- 1Nanjing Lishui District Hospital of Traditional Chinese Medicine, Nanjing, China
- 2Clinical College of Traditional Chinese Medicine Hospital in Lishui, Jiangsu Health Vocational College, Nanjing, China
- 3Affiliated Hospital of Integrated Traditional Chinese and Western Medicine, Nanjing University of Chinese Medicine, Nanjing, China
- 4Tonglu Hospital of Traditional Chinese Medicine, Tonglu, China
- 5Wuxi Hospital Affiliated to Nanjing University of Chinese Medicine, Wuxi, China
Introduction: Premature ovarian insufficiency (POI) has affected about 3.7% of women of reproductive age and is a major factor contributing to infertility. Bushen Huoxue formula (BHF), a traditional Chinese medicine prescription, is clinically used to treat POI in China. This study aims to investigate the potential mechanisms of BHF in combating POI using corticosterone-induced rats and palmitic acid (PA)-challenged human ovarian granulosa cells (GCs).
Methods: Initially, ultra performance liquid chromatography tandem mass spectrometry was employed to analyze the components of BHF. The pharmacodynamic parameters evaluated included body weight, ovaries index, and serum hormone in rats. Follicle numbers were observed using H&E staining. Additionally, PCNA and TUNEL staining were used to assess GCs proliferation and apoptosis, respectively. Lipid accumulation and ROS levels were examined using Oil Red O and ROS staining. Protein expressions were determined by western blot. To probe mechanisms, cell viability and E2 levels in BHF-treated, PA-stimulated GCs were determined using MTT and ELISA, respectively. Cell apoptosis and ROS levels were assessed using TUNEL and ROS staining. Proteins related to lipid metabolism and autophagy in PA-stimulated GCs were studied using agonists.
Results: Our results shown that BHF effectively normalized serum hormone levels, including follicle-stimulating hormone (FSH), anti-Müllerian hormone (AMH), estradiol (E2), and luteinizing hormone (LH). Concurrently, BHF also significantly reduced follicular atresia and promoted cell proliferation while inhibiting apoptosis in POI rats. Furthermore, BHF mitigated ovarian lipid accumulation by modulating lipid metabolism, which included reducing lipid synthesis (expression of peroxisome proliferator-activated receptor γ and CCAAT/enhancer binding protein α), increasing lipid catabolism (expression of adipose triglyceride lipase), and enhancing lipid oxidation (expression of carnitine palmitoyl transferase 1A). Mechanistically, the therapeutic effects of BHF on POI were linked with alleviation of lipid deposition-induced reactive oxygen species (ROS) accumulation and excessive autophagy, corroborating the results in PA-challenged GCs. After treatment with elesclomol (a ROS inducer) and rapamycin (an autophagy inducer) in GCs, the effects of BHF were almost counteracted under model conditions.
Conclusion: These findings suggest that BHF alleviates the symptoms of POI by altering lipid metabolism and reducing lipid accumulation-induced ROS and autophagy, offering evidence for BHF’s efficacy in treating POI clinically.
1 Introduction
Premature ovarian insufficiency (POI) is characterized by a decline in ovarian function before the age of 40, affecting approximately 3.7% of women of reproductive age globally (Golezar et al., 2019). Diagnosis is based on clinical features including menstrual irregularities or amenorrhea, elevated gonadotrophins (FSH level > 25 IU/L on two separate tests at least 4 weeks apart), and reduced estradiol (E2) levels (Ishizuka, 2021). Studies have shown that the natural pregnancy rate in women with POI is below 5%, making it a significant factor in infertility among women of reproductive age (Bidet et al., 2011). Furthermore, POI is associated with increased risks of neurological dysfunction, type 2 diabetes, cardiovascular diseases, osteoporosis, and reduced life expectancy (Tucker et al., 2016).
Current evidence suggests that POI etiologies are linked to environmental factors, iatrogenic injuries, genetic defects, autoimmune dysfunctions, and metabolic abnormalities (Huhtaniemi et al., 2018; Jiao et al., 2021; Wang, et al., 2020b). These complex exogenous and endogenous factors contribute to abnormal follicle activation, hindered recruitment of dominant follicles, impeded maturation, and increased follicular atresia, ultimately leading to POI (Li Z. et al., 2021). Apoptosis in granulosa cells (GCs) can initiate follicle atresia and lead to oocyte loss, which is a critical factor in POI development (Li D. et al., 2021). Prior research on GC apoptosis has focused on reactive oxygen species (ROS) generation, cytokine, and hormone disruptions (Matsuda et al., 2012). However, recent studies show that imbalances in lipogenesis and lipolysis, causing lipid deposition and subsequent lipotoxicity and ROS generation, are key in GCs apoptosis (Shaoyong et al., 2022). In POI patients, triglyceride (TG) and free fatty acid (FFA) levels are significantly elevated in follicular fluid compared to healthy individuals (Huang et al., 2022; Wang L. et al., 2020). High FFA levels in follicular fluid have been shown to suppress GCs proliferation, as observed in dairy cows (Wang Y. et al., 2020). Additionally, FFAs induce apoptosis in GCs (human primary GCs and human KGN cells) in a dose-dependent manner (Baddela et al., 2020) and elevate ROS content (Ma et al., 2022), which inhibits proliferation and promotes senescence in GCs by triggering autophagy (Sun et al., 2021). Thus, reducing lipid deposition emerges as a potential therapeutic approach for POI.
While hormone replacement therapy (HRT) is commonly used for treating POI in clinical settings, it often results in limited clinical effectiveness or adverse side effects (Webber et al., 2017). HRT is not reported to aid in follicular development or reverse ovarian function decline (Li et al., 2020). Recent studies have shown that Traditional Chinese Medicines may delay ovarian failure and have a high safety profile in POI treatment (Li et al., 2020). Bushen Huoxue formula (BHF), a traditional clinical prescription, is widely used in China for ovarian failure-related diseases (Zhongwei et al., 2019). Our previous study indicated that BHF significantly enhanced ovarian function and restored triglyceride homeostasis in psychological stress-induced POI rats (Miao et al., 2020). Interestingly, BHF also showed efficacy in regulating lipid metabolism and reducing ROS in diabetic retinopathy rats and nucleus pulposus cells (Gao et al., 2022; Wang et al., 2020c; Li, et al., 2020). However, the specific role of BHF in treating POI by improving lipid levels remains unclear.
In this context, we hypothesize that BHF treatment in POI may rectify impaired lipid deposition, thereby reducing ROS and autophagy. To explore this, we employed FFA-stimulated GCs and corticosterone-induced rats to investigate BHF therapeutic effectiveness and the underlying mechanisms in POI treatment.
2 Materials and methods
2.1 Drugs and reagents
BHF consists of eleven medicinal herbs (Table 1) which were purchased from Nanjing Lishui District Hospital of Traditional Chinese Medicine (Nanjing, China). Progynova was provided by DELPHARM Lille S.A.S. Corticosterone was purchased from Shanghai Aladdin Bio-Chem Technology Co., Ltd. (Shanghai, China). Estradiol (E2) and follicle-stimulating hormone (FSH) ELISA kits were obtained from the Elabscience Biotechnology Co., Ltd. (Wuhan, China). Corticosterone, Luteinizing Hormone (LH), and anti-Müllerian hormone (AMH) ELISA kits were from the Wuhan Xinqidi Biotech Co., Ltd. (Wuhan, China). BCA protein assay kits were bought from Beyotime (Shanghai, China). Primary antibodies against PPARγ, c/EBPα, ATGL, and CPT1A were supplied by Proteintech (Chicago, United States). LC3B and p62 were purchased by ABclonal (Wuhan, China).
2.2 Preparation and qualitative analysis of BHF
BHF was prepared using a previously established method. Initially, In our experiment, all herbs were soaked in distilled water at ten times their volume (v/m) for 30 min at 24°C, followed by decoction for an additional 30 min at 100°C. The mixture was then filtered through gauze. The herb residue was re-extracted under identical conditions. The two filtrates were consolidated and concentrated under depressurized conditions, followed by lyophilization to powder for qualitative analysis and subsequent experiments.
For the analysis, 500 mg of BHF powder was immersed in 1 mL of 70% methanol and ground for 3 min using an automatic sample rapid grinder (jxfstprp-48, 70 Hz). The solution was then centrifuged at 12,000 rpm for 10 min at 4°C and filtered through a 0.22 μm PTFE filter. UPLC-MS/MS was used for analysis. UPLC was performed on a Thermo Vanquish UHPLC using a Zorbax Eclipse C18 (1.8 μm × 2.1 mm × 100 mm) column at 30°C, with pure acetonitrile as solvent A and 0.1% formic acid as solvent B. The gradient elution program was as follows: 5% A at 0–2 min, 5%–30% A at 2–6 min, 30% A at 6–7 min, 30%–78% A at 7–12 min, 78% A at 12–14 min, 78%–95% A at 14–17 min, 95% A at 17–20 min, 95%–5% A at 20–21 min, and 5% A at 21–25 min. The flow rate was set at 0.3 mL/min, with an injection volume of 2 μL. Mass spectrometry was conducted on a Q-Exactive HF from Thermo Fisher in both positive and negative ionization modes. The parameters were: heater temperature at 325°C; sheath gas flow at 45 arb (arbitrary units); electrospray voltage at 3.5 KV; capillary temperature at 330°C; S-Lens RF level at 55%; full scan (m/z 100–1,500) and data-dependent MS2 (TopN = 10); resolutions at 120,000 (MS1) and 60,000 (MS2).
2.3 Animals and experimental design
Fifty female Sprague-Dawley (SD) rats were sourced from Nanjing Kaisijia Biotechnology Co., Ltd. They were housed at the Animal Center of the Jiangsu Province Institute of Traditional Chinese Medicine under standard conditions (23°C ± 2°C temperature, 50% ± 5% humidity, and a 12-h light/dark cycle) for 7 days. All animal experimental procedures were in strict compliance with European community guidelines and approved by the Ethics Committee of Jiangsu Province Institute of Traditional Chinese Medicine (Permission No. AEWC-20220615-214).
In this research, we utilized corticosterone to establish a POI model (Miao et al., 2020). The rats were randomly divided into five groups (n = 10/group): normal control (NC), model control (MC), Progynova treatment (PT) as the positive control, and two BHF treatment groups with low (L-BHF) and high (H-BHF) dosages. The rats of NC received subcutaneous saline injections, while the rest were administered corticosterone (40 mg/kg, w/w) for 3 weeks to induce POI. Since the first day of modeling, rats were given drugs administration at a volume of 10 mL/kg. The NC and MC groups were received saline plus 0.5% carboxymethylcellulose, while rats in the PT and BHF treatment groups were orally administered PT (10 mg/mL) or BHF (L-BHF: 171.5 mg/mL of lyophilized powder, H-BHF: 686 mg/mL of lyophilized powder) once daily for 3 weeks. The BHF dosage was selected based on prior research (Miao et al., 2020). And all subsequent experiments were only carried out after the treatment had been completed.
2.4 Assessment of ovarian function
Body weight was monitored weekly during the experiment. At its conclusion, tissue and blood samples were collected for analysis. Serum levels of corticosterone, FSH, LH, AMH and E2 were measured using ELISA kits per the manufacturer’s instructions. Ovaries were rapidly excised, washed with PBS, and weighed to calculate the ovarian index (ovarian weight/body weight). Sections of the ovaries were fixed in 4% paraformaldehyde for a minimum of 24 h, embedded in paraffin, sectioned into 5 μm slices, and stained with Hematoxylin and Eosin (H&E) to assess follicular changes.
2.5 Cells culture and viability assay
The human ovarian granulosa cell line (KGN cells) was obtained from EK Bioscience (Shanghai, China) and cultured in DMEM/F-12 medium (Jiangsu KeyGEN BioTECH Corp., Ltd., China) with 10% FBS (Gibco, United Kingdom) in a 37°C, 5% CO2 incubator (Thermo Fisher Scientific, Waltham, MA, United States). KGN cells in the logarithmic growth phase were plated in 96-well plates at a density of 8 × 103 cells/well for 12 h. The cells were then incubated with varying concentrations of palmitic acid (PA, 50, 100, 200 μM), BHF (200, 400 μg/mL), or a combination of PA and BHF for 24 h. Subsequently, 10 μL MTT solution was added to each well and incubated at 37°C for 4 h. After discarding the medium, 100 μL DMSO was added to each well. Cell viability was determined at 490 nm using a microplate reader (SpectraMax® i3x, Molecular Devices, United States) and calculated as follows: cell viability (%) = [OD 490 (sample)/OD 490 (control)] × 100%.
2.6 E2 Enzyme-linked immunosorbent assay
KGN cells were seeded in 24-well plates at a density of 3 × 104 cells/well for 12 h, followed by treatment with PA, with or without BHF (200, 400 μg/mL), under FSH (50 ng/mL) stimulation for 24 h. Culture supernatants were then collected, and E2 concentrations were measured using the E2 Human ELISA Kit according to the manufacturer’s instructions. E2 levels were determined based on standard curves.
2.7 Oil red O and Nile Red staining
Ovarian tissues were fixed in 4% paraformaldehyde, embedded in Tissue-Tek OCT, and sectioned into 5-μm-thick slices for Oil Red O staining. Neutral lipids were visualized and imaged using an inverted microscope (Leica DM 11, Germany).
For Nile Red staining of KGN, cells were seeded into 6-well plates and treated with PA (50, 100, 200, 400 μM) or PA (200 μM) combined with or without BHF (200, 400 μg/mL) for 24 h. Post-treatment, cells were washed with PBS, fixed with 4% paraformaldehyde for 10 min, and stained using a Nile Red staining kit. After being rinsed twice with PBS, lipid droplets were visualized and photographed as mentioned above.
2.8 Immunohistochemistry
Immunohistochemistry was performed on paraffin-embedded sections using the avidin-biotin complex method (Zhao et al., 2021). Slides were incubated overnight at 4°C with primary antibodies against PCNA. Subsequently, biotinylated anti-rabbit IgG secondary antibodies and DAPI (Beyotime, China) were applied in sequence, with protection from light. Images were captured using a light microscope (Leica DM 11, Germany) and analyzed with Image-Pro Plus 6.0 software (Media Cybernetics, Maryland, United States).
2.9 Immunofluorescence
For immunofluorescence, sections from paraffin-embedded tissues were stained, followed by TUNEL and ROS staining according to standard protocols. GC cells were also fixed and stained with TUNEL and ROS. Images were acquired using a Nikon TS2R-FL fluorescent inverted microscope and quantified with Image-Pro Plus 6.0 software (Media Cybernetics, Maryland, United States).
2.10 Immunoblot analysis
Ovarian tissue and KGN cells were lysed using RIPA buffer supplemented with protease and phosphatase inhibitors. Protein concentrations were quantified using a BCA kit, and equal amounts of protein (30 μg/lane) were separated by SDS-PAGE, then transferred onto nitrocellulose filters. The membranes were blocked with 5% skimmed milk for 2 h and incubated with specific primary antibodies overnight at 4°C. After washing with TBST, membranes were incubated with horseradish peroxidase-conjugated secondary antibodies. Protein bands were visualized using ChemiScope S6 (Clinx Science Instruments Co., Ltd., Shanghai, China) and analyzed using ImageJ software. The primary and secondary antibodies used were: anti-PPARγ (Mouse, Proteintech, 1:1,000), anti-c/EBPα (Rabbit, Proteintech, 1:1,000), anti-ATGL (Rabbit, Proteintech, 1:1,000), anti-CPT1 (Mouse, Proteintech, 1:1,000), anti-LC3B (Rabbit, ABclonal, 1:1,000), anti-p62 (Rabbit, ABclonal, 1:1,000), anti-GAPDH (Mouse, Proteintech, 1:2,000), and anti-β-actin (Rabbit, Beyotime, 1:2,000).
2.11 Statistical analyses
Data were presented as mean ± SEM. Statistical analyses were conducted using Prism 8.4.0 (GraphPad Software, Inc., United States). Normality and homogeneity of variance tests were respectively performed with Shapiro-Wilk and Brown-Forsythe tests before proceeding with the statistical analysis (both p > 0.05 in the present study. A One-Way ANOVA with a subsequent LSD test was applied for multiple group comparisons, or an independent samples t-test for comparisons between two groups. A p-value < 0.05 was considered statistically significant.
3 Results
3.1 Chemical components analysis of BHF
UPLC-MS/MS was utilized to identify the chemical components of BHF extract. The total ion chromatograms (TIC) of BHF are depicted in Figures 1A, B. Using Compound Discoverer 3.3, along with mzCloud and mzVault databases, 350 ingredients were identified in BHF. The top 23 compounds are enumerated in Table 2.
3.2 BHF alleviated ovarian function in POI rats
The therapeutic effects of BHF on POI were assessed using 8-week-old female Sprague-Dawley rats, subjected to BHF for 3 weeks under CORT-induced conditions (Figure 2A). Figure 2B shows that the body weights in the MC group decreased significantly compared to the NC group, whereas BHF treatment notably increased body weight at the end of the experiment. Serum corticosterone levels (Figure 2C), indicative of stress response, were significantly higher in the MC group than in the NC group. However, BHF administration reduced these changes. Ovarian function assessments revealed that ovary index, E2, AMH, and LH levels were considerably lower in the MC group compared to the NC group. BHF intervention notably elevated these parameters (Figures 2D–G). BHF treatment also reversed elevated FSH levels in POI rats (Figure 2H). Ovarian morphology, evaluated by H&E staining (Figure 2I), showed that MC rats had an increased number of atretic follicles, while BHF treatment significantly reduced them (Figure 2M). We found that the numbers of primary, preantral, and antral follicles were also exhibited a trend of increasing in BHF-treated POI rats, although there were no significant differences (Figures 2J–L). These findings suggest that BHF restored hormone synthesis and reduced excessive follicular atresia, thereby maintaining ovarian function.
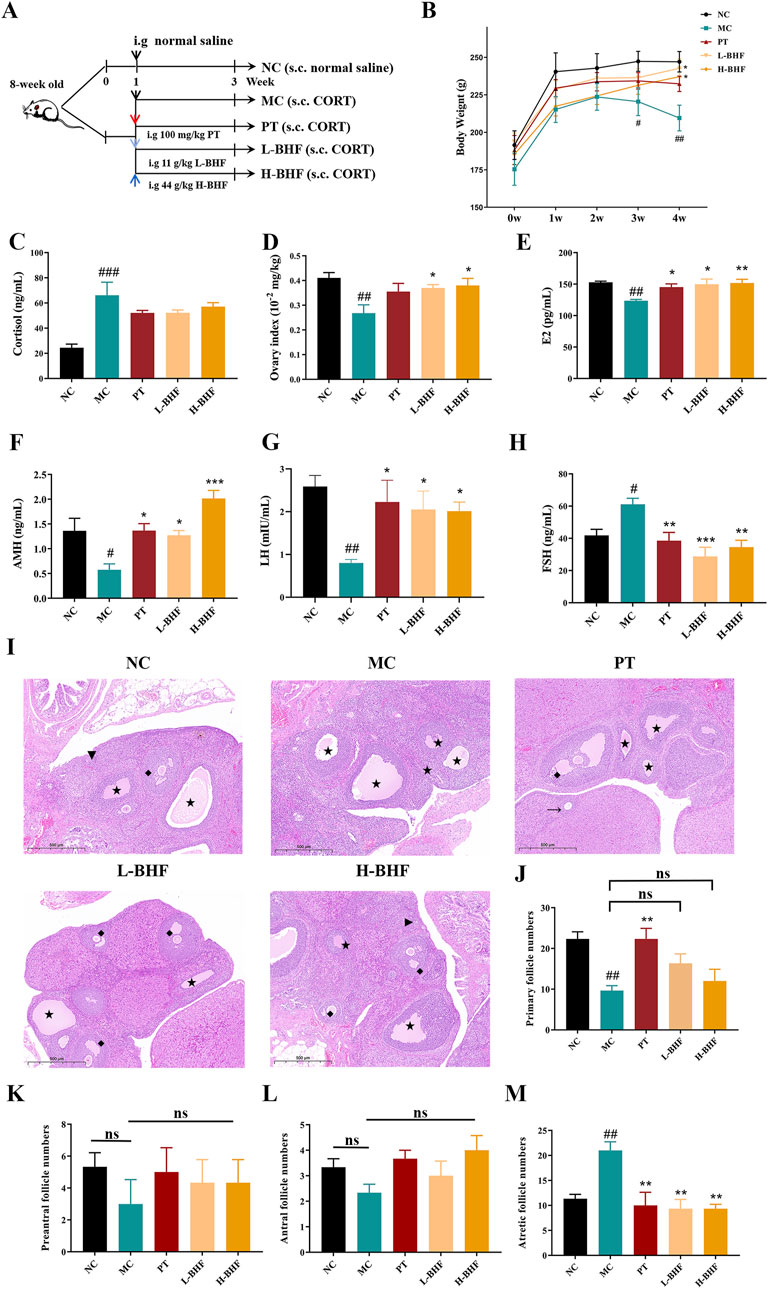
Figure 2. BHF’s impact on body weight and ovarian function in CORT-induced POI rats: (A) Study design workflow. (B) Body weight over the weeks. (C) Serum cortisol levels. (D) Ovarian index (ovarian weight/body weight). (E–H) Serum levels of E2, AMH, LH, and FSH. (I) H&E staining ovarian micrographs. (J–M) Numbers of primary follicles (Triangle), preantral follicles (arrow), antral follicles (rhomboid), and atretic follicles (Five-pointed star). Data are expressed as mean ± SEM. #p < 0.05, ##p < 0.01, and ###p < 0.001 vs. the NC group. *p < 0.05, **p < 0.01, and ***p < 0.001 vs the MC group. NC, normal control. MC, model control. PT, positive control. L-BHF, BHF treatment with low dosage. H-BHF, BHF treatment with high dosage.
3.3 BHF alleviated proliferation inhibition and apoptosis in rat ovary tissues
Proliferation and apoptosis in follicles were evaluated using PCNA and TUNEL staining, respectively. Figure 3 illustrates that the proportion of PCNA-positive areas in follicles was significantly reduced in the MC group compared to the NC group, but markedly increased following BHF treatment. Conversely, TUNEL staining revealed increased apoptosis in the MC group relative to the NC group. BHF intervention notably lessened this effect. These findings indicate that BHF effectively reduced both proliferation inhibition and apoptosis in GCs within follicles.
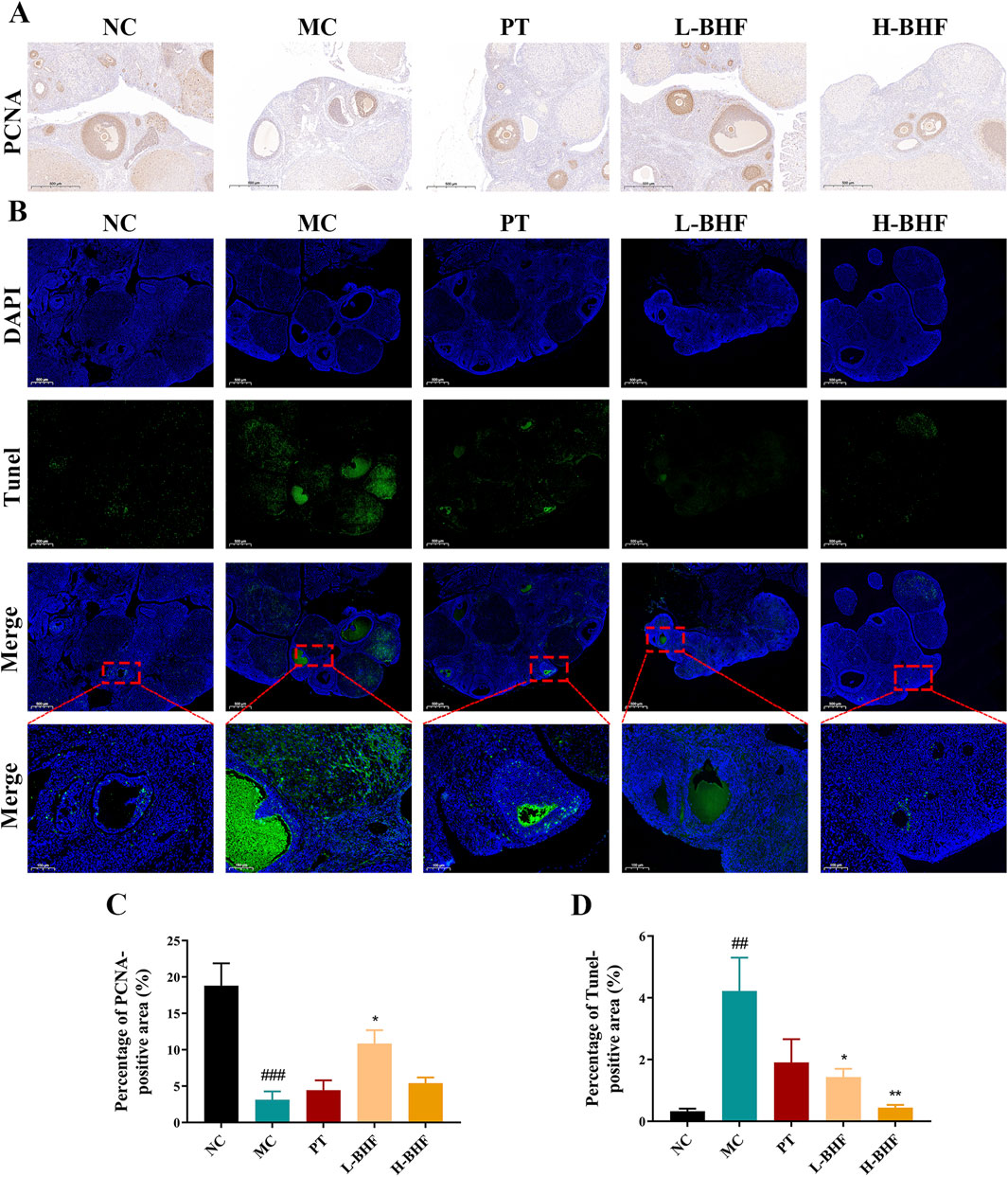
Figure 3. Proliferation and apoptosis in rat ovarian sections using PCNA and TUNEL staining across all groups. (A) Immunohistochemistry staining of PCNA (magnification, ×100). (B) Immunofluorescence staining of TUNEL (magnification, ×100). (C, D) Quantitative analysis of positive areas in the ovaries. Data were expressed as mean ± SEM. ##p < 0.01 and ###p < 0.001 vs the NC group. *p < 0.05 and **p < 0.01 vs the MC group. NC, normal control. MC, model control. PT, positive control. L-BHF, BHF treatment with low dosage. H-BHF, BHF treatment with high dosage.
3.4 BHF modulated lipid metabolism, alleviated ROS and excessive autophagy in POI rats
To assess the impact of BHF on lipid accumulation, ROS, and autophagy, ovarian lipid deposition levels were first examined using Oil Red O staining (Figure 4A). BHF treatment significantly decreased lipid accumulation in the ovaries of POI rats. Further investigation into lipid metabolism-related proteins (Figures 4B–F) showed that BHF therapy reduced lipogenesis protein expression (PPARγ and C/EBPα), increased lipolysis protein levels (ATGL), and boosted fatty acid β-oxidation protein expression (CPT1A). ROS levels and autophagy status in the ovaries were also evaluated post-BHF treatment. Results indicated that ROS levels and LC3II expression were substantially higher in the MC group compared to the NC group, while significantly reduced after BHF treatment. In contrast, P62 expression, markedly decreased in the MC group, was restored with BHF administration (Figures 4G–H). Overall, these data demonstrate that BHF treatment reduced lipid accumulation, ROS production, and excessive autophagy.
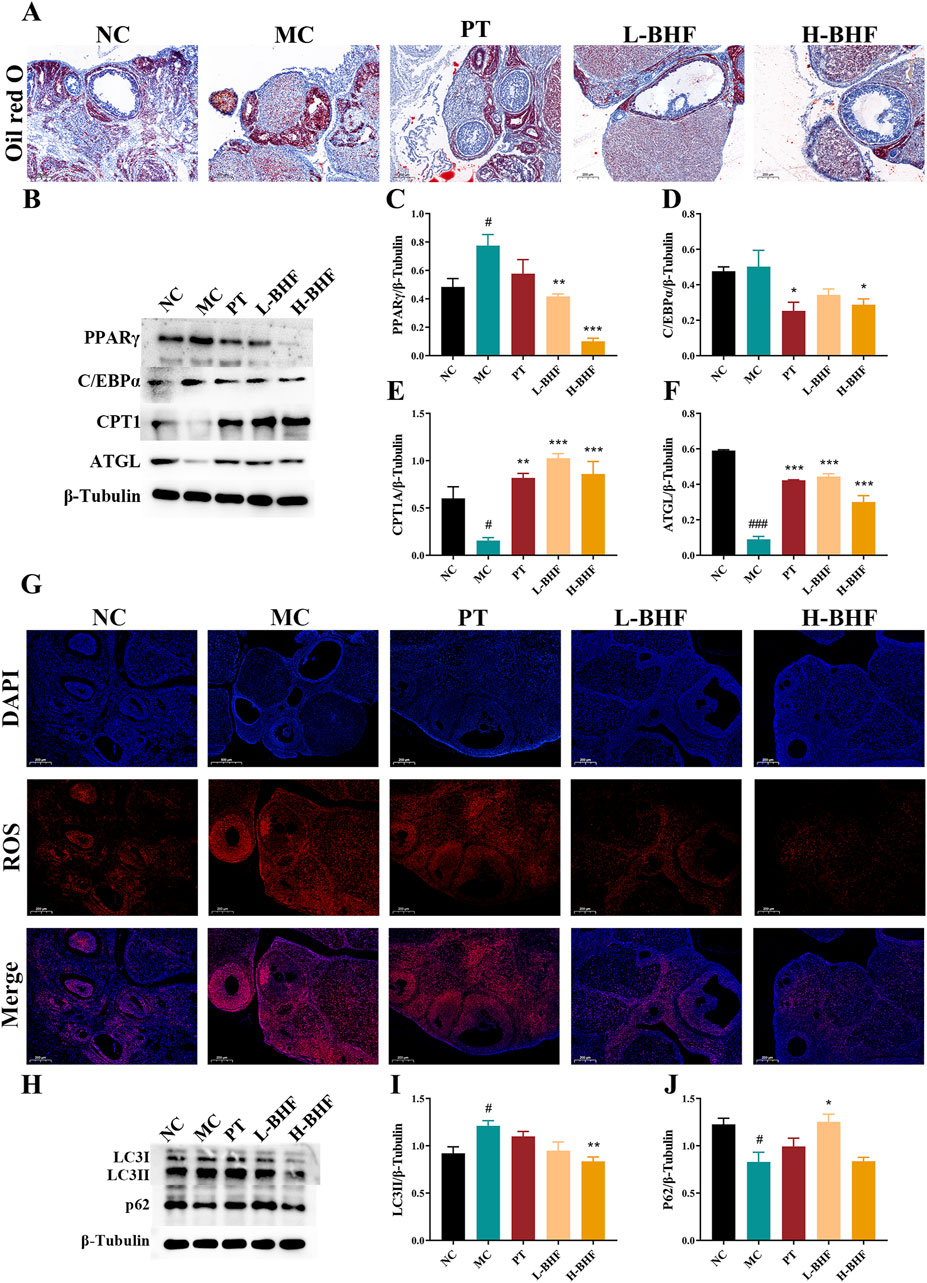
Figure 4. BHF improved lipid metabolism, ROS and autophagy in POI rats (A) Oil Red O staining (magnification ×100). (B) Expression levels of PPARγ, c/EBPα, CPT1A and ATGL. (C–F) Semi-quantitative analysis of PPARγ, c/EBPα, CPT1A and ATGL. (G) ROS staining (magnification ×100). (H) Expression levels of LC3 and P62. (I, J) Semi-quantitative analysis of LC3 and P62. Data were expressed as mean ± SEM. #p < 0.05 and ###p < 0.001 vs the NC group. *p < 0.05, **p < 0.01 and ***p < 0.001 vs the MC group. NC, normal control. MC, model control. PT, positive control. L-BHF, BHF treatment with low dosage. H-BHF, BHF treatment with high dosage.
3.5 BHF mitigated PA-induced KGN cells injury via regulating lipid metabolism
To understand how BHF diminishes the injury of GCs, a lipid-loading KGN cell model was established using PA. Figure 5A demonstrates that 24-h incubation with PA (50, 100, 200, and 400 μM) significantly decreased cell viability in KGN cells in a dose-dependent manner. Consequently, PA at 200 μM for 24 h was selected for subsequent experiments. BHF cytotoxicity was then evaluated in KGN cells. Results indicated that up to 400 μg/mL of BHF did not exhibit cytotoxic effects (Figure 5B). The impact of BHF at 200 and 400 μg/mL on PA (200 μM) stimulated KGN cells was further examined. Figures 5C–E show that BHF treatment not only significantly improved cell viability and E2 levels but also notably reduced apoptosis in PA-induced KGN cells. Additional analysis revealed that BHF decreased PA-induced lipid accumulation (Figure 5F). PA treatment increased PPARγ and C/EBPα expression, which was negated following BHF treatment. Expectedly, ATGL and CPT1A expression markedly decreased with PA treatment but were substantially reversed upon co-incubation with BHF (Figures 5G–K). Collectively, these findings indicate that BHF protects GCs by regulating lipid metabolism, thereby reducing lipid accumulation.
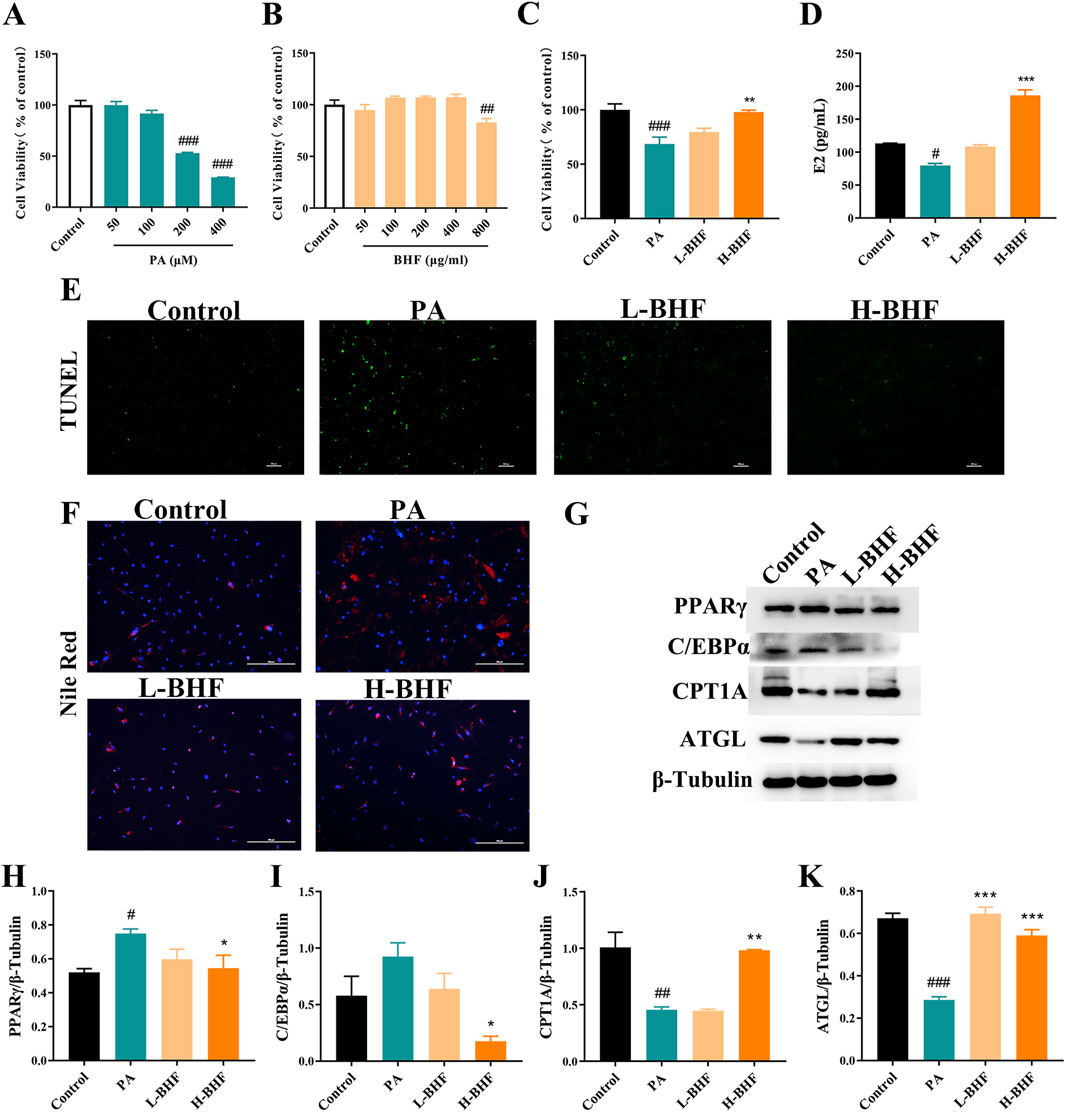
Figure 5. BHF improved lipid metabolism, ROS and autophagy in KGN cells. (A–C) Cell viability assays. (D) E2 levels assays. (E, F) TUNEL and Nile Red staining (magnification ×100). (G) Expression levels of PPARγ, c/EBPα, CPT1A and ATGL. (H–K) Semi-quantitative analysis of PPARγ, c/EBPα, CPT1A and ATGL. Data were expressed as mean ± SEM. #p < 0.05 and ###p < 0.001 vs the NC group. *p < 0.05, **p < 0.01 and ***p < 0.001 vs the MC group.
3.6 BHF inhibited ROS accumulation and autophagy in PA-induced KGN cells
The effect of BHF on ROS and autophagy was evaluated in PA-stimulated KGN cells. Figures 6A–C shows that 24-h BHF treatment significantly reduced ROS levels and LC3II expression. The same treatment also increased p62 expression in PA-stimulated KGN cells (Figures 6B, D). Our data demonstrated that BHF was most effective at a concentration of 400 μg/mL, which was used for further experiments. The effects of BHF on E2 levels, apoptosis, ROS, and autophagy were partially negated by treatment with elesclomol (a ROS inducer) and rapamycin (an autophagy inducer). Co-incubation with elesclomol and rapamycin almost entirely counteracted the effects of BHF under model conditions (Figures 6E–I). In conclusion, BHF was found to inhibit cell apoptosis and enhance E2 secretion by reducing ROS accumulation and hyperactivation of autophagy.
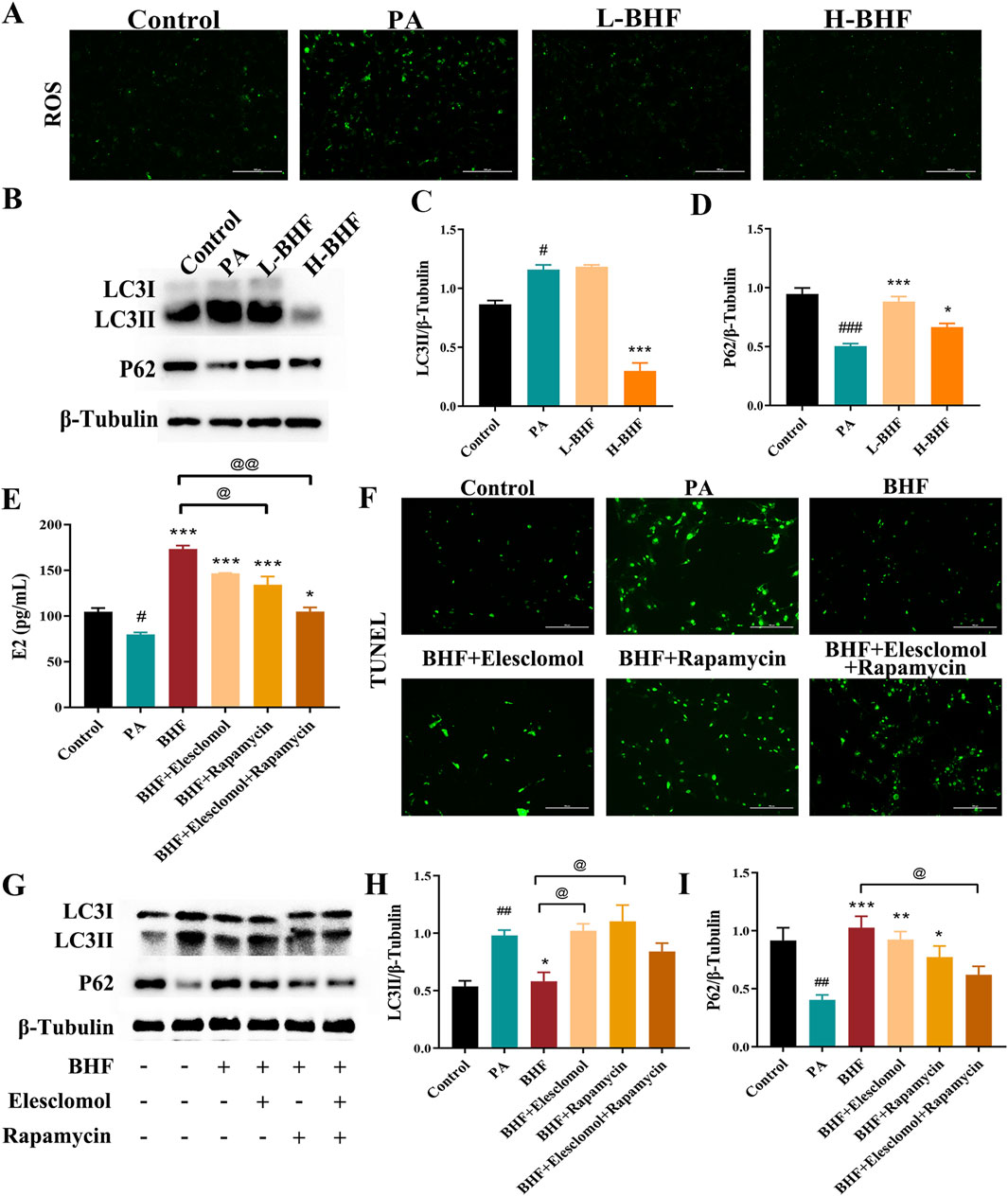
Figure 6. BHF inhibited ROS and autophagy in KGN cells. (A) ROS staining (magnification ×100). (B) Expression levels of LC3 and P62. (C, D) Semi-quantitative analysis of LC3 and P62. (E) E2 levels assays. (F) TUNEL staining (magnification ×100). (G) LC3 and P62 expression levels with and without elesclomol or rapamycin addition. (H, I) Semi-quantitative analysis of LC3 and P62 with and without elesclomol or rapamycin addition. Data were expressed as mean ± SEM. #p < 0.05 and ###p < 0.001 vs the NC group. *p < 0.05 and ***p < 0.001 vs the MC group. @p < 0.05 and @@p < 0.05 vs the BHF group.
4 Discussion
POI, a major factor contributing to infertility, significantly affects the quality of life in women. Currently, there are no specific, clinically proven treatments for POI. However, recent studies suggest that Traditional Chinese Medicines offer promising benefits in managing POI (Xiu et al., 2023; Zhou et al., 2021). BHF, a formula based on clinical experience, has shown protective effects against POI (Miao et al., 2020). Yet, its underlying mechanism remains to be fully understood. In this study, we found that BHF effectively countered hormonal imbalances (E2, LH, FSH, and AMH) and reduced follicular depletion in POI rats. Both in vivo and in vitro experiments indicated that BHF reduced proliferation inhibition and apoptosis in GCs by decreasing lipid deposition-induced ROS accumulation and autophagy.
BHF comprises eleven traditional Chinese medicinal herbs and has complex chemical constituents. This research identified the top 23 compounds in BHF (Table 2) with pharmacological activities like lipid-lowering, insulin sensitization, antithrombotic, anti-inflammatory, and antioxidant effects (Jang et al., 2017; Ma et al., 2017; Zhang et al., 2014). Notably, paeoniflorin, isomangiferin, sucrose, icariin, benzoylpaeoniflorin, neomangiferin, and chlorogenic acid are known lipid metabolism regulators (Bi et al., 2023; Geidl-Flueck et al., 2021; Ma et al., 2017; Shao et al., 2021; Xu et al., 2021; Zhou et al., 2015). Our previous proteomic screening indicated that differentially expressed proteins in BHF-treated POI rats primarily involved triglyceride homeostasis and cholesterol metabolism (Miao et al., 2020). Recent studies have also highlighted that cholesteryl ester and triacylglycerol are key metabolites in diminished ovarian reserve rats treated with BHF (Zeng et al., 2023). Therefore, BHF’s efficacy in alleviating POI may be attributed to its lipid-regulating properties.
Lipids are recognized as crucial for ovarian function maintenance. Research indicates that lipids not only provide energy to oocytes but also serve as precursors for steroid hormones synthesized in granulosa and theca cells (Liu et al., 2022). However, lipid accumulation can cause significant ovarian damage. Previous studies have demonstrated that excessive adipose tissue accelerates ovarian follicle loss in rats, leading to premature ovarian failure (Nteeba et al., 2014). Additionally, FFA accumulation in ovaries correlates with oocyte mitochondrial dysregulation and apoptosis in cumulus-oocyte complexes, contributing to ovarian function depletion (Wu et al., 2022). Lipid-lowering agents, such as rosuvastatin, curcumin, and resveratrol, have shown protective effects on ovarian function in POI models (Elkady et al., 2019; Said et al., 2016; Yan et al., 2018). In this study, lipid deposition in POI rat ovaries and PA-induced lipid accumulation leading to reduced E2 secretion and increased apoptosis in GCs were observed. These effects were reversed with BHF treatment, suggesting that the efficacy of BHF against POI is linked to its lipid accumulation reduction. Further, BHF regulated lipid metabolism by inhibiting lipid synthesis (PPARγ and c/EBPα expression), enhancing lipid catabolism (ATGL expression), and boosting lipid oxidation (CPT1A expression). Thus, BHF treatment in POI might be related to reprogramming lipid metabolism to alleviate lipid accumulation. Notably, premature menopause, especially in POI patients, increases cardiovascular risk factors such as weight gain, visceral adiposity, and lipid abnormalities (van Lennep et al., 2016; van Lennep et al., 2023). The 2019 ACC/AHA guideline also considers premature menopause (before age 40 years) a risk-enhancing factor for atherosclerotic cardiovascular disease, recommending statin treatment for women at borderline or intermediate risk (Arnett et al., 2019). Therefore, combining effective POI treatments with lipid-lowering drugs may be a novel strategy for future POI management.
Moreover, disrupted lipid metabolism leads to elevated ROS levels, implicated in conditions like fatty liver disease and colon cancer (Abulikemu et al., 2023; Shi et al., 2022). Previous studies have established that excessive ROS can cause oxidative damage, triggering atresia in follicles and eventually resulting in premature ovarian failure (Agarwal et al., 2012). Our data indicate that BHF treatments significantly reduced ROS accumulation in corticosterone-induced rats and PA-challenged GCs. The use of elesclomol, a ROS inducer, partially reversed the effects of BHF in PA-challenged GCs. These findings suggest that BHF improves POI by reducing lipid-induced ROS accumulation.
Autophagy is crucial for maintaining cellular homeostasis by eliminating misfolded proteins or defective organelles. However, its hyperactivation can lead to the extensive degradation of vital cell components. There is substantial evidence that excessive autophagy induces apoptosis in GCs (Zhou et al., 2019). Duerrschmidt N et al. reported that low-density lipoprotein-induced autophagic death in GCs contributes to follicular atresia (Duerrschmidt et al., 2006). Furthermore, chronic stress is known to accelerate GC senescence, which is linked to ROS-induced autophagy (Sun et al., 2021). Current therapeutic approaches, such as melatonin, hyperoside, and human umbilical cord-derived mesenchymal stem cells, aim to reduce autophagy to improve POI (Dai et al., 2023; Xie et al., 2021; Zhu et al., 2022). Our findings show that BHF reduced the accumulation of LC3II and the decrease in P62 in CORT-induced rats and PA-challenged GCs, thereby inhibiting excessive autophagy. Co-treatment with rapamycin, an autophagy inducer, partially nullified BHF’s anti-apoptotic and E2 enhancing effects in PA-induced GCs. Notably, the effects of BHF were almost negated under co-incubation with rapamycin and elesclomol in PA-induced GCs. These results suggest that BHF attenuates lipid-induced autophagy, thereby boosting E2 secretion. Conversely, inadequate autophagy can impair GC differentiation and E2 synthesis (Shao et al., 2022). Given that autophagy has varied effects on cell proliferation or apoptosis depending on cellular conditions (Mazure and Pouyssegur, 2010), we speculate that the observed differences could be due to the status of GCs arising from the various model establishment methods.
5 Conclusion
In summary, our study has shown that BHF has beneficial effects on POI rats by enhancing hormonal balance and reducing follicular depletion. Further analysis indicated that the mechanisms of BHF are closely related to its ability to reduce lipid accumulation-induced ROS and autophagy in ovaries and GCs by altering lipid metabolism. These findings suggest that modulation of lipid metabolism could be a promising therapeutic approach for the treatment of POI. Notwithstanding, our study also exists some limitations. Firstly, the chemical components of BHF were identified in this paper. However, the active ingredients should be screened based on the curative effects of improving POI. Secondly, this experiment only provided preliminary detection of the representative proteins in lipid metabolism and did not carry out a full screening. Finally, this experiment did not probe the upstream regulators of lipid metabolism in the treatment POI. Therefore, the pathways and critical targets of BHF modulation lipid metabolism in POI will be the subject of a future study.
Data availability statement
The original contributions presented in the study are included in the article/supplementary material, further inquiries can be directed to the corresponding authors.
Ethics statement
The animal studies were approved by Jiangsu Province Institute of Traditional Chinese Medicine (Permission No. AEWC-20220615-214). The studies were conducted in accordance with the local legislation and institutional requirements. Written informed consent was obtained from the owners for the participation of their animals in this study.
Author contributions
TL: Data curation, Formal Analysis, Investigation, Methodology, Software, Writing–original draft. YW: Investigation, Writing–review and editing. BJ: Methodology, Writing–review and editing. RH: Formal Analysis, Methodology, Writing–original draft. BZ: Data curation, Formal Analysis, Writing–original draft. XB: Methodology, Writing–review and editing. PW: Conceptualization, Resources, Writing–review and editing. YZ: Conceptualization, Writing–original draft. XS: Conceptualization, Funding acquisition, Resources, Writing–original draft. JZ: Conceptualization, Resources, Supervision, Writing–review and editing.
Funding
The author(s) declare that financial support was received for the research, authorship, and/or publication of this article. This research was funded by the National Natural Science Foundation of China (82405468), Development Plan Project of Jiangsu Province Traditional Chinese Medicine Science and Technology (MS2022056), the Development Special Fund Project Plan of Nanjing Health Science and Technology (YKK22243), Nanjing Pharmaceutical Association-Hospital pharmacy Scientific Research Fund Project of Changzhou Siyao (2021YX018), and the Scientific Project of the Nanjing Lishui District Hospital of Traditional Chinese Medicine (LZY202210), Jiangsu Health Vocational College’s Special Research Project on “Medical Education Collaboration and Chinese Western Integration” (YJXTL202301), the Postgraduate Research and Practice Innovation Program of Jiangsu Province (SJCX23_0771), and the Science and Technology Program for Traditional Chinese Medicine of Zhejiang Province (2023ZL639).
Conflict of interest
The authors declare that the research was conducted in the absence of any commercial or financial relationships that could be construed as a potential conflict of interest.
Publisher’s note
All claims expressed in this article are solely those of the authors and do not necessarily represent those of their affiliated organizations, or those of the publisher, the editors and the reviewers. Any product that may be evaluated in this article, or claim that may be made by its manufacturer, is not guaranteed or endorsed by the publisher.
Abbreviations
BHF, Bushen Huoxue formula; POI, premature ovarian insufficiency; PA, palmitic acid; FSH, follicle-stimulating hormone; AMH, anti-Müllerian hormone; E2, estradiol; LH, luteinizing hormone; GCs, granulosa cells; ROS, reactive oxygen species; TG, triglyceride; FFA, free fatty acid; HRT, hormone replacement therapy.
References
Abulikemu, A., Zhao, X., Xu, H., Li, Y., Ma, R., Yao, Q., et al. (2023). Silica nanoparticles aggravated the metabolic associated fatty liver disease through disturbed amino acid and lipid metabolisms-mediated oxidative stress. Redox Biol. 59, 102569. doi:10.1016/j.redox.2022.102569
Agarwal, A., Aponte-Mellado, A., Premkumar, B. J., Shaman, A., and Gupta, S. (2012). The effects of oxidative stress on female reproduction: a review. Reprod. Biol. Endocrinol. 10, 49. doi:10.1186/1477-7827-10-49
Arnett, D. K., Blumenthal, R. S., Albert, M. A., Buroker, A. B., Goldberger, Z. D., Hahn, E. J., et al. (2019). 2019 ACC/AHA guideline on the primary prevention of cardiovascular disease: a report of the American College of cardiology/American heart association task force on clinical Practice guidelines. Circulation 140 (11), e596–e646. doi:10.1161/CIR.0000000000000678
Baddela, V. S., Sharma, A., and Vanselow, J. (2020). Non-esterified fatty acids in the ovary: friends or foes? Reprod. Biol. Endocrinol. 18 (1), 60. doi:10.1186/s12958-020-00617-9
Bi, Y., Wang, X., Han, L., Tian, Y., Bo, T., Li, C., et al. (2023). Selective inhibition of organic cation transporter 1 by benzoylpaeoniflorin attenuates hepatic lipid accumulation through AMPK activation. J. Nat. Prod. 86 (1), 191–198. doi:10.1021/acs.jnatprod.2c00927
Bidet, M., Bachelot, A., Bissauge, E., Golmard, J. L., Gricourt, S., Dulon, J., et al. (2011). Resumption of ovarian function and pregnancies in 358 patients with premature ovarian failure. J. Clin. Endocrinol. Metab. 96 (12), 3864–3872. doi:10.1210/jc.2011-1038
Dai, W., Yang, H., Xu, B., He, T., Liu, L., Ma, X., et al. (2023). Human umbilical cord-derived mesenchymal stem cells (hUC-MSCs) alleviate excessive autophagy of ovarian granular cells through VEGFA/PI3K/AKT/mTOR pathway in premature ovarian failure rat model. J. Ovarian Res. 16 (1), 198. doi:10.1186/s13048-023-01278-z
Duerrschmidt, N., Zabirnyk, O., Nowicki, M., Ricken, A., Hmeidan, F. A., Blumenauer, V., et al. (2006). Lectin-like oxidized low-density lipoprotein receptor-1-mediated autophagy in human granulosa cells as an alternative of programmed cell death. Endocrinology 147 (8), 3851–3860. doi:10.1210/en.2006-0088
Elkady, M. A., Shalaby, S., Fathi, F., and El-Mandouh, S. (2019). Effects of quercetin and rosuvastatin each alone or in combination on cyclophosphamide-induced premature ovarian failure in female albino mice. Hum. Exp. Toxicol. 38 (11), 1283–1295. doi:10.1177/0960327119865588
Gao, S., Li, N., Chen, R., Su, Y., Song, Y., and Liang, S. (2022). Bushen Huoxue formula modulates autophagic flux and inhibits apoptosis to protect nucleus pulposus cells by restoring the AMPK/SIRT1 pathway. Biomed. Res. Int. 2022, 8929448. doi:10.1155/2022/8929448
Geidl-Flueck, B., Hochuli, M., Nemeth, A., Eberl, A., Derron, N., Kofeler, H. C., et al. (2021). Fructose- and sucrose- but not glucose-sweetened beverages promote hepatic de novo lipogenesis: a randomized controlled trial. J. Hepatol. 75 (1), 46–54. doi:10.1016/j.jhep.2021.02.027
Golezar, S., Ramezani Tehrani, F., Khazaei, S., Ebadi, A., and Keshavarz, Z. (2019). The global prevalence of primary ovarian insufficiency and early menopause: a meta-analysis. Climacteric 22 (4), 403–411. doi:10.1080/13697137.2019.1574738
Huang, L., Wang, H., Shi, M., Kong, W., and Jiang, M. (2022). Lipid profile in patients with primary ovarian insufficiency: a systematic review and meta-analysis. Front. Endocrinol. (Lausanne) 13, 876775. doi:10.3389/fendo.2022.876775
Huhtaniemi, I., Hovatta, O., La Marca, A., Livera, G., Monniaux, D., Persani, L., et al. (2018). Advances in the molecular pathophysiology, genetics, and treatment of primary ovarian insufficiency. Trends Endocrinol. Metab. 29 (6), 400–419. doi:10.1016/j.tem.2018.03.010
Ishizuka, B. (2021). Current understanding of the etiology, symptomatology, and treatment options in premature ovarian insufficiency (POI). Front. Endocrinol. (Lausanne) 12, 626924. doi:10.3389/fendo.2021.626924
Jang, S. A., Park, D. W., Kwon, J. E., Song, H. S., Park, B., Jeon, H., et al. (2017). Quinic acid inhibits vascular inflammation in TNF-α-stimulated vascular smooth muscle cells. Biomed. Pharmacother. 96, 563–571. doi:10.1016/j.biopha.2017.10.021
Jiao, X., Zhang, X., Li, N., Zhang, D., Zhao, S., Dang, Y., et al. (2021). T(reg) deficiency-mediated T(H) 1 response causes human premature ovarian insufficiency through apoptosis and steroidogenesis dysfunction of granulosa cells. Clin. Transl. Med. 11 (6), e448. doi:10.1002/ctm2.448
Li, D., Xu, W., Wang, X., Dang, Y., Xu, L., Lu, G., et al. (2021a). lncRNA DDGC participates in premature ovarian insufficiency through regulating RAD51 and WT1. Mol. Ther. Nucleic Acids 26, 1092–1106. doi:10.1016/j.omtn.2021.10.015
Li, Y., Xia, G., Tan, Y., and Shuai, J. (2020). Acupoint stimulation and Chinese herbal medicines for the treatment of premature ovarian insufficiency: a systematic review and meta-analysis. Complement. Ther. Clin. Pract. 41, 101244. doi:10.1016/j.ctcp.2020.101244
Li, Z., Zhang, M., Tian, Y., Li, Q., and Huang, X. (2021b). Mesenchymal stem cells in premature ovarian insufficiency: mechanisms and prospects. Front. Cell Dev. Biol. 9, 718192. doi:10.3389/fcell.2021.718192
Liu, T., Qu, J., Tian, M., Yang, R., Song, X., Li, R., et al. (2022). Lipid metabolic process involved in oocyte maturation during folliculogenesis. Front. Cell Dev. Biol. 10, 806890. doi:10.3389/fcell.2022.806890
Ma, Y., Zheng, L., Wang, Y., Gao, Y., and Xu, Y. (2022). Arachidonic acid in follicular fluid of PCOS induces oxidative stress in a human ovarian granulosa tumor cell line (KGN) and upregulates GDF15 expression as a response. Front. Endocrinol. (Lausanne) 13, 865748. doi:10.3389/fendo.2022.865748
Ma, Z., Liu, H., Wang, W., Guan, S., Yi, J., and Chu, L. (2017). Paeoniflorin suppresses lipid accumulation and alleviates insulin resistance by regulating the Rho kinase/IRS-1 pathway in palmitate-induced HepG2Cells. Biomed. Pharmacother. 90, 361–367. doi:10.1016/j.biopha.2017.03.087
Matsuda, F., Inoue, N., Manabe, N., and Ohkura, S. (2012). Follicular growth and atresia in mammalian ovaries: regulation by survival and death of granulosa cells. J. Reprod. Dev. 58 (1), 44–50. doi:10.1262/jrd.2011-012
Mazure, N. M., and Pouyssegur, J. (2010). Hypoxia-induced autophagy: cell death or cell survival? Curr. Opin. Cell Biol. 22 (2), 177–180. doi:10.1016/j.ceb.2009.11.015
Miao, M., Gao, M., Li, T., Jiang, C., Lu, Y., Chen, S., et al. (2020). Tandem mass tag-based proteomic analysis reveals the treatment mechanism of Bushen Huoxue Formula on psychological stress-induced premature ovarian insufficiency. J. Ethnopharmacol. 258, 112870. doi:10.1016/j.jep.2020.112870
Nteeba, J., Ganesan, S., and Keating, A. F. (2014). Progressive obesity alters ovarian folliculogenesis with impacts on pro-inflammatory and steroidogenic signaling in female mice. Biol. Reprod. 91 (4), 86. doi:10.1095/biolreprod.114.121343
Said, R. S., El-Demerdash, E., Nada, A. S., and Kamal, M. M. (2016). Resveratrol inhibits inflammatory signaling implicated in ionizing radiation-induced premature ovarian failure through antagonistic crosstalk between silencing information regulator 1 (SIRT1) and poly(ADP-ribose) polymerase 1 (PARP-1). Biochem. Pharmacol. 103, 140–150. doi:10.1016/j.bcp.2016.01.019
Shao, T., Ke, H., Liu, R., Xu, L., Han, S., Zhang, X., et al. (2022). Autophagy regulates differentiation of ovarian granulosa cells through degradation of WT1. Autophagy 18 (8), 1864–1878. doi:10.1080/15548627.2021.2005415
Shao, W., Xu, J., Xu, C., Weng, Z., Liu, Q., Zhang, X., et al. (2021). Early-life perfluorooctanoic acid exposure induces obesity in male offspring and the intervention role of chlorogenic acid. Environ. Pollut. 272, 115974. doi:10.1016/j.envpol.2020.115974
Shaoyong, W., Liu, Y., Xu, B., Pan, B., Xianmi, X., Wang, Y., et al. (2022). Exposure to BDE-47 causes female infertility risk and induces oxidative stress and lipotoxicity-mediated ovarian hormone secretion disruption in mice. Sci. Total Environ. 842, 156885. doi:10.1016/j.scitotenv.2022.156885
Shi, J., Ju, R., Gao, H., Huang, Y., Guo, L., and Zhang, D. (2022). Targeting glutamine utilization to block metabolic adaptation of tumor cells under the stress of carboxyamidotriazole-induced nutrients unavailability. Acta Pharm. Sin. B 12 (2), 759–773. doi:10.1016/j.apsb.2021.07.008
Sun, J., Guo, Y., Fan, Y., Wang, Q., Zhang, Q., and Lai, D. (2021). Decreased expression of IDH1 by chronic unpredictable stress suppresses proliferation and accelerates senescence of granulosa cells through ROS activated MAPK signaling pathways. Free Radic. Biol. Med. 169, 122–136. doi:10.1016/j.freeradbiomed.2021.04.016
Tucker, E. J., Grover, S. R., Bachelot, A., Touraine, P., and Sinclair, A. H. (2016). Premature ovarian insufficiency: new perspectives on genetic cause and phenotypic spectrum. Endocr. Rev. 37 (6), 609–635. doi:10.1210/er.2016-1047
van Lennep, J. E. R., Heida, K. Y., Bots, M. L., and Hoek, A.Collaborators of the Dutch Multidisciplinary Guideline Development Group on Cardiovascular Risk Management after Reproductive Disorders (2016). Cardiovascular disease risk in women with premature ovarian insufficiency: a systematic review and meta-analysis. Eur. J. Prev. Cardiol. 23 (2), 178–186. doi:10.1177/2047487314556004
van Lennep, J. E. R., Tokgozoglu, L. S., Badimon, L., Dumanski, S. M., Gulati, M., Hess, C. N., et al. (2023). Women, lipids, and atherosclerotic cardiovascular disease: a call to action from the European Atherosclerosis Society. Eur. Heart J. 44 (39), 4157–4173. doi:10.1093/eurheartj/ehad472
Wang, L., Ma, J., Kang, Y., Zhang, N., Li, X., Wang, H., et al. (2020a). Dysregulation of follicle fatty acid is a potential driver of human primary ovarian insufficiency. J. Mol. Cell Biol. 12 (10), 817–819. doi:10.1093/jmcb/mjaa044
Wang, X., Li, Y., Xie, M., Deng, L., Zhang, M., and Xie, X. (2020b). Urine metabolomics study of Bushen Huoxue Prescription on diabetic retinopathy rats by UPLC-Q-exactive Orbitrap-MS. Biomed. Chromatogr. 34 (4), e4792. doi:10.1002/bmc.4792
Wang, X., Zhang, X., Dang, Y., Li, D., Lu, G., Chan, W. Y., et al. (2020c). Long noncoding RNA HCP5 participates in premature ovarian insufficiency by transcriptionally regulating MSH5 and DNA damage repair via YB1. Nucleic Acids Res. 48 (8), 4480–4491. doi:10.1093/nar/gkaa127
Wang, Y., Li, C., Ali, I., Li, L., and Wang, G. (2020d). N-acetylcysteine modulates non-esterified fatty acid-induced pyroptosis and inflammation in granulosa cells. Mol. Immunol. 127, 157–163. doi:10.1016/j.molimm.2020.09.011
Webber, L., Anderson, R. A., Davies, M., Janse, F., and Vermeulen, N. (2017). HRT for women with premature ovarian insufficiency: a comprehensive review. Hum. Reprod. Open 2017 (2), hox007. doi:10.1093/hropen/hox007
Wu, M., Huang, Y., Zhu, Q., Zhu, X., Xue, L., Xiong, J., et al. (2022). Adipose tissue and ovarian aging: potential mechanism and protective strategies. Ageing Res. Rev. 80, 101683. doi:10.1016/j.arr.2022.101683
Xie, Q. E., Wang, M. Y., Cao, Z. P., Du, X., Ji, D. M., Liang, D., et al. (2021). Melatonin protects against excessive autophagy-induced mitochondrial and ovarian reserve function deficiency though ERK signaling pathway in Chinese hamster ovary (CHO) cells. Mitochondrion 61, 44–53. doi:10.1016/j.mito.2021.09.009
Xiu, Z., Tang, S., Kong, P., Yan, M., Tong, X., Liu, X., et al. (2023). Zigui-Yichong-Fang protects against cyclophosphamide-induced premature ovarian insufficiency via the SIRT1/Foxo3a pathway. J. Ethnopharmacol. 314, 116608. doi:10.1016/j.jep.2023.116608
Xu, Y., Jiang, Y., Jia, B., Wang, Y., and Li, T. (2021). Icariin stimulates osteogenesis and suppresses adipogenesis of human bone mesenchymal stem cells via miR-23a-mediated activation of the Wnt/β-catenin signaling pathway. Phytomedicine 85, 153485. doi:10.1016/j.phymed.2021.153485
Yan, Z., Dai, Y., Fu, H., Zheng, Y., Bao, D., Yin, Y., et al. (2018). Curcumin exerts a protective effect against premature ovarian failure in mice. J. Mol. Endocrinol. 60 (3), 261–271. doi:10.1530/JME-17-0214
Zeng, P., Zhou, H., Guo, P., Han, N., Zhang, X., Yin, Z., et al. (2023). Bushen Huoxue formula for the treatment of diminished ovarian reserve: a combined metabolomics and integrated network pharmacology analysis. Heliyon 9 (9), e20104. doi:10.1016/j.heliyon.2023.e20104
Zhang, Q. C., Zhao, Y., and Bian, H. M. (2014). Anti-thrombotic effect of a novel formula from Corni fructus with malic acid, succinic acid and citric acid. Phytother. Res. 28 (5), 722–727. doi:10.1002/ptr.5052
Zhao, S., Cheng, L., Shi, Y., Li, J., Yun, Q., and Yang, H. (2021). MIEF2 reprograms lipid metabolism to drive progression of ovarian cancer through ROS/AKT/mTOR signaling pathway. Cell Death Dis. 12 (1), 18. doi:10.1038/s41419-020-03336-6
Zhongwei, P., Ye, Y., and Wang, P. (2019). Curative effect of Bushen Huoxue decoction on premature ovarian insufficiency of type of kidney deficiency and blood stasis. Mod. J. Integr. Traditional Chin. West. Med. 28 (23), 2518–2522.
Zhou, C., Zhou, J., Han, N., Liu, Z., Xiao, B., and Yin, J. (2015). Beneficial effects of neomangiferin on high fat diet-induced nonalcoholic fatty liver disease in rats. Int. Immunopharmacol. 25 (1), 218–228. doi:10.1016/j.intimp.2015.01.027
Zhou, F., Song, Y., Liu, X., Zhang, C., Li, F., Hu, R., et al. (2021). Si-Wu-Tang facilitates ovarian function through improving ovarian microenvironment and angiogenesis in a mouse model of premature ovarian failure. J. Ethnopharmacol. 280, 114431. doi:10.1016/j.jep.2021.114431
Zhou, J., Peng, X., and Mei, S. (2019). Autophagy in ovarian follicular development and atresia. Int. J. Biol. Sci. 15 (4), 726–737. doi:10.7150/ijbs.30369
Keywords: premature ovarian insufficiency, bushen huoxue formula, lipid, ROS, autophagy
Citation: Li T, Wei Y, Jiao B, Hao R, Zhou B, Bian X, Wang P, Zhou Y, Sun X and Zhang J (2024) Bushen Huoxue formula attenuates lipid accumulation evoking excessive autophagy in premature ovarian insufficiency rats and palmitic acid-challenged KGN cells by modulating lipid metabolism. Front. Pharmacol. 15:1425844. doi: 10.3389/fphar.2024.1425844
Received: 30 April 2024; Accepted: 03 September 2024;
Published: 16 September 2024.
Edited by:
Hui Hui Zhao, Beijing University of Chinese Medicine, ChinaReviewed by:
Johannes Ott, Medical University of Vienna, AustriaMuhammad Babar Khawar, Chinese Academy of Sciences (CAS), China
Copyright © 2024 Li, Wei, Jiao, Hao, Zhou, Bian, Wang, Zhou, Sun and Zhang. This is an open-access article distributed under the terms of the Creative Commons Attribution License (CC BY). The use, distribution or reproduction in other forums is permitted, provided the original author(s) and the copyright owner(s) are credited and that the original publication in this journal is cited, in accordance with accepted academic practice. No use, distribution or reproduction is permitted which does not comply with these terms.
*Correspondence: Jian Zhang, emhhbmdqaWFuQGpzYXRjbS5jb20=; Xia Sun, Mjg5ODczN0BxcS5jb20=
†These authors have contributed equally to this work.