- 1Yunnan Provincial Key Laboratory of Integrated Traditional Chinese and Western Medicine for Chronic Diseasein Prevention and Treatment, School of Basic Medical Sciences, Yunnan University of Chinese Medicine, Kunming, Yunnan, China
- 2Xiyuan Hospital, China Academy of Chinese Medical Sciences, Beijing, China
- 3College of Traditional Chinese Medicine, Yunnan University of Chinese Medicine, Kunming, Yunnan, China
- 4First Clinical Medical College, Yunnan University of Chinese Medicine, Kunming, Yunnan, China
As a medicinal and edible homologous Chinese herb, Polygonatum sibiricum has been used as a primary ingredient in various functional and medicinal products. Damage to the intestinal mucosal barrier can lead to or worsen conditions such as type 2 diabetes and Alzheimer’s disease. Traditional Chinese medicine and its bioactive components can help prevent and manage these conditions by restoring the integrity of the intestinal mucosal barrier. This review delves into the mode of action of P. sibiricum polysaccharide in disease prevention and management through the restoration of the intestinal barrier. Polysaccharide from P. sibiricum effectively treats conditions by repairing the intestinal mucosal barrier, offering insights for treating complex diseases and supporting the application of P. sibiricum in clinical settings.
1 Introduction
Polygonum sibiricum (PS; in Chinese: Huang Jing) is the dry rhizome of Polygonum kingianum (PK; in Chinese: Dian Huang Jing or Yunnan Huang Jing) and Polygonum cyrtonema (PC; in Chinese: Duohua Huang Jing). PS is a member of the lily family. In traditional Chinese medicine (TCM), PS has the effects of replenishing qi and nourishing yin, strengthening the spleen, moistening the lung, and tonifying the kidney (Chen et al., 2021). In 2002, the Chinese Ministry of Health included PS in the list of substances that are both food and medicine. Polygonatum sibiricum polysaccharide (PSP) is the main active ingredient in PS (Liu S. et al., 2022). PSP has been reported to have many pharmacological applications and biological activities such as antioxidant activity and anti-aging activity. In addition, it has been shown to enhance immunity in immunosuppressed mice by improving gut microbes and activating macrophages (He et al., 2022).
The intestinal mucosal barrier is a multilayered tissue structure with specific functions and is the surface on which the organism interacts with the external environment (Farhadi et al., 2003). In recent years, the intestinal tract has become a research hotspot in the field of TCM, and the intestinal mucosal barrier is an important target for the action of most TCM and the treatment of diseases (Che et al., 2022). The intestinal mucosal barrier is the first line of defense against infiltration of luminal contents and performs many biological functions (Wu et al., 2023). It not only serves as a site for the absorption of food nutrients and microbial metabolites, but also acts as a barrier to prevent microbial invasion of the tissues and moderates the inflammatory response to the myriad of contents in the intestinal lumen. In addition, the intestinal mucosal barrier is plays an important role in immune defenses and in the maintenance of the integrity of the intestinal mucosa. According to a study published in Carbohydr Polymers, PSP can repair the intestinal mucosal barrier (Gong et al., 2024). In view of this, this paper will summarize the mechanism of PSP in repairing the intestinal mucosal barrier for the treatment of diseases, with a view to providing ideas for the clinical treatment of multisystemic diseases so as to provide a theoretical background for the development and clinical application of PS.
2 PS and PSP
2.1 PS
The name “Huangjing” derives from the traditional Chinese theory of “absorbing the essence of heaven and earth” (Zhao et al., 2018). Modern pharmacological studies have shown that PS has anticancer, anti-aging, blood sugar regulation, and anti-fatigue effects (Li X. L. et al., 2023). It is a medicinal and food herb, which has been developed into functional foods such as PS tea and PS liquor. PS is obtained from fruits or vegetables in daily life (Li X. L. et al., 2023), which replenishes the nutrients required for human growth and development (Xie et al., 2021), such as Zn, Ni, Pb, Sn, Mg, Mn, Fe, Li, Ca, glutamic acid, and aspartic acid. (Yao-Yao et al., 2022; Chen et al., 2024). PS has the potential to be developed into a novel and promising functional health food for older individuals. Studies have shown that PS can activate the BDNF/TrKB pathway to improve cognitive dysfunction and synaptic plasticity in naturally aging rats (Zhang et al., 2022). Furthermore, PS can effectively regulate glucose and lipid metabolism during the development of type 2 diabetes (T2D) as an alternative functional food (Wang G. et al., 2022). In the Chinese Pharmacopoeia, the clinical dosage of PS is 9–15 g. In current clinical records, the dosage of PS is mostly 12–15 g (Xu et al., 2024). More importantly, since ancient times, China has had a history of combining PS with other medicinal drugs to prevent and treat diseases, for example, Erjingwan during the Song dynasty. Erjingwan can reduce intestinal inflammatory reactions, restore intestinal mucosal barrier function, and reduce the level of neuroinflammatory factors by regulating the intestinal bacterial flora and participating in the metabolism of short-chain fatty acids (SCFA), thus representing a suitable treatment for Alzheimer’s disease (AD) (Li-ping et al., 2023). Most traditional Chinese medicines are taken orally, increasing the interactions between drugs and intestinal microorganisms, which may be the key to their efficacy. It is evident that targeting the regulation of intestinal microecology could be an important strategy for disease control (Chen and Houkai, 2020). Repairing the intestinal mucosal barrier by increasing the abundance of probiotics can alleviate the development of diseases such as functional dyspepsia,antibiotic-associated diarrhea and T2D (Medina-Vera et al., 2019; Mekonnen et al., 2020; Shen et al., 2024). In modern use, PS is often paired with other medicinal drugs to treat diseases. Yongwei (Fu Yong-wei, 2022) showed that Polygonatum and Euryaleferox powder composed of PS and Semen Euryales, Dioscorea opposita Thunb, Semen ziziphi spinosae, Chinese-date, Radix codonopsis, and Patchouli could significantly increase the abundance of Bifidobacteria and Lactobacilli in the intestinal tract, decrease that of Escherichia coli and Enterococci, and increase the body’s immune system and digestive and absorptive capacity, which in turn achieved an ameliorative effect on functional dyspepsia. Liu et al. (Liu Ying et al., 2023) showed that PS paired with Polygonatum odoratum and Mulberry leaf restored the intestinal mucosal barrier in rats with antibiotic-associated diarrhea by restoring the diversity and abundance of the intestinal flora, decreasing the content of pro-inflammatory inflammatory factors, alleviating inflammatory symptoms, and restoring the intestinal mucosal barrier. Song et al. (Song, 2023) showed that the compatibility of PS with Pueraria lobata, Mulberry leaf, Poria cocos, and Lycium barbarum can alleviate T2D by reducing systemic chronic low-grade inflammation caused by lipopolysaccharide (LPS) by regulating the proportion of Firmicutes and Bacteroides (F/B) in the intestinal flora (as shown in Table 1). Thus, the demand for PS has increased annually to 4,000 tons (Zhang J. et al., 2021).
2.2 PSP
The active constituents of PS include polysaccharides, steroidal saponins, and volatile oils, among which polysaccharides are the most important active constituents of PS. Polysaccharides are one of the four basic substances that constitute life, consisting of at least 10 or more monosaccharide molecules through condensation, and with the loss of water molecules forms polymers, the molecular structural formula is (C6H10O5)n and is widely found in nature, mainly in plant cell walls, animal cell membranes, and microbial cell walls (Xu et al., 2019). Polysaccharide content ranges from 11% to 22% and comprises five components, namely PSW1B-b, PSW2A-1, PSW3A-1, PSW4A, and PSW5B (Liu S. et al., 2022). The main distribution of the relative molecular masses of homogeneous polysaccharides isolated from PS to date ranges from 1.80 × 103 to 6.28 × 105 and predominantly consists of galactose (Gal) and mannose (Man), but also includes fructose (Fru), glucose (Glc), arabinose (Ara), and rhamnose (Rha), as well as small amounts of glucuronic acid (GlcA) and xylose (Xyl) (Liu S. et al., 2022). Pharmacological studies have shown that PSP exerts antidepressant (Shen et al., 2021), anti-aging (Ma et al., 2021), anticancer (Long et al., 2018) and antidiabetic (Li et al., 2020) effects. PSP can exert anti-inflammatory effects through the TLR4/Myd88/NF-κB pathway (Liu T. Y. et al., 2020). In the LPS-induced acute liver injury (SALI) murine model, PSP treatment significantly and inversely reduced serum levels of inflammatory cytokines tumor necrosis factor (TNF) - α and interleukin (IL)-6, as well as pyroptosis-associated inflammatory cytokines IL-18 and IL-1β, compared with controls (Xiao et al., 2022). PSP improves the tumor microenvironment and dose-dependently inhibits migration, invasion, and epithelial mesenchymal transition of hepatocellular carcinoma cells (Xu et al., 2023a). Long et al. (2018) demonstrated that PSP exerts anticancer effects through the TLR4/MAPK/NF-κB signaling pathway. In addition, PSP can prevent MPP-induced neurotoxicity in a dose-dependent manner, while inhibiting the production of reactive oxygen species and increasing the ratio of reduced glutathione/oxidized glutathione, resulting in antiapoptotic and antioxidant effects (Huang et al., 2021). At the same time, PSP can prevent depressive-like behavior as well as synaptic and neuronal damage by reducing ROS (Shen et al., 2021).
Different varieties of PS have different PSP content and acidic/alkaline differences. Polysaccharide content in PS and PC is higher than PK, whereas PS is dominated by neutral polysaccharides and PC comprised mainly acidic polysaccharides (Tao et al., 2023). After PS is washed, cut, dried and crushed, PSP can be extracted in three steps: removal of impurities, cell wall isolation, and polysaccharide isolation (Chen et al., 2020). Oligofructose with a polymerization degree between 5 and 10 is the main component of PSP (Singh et al., 2016). Oligofructans are oligosaccharides widely found in natural plants, which can be obtained by direct extraction or by enzyme synthesis from different substrates, and consist of linear chains of fructose units linked by β (2-1) (Sabater-Molina et al., 2009; Silva et al., 2023). Oligofructose has been shown to improve cognitive dysfunction (Sun et al., 2019), diabetes mellitus (Pengrattanachot et al., 2022), and nonalcoholic fatty liver disease (Huang et al., 2023), and is now recognized as a prebiotic that selectively promotes colonization and activity of beneficial bacteria, improves metabolism of the intestinal flora, reduces inflammation, and enhances host immunity (Huang et al., 2023). He et al. (2021) isolated two oligofructans from PC polysaccharide (PCP) with a polymerization degree between 5 and 10. Zhang J. et al. (2021) also extracted oligosaccharides from PCP.
Processing (in Chinese: Paozhi) is an important feature of Chinese medicine that originated in the early stages of its history and continues to evolve. Different herbs require different processing methods, and the effects of different processing methods on herbs are complex. Food PS are mostly processed by water or by steaming with wine to eliminate the side effect of making the mouth numb (Gan et al., 2019). Steam-processed PS has been used as a food source for thousands of years, and the Chinese Qing Dynasty medical book Yao Pin Jian Yi recorded that “long time steaming system is ripe, and it becomes purple black,” which believed that the original function of PS could be enhanced after steaming. The reason for this is that during the steaming process, PS can undergo acid methanolysis to hydrolyze polysaccharides, producing different types of secondary polysaccharides including oligosaccharides and monosaccharides (Bian et al., 2022). Wang et al. (Wang J. et al., 2022) also confirmed that the main components of polysaccharides in PS after steaming are Gal and GalA, and a small amount of Man, Glc, Ara, Rha, and GlcN. Repetitive cooking and drying of polysaccharides obtained from the rhizomes of PSP is considered a key step to improve their nutritional activity (Liu et al., 2024). Different steaming times result in significant changes in the structure of PSP, which have a significant modulating effect on immune function. Su et al. (2023) demonstrated that PSP with the gradual increase in steaming time can lead to a gradual increase in the number of CD4+/CD8+T-lymphocytes along with a gradual increase in the number of CD4+/CD8+T-lymphocytes.
In summary, polysaccharides of natural origin, especially those of traditional tonic Chinese medicines, exhibit a variety of pharmacological activities and have attracted the attention of drug developers. However, their mechanisms remain to be elucidated. Increasing research on intestinal microecology in recent years has determined that herbal polysaccharides can target the repair of the intestinal mucosal barrier for the treatment of multisystemic diseases (Yue et al., 2022); therefore, it is particularly important to gain a deeper understanding of PSP and its relationship with the intestinal mucosal barrier.
3 Intestinal mucosal barrier
The intestine is the largest digestive organ in the body and is in very close contact with the outside world; therefore, a strong barrier system has evolved in the intestine (Zhou et al., 2020). The intestinal mucosal barrier is the largest and most important barrier against the external environment and plays an important role in maintaining homeostasis (Groschwitz and Hogan, 2009). Under normal conditions, the intestinal mucosal barrier can be classified as a mechanical, chemical, immune, and biological barrier (Khoshbin and Camilleri, 2020). The mechanical (physical) barrier comprises a mucous layer, glycocalyx on intestinal epithelial cells (IECs), and cell connections involved in the physical barrier (Kayama et al., 2020),which serve to prevent harmful substances and bacteria from entering the intestinal cavity and causing inflammation (Peng et al., 2024). The mechanical barrier also provides intestinal motility and regulation of intestinal peristalsis to promote the elimination of food residues in the intestine, reducing the probability of bacteria staying in the intestinal epithelial for long periods or passing through the intestinal epithelium (Vanuytsel et al., 2023). The chemical barrier mainly comprises metabolites from the intestine and innate immune molecules, such as mucin, antimicrobial peptides, and lysozyme secreted by IECs. Mucins, especially mucin-2 (Muc-2), are important components of the skeleton of the intestinal mucus layer of the chemical barrier (Hansson and Johansson, 2010). The immune barrier mainly comprises secretory immunoglobulin A(SIgA). on the surface of the epithelial, gut-associated lymphoid tissue, and immune cells distributed in the epithelial and sub epithelial layers of the intestine. SIgA is the most abundant immunoglobulin in the intestine, and is mainly present in the upper layer of epithelial mucus. It is the main effector factor of the local immune response in the intestinal epithelium, defending against pathogen adhesion and colonization in the intestine (Peterson and Artis, 2014). The number of CD8+ and CD4+ lymphocytes in the intestinal epithelium increases upon stimulation, promoting the secretion of interleukin (IL)-6, IL-10, and interferon-α. Various cytokines produce cytotoxicity to combat pathogens, repair theintestinal barrier, and promote intestinal healing (Sarrabayrouse et al., 2015). The intestinal flora is a general term for microorganisms living in the human gut (Che et al., 2022). It is not only an important component of the biological barrier, but it also plays an important role in the functioning of other barriers and promotes the joint functioning with the intestinal mucosal barrier (Zheng et al., 2023).
3.1 Intestinal flora as a basis for the functioning of the intestinal mucosal barrier
The intestinal tract of healthy adults contains approximately 1013 to 1014 microorganisms with a complex structure that is influenced by factors such as genotype, diet, age, disease, and lifestyle (Adak and Khan, 2019). Under physiological conditions, the large intestinal flora remains relatively stable and constitutes a complex microecological environment. Intestinal specialized anaerobic bacteria (e.g., Bifidobacterium bifidum, Lactobacillus lactis) can defend against pathogen invasion, synthesize vitamins, produce a number of enzymes, and participate in nutrient metabolism, and can form an intestinal biobarrier through the phosphoric acid wall and interconnection with the human intestinal mucosa to prevent invasion of harmful microorganisms or toxins, which constitutes a biobarrier (Albillos et al., 2020). The tight junction (TJ) between the intestinal epithelial cells (IEC) is a major component of the mechanical barrier and is responsible for closing the intercellular space and preventing harmful substances and bacteria from entering the intestinal lumen and triggering an inflammatory response (Kaminsky et al., 2021; Wu et al., 2023). Changes in intestinal flora can lead to TJ disruption and increased intestinal permeability. Studies have shown that F/B is associated with LPS production, and a dysregulation of the ratio of the two promotes LPS production and activates the TLR4/MyD88/NF-κB pathway inducing inflammatory factors such as IL-1β, IL-6 and TNF-α to disrupt TJs and increase intestinal permeability (Martin-Subero et al., 2016; Zou et al., 2023). In the chemical barrier, mucins are secreted by cup cells and form a protective homeostatic barrier between the resident microbiota and potential immune cells in the colon (Bergstrom and Xia, 2013). In ulcerative colitis (UC) model mice, the number of goblet cells and the expression of Muc-2 are significantly reduced and is accompanied by dysregulation of the intestinal flora (Hu et al., 2021). Dysregulation of the intestinal flora leads to dysregulation of metabolites and proteins, including SCFAs, vitamins, tryptophan, and bile acids. In turn, these metabolites (predominantly SCFAs) can stimulate goblet cells to promote Muc-2 expression through the β-oxidation-like pathway-induced hypoxia-specific activation of HIF-2α (Hsieh et al., 2015; Ma et al., 2022). SIgA, the most abundant immunoglobulin in the intestine, is a major effector of the local immune response of IECs against adhesion and colonization of pathogens in the intestine (Peterson and Artis, 2014). Intestinal SIgA is closely linked to the intestinal flora, and studies have shown that germ-free mice produce a small amount of SIgA, which increases rapidly after bacterial colonization (Bos et al., 1989). Among them, Bifidobacterium and Lactobacillus promote the secretion of SIgA, and in addition, Bifidobacterium and Lactobacillus mediate the balance of Treg/Th17 in relation to this, which affects cytokine homeostasis (de Toro-Martín et al., 2017).
3.2 Damage to the intestinal mucosal barrier induces and exacerbates multisystemic diseases
There is growing evidence indicating that the intestinal mucosal barrier is impaired not only in digestive disorders but also in central nervous system (CNS) disorders, metabolic disorders, and in age-related diseases. The mechanisms by which the “gut-brain axis” and the “gut-liver axis” affect other organ diseases through the gut barrier and the gut microbiota have become a hot research topic.
The intestinal mucosal barrier can be impaired in a variety of diseases. Increased translocation of bacteria, endotoxins, and other inflammatory mediators can activate relevant pathways leading to progressive exacerbation of, for example, neurodegenerative and endocrine diseases (Ghosh et al., 2021). Furthermore, aging-induced decline in intestinal mucosal barrier function can lead to the progressive exacerbation of associated diseases (Gong et al., 2023). Aging causes a significant increase in the abundance of LPS-secreting Mycobacterium anisopliae and a significant decrease in the abundance of butyrate-metabolizing Bacteroides thickettsiae, which increase inflammatory mediators and reactive oxygen species (ROS), induce TJ dysregulation, and lead to intestinal mucosal barrier damage (Wyss-Coray, 2016; Takiishi et al., 2017). Aging underlies neurodegeneration and dementia (Lechin and van der Dijs, 2009), such as AD and Parkinson’s disease (PD). Clinical studies have shown that these degenerative neuropathies are usually accompanied by damage to the intestinal mucosal barrier and increased abundance of Gram-negative bacteria, which releases LPS and circulating pro-inflammatory factors into the bloodstream, destroying the integrity of the blood-brain barrier, activating microglial cells and promoting non-programmed cell death, which further exacerbates the progression of the disease (Yang et al., 2021; Mayer et al., 2022). The function of the intestinal mucosal barrier also plays an important role in regulating metabolic diseases (e.g., obesity, T2D) (Gong et al., 2023; Zhou et al., 2023). A high-fat diet (HFD) alters the composition of the intestinal flora, leading to decreased expression of TJ protein, increased intestinal permeability, translocation of bacteria and bacterial products, and generation of systemic chronic low-grade inflammation leading to metabolic disorders (Lau et al., 2016). During T2D progression, diet-driven unfavorable microbiota composition can lead to disturbed gut flora composition with intestinal mucosal barrier damage, reduced probiotic (including butyric acid-producing bacteria) populations, increased populations of conditionally pathogenic bacteria, and increased production of pro-inflammatory LPS, which in turn exacerbate insulin resistance and metabolic disturbances in T2D via the NF-κB and the JNK signaling pathways (Lewis and Taylor, 2020; Meng et al., 2023). SCFAs can be involved in the regulation of secretion of intestinal peptides that regulate appetite and insulin secretion, such as glucagon-like peptide 1 (GLP-1) (Wang et al., 2020). The number of butyric acid-producing bacteria is also decreased in the intestinal tract of patients with T2D (Liu Q. et al., 2021) (as shown in Figure 1).
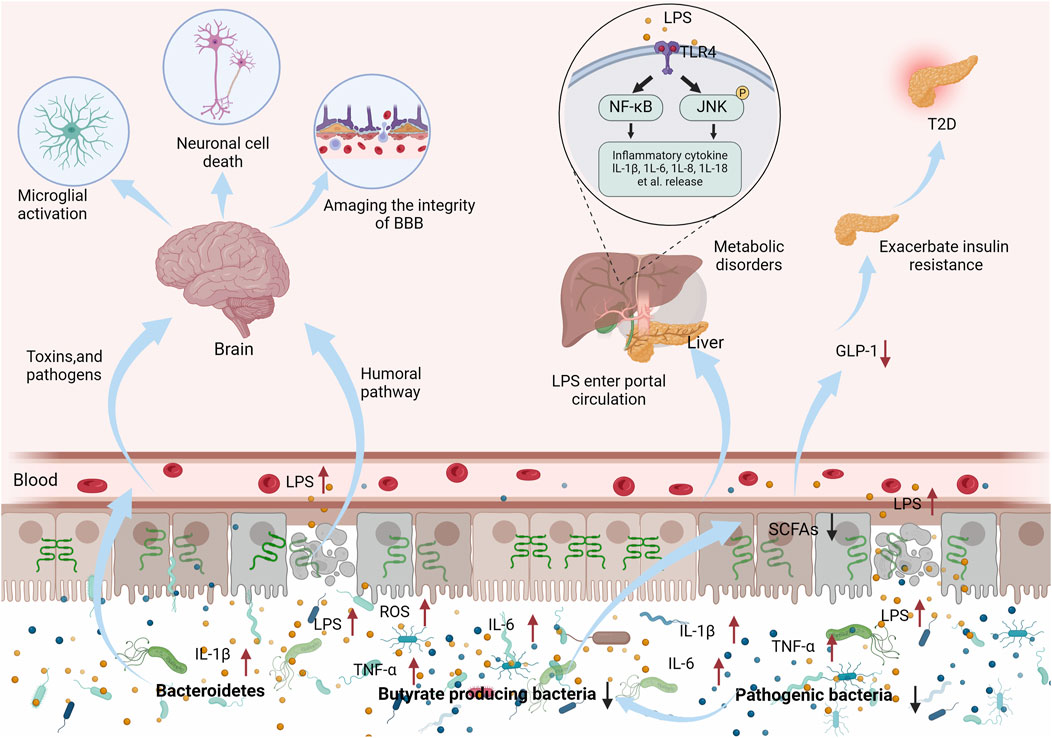
Figure 1. Dysbiosis of intestinal flora and reduction of SCFA can lead to damage of the intestinal mucosal barrier and entry of inflammatory factors and toxins into the bloodstream, exacerbating the development of neurological and endocrine disorders.
Currently, there are few therapeutic approaches specifically designed to target intestinal mucosal barrier function, and the main clinical focus is on enhancing beneficial flora or metabolites or fecal transplantation techniques (Li D. et al., 2023). Effective ingredients in TCM can repair or alleviate damage to the intestinal mucosal barrier, thus exerting the function of alleviating or treating digestive, non-gastrointestinal, and central nervous system diseases (Dong et al., 2022). Liu et al. (Liu F. Y. et al., 2021) showed that the active ingredient gastrodin in the medicinal food Rhizoma Gastrodiae could repair the intestinal mucosal barrier and alleviate atherosclerosis by improving the intestinal bacterial flora and restoring the TJ between IECs. Cui et al. (Cui et al., 2022) demonstrated through experimental studies that curcumin in the medicinal herb ginger treats PD by modulating the intestinal microbiota Lactobacillaceae and Aerococceae, as well as key metabolites tyrosine and dopamine. Therefore, PSP, which is also a major component of PS, can also prevent and control multisystemic diseases by repairing intestinal mucosal barrier damage.
4 Mechanisms of intestinal mucosal barrier repair by PSP
4.1 PSP regulates intestinal flora and metabolites to repair the intestinal mucosal barrier
As the basic backbone of PSP, oligofructose as a prebiotic can improve host metabolism by improving intestinal mucosal barrier function by altering the composition of the gut microbiota (Guarino et al., 2020; Wang et al., 2020). Prebiotic intake favors the growth of probiotics such as Lactobacillus and Bifidobacterium (Mohanty, 2018; Farias et al., 2019). PS oligofructose promotes an increase in probiotics such as Bifidobacterium, Heterobacterium, and Alistipes (Xu J. et al., 2023). Probiotics are defined as live microorganisms that improve health by increasing the beneficial components of the intestinal microbiota, reducing pathogen adhesion and intestinal permeability, modulating the immune response, and ensuring appropriate metabolic energy levels (Hill et al., 2014; Serek and Oleksy-Wawrzyniak, 2021). Current studies have shown that probiotics (e.g., Lactobacillus, Bifidobacterium, Bacteroides thetaiotaomicron, and Akkermansia muciniphila) are important in maintaining intestinal epithelial homeostasis and influencing intestinal barrier function (Liu Q. et al., 2020; Guarino et al., 2020). Yang et al. (2020) demonstrated that the probiotic preparations consisting of Bifidobacterium lactis, Lactobacillus casei, B. bifidum, and Lactobacillus acidophilus, significantly repaired intestinal mucosal barrier damage and inflammatory responses due to aging. Probiotics can produce SCFA through digestion, fermentation, and metabolism of dietary fiber, protein, and glycoprotein (Meng et al., 2023). Study has shown that, After entering the body,PSP promotes the proliferation of probiotic bacteria, including Allobaculum, Blautia, Phascolarctobacterium, Roseburia intestinalis, M. anisopliae, Rhodococcus tumefaciens, Lactobacillus, and Prevotella, all of which promote the production of SCFAs. Roseburia intestinalis metabolizes butyric acid, whereas R. tumefaciens and Prevotella metabolize isobutyric, butyric, and valeric acids (Wang et al., 2018). In vitro study also indicate that PSP increases the abundance of probiotics such as Bifidobacteria and concomitantly increases SCFAs (Xu et al., 2023c). SCFA activates G protein-coupled receptors (GPRs) 43 (FFAR2), GPR41 (FFAR3) proteins and olfactory receptor 78 (Olfr78) protein, which mediate the PI3K/Akt/mTOR pathway to enhance intestinal mucosal barrier function (Tang et al., 2022). Butyrate can also activate GPR109 protein, which in turn inhibits the release of pro-inflammatory factors such as TNF-α, IL-12, and IL-1β, affecting the health of the intestinal mucosal barrier (Dang et al., 2021). SCFAs are also important fuels for IECs, which regulate IEC function through different mechanisms in order to modulate their proliferation, differentiation, and function of subpopulations of intestinal endocrine cells, which influence intestinal motility and enhance intestinal barrier function (Martin-Gallausiaux et al., 2021). TJ injury is considered an important causal factor for intestinal mucosal barrier injury and intestinal inflammatory diseases (Zhang et al., 2023). SCFAs enhance extracellular TJ proteins (e.g., ZO-1, occludin) to reduce paracellular permeability, controlling paracellular infiltration of contents from the lumen of the intestinal tract into intestinal tissues and the somatic circulation (Xia et al., 2020; Su et al., 2022). (as shown in Figure 2)The study shows that PSP significantly increased Akkermansia abundance and the content of propionic acid and isovaleric acid in SCFA, and further by transplanting PSP-associated intestinal flora, it significantly increased the expression of TJ protein and decreased the content of pro-inflammatory cytokines and LPS in colon, which further confirms that PSP can repair the intestinal mucosal barrier by enhancing probiotics and increasing SCFA (Mengting, 2017).
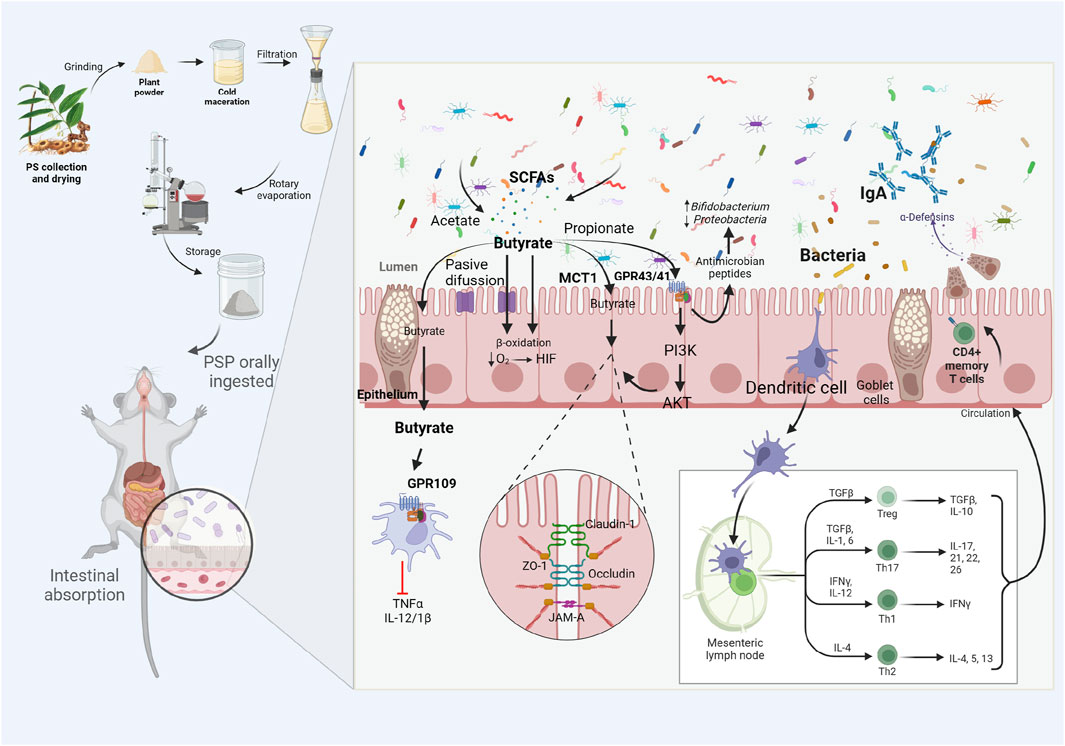
Figure 2. PSP acts as a prebiotic, promoting the production of probiotics and SCFAs, activating related reactions and downstream pathways, inhibiting the release of pro-inflammatory cytokines and increasing the expression of TJ proteins, thereby repairing the intestinal mucosal barrier.In addition, PSP can also repair the intestinal mucosal barrier by modulating immune cells (e.g., Th17/Treg and Th1/Th2).
4.2 PSP modulates immune cells to repair intestinal mucosal barrier
PSP has a significant effect on the regulation of the immune system.PSP promotes the expression of mucins in the jejunum as well as the expression of secretory immunoglobulin, SIgA.These results reveal that PSP improves the immune response of the intestinal mucosa.The results of the study show that PSP can improve the immune response of the intestinal mucosa (Li L. X. et al., 2022). Diagnosis of CD4+/CD8+T-lymphocytes ratio assesses the immune status,It was shown that PSP increased the ratio of CD4+/CD8+T-lymphocytes in a mouse model of cyclophosphamide-induced immunosuppression and significantly increased the splenic index and thymic index, as well as increased the expression of IL-2, IFN-γ, IgA and IgM (Su et al., 2023). During inflammatory progression, the initial CD4+ T lymphocytes are stimulated by antigenic signals and can differentiate into different T lymphocytes under different conditions, including Th1- and Th2-type effector cells, helper T cells 17 (Th17), and regulatory T cells (Tregs), and the balance of Th1/Th2 cells and Th17/Treg cells is important for intestinal mucosal immunity (Li L. X. et al., 2022). Th17 and Treg cells are two different subtypes of T helper cells, which play important roles in regulating the immune system. The former mainly exacerbate the inflammatory response by releasing the inflammatory factor IL-17, whereas Treg cells inhibit the activity of other immune cells by secreting anti-inflammatory cytokines, such as IL-1, IL-2, and transforming growth factor (TGF)-β, which suppresses the activity of the antigen-presenting cells and T-cell function, reducing the production of inflammatory cytokines and antibodies (Li Z. et al., 2022). Th1 and Th2 are phenotypes of host-specific immune responses mediated by CD4+T-lymphocytes in the pathogenesis of inflammatory diseases, and Th1/Th2 balance controls immune and inflammatory responses (Matia-Garcia et al., 2021). Th1 secretes pro-inflammatory cytokines such as IFN-γ, TNF-α, IL-1β, IL-2, IL-6, IL-8, IL-12, etc., and Th2 secretes anti-inflammatory cytokines such as IL-4, IL-10, IL-13, etc., in which IFN-γ and IL-12 secreted by Th1 inhibit the activity of Th2, and IL-4 and IL-10 secreted by Th2 inhibit the activity of Th1. Th1 activity, indicating that Th1 and Th2 can antagonize each other in pro-inflammatory/anti-inflammatory (Martino et al., 2012; Woda et al., 2016). It was shown that PSP enhanced intestinal function in aged mice by suppressing the expression of Th17 and Th1-related pro-inflammatory cytokines, including IL-6, IL-1β, and TNF-α, and increasing the expression of inflammation suppressor cytokines IL-4 and IL-10 secreted by Treg and Th2 cells in colonic tissues (Li L. X. et al., 2022). (as shown in Figure 2).
5 PSP repairs intestinal barrier damage in disease states to combat multisystemic diseases
5.1 Diseases of the nervous system
PS has been shown to improve memory (Che et al., 2022). Previous studies have confirmed that PSP prevents Aβ (Sabater-Molina et al., 2009; Liu et al., 2024) and MPP-induced neurotoxicity through the PI3K/Akt and Nrf2 signaling pathways (Zhang et al., 2015; Huang et al., 2021). AD, a central neurodegenerative disease, is the most common type of dementia, with progressive memory deficits, impaired cognitive perception of objects, marked personality changes and verbal expression problems, among other typical symptoms (Kesika et al., 2021). Studies have shown that Aβ deposits were found in the intestinal tracts of cadaver AD patients; the same was found in the intestinal tracts of APP/PS1 mice with impaired intestinal mucosal barrier and inflammation (Liu G. et al., 2023). Luo et al. (2022) isolated and purified the monomeric polysaccharide PSP-1 from PSP to improve cognitive dysfunction in 5xFAD mice by decreasing Helicobacter pylori abundance, increasing the abundance of probiotic bacteria, such as Akkermansia, rebuilding the intestinal microbiota composition, decreasing the inflammatory response and intestinal Aβ deposition, repairing the intestinal mucosal barrier and improving cognitive dysfunction in 5xFAD mice. Aging can also cause cognitive deficits that progressively worsen with age. Among them, a change in the ratio of F/B due to aging is an important cause of cognitive impairment. Liu Z., (2023) showed that PSP could alleviate cognitive impairment by restoring the F/B ratio of the intestinal flora. External stress not only triggers depression, but also leads to an imbalance in the intestinal flora, which increases intestinal permeability, providing a pathway for lumen-derived molecules, toxins (e.g., LPS), and pathogens to reach the brain parenchyma, activate local immune cells and trigger neuroinflammation, which in turn affects the onset and development of depression (Liu L. et al., 2023). Zhang (Zhang et al., 2023) and others showed that PSP remodeled the intestinal flora, increased the expression of TJ proteins ZO-1 and occludin, and reduced neuroinflammation formed by the entry of LPS and inflammatory factors into the bloodstream to alleviate depression. In addition, through transplantation of PSP-associated intestinal flora, could also increase the expression of TJ proteins, reduce LPS, and inhibit inflammatory responses in the hippocampal area, and alleviate depression, effects similar to that of direct administration of PSP (Table 2).
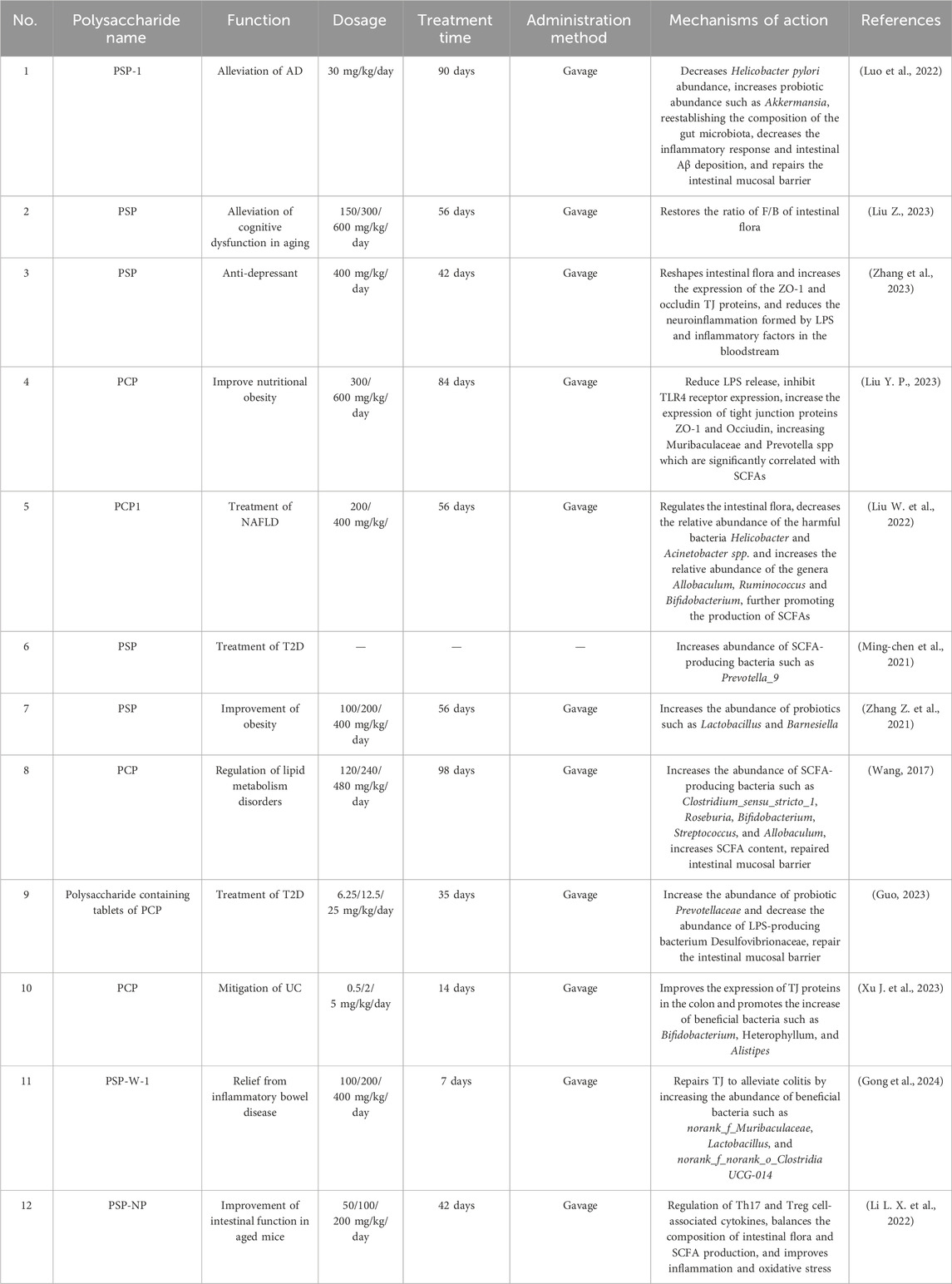
Table 2. Mechanisms of PSP against multisystemic diseases by repairing the intestinal mucosal barrier.
5.2 Endocrine system diseases
Intestinal mucosal barrier damage is strongly associated with the development of metabolic disorders (Chelakkot et al., 2018). Studies have shown that PS aqueous extracts can effectively reduce HFD-induced obesity and hepatic steatosis by increasing beneficial bacteria, decreasing colonic fat digestion/absorption, and increasing hepatic lipid metabolism (Ou et al., 2024). As a major component in PS, PSP also has potential in the treatment of obesity, lipid metabolism disorders and non-alcoholic fatty liver disease (NAFLD). Yapeng Liu showed that PCP can reduce LPS release, inhibit TLR4 receptor expression, reduce inflammatory response, increase the expression of TJ proteins ZO-1 and Occiudin, repair the intestinal mucosal barrier, and improve nutritional obesity by increasing Muribaculaceae and Prevotella spp which are significantly correlated with SCFAs (Liu Y. P., 2023). Liu W. et al., (2022) extracted the neutral polysaccharide PCP1 from PC. PCP1 has a protective effect against NAFLD by a mechanism that regulates the intestinal flora, decreases the relative abundance of the harmful bacteria Helicobacter and Acinetobacter spp. and increases the relative abundance of the genera Allobaculum, Ruminococcus and Bifidobacterium, further promoting the production of SCFAs such as isobutyric acid and isovaleric acid. Modern medicine has shown that PSP shows great potential in the prevention and treatment of endocrine diseases such as diabetes and hyperlipidemia. In China, in addition to the Han ethnic group, the ethnic groups of Tibetan, Mongolian, Miao, Yi, Qiang, and Tu also use PS for the prevention and treatment of T2D (Shi et al., 2023). PSP can affect glucose and lipid metabolism by regulating the intestinal microbiota, especially by increasing the abundance of bacteria producing SCFA (Zhang H. Y. et al., 2021). Yang et al. (Ming-chen et al., 2021) showed that the abundance of SCFA-producing bacteria Prevotella_9 increased in T2D mice after PSP intervention. Zhang et al. (Zhang Z. et al., 2021) reported that PSP fermentation increased the abundance of probiotics such as Lactobacillus and Barnesiella in obese model mice. Wang (2017) reported that PCP improved intestinal flora structure and function, increased the abundance of SCFA-producing bacteria Clostridium_sensu_stricto_1, Roseburia, Bifidobacterium, Streptococcus, and Allobaculum, increased SCFA content, repaired the intestinal mucosal barrier, and alleviated lipid metabolism disorders. Xinyue Guo (Guo, 2023) developed a polysaccharide containing tablets (containing tablets weighing about 0.6 g/tablet and containing no less than 0.28 g/tablet of PCP) with PCP as raw material and relevant excipients, which can increase the abundance of probiotic Prevotellaceae and decrease the abundance of LPS-producing bacterium Desulfovibrionaceae, repair the intestinal mucosal barrier, and treat T2D.(summarized in Table 2).
5.3 Diseases of the digestive system
Damage to the intestinal mucosal barrier is the pathogenesis of many digestive disorders, and dysregulation of intestinal flora or metabolites can disrupt the normal immune balance in the intestinal tract and activate abnormal immune responses in the host, exacerbating intestinal inflammation and damage to mucosal tissues (Wang S. et al., 2023). PS and PSP are not traditional drugs for the treatment of digestive disorders, but have also received attention from researchers due to their role in regulating intestinal flora and metabolites to repair the intestinal mucosal barrier. Xu J. et al. (2023) found that oligofructose isolated from PCP could reduce the levels of inflammatory factors, matrix metalloproteinases, and oxidative stress in the colonic tissues of mice with UC, improve the expression of TJ proteins in the colon, and promote the increase of beneficial bacteria such as Bifidobacterium, Heterophyllum, and Alistipes, which could repair the intestinal mucosal barrier and thus improve UC at multiple levels. Gong et al. (2024) isolated a water-soluble polysaccharide called PSP-W-1 from steamed PS, which improve the expression of TJ proteins to alleviate colitis by increasing the abundance of beneficial bacteria norank_f_Muribaculaceae, Lactobacillus, and norank_f_norank_o_Clostridia UCG-014. The anti-aging effect of PSP by repairing the intestinal mucosal barrier is applicable not only to neurodegenerative diseases, but also to the treatment of intestinal disorders caused by aging. Li L. X. et al. (2022) showed that the mechanism of neutral polysaccharide of PSP (PSP-NP) in improving intestinal function in aged mice was to egulation of Th17 and Treg cell-associated cytokines, balance the composition of intestinal flora and SCFA production, and to improve inflammation and oxidative stress to repair the intestinal mucosal barrier (summarized in Table 2).
6 Discussion and perspectives
Entering the twenty-first century, humankind has encountered unprecedented challenges such as a century of change, the epidemic of the century, regional conflicts, and natural disasters, as well as the complex and serious global food security situation. At the social level, environmental pollution and the increasing competition in study, work, and life have caused people to be under increasing pressure, which has led to the emergence of various diseases. Therefore, it is important to find safe and effective medicines to maintain health. PS is a traditional Chinese herbal medicine and has a history of use of more than 2,000 years. Given the medicinal and food functions of PS, it has been influential in preventing diseases and performing healthcare functions. PS is starch-free, nutritionally diverse, and grows primarily in the understory of mountain forests, thickets, or along the shaded side of mountain slopes, without occupying farmland or forest land. It can not only maintain human health through “food as medicine” and “nutritional diversity”, but also improves concerns of global food shortage and promotes economic development (Si et al., 2024). In this review, we provide an overview of the properties of PSP, as the main active ingredient in PS. PSP can be used as a prebiotic to promote the increase in probiotics and their metabolites SCFAs, activate related receptors, enhance related TJs, and repair the IEC, and prevent and control multisystem diseases.
However, it should be acknowledged that current studies on the single mechanism of PSP repair of the intestinal mucosal barrier have focused mainly on the repair of the intestinal mucosal barrier by SCFA, a metabolite of the regulatory flora. In existing reports, other metabolites such as tryptophan and bile acids have achieved protective and repairing effects on the intestinal mucosal barrier (Wang S. et al., 2023); therefore, future research on the mechanism of PSP that repairs the intestinal mucosal barrier should consider whether PSP works by regulating other metabolites. In this review, we report that the intestinal flora is the basis of other barriers, and plant-based polysaccharides can also promote repair of the intestinal mucosal barrier by targeting intestinal flora and regulating the dynamic balance of Th1/Th2 and Th17/Treg (Wang X. et al., 2023). Although, there are a few reports indicating that PSP can regulate immune cell balance through intestinal flora or repair the intestinal mucosal barrier, and thus, act as a potential immune modulating drug, which could be the focus of future research. Furthermore, the extraction process, purity control, preparation method, and selection of the dosage form of PSP will also affect the efficacy of the drug. Thus, additional detailed studies and research are needed to support the translation of PSP into drugs or health products.
Author contributions
YR: Writing–review and editing, Writing–original draft, Conceptualization. YS: Writing–review and editing, Writing–original draft, Validation. Y-YL: Writing–review and editing, Visualization. SW: Writing–review and editing, Data curation. QL: Writing–review and editing, Visualization. C-YD: Writing–review and editing, Visualization, Methodology. LS: Writing–review and editing, Visualization, Methodology. X-YL: Writing–review and editing, Supervision. J-LY: Writing–review and editing, Supervision, Funding acquisition.
Funding
The author(s) declare that financial support was received for the research, authorship, and/or publication of this article. This project was organized by the Cultivating Plan Program for the Leader in Science and Technology of Yunnan Province (202304BQ040021); Yunnan Provincial Department of Science and Technology’s Science and Technology Talent and Platform Plan (2019DG016); and Yunnan Provincial Major Science and Technology Special Plan (202102AE090031).
Acknowledgments
All authors thank Professor Cheng Lu of the Institute of Basic Research in Clinical Medicine, China Academy of Chinese Medical Sciences, for his guidance in writing the manuscript. The figures in this review have obtained permission to use BioRender content, including icons, templates, and other original artworks, which appear in the accompanying complete graphics in accordance with BioRender's academic license terms. This license allows the sublicense of BioRender content for journal publications.
Conflict of interest
The authors declare that the research was conducted in the absence of any commercial or financial relationships that could be construed as a potential conflict of interest.
Publisher’s note
All claims expressed in this article are solely those of the authors and do not necessarily represent those of their affiliated organizations, or those of the publisher, the editors and the reviewers. Any product that may be evaluated in this article, or claim that may be made by its manufacturer, is not guaranteed or endorsed by the publisher.
References
Adak, A., and Khan, M. R. (2019). An insight into gut microbiota and its functionalities. Cell Mol. Life Sci. 76 (3), 473–493. doi:10.1007/s00018-018-2943-4
Albillos, A., de Gottardi, A., and Rescigno, M. (2020). The gut-liver axis in liver disease: pathophysiological basis for therapy. J. Hepatol. 72 (3), 558–577. doi:10.1016/j.jhep.2019.10.003
Bergstrom, K. S., and Xia, L. (2013). Mucin-type O-glycans and their roles in intestinal homeostasis. Glycobiology 23 (9), 1026–1037. Epub 2013 Jun 10. PMID: 23752712; PMCID: PMC3858029. doi:10.1093/glycob/cwt045
Bian, Z., Li, C., Peng, D., Wang, X., and Zhu, G. (2022). Use of steaming process to improve biochemical activity of polygonatum sibiricum polysaccharides against D-galactose-induced memory impairment in mice. Int. J. Mol. Sci. 23 (19), 11220. doi:10.3390/ijms231911220
Bos, N. A., Kimura, H., Meeuwsen, C. G., De Visser, H., Hazenberg, M. P., Wostmann, B. S., et al. (1989). Serum immunoglobulin levels and naturally occurring antibodies against carbohydrate antigens in germ-free BALB/c mice fed chemically defined ultrafiltered diet. Eur. J. Immunol. 19 (12), 2335–2339. doi:10.1002/eji.1830191223
Che, Q., Luo, T., Shi, J., He, Y., and Xu, D. L. (2022). Mechanisms by which traditional Chinese medicines influence the intestinal flora and intestinal barrier. Front. Cell Infect. Microbiol. 12, 863779. doi:10.3389/fcimb.2022.863779
Chelakkot, C., Ghim, J., and Ryu, S. H. (2018). Mechanisms regulating intestinal barrier integrity and its pathological implications. Exp. Mol. Med. 50 (8), 103–109. doi:10.1038/s12276-018-0126-x
Chen, D., Han, Z., and Si, J. (2021). Huangjing (Polygonati rhizoma) is an emerging crop with great potential to fight chronic and hidden hunger. Sci. China Life Sci. 64 (9), 1564–1566. doi:10.1007/s11427-021-1958-2
Chen, Z., Liu, J., Kong, X., and Li, H. (2020). Characterization and immunological activities of polysaccharides from polygonatum sibiricum. Biol. Pharm. Bull. 43 (6), 959–967. doi:10.1248/bpb.b19-00978
Chen, L., and Houkai, L. (2020). Targeting microbiome: new opportunities and challenges in the study of pharmacodynamic mechanism of traditional Chinese medicine. Sh. J. TCM 54 (02), 15–20+14. in China. doi:10.16305/j.1007-1334.2020.02.004
Chen, M., Huang, Z., Dong, Z., Ying, L. U., Tang, Qi, Zou, H., et al. (2024). Effect of processing on amino acid composition and nutritional value of protein from Polygonatum. Food Ferment. Industries, 1–11. in China. doi:10.13995/j.cnki.11-1802/ts.035261
Cui, C., Han, Y., Li, H., Yu, H., Zhang, B., and Li, G. (2022). Curcumin-driven reprogramming of the gut microbiota and metabolome ameliorates motor deficits and neuroinflammation in a mouse model of Parkinson's disease. Front. Cell Infect. Microbiol. 12, 887407. doi:10.3389/fcimb.2022.887407
Dang, G., Wu, W., Zhang, H., and Everaert, N. (2021). A new paradigm for a new simple chemical: butyrate and immune regulation. Food Funct. 12 (24), 12181–12193. doi:10.1039/d1fo02116h
de Toro-Martín, J., Arsenault, B. J., Després, J. P., and Vohl, M. C. (2017). Precision nutrition: a review of personalized nutritional approaches for the prevention and management of metabolic syndrome. Nutrients 9 (8), 913. doi:10.3390/nu9080913
Dong, Y., Fan, H., Zhang, Z., Jiang, F., Li, M., Zhou, H., et al. (2022). Berberine ameliorates DSS-induced intestinal mucosal barrier dysfunction through microbiota-dependence and Wnt/β-catenin pathway. Int. J. Biol. Sci. 18 (4), 1381–1397. doi:10.7150/ijbs.65476
Farhadi, A., Banan, A., Fields, J., and Keshavarzian, A. (2003). Intestinal barrier: an interface between health and disease. J. Gastroenterol. Hepatol. 18 (5), 479–497. doi:10.1046/j.1440-1746.2003.03032.x
Farias, D. de P. A., FernandesNeri-Numa, F., AngelicaPastore, I., and Maria, G. (2019). Prebiotics: trends in food, health and technological applications. Trends Food Sci. and Technol., 93. doi:10.1016/j.tifs.2019.09.004
Fu Yong-wei, (2022) “Study on the preparation of functional food based on polygonatum and euryale ferox and euryale ferox and the improvement of Gastrointestinal function,”. Chongqing: Chongqing Technology and Business University. doi:10.27713/d.cnki.gcqgs.2022.000641
Gan, X. F., Wei, G. L., Li, T. T., Qu, Z. Y., Xu, S., He, M., et al. (2019). Study on content of polysaccharide during processing of polygonatum sibiricum by RSM and PMP-HPLC characteristic map technology. Chin. Traditional Herb. Drugs 50, 4932–4941. doi:10.7501/j.issn.0253-2670.2019.20.014
Ghosh, S., Whitley, C. S., Haribabu, B., and Jala, V. R. (2021). Regulation of intestinal barrier function by microbial metabolites. Cell Mol. Gastroenterol. Hepatol. 11 (5), 1463–1482. doi:10.1016/j.jcmgh.2021.02.007
Gong, C. X., Ma, C., Irge, D. D., Li, S. M., Chen, S. M., Zhou, S. X., et al. (2023). Gastrodia elata and parishin ameliorate aging induced 'leaky gut' in mice: correlation with gut microbiota. Biomed. J. 46 (4), 100547. doi:10.1016/j.bj.2022.07.001
Gong, H., Gan, X., Qin, B., Chen, J., Zhao, Y., Qiu, B., et al. (2024). Structural characteristics of steamed Polygonatum cyrtonema polysaccharide and its bioactivity on colitis via improving the intestinal barrier and modifying the gut microbiota. Carbohydr. Polym. 327, 121669. doi:10.1016/j.carbpol.2023.121669
Groschwitz, K. R., and Hogan, S. P. (2009). Intestinal barrier function: molecular regulation and disease pathogenesis. J. Allergy Clin. Immunol. 124 (1), 3–20. doi:10.1016/j.jaci.2009.05.038
Guarino, M. P. L., Altomare, A., Emerenziani, S., Di Rosa, C., Ribolsi, M., Balestrieri, P., et al. (2020). Mechanisms of action of prebiotics and their effects on gastro-intestinal disorders in adults. Nutrients 12 (4), 1037. doi:10.3390/nu12041037
Guo, Y. Y. (2023) “Preparation technology of polygonatum cyrtonema Hua polysaccharide buccal tablets and evaluation of its intestinal flora regulation and health care function,”. China: Chengdu University. doi:10.27917/d.cnki.gcxdy.2023.000331
Hansson, G. C., and Johansson, M. E. (2010). The inner of the two Muc2 mucin-dependent mucus layers in colon is devoid of bacteria. Gut Microbes 1 (1), 51–54. doi:10.4161/gmic.1.1.10470
He, L., Yan, B., Yao, C., Chen, X., Li, L., Wu, Y., et al. (2021). Oligosaccharides from polygonatum cyrtonema hua: structural characterization and treatment of LPS-induced peritonitis in mice. Carbohydr. Polym. 255, 117392. doi:10.1016/j.carbpol.2020.117392
He, Y., Huang, L., Jiang, P., Xu, G., and Sun, T. (2022). Immunological regulation of the active fraction from Polygonatum sibiricum F. Delaroche based on improvement of intestinal microflora and activation of RAW264.7 cells. J. Ethnopharmacol. 293, 115240. doi:10.1016/j.jep.2022.115240
Hill, C., Guarner, F., Reid, G., Gibson, G. R., Merenstein, D. J., Pot, B., et al. (2014). Expert consensus document. The International Scientific Association for Probiotics and Prebiotics consensus statement on the scope and appropriate use of the term probiotic. Nat. Rev. Gastroenterol. Hepatol. 11 (8), 506–514. doi:10.1038/nrgastro.2014.66
Hsieh, C. Y., Osaka, T., Moriyama, E., Date, Y., Kikuchi, J., and Tsuneda, S. (2015). Strengthening of the intestinal epithelial tight junction by Bifidobacterium bifidum. Physiol. Rep. 3 (3), e12327. doi:10.14814/phy2.12327
Hu, J., Huang, H., Che, Y., Ding, C., Zhang, L., Wang, Y., et al. (2021). Qingchang Huashi Formula attenuates DSS-induced colitis in mice by restoring gut microbiota-metabolism homeostasis and goblet cell function. J. Ethnopharmacol. 266, 113394. doi:10.1016/j.jep.2020.113394
Huang, S., Yuan, H., Li, W., Liu, X., Zhang, X., Xiang, D., et al. (2021). Polygonatum sibiricum polysaccharides protect against MPP-induced neurotoxicity via the akt/mTOR and Nrf2 pathways. Oxid. Med. Cell Longev. 2021, 8843899. doi:10.1155/2021/8843899
Huang, X., Chen, Q., Fan, Y., Yang, R., Gong, G., Yan, C., et al. (2023). Fructooligosaccharides attenuate non-alcoholic fatty liver disease by remodeling gut microbiota and association with lipid metabolism. Biomed. Pharmacother. 159, 114300. doi:10.1016/j.biopha.2023.114300
Kaminsky, L. W., Al-Sadi, R., and Ma, T. Y. (2021). IL-1β and the intestinal epithelial tight junction barrier. Front. Immunol. 12, 767456. doi:10.3389/fimmu.2021.767456
Kayama, H., Okumura, R., and Takeda, K. (2020). Interaction between the microbiota, epithelia, and immune cells in the intestine. Annu. Rev. Immunol. 38, 23–48. doi:10.1146/annurev-immunol-070119-115104
Kesika, P., Suganthy, N., Sivamaruthi, B. S., and Chaiyasut, C. (2021). Role of gut-brain axis, gut microbial composition, and probiotic intervention in Alzheimer's disease. Life Sci. 264, 118627. doi:10.1016/j.lfs.2020.118627
Khoshbin, K., and Camilleri, M. (2020). Effects of dietary components on intestinal permeability in health and disease. Am. J. Physiol. Gastrointest. Liver Physiol. 319 (5), G589–G608. doi:10.1152/ajpgi.00245.2020
Lau, E., Marques, C., Pestana, D., Santoalha, M., Carvalho, D., Freitas, P., et al. (2016). The role of I-FABP as a biomarker of intestinal barrier dysfunction driven by gut microbiota changes in obesity. Nutr. Metab. (Lond) 13, 31. doi:10.1186/s12986-016-0089-7
Lechin, F., and van der Dijs, B. (2009). Central nervous system plus autonomic nervous system disorders responsible for gastrointestinal and pancreatobiliary diseases. Dig. Dis. Sci. 54 (3), 458–470. doi:10.1007/s10620-008-0369-9
Lewis, C. V., and Taylor, W. R. (2020). Intestinal barrier dysfunction as a therapeutic target for cardiovascular disease. Am. J. Physiol. Heart Circ. Physiol. 319 (6), H1227–H1233. doi:10.1152/ajpheart.00612.2020
Li, D., Tang, W., Wang, Y., Gao, Q., Zhang, H., Zhang, Y., et al. (2023b). An overview of traditional Chinese medicine affecting gut microbiota in obesity. Front. Endocrinol. (Lausanne) 14, 1149751. doi:10.3389/fendo.2023.1149751
Li, L. X., Feng, X., Tao, M. T., Paulsen, B. S., Huang, C., Feng, B., et al. (2022a). Benefits of neutral polysaccharide from rhizomes of Polygonatum sibiricum to intestinal function of aged mice. Front. Nutr. 9, 992102. doi:10.3389/fnut.2022.992102
Li, R., Tao, A., Yang, R., Fan, M., Zhang, X., Du, Z., et al. (2020). Structural characterization, hypoglycemic effects and antidiabetic mechanism of a novel polysaccharides from Polygonatum kingianum Coll. et Hemsl. Biomed. Pharmacother. 131, 110687. doi:10.1016/j.biopha.2020.110687
Li, X. L., Ma, R. H., Zhang, F., Ni, Z. J., Thakur, K., Wang, S., et al. (2023a). Evolutionary research trend of Polygonatum species: a comprehensive account of their transformation from traditional medicines to functional foods. Crit. Rev. Food Sci. Nutr. 63 (19), 3803–3820. doi:10.1080/10408398.2021.1993783
Li, Z., Lin, M., Li, Y., Shao, J., Huang, R., Qiu, Y., et al. (2022b). Total flavonoids of Sophora flavescens and kurarinone ameliorated ulcerative colitis by regulating Th17/Treg cell homeostasis. J. Ethnopharmacol. 297, 115500. doi:10.1016/j.jep.2022.115500
Li-ping, H., Long-hui, L., Xi-yang, Y., Yong-jue, X., wei, X.-Z., Xu-dong, Z., et al. (2023). Exploring the effects and mechanisms of Erjingwan on cognitive functions of AD model rats based on intestinal flora. Pharmacol. Clin. Chin. Med. 39 (09), 2–8. doi:10.13412/j.cnki.zyyl.20230524.003
Liu, F. Y., Wen, J., Hou, J., Zhang, S. Q., Sun, C. B., Zhou, L. C., et al. (2021b). Gastrodia remodels intestinal microflora to suppress inflammation in mice with early atherosclerosis. Int. Immunopharmacol. 96, 107758. doi:10.1016/j.intimp.2021.107758
Liu, G., Yu, Q., Zhu, H., Tan, B., Yu, H., Li, X., et al. (2023b). Amyloid-β mediates intestinal dysfunction and enteric neurons loss in Alzheimer's disease transgenic mouse. Cell Mol. Life Sci. 80 (12), 351. doi:10.1007/s00018-023-04948-9
Liu, L., Wang, H., Chen, X., Zhang, Y., Zhang, H., and Xie, P. (2023c). Gut microbiota and its metabolites in depression: from pathogenesis to treatment. EBioMedicine 90, 104527. doi:10.1016/j.ebiom.2023.104527
Liu, Q., Liu, S., Cao, H., Ji, W., Li, C., Huan, Y., et al. (2021a). Ramulus mori (sangzhi) alkaloids (SZ-A) ameliorate glucose metabolism accompanied by the modulation of gut microbiota and ileal inflammatory damage in type 2 diabetic KKAy mice. Front. Pharmacol. 12, 642400. doi:10.3389/fphar.2021.642400
Liu, Q., Yu, Z., Tian, F., Zhao, J., Zhang, H., Zhai, Q., et al. (2020b). Surface components and metabolites of probiotics for regulation of intestinal epithelial barrier. Microb. Cell Fact. 19 (1), 23. doi:10.1186/s12934-020-1289-4
Liu, R., Zhang, X., Cai, Y., Xu, S., Xu, Q., Ling, C., et al. (2024). Research progress on medicinal components and pharmacological activities of polygonatum sibiricum. J. Ethnopharmacol. 328, 118024. doi:10.1016/j.jep.2024.118024
Liu, S., Jia, Q. J., Peng, Y. Q., Feng, T. H., Hu, S. T., Dong, J. E., et al. (2022a). Advances in mechanism research on polygonatum in prevention and treatment of diabetes. Front. Pharmacol. 13, 758501. doi:10.3389/fphar.2022.758501
Liu, T. Y., Zhao, L. L., Chen, S. B., Hou, B. C., Huang, J., Hong, X., et al. (2020a). Polygonatum sibiricum polysaccharides prevent LPS-induced acute lung injury by inhibiting inflammation via the TLR4/Myd88/NF-κB pathway. Exp. Ther. Med. 20 (4), 3733–3739. doi:10.3892/etm.2020.9097
Liu, W., Shao, T., Tian, L., Ren, Z., Gao, L., Tang, Z., et al. (2022b). Structural elucidation and anti-nonalcoholic fatty liver disease activity of Polygonatum cyrtonema Hua polysaccharide. Food Funct. 13 (24), 12883–12895. PMID: 36441628. doi:10.1039/d2fo03384d
Liu, Y., Tang, Z., He, P., Peng, T., Liu, X., Peng, T., et al. (2023a). Recovery and regulatio no fIntestinal microflorain ratswith antibiotic-associated diarrhea by compatibility of polygonatum. Asia-Pacific Tradit. Med. 19 (09), 27–34.
Liu, Y. P. (2023). Mechanism of polygonatum cyrtonema polysaccharide in reducing nutritional obesity by regulating intestinal flora [dissertation/master's thesis]. Hefei(AH): Anhui University of Chinese Medicine. doi:10.26922/d.cnki.ganzc.2023.000484
Liu, Z. (2023). Discussion on the anti-brain aging effect and mechanism of polysaccharides of Polygonatum sibiricum Polysaccharide based on intestinal flora and metabolomics [dissertation/master's thesis].Zhengzhou(HN). Hunan Univ. Chin. Med. doi:10.27138/d.cnki.ghuzc.2023.000454
Long, T., Liu, Z., Shang, J., Zhou, X., Yu, S., Tian, H., et al. (2018). Polygonatum sibiricum polysaccharides play anti-cancer effect through TLR4-MAPK/NF-κB signaling pathways. Int. J. Biol. Macromol. 111, 813–821. doi:10.1016/j.ijbiomac.2018.01.070
Luo, S., Zhang, X., Huang, S., Feng, X., Zhang, X., and Xiang, D. (2022). A monomeric polysaccharide from Polygonatum sibiricum improves cognitive functions in a model of Alzheimer's disease by reshaping the gut microbiota. Int. J. Biol. Macromol. 213, 404–415. doi:10.1016/j.ijbiomac.2022.05.185
Ma, S., Yeom, J., and Lim, Y. H. (2022). Specific activation of hypoxia-inducible factor-2α by propionate metabolism via a β-oxidation-like pathway stimulates MUC2 production in intestinal goblet cells. Biomed. Pharmacother. 155, 113672. doi:10.1016/j.biopha.2022.113672
Ma, W., Wei, S., Peng, W., Sun, T., Huang, J., Yu, R., et al. (2021). Antioxidant effect of polygonatum sibiricum polysaccharides in D-galactose-induced heart aging mice. Biomed. Res. Int. 2021, 6688855. doi:10.1155/2021/6688855
Martin-Gallausiaux, C., Marinelli, L., Blottière, H. M., Larraufie, P., and Lapaque, N. (2021)). SCFA: mechanisms and functional importance in the gut. Proc. Nutr. Soc. 80 (1), 37–49. doi:10.1017/S0029665120006916
Martino, M., Rocchi, G., Escelsior, A., and Fornaro, M. (2012). Immunomodulation mechanism of antidepressants: interactions between serotonin/norepinephrine balance and Th1/Th2 balance. Curr. Neuropharmacol. 10 (2), 97–123. doi:10.2174/157015912800604542
Martin-Subero, M., Anderson, G., Kanchanatawan, B., Berk, M., and Maes, M. (2016). Comorbidity between depression and inflammatory bowel disease explained by immune-inflammatory, oxidative, and nitrosative stress; tryptophan catabolite; and gut-brain pathways. CNS Spectr. 21 (2), 184–198. doi:10.1017/S1092852915000449
Matia-Garcia, I., Vadillo, E., Pelayo, R., Muñoz-Valle, J. F., García-Chagollán, M., Loaeza-Loaeza, J., et al. (2021). Th1/Th2 balance in young subjects: relationship with cytokine levels and metabolic profile. J. Inflamm. Res. 14, 6587–6600. doi:10.2147/JIR.S342545
Mayer, E. A., Nance, K., and Chen, S. (2022). The gut-brain Axis. Annu. Rev. Med. 73, 439–453. doi:10.1146/annurev-med-042320-014032
Medina-Vera, I., Sanchez-Tapia, M., Noriega-López, L., Granados-Portillo, O., Guevara-Cruz, M., Flores-López, A., et al. (2019). A dietary intervention with functional foods reduces metabolic endotoxaemia and attenuates biochemical abnormalities by modifying faecal microbiota in people with type 2 diabetes. Diabetes Metab. 45 (2), 122–131. doi:10.1016/j.diabet.2018.09.004
Mekonnen, S. A., Merenstein, D., Fraser, C. M., and Marco, M. L. (2020). Molecular mechanisms of probiotic prevention of antibiotic-associated diarrhea. Curr. Opin. Biotechnol. 61, 226–234. doi:10.1016/j.copbio.2020.01.005
Meng, Q., Xu, Y., Li, Y., and Wang, Y. (2023). Novel studies on Drosophila melanogaster model reveal the roles of JNK-Jak/STAT axis and intestinal microbiota in insulin resistance. J. Drug Target 31 (3), 261–268. doi:10.1080/1061186X.2022.2144869
Mengting, T. (2017). The mechanism of action of polysaccharides from Polygonatum sibiricumin the treatment of COPD by regulating Th17/Treg balance based on the gut-lung axis. Yaan(YA): Sichuan Agricultural University. [dissertation/master’s thesis]
Ming-chen, Y., Meng-xin, Y., Lu, W., Yi-hong, B., and Yang-yang, C. (2021). In vitro digestion properties of polygonatum sibiricum polysaccharide and its regulatory action on the gut microbiota in T2DM mice. Mod. Food Sci. Technol. 37 (08), 14–21. doi:10.13982/j.mfst.1673-9078.2021.8.1181
Mohanty, S. S. P. S. (2018). Prebiotics and synbiotics: recent concepts in nutrition. Food Biosci., 26.
Ou, X., Chen, J., Li, B., Yang, Y., Liu, X., Xu, Z., et al. (2024). Multiomics reveals the ameliorating effect and underlying mechanism of aqueous extracts of polygonatum sibiricum rhizome on obesity and liver fat accumulation in high-fat diet-fed mice. Phytomedicine 132, 155843. doi:10.1016/j.phymed.2024.155843
Peng, Y., Huang, Y., Li, H., Li, C., Wu, Y., Wang, X., et al. (2024). Associations between rheumatoid arthritis and intestinal flora, with special emphasis on RA pathologic mechanisms to treatment strategies. Microb. Pathog. 188, 106563. doi:10.1016/j.micpath.2024.106563
Pengrattanachot, N., Thongnak, L., and Lungkaphin, A. (2022). The impact of prebiotic fructooligosaccharides on gut dysbiosis and inflammation in obesity and diabetes related kidney disease. Food Funct. 13 (11), 5925–5945. doi:10.1039/d1fo04428a
Peterson, L. W., and Artis, D. (2014). Intestinal epithelial cells: regulators of barrier function and immune homeostasis. Nat. Rev. Immunol. 14 (3), 141–153. doi:10.1038/nri3608
Sabater-Molina, M., Larqué, E., Torrella, F., and Zamora, S. (2009). Dietary fructooligosaccharides and potential benefits on health. J. Physiol. Biochem. 65 (3), 315–328. doi:10.1007/BF03180584
Sarrabayrouse, G., Alameddine, J., Altare, F., and Jotereau, F. (2015). Microbiota-specific CD4CD8αα tregs: role in intestinal immune homeostasis and implications for IBD. Front. Immunol. 6, 522. doi:10.3389/fimmu.2015.00522
Serek, P., and Oleksy-Wawrzyniak, M. (2021). The effect of bacterial infections, probiotics and zonulin on intestinal barrier integrity. Int. J. Mol. Sci. 22 (21), 11359. doi:10.3390/ijms222111359
Shen, F., Song, Z., Xie, P., Li, L., Wang, B., Peng, D., et al. (2021). Polygonatum sibiricum polysaccharide prevents depression-like behaviors by reducing oxidative stress, inflammation, and cellular and synaptic damage. J. Ethnopharmacol. 275, 114164. doi:10.1016/j.jep.2021.114164
Shen, X., Xie, A., Li, Z., Jiang, C., Wu, J., Li, M., et al. (2024). Research progress for probiotics regulating intestinal flora to improve functional dyspepsia: a review. Foods 13 (1), 151. doi:10.3390/foods13010151
Shi, Y., Si, D., Chen, D., Zhang, X., Han, Z., Yu, Q., et al. (2023). Bioactive compounds from Polygonatum genus as anti-diabetic agents with future perspectives. Food Chem. 408, 135183. doi:10.1016/j.foodchem.2022.135183
Si, J., Qiu, Y., Sun, Y., Liu, J., Chen, D., Yan, S., et al. Development strategy of huangjing industry strategic study of CAE (2024) 1–8. doi:10.15302/J-SSCAE-2023.07.035
Silva, R. S. D., Mendonça, I. P., Paiva, I. H. R., Souza, J. R. B., and Peixoto, C. A. (2023). Fructooligosaccharides and galactooligosaccharides improve hepatic steatosis via gut microbiota-brain axis modulation. Int. J. Food Sci. Nutr. 74 (7), 760–780. doi:10.1080/09637486.2023.2262779
Singh, R. S., Singh, R. P., and Kennedy, J. F. (2016). Recent insights in enzymatic synthesis of fructooligosaccharides from inulin. Int. J. Biol. Macromol. 85, 565–572. Epub 2016 Jan 11. doi:10.1016/j.ijbiomac.2016.01.026
Song, M. (2023). Exploring the intervention effect of sangge huazhuo decoction on prediabetes based on the intestinal flora endotoxin mechanism [dissertation/master’s thesis].Jinan(SD). Shandong Univ. Chin. Med. doi:10.27282/d.cnki.gsdzu.2023.000985
Su, J., Wang, Y., Yan, M., He, Z., Zhou, Y., Xu, J., et al. (2022). The beneficial effects of Polygonatum sibiricum Red. superfine powder on metabolic hypertensive rats via gut-derived LPS/TLR4 pathway inhibition. Phytomedicine 106, 154404. doi:10.1016/j.phymed.2022.154404
Su, L. L., Li, X., Guo, Z. J., Xiao, X. Y., Chen, P., Zhang, J. B., et al. (2023). Effects of different steaming times on the composition, structure and immune activity of Polygonatum Polysaccharide. J. Ethnopharmacol. 310, 116351. doi:10.1016/j.jep.2023.116351
Sun, J., Liu, S., Ling, Z., Wang, F., Ling, Y., Gong, T., et al. (2019). Fructooligosaccharides ameliorating cognitive deficits and neurodegeneration in APP/PS1 transgenic mice through modulating gut microbiota. J. Agric. Food Chem. 67 (10), 3006–3017. doi:10.1021/acs.jafc.8b07313
Takiishi, T., Fenero, C. I. M., and Câmara, N. O. S. (2017). Intestinal barrier and gut microbiota: shaping our immune responses throughout life. Tissue Barriers 5 (4), e1373208. doi:10.1080/21688370.2017.1373208
Tang, G., Du, Y., Guan, H., Jia, J., Zhu, N., Shi, Y., et al. (2022). Butyrate ameliorates skeletal muscle atrophy in diabetic nephropathy by enhancing gut barrier function and FFA2-mediated PI3K/Akt/mTOR signals. Br. J. Pharmacol. 179 (1), 159–178. doi:10.1111/bph.15693
Tao, M. T., Li, L. X., Wang, H. Y., Peng, Y. M., Huang, X. Y., and Zhao, Y. Z. (2023). Comparison of polysaccharide components and anti-inflammatory and antioxidant effects of Huangjing, Duohua Huangjing, and Dianhuangjing. Chin. Tradit. Pat. Med. 45 (04), 1367–1372. doi:10.3969/j.issn.1001-1528.2023.04.059
Vanuytsel, T., Bercik, P., and Boeckxstaens, G. (2023). Understanding neuroimmune interactions in disorders of gut-brain interaction: from functional to immune-mediated disorders. Gut 72 (4), 787–798. doi:10.1136/gutjnl-2020-320633
Wang, F. Y. (2017). Effects and mechanisms of polysaccharide from Polygonatum kingianum on lipid metabolism disorder in rats[dissertation/master's thesis]. Kunming(YN): Yunnan University of Traditional Chinese Medicine.
Wang, G., Liu, Z., Liang, D., Yu, J., Wang, T., Zhou, F., et al. (2022a). Aqueous extract of Polygonatum sibiricum ameliorates glucose and lipid metabolism via PI3K/AKT signaling pathway in high-fat diet and streptozotocin-induced diabetic mice. J. Food Biochem. 46 (12), e14402. doi:10.1111/jfbc.14402
Wang, J., Wang, F., Yuan, L., Ruan, H., Zhu, Z., Fan, X., et al. (2022b). Blood-enriching effects and immune-regulation mechanism of steam-processed polygonatum sibiricum polysaccharide in blood deficiency syndrome mice. Front. Immunol. 13, 813676. doi:10.3389/fimmu.2022.813676
Wang, S., Li, L., Chen, Y., Liu, Q., Zhou, S., Li, N., et al. (2023a). Houttuynia cordata thunb. alleviates inflammatory bowel disease by modulating intestinal microenvironment: a research review. Front. Immunol. 14, 1306375. doi:10.3389/fimmu.2023.1306375
Wang, X., Li, X., Zhang, L., An, L., Guo, L., Huang, L., et al. (2023b). Recent progress in plant-derived polysaccharides with prebiotic potential for intestinal health by targeting gut microbiota: a review. Crit. Rev. Food Sci. Nutr. 31, 1–30. doi:10.1080/10408398.2023.2248631
Wang, Y., Dilidaxi, D., Wu, Y., Sailike, J., Sun, X., and Nabi, X. H. (2020). Composite probiotics alleviate type 2 diabetes by regulating intestinal microbiota and inducing GLP-1 secretion in db/db mice. Biomed. Pharmacother. 125, 109914. doi:10.1016/j.biopha.2020.109914
Wang, Y., Fei, Y., Liu, L., Xiao, Y., Pang, Y., Kang, J., et al. (2018). Polygonatum odoratum polysaccharides modulate gut microbiota and mitigate experimentally induced obesity in rats. Int. J. Mol. Sci. 19 (11), 3587. doi:10.3390/ijms19113587
Woda, A., Picard, P., and Dutheil, F. (2016). Dysfunctional stress responses in chronic pain. Psychoneuroendocrinology 71, 127–135. doi:10.1016/j.psyneuen.2016.05.017
Wu, J., Yang, Y., Liu, L., Zhu, W., Liu, M., Yu, X., et al. (2023). ROS-AMPK/mTOR-dependent enterocyte autophagy is involved in the regulation of Giardia infection-related tight junction protein and nitric oxide levels. Front. Immunol. 14, 1120996. doi:10.3389/fimmu.2023.1120996
Wu, Y., Tang, L., Wang, B., Sun, Q., Zhao, P., and Li, W. The role of autophagy in maintaining intestinal epithelial barrier. J. Cell Physiol. 20 (11), 19406–19419. doi:10.1002/jcp.28722
Wyss-Coray, T. (2016). Ageing, neurodegeneration and brain rejuvenation. Nature 539 (7628), 180–186. doi:10.1038/nature20411
Xia, W., Khan, I., Li, X. A., Huang, G., Yu, Z., Leong, W. K., et al. (2020). Adaptogenic flower buds exert cancer preventive effects by enhancing the SCFA-producers, strengthening the epithelial tight junction complex and immune responses. Pharmacol. Res. 159, 104809. doi:10.1016/j.phrs.2020.104809
Xiao, L., Qi, L., Zhang, G., Liu, H., Gu, Y., Zhang, L., et al. (2022). Polygonatum sibiricum polysaccharides attenuate lipopoly-saccharide-induced septic liver injury by suppression of pyroptosis via NLRP3/GSDMD signals. Molecules 27 (18), 5999. doi:10.3390/molecules27185999
Xie, Y., Mu, C., Kazybay, B., Sun, Q., Kutzhanova, A., Nazarbek, G., et al. (2021). Network pharmacology and experimental investigation of Rhizoma polygonati extract targeted kinase with herbzyme activity for potent drug delivery. Drug Deliv. 28 (1), 2187–2197. doi:10.1080/10717544.2021.1977422
Xu, J., Tang, C., Din, A. U., Lu, Y., Ma, X., Zhang, T., et al. (2023b). Oligosaccharides of Polygonatum Cyrtonema Hua ameliorates dextran sulfate sodium-induced colitis and regulates the gut microbiota. Biomed. Pharmacother. 161, 114562. doi:10.1016/j.biopha.2023.114562
Xu, Ma, Yu, J., Liu, Y., and Lei, Z. (2024). Clinical application of polygonatum sibiricum to tonify kidney qi in the identification and treatment of hypertension in the elderly. J. Liaoning Univ. Traditional Chin. Med., 1–23. Available at: http://kns.cnki.net/kcms/detail/21.1543.r.20240516.1520.004.html.
Xu, Y., Guo, Y., Lu, C., Yu, L., Fang, C., and Li, C. (2023a). Polygonatum sibiricum polysaccharide inhibited liver cancer in a simulated tumor microenvironment by eliminating TLR4/STAT3 pathway. Biol. Pharm. Bull. 46 (9), 1249–1259. doi:10.1248/bpb.b23-00198
Xu, Y., Wu, Y. J., Sun, P. L., Zhang, F. M., Linhardt, R. J., and Zhang, A. Q. (2019). Chemically modified polysaccharides: synthesis, characterization, structure activity relationships of action. Int. J. Biol. Macromol. 132, 970–977. doi:10.1016/j.ijbiomac.2019.03.213
Xu, Y., Ye, Y., Liu, C., Chen, B., Ji, J., Sun, J., et al. (2023c). Positive effects of steamed Polygonatum sibiricum polysaccharides including a glucofructan on fatty acids and intestinal microflora. Food Chem. 402, 134068. doi:10.1016/j.foodchem.2022.134068
Yang, S., Hu, T., Liu, H., Lv, Y. L., Zhang, W., Li, H., et al. (2021). Akebia saponin D ameliorates metabolic syndrome (MetS) via remodeling gut microbiota and attenuating intestinal barrier injury. Biomed. Pharmacother. 138, 111441. doi:10.1016/j.biopha.2021.111441
Yang, X., Yu, D., Xue, L., Li, H., and Du, J. (2020). Probiotics modulate the microbiota-gut-brain axis and improve memory deficits in aged SAMP8 mice. Acta Pharm. Sin. B 10 (3), 475–487. doi:10.1016/j.apsb.2019.07.001
Yao-Yao, D., Tao, Z., Yu-shuang, Q., and Peng, W. (2022). Research progress of taishan rhizoma polygonati. China Fruit and Veg. 42 (02), 21–28. doi:10.19590/j.cnki.1008-1038.2022.02.004
Yue, B., Zong, G., Tao, R., Wei, Z., and Lu, Y. (2022). Crosstalk between traditional Chinese medicine-derived polysaccharides and the gut microbiota: a new perspective to understand traditional Chinese medicine. Phytother. Res. 36 (11), 4125–4138. doi:10.1002/ptr.7607
Zhang, H., Cao, Y., Chen, L., Wang, J., Tian, Q., Wang, N., et al. (2015). A polysaccharide from Polygonatum sibiricum attenuates amyloid-β-induced neurotoxicity in PC12 cells. Carbohydr. Polym. 117, 879–886. doi:10.1016/j.carbpol.2014.10.034
Zhang, H. Y., Tian, J. X., Lian, F. M., Li, M., Liu, W. K., Zhen, Z., et al. (2021b). Therapeutic mechanisms of traditional Chinese medicine to improve metabolic diseases via the gut microbiota. Biomed. Pharmacother. 133, 110857. doi:10.1016/j.biopha.2020.110857
Zhang, J., Chen, H., Luo, L., Zhou, Z., Wang, Y., Gao, T., et al. (2021a). Structures of fructan and galactan from Polygonatum cyrtonema and their utilization by probiotic bacteria. Carbohydr. Polym. 267, 118219. doi:10.1016/j.carbpol.2021.118219
Zhang, X., Ni, L., Hu, S., Yue, B., Chen, X., Yuan, D., et al. (2022). Polygonatum sibiricum ameliorated cognitive impairment of naturally aging rats through BDNF-TrkB signaling pathway. J. Food Biochem. 46 (12), e14510. doi:10.1111/jfbc.14510
Zhang, Y., Sun, Y., Liu, Y., Liu, J., Sun, J., Liu, X., et al. (2023). Polygonum sibiricum polysaccharides exert the antidepressant-like effects in chronic unpredictable mild stress-induced depressive mice by modulating microbiota-gut-brain axis. Phytother. Res. 37 (8), 3408–3423. doi:10.1002/ptr.7813
Zhang, Z., Bao, Z. Y., Sun, J. J., Wei, G., and Yang, K. X. (2021c). The effect of fermented Huangjing polysaccharide on the gut microbiota of obese mice. J. South China Univ. Technol. Nat. Sci. Ed. 49 (03), 95–105+113. in China. doi:10.12141/j.issn.1000-565X.200219
Zhao, P., Zhao, C., Li, X., Gao, Q., Huang, L., Xiao, P., et al. (2018). The genus Polygonatum: a review of ethnopharmacology, phytochemistry and pharmacology. J. Ethnopharmacol. 214, 274–291. doi:10.1016/j.jep.2017.12.006
Zheng, Y., Chen, M., Zhang, Y., Wang, G., and Zhao, H. (2023). Lead exposure disrupted ileal barrier of developmental Japanese quails(Coturnix japonica): histopathological damages, microbiota dysbiosis and immune disorder. Ecotoxicol. Environ. Saf. 264, 115488. doi:10.1016/j.ecoenv.2023.115488
Zhou, B., Yuan, Y., Zhang, S., Guo, C., Li, X., Li, G., et al. (2020). Intestinal flora and disease mutually shape the regional immune system in the intestinal tract. Front. Immunol. 11, 575. doi:10.3389/fimmu.2020.00575
Zhou, Z., Wang, D., Xu, X., Dai, J., Lao, G., Zhang, S., et al. (2023). Myofibrillar protein-chlorogenic acid complexes ameliorate glucose metabolism via modulating gut microbiota in a type 2 diabetic rat model. Food Chem. 409, 135195. doi:10.1016/j.foodchem.2022.135195
Zou, Y., Yan, H., Li, C., Wen, F., Jize, X., Zhang, C., et al. (2023). A pectic polysaccharide from codonopsis pilosula alleviates inflammatory response and oxidative stress of aging mice via modulating intestinal microbiota-related gut-liver Axis. Antioxidants (Basel) 12 (9), 1781. doi:10.3390/antiox12091781
Keywords: Polygonatum sibiricum, Polygonatum sibiricum polysaccharide, homology of medicine and food, intestinal mucosal barrier, short-chain fatty acids
Citation: Ren Y, Sun Y, Liao Y-Y, Wang S, Liu Q, Duan C-Y, Sun L, Li X-Y and Yuan J-L (2024) Mechanisms of action and applications of Polygonatum sibiricum polysaccharide at the intestinal mucosa barrier: a review. Front. Pharmacol. 15:1421607. doi: 10.3389/fphar.2024.1421607
Received: 22 April 2024; Accepted: 01 August 2024;
Published: 19 August 2024.
Edited by:
Gang Chen, Shenyang Pharmaceutical University, ChinaCopyright © 2024 Ren, Sun, Liao, Wang, Liu, Duan, Sun, Li and Yuan. This is an open-access article distributed under the terms of the Creative Commons Attribution License (CC BY). The use, distribution or reproduction in other forums is permitted, provided the original author(s) and the copyright owner(s) are credited and that the original publication in this journal is cited, in accordance with accepted academic practice. No use, distribution or reproduction is permitted which does not comply with these terms.
*Correspondence: Xiao-Ya Li, bGl4aWFveWFAeW51Y20uZWR1LmNu; Jia-Li Yuan, Mjc0ODEzMjgwMEBxcS5jb20=
†These authors have contributed equally to this work and share first authorship