeptide Y-induced secretion of endothelin-1 in right but not left human ventricular endocardial endothelial cells. Neuropeptides 55, 145–153. doi:10.1016/j.npep.2016.01.001
Adebiyi, A., Thomas-Gatewood, C. M., Leo, M. D., Kidd, M. W., Neeb, Z. P., and Jaggar, J. H. (2012). An elevation in physical coupling of type 1 inositol 1,4,5-trisphosphate (IP3) receptors to transient receptor potential 3 (TRPC3) channels constricts mesenteric arteries in genetic hypertension. Hypertension 60, 1213–1219. doi:10.1161/HYPERTENSIONAHA.112.198820
Alexandre, J., Cautela, J., Ederhy, S., Damaj, G. L., Salem, J. E., Barlesi, F., et al. (2020). Cardiovascular toxicity related to cancer treatment: a pragmatic approach to the American and European cardio-oncology guidelines. J. Am. Heart Assoc. 9, e018403. doi:10.1161/JAHA.120.018403
Aliabadi, P., Sadri, M., Siri, G., Ebrahimzadeh, F., Yazdani, Y., Gusarov, A. M., et al. (2022). Restoration of miR-648 overcomes 5-FU-resistance through targeting ET-1 in gastric cancer cells in-vitro. Pathol. Res. Pract. 239, 154139. doi:10.1016/j.prp.2022.154139
Amiri, F., Virdis, A., Neves, M. F., Iglarz, M., Seidah, N. G., Touyz, R. M., et al. (2004). Endothelium-restricted overexpression of human endothelin-1 causes vascular remodeling and endothelial dysfunction. Circulation 110, 2233–2240. doi:10.1161/01.CIR.0000144462.08345.B9
Black, H. R., Bakris, G. L., Weber, M. A., Weiss, R., Shahawy, M. E., Marple, R., et al. (2007). Efficacy and safety of darusentan in patients with resistant hypertension: results from a randomized, double-blind, placebo-controlled dose-ranging study. J. Clin. Hypertens. (Greenwich) 9, 760–769. doi:10.1111/j.1524-6175.2007.07244.x
Bonvallet, S. T., Oka, M., Yano, M., Zamora, M. R., McMurtry, I. F., and Stelzner, T. J. (1993). BQ123, an ETA receptor antagonist, attenuates endothelin-1-induced vasoconstriction in rat pulmonary circulation. J. Cardiovasc Pharmacol. 22, 39–43. doi:10.1097/00005344-199307000-00007
Camarda, N., Travers, R., Yang, V. K., London, C., and Jaffe, I. Z. (2022). VEGF receptor inhibitor-induced hypertension: emerging mechanisms and clinical implications. Curr. Oncol. Rep. 24, 463–474. doi:10.1007/s11912-022-01224-0
Cao, Y., Fang, Y., Mu, J., and Liu, X. (2016). High salt medium activates RhoA/ROCK and downregulates eNOS expression via the upregulation of ADMA. Mol. Med. Rep. 14, 606–612. doi:10.3892/mmr.2016.5241
Carneiro, F. S., Nunes, K. P., Giachini, F. R., Lima, V. V., Carneiro, Z. N., Nogueira, E. F., et al. (2008). Activation of the ET-1/ETA pathway contributes to erectile dysfunction associated with mineralocorticoid hypertension. J. Sex. Med. 5, 2793–2807. doi:10.1111/j.1743-6109.2008.01009.x
Chen, W. H., Chen, C. H., Hsu, M. C., Chang, R. W., Wang, C. H., and Lee, T. S. (2024). Advances in the molecular mechanisms of statins in regulating endothelial nitric oxide bioavailability: interlocking biology between eNOS activity and L-arginine metabolism. Biomed. Pharmacother. 171, 116192. doi:10.1016/j.biopha.2024.116192
Chen, Y., Su, X., Qin, Q., Yu, Y., Jia, M., Zhang, H., et al. (2020). New insights into phenotypic switching of VSMCs induced by hyperhomocysteinemia: role of endothelin-1 signaling. Biomed. Pharmacother. 123, 109758. doi:10.1016/j.biopha.2019.109758
Chen, Y. C., Chung, C. C., Lin, Y. K., and Chen, Y. J. (2018). Genetic and ethnic modulation of cardiovascular toxicity of vascular endothelial growth factor inhibitors. Ann. Med. 50, 46–56. doi:10.1080/07853890.2017.1383629
Choraghe, R. P., Kołodziej, T., Buser, A., Rajfur, Z., and Neumann, A. K. (2020). RHOA-mediated mechanical force generation through Dectin-1. J. Cell Sci. 133, jcs236166. doi:10.1242/jcs.236166
Chuang, C. L., Chang, C. C., Hsu, S. J., Huang, H. C., Lee, F. Y., Huang, L. J., et al. (2018). Endotoxemia-enhanced renal vascular reactivity to endothelin-1 in cirrhotic rats. Am. J. Physiol. Gastrointest. Liver Physiol. 315, G752–g761. doi:10.1152/ajpgi.00302.2017
Ciccarese, C., Iacovelli, R., Porta, C., Procopio, G., Bria, E., Astore, S., et al. (2021). Efficacy of VEGFR-TKIs plus immune checkpoint inhibitors in metastatic renal cell carcinoma patients with favorable IMDC prognosis. Cancer Treat. Rev. 100, 102295. doi:10.1016/j.ctrv.2021.102295
Coelho, S. C., Berillo, O., Caillon, A., Ouerd, S., Fraulob-Aquino, J. C., Barhoumi, T., et al. (2018). Three-Month endothelial human endothelin-1 overexpression causes blood pressure elevation and vascular and kidney injury. Hypertension 71, 208–216. doi:10.1161/HYPERTENSIONAHA.117.09925
Croxtall, J. D., and Keam, S. J. (2008). Ambrisentan. Drugs 68, 2195–2204. doi:10.2165/00003495-200868150-00008
Danaietash, P., Verweij, P., Wang, J. G., Dresser, G., Kantola, I., Lawrence, M. K., et al. (2022). Identifying and treating resistant hypertension in PRECISION: a randomized long-term clinical trial with aprocitentan. J. Clin. Hypertens. (Greenwich) 24, 804–813. doi:10.1111/jch.14517
Davenport, A. P., Hyndman, K. A., Dhaun, N., Southan, C., Kohan, D. E., Pollock, J. S., et al. (2016). Endothelin. Pharmacol. Rev. 68, 357–418. doi:10.1124/pr.115.011833
Davenport, A. P., and Maguire, J. J. (2006). Endothelin. Handb. Exp. Pharmacol., 295–329. doi:10.1007/3-540-32967-6_9
Du, D., Liu, C., Qin, M., Zhang, X., Xi, T., Yuan, S., et al. (2022). Metabolic dysregulation and emerging therapeutical targets for hepatocellular carcinoma. Acta Pharm. Sin. B 12, 558–580. doi:10.1016/j.apsb.2021.09.019
Eid, A. H. (2024). A chance to grow and excel as an associate editor of pharmacological reviews-editorial. Pharmacol. Rev. 76, 321–322. doi:10.1124/pharmrev.124.001101
Enseleit, F., Lüscher, T. F., and Ruschitzka, F. (2008). Darusentan: a new perspective for treatment of resistant hypertension? Expert Opin. Investig. Drugs 17, 1255–1263. doi:10.1517/13543784.17.8.1255
Facemire, C. S., Nixon, A. B., Griffiths, R., Hurwitz, H., and Coffman, T. M. (2009). Vascular endothelial growth factor receptor 2 controls blood pressure by regulating nitric oxide synthase expression. Hypertension 54, 652–658. doi:10.1161/HYPERTENSIONAHA.109.129973
Fallahi, P., Ferrari, S. M., Galdiero, M. R., Varricchi, G., Elia, G., Ragusa, F., et al. (2022). Molecular targets of tyrosine kinase inhibitors in thyroid cancer. Semin. Cancer Biol. 79, 180–196. doi:10.1016/j.semcancer.2020.11.013
Fan, F., Yang, C., Piao, E., Shi, J., and Zhang, J. (2024). Mechanisms of chondrocyte regulated cell death in osteoarthritis: focus on ROS-triggered ferroptosis, parthanatos, and oxeiptosis. Biochem. Biophys. Res. Commun. 705, 149733. doi:10.1016/j.bbrc.2024.149733
Farhan, M., Silva, M., Xingan, X., Huang, Y., and Zheng, W. (2020). Role of FOXO transcription factors in cancer metabolism and angiogenesis. Cells 9, 1586. doi:10.3390/cells9071586
Finch, J., and Conklin, D. J. (2016). Air pollution-induced vascular dysfunction: potential role of endothelin-1 (ET-1) system. Cardiovasc Toxicol. 16, 260–275. doi:10.1007/s12012-015-9334-y
Gentile, M., Martino, M., Recchia, A. G., Vigna, E., Morabito, L., and Morabito, F. (2016). Sorafenib for the treatment of multiple myeloma. Expert Opin. Investig. Drugs 25, 743–749. doi:10.1517/13543784.2016.1169272
Georgianos, P. I., and Agarwal, R. (2023). Hypertension in chronic kidney disease-treatment standard 2023. Nephrol. Dial. Transpl. 38, 2694–2703. doi:10.1093/ndt/gfad118
Grünig, E., Jansa, P., Fan, F., Hauser, J. A., Pannaux, M., Morganti, A., et al. (2024). Randomized trial of macitentan/tadalafil single-tablet combination therapy for pulmonary arterial hypertension. J. Am. Coll. Cardiol. 83, 473–484. doi:10.1016/j.jacc.2023.10.045
Gu, X., Zhao, L., Zhu, J., Gu, H., Li, H., Wang, L., et al. (2015). Serum mimecan is associated with arterial stiffness in hypertensive patients. J. Am. Heart Assoc. 4, e002010. doi:10.1161/JAHA.115.002010
Harrison, M., Zinovkin, D., and Pranjol, M. Z. I. (2024). Endothelin-1 and its role in cancer and potential therapeutic opportunities. Biomedicines 12, 511. doi:10.3390/biomedicines12030511
Hopfner, R. L., Hasnadka, R. V., Wilson, T. W., McNeill, J. R., and Gopalakrishnan, V. (1998). Insulin increases endothelin-1-evoked intracellular free calcium responses by increased ET(A) receptor expression in rat aortic smooth muscle cells. Diabetes 47, 937–944. doi:10.2337/diabetes.47.6.937
Hosseinbalam, M., Nouri, R., Farajzadegan, Z., and Mottaghi, P. (2023). Effectiveness of bosentan in the treatment of systemic sclerosis-related digital ulcers: systematic review and meta-analysis. J. Res. Med. Sci. 28, 3. doi:10.4103/jrms.jrms_386_22
Hsieh, J. J., Purdue, M. P., Signoretti, S., Swanton, C., Albiges, L., Schmidinger, M., et al. (2017). Renal cell carcinoma. Nat. Rev. Dis. Prim. 3, 17009. doi:10.1038/nrdp.2017.9
Hwang, S., Lee, W., Ravi, D., Devine, W., Yong, M., Diebold, R. B., et al. (2024). Novel small molecule ROCK2 inhibitor GNS-3595 attenuates pulmonary fibrosis in preclinical studies. Am. J. Respir. Cell Mol. Biol. 71, 430–441. doi:10.1165/rcmb.2023-0401OC
Iglarz, M., Bossu, A., Wanner, D., Bortolamiol, C., Rey, M., Hess, P., et al. (2014). Comparison of pharmacological activity of macitentan and bosentan in preclinical models of systemic and pulmonary hypertension. Life Sci. 118, 333–339. doi:10.1016/j.lfs.2014.02.018
Inoue, A., Yanagisawa, M., Kimura, S., Kasuya, Y., Miyauchi, T., Goto, K., et al. (1989). The human endothelin family: three structurally and pharmacologically distinct isopeptides predicted by three separate genes. Proc. Natl. Acad. Sci. U. S. A. 86, 2863–2867. doi:10.1073/pnas.86.8.2863
Iwagawa, T., Saita, K., Sagara, H., and Watanabe, S. (2023). Downregulation of VEGF in the retinal pigment epithelium followed by choriocapillaris atrophy after NaIO3 treatment in mice. Exp. Eye Res. 234, 109598. doi:10.1016/j.exer.2023.109598
Jin, J., Xie, Y., Zhang, J. S., Wang, J. Q., Dai, S. J., He, W. F., et al. (2023). Sunitinib resistance in renal cell carcinoma: from molecular mechanisms to predictive biomarkers. Drug Resist Updat 67, 100929. doi:10.1016/j.drup.2023.100929
Kai, Y., Kon, R., Ikarashi, N., Chiba, Y., Kamei, J., and Sakai, H. (2019). Role of Rac1 in augmented endothelin-1-induced bronchial contraction in airway hyperresponsive mice. J. Pharmacol. Sci. 141, 106–110. doi:10.1016/j.jphs.2019.09.011
Kappers, M. H., de Beer, V. J., Zhou, Z., Danser, A. H., Sleijfer, S., Duncker, D. J., et al. (2012). Sunitinib-induced systemic vasoconstriction in swine is endothelin mediated and does not involve nitric oxide or oxidative stress. Hypertension 59, 151–157. doi:10.1161/HYPERTENSIONAHA.111.182220
Kappers, M. H., Smedts, F. M., Horn, T., van Esch, J. H., Sleijfer, S., Leijten, F., et al. (2011). The vascular endothelial growth factor receptor inhibitor sunitinib causes a preeclampsia-like syndrome with activation of the endothelin system. Hypertension 58, 295–302. doi:10.1161/HYPERTENSIONAHA.111.173559
Kappers, M. H., van Esch, J. H., Sluiter, W., Sleijfer, S., Danser, A. H., and van den Meiracker, A. H. (2010). Hypertension induced by the tyrosine kinase inhibitor sunitinib is associated with increased circulating endothelin-1 levels. Hypertension 56, 675–681. doi:10.1161/HYPERTENSIONAHA.109.149690
Karam, H., Heudes, D., Bruneval, P., Gonzales, M. F., Löffler, B. M., Clozel, M., et al. (1996). Endothelin antagonism in end-organ damage of spontaneously hypertensive rats. Comparison with angiotensin-converting enzyme inhibition and calcium antagonism. Hypertension 28, 379–385. doi:10.1161/01.hyp.28.3.379
Kim, J. S., Choi, G. H., Jung, Y., Kim, K. M., Jang, S. J., Yu, E. S., et al. (2018). Downregulation of Raf-1 kinase inhibitory protein as a sorafenib resistance mechanism in hepatocellular carcinoma cell lines. J. Cancer Res. Clin. Oncol. 144, 1487–1501. doi:10.1007/s00432-018-2672-y
Kobayashi, N., Takeshima, H., Fukushima, H., Koguchi, W., Mamada, Y., Hirata, H., et al. (2009). Cardioprotective effects of pitavastatin on cardiac performance and remodeling in failing rat hearts. Am. J. Hypertens. 22, 176–182. doi:10.1038/ajh.2008.333
Kostov, K. (2021). The causal relationship between endothelin-1 and hypertension: focusing on endothelial dysfunction, arterial stiffness, vascular remodeling, and blood pressure regulation. Life (Basel) 11, 986. doi:10.3390/life11090986
Koyama, Y., Hayashi, M., Nagae, R., Tokuyama, S., and Konishi, T. (2014). Endothelin-1 increases the expression of VEGF-R1/Flt-1 receptors in rat cultured astrocytes through ETB receptors. J. Neurochem. 130, 759–769. doi:10.1111/jnc.12770
Kumar, S., Oishi, P. E., Rafikov, R., Aggarwal, S., Hou, Y., Datar, S. A., et al. (2013). Tezosentan increases nitric oxide signaling via enhanced hydrogen peroxide generation in lambs with surgically induced acute increases in pulmonary blood flow. J. Cell Biochem. 114, 435–447. doi:10.1002/jcb.24383
Lankhorst, S., Baelde, H. J., Kappers, M. H., Smedts, F. M., Hansen, A., Clahsen-van Groningen, M. C., et al. (2015). Greater sensitivity of blood pressure than renal toxicity to tyrosine kinase receptor inhibition with sunitinib. Hypertension 66, 543–549. doi:10.1161/HYPERTENSIONAHA.115.05435
Lankhorst, S., Kappers, M. H., van Esch, J. H., Danser, A. H., and van den Meiracker, A. H. (2014). Hypertension during vascular endothelial growth factor inhibition: focus on nitric oxide, endothelin-1, and oxidative stress. Antioxid. Redox Signal 20, 135–145. doi:10.1089/ars.2013.5244
Laudette, M., Sainte-Marie, Y., Cousin, G., Bergonnier, D., Belhabib, I., Brun, S., et al. (2021). Cyclic AMP-binding protein Epac1 acts as a metabolic sensor to promote cardiomyocyte lipotoxicity. Cell Death Dis. 12, 824. doi:10.1038/s41419-021-04113-9
Le, X., Nilsson, M., Goldman, J., Reck, M., Nakagawa, K., Kato, T., et al. (2021). Dual EGFR-VEGF pathway inhibition: a promising strategy for patients with EGFR-mutant NSCLC. J. Thorac. Oncol. 16, 205–215. doi:10.1016/j.jtho.2020.10.006
Lei, T., Xu, T., Zhang, N., Zou, X., Kong, Z., Wei, C., et al. (2023). Anlotinib combined with osimertinib reverses acquired osimertinib resistance in NSCLC by targeting the c-MET/MYC/AXL axis. Pharmacol. Res. 188, 106668. doi:10.1016/j.phrs.2023.106668
Leite, L. N., Lacchini, R., Carnio, E. C., Queiroz, R. H., Tanus-Santos, J. E., de Oliveira, A. M., et al. (2013). Ethanol consumption increases endothelin-1 expression and reactivity in the rat cavernosal smooth muscle. Alcohol Alcohol 48, 657–666. doi:10.1093/alcalc/agt057
Leo, F., Suvorava, T., Heuser, S. K., Li, J., LoBue, A., Barbarino, F., et al. (2021). Red blood cell and endothelial eNOS independently regulate circulating nitric oxide metabolites and blood pressure. Circulation 144, 870–889. doi:10.1161/CIRCULATIONAHA.120.049606
Li, C., Ma, L., Wang, Q., Shao, X., Guo, L., Chen, J., et al. (2022). Rho kinase inhibition ameliorates vascular remodeling and blood pressure elevations in a rat model of apatinib-induced hypertension. J. Hypertens. 40, 675–684. doi:10.1097/hjh.0000000000003060
Li, S. (2021). Anlotinib: a novel targeted drug for bone and soft tissue sarcoma. Front. Oncol. 11, 664853. doi:10.3389/fonc.2021.664853
Lin, X., Ouyang, S., Zhi, C., Li, P., Tan, X., Ma, W., et al. (2022). Focus on ferroptosis, pyroptosis, apoptosis and autophagy of vascular endothelial cells to the strategic targets for the treatment of atherosclerosis. Arch. Biochem. Biophys. 715, 109098. doi:10.1016/j.abb.2021.109098
Liu, D., Song, Y., Chen, H., You, Y., Zhu, L., Zhang, J., et al. (2023b). Anti-VEGFR2 F(ab')(2) drug conjugate promotes renal accumulation and glomerular repair in diabetic nephropathy. Nat. Commun. 14, 8268. doi:10.1038/s41467-023-43847-2
Liu, Z. L., Chen, H. H., Zheng, L. L., Sun, L. P., and Shi, L. (2023a). Angiogenic signaling pathways and anti-angiogenic therapy for cancer. Signal Transduct. Target Ther. 8, 198. doi:10.1038/s41392-023-01460-1
Lv, B., Chen, J., and Liu, X. L. (2022). Anlotinib-induced hypertension: current concepts and future prospects. Curr. Pharm. Des. 28, 216–224. doi:10.2174/1381612827666211006145141
Ma, J., Li, Y., Yang, X., Liu, K., Zhang, X., Zuo, X., et al. (2023). Signaling pathways in vascular function and hypertension: molecular mechanisms and therapeutic interventions. Signal Transduct. Target Ther. 8, 168. doi:10.1038/s41392-023-01430-7
Maguire, J. J., and Davenport, A. P. (2015). Endothelin receptors and their antagonists. Semin. Nephrol. 35, 125–136. doi:10.1016/j.semnephrol.2015.02.002
Mangana, J., Levesque, M. P., Karpova, M. B., and Dummer, R. (2012). Sorafenib in melanoma. Expert Opin. Investig. Drugs 21, 557–568. doi:10.1517/13543784.2012.665872
Mao, L., Lian, B., Li, C., Bai, X., Zhou, L., Cui, C., et al. (2023). Camrelizumab plus apatinib and temozolomide as first-line treatment in patients with advanced acral melanoma: the CAP 03 phase 2 nonrandomized clinical trial. JAMA Oncol. 9, 1099–1107. doi:10.1001/jamaoncol.2023.1363
Mazzotta, E., Grants, I., Villalobos-Hernandez, E., Chaudhuri, S., McClain, J. L., Seguella, L., et al. (2023). BQ788 reveals glial ET(B) receptor modulation of neuronal cholinergic and nitrergic pathways to inhibit intestinal motility: linked to postoperative ileus. Br. J. Pharmacol. 180, 2550–2576. doi:10.1111/bph.16145
Mourad, J. J., des Guetz, G., Debbabi, H., and Levy, B. I. (2008). Blood pressure rise following angiogenesis inhibition by bevacizumab. A crucial role for microcirculation. Ann. Oncol. 19, 927–934. doi:10.1093/annonc/mdm550
Muhammad, R. N., Sallam, N., and El-Abhar, H. S. (2020). Activated ROCK/Akt/eNOS and ET-1/ERK pathways in 5-fluorouracil-induced cardiotoxicity: modulation by simvastatin. Sci. Rep. 10, 14693. doi:10.1038/s41598-020-71531-8
Narayan, V., Liu, T., Song, Y., Mitchell, J., Sicks, J., Gareen, I., et al. (2023). Early increases in blood pressure and major adverse cardiovascular events in patients with renal cell carcinoma and thyroid cancer treated with VEGFR TKIs. J. Natl. Compr. Canc Netw. 21, 1039–1049.e10. doi:10.6004/jnccn.2023.7047
Neves, K. B., Rios, F. J., Jones, R., Evans, T. R. J., Montezano, A. C., and Touyz, R. M. (2019). Microparticles from vascular endothelial growth factor pathway inhibitor-treated cancer patients mediate endothelial cell injury. Cardiovasc Res. 115, 978–988. doi:10.1093/cvr/cvz021
Ortega Mateo, A., and de Artiñano, A. A. (1997). Highlights on endothelins: a review. Pharmacol. Res. 36, 339–351. doi:10.1006/phrs.1997.0246
Pannucci, P., Van Daele, M., Cooper, S. L., Wragg, E. S., March, J., Groenen, M., et al. (2023). Role of endothelin ET(A) receptors in the hypertension induced by the VEGFR-2 kinase inhibitors axitinib and lenvatinib in conscious freely-moving rats. Biochem. Pharmacol. 228, 116007. doi:10.1016/j.bcp.2023.116007
Peppiatt-Wildman, C. M., Albert, A. P., Saleh, S. N., and Large, W. A. (2007). Endothelin-1 activates a Ca2+-permeable cation channel with TRPC3 and TRPC7 properties in rabbit coronary artery myocytes. J. Physiol. 580, 755–764. doi:10.1113/jphysiol.2006.126656
Pérez-Gutiérrez, L., and Ferrara, N. (2023). Biology and therapeutic targeting of vascular endothelial growth factor A. Nat. Rev. Mol. Cell Biol. 24, 816–834. doi:10.1038/s41580-023-00631-w
Plimack, E. R., Powles, T., Stus, V., Gafanov, R., Nosov, D., Waddell, T., et al. (2023). Pembrolizumab plus axitinib versus sunitinib as first-line treatment of advanced renal cell carcinoma: 43-month follow-up of the phase 3 KEYNOTE-426 study. Eur. Urol. 84, 449–454. doi:10.1016/j.eururo.2023.06.006
Porta, M., and Striglia, E. (2020). Intravitreal anti-VEGF agents and cardiovascular risk. Intern Emerg. Med. 15, 199–210. doi:10.1007/s11739-019-02253-7
Ramalingam, S. S., Vansteenkiste, J., Planchard, D., Cho, B. C., Gray, J. E., Ohe, Y., et al. (2020). Overall survival with osimertinib in untreated, EGFR-mutated advanced NSCLC. N. Engl. J. Med. 382, 41–50. doi:10.1056/NEJMoa1913662
Rattan, S. (2017). Ca2+/calmodulin/MLCK pathway initiates, and RhoA/ROCK maintains, the internal anal sphincter smooth muscle tone. Am. J. Physiol. Gastrointest. Liver Physiol. 312, G63–g66. doi:10.1152/ajpgi.00370.2016
Réa, D., and Hughes, T. P. (2022). Development of asciminib, a novel allosteric inhibitor of BCR-ABL1. Crit. Rev. Oncol. Hematol. 171, 103580. doi:10.1016/j.critrevonc.2022.103580
Rosanò, L., and Bagnato, A. (2016). β-arrestin1 at the cross-road of endothelin-1 signaling in cancer. J. Exp. Clin. Cancer Res. 35, 121. doi:10.1186/s13046-016-0401-4
Sarkar, J., Chakraborti, T., Chowdhury, A., Bhuyan, R., and Chakraborti, S. (2019). Protective role of epigallocatechin-3-gallate in NADPH oxidase-MMP2-Spm-Cer-S1P signalling axis mediated ET-1 induced pulmonary artery smooth muscle cell proliferation. J. Cell Commun. Signal 13, 473–489. doi:10.1007/s12079-018-00501-7
Schlaich, M. P., Bellet, M., Weber, M. A., Danaietash, P., Bakris, G. L., Flack, J. M., et al. (2022). Dual endothelin antagonist aprocitentan for resistant hypertension (PRECISION): a multicentre, blinded, randomised, parallel-group, phase 3 trial. Lancet 400, 1927–1937. doi:10.1016/S0140-6736(22)02034-7
Sedeek, M. H., Llinas, M. T., Drummond, H., Fortepiani, L., Abram, S. R., Alexander, B. T., et al. (2003). Role of reactive oxygen species in endothelin-induced hypertension. Hypertension 42, 806–810. doi:10.1161/01.HYP.0000084372.91932.BA
Shaw, P., Dwivedi, S. K. D., Bhattacharya, R., Mukherjee, P., and Rao, G. (2024). VEGF signaling: role in angiogenesis and beyond. Biochim. Biophys. Acta Rev. Cancer 1879, 189079. doi:10.1016/j.bbcan.2024.189079
Shen, G., Zheng, F., Ren, D., Du, F., Dong, Q., Wang, Z., et al. (2018). Anlotinib: a novel multi-targeting tyrosine kinase inhibitor in clinical development. J. Hematol. Oncol. 11, 120. doi:10.1186/s13045-018-0664-7
Simonetti, O., Lucarini, G., Campanati, A., Goteri, G., Zizzi, A., Marconi, B., et al. (2009). VEGF, survivin and NOS overexpression in psoriatic skin: critical role of nitric oxide synthases. J. Dermatol Sci. 54, 205–208. doi:10.1016/j.jdermsci.2008.12.012
Sobrano Fais, R., Menezes da Costa, R., Carvalho Mendes, A., Mestriner, F., Comerma-Steffensen, S. G., Tostes, R. C., et al. (2023). NLRP3 activation contributes to endothelin-1-induced erectile dysfunction. J. Cell Mol. Med. 27, 1–14. doi:10.1111/jcmm.17463
Soria, J. C., Ohe, Y., Vansteenkiste, J., Reungwetwattana, T., Chewaskulyong, B., Lee, K. H., et al. (2018). Osimertinib in untreated EGFR-mutated advanced non-small-cell lung cancer. N. Engl. J. Med. 378, 113–125. doi:10.1056/NEJMoa1713137
Sourdon, J., Facchin, C., Certain, A., Viel, T., Robin, B., Lager, F., et al. (2021). Sunitinib-induced cardiac hypertrophy and the endothelin axis. Theranostics 11, 3830–3838. doi:10.7150/thno.49837
Sourdon, J., Lager, F., Viel, T., Balvay, D., Moorhouse, R., Bennana, E., et al. (2017). Cardiac metabolic deregulation induced by the tyrosine kinase receptor inhibitor sunitinib is rescued by endothelin receptor antagonism. Theranostics 7, 2757–2774. doi:10.7150/thno.19551
Sun, H., Zheng, J., Xiao, J., Yue, J., Shi, Z., Xuan, Z., et al. (2022). TOPK/PBK is phosphorylated by ERK2 at serine 32, promotes tumorigenesis and is involved in sorafenib resistance in RCC. Cell Death Dis. 13, 450. doi:10.1038/s41419-022-04909-3
Sun, Q., Zhou, J., Zhang, Z., Guo, M., Liang, J., Zhou, F., et al. (2014). Discovery of fruquintinib, a potent and highly selective small molecule inhibitor of VEGFR 1, 2, 3 tyrosine kinases for cancer therapy. Cancer Biol. Ther. 15, 1635–1645. doi:10.4161/15384047.2014.964087
Taneja, G., Sud, A., Pendse, N., Panigrahi, B., Kumar, A., and Sharma, A. K. (2019). Nano-medicine and vascular endothelial dysfunction: options and delivery strategies. Cardiovasc Toxicol. 19, 1–12. doi:10.1007/s12012-018-9491-x
Tapia, J. C., and Niechi, I. (2019). Endothelin-converting enzyme-1 in cancer aggressiveness. Cancer Lett. 452, 152–157. doi:10.1016/j.canlet.2019.03.033
Tavakolian, S., Tabaeian, S. P., Namazi, A., Faghihloo, E., and Akbari, A. (2024). Role of the VEGF in virus-associated cancers. Rev. Med. Virol. 34, e2493. doi:10.1002/rmv.2493
Tocci, P., Blandino, G., and Bagnato, A. (2021). YAP and endothelin-1 signaling: an emerging alliance in cancer. J. Exp. Clin. Cancer Res. 40, 27. doi:10.1186/s13046-021-01827-8
Tocci, P., Roman, C., Sestito, R., Di Castro, V., Sacconi, A., Molineris, I., et al. (2023). Targeting tumor-stroma communication by blocking endothelin-1 receptors sensitizes high-grade serous ovarian cancer to PARP inhibition. Cell Death Dis. 14, 5. doi:10.1038/s41419-022-05538-6
Touyz, R. M., and Herrmann, J. (2018). Cardiotoxicity with vascular endothelial growth factor inhibitor therapy. NPJ Precis. Oncol. 2, 13. doi:10.1038/s41698-018-0056-z
Tsai, S. H., Lu, G., Xu, X., Ren, Y., Hein, T. W., and Kuo, L. (2017). Enhanced endothelin-1/Rho-kinase signalling and coronary microvascular dysfunction in hypertensive myocardial hypertrophy. Cardiovasc Res. 113, 1329–1337. doi:10.1093/cvr/cvx103
Ushio-Fukai, M., and Alexander, R. W. (2004). Reactive oxygen species as mediators of angiogenesis signaling: role of NAD(P)H oxidase. Mol. Cell Biochem. 264, 85–97. doi:10.1023/b:mcbi.0000044378.09409.b5
Versmissen, J., Mirabito Colafella, K. M., Koolen, S. L. W., and Danser, A. H. J. (2019). Vascular cardio-oncology: vascular endothelial growth factor inhibitors and hypertension. Cardiovasc Res. 115, 904–914. doi:10.1093/cvr/cvz022
Wang, Q., Gao, S., Shou, Y., Jia, Y., Wei, Z., Liu, Y., et al. (2023). AIM2 promotes renal cell carcinoma progression and sunitinib resistance through FOXO3a-ACSL4 axis-regulated ferroptosis. Int. J. Biol. Sci. 19, 1266–1283. doi:10.7150/ijbs.79853
Wang, W., He, Q., Li, C., Zhuang, C., Zhang, H., Wang, Q., et al. (2022c). Research on the mechanism and prevention of hypertension caused by apatinib through the RhoA/ROCK signaling pathway in a Mouse model of gastric cancer. Front. Cardiovasc Med. 9, 873829. doi:10.3389/fcvm.2022.873829
Wang, W., He, Q., Zhang, H., Zhuang, C., Wang, Q., Li, C., et al. (2021). A narrative review on the interaction between genes and the treatment of hypertension and breast cancer. Ann. Transl. Med. 9, 894. doi:10.21037/atm-21-2133
Wang, W., He, Q., Zhuang, C., Zhang, H., Fan, X., Wang, Q., et al. (2022a). Apatinib through activating the RhoA/ROCK signaling pathway to cause dysfunction of vascular smooth muscle cells. Appl. Biochem. Biotechnol. 194, 5367–5385. doi:10.1007/s12010-022-04020-5
Wang, W., Li, G., Ma, J., Fan, X., Lu, J., Sun, Q., et al. (2024). Microvascular rarefaction caused by the NOTCH signaling pathway is a key cause of TKI-apatinib-induced hypertension and cardiac damage. Front. Pharmacol. 15, 1346905. doi:10.3389/fphar.2024.1346905
Wang, X., Jiang, S., Fei, L., Dong, F., Xie, L., Qiu, X., et al. (2022b). Tacrolimus causes hypertension by increasing vascular contractility via RhoA (ras Homolog family member A)/ROCK (Rho-Associated protein kinase) pathway in mice. Hypertension 79, 2228–2238. doi:10.1161/HYPERTENSIONAHA.122.19189
Watanabe, T., Awane, Y., Ikeda, S., Fujiwara, S., Kubo, K., Kikuchi, T., et al. (1995). Pharmacology of a non-selective ETA and ETB receptor antagonist, TAK-044 and the inhibition of myocardial infarct size in rats. Br. J. Pharmacol. 114, 949–954. doi:10.1111/j.1476-5381.1995.tb13296.x
Wilcox, C. S., Wang, C., and Wang, D. (2019). Endothelin-1-Induced microvascular ROS and contractility in angiotensin-II-infused mice depend on COX and TP receptors. Antioxidants (Basel) 8, 193. doi:10.3390/antiox8060193
Wilhelm, S. M., Adnane, L., Newell, P., Villanueva, A., Llovet, J. M., and Lynch, M. (2008). Preclinical overview of sorafenib, a multikinase inhibitor that targets both Raf and VEGF and PDGF receptor tyrosine kinase signaling. Mol. Cancer Ther. 7, 3129–3140. doi:10.1158/1535-7163.MCT-08-0013
Wolf, D., Tseng, N., Seedorf, G., Roe, G., Abman, S. H., and Gien, J. (2014). Endothelin-1 decreases endothelial PPARγ signaling and impairs angiogenesis after chronic intrauterine pulmonary hypertension. Am. J. Physiol. Lung Cell Mol. Physiol. 306, L361–L371. doi:10.1152/ajplung.00277.2013
Wu, J., Liang, J., Liu, R., Lv, T., Fu, K., Jiang, L., et al. (2023). Autophagic blockade potentiates anlotinib-mediated ferroptosis in anaplastic thyroid cancer. Endocr. Relat. Cancer 30, e230036. doi:10.1530/ERC-23-0036
Wykoff, C. C., Abreu, F., Adamis, A. P., Basu, K., Eichenbaum, D. A., Haskova, Z., et al. (2022). Efficacy, durability, and safety of intravitreal faricimab with extended dosing up to every 16 weeks in patients with diabetic macular oedema (YOSEMITE and RHINE): two randomised, double-masked, phase 3 trials. Lancet 399, 741–755. doi:10.1016/S0140-6736(22)00018-6
Xia, Y., Tang, W., Qian, X., Li, X., Cheng, F., Wang, K., et al. (2022). Efficacy and safety of camrelizumab plus apatinib during the perioperative period in resectable hepatocellular carcinoma: a single-arm, open label, phase II clinical trial. J. Immunother. Cancer 10, e004656. doi:10.1136/jitc-2022-004656
Xie, C., Zhou, X., Liang, C., Li, X., Ge, M., Chen, Y., et al. (2021). Apatinib triggers autophagic and apoptotic cell death via VEGFR2/STAT3/PD-L1 and ROS/Nrf2/p62 signaling in lung cancer. J. Exp. Clin. Cancer Res. 40, 266. doi:10.1186/s13046-021-02069-4
Xu, Q., Wang, J., Sun, Y., Lin, Y., Liu, J., Zhuo, Y., et al. (2022). Efficacy and safety of sintilimab plus anlotinib for PD-L1-positive recurrent or metastatic cervical cancer: a multicenter, single-arm, prospective phase II trial. J. Clin. Oncol. 40, 1795–1805. doi:10.1200/JCO.21.02091
Xu, X., Ye, W., Chen, H., Liu, M., Jiang, W., and Fang, Z. (2021). Association of endothelial nitric oxide synthase intron 4a/b gene polymorphisms and hypertension: a systematic review and meta-analysis. J. Int. Med. Res. 49, 300060520979230. doi:10.1177/0300060520979230
Yingze, Y., Zhihong, J., Tong, J., Yina, L., Zhi, Z., Xu, Z., et al. (2022). NOX2-mediated reactive oxygen species are double-edged swords in focal cerebral ischemia in mice. J. Neuroinflammation 19, 184. doi:10.1186/s12974-022-02551-6
Yu, T., Jing, M., Gao, Y., Liu, C., Liu, L., Jia, H., et al. (2020). Study on the relationship between hyperthyroidism and vascular endothelial cell damage. Sci. Rep. 10, 6992. doi:10.1038/s41598-020-62796-0
Zamorano, J. L., Lancellotti, P., Rodriguez Muñoz, D., Aboyans, V., Asteggiano, R., Galderisi, M., et al. (2016). 2016 ESC Position Paper on cancer treatments and cardiovascular toxicity developed under the auspices of the ESC Committee for Practice Guidelines: The Task Force for cancer treatments and cardiovascular toxicity of the European Society of Cardiology (ESC). Eur. Heart J. 37, 2768–2801. doi:10.1093/eurheartj/ehw211
Zhang, W. M., Yip, K. P., Lin, M. J., Shimoda, L. A., Li, W. H., and Sham, J. S. (2003). ET-1 activates Ca2+ sparks in PASMC: local Ca2+ signaling between inositol trisphosphate and ryanodine receptors. Am. J. Physiol. Lung Cell Mol. Physiol. 285, L680–L690. doi:10.1152/ajplung.00067.2003
Zhao, J., Zhao, L., Guo, W., Wang, S., Tao, X., Li, L., et al. (2023). Efficacy, safety, and biomarker analysis of neoadjuvant camrelizumab and apatinib in patients with resectable NSCLC: a phase 2 clinical trial. J. Thorac. Oncol. 18, 780–791. doi:10.1016/j.jtho.2023.02.019
Zhao, L., Yuan, F., Pan, N., Yu, Y., Yang, H., Liu, Y., et al. (2021). CFTR deficiency aggravates Ang II induced vasoconstriction and hypertension by regulating Ca2+ influx and RhoA/Rock pathway in VSMCs. Front. Biosci. Landmark Ed. 26, 1396–1410. doi:10.52586/5034
Zheng, D., Liu, J., Piao, H., Zhu, Z., Wei, R., and Liu, K. (2022). ROS-triggered endothelial cell death mechanisms: focus on pyroptosis, parthanatos, and ferroptosis. Front. Immunol. 13, 1039241. doi:10.3389/fimmu.2022.1039241
Zhuang, R., Wu, J., Lin, F., Han, L., Liang, X., Meng, Q., et al. (2018). Fasudil preserves lung endothelial function and reduces pulmonary vascular remodeling in a rat model of end-stage pulmonary hypertension with left heart disease. Int. J. Mol. Med. 42, 1341–1352. doi:10.3892/ijmm.2018.3728
Ziemba, B. P., and Falke, J. J. (2018). A PKC-MARCKS-PI3K regulatory module links Ca2+ and PIP3 signals at the leading edge of polarized macrophages. PLoS One 13, e0196678. doi:10.1371/journal.pone.0196678
Keywords: hypertension, tyrosine kinase inhibitors, endothelin receptor antagonists, endothelin, aprocitentan
Citation: He Q, Lin J, Mo C, Li G, Lu J, Sun Q, Cao L, Gan H, Sun Q, Yao J, Lian S and Wang W (2025) Endothelin receptor antagonists (ERAs) can potentially be used as therapeutic drugs to reduce hypertension caused by small molecule tyrosine kinase inhibitors (TKIs). Front. Pharmacol. 15:1463520. doi: 10.3389/fphar.2024.1463520
Received: 12 July 2024; Accepted: 23 December 2024;
Published: 09 January 2025.
Edited by:
Awanish Mishra, National Institute of Pharmaceutical Education and Research, IndiaReviewed by:
Sarah Allegra, San Luigi Gonzaga University Hospital, ItalyHitesh Singh Chaouhan, National Institute of Neurological Disorders and Stroke (NIH), United States
Copyright © 2025 He, Lin, Mo, Li, Lu, Sun, Cao, Gan, Sun, Yao, Lian and Wang. This is an open-access article distributed under the terms of the Creative Commons Attribution License (CC BY). The use, distribution or reproduction in other forums is permitted, provided the original author(s) and the copyright owner(s) are credited and that the original publication in this journal is cited, in accordance with accepted academic practice. No use, distribution or reproduction is permitted which does not comply with these terms.
*Correspondence: WenJuan Wang, d3dqeXkwNzEyQDEyNi5jb20=