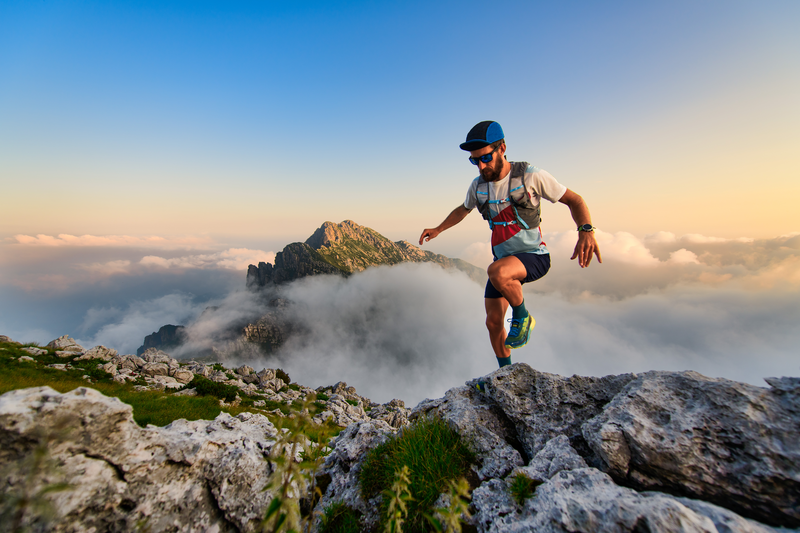
94% of researchers rate our articles as excellent or good
Learn more about the work of our research integrity team to safeguard the quality of each article we publish.
Find out more
ORIGINAL RESEARCH article
Front. Pharmacol. , 22 August 2024
Sec. Ethnopharmacology
Volume 15 - 2024 | https://doi.org/10.3389/fphar.2024.1386604
This article is part of the Research Topic Quality control for Efficacy and Safety of Herbal Medicinal Products: Volume II View all 12 articles
Introduction: Increasing evidence shows that hyperactive aryl hydrocarbon receptor (AHR) signalling is involved in renal disease. However, no currently available intervention strategy is effective in halting disease progression by targeting the AHR signalling. Our previous study showed that barleriside A (BSA), a major component of Plantaginis semen, exhibits renoprotective effects.
Methods: In this study, we determined the effects of BSA on AHR expression in 5/6 nephrectomized (NX) rats. We further determined the effect of BSA on AHR, nuclear factor kappa B (NF-ƙB), and the nuclear factor erythroid 2-related factor 2 (Nrf2) signalling cascade in zymosan-activated serum (ZAS)-stimulated MPC5 cells.
Results: BSA treatment improved renal function and inhibited intrarenal nuclear AHR protein expression in NX-treated rats. BSA mitigated podocyte lesions and suppressed AHR mRNA and protein expression in ZAS-stimulated MPC5 cells. BSA inhibited inflammation by improving the NF-ƙB and Nrf2 pathways in ZAS-stimulated MPC5 cells. However, BSA did not markedly upregulate the expression of podocyte-specific proteins in the ZAS-mediated MPC5 cells treated with CH223191 or AHR siRNA compared to untreated ZAS-induced MPC5 cells. Similarly, the inhibitory effects of BSA on nuclear NF-ƙB p65, Nrf2, and AHR, as well as cytoplasmic cyclooxygenase-2, heme oxygenase-1, and AHR, were partially abolished in ZAS-induced MPC5 cells treated with CH223191 or AHRsiRNA compared with untreated ZAS-induced MPC5 cells. These results indicated that BSA attenuated the inflammatory response, partly by inhibiting AHR signalling.
Discussion: Both pharmacological and siNRA findings suggested that BSA mitigated podocyte lesions by improving the NF-ƙB and Nrf2 pathways via inhibiting AHR signalling. Therefore, BSA is a high-affinity AHR antagonist that abolishes oxidative stress and inflammation.
Aryl hydrocarbon receptor (AHR) is a cytoplasmic ligand-mediated transcription factor (Cao et al., 2022; Ouyang et al., 2020). The biological functions of AHR include immune regulation, cell cycle regulation, mucosal barrier function, and organogenesis, which are associated with ligand-mediated receptor activation (da Silva et al., 2022; Ouyang et al., 2020; Wu et al., 2022). AHR can transcribe various drug-metabolizing enzymes including cytochrome P450 family 1 subfamily A member 1 (CYP1A1), cytochrome P450 family 1 subfamily A member 2 (CYP1A2), and cytochrome P450 family 1 subfamily B member 1 (CYP1B1) (Miao et al., 2020). High-affinity AHR ligands have been identified as xenobiotics, such as 2,3,7,8-tetrachlorodibenzo-p-dioxin (Cao et al., 2022). Previous study has suggested that hyperactive AHR signal was implicated in patients with podocyte damage-associated renal disease such as immunoglobulin A nephropathy (IgAN), diabetic kidney disease (DKD) and idiopathic membranous nephropathy (IMN) (Miao et al., 2020). Our previous study showed increased intrarenal AHR mRNA and protein expression in patients with chronic kidney disease (CKD) (Miao et al., 2022). However, no currently available therapy is effective for halting disease progression. Therefore, identification of novel AHR ligands plays a critical role in targeting this enigmatic receptor for the treatment of various diseases.
Mounting evidence suggests that traditional Chinese medicines (TCM) are a key source of new drugs and are used for treatment of various diseases (Newman and Cragg, 2020; Hou et al., 2022; Li, et al., 2022a; Marena et al., 2022; Zhao et al., 2022a; Kong et al., 2023). Natural compounds form TCM were widely used for improving renal function and treating renal injury (Ren et al., 2021; Hu et al., 2022; Zhou et al., 2022; Shao et al., 2023; Sun et al., 2023). Substantial achievements have shown a myriad of natural compounds that can directly modulate AHR signalling (Goya-Jorge et al., 2021). Our previous studies have demonstrated that a number of flavonoids, such as barleriside A (BSA), rhoifolin, 5,7,3′,4′,5′-pentahydroxy flavanone and 5,6,7,8,3′,4′-hexamethoxyflavone, and lignans, including matairesinol and erythro-guaiacylglycerol-β-ferulic acid ether as AHR antagonists, attenuate renal fibrosis by suppressing AHR signalling (Miao et al., 2020; Cao et al., 2022; Miao et al., 2022). In addition, astragaloside IV attenuates renal damage and AHR signalling in mice (Mo et al., 2023). In vitro experiments have shown that astragaloside IV suppresses inflammation and AHR signalling in indoxyl sulfate-treated HK-2 cells (Mo et al., 2023). Moreover, a previous study showed that lycopene mitigated Di (2-ethylhexyl) phthalate-induced renal cell injury by suppressing AHR signal (Li et al., 2021). Collectively, naturally derived compounds, such as AHR inhibitors, attenuated renal fibrosis.
Numerous studies have reported that inflammation plays a central role in CKD (Singh et al., 2022; Wang et al., 2023b; Yuan et al., 2022). Cyclooxygenase-2 (COX-2) is produced by transcription factors including AHR and nuclear factor kappa B (NF-ƙB) p65. Several studies have shown that AHR interacts with NF-ƙB in CKD (Addi et al., 2019; Brito et al., 2019; Curran and Kopp, 2022). Our recent study showed that inhibitor of kappa B (IƙB)/NF-ƙB pathway was a downstream target of AHR signal in IMN (Wang et al., 2023b). However, there are no agents that inhibit NF-ƙB pathway by targeting AHR signalling. Plantaginis semen is widely used as a diuretic to improve renal function and treat renal diseases in patients (Wen et al., 2023a). Our previous study showed that BSA, a major component of P. semen, exhibits renoprotective effects (Miao et al., 2020). In this study, we first determined the effect of BSA on AHR signals in 5/6 nephrectomized (NX) rats. We further revealed that BSA, an AHR antagonist, ameliorated podocyte injury through IƙB/NF-ƙB and kelch-like ECH-associated protein 1 (Keap1)/nuclear factor erythroid 2-related factor 2 (Nrf2) signalling cascade in zymosan activation serum (ZAS)-stimulated MPC5 cells. Our study will uncover that BSA ameliorate podocyte damage-associated renal disease by improving IƙB/NF-ƙB and Keap1/Nrf2 pathways via suppressing hyperactive AHR expression.
Zymosan A was purchased from Sigma–Aldrich (St. Louis, MO, USA). Primary antibodies, Healthy human and Western quick horseradish peroxidase chemiluminescent substrate were presented in the previous publications (Wang et al., 2023).
Male Sprague Dawley rats were purchased from the Animal Center of the Xi’an Jiaotong University (Xi’an, Shaanxi, China). NX rats were reproduced as described in the previous publication (Miao et al., 2020). Rats were divided into three groups: Sham, NX and NX + BSA (n = 8/group). BSA was administered at 10 mg/kg/day by gastric irrigation for 4 weeks from ninth to 12th week. Urine was collected for 24 h after 12 weeks. All rats were euthanized after anesthetization with 10% urethane. Serum and kidney tissue samples were also collected. The animal care and experiments are approved by Ethics Committee for Animal Experiments of University (No. 20200713-06).
Mouse podocyte cell culture was performed as described in the previous publication (Wang et al., 2023a). C5b-9 was prepared as described in our previous study (Wang et al., 2023a). MPC5 cells were stimulated with 10% ZAS for 24 h in the absence or presence of BSA (20 μM) and CH223191 (10 μM). The treated cells were collected.
Creatinine and urea levels in serum were measured using a Beckman AU680 automatic analyzer. Proteinuria was measured using a Roche Cobas C501 Chemistry Analyzer.
Extracted total RNA, quantitative RT-PCR and specific primers were shown in the previous publications (Miao et al., 2020; Cao et al., 2022; Miao et al., 2022).
Kidneys were incubated overnight at 4°C with an anti-AHR primary antibody, and then incubated with a secondary antibody. Analysis was carried out using a light microscope.
MPC5 cells were incubated with antibodies against podocin, AHR, NF-ƙB p65, COX-2, Nrf2, and haem oxygenase-1 (HO-1). Details of the immunofluorescence methods are presented in the previous publication (Wang et al., 2023).
Western blot analysis was performed in the previous publication (Cao et al., 2022). The expression levels were normalized to those of α-tubulin and histone H3. The relative levels were quantified using the ImageJ software.
The experimental results are presented as the mean ± standard error of mean. The statistical significance was analyzed using one-way ANOVA using the GraphPad Prism software. The values for P < 0.05 were considered statistically significant.
Compared to Sham rats, NX rats presented a markedly increase in the serum levels of creatinine and urea, as well as proteinuria levels, while BSA treatment markedly reduced levels of three renal function markers in NX-induced CKD rats (Figure 1A), indicating that BSA improved renal function in NX-induced CKD rats.
Figure 1. BSA inhibits AHR expression in the NX-induced rats. (A) Serum levels of creatinine and urea and proteinuria in the control and NX-induced rats with or without BSA. (B) Cytoplasm and nuclei AHR protein expression in the renal tissues of control and NX-induced rats with or without BSA. (C) Protein levels of cytoplasm and nuclei AHR in the renal tissues of control and NX-induced rats with or without BSA. (D) Immunohistochemical analysis with intrarenal anti-AHR antibody in the control and NX-induced rats with or without BSA. (E) Protein expression levels of podocin and nephrin in the ZAS-induced MPC5 cells treated with the different concentrations of BSA. (F) Quantitative analysis of protein expression of podocin and nephrin in ZAS-induced MPC5 cells treated with the different concentrations of BSA. (G) Immunofluorescent analysis with anti-podocin antibody in the control and ZAS-stimulated MPC5 cells with or without BSA. *P < 0.05, **P < 0.01 compared with sham or CTL; #P < 0.05, ##P < 0.01 compared with NX or ZAS-stimulated MPC5 cells.
Compared to Sham rats, NX rats presented a significant reduction in intrarenal cytoplasmic AHR protein expression in NX-induced CKD rats, which was accompanied by a significant increase in intrarenal nuclei AHR protein expression in NX-induced CKD rats (Figures 1B, C). BSA treatment markedly preserved cytoplasmic AHR protein expression and markedly reduced nuclear AHR protein expression in renal tissues of NX-induced CKD rats (Figures 1B, C). This result was further verified using immunohistochemical analysis (Figure 1D). These data suggest that activating AHR signalling in CKD and AHR may be an effective therapeutic target. BSA is a novel aryl hydrocarbon receptor antagonist.
To elucidate the renoprotective mechanism of BSA, we first determined its effect on ZAS-stimulated MPC5 cells. BSA could markedly inhibit the downregulation of the protein expression of podocin and nephrin in ZAS-treated MPC5 cells in a concentration-dependent manner (10–40 μM) within 24 h (Figures 1E, F). The concentrations of 20 and 40 μM BSA had a stronger effect on the upregulated podocin and nephrin protein expressions than did 10 μM BSA (Figures 1E, F). But, the effect at 20 μM was similar to that observed at 40 μM. Therefore, 20 μM was used for this experiment. Immunofluorescence staining further verified that BSA treatment preserved podocin expression in the ZAS-stimulated MPC5 cells (Figure 1G).
Compared to ZAS-stimulated MPC5 cells, BSA treatment markedly inhibited the mRNA expression of AHR and its four target genes, such as CYP1A1, CYP1A2, CYP1B1 and COX-2 in ZAS-induced MPC5 cells (Figure 2A), which was accompanied by reduced AHR nuclear translocation (Figure 2B), which was in line with the protein expression of increasing cytoplasmic AHR and decreasing nuclear AHR (Figures 2C, D). Luciferase assay uncovered that BSA treatment markedly inhibited AHR-driven reporter activity in ZAS-stimulated MPC5 cells (Figure 2E). These data indicate that BSA inhibits activating AHR signalling in ZAS-stimulated MPC5 cells.
Figure 2. BSA inhibits AHR signalling in the ZAS-stimulated MPC5 cells. (A) The mRNA levels of AHR and its target genes, including CYP1A1, CYP1A2, CYP1B1 and COX-2 in the control and ZAS-stimulated MPC5 cells with or without BSA. (B) Immunofluorescent analysis with anti-AHR antibody in the control and ZAS-stimulated MPC5 cells with or without BSA. (C) Cytoplasm and nuclei AHR protein expression in the control and ZAS-stimulated MPC5 cells with or without BSA. (D) Protein levels of cytoplasm and nuclei AHR in the control and CBSA-induced MN rats with or without MSG. (E) Luciferase assay of AHR activation in the control and ZAS-stimulated MPC5 cells with or without BSA. *P < 0.05, **P < 0.01 compared with CTL; #P < 0.05, ##P < 0.01 compared with ZAS-stimulated MPC5 cells.
Compared to ZAS-stimulated MPC5 cells, BSA treatment markedly reduced nuclear p65 expression in ZAS-stimulated MPC5 cells (Figures 3A–C). This is acspanied by markedly inhibiting protein expressions of p-IƙBα and p65 downstream target gene products such as COX-2, inducible nitric oxide synthase (iNOS), monocyte chemotactic protein-1 (MCP-1), 12-lipoxygenase (12-LOX), p67phox and p67phox in the ZAS-stimulated MPC5 cells (Figures 3B, C), which were consistent with markedly inhibiting cytoplasm COX-2 protein expression in the ZAS-stimulated MPC5 cells (Figure 3D). Therefore, these data suggest that BSA inhibits the hyperactive IƙB/NF-ƙB pathway in ZAS-stimulated MPC5 cells.
Figure 3. BSA inhibits activation of IƙB/NF-ƙB pathway in the ZAS-stimulated MPC5 cells. (A) Immunofluorescence analysis with anti-p65 antibody in the control and ZAS-stimulated MPC5 cells with or without BSA. (B) Protein expression of p-IƙBα and nuclei p65 and its downstream gene products including COX-2, MCP-1, iNOS, 12-LOX, p47phox and p67phox in the control and ZAS-stimulated MPC5 cells with or without BSA. (C) Protein levels of p-IƙBα, NF-ƙB p65, COX-2, MCP-1, iNOS, 12-LOX, p47phox and p67phox in the control and ZAS-stimulated MPC5 cells with or without BSA. (D) Immunofluorescence analysis with and COX-2 antibody in the control and ZAS-stimulated MPC5 cells with or without BSA. *P < 0.05, **P < 0.01 compared with CTL; #P < 0.05, ##P < 0.01 compared with ZAS-stimulated MPC5 cells.
Compared to untreated ZAS-stimulated MPC5 cells, treatment with BSA markedly increased nuclear Nrf2 expression in ZAS-stimulated MPC5 cells (Figures 4A–C). This is also accompanied by markedly reduced protein expression of Keap1 and increased Nrf2 target gene products, such as HO-1, catalase, glutamate-cysteine ligase catalytic subunit (GCLC), glutamate-cysteine ligase modifier subunit (GCLM), manganese superoxide dismutase (MnSOD), and nicotinamide adenine dinucleotide phosphate quinone dehydrogenase 1 (NQO-1), in ZAS-stimulated MPC5 cells treated with BSA compared to untreated ZAS-stimulated MPC5 cells (Figures 4B, C). In addition, BSA treatment markedly increased cytoplasmic HO-1 protein expression in ZAS-stimulated MPC5 cells (Figure 4D). These results suggest that BSA improves the impaired Keap1/Nrf2 pathway in ZAS-induced MPC5 cells. Collectively, these results indicate that BSA improves the activating IƙB/NF-ƙB and impaired Keap1/Nrf2 pathway in MPC5 cells.
Figure 4. BSA improves impairment of Keap1/Nrf2 pathway in the ZAS-stimulated MPC5 cells. (A) Immunofluorescence analysis with anti-Nrf2 antibody in the control and ZAS-stimulated MPC5 cells with or without BSA. (B) Protein expression of Nrf2, Keap1, HO-1, catalase, GCLC, GCLM, MnSOD and NQO-1 in the control and ZAS-stimulated MPC5 cells with or without BSA. (C) Protein levels of Nrf2, Keap1, HO-1, catalase, GCLC, GCLM, MnSOD and NQO-1 in the control and ZAS-stimulated MPC5 cells with or without BSA. (D) Immunofluorescence analysis with anti-HO-1 antibody in the control and ZAS-stimulated MPC5 cells with or without BSA. *P < 0.05, **P < 0.01 compared with CTL; #P < 0.05, ##P < 0.01 compared with ZAS-stimulated MPC5 cells.
We determine whether BSA mitigates MPC5 cell damage by improving IƙB/NF-ƙB and Keap1/Nrf2 pathways via suppressing AHR signalling. BSA treatment downregulated the expression of podocyte-specific proteins in ZAS-mediated MPC5 cells (Figures 5A, B). However, BSA did not significantly upregulate podocyte protein expression in ZAS-induced MPC5 cells treated with CH223191 compared with only ZAS-mediated MPC5 cells (Figures 5A, B). Similarly, the inhibitory effect of BSA on nuclear NF-ƙB p65 and Nrf2 as well as cytoplasmic COX-2, MCP-1, HO-1 and catalase was partially abolished in ZAS-mediated MPC5 cells treated with CH223191 compared with only ZAS-induced MPC5 cells (Figures 5C, D). The results were also demonstrated in the ZAS-induced AHR siRNA-transfected MPC5 cells treated with BSA (Figures 6A, D). These results indicate that BSA attenuates inflammatory response via inhibiting AHR signalling. Totally, both pharmacological and siRNA results demonstrate that BSA attenuates podocyte lesion by modulating IƙB/NF-ƙB and Keap1/Nrf2 pathways via suppressing AHR signalling.
Figure 5. BSA inhibits podocyte injury through improving IƙB/NF-ƙB and Keap1/Nrf2 pathways via AHR signalling in the ZAS-induced MPC5 cells with BSA and/or CH223191. (A) Podocyte-specific protein expression in the ZAS-induced MPC5 cells with BSA and/or CH223191. (B) Podocyte-specific protein levels in the ZAS-induced MPC5 cells with BSA and/or CH223191. (C) Protein expression of NF-ƙB p65, Nrf2, COX-2, MCP-1, HO-1 and catalase in the ZAS-induced MPC5 cells with BSA and/or CH223191. (D) Protein levels of NF-κB p65, Nrf2, COX-2, MCP-1, HO-1 and catalase in the ZAS-induced MPC5 cells with BSA and/or CH223191. *P < 0.05, **P < 0.01 compared with CTL; #P < 0.05, ##P < 0.01 compared with ZAS-stimulated MPC5 cells.
Figure 6. BSA inhibits podocyte injury through improving IƙB/NF-ƙB and Keap1/Nrf2 pathways via AHR signalling in the ZAS-induced AHRsiRNA-transfected MPC5 cells treated with BSA. (A) Podocyte-specific protein expression in the ZAS-induced AHRsiRNA-transfected MPC5 cells treated with BSA. (B) Podocyte-specific protein levels in the ZAS-induced AHRsiRNA-transfected MPC5 cells treated with BSA. (C) Protein expression of NF-ƙB p65, Nrf2, COX-2, MCP-1, HO-1 and catalase in the ZAS-induced AHRsiRNA-transfected MPC5 cells treated with BSA. (D) Protein levels of NF-ƙB p65, Nrf2, COX-2, MCP-1, HO-1 and catalase in the ZAS-induced AHRsiRNA-transfected MPC5 cells treated with BSA. *P < 0.05, **P < 0.01 compared with CTL; #P < 0.05, ##P < 0.01 compared with ZAS-stimulated MPC5 cells.
Increasing publications have suggested that TCM improved various diseases by regulating AHR signalling (Wen et al., 2023b; Ying et al., 2024; Zhang et al., 2023; Wang et al., 2024). Accumulated evidence has showed increasing serum AHR activity in CKD patients (Dou et al., 2018; Kim et al., 2013; Kim et al., 2020). Dou et al. demonstrated that CKD patients with stages 3–5 showed strong serum AHR-activating potential and upregulated mRNA levels of CYP1A1 and AHR repressor in whole blood compared to serum from healthy controls (Dou et al., 2018). Kim et al. demonstrated that serum AHR transactivation activity was higher in DKD patients with microalbuminuria and macroalbuminuria than in those with normoalbuminuria (Kim et al. (2013), indicating that high serum AHR transactivation is a high risk factor for DKD. The same research group further demonstrated that serum AHR transactivation activity was increased in non-dialysis CKD patients compared to patients on dialysis, whereas its activity was increased in patients undergoing hemodialysis compared to undergoing peritoneal dialysis (Kim et al., 2020). Hemodialysis treatment could decrease AHR transactivation activity in patients with hemodialysis dialysis (Kim et al., 2020). Some studies have shown hyperactive AHR signalling in renal tissues of CKD patients and animal models (Miao et al., 2020; Miao et al., 2022; Cao et al., 2022; Miao et al., 2024). First, our previous study demonstrated increased intrarenal mRNA expression of AHR and its genes, such as CYP1A1, CYP1A2 and CYP1B1 in CKD patients at five stages, accompanied by elevating AHR nuclear translocation (Miao et al., 2022). Second, our previous study revealed elevated intrarenal AHR nuclear translocation in patients with DKD, IgAN and IMN (Miao et al., 2020). Our latest study further showed increased intrarenal mRNA expression of AHR and its genes, including CYP1A1, CYP1A2 and CYP1B1 in patients with IMN, accompanied by elevated AHR nuclear translocation (Miao et al., 2024; Wang et al., 2023). Similar findings were also demonstrated in several rat or mice models treated with NX, adenine, unilateral ureteral obstruction and cationic bovine serum albumin (CBSA) (Miao et al., 2020; Cao et al., 2022; Miao et al., 2022). These data show that AHR signalling is activated in various pathological types of CKD. Therefore, AHR is a promising therapeutic target for improving renal function in CKD patients.
Natural products have been demonstrated to be effective therapies for intervention in glomerular-related diseases including glomerulonephritis (Wang et al., 2021; Zhao et al., 2022b; Qin et al., 2023), DKD (Huang et al., 2022; Li et al., 2022b; Liu et al., 2022b; Pei et al., 2022) and IMN (Miao et al., 2024; Wang et al., 2023). In this study, we identified BSA as an AHR antagonist and it could ameliorate podocyte lesion through improving IƙB/NF-ƙB and Keap1/Nrf2 pathways (Figure 7). BSA inhibited the mRNA expression of AHR, CYP1A1, CYP1A2, CYP1B1 and COX-2 in ZAS-stimulated MPC5 cells, which was accompanied by inhibiting nuclear translocation of AHR. Accumulating evidence suggests that many natural product-derived components can directly regulate AHR signalling. Previous studies have shown that AHR ligands from vegetable extracts mediate CYP1A1 activity (Zhao et al., 2019). Cruciferous family members, including broccoli, cauliflower, white cabbage, and Brussels sprouts, contain rich sources of AHR ligands, such as indole-3-carbinol and indole-3-acetonitrile (Zhao et al., 2019). Polyphenols are common components of the plant kingdom. Polyphenols are divided into five categories according to their chemical structures: phenolic acids, flavonoids, lignans, tannins and stilbenes. Extensive studies have demonstrated that phenolic acids and flavonoids are the most affluent polyphenolic components in diet and can be classified into flavanols, flavonols, flavones, flavanones, isoflavones, anthocyanins and proanthocyanidins (Zhao et al., 2019).
Figure 7. BSA as a novel AHR antagonist targeted oxidative stress and inflammation. Treatment with BSA suppressed intrarenal AHR expression at both mRNA and protein levels in NX rats. Hyperactive AHR and IƙB/NF-ƙB pathways and impaired Keap1/Nrf2 pathway were demonstrated in the ZAS-stimulated MPC5 cells. However, treatment with BSA could suppress hyperactive AHR and IƙB/NF-ƙB pathways and enhance impaired Keap1/Nrf2 pathway in the ZAS-stimulated MPC5 cells. Mechanistically, both pharmacological and genetic methods suggested that BSA ameliorated podocyte injury by improving IƙB/NF-ƙB and Keap1/Nrf2 pathways via AHR signalling. Therefore, BSA suppressed intrarenal AHR expression at both mRNA and protein levels by using in vivo and in vitro experiments. BSA was demonstrated to be as a high-affinity AHR antagonist that abolished oxidative stress and inflammation. AIP, AHR interacting protein; ARE, antioxidant response element; ARE/EpRE antioxidant/electrophile response element; ARNT, aryl hydrocarbon receptor nuclear translocator; CUL3, Cullin3; HSP90, heat shock protein 90; Rbx1, ring-Box 1; sMaf, small musculoaponeurotic fibrosarcoma; Ub, ubiquitin.
Recent studies suggested that TCM including Bupi Yishen formula, Dahuang Fuzi decoction and Jian-Pi-Yi-Shen formula attenuated CKD by inhibiting AHR signalling (Mo et al., 2021; Gu et al., 2022; Liu et al., 2022a). Our previous publications have demonstrated that some compounds such as matairesinol, rhoifolin, 5,6,7,8,3′,4′-hexamethoxyflavone, 5,7,3′,4′,5′-pentahydroxy flavanone and erythro-guaiacylglycerol-β-ferulic acid ether attenuated renal fibrosis by suppressing AHR signalling (Miao et al., 2020; Cao et al., 2022; Miao et al., 2022). BSA is a flavonoid glycoside. Previous studies suggested that BSA could decrease the activities of superoxide scavenging and xanthine oxidase, as well as inhibit the protein expression of extracellular matrix proteins, including collagen I, α-smooth muscle actin, and fibronectin in NRK-52E cells mediated by 1-aminopyrene (Karim et al., 2009; Miao et al., 2020). Our previous study showed that BSA inhibited mRNA expression of AHR, CYP1A1, CYP1A2 and CYP1B1 in renal tissues of NX-induced rats and NRK-52E cells mediated by 1-aminopyrene, which was accompanied by the protein expression of upregulated cytoplasmic AHR and downregulated nuclear AHR (Miao et al., 2020). Molecular ligand docking analysis revealed that BSA could bind to the active AHR site and exhibited a strong interaction with AHR. Collectively, the current study and other studies suggest that BSA is an effective AHR antagonist and suppresses AHR expression using in vivo and in vitro experiments (Figure 7).
Mechanistically, this study further illuminated that treatment with BSA mitigated podocyte lesion by suppressing hyperactive IƙB/NF-ƙB pathway and enhancing impaired Keap1/Nrf2 pathway via inhibiting AHR signalling in the ZAS-stimulated MPC5 cells (Figure 7). Both oxidative stress and inflammation change expression of a number of genes, including NF-ƙB and Nrf2. Our latest study showed that the NF-ƙB signalling was a downstream target of AHR pathway in IMN (Wang et al., 2023). Several studies have demonstrated that AHR interacts with NF-ƙB in CKD (Addi et al., 2019; Brito et al., 2019; Curran and Kopp, 2022). Brito et al. reported that increasing AHR protein levels were positively associated with increasing NF-ƙB protein levels in hemodialysis and non-dialysis-dependent patients (Brito et al., 2019). Our latest study showed increased protein expression of nuclear AHR and cytoplasmic COX-2 in the renal tissues of IMN patients (Wang et al., 2023). In addition, increased protein expression of nuclear AHR and cytoplasmic COX-2 was observed in renal tissues of rats treated with CBSA and ZAS-mediated MPC5 cells (Ma et al., 2023; Wang et al., 2023). Treatment with Moshen granules inhibited their expression in the renal tissues of rats treated with CBSA (Ma et al., 2023). Addi et al. demonstrated that an AHR ligand indole-3 acetic acid mediated activating tissue factor via AHR/NF-ƙB pathway (Addi et al., 2019). This research group further revealed that COX-2 levels were markedly suppressed in indole-3 acetic acid-induced umbilical vein endothelial cells treated with BAY 11-7082 and CH223191 (Dou et al., 2015). In addition, Lee et al. reported that ochratoxin A-treated HK-2 cells showed increased mRNA expression of AHR and its target genes, such as CYP1A1 and CYP1A2 representing phase I enzymes, as well as upregulated mRNA expression of phase II enzymes, such as GCLC, NQO1 and HO-1 by the activation of Nrf2 translocation (Lee et al., 2018). However, AHR deficiency ameliorates oxidative stress-induced macrophage infiltration, activating mesangial cell and kidney fibrosis in DKD mice (Lee et al., 2016).
Accumulated evidence has reported that renoprotective effect of natural products were associated with suppressing AHR, IƙB/NF-ƙB and Keap1/Nrf2 pathways. Dhulkifle et al. reported that treatment with 6-formylindolo(3,2-b)carbazole improved septic acute kidney injury and inflammation by increasing intrarenal mRNA expression of H O -1 and NQO1 via AHR and Nrf2 (Dhulkifle et al., 2023). Recent publication showed that Dahuang Fuzi decoction blunted CKD by suppressing AHR/NF-ƙB pathway (Gu et al., 2022). Moreover, Zhao et al. showed that the beneficial effect of Tangshen formula for NF-ƙB p-p65 expression was related to AHR inhibition in renal tissues of DKD rats (Zhao et al., 2020). Our earlier publication showed that poricoic acids abolished AHR, IƙB/NF-ƙB and Keap1/Nrf2 pathways in mice with renal fibrosis (Wang et al., 2020). Collectively, this study demonstrated that BSA dampened podocyte lesion partly by modulating IƙB/NF-ƙB and Keap1/Nrf2 pathways via attenuating AHR signalling.
In conclusion, this study showed that treatment with BSA suppressed AHR expression at both the mRNA and protein levels in the renal tissues of NX rats and ZAS-stimulated MPC5 cells. We further illuminated that BSA mitigated podocyte lesion by suppressing hyperactive IƙB/NF-ƙB pathway and enhancing hypoactive Keap1/Nrf2 pathway via inhibiting AHR signalling in the ZAS-stimulated MPC5 cells. Mechanistically, both pharmacological and genetic results suggested that BSA ameliorated podocyte damage by modulating IƙB/NF-ƙB and Keap1/Nrf2 pathways via AHR signalling. Therefore, BSA is a high-affinity AHR antagonist that abolishes oxidative stress and inflammation. These findings may provide a leading drug for treating podocyte damage-related renal disease through oxidative stress and inflammation.
The original contributions presented in the study are included in the article/Supplementary Material, further inquiries can be directed to the corresponding authors.
The animal study was approved by Zhejiang Chinese Medical University. The study was conducted in accordance with the local legislation and institutional requirements. No potentially identifiable images or data are presented in this study.
X-JL: Data curation, Investigation, Project administration, Supervision, Writing–review and editing. Y-NW: Investigation, Resources, Software, Supervision, Visualization, Writing–review and editing. W-FW: Data curation, Investigation, Supervision, Validation, Writing–review and editing. XN: Software, Supervision, Writing–review and editing. HM: Conceptualization, Funding acquisition, Project administration, Software, Supervision, Visualization, Writing–review and editing. Y-YZ: Conceptualization, Data curation, Funding acquisition, Methodology, Project administration, Software, Supervision, Validation, Writing–original draft.
The author(s) declare that financial support was received for the research, authorship, and/or publication of this article. This study was supported by the National Natural Science Foundation of China (Nos 82074002, 82274079, and 82274192), the National Key Research and Development Program of China (No. 2019YFC1709405) and the Shaanxi Key Science and Technology Plan Project (No. 2023-ZDLSF-26).
The authors declare that the research was conducted in the absence of any commercial or financial relationships that could be construed as a potential conflict of interest.
The author(s) declared that they were an editorial board member of Frontiers, at the time of submission. This had no impact on the peer review process and the final decision.
All claims expressed in this article are solely those of the authors and do not necessarily represent those of their affiliated organizations, or those of the publisher, the editors and the reviewers. Any product that may be evaluated in this article, or claim that may be made by its manufacturer, is not guaranteed or endorsed by the publisher.
The Supplementary Material for this article can be found online at: https://www.frontiersin.org/articles/10.3389/fphar.2024.1386604/full#supplementary-material
Addi, T., Poitevin, S., McKay, N., El Mecherfi, K. E., Kheroua, O., Jourde-Chiche, N., et al. (2019). Mechanisms of tissue factor induction by the uremic toxin indole-3 acetic acid through aryl hydrocarbon receptor/nuclear factor-kappa B signaling pathway in human endothelial cells. Arch. Toxicol. 93 (1), 121–136. doi:10.1007/s00204-018-2328-3
Brito, J. S., Borges, N. A., Anjos, J. S. D., Nakao, L. S., Stockler-Pinto, M. B., Paiva, B. R., et al. (2019). Aryl hydrocarbon receptor and uremic toxins from the gut microbiota in chronic kidney disease patients: is there a relationship between them? Biochemistry 58 (15), 2054–2060. doi:10.1021/acs.biochem.8b01305
Cao, G., Miao, H., Wang, Y. N., Chen, D. Q., Wu, X. Q., Chen, L., et al. (2022). Intrarenal 1-methoxypyrene, an aryl hydrocarbon receptor agonist, mediates progressive tubulointerstitial fibrosis in mice. Acta Pharmacol. Sin. 43 (11), 2929–2945. doi:10.1038/s41401-022-00914-6
Curran, C. S., and Kopp, J. B. (2022). Aryl hydrocarbon receptor mechanisms affecting chronic kidney disease. Front. Pharmacol. 13, 782199. doi:10.3389/fphar.2022.782199
da Silva, J. F., Bolsoni, J. A., da Costa, R. M., Alves, J. V., Bressan, A. F. M., Silva, L. E. V., et al. (2022). Aryl hydrocarbon receptor (AhR) activation contributes to high-fat diet-induced vascular dysfunction. Br. J. Pharmacol. 179 (12), 2938–2952. doi:10.1111/bph.15789
Dhulkifle, H., Sayed, T. S., Abunada, H. H., Abulola, S. M., Alhoshani, A., Korashy, H. M., et al. (2023). 6-Formylindolo(3,2-b)carbazole dampens inflammation and reduces endotoxin-induced kidney injury via Nrf2 activation. Chem. Res. Toxicol. 36 (3), 552–560. doi:10.1021/acs.chemrestox.3c00002
Dou, L., Poitevin, S., Sallee, M., Addi, T., Gondouin, B., McKay, N., et al. (2018). Aryl hydrocarbon receptor is activated in patients and mice with chronic kidney disease. Kidney Int. 93 (4), 986–999. doi:10.1016/j.kint.2017.11.010
Dou, L., Sallee, M., Cerini, C., Poitevin, S., Gondouin, B., Jourde-Chiche, N., et al. (2015). The cardiovascular effect of the uremic solute indole-3 acetic acid. J. Am. Soc. Nephrol. 26 (4), 876–887. doi:10.1681/asn.2013121283
Goya-Jorge, E., Jorge Rodríguez, M. E., Veitía, M. S., and Giner, R. M. (2021). Plant occurring flavonoids as modulators of the aryl hydrocarbon receptor. Molecules 26 (8), 2315. doi:10.3390/molecules26082315
Gu, M., Ying, P., Miao, Z., Yu, X., Bao, R., Xiao, J., et al. (2022). Treatment of modified Dahuang Fuzi decoction on cognitive impairment induced by chronic kidney disease through regulating AhR/NF-κB/JNK signal pathway. Evid. Based Complement. Altern. Med. 2022, 8489699. doi:10.1155/2022/8489699
Hou, J. J., Zhang, Z. J., Wu, W. Y., He, Q. Q., Zhang, T. Q., Liu, Y. W., et al. (2022). Mass spectrometry imaging: new eyes on natural products for drug research and development. Acta Pharmacol. Sin. 43 (12), 3096–3111. doi:10.1038/s41401-022-00990-8
Hu, J., Gu, W., Ma, N., Fan, X., and Ci, X. (2022). Leonurine alleviates ferroptosis in cisplatin-induced acute kidney injury by activating the Nrf2 signalling pathway. Br. J. Pharmacol. 179 (15), 3991–4009. doi:10.1111/bph.15834
Huang, D., Shen, P., Wang, C., Gao, J., Ye, C., and Wu, F. (2022). Calycosin plays a protective role in diabetic kidney disease through the regulation of ferroptosis. Pharm. Biol. 60 (1), 990–996. doi:10.1080/13880209.2022.2067572
Karim, A., Noor, A. T., Malik, A., Qadir, M. I., and Choudhary, M. I. (2009). Barlerisides A and B, new potent superoxide scavenging phenolic glycosides from Barleria acanthoides. J. Enzyme Inhib. Med. Chem. 24 (6), 1332–1335. doi:10.3109/14756360902887046
Kim, J. T., Kim, S. H., Min, H. K., Jeon, S. J., Sung, S. A., Park, W. H., et al. (2020). Effect of dialysis on aryl hydrocarbon receptor transactivating activity in patients with chronic kidney disease. Yonsei Med. J. 61 (1), 56–63. doi:10.3349/ymj.2020.61.1.56
Kim, J. T., Kim, S. S., Jun, D. W., Hwang, Y. H., Park, W. H., Pak, Y. K., et al. (2013). Serum arylhydrocarbon receptor transactivating activity is elevated in type 2 diabetic patients with diabetic nephropathy. J. Diabetes Investig. 4 (5), 483–491. doi:10.1111/jdi.12081
Kong, Y. D., Qi, Y., Cui, N., Zhang, Z. H., Wei, N., Wang, C. F., et al. (2023). The traditional herb Polygonum hydropiper from China: a comprehensive review on phytochemistry, pharmacological activities and applications. Pharm. Biol. 61 (1), 799–814. doi:10.1080/13880209.2023.2208639
Lee, H. J., Pyo, M. C., Shin, H. S., Ryu, D., and Lee, K. W. (2018). Renal toxicity through AhR, PXR, and Nrf2 signaling pathway activation of ochratoxin A-induced oxidative stress in kidney cells. Food Chem. Toxicol. 122, 59–68. doi:10.1016/j.fct.2018.10.004
Lee, W. J., Liu, S. H., Chiang, C. K., Lin, S. Y., Liang, K. W., Chen, C. H., et al. (2016). Aryl hydrocarbon receptor deficiency attenuates oxidative stress-related mesangial cell activation and macrophage infiltration and extracellular matrix accumulation in diabetic nephropathy. Antioxid. Redox Signal 24 (4), 217–231. doi:10.1089/ars.2015.6310
Li, C., Jia, W. W., Yang, J. L., Cheng, C., and Olaleye, O. E. (2022a). Multi-compound and drug-combination pharmacokinetic research on Chinese herbal medicines. Acta Pharmacol. Sin. 43 (12), 3080–3095. doi:10.1038/s41401-022-00983-7
Li, J., Zhang, J., Yang, M., Huang, X., Zhang, M., Fang, X., et al. (2022b). Kirenol alleviates diabetic nephropathy via regulating TGF-β/Smads and the NF-κB signal pathway. Pharm. Biol. 60 (1), 1690–1700. doi:10.1080/13880209.2022.2112239
Li, M. Z., Zhao, Y., Wang, H. R., Talukder, M., and Li, J. L. (2021). Lycopene preventing DEHP-induced renal cell damage is targeted by aryl hydrocarbon receptor. J. Agric. Food Chem. 69 (43), 12853–12861. doi:10.1021/acs.jafc.1c05250
Liu, X., Deng, R., Chen, Y., Huang, S., Lu, J., Zheng, L., et al. (2022a). Jian-Pi-Yi-Shen Formula improves adenine-induced chronic kidney disease via regulating tryptophan metabolism and aryl hydrocarbon receptor signaling. Front. Pharmacol. 13, 922707. doi:10.3389/fphar.2022.922707
Liu, X. Q., Jiang, L., Li, Y. Y., Huang, Y. B., Hu, X. R., Zhu, W., et al. (2022b). Wogonin protects glomerular podocytes by targeting Bcl-2-mediated autophagy and apoptosis in diabetic kidney disease. Acta Pharmacol. Sin. 43 (1), 96–110. doi:10.1038/s41401-021-00721-5
Ma, S.-X., Li, X.-J., Duan, T.-T., Pei, M., Zou, L., Yu, X.-Y., et al. (2023). Moshen granule ameliorates membranous nephropathy by regulating NF-ƙB/Nrf2 pathways via aryl hydrocarbon receptor signalling. Heliyon 9 (9), e20019. doi:10.1016/j.heliyon.2023.e20019
Marena, G. D., Ramos, M., Carvalho, G. C., Junior, J. A. P., Resende, F. A., Corrêa, I., et al. (2022). Natural product-based nanomedicine applied to fungal infection treatment: a review of the last 4 years. Phytother. Res. 36 (7), 2710–2745. doi:10.1002/ptr.7460
Miao, H., Cao, G., Wu, X. Q., Chen, Y. Y., Chen, D. Q., Chen, L., et al. (2020). Identification of endogenous 1-aminopyrene as a novel mediator of progressive chronic kidney disease via aryl hydrocarbon receptor activation. Br. J. Pharmacol. 177 (15), 3415–3435. doi:10.1111/bph.15062
Miao, H., Wang, Y. N., Yu, X. Y., Zou, L., Guo, Y., Su, W., et al. (2024). Lactobacillus species ameliorate membranous nephropathy through inhibiting the aryl hydrocarbon receptor pathway via tryptophan-produced indole metabolites. Br. J. Pharmacol. 181 (1), 162–179. doi:10.1111/bph.16219
Miao, H., Wu, X. Q., Wang, Y. N., Chen, D. Q., Chen, L., Vaziri, N. D., et al. (2022). 1-Hydroxypyrene mediates renal fibrosis through aryl hydrocarbon receptor signalling pathway. Br. J. Pharmacol. 179 (1), 103–124. doi:10.1111/bph.15705
Mo, Y., Hu, D., Yu, W., Ji, C., Li, Y., Liu, X., et al. (2023). Astragaloside IV attenuates indoxyl sulfate-induced injury of renal tubular epithelial cells by inhibiting the aryl hydrocarbon receptor pathway. J. Ethnopharmacol. 308, 116244. doi:10.1016/j.jep.2023.116244
Mo, Y., Jie, X., Wang, L., Ji, C., Gu, Y., Lu, Z., et al. (2021). Bupi Yishen formula attenuates kidney injury in 5/6 nephrectomized rats via the tryptophan-kynurenic acid-aryl hydrocarbon receptor pathway. BMC Complement. Med. Ther. 21 (1), 207. doi:10.1186/s12906-021-03376-1
Newman, D. J., and Cragg, G. M. (2020). Natural products as sources of new drugs over the nearly four decades from 01/1981 to 09/2019. J. Nat. Prod. 83 (3), 770–803. doi:10.1021/acs.jnatprod.9b01285
Ouyang, L., Yan, B., Liu, Y., Mao, C., Wang, M., Liu, N., et al. (2020). The deubiquitylase UCHL3 maintains cancer stem-like properties by stabilizing the aryl hydrocarbon receptor. Signal Transduct. Target Ther. 5 (1), 78. doi:10.1038/s41392-020-0181-3
Pei, D., Tian, S., Bao, Y., Zhang, J., Xu, D., and Piao, M. (2022). Protective effect of salidroside on streptozotocin-induced diabetic nephropathy by inhibiting oxidative stress and inflammation in rats via the Akt/GSK-3β signalling pathway. Pharm. Biol. 60 (1), 1732–1738. doi:10.1080/13880209.2022.2116055
Qin, X., Chen, H., Zhu, X., Xu, X., and Gao, J. (2023). Identification of Rab7 as an autophagy marker: potential therapeutic approaches and the effect of Qi Teng Xiao Zhuo granule in chronic glomerulonephritis. Pharm. Biol. 61 (1), 1120–1134. doi:10.1080/13880209.2023.2233998
Ren, Q., Tao, S., Guo, F., Wang, B., Yang, L., Ma, L., et al. (2021). Natural flavonol fisetin attenuated hyperuricemic nephropathy via inhibiting IL-6/JAK2/STAT3 and TGF-β/SMAD3 signaling. Phytomedicine 87, 153552. doi:10.1016/j.phymed.2021.153552
Shao, Y. F., Tang, B. B., Ding, Y. H., Fang, C. Y., Hong, L., Shao, C. X., et al. (2023). Kaempferide ameliorates cisplatin-induced nephrotoxicity via inhibiting oxidative stress and inducing autophagy. Acta Pharmacol. Sin. 44 (7), 1442–1454. doi:10.1038/s41401-023-01051-4
Singh, B., Kumar, A., Singh, H., Kaur, S., Arora, S., and Singh, B. (2022). Protective effect of vanillic acid against diabetes and diabetic nephropathy by attenuating oxidative stress and upregulation of NF-κB, TNF-α and COX-2 proteins in rats. Phytother. Res. 36 (3), 1338–1352. doi:10.1002/ptr.7392
Sun, M. Y., Ye, H. J., Zheng, C., Jin, Z. J., Yuan, Y., and Weng, H. B. (2023). Astragalin ameliorates renal injury in diabetic mice by modulating mitochondrial quality control via AMPK-dependent PGC1α pathway. Acta Pharmacol. Sin. 44 (8), 1676–1686. doi:10.1038/s41401-023-01064-z
Wang, M., Hu, H. H., Chen, Y. Y., Chen, L., Wu, X. Q., and Zhao, Y. Y. (2020). Novel poricoic acids attenuate renal fibrosis through regulating redox signalling and aryl hydrocarbon receptor activation. Phytomedicine 79, 153323. doi:10.1016/j.phymed.2020.153323
Wang, X., Xie, X., Li, Y., Xie, X., Huang, S., Pan, S., et al. (2024). Quercetin ameliorates ulcerative colitis by activating aryl hydrocarbon receptor to improve intestinal barrier integrity. Phytother. Res. 38 (1), 253–264. doi:10.1002/ptr.8027
Wang, Y. N., Miao, H., Hua, M. R., Yang, J. Z., Pei, M., Yu, H. X., et al. (2023a). Moshen granule ameliorates membranous nephropathy by blocking intrarenal renin-angiotensin system signalling via the Wnt1/β-catenin pathway. Phytomedicine 114, 154763. doi:10.1016/j.phymed.2023.154763
Wang, Y. N., Miao, H., Yu, X. Y., Guo, Y., Su, W., Liu, F., et al. (2023b). Oxidative stress and inflammation are mediated via aryl hydrocarbon receptor signalling in idiopathic membranous nephropathy. Free Radic. Biol. Med. 207, 89–106. doi:10.1016/j.freeradbiomed.2023.07.014
Wang, Y. W., Zeng, Q., and Yu, R. H. (2021). Treatment of membranoproliferative glomerulonephritis with traditional Chinese medicine and rituximab: a case report. 8, 3.doi:10.4103/imna.imna_10_21Integr Med Nephrol Androl. 8 3
Wen, S. Y., Wei, B. Y., Ma, J. Q., Wang, L., and Chen, Y. Y. (2023a). Phytochemicals, biological activities, molecular mechanisms, and future prospects of Plantago asiatica L. J. Agric. Food Chem. 71 (1), 143–173. doi:10.1021/acs.jafc.2c07735
Wen, Y., Zhan, Y., Tang, S., Liu, F., Wu, R., Kong, P., et al. (2023b). Zhizhu decoction alleviates slow transit constipation by regulating aryl hydrocarbon receptor through gut microbiota. Pharm. Biol. 61 (1), 111–124. doi:10.1080/13880209.2022.2157020
Wu, S. M., Tsai, J. J., Pan, H. C., Arbiser, J. L., Elia, L., and Sheu, M. L. (2022). Aggravation of pulmonary fibrosis after knocking down the aryl hydrocarbon receptor in the insulin-like growth factor 1 receptor pathway. Br. J. Pharmacol. 179 (13), 3430–3451. doi:10.1111/bph.15806
Ying, Y., Song, L. Y., Pang, W. L., Zhang, S. Q., Yu, J. Z., Liang, P. T., et al. (2024). Astragalus polysaccharide protects experimental colitis through an aryl hydrocarbon receptor-dependent autophagy mechanism. Br. J. Pharmacol. 181 (5), 681–697. doi:10.1111/bph.16229
Yuan, Q., Tang, B., and Zhang, C. (2022). Signaling pathways of chronic kidney diseases, implications for therapeutics. Signal Transduct. Target Ther. 7 (1), 182. doi:10.1038/s41392-022-01036-5
Zhang, C., Fan, S., Zhao, J. Q., Jiang, Y., Sun, J. X., and Li, H. J. (2023). Transcriptomics and metabolomics reveal the role of CYP1A2 in psoralen/isopsoralen-induced metabolic activation and hepatotoxicity. Phytother. Res. 37 (1), 163–180. doi:10.1002/ptr.7604
Zhao, H., Chen, L., Yang, T., Feng, Y. L., Vaziri, N. D., Liu, B. L., et al. (2019). Aryl hydrocarbon receptor activation mediates kidney disease and renal cell carcinoma. J. Transl. Med. 17 (1), 302. doi:10.1186/s12967-019-2054-5
Zhao, T., Zhang, H., Yin, X., Zhao, H., Ma, L., Yan, M., et al. (2020). Tangshen formula modulates gut microbiota and reduces gut-derived toxins in diabetic nephropathy rats. Biomed. Pharmacother. 129, 110325. doi:10.1016/j.biopha.2020.110325
Zhao, Y., Deng, S., Bai, Y., Guo, J., Kai, G., Huang, X., et al. (2022a). Promising natural products against SARS-CoV-2: structure, function, and clinical trials. Phytother. Res. 36 (10), 3833–3858. doi:10.1002/ptr.7580
Zhao, Y. L., Zhang, X. H., Guo, F., Wei, Y., Shang, J. H., and Luo, X. D. (2022b). Yi Shen An, a Chinese traditional prescription, ameliorates membranous glomerulonephritis induced by cationic bovine serum albumin in rats. Pharm. Biol. 60 (1), 163–174. doi:10.1080/13880209.2021.2021947
Keywords: aryl hydrocarbon receptor, oxidative stress and inflammation, nuclear factor kappa B, Nrf2, chronic kidney disease, podocyte injury, Plantaginis semen, barleriside A
Citation: Li X-J, Wang Y-N, Wang W-F, Nie X, Miao H and Zhao Y-Y (2024) Barleriside A, an aryl hydrocarbon receptor antagonist, ameliorates podocyte injury through inhibiting oxidative stress and inflammation. Front. Pharmacol. 15:1386604. doi: 10.3389/fphar.2024.1386604
Received: 15 February 2024; Accepted: 12 August 2024;
Published: 22 August 2024.
Edited by:
Jian-bo Yang, National Institutes for Food and Drug Control, ChinaReviewed by:
Ahmed A. Elmarakby, Augusta University, United StatesCopyright © 2024 Li, Wang, Wang, Nie, Miao and Zhao. This is an open-access article distributed under the terms of the Creative Commons Attribution License (CC BY). The use, distribution or reproduction in other forums is permitted, provided the original author(s) and the copyright owner(s) are credited and that the original publication in this journal is cited, in accordance with accepted academic practice. No use, distribution or reproduction is permitted which does not comply with these terms.
*Correspondence: Xiaoli Nie, bnhsMTE3QDE2My5jb20=; Hua Miao, aG1pYW83N0AxNjMuY29t; Ying-Yong Zhao, emhhb3l5YnJAMTYzLmNvbQ==
Disclaimer: All claims expressed in this article are solely those of the authors and do not necessarily represent those of their affiliated organizations, or those of the publisher, the editors and the reviewers. Any product that may be evaluated in this article or claim that may be made by its manufacturer is not guaranteed or endorsed by the publisher.
Research integrity at Frontiers
Learn more about the work of our research integrity team to safeguard the quality of each article we publish.