- 1Faculty of Chinese Medicine and State Key Laboratory of Quality Research in Chinese Medicines, Macau University of Science and Technology, Macau, Macao SAR, China
- 2Henan University of Chinese Medicine, Zhengzhou, China
- 3Zunyi Medical University, Zhuhai, China
Background: Depression is a severe mental disorder that poses a significant threat to both the physical and mental wellbeing of individuals. Currently, there are various methods for treating depression, including traditional Chinese herbal formulations like Chaihu-Shugan-San (CSS), which have shown effective antidepressant effects in both clinical and animal research.
Objective: This review aims to provide a comprehensive synthesis of evidence related to CSS, considering both preclinical and clinical studies, to uncover its potential multi-level, multi-pathway, and multi-target mechanisms for treating depression and identify its active ingredients.
Methods: A thorough search was conducted in electronic databases, including PubMed, MEDLINE, Web of Science, Google Scholar, CNKI, and Wanfang, using keywords such as “Chaihu Shugan” and “depression” to retrieve relevant literature on CSS and its active ingredients. The review process adhered to the PRISMA guidelines.
Results: This review consolidates the mechanisms underlying antidepressant effects of CSS and its active ingredients. It emphasizes its involvement in the regulation of monoaminergic neurotransmitter systems, synaptic plasticity, and the hypothalamic-pituitary-adrenal axis, among other aspects.
Conclusion: CSS exerts a pivotal role in treating depression through various pathways, including the monoaminergic neurotransmitter system, the hypothalamic-pituitary-adrenal axis, synaptic plasticity, inflammation, brain-derived neurotrophic factor levels, and the brain-gut axis. This review facilitates a comprehensive understanding of the current state of CSS research, fostering an in-depth exploration of the etiological mechanisms of depression and the potential discovery of novel antidepressant drugs.
1 Introduction
Depression has emerged as one of the most prevalent global public health issues, with its incidence steadily rising year by year. Depression patients commonly exhibit persistent low mood, insomnia, extreme fatigue, lack of energy, self-doubt, and excessive self-blame. They may experience unexplained physical symptoms, and in some cases, thoughts of self-harm or suicide may be present. Both the recurrence rate and the disability rate associated with depression are substantial (GBD, 2019 Mental Disorders Collaborators, 2022). This debilitating condition affects a staggering 350 million individuals worldwide, which continues to grow.
The economic burden of depression is substantial, with annual cost estimates reaching as high as 200 billion dollars. This accounts for the expenses associated with depression treatment and the productivity impact related to work, such as absenteeism and missed deadlines. Consequently, the societal impact of depression is becoming increasingly severe (Greenberg et al., 2015).
Projections from a study indicate that by 2030, depression is poised to become the second-largest contributor to the global disease burden (Mathers and Loncar, 2006). The likelihood of severe depression is significantly higher among individuals with common physical illnesses such as cardiovascular diseases, cancer, and neurodegenerative diseases compared to the general population. Conversely, individuals with depression are at a greater risk of developing various physical illnesses like cardiovascular diseases (Basiri et al., 2023), stroke (Ji et al., 2023), and diabetes (Qi et al., 2023). This high level of comorbidity is associated with worse outcomes, reduced treatment compliance, increased mortality rates, higher healthcare utilization, and costs. Comorbidities can also lead to a range of clinical challenges, including more complex treatment regimens, issues related to adaptive health behaviors, drug interactions, and adverse events caused by medications used for both physical and mental disorders (Berk et al., 2023). There are numerous risk factors associated with the onset of depression, including stress, pain, physical illnesses, and cognitive decline. Currently, scholars are primarily focusing on the pathophysiology of depression, particularly in relation to the monoaminergic system, the glutamatergic system, the hypothalamic-pituitary-adrenal axis, inflammation, gut microbiota, and neurogenesis (Emiko Tsugiyama et al., 2023; Reyes-Lizaola et al., 2023). Since the 1950s, commonly used clinical antidepressant medications have primarily targeted the increase of serotonin or acted directly on serotonin receptors (Murrough and Charney, 2012; Undurraga and Baldessarini, 2012). Although 35%–50% of patients do not respond to these medications, selective serotonin reuptake inhibitors (SSRIs) continue to be the mainstay of antidepressant therapy. Additionally, drowsiness and gastrointestinal reactions are common adverse effects of these drugs. Improvement in depressive symptoms typically takes at least 2 weeks or more after starting treatment (Trivedi et al., 2006; Trivedi et al., 2008). Reportedly, traditional Chinese medicine has demonstrated high efficacy in the treatment of depression with minimal side effects, making it considered a favorable approach for managing depressive symptoms. A substantial body of research indicates that traditional Chinese medicine may significantly alleviate the symptoms of depression and other disorders characterized by depressive behaviors (Moreira et al., 2023).
Chaihu-Shugan-San (CSS) is a traditional Chinese herbal formula that was originally documented in the Ming Dynasty’s “Yi Xue Tong Zhi.” It comprises seven botanical drugs, including Bupleurum chinense DC. [Apiaceae; Bupleuri radix], Citrus reticulata Blanco [Rutaceae; Citri reticulatae pericarpium], Paeonia lactiflora Pall. [Paeoniaceae; Paeoniae radix alba], Cirtus aurantium L. [Rutaceae; Aurantii fructus], Cyperus rotundus L. [Cyperaceae; Cyperi rhizoma], Ligusticum chuanxiong Hort. [Apiaceae; Chuanxiong rhizoma], and Glycyrrhiza uralensis Fisch. [Fabaceae; Glycyrrhizae radix et rhizoma], and the ratio is 4:4:3:3:3:3:1 (Li et al., 2022). The specific preparation method involves mixing 7 types of botanical drugs in the respective proportions, soaking them in water at 25°C for 0.5 h, and then heating to 100°C and boiling for 30 min. The first filtrate is collected in a beaker. The botanical drug residues are refluxed and heated in the same volume of water for 30 min, and then the second filtrate is collected. The two filtrates are integrated and filtered through 5 layers of cotton gauze (Yan et al., 2020; Zhu et al., 2021). All plant names or species were validated using http://mpns.kew.org/mpns-portal/ and Chinese Pharmacopoeia, as depicted in Figure 1. CSS has been long used in clinical practice for the treatment of liver qi stagnation combined with depression. Clinical research has shown that CSS exhibits significant efficacy and safety in alleviating depressive symptoms in patients, leading to improvements in depression symptomatology, increased response rates (Wang et al., 2012), and enhanced HAMD scores (Ding et al., 2018). Moreover, CSS has demonstrated positive antidepressant effects in various populations, such as postpartum depression, perimenopausal depression (Cai and Bao, 2023), post-stroke depression (Fan et al., 2023), and geriatric depression (Xiong, 2021).
Additionally, research suggests that CSS not only displays antidepressant effects in common rodent models of depression, including chronic unpredictable mild stress (CUMS) (Liu et al., 2018) or combined social isolation (Zhang et al., 2011a), chronic restraint stress (CRS) (Qian et al., 2020) or combined social isolation (Lijuan et al., 2012), and LPS-induced depression model (Xuesong et al., 2023).
Prior research has identified several potential antidepressant mechanisms of CSS. These mechanisms include the regulation of monoaminergic neurotransmitters (Hwang et al., 2020), effects on the HPA axis, anti-inflammatory and neuroplasticity actions (Li et al., 2019), primarily affecting regions such as the hippocampus (Zhu et al., 2021), prefrontal cortex (Zhang et al., 2021a) and amygdala (Ying et al., 2011). Extensive research employing various techniques and methods has been conducted to investigate the antidepressant mechanisms of CSS and its active ingredients. However, due to certain limitations, including its complex composition, the exact antidepressant mechanisms of CSS remain to be fully elucidated. Therefore, this paper provides a comprehensive review of its antidepressant mechanisms and active ingredients, with the hope that our findings will contribute to further research on CSS in the context of depression.
2 Methods
2.1 Search strategy
We searched for CSS about depressive disorders using the terms described below to obtain data from several electronic databases from the inception of each database to October 2023. Specifically, we searched using PubMed, Web of Science, Google Scholar, Cochrane Library, Chinese National Knowledge Infrastructure, VIP Information, and Wanfang Database. We used the search strategy of combining words (“Depression” OR “Depressive Symptoms” OR “Symptom, Depressive”) AND (“Chaihushugansan” OR “Chai Hu Shu Gan San” OR “Chai-Hu-Shu-Gan-San” OR “Chaihu Shugan San” OR “Chai Hu Shu Gan formula” OR “Chai Hu Shu Gan powder”).
2.2 Inclusion and exclusion criteria
The inclusion criteria were defined as follows: 1) Studies, both in vivo and in vitro, that evaluated the effects of CSS in the treatment of depression; 2) Any intervention in the experimental group that involved the use of an CSS prescription for depression, comprising the seven botanical drugs mentioned earlier, at any dosage, frequency, or administration method; 3) No restrictions on the species, sex, age, or weight of animals, and the species of cells under investigation; and 4) Studies that focused on depression. The exclusion criteria were as follows: 1) The use of CSS as an adjuvant drug in the intervention group; 2) Duplicate studies; 3) Inadequate outcome measures or incomplete data in the studies.
3 Clinical studies on Chaihu-Shugan-San
3.1 Clinical research and safety of CSS for depression
Multiple meta-analyses have demonstrated that when CSS is used in conjunction with antidepressant drugs such as fluoxetine, venlafaxine, paroxetine, etc., the efficacy in treating depression significantly surpasses that of using antidepressants alone, indicating that the combination of CSS with antidepressants effectively enhances the treatment outcomes (Zeng et al., 2017; Sun et al., 2020). In a study involving 40 postpartum depression patients who received 4 weeks of CSS treatment, it was found that the patients experienced a reduction in their postpartum depression scores, as measured by the Edinburgh Postartum Depression Scale (EPDS) and the Hamilton Depression Scale (HAMD). Simultaneously, their hormone levels, including luteotropic hormone (LH), estradiol (E2), and follicular stimulating hormone (FSH), increased. This suggests that CSS can effectively ameliorate clinical symptoms of depression and regulate hormone levels. In a clinical study involving 120 geriatric depression patients, after CSS treatment, it was observed that HAMD scores, Pittsburgh Sleep Quality Index (PSQI) scores, and Quality of Life (QOL) scores all improved. This indicates that CSS can effectively reduce the severity of depression, improve sleep quality and life quality of patients (Xiong et al., 2019).
When CSS was used as the primary treatment, a network meta-analysis of seven traditional Chinese medicines as adjunctive therapy for post-stroke depression revealed that CSS has unique advantages in enhancing clinical efficacy compared to some other traditional Chinese medicines (Yu et al., 2022). In a clinical randomized controlled trial involving 86 patients, CSS demonstrated a significantly higher clinical effectiveness rate compared to the control group (97.67% vs. 81.40%) and substantially alleviated depression levels of patients (Zhang, 2016). Results from a clinical study indicated that, when used as an adjunctive medication, clinical effectiveness of CSS (overall efficacy rate of 97.20%) was significantly better than the control group receiving only psychotherapy (overall efficacy rate of 91.42%) (Chen et al., 2021).
3.2 Clinical application of CSS in the treatment of depression
In a systematic review and meta-analysis assessing the safety and efficacy of CSS in treating depression, it was observed that the adverse reaction rate of CSS combined with antidepressants was lower than that of the group receiving antidepressants alone (Zeng et al., 2017; Sun et al., 2020). An analysis of a randomized controlled trial involving 66 patients treated with CSS for post-stroke depression showed that CSS significantly improved scores on related scales, including the Hamilton Anxiety Rating Scale, and that CSS in combination with antidepressants effectively treated post-stroke depression with lower side effects compared to the control group (6.06% vs. 27.27%) (Tao et al., 2023). These studies suggest that the likelihood of CSS interacting with other medications is quite low.
However, further investigation is needed to assess the safety of using CSS alone. In a meta-analysis involving CSS for Parkinson’s disease patients, data from 11 trials showed no adverse reactions, indicating that CSS could be a potential treatment option for patients with comorbid depression (Huber et al., 2023). A meta-analysis of 24 studies using CSS or its modified formulas for treating depression reported neurological symptoms such as dizziness, headache, sleep disturbances, hyperactivity, blurred vision, and fatigue, as well as gastrointestinal symptoms including dry mouth, diarrhea, anorexia, nausea, vomiting, and constipation. However, the incidence of adverse reactions was lower than in the control group (Zhao et al., 2023b).
4 The antidepressant mechanisms of CSS
4.1 Regulation of monoaminergic neurotransmitter systems
Damage to the monoaminergic neurotransmission and the accompanying decrease in 5-HT and NE concentrations are major factors in the pathogenesis of depression and are the targets of most antidepressants (Perez-Caballero et al., 2019). Currently, most antidepressants work by inhibiting the reuptake of monoamines, thereby increasing monoamine levels in the synaptic cleft (Lee et al., 2010). However, selective serotonin reuptake inhibitors may lead to withdrawal symptoms such as nausea, vomiting, and diarrhea, limiting their clinical use (Lee et al., 2010). As shown in Figure 2, After treatment with CSS, the content of 5-HT, 5-HIAA, and DA in the hippocampus and hypothalamus of rats increased, while the NE content decreased. This suggests that CSS exerts its antidepressant effects by regulating levels of monoaminergic neurotransmitters like 5-HT and DA in the rat brain (Yufeng et al., 2016). Furthermore, earlier research has also yielded the same conclusion (Wang et al., 2014). MAO-A is involved in the metabolism of 5-HT and NE, and some antidepressants can exert their effects by inhibiting MAO activity (Jahanabadi et al., 2023). Studies have shown that CSS effectively reduces the activity of MAO enzymes, regulating the expression of 5-HT and NE, thereby achieving its antidepressant effects (Haiying et al., 2011).
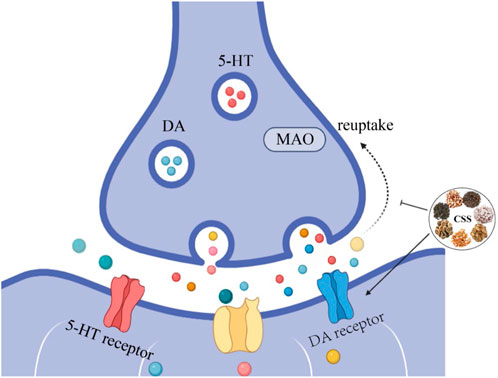
Figure 2. CSS Increases Monoaminergic Neurotransmitter Levels. CSS facilitates the synthesis of monoaminergic neurotransmitters, leading to an increase in the concentration of these neurotransmitters. Inhibiting the reuptake of monoaminergic neurotransmitters enhances their respective signaling pathways, elevates monoaminergic neurotransmitter levels, and alleviates depressive symptoms.
Tryptophan (Trp) is an essential amino acid taken in by the human body from external sources. It serves as the primary metabolic precursor for the production of serotonin (5-HT) and kynurenine (KYN), which regulate various metabolic activities (Zádori et al., 2018). Under normal physiological conditions, the activity of indoleamine-2,3-dioxygenase (IDO) is low, and Trp is primarily metabolized by tryptophan-2,3-dioxygenase (TDO) in the liver. However, in the presence of inflammation or stress, proinflammatory cytokines can induce a significant increase in IDO activity, and elevated glucocorticoids further activate TDO, directing Trp toward the kynurenine pathway (KP), resulting in a decrease in central and peripheral 5-HT levels (Murakami et al., 2016). In normal mammals, approximately 40% of brain KYN comes from central metabolism, while the remaining 60% originates from the periphery. Therefore, peripheral KYN levels may to some extent represent the levels of central KP metabolites (Li et al., 2020a). In 72 untreated depressive patients, it was found that the levels of KP metabolites in cerebrospinal fluid were closely correlated with those in plasma, with the KYN/Trp ratio being the most correlated indicator (Haroon et al., 2020). In mice after treatment with CSS, the ratio of 5-HT/TRP in the liver significantly increased, while the ratio of KYN/TRP in tissues such as the liver, colon, and brain significantly decreased (Mengyu et al., 2021). Additionally, CSS reduced the activity and expression levels of TDO in the liver, leading to an increase in mouse 5-HT expression levels and the alleviation of depression-like behavior.
4.2 Maintaining homeostasis in the regulated HPA axis
One prominent feature of depression is the dysfunction of the hypothalamic-pituitary-adrenal (HPA) axis (Menke, 2023). HPA axis hyperactivity is particularly common in individuals with depression and can affect their cognitive functions (Cherian et al., 2019). Excessive stress stimuli to the brain result in cortical impact, triggering the release of signals from the hypothalamus. This leads to increased secretion of corticotropin-releasing factor (CRF) in the HPA axis. Excess CRF is transported through the portal venous system to the pituitary, where it stimulates the synthesis of adrenocorticotropic hormone (ACTH). This, in turn, promotes the production of cortisol (CORT) by the adrenal cortex (Dwyer et al., 2020). CRF is a key regulator of the body’s stress response and is closely associated with various psychiatric disorders, including depression. Overproduction of CRF is a mechanism that activates the HPA axis (Montgomery et al., 2023). Furthermore, cortisol (CORT), which acts downstream of the HPA axis, plays a significant role in the pathogenesis of depression. The CORT levels in individuals with depression are closely related to their clinical symptoms (Holloway et al., 2023). In a study involving perimenopausal depression rats treated with CSS for 21 days, both CRH and CORT levels significantly increased, indicating that CSS can regulate CRH and CORT levels to restore HPA axis function (Shengqiang et al., 2015). Similarly, in the case of CUMS combined with social isolation-induced depression in rats, CSS exhibited the same antidepressant effects (Yunhui et al., 2009).
During stress, corticotropin-releasing hormone (CRH) secreted by the paraventricular nucleus (PVN) of the hypothalamus stimulates the secretion of ACTH and glucocorticoids (GC); corticosterone in rodents (Kim et al., 2019). Receptors for glucocorticoids primarily include mineralocorticoid receptors (MR) and glucocorticoid receptors (GR). During stress, the expression of GC increases dramatically. After crossing the blood-brain barrier, GC acts on hippocampal GR. The GC-GR complex formed in response to high GC concentrations inhibits CRH transcription in the hypothalamus, providing negative feedback regulation on the HPA axis, thus suppressing HPA axis hyperactivity (de Kloet and Joëls, 2023). GC levels are crucial indicators for evaluating HPA axis function. The hippocampus, as the central regulator of the HPA axis, is rich in GR and mineralocorticoid receptors (MR). It is highly susceptible to the influence of high concentrations of glucocorticoids during stress (Gong et al., 2023). As illustrated in Figure 3, when CSS was orally administered to CUMS rats continuously for 21 days, it was found that CRH levels increased and GR levels decreased in the hippocampus compared to the depression model group. This indicates that CSS can effectively weaken the negative feedback effect of GC on the HPA axis, alleviating HPA axis hyperactivity. Thus, CSS may regulate HPA axis hyperactivity by acting on the hippocampus to achieve its antidepressant effect (Fan et al., 2015).
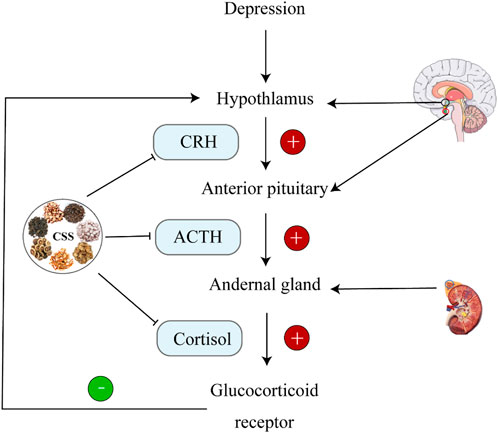
Figure 3. CSS Regulates HPA Axis Homeostasis. CSS maintains HPA axis homeostasis by inhibiting ACTH, CRH, and CORT.
4.3 Upregulation of BDNF levels
Brain-derived neurotrophic factor (BDNF) is a crucial member of the neurotrophic factor family, initially discovered in pig brain in the 1980s (Leibrock et al., 1989). BDNF is primarily synthesized in neurons and is distributed throughout the central nervous system (CNS). It plays a role in synaptic plasticity recovery, 5-HT signal transduction, and regulating the levels of 5-HT in the brain (Bhattarai et al., 2020; Costa et al., 2022). Numerous studies have shown that BDNF is closely associated with the occurrence, development, and treatment of depression. In the field of neurobiology, BDNF is one of the most studied neurotrophic factors (Amagase et al., 2023; Wang et al., 2023a). Changes in BDNF activity and levels in the brain are closely related to the development of depression. Autopsy results have revealed that the expression levels of BDNF in the plasma of depressed patients are lower than in the control group (Gadad et al., 2021). Furthermore, research suggests that developing antidepressants targeting BDNF might be one of the most effective strategies for future antidepressant drug development (Deltheil et al., 2009; Furukawa et al., 2019).
In a study involving CUMS rats subjected to 28 days of CSS oral administration, it was observed that compared to the depression model group, the expression of BDNF in the prefrontal cortex of rats significantly increased. This indicates that CSS can exert effects similar to the antidepressant venlafaxine by regulating BDNF expression levels (Zhang and Liu, 2020). Tropomyosin receptor kinase B (TrkB) is a specific receptor for BDNF, and it has been confirmed that activating BDNF-TrkB signaling can have an antidepressant effect (Ma et al., 2020). Figure 4 After intervening with CSS in depression model rats, it was found that compared to the model group, rats in the CSS group exhibited significantly increased expression of BDNF and its receptor TrkB in the hippocampus, prefrontal cortex, and amygdala, suggesting that the mechanism through which CSS alleviates depressive states might be related to the increased expression of BDNF and its receptor TrkB in these brain regions (Ying et al., 2011).
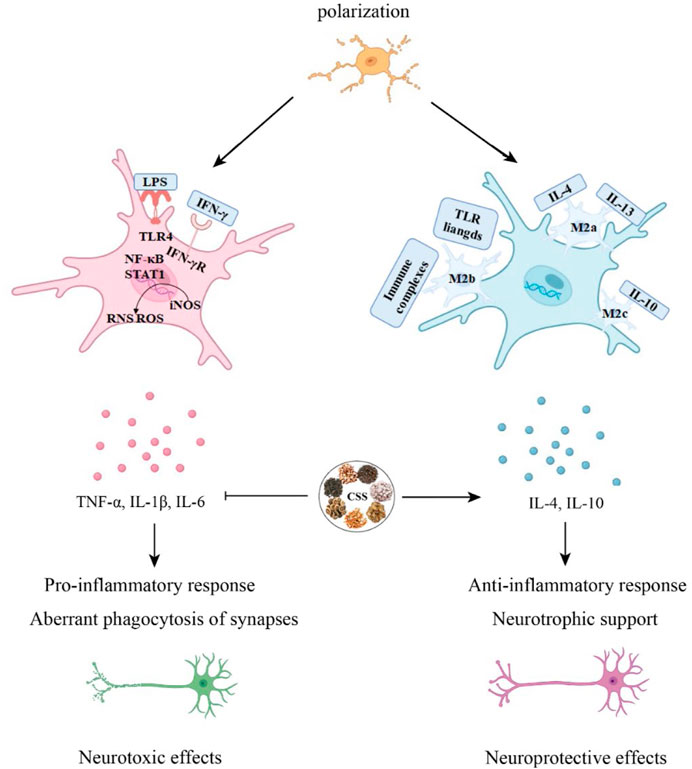
Figure 4. CSS alleviates neuroinflammation. Microglia can differentiate into M1 and M2 phenotypes. CSS is capable of exerting neuroprotective effects by modulating the immune response through the regulation of pro-inflammatory and anti-inflammatory cytokines in microglial activation.
4.4 Promoting synaptic plasticity
Synapses are the fundamental structural units responsible for transmitting information in neurons. Changes in their morphology, quantity, density, and signal transmission efficiency are collectively referred to as synaptic plasticity (Suvrathan et al., 2016; Sweatt, 2016). Synaptic plasticity serves as the neurobiological foundation for the growth and development of the nervous system, repair after injury, and the processes of learning and memory (Sehgal et al., 2013). Figure 5 When examining synaptic structural changes under electron microscopy, it is observed that in comparison to the model group, synaptic improvements occur in the hippocampal region of CUMS-depressed rats following CSS intervention, with an increase in synaptic quantity. This suggests that CSS can effectively enhance synaptic plasticity in the hippocampal region of depressed rats (Fan et al., 2018).
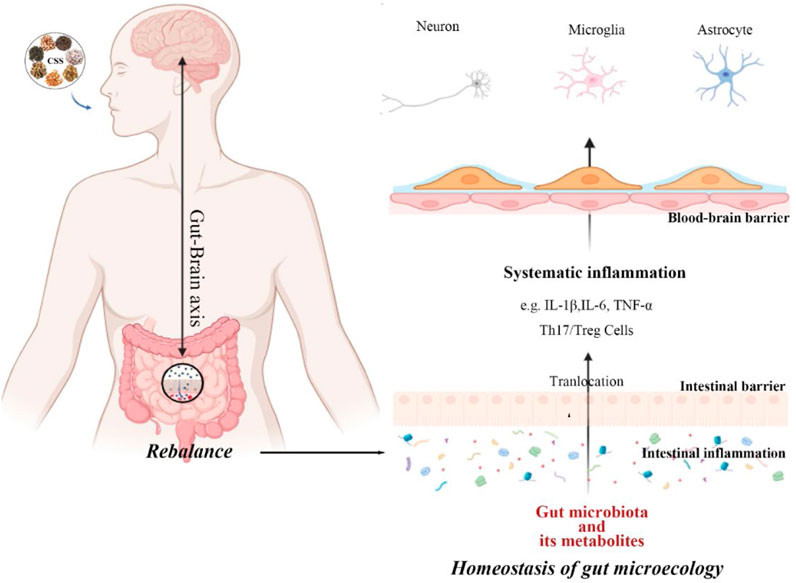
Figure 5. CSS restores dysbiosis of the gut microbiota. CSS exerts a protective effect on neuronal cells by regulating the gut microbiota through the brain-gut axis, activating the systemic immune response, and crossing the blood-brain barrier.
Two specific protein markers, PSD95 and SYN, are used to measure synaptic plasticity. PSD95 is abundantly expressed in the postsynaptic density region and interacts with membrane receptors, ion channels, and cell adhesion molecules, thereby participating in the regulation of synaptic plasticity and learning and memory abilities. SYN is a crucial presynaptic vesicle membrane protein, and its expression accurately reflects synaptic distribution, quantity, and density, making it the most direct indicator of changes in synaptic plasticity. Research indicates that the continuous administration of CSS through oral gavage to depressed rats for 2 weeks results in a significant increase in the deposition of Syn-positive substances in the CSS group compared to the model group. This suggests that CSS can effectively inhibit hippocampal neuronal apoptosis, impact the expression of SYN and PSD95, promote the recovery of damaged synaptic structure and function, and consequently ameliorate depressive-like behaviors in rats (Lan et al., 2023).
NMDAR (N-methyl-D-aspartate receptor) is a glutamate-gated ion channel that regulates neuronal survival, dendritic and axonal development in neurons, excitatory synaptic plasticity, and the formation of neuronal circuits (Tashiro et al., 2006). NMDAR is considered a necessary condition for dendritic spine structural remodeling and is believed to be the cellular basis of learning and memory (Matsuzaki et al., 2004). Downregulation of hippocampal NR2A/NR2B has been associated with cognitive impairment (Luciano-Jaramillo et al., 2019). According to the NMDAR signaling pathway, TrkB is rapidly and persistently activated, serving as a crucial binding partner for BDNF in various forms of synaptic plasticity. Studies indicate that the increase in BDNF exerts neuroprotective effects by transducing the NR2A-CaMKIV-TORC1 pathway (Xu et al., 2015). CSS enhances the expression of BDNF, NR2A, and NR2B in the hippocampus, suggesting that the antidepressant effects of CSS may be mediated through the transduction of the NR2A-CaMKIV-TORC1 pathway, impacting hippocampal neuronal development and thus restoring the neural function of damaged synapses (Xu et al., 2021).
4.5 Alleviating neuroinflammation
Neuroinflammation is an innate immune response of the nervous system (Meseguer et al., 2014), and it plays a role in the pathological processes of various neurological and psychiatric disorders. Patients with severe depression and animal models of depression often exhibit elevated levels of pro-inflammatory cytokines such as IL-1β, IL-6, and TNF-α (Yang et al., 2023). Cytokines are secreted proteins with growth, differentiation, and activation functions. They regulate and determine the nature of immune responses, control immune cell trafficking, and organize the cellular arrangement of immune organs (Borish and Steinke, 2003). In the context of neurodevelopment, the role of cytokines is reflected in pro-inflammatory cytokines impairing neurogenesis, while anti-inflammatory cytokines protect or promote neurogenesis (Soares et al., 2023).
Neuroglial cells play a significant role in the normal physiological processes of the central nervous system, including synaptic transmission, neural plasticity, regulation of neurons, and the local microenvironment (Fang et al., 2023). Microglial cells are the resident immune cells of the CNS, constituting approximately 5%–10% of CNS cells in mice and 0.5%–16.6% in human CNS cells. They participate in pathogen clearance, phagocytosis of dead or apoptotic cells, and the promotion or inhibition of inflammation to maintain brain health. Under normal physiological conditions, microglial cell bodies have elongated processes and continually monitor changes in the brain environment. However, in response to pathological stimuli, microglia are rapidly activated, their numbers increase, and their cell bodies enlarge while their processes become shorter (Zhu et al., 2023). Under pathological conditions, microglia transition from a resting state to an activated state, adopting two polarization phenotypes, M1 and M2. M1 microglial cells secrete many inflammatory mediators to engulf pathogens, while M2 microglial cells secrete protective cell regulators and participate in repairing local tissues and the microenvironment, thus protecting neural tissue (Smith et al., 2012; Pozzo et al., 2019). In an in vitro post-stroke depression model, microglial cell polarization shifts toward the M1 phenotype. However, treatment with GSK3β overexpression virus, CSS, or JAK-STAT3 inhibitors can reverse this polarization. Analysis of inflammation-related protein levels and expression of the JAK/STAT3-GSK3β/PTEN/Akt pathway reveals that the antidepressant effects of CSS may be achieved through the activation of the JAK/STAT3-GSK3β/PTEN/Akt pathway, regulating microglial cell polarization and suggesting that CSS exerts its antidepressant effects by inhibiting neuroinflammation (Fan et al., 2023). CSS can inhibit neuroinflammation in the brain by activating the important SIRT1/NF-κB inflammation signaling pathway in the prefrontal cortex of rats, thus exhibiting its antidepressant effects (Di and Liu, 2021). CSS can reduce the levels of serum TNF-α and IL-6 in depressed rats. It can also effectively inhibit the activation of hippocampal p38 mitogen-activated protein kinase (p38MAPK) and activate the extracellular signal-regulated kinase 5 (ERK5) signaling pathway to achieve its antidepressant effects (Qiu, 2014).
4.6 Regulation of biological activities within gut
Research has shown that the composition of the gut microbiota is altered in individuals with depression compared to healthy individuals, particularly in terms of microbial diversity and the relative abundance of specific bacterial taxa. This suggests a connection between gut dysbiosis and depression. In depression, the phyla Firmicutes, Actinobacteria, and Bacteroidetes are the most affected (Nikolova et al., 2021). After 28 days of gastric gavage treatment with CSS in CUMS, fresh fecal samples were collected and analyzed. It was observed that in the CSS group, the abundance of Firmicutes significantly increased, and the abundance of Actinobacteria decreased markedly compared to the model group. This indicates that CSS can regulate gut microbiota at the phylum level, mitigating the dysbiosis caused by chronic stress (Meng et al., 2020). In CRS mice, CSS intervention led to an increase in the relative abundance of Actinobacteria. While the results differ, a study that performed 16s rRNA sequencing of gut microbiota in patients with depression found a significant disruption in gut microbiota composition (Han et al., 2021). Differences in bacterial strain abundance led to a reduced ability of MDD patients to produce short-chain fatty acids (SCFA). The lack of SCFAs may weaken gut barrier function (Zheng et al., 2016). Metabolites from the gut, microbial cell components, and even the gut microbiota itself translocate systemically through a compromised gut barrier, often referred to as “leaky gut.” This exacerbates systemic inflammation, such as Th17/Treg imbalance, IL-6, IL-1β, and TNF-α, all of which are closely related to the pathogenesis of depression. Further induced immune responses may be related to the onset and development of depression (Młynarska et al., 2022). Therefore, the antidepressant effect of CSS may be achieved through the regulation of gut microbiota, reshaping the structure and composition of the gut microbiota, and exerting its effects through the microbiota-gut-brain axis (Ma et al., 2022).
4.7 System biology research
Traditional Chinese botanical drug formulations are characterized by complex compositions, making it challenging to characterize functional biomarker ingredients and understand the intricate compatibility rules of multiple ingredients. Research indicates that employing multi-omics approaches is more advantageous for identifying critical ingredients in traditional Chinese medicine (TCM), which is approach helps identify systemic targets for diseases and facilitate a deeper exploration of the mechanisms behind TCM formulations (Wang et al., 2021c). Commonly used methods in this context include network pharmacology, proteomics, and metabolomics.
Network pharmacology analysis has revealed 24 potential targets for the treatment of depression by CSS. Functional enrichment analysis indicates that the PI3K/AKT signaling pathway may be significantly influenced by CSS in MDD treatment. In vivo experiments demonstrate that CSS can ameliorate depressive-like behaviors in CUMS mice and promote neurogenesis. Additionally, CSS can increase the phosphorylation of PI3K/PI3K and AKT/AKT levels while reducing GSK3β/GSK3β levels in the hippocampus of CUMS mice (Zhang et al., 2021b). Research utilizing combined network pharmacology and bioinformatics analysis has identified that the mechanism of CSS primarily focuses on two main cascading signal modules. The first module involves a cascade reaction through ADCYAP1-ADCYAP2R3-GNAS-ADCY3-cAMP-PRKACA, controlling downstream genes like GRIA2, GRIN8A, GSK1A, CREB1, BDNF, FOS, ATF1, MAPK1, and JUND, which play a role in treating depression. The second module targets FOS for depression treatment through the DRD1/5-GNAQ-PLC B1-DAG-PRKCA cascade signal (Wang et al., 2021b).
Using quantitative proteomics and bioinformatics analysis methods to identify and analyze CSS, it appears that CSS may exert its antidepressant effects by influencing the synthesis, release, reuptake, and degradation pathways of GABA. Proteins such as Gad2, Vamp2, and Pde2a may be associated with the alleviation of depression by CSS. Therefore, these proteins may serve as potential antidepressant targets for CSS. Previous studies have demonstrated that CSS exerts its antidepressant effects by modulating the HPA axis and inhibiting neurotransmitter reuptake, such as norepinephrine, serotonin, and dopamine (Zhang et al., 2011b). Hence, it is hypothesized that CSS affects the glutamate and GABA signaling pathways via the HPA axis for depression treatment (Zhu et al., 2021) Table 1.
Serum metabolomics results indicate that 16 proteins and 63 genes are regulated by CSS. Sphingolipid metabolism and arachidonic acid metabolism appear to be the most significantly affected metabolic pathways related to depression induced by CUMS. Multiple studies have demonstrated that depression often accompanies sphingolipid metabolism disturbances (Li et al., 2022), and clinically, low levels of arachidonic acid in blood are associated with adult depression (Tsuchimine et al., 2015). Metabolomic studies of the hippocampus and serum in chronic variable stress (CVS)-induced rats reveal 10 metabolites from the hippocampus and 11 from serum that are considered potential biomarkers involved in the development of depression. CSS collaboratively regulates metabolic network abnormalities, including energy metabolism, neurotransmitter synthesis, tryptophan, phospholipids, fatty acids, bile acid metabolism, bone loss, and liver detoxification. CSS reverses the decrease in BDNF, ERK2/1, and pERK2 in CVS rats, suggesting that the ERK signaling system may be one of the targets for antidepressant effects of CSS (Su et al., 2014).
In summary, antidepressant effects of CSS are believed to be primarily related to monoamine neurotransmitters, the HPA axis, BDNF, synaptic plasticity, gut microbiota, and inflammatory responses (Figure 6; Table 2). It is thought to directly regulate these factors or indirectly act on various related proteins, receptors, or signaling pathways, which are primarily concentrated in the brain, the main targeted organ in current depression research.
5 Research on the antidepressant-like effects of the active ingredients of CSS
The ingredients detected in CSS using UPLC-QTOF-MS include ferulic acid, naringin, hesperidin, meranzin hydrate, glycyrrhizic acid, saikosaponin A, nobiletin, and hesperetin (Liu et al., 2020b). Additional ingredients such as ferulic acid, naringin, hesperidin, meranzin hydrate, glycyrrhizic acid, saikosaponin A, nobiletin, and hesperetin have also been identified in CSS.
HPLC analysis of CSS primarily identifies ingredients like naringin, neohesperidin, hesperidin, paeoniflorin, and glycyrrhetinic acid (Zhu et al., 2020b). LC-MS/MS analysis has revealed the presence of ingredients like paeoniflorin, ferulic acid, naringin, glycyrrhizic acid, saikosaponin A, chenpi flavonoid, and nobiletin in the water extract of CSS (Lei et al., 2021). These ingredients are considered the active ingredients responsible for the antidepressant-like effects of CSS.
In accordance with the 2020 edition of the Chinese Pharmacopoeia, the content of active ingredients in traditional Chinese medicine is regulated. For CSS, the minimum content of certain active ingredients is specified: Bupleuri radix should contain a total of not less than 0.30% of saikosaponin A (C42H68O13) and saikosaponin D (C42H68O13). Paeonia radix alba should contain not less than 1.2% of paeoniflorin (C23H28O11). Chuanxiong rhizoma should contain not less than 0.10% of ferulic acid (C10H10O4). Aurantii fructus should contain not less than 4.0% of naringin (C27H32O14). Citri reticulatae pericarpium should contain not less than 3.5% of hesperidin (C28H34O15). Cyperi rhizoma should contain not less than 1.0% of volatile oil, with Nootkatone as the characteristic ingredients. Glycyrrhizae radix et rhizome should contain not less than 0.50% of glycyrrhizin (C21H22O9) and not less than 2.0% of glycyrrhizic acid (C42H62O16). These standards ensure the quality and potency of CSS in traditional Chinese medicine. (They are listed in Table 3; Table 4).
5.1 Research on the antidepressant-like effects of saikosaponin
Saikosaponin possess a wide range of anti-inflammatory and immune-regulatory properties, making them effective ingredients in the treatment of depression with botanical drug medicine (Liu et al., 2020a; Wang et al., 2023b). The antidepressant effect of saikosaponin A may be associated with the modulation of DA levels and the transmembrane protein 2 rich in proline (PRRT2) in the hippocampus of depressive rats induced by CUMS (Guo et al., 2020). Saikosaponin A have been demonstrated to increase the expression of BDNF. In the MCAO + CUMS model, the administration of Chaihu Saponins significantly upregulated the protein levels of p-CREB, BDNF, Bax, and Caspase-3, promoting BDNF and Bcl-2 expression, consequently preventing hippocampal neuronal apoptosis and improving depressive-like behavior in post-stroke depression rats (Wang et al., 2021a).
As a critical regulator of the pro-inflammatory cytokine signaling pathway, NF-κB plays a pivotal role in immune responses, including stress, and has been associated with cognitive and memory impairments as well as depressive symptoms (Singh and Singh, 2020). Saikosaponin D can negatively regulate NF-κB, which, in turn, downregulates FGF2 expression through miR-155 downregulation. FGF2, as a key growth factor in synaptic plasticity and neuron growth, enhances synaptic plasticity and protects neurons, contributing to the antidepressant effect (Chao et al., 2020).
Saikosaponin A exhibits an antidepressant-like effect on peri-menopausal depressive rats induced by CUMS, and Saikosaponin A restores the hyperactivity of the HPA axis and pro-inflammatory cytokines while promoting BDNF-TrkB signaling in the hippocampus. SSA plays an antidepressant-like role in peri-menopausal rats by restoring hippocampal neuroendocrine, neuroinflammatory, and neurotrophic systems (Chen et al., 2018). Microglial cells serve as the first line of immune defense in the central nervous system, responding rapidly to external threats and secreting a substantial amount of oxidative stress substances and inflammatory cytokines, such as nitric oxide, reactive oxygen species, and IL-1β (Arioz et al., 2019). Activated microglial cells aim to remove antigens and restore brain homeostasis, but excessive activation can lead to neuronal damage. Saikosaponin D pretreatment effectively inhibits the release of inflammation-related cytokines (IL-1β, IL-6, and TNFα) mediated by activated microglial cells in vitro and in vivo. Saikosaponin D exerts its anti-inflammatory action by inhibiting the translocation and extracellular release of HMGB1, and inhibiting downstream TLR4/NF-κB signaling (Su et al., 2020). The elevated levels of glutamate induced by CUMS can lead to synaptic damage, which can be reversed through treatment with SSD. The amelioration of glutamate-induced synaptic injury appears to be mediated through the regulation of the Homer1-mGluR5 pathway and downstream mTOR signaling. It was observed that SSD treatment attenuated the increase in glutamate levels in the hippocampal CA1 region induced by chronic stress, which is known to contribute to synaptic damage and enhance the expression of synaptic proteins. Therefore, SSD may alleviate depressive-like behavior in CUMS-exposed rats by modulating the Homer1-mGluR5 and mTOR signaling pathways. These findings suggest that SSD can serve as a natural neuroprotective agent for the prevention of depression (Liu et al., 2022b). Therefore, saikosaponin A and saikosaponin D are promising ingredients in inhibiting the inflammatory response, which might be a potential mechanism underlying their antidepressant effects.
5.2 Research on the antidepressant-like effects of paeoniflorin
Paeoniflorin is a monoterpenoid glycoside obtained from the roots of peony or moutan, typically extracted from Bai Shao, a traditional low-toxicity Chinese botanical drug medicine widely used in the treatment of depression. Regardless of the type of stress animals are exposed to, reduced BDNF levels can potentially impact various brain functions, such as neurogenesis, neuron survival, and plasticity, promoting depressive-like behavior in stressed animals. Paeoniflorin exerts neuroprotective effects through the ERK-CREB signaling pathway, ameliorating depressive-like behavior in CUMS rats (Zhong et al., 2018). In an acute depression animal model induced by forced swimming, paeoniflorin significantly increases the serum and hippocampal BDNF levels in rats, offering protection against hippocampal pathological changes (Mu et al., 2020).
Oxidative stress is defined as an imbalance between the generation of reactive oxygen species (ROS) and the antioxidant capacity of cells, which is a major contributor to depression (Liu et al., 2023). Paeoniflorin can exhibit antidepressant effects through a multitarget pharmacological approach. For example, rats subjected to the forced swim test (FST) exhibit decreased plasma and hippocampal levels of serotonin, norepinephrine, and dopamine, as well as reduced plasma BDNF and superoxide dismutase (SOD) levels (Mu et al., 2020).
The HPA axis is associated with the pathophysiology of depression. Experimental evidence indicates that paeoniflorin can reverse depressive-like behavior. Neurogranin (Ng), a recently discovered postsynaptic protein widely distributed in the hippocampus, is found to be significantly lower in the hippocampus of depressive rats (Xiang et al., 2020). Paeoniflorin significantly increases the expression of Ng protein in the hippocampus. Furthermore, paeoniflorin decreases hippocampal glutamate (Glu) levels by inhibiting the expression of SNAP25, VAMP2, Syntaxin1a, and related proteins, as well as EAAT2/3, NR1, and NR2A proteins. These findings suggest that paeoniflorin can improve depressive-like behavior through modulation of the HPA axis, GR function, and Glu transporters (Li et al., 2020c).
Growing evidence suggests a close relationship between neuroinflammation and depression, making the inhibition of neuroinflammation a crucial treatment strategy for depression (Wei et al., 2023). Paeoniflorin can inhibit the TLR4/NF-κB/NLRP3 signaling pathway in the mouse hippocampus induced by LPS, reducing the levels of pro-inflammatory cytokines and microglial activation. Fibroblast growth factor-2 (FGF-2) plays a role in regulating neuron proliferation and differentiation (Koizumi, 2021). Paeoniflorin can increase FGF-2 levels and dendritic spine density. Therefore, paeoniflorin may exert its neuroprotective and antidepressant effects by inhibiting hippocampal microglial activation and activating the FGF-2/FGFR1 signaling pathway in neurons (Cheng et al., 2021).
5.3 Research on the antidepressant-like effects of ferulic acid
Ferulic acid (FA) is an aromatic acid originating from Chuanxiong (Szechuan lovage rhizome) and has shown antidepressant effects in clinical or animal studies (Zhang et al., 2018b). Among the various pharmacological actions of FA, its potential antidepressant effects have gained increasing attention. FA is a potent antioxidant that can scavenge hydrogen peroxide, hydroxyl radicals, superoxide radicals, and peroxynitrite (Xie et al., 2020a). Additionally, FA possesses characteristics of anti-apoptosis, anti-tumor, and anti-thrombotic properties. It can also inhibit neuroinflammatory responses and promote neurogenesis in the hippocampus (Kaur et al., 2022).
Hippocampal SIRT6 is involved in the in vivo lentivirus-mediated knockdown of depression induced by chronic unpredictable stress (CUS). Knockdown of hippocampal SIRT6 can prevent CUS-induced depressive-like phenotypes (Li et al., 2018). The PI3K/AKT signaling pathway is implicated in mediating antidepressant effects. The hippocampal SIRT6/AKT/CRMP2 signaling pathway is involved in the mechanism of antidepressant action of FA. CRMP2 is well-characterized as a target for neuronal plasticity and behavior regulation, regulating various aspects of neuron development, including axon guidance, dendritic morphology, and synaptic plasticity. Overexpression of CRMP2 effectively prevents acute axonal degeneration (Zhang et al., 2018a). CRMP2 activity is regulated by the SIRT6/AKT signaling pathway. Therefore, FA effectively activates the AKT/CRMP2 signaling pathway, improving CUS-induced depressive-like behavior and exerting neuroprotective effects through the SIRT6/AKT/CRMP2 pathway (Li et al., 2020b).
Ferulic acid can ameliorate depression in mice through antioxidative pathways. Studies have shown that ferulic acid increases the activities of SOD, catalase (CAT), and glutathione peroxidase (GSH-Px) in the blood, hippocampus, and cerebral cortex, while reducing levels of thiobarbituric acid reactive substances (TBA-RS). When the antioxidant defense system is modulated, ferulic acid can exert its antidepressant effects (Lenzi et al., 2015). In CUMS mice, FA improves depressive-like behavior in the tail suspension test (TST) and sucrose preference test (SPT). FA inhibits the activation of the NLRP3 inflammasome, the NF-κB signaling pathway, and the expression of IL-1β, IL-6, and TNF-α, suggesting that anti-inflammatory mechanisms are involved in antidepressant effects of ferulic acid in stressed mice (Liu et al., 2017).
Mitochondrial energy homeostasis mediated by ferulic acid is another potential mechanism of its antidepressant action. Dysfunction of mitochondria can lead to abnormal energy metabolism, triggering depressive episodes. Ferulic acid can promote glycogen metabolism in the brain, increase ATP levels, and activate neural function. FA also upregulates the gene expression of Ddc and Ppp1r1b, which are involved in dopamine signaling. FA treatment increases brain dopamine and norepinephrine levels, and thus, FA exerts its antidepressant effect by promoting dopamine synthesis (Sasaki et al., 2019).
Dysbiosis of the gut microbiota is closely associated with the onset of depression. FA effectively reduces the levels of pro-inflammatory cytokines, such as IL-6, IL-1β, TNF-α, while increasing the anti-inflammatory cytokine IL-10 in serum. This suggests that FA reduces systemic inflammation. 16S rRNA sequencing reveals that FA intervention reduces the abundance of the Proteobacteria phylum and significantly increases the abundance of Lactobacillus spp., Allobaculum spp., and Unspecified_S24_7. FA may exert its antidepressant effects by upregulating short-chain fatty acids. Furthermore, UHPLC-QTOF-MS/MS detection reveals that FA affects 459 microbial metabolites, primarily involved in tryptophan or thiamine metabolism. Tryptophan is an amino acid clinically used to alleviate depression, and a causal relationship exists between disrupted thiamine metabolism and depression (Wilson et al., 2023). Therefore, FA may exert its antidepressant effects by inhibiting pro-inflammatory cytokines, remodeling the gut microbiota, and modulating microbial metabolism (Hao et al., 2021).
5.4 Research on the antidepressant-like effects of naringin
Naringin is an active ingredient derived from the pericarp of citrus fruits, exhibiting a wide range of biological activities, such as anti-inflammatory and antioxidative properties (Kim and Kim, 2017), and has been employed in alleviating various neurological disorders (Emran et al., 2022). Adult hippocampal neurogenesis is crucial in the establishment of circuitry networks involving emotion-regulating regions like the amygdala and hypothalamus. Naringin promotes neuronal differentiation in normal mice, induces neural stem/progenitor cell (NSPC) migration to the subventricular zone-olfactory bulb (SVZ-OB) system, accelerates neuronal maturation in CORT-induced depressed mice, and enhances dendritic arborization, thereby exerting its antidepressant and anxiolytic effects via the promotion of neuronal differentiation target CREB (Gao et al., 2022). Moreover, naringin enhances BDNF through the cAMP-CREB-BDNF signaling pathway while suppressing neuroinflammation and neuronal apoptosis, ameliorating depressive behaviors in CORT-induced depressed mice (Zhang et al., 2023). Neurobehavioral characteristics of naringin are also accompanied by increased neuro-antioxidative and cholinergic activities. Furthermore, it significantly reduces the levels of malondialdehyde and nitrites, indicating involvement in the oxidative/nitrosative pathways, suggesting that naringin treatment may contribute to functional behavioral effects through mechanisms associated with enhanced cholinergic transmission, antioxidant defense systems, and the inhibition of lipid peroxidation and nitrosative processes (Ben-Azu et al., 2019). Rutin increases the levels of GAD67 in the striatum, prefrontal cortex, and hippocampus while reducing AChE activity. Additionally, Rutin region-dependently decreases TNF-α, IL-6, malondialdehyde, and nitrite concentrations while increasing glutathione levels. Our study suggests that Naringin attenuates SDS-induced depressive-like behaviors by increasing GAD67 synthesis, inhibiting AChE activity, oxidative and nitrosative stress, and neuroinflammation processes in stress-sensitive brain regions (Oladapo et al., 2021).
5.5 Research on the antidepressant-like effects of hesperidin
Hesperidin, an active ingredient derived from tangerine peel, possesses antioxidative, anti-inflammatory, and antiviral properties, while also promoting neurogenesis and enhancing memory function (Li et al., 2023). Studies have indicated that hesperidin exhibits antidepressant effects (Li et al., 2016). Hesperidin has been found to decrease the expression of the NLRP3 inflammasome (NLRP3, caspase-1, and ASC) in the prefrontal cortex (PFC) of rats induced with CUMS. It reduces the number of activated microglia in the PFC and levels of pro-inflammatory cytokines (IL-1β, IL-6, and TNF-α). Elevated levels of pro-inflammatory cytokines released by activated microglia contribute to neuroinflammatory responses, and hesperidin can inhibit the activation of pro-inflammatory cytokines in microglia induced by LPS and the NLRP3 signaling pathway (Xie et al., 2020b). In a comorbid diabetic depression rat model, hesperidin also exerts antidepressant effects. Hesperidin reduces depressive behaviors in diabetic rats by enhancing the function of Glo-1 in the amygdala and hippocampus, thereby reducing the formation of advanced glycation end products (AGEs) and oxidative stress-induced damage. Furthermore, the upregulation of Glo-1 by hesperidin is associated with the activation of the Nrf2/ARE signaling pathway, further elucidating the molecular mechanisms through which hesperidin inhibits the formation of brain AGEs and oxidative stress in diabetic rats. In vitro studies suggest that hesperidin treatment can reverse the reduced expression of Nrf2 induced by high glucose (HG). Therefore, the antidepressant-like effects of hesperidin are primarily mediated through the activation of the Nrf2/ARE/Glo-1 pathway (Zhu et al., 2020a). In a rat model of depression induced by PTSD, hesperidin can ameliorate depressive-like behavior in rats by inhibiting the activity of MAO-A and the expression of tryptophan hydroxylase-1 in the hippocampus. This leads to an increase in the release of serotonin (5-HT) (Lee et al., 2021).
5.6 Research on the antidepressant-like effects o research on the antidepressant-like effects of nootkatone
Nootkatone is the primary monomeric ingredient found in lotus plumule and exhibits various pharmacological properties, including antimicrobial, antioxidant, and anti-allergic activities. Nootkatone functions as a neuroprotective agent and improves cognitive impairments by inhibiting neuroinflammation through the TLR4/NF-κB/NLRP3 pathway in the hippocampus (Wang et al., 2018). Recent studies have shown that nootkatone also possesses antidepressant and anxiolytic effects by activating the Keap1/Nrf2/HO-1 antioxidant pathway in response to liver injury-induced depression (Yan et al., 2021). Nootkatone significantly increases the reduced expression of PKA and p-CREB caused by CUS, suggesting that the PKA/CREB pathway may be involved in regulating BDNF expression in response to nootkatone treatment. Additionally, Nootkatone improves neurogenesis in the hippocampal dentate gyrus, indicating that it may exert antidepressant effects by enhancing neurogenesis. Therefore, nootkatone likely exhibits antidepressant activity through increasing neurogenesis in the hippocampal dentate gyrus and activating the PKA/CREB pathway to enhance BDNF expression (Wang et al., 2022). In a CUMS-induced depression model, Nootkatone improves depressive symptoms by inhibiting neuroinflammation mediated by the NF-κB/NLRP3 pathway (Zhao et al., 2023a).
5.7 Research on the antidepressant-like effects of glycyrrhizin
Glycyrrhizin, the principal active ingredient in licorice, possesses pharmacological properties such as anti-inflammatory and antioxidant effects. A metabolomics analysis of the effects of Glycyrrhizin on depression in rats was conducted using UHPLC-Q-TOF MS and UHPLC-TQ MS, revealing the screening of 47 different metabolites in the urine of depressed rats. This suggests that Glycyrrhizin may exert its antidepressant effects through metabolic pathways (Yang et al., 2020). Glycyrrhizin can lower the levels of FGF-2 in the hippocampus of LPS-induced depressive model mice, increase dendritic spine density, and significantly reduce the number of Iba-1 positive cells. This indicates that Glycyrrhizin may exert its antidepressant effects by inhibiting the activation of microglial cells, suppressing the release of pro-inflammatory cytokines, and protecting dendritic spine morphology in the hippocampal region (Chen et al., 2020). Research also suggests that Glycyrrhizin exerts its antidepressant effects in part by inhibiting the levels of cytokines triggered by the NLRP3 inflammasome, highlighting the role of antioxidative stress and the inhibition of NLRP3 inflammasome activation in antidepressant properties of Glycyrrhizin (Liu et al., 2022a). Glycyrrhizin is applicable in various depression models, as it can reduce the levels of FSH and estradiol E2 in the serum, improve the decreased 5-HT and NE levels in the hypothalamus, and increase the levels of monoamine neurotransmitters, thereby ameliorating depressive-like behavior in perimenopausal rats (Lan et al., 2020).
6 Discussion
Extensive research has confirmed that the antidepressant mechanisms of CSS are primarily associated with the monoaminergic neurotransmitter system, the HPA axis, synaptic plasticity, BDNF, gut microbiota, neuroinflammation, among other factors. Additionally, the therapeutic effects of CSS extend to various brain regions, such as the hippocampus, prefrontal cortex, amygdala, and hypothalamus, as well as regulatory systems outside the central nervous system, including the liver, gastrointestinal tract, and endocrine system. Studies on the main active ingredients of CSS, such as saikosaponin, paeoniflorin, ferulic acid, and Nootkatone, have demonstrated their antidepressant effects. This suggests that antidepressant properties of CSS involve multiple targets, levels, and systems, making it a promising treatment for depression. However, the safety of the antidepressant mechanisms of CSS has not been fully elucidated. Therefore, it is crucial to identify and assess the quality of the effective ingredients within CSS.
While the efficacy of CSS as a holistic formula is well-established, the mechanistic explanations remain uncertain due to the limited comprehensive, systematic, and in-depth exploratory studies combining clinical trials, animal experiments, and cellular research. Further investigations using techniques like high-performance liquid chromatography and metabolomics are needed to explore which medicinal molecules can effectively cross the blood-brain barrier. Identifying the key molecules and understanding whether they exert antidepressant effects through gut microbiota or metabolic pathways is also an important area of research. Based on our current understanding and the limited scope of this paper, we have only discussed the mechanisms by which a subset of the identified active metabolites in CSS exert their effects. However, it is important to note that a botanical drug decoction may contain thousands of compounds, targeting multiple molecular pathways. Therefore, further identification of the effective ingredients in the decoction and their antidepressant effects remains a crucial area for future research (Xu et al., 2023).
7 Limitations and further perspective
While research on the mechanisms underlying the antidepressant effects of CSS and its active components has made certain advancements, there are inherent limitations in the existing studies. Firstly, most traditional Chinese medicine (TCM) compounds are characterized by intricate active ingredients, and previous research on the quality control of CSS lacks a standardized approach. This issue is not unique to CSS but is a common challenge in the study of various traditional botanical drug formulations. Discrepancies in the drug proportions of CSS have been observed in different studies, stemming from the clinical emphasis in TCM on individualized treatment based on specific patient conditions. However, such variations in drug composition adjustments are challenging to replicate in basic research. Therefore, it is imperative to establish reasonable research standards for prescription formulation in the course of basic research. Given the complexity of Chinese botanical drug ingredients, the mechanisms of action are not confined to a single component or target but involve numerous targets. Nevertheless, many experimental methods, primarily animal studies, tend to focus on revealing single pathways or individual target effects. Consequently, comprehensively and adequately exploring and elucidating the pharmacological mechanisms of CSS’s active components and the interactions between different mechanisms pose challenging tasks. In the future, TCM research could benefit from a multi-omics approach (metabolomics, transcriptomics, and proteomics) to extensively screen molecular mechanisms and potential targets for treating depression, leveraging the advantageous synergies of TCM in targeting multiple pathways, mechanisms, levels, and factors.
Secondly, studies on Chinese botanical drug medicine often underscore the absorption of relevant components into the bloodstream, their distribution in organs, metabolism, and excretion. However, the chemical composition of Chinese botanical drug medicine is highly intricate, and not all components may be absorbed into the bloodstream. Previous observations have noted that some botanical drug medicine components exhibit low bioavailability, with very low or undetectable concentrations in the blood. Enhancing the bioavailability of these components and focusing on constructing precise and efficient targeted systems are conducive to significantly improving treatment efficacy. However, there is limited research on enhancing the bioavailability of CSS in treating depression. Therefore, future studies should explore effective strategies for enhancing drug efficacy to provide clinical assistance, rather than merely validating its pharmacological mechanisms.
In practical clinical applications, Chinese botanical drug medicine is often a combination of prescribed herbs. However, current research predominantly concentrates on verifying the anti-depressive effects of individual components in the medication, deviating from traditional efficacy and prescription rules. Through extensive literature review and summarization, we propose a new research direction. Ferulic acid is identified as a crucial active component in CSS, with research indicating that its antidepressant effects at a dose of 50 mg/kg are similar to the overall prescription of CSS. Hence, exploring a novel combination approach by adding 50 mg/kg of ferulic acid to the overall CSS prescription may enhance the overall efficacy of CSS. This will be a focus of our future research.
It is essential to acknowledge that CSS lacks high-quality standardized clinical trials, including large-scale multicenter, randomized double-blind controlled trials, to validate its clinical efficacy in treating depression. Recognizing the involvement of multiple factors in the occurrence and development of diseases is crucial. For complex disorders like depression, a single etiology cannot fully explain its onset and progression. Therefore, the metabolism and transformation of CSS within the human body should be incorporated into future research. Integrated research utilizing various technologies and methods is necessary to comprehensively elucidate the antidepressant mechanisms of CSS, paving the way for new combination methods to increase drug effectiveness. This will establish a reliable scientific foundation for botanical drug antidepressant development and promote advancements in the field of TCM for treating depression.
Author contributions
ZG: Writing–review and editing, Writing–original draft, Visualization, Software, Investigation, Formal Analysis, Data curation, Conceptualization. TL: Writing–review and editing, Methodology. JY: Writing–review and editing, Conceptualization. YL: Writing–review and editing, Supervision, Methodology, Investigation. LX: Writing–review and editing, Funding acquisition. MC: Writing–review and editing, Funding acquisition.
Funding
The author(s) declare financial support was received for the research, authorship, and/or publication of this article. The Science and Technology Development Fund, Macau SAR (file No. 0114/2022/A); The Project of Guizhou Provincial Natural Science Foundation, QKH-J [2020]1Y378; Special Scientific Research Project for the Construction of “Double First-Class” in Traditional Chinese Medicine in Henan Province (Project No. HSRPDFCTCM-2023-1-18, Research on the Compatibility of Prescriptions Based on Changes in Key Pharmacodynamic Ingredient Content.
Acknowledgments
We sincerely thank JY for his selfless support to the project.
Conflict of interest
The authors declare that the research was conducted in the absence of any commercial or financial relationships that could be construed as a potential conflict of interest.
Publisher’s note
All claims expressed in this article are solely those of the authors and do not necessarily represent those of their affiliated organizations, or those of the publisher, the editors and the reviewers. Any product that may be evaluated in this article, or claim that may be made by its manufacturer, is not guaranteed or endorsed by the publisher.
References
Amagase, Y., Kambayashi, R., Sugiyama, A., and Takei, Y. (2023). Peripheral regulation of central brain-derived neurotrophic factor expression through the vagus nerve. Int. J. Mol. Sci. 24, 3543. doi:10.3390/ijms24043543
Arioz, B. I., Tastan, B., Tarakcioglu, E., Tufekci, K. U., Olcum, M., Ersoy, N., et al. (2019). Melatonin attenuates LPS-induced acute depressive-like behaviors and microglial NLRP3 inflammasome activation through the SIRT1/nrf2 pathway. Front. Immunol. 10, 1511. doi:10.3389/fimmu.2019.01511
Basiri, R., Seidu, B., and Rudich, M. (2023). Exploring the interrelationships between diabetes, nutrition, anxiety, and depression: implications for treatment and prevention strategies. Nutrients 15, 4226. doi:10.3390/nu15194226
Ben-Azu, B., Nwoke, E. E., Aderibigbe, A. O., Omogbiya, I. A., Ajayi, A. M., Olonode, E. T., et al. (2019). Possible neuroprotective mechanisms of action involved in the neurobehavioral property of naringin in mice. Biomed. Pharmacother. 109, 536–546. doi:10.1016/j.biopha.2018.10.055
Berk, M., KöHLER-Forsberg, O., Turner, M., Penninx, B., Wrobel, A., Firth, J., et al. (2023). Comorbidity between major depressive disorder and physical diseases: a comprehensive review of epidemiology, mechanisms and management. World Psychiatry 22, 366–387. doi:10.1002/wps.21110
Bhattarai, P., Cosacak, M. I., Mashkaryan, V., Demir, S., Popova, S. D., Govindarajan, N., et al. (2020). Neuron-glia interaction through Serotonin-BDNF-NGFR axis enables regenerative neurogenesis in Alzheimer's model of adult zebrafish brain. PLoS Biol. 18, e3000585. doi:10.1371/journal.pbio.3000585
Borish, L. C., and Steinke, J. W. (2003). 2. Cytokines and chemokines. J. Allergy Clin. Immunol. 111, S460–S475. doi:10.1067/mai.2003.108
Cai, W., and Bao, H. (2023). Clinical study of Chaihu Shugan powder in the treatment of postpartum depression with stagnation of liver qi. Henan Tradit. Chin. Med. 43, 1208–1212.
Chao, B., Huang, S., Pan, J., Zhang, Y., and Wang, Y. (2020). Saikosaponin d downregulates microRNA-155 and upregulates FGF2 to improve depression-like behaviors in rats induced by unpredictable chronic mild stress by negatively regulating NF-κB. Brain Res. Bull. 157, 69–76. doi:10.1016/j.brainresbull.2020.01.008
Chen, GAIHUA, Lixia, H. A. O., Jinyun, P. E. I., Qiyan, D. A. I., Jianyun, G. U. O., and Zhao, S. (2021). Clinical efficacy of Chaihu Shuohe San combined with psychotherapy on post-stroke depression and liver qi stagnation. J. Shanxi Univ. Traditional Chin. Med. 22, 371–374.
Chen, X. Q., Chen, S. J., Liang, W. N., Wang, M., Li, C. F., Wang, S. S., et al. (2018). Saikosaponin A attenuates perimenopausal depression-like symptoms by chronic unpredictable mild stress. Neurosci. Lett. 662, 283–289. doi:10.1016/j.neulet.2017.09.046
Cheng, J., Chen, M., Wan, H. Q., Chen, X. Q., Li, C. F., Zhu, J. X., et al. (2021). Paeoniflorin exerts antidepressant-like effects through enhancing neuronal FGF-2 by microglial inactivation. J. Ethnopharmacol. 274, 114046. doi:10.1016/j.jep.2021.114046
Chen, M., Zhang, Q. P., Zhu, J. X., Cheng, J., Liu, Q., Xu, G. H., et al. (2020). Involvement of FGF-2 modulation in the antidepressant-like effects of liquiritin in mice. Eur. J. Pharmacol. 881, 173297. doi:10.1016/j.ejphar.2020.173297
Cherian, K., Schatzberg, A. F., and Keller, J. (2019). HPA axis in psychotic major depression and schizophrenia spectrum disorders: cortisol, clinical symptomatology, and cognition. Schizophr. Res. 213, 72–79. doi:10.1016/j.schres.2019.07.003
Costa, R. O., Martins, L. F., Tahiri, E., and Duarte, C. B. (2022). Brain-derived neurotrophic factor-induced regulation of RNA metabolism in neuronal development and synaptic plasticity. Wiley Interdiscip. Rev. RNA 13, e1713. doi:10.1002/wrna.1713
De Kloet, E. R., and JoëLS, M. (2023). The cortisol switch between vulnerability and resilience. Mol. Psychiatry. doi:10.1038/s41380-022-01934-8
Deltheil, T., Tanaka, K., Reperant, C., Hen, R., David, D. J., and Gardier, A. M. (2009). Synergistic neurochemical and behavioural effects of acute intrahippocampal injection of brain-derived neurotrophic factor and antidepressants in adult mice. Int. J. Neuropsychopharmacol. 12, 905–915. doi:10.1017/S1461145709000017
Di, XIAO, and Liu, J. (2021). To study the effect of Chaihu Shugan powder on SIRT1/NF-κB signaling pathway in the prefrontal cortex of depression model rats. J. Hubei Univ. Chin. Med. 23, 9–14.
Ding, H., Wang, B., and Xu, Q. (2018). Meta-analysis of clinical RCTS of Chaihu Shugan powder in the treatment of depression. Drug Eval. China 35, 300–304.
Dwyer, J. B., Aftab, A., Radhakrishnan, R., Widge, A., Rodriguez, C. I., Carpenter, L. L., et al. (2020). Hormonal treatments for major depressive disorder: state of the art. Am. J. Psychiatry 177, 686–705. doi:10.1176/appi.ajp.2020.19080848
Emiko Tsugiyama, L., Carlos Macedo Moraes, R., Andrade Cavalcante Moraes, Y., and Francis-Oliveira, J. (2023). Promising new pharmacological targets for depression: the search for efficacy. Drug Discov. Today 28, 103804. doi:10.1016/j.drudis.2023.103804
Emran, T. B., Islam, F., Nath, N., Sutradhar, H., Das, R., Mitra, S., et al. (2022). Naringin and naringenin polyphenols in neurological diseases: understandings from a therapeutic viewpoint. Life (Basel) 13, 99. doi:10.3390/life13010099
Fan, DAHUA, Sun, NINGNING, Zhengzhi, W., Huanmin, L. U. O., and Cao, M. (2018). Molecular mechanism of Chaihu-Shugan powder regulating hippocampal neuroplasticity in depression model of liver-depression syndrome. World J. Integr. Chin. West. Med. 13, 1190–1193+1206.
Fan, WEIHONG, Fang, Y. U., and Yao, J. (2015). Effect of Chaihu Shugan powder on regulating HPA axis function in hippocampus of chronic stress depression model rats. Chin. J. Basic Med. Traditional Chin. Med. 21, 50–52.
Fang, S., Wu, Z., Guo, Y., Zhu, W., Wan, C., Yuan, N., et al. (2023). Roles of microglia in adult hippocampal neurogenesis in depression and their therapeutics. Front. Immunol. 14, 1193053. doi:10.3389/fimmu.2023.1193053
Fan, Q., Liu, Y., Sheng, L., Lv, S., Yang, L., Zhang, Z., et al. (2023). Chaihu-Shugan-San inhibits neuroinflammation in the treatment of post-stroke depression through the JAK/STAT3-GSK3β/PTEN/Akt pathway. Biomed. Pharmacother. 160, 114385. doi:10.1016/j.biopha.2023.114385
Furukawa, Y., Hara, R. I., Nakaya, M., Okuyama, S., Sawamoto, A., and Nakajima, M. (2019). Citrus auraptene induces glial cell line-derived neurotrophic factor in C6 cells. Int. J. Mol. Sci. 21, 253. doi:10.3390/ijms21010253
Gadad, B. S., Vargas-Medrano, J., Ramos, E. I., Najera, K., Fagan, M., Forero, A., et al. (2021). Altered levels of interleukins and neurotrophic growth factors in mood disorders and suicidality: an analysis from periphery to central nervous system. Transl. Psychiatry 11, 341. doi:10.1038/s41398-021-01452-1
Gao, C., Wu, M., Du, Q., Deng, J., and Shen, J. (2022). Naringin mediates adult hippocampal neurogenesis for antidepression via activating CREB signaling. Front. Cell Dev. Biol. 10, 731831. doi:10.3389/fcell.2022.731831
GBD 2019 Mental Disorders Collaborators (2022). Global, regional, and national burden of 12 mental disorders in 204 countries and territories, 1990-2019: a systematic analysis for the Global Burden of Disease Study 2019. Lancet Psychiatry 9, 137–150. doi:10.1016/S2215-0366(21)00395-3
Gong, W., Chen, J., Xu, S., Li, Y., Zhou, Y., and Qin, X. (2023). The regulatory effect of Angelicae Sinensis Radix on neuroendocrine-immune network and sphingolipid metabolism in CUMS-induced model of depression. J. Ethnopharmacol. 319, 117217. doi:10.1016/j.jep.2023.117217
Greenberg, P. E., Fournier, A.-A., Sisitsky, T., Pike, C. T., and Kessler, R. C. (2015). The economic burden of adults with major depressive disorder in the United States (2005 and 2010). J. Clin. psychiatry 76, 155–162. doi:10.4088/JCP.14m09298
Guo, J., Zhang, F., Gao, J., Guan, X., Liu, B., Wang, X., et al. (2020). Proteomics-based screening of the target proteins associated with antidepressant-like effect and mechanism of Saikosaponin A. J. Cell Mol. Med. 24, 174–188. doi:10.1111/jcmm.14695
Haiying, DONG, Guihua, XING, Deshui, L. I. U., and Zhang, X. (2011). Effects of Chaihu Shuohe San on the activities of hippocampal monoamine oxidase and acetylcholinesterase in rats with depression model. Chin. J. Gerontology 31, 809–811.
Han, S. K., Kim, J. K., Park, H. S., Shin, Y. J., and Kim, D. H. (2021). Chaihu-Shugan-San (Shihosogansan) alleviates restraint stress-generated anxiety and depression in mice by regulating NF-κB-mediated BDNF expression through the modulation of gut microbiota. Chin. Med. 16, 77. doi:10.1186/s13020-021-00492-5
Hao, W. Z., Ma, Q. Y., Tao, G., Huang, J. Q., and Chen, J. X. (2021). Oral coniferyl ferulate attenuated depression symptoms in mice via reshaping gut microbiota and microbial metabolism. Food Funct. 12, 12550–12564. doi:10.1039/d1fo02655k
Haroon, E., Welle, J. R., Woolwine, B. J., Goldsmith, D. R., Baer, W., Patel, T., et al. (2020). Associations among peripheral and central kynurenine pathway metabolites and inflammation in depression. Neuropsychopharmacology 45, 998–1007. doi:10.1038/s41386-020-0607-1
Holloway, A. L., Schaid, M. D., and Lerner, T. N. (2023). Chronically dysregulated corticosterone impairs dopaminergic transmission in the dorsomedial striatum by sex-divergent mechanisms. Neuropsychopharmacology 48, 1328–1337. doi:10.1038/s41386-023-01551-1
Huber, K., Szerenos, E., Lewandowski, D., Toczylowski, K., and Sulik, A. (2023). The role of adipokines in the pathologies of the central nervous system. Int. J. Mol. Sci. 24, 14684. doi:10.3390/ijms241914684
Hwang, M., Kim, J. N., Lee, J. R., Kim, S. C., and Kim, B. J. (2020). Effects of chaihu-shugan-san on small intestinal interstitial cells of cajal in mice. Biol. Pharm. Bull. 43, 707–715. doi:10.1248/bpb.b19-01058
Jahanabadi, S., Amiri, S., Karkeh-Abadi, M., and Razmi, A. (2023). Natural psychedelics in the treatment of depression; a review focusing on neurotransmitters. Fitoterapia 169, 105620. doi:10.1016/j.fitote.2023.105620
Ji, Y., Du, Z., Zheng, K., Jiang, Y., Ren, C., Zhu, H., et al. (2023). Bidirectional causal association between ischemic stroke and five mental disorders. Acta Psychiatr. Scand. 148, 359–367. doi:10.1111/acps.13606
Kaur, R., Sood, A., Lang, D. K., Arora, R., Kumar, N., Diwan, V., et al. (2022). Natural products as sources of multitarget compounds: advances in the development of ferulic acid as multitarget therapeutic. Curr. Top. Med. Chem. 22, 347–365. doi:10.2174/1568026622666220117105740
Kim, H. J., and Kim, B. J. (2017). Naringenin inhibits pacemaking activity in interstitial cells of Cajal from murine small intestine. Integr. Med. Res. 6, 149–155. doi:10.1016/j.imr.2017.02.001
Kim, J. S., Han, S. Y., and Iremonger, K. J. (2019). Stress experience and hormone feedback tune distinct components of hypothalamic CRH neuron activity. Nat. Commun. 10, 5696. doi:10.1038/s41467-019-13639-8
Koizumi, S. (2021). Glial purinergic signals and psychiatric disorders. Front. Cell Neurosci. 15, 822614. doi:10.3389/fncel.2021.822614
Lan, X. Y., Yu, H., Chen, Q. J., Zhai, S., Zhang, C. F., Li, F., et al. (2020). Effect of liquiritin on neuroendocrine-immune network in menopausal rat model. Phytother. Res. 34, 2665–2674. doi:10.1002/ptr.6696
Lan, Y. U., Lihua, SONG, Chang, L. I. U., Ren, JIXIANG, Yunlong, X. U., and Nan, H. (2023). Mechanism of Chaihu Shugan powder on hippocampus of depressed rats. Chin. J. Gerontology 43, 1640–1645.
Lee, H. J., Lee, S. Y., Kim, J. H., Sung, I. K., Park, H. S., Jin, C. J., et al. (2010). Depressive mood and quality of life in functional gastrointestinal disorders: differences between functional dyspepsia, irritable bowel syndrome and overlap syndrome. Gen. Hosp. Psychiatry 32, 499–502. doi:10.1016/j.genhosppsych.2010.05.002
Lee, B., Choi, G. M., and Sur, B. (2021). Antidepressant-like effects of hesperidin in animal model of post-traumatic stress disorder. Chin. J. Integr. Med. 27, 39–46. doi:10.1007/s11655-020-2724-4
Lei, W. U., Yalin, L. I. U., and Huang, X. (2021). Quality markers of Chaihu Shugan powder for antidepressant efficacy based on biological prescription analysis pharmacological strategy. Chin. Herb. Med. 52, 2617–2625.
Leibrock, J., Lottspeich, F., Hohn, A., Hofer, M., Hengerer, B., Masiakowski, P., et al. (1989). Molecular cloning and expression of brain-derived neurotrophic factor. Nature 341, 149–152. doi:10.1038/341149a0
Lenzi, J., Rodrigues, A. F., RóS Ade, S., De Castro, A. B., De Lima, D. D., Magro, D. D., et al. (2015). Ferulic acid chronic treatment exerts antidepressant-like effect: role of antioxidant defense system. Metab. Brain Dis. 30, 1453–1463. doi:10.1007/s11011-015-9725-6
Li, C. C., Jiang, N., Gan, L., Zhao, M. J., Chang, Q., Liu, X. M., et al. (2020a). Peripheral and cerebral abnormalities of the tryptophan metabolism in the depression-like rats induced by chronic unpredicted mild stress. Neurochem. Int. 138, 104771. doi:10.1016/j.neuint.2020.104771
Li, C. F., Chen, S. M., Chen, X. M., Mu, R. H., Wang, S. S., Geng, D., et al. (2016). ERK-dependent brain-derived neurotrophic factor regulation by hesperidin in mice exposed to chronic mild stress. Brain Res. Bull. 124, 40–47. doi:10.1016/j.brainresbull.2016.03.016
Li, Y. C., Zheng, X. X., Xia, S. Z., Li, Y., Deng, H. H., Wang, X., et al. (2020c). Paeoniflorin ameliorates depressive-like behavior in prenatally stressed offspring by restoring the HPA axis- and glucocorticoid receptor-associated dysfunction. J. Affect Disord. 274, 471–481. doi:10.1016/j.jad.2020.05.078
Lijuan, H. E., Wang, YULAI, Yufeng, M. A., Guo, R., Zhang, X., Dan, L., et al. (2012). Effects of Chaihu Shugan powder on behavior and plasma IL-6 and TNF-α in rats with stagnation of liver-qi syndrome. Beijing Tradit. Chin. Med. 31, 380–382.
Li, L., Yu, A. L., Wang, Z. L., Chen, K., Zheng, W., Zhou, J. J., et al. (2019). Chaihu-Shugan-San and absorbed meranzin hydrate induce anti-atherosclerosis and behavioral improvements in high-fat diet ApoE(-/-) mice via anti-inflammatory and BDNF-TrkB pathway. Biomed. Pharmacother. 115, 108893. doi:10.1016/j.biopha.2019.108893
Liu, C. Y., Chen, J. B., Liu, Y. Y., Zhou, X. M., Zhang, M., Jiang, Y. M., et al. (2022b). Saikosaponin D exerts antidepressant effect by regulating Homer1-mGluR5 and mTOR signaling in a rat model of chronic unpredictable mild stress. Chin. Med. 17, 60. doi:10.1186/s13020-022-00621-8
Liu, Y. M., Shen, J. D., Xu, L. P., Li, H. B., Li, Y. C., and Yi, L. T. (2017). Ferulic acid inhibits neuro-inflammation in mice exposed to chronic unpredictable mild stress. Int. Immunopharmacol. 45, 128–134. doi:10.1016/j.intimp.2017.02.007
Liu, C., Yuan, D., Zhang, C., Tao, Y., Meng, Y., Jin, M., et al. (2022a). Liquiritin alleviates depression-like behavior in CUMS mice by inhibiting oxidative stress and NLRP3 inflammasome in Hippocampus. Evid. Based Complement. Altern. Med. 2022, 7558825. doi:10.1155/2022/7558825
Liu, L., Liu, M., Xiu, J., Zhang, B., Hu, H., Qiao, M., et al. (2023). Stimuli-responsive nanoparticles delivered by a nasal-brain pathway alleviate depression-like behavior through extensively scavenging ROS. Acta Biomater. 171, 451–465. doi:10.1016/j.actbio.2023.09.038
Liu, M., Zhang, G., Naqvi, S., Zhang, F., Kang, T., Duan, Q., et al. (2020a). Cytotoxicity of Saikosaponin A targets HEKa cell through apoptosis induction by ROS accumulation and inflammation suppression via NF-κB pathway. Int. Immunopharmacol. 86, 106751. doi:10.1016/j.intimp.2020.106751
Liu, Q., Sun, N. N., Wu, Z. Z., Fan, D. H., and Cao, M. Q. (2018). Chaihu-Shugan-San exerts an antidepressive effect by downregulating miR-124 and releasing inhibition of the MAPK14 and Gria3 signaling pathways. Neural Regen. Res. 13, 837–845. doi:10.4103/1673-5374.232478
Liu, Y., Wang, W., Chen, Y., Yan, H., Wu, D., Xu, J., et al. (2020b). Simultaneous quantification of nine components in the plasma of depressed rats after oral administration of Chaihu-Shugan-San by ultra-performance liquid chromatography/quadrupole-time-of-flight mass spectrometry and its application to pharmacokinetic studies. J. Pharm. Biomed. Anal. 186, 113310. doi:10.1016/j.jpba.2020.113310
Li, W., Liu, X., and Qiao, H. (2020b). Downregulation of hippocampal SIRT6 activates AKT/CRMP2 signaling and ameliorates chronic stress-induced depression-like behavior in mice. Acta Pharmacol. Sin. 41, 1557–1567. doi:10.1038/s41401-020-0387-5
Li, W., Zhou, R., Zheng, J., Sun, B., Jin, X., Hong, M., et al. (2022). Chaihu-Shugan-San ameliorates tumor growth in prostate cancer promoted by depression via modulating sphingolipid and glycerinphospholipid metabolism. Front. Pharmacol. 13, 1011450. doi:10.3389/fphar.2022.1011450
Li, W., Zhu, Y., Liu, X., Hou, J., Fang, J., Shen, J., et al. (2018). Phencynonate mediates antidepressant response by activating sirtuin 6-SOD2/Prdx6 pathway. Biochem. Biophys. Res. Commun. 505, 898–904. doi:10.1016/j.bbrc.2018.10.017
Li, X., Huang, W., Tan, R., Xu, C., Chen, X., Li, S., et al. (2023). The benefits of hesperidin in central nervous system disorders, based on the neuroprotective effect. Biomed. Pharmacother. 159, 114222. doi:10.1016/j.biopha.2023.114222
Luciano-Jaramillo, J., Sandoval-GarcíA, F., VáZQUEZ-Del Mercado, M., GutiéRREZ-Mercado, Y. K., Navarro-HernáNDEZ, R. E., MartíNEZ-GarcíA, E. A., et al. (2019). Downregulation of hippocampal NR2A/2B subunits related to cognitive impairment in a pristane-induced lupus BALB/c mice. PLoS One 14, e0217190. doi:10.1371/journal.pone.0217190
Ma, C., Yuan, D., Renaud, S. J., Zhou, T., Yang, F., Liou, Y., et al. (2022). Chaihu-shugan-san alleviates depression-like behavior in mice exposed to chronic unpredictable stress by altering the gut microbiota and levels of the bile acids hyocholic acid and 7-ketoDCA. Front. Pharmacol. 13, 1040591. doi:10.3389/fphar.2022.1040591
Ma, G., Zhang, J., Yang, X., Guo, P., Hou, X., Fan, Y., et al. (2020). TMEM16A-encoded anoctamin 1 inhibition contributes to chrysin-induced coronary relaxation. Biomed. Pharmacother. 131, 110766. doi:10.1016/j.biopha.2020.110766
Mathers, C. D., and Loncar, D. (2006). Projections of global mortality and burden of disease from 2002 to 2030. PLoS Med. 3, e442. doi:10.1371/journal.pmed.0030442
Matsuzaki, M., Honkura, N., Ellis-Davies, G. C., and Kasai, H. (2004). Structural basis of long-term potentiation in single dendritic spines. Nature 429, 761–766. doi:10.1038/nature02617
Meng, Y. U., Zhang, HONGMEI, Zhang, HONGWU, Wang, T. A. O., Hai, SHANG, Liyan, M. A., et al. (2020). To investigate the regulatory effects of Chaihu Shugan powder on fecal metabolites and intestinal flora in depression model rats. Int. J. Pharm. Res. 47, 229–235.
Mengyu, CONG, Xiaoxia, LIANG, Chen, FENGLIAN, and Cao, C. (2021). Mechanisms of antidepressant effects and common pharmacodynamic substances in the regulation of tryptophan metabolism by liver-sparing and spleen-regulating formulae. Chin. J. Traditional Chin. Med. 46, 3633–3642.
Menke, A. (2023). The HPA Axis as target for depression. Curr. Neuropharmacol. 22, 904–915. doi:10.2174/1570159X21666230811141557
Meseguer, V., Alpizar, Y. A., Luis, E., Tajada, S., Denlinger, B., Fajardo, O., et al. (2014). TRPA1 channels mediate acute neurogenic inflammation and pain produced by bacterial endotoxins. Nat. Commun. 5, 3125. doi:10.1038/ncomms4125
Młynarska, E., Gadzinowska, J., Tokarek, J., Forycka, J., Szuman, A., Franczyk, B., et al. (2022). The role of the microbiome-brain-gut Axis in the pathogenesis of depressive disorder. Nutrients 14, 1921. doi:10.3390/nu14091921
Montgomery, K. R., Bridi, M. S., Folts, L. M., Marx-Rattner, R., Zierden, H. C., Wulff, A. B., et al. (2023). Chemogenetic activation of CRF neurons as a model of chronic stress produces sex-specific physiological and behavioral effects. Neuropsychopharmacology 49, 443–454. doi:10.1038/s41386-023-01739-5
Moreira, J., Machado, M., Dias-Teixeira, M., Ferraz, R., Delerue-Matos, C., and Grosso, C. (2023). The neuroprotective effect of traditional Chinese medicinal plants-A critical review. Acta Pharm. Sin. B 13, 3208–3237. doi:10.1016/j.apsb.2023.06.009
Mu, D. Z., Xue, M., Xu, J. J., Hu, Y., Chen, Y., Ren, P., et al. (2020). Antidepression and prokinetic effects of paeoniflorin on rats in the forced swimming test via polypharmacology. Evid. Based Complement. Altern. Med. 2020, 2153571. doi:10.1155/2020/2153571
Murakami, Y., Ishibashi, T., Tomita, E., Imamura, Y., Tashiro, T., Watcharanurak, K., et al. (2016). Depressive symptoms as a side effect of Interferon-α therapy induced by induction of indoleamine 2,3-dioxygenase 1. Sci. Rep. 6, 29920. doi:10.1038/srep29920
Murrough, J. W., and Charney, D. S. (2012). Is there anything really novel on the antidepressant horizon? Curr. psychiatry Rep. 14, 643–649. doi:10.1007/s11920-012-0321-8
Nikolova, V. L., Smith, M. R. B., Hall, L. J., Cleare, A. J., Stone, J. M., and Young, A. H. (2021). Perturbations in gut microbiota composition in psychiatric disorders: a review and meta-analysis. JAMA Psychiatry 78, 1343–1354. doi:10.1001/jamapsychiatry.2021.2573
Oladapo, O. M., Ben-Azu, B., Ajayi, A. M., Emokpae, O., Eneni, A. O., Omogbiya, I. A., et al. (2021). Naringin confers protection against psychosocial defeat stress-induced neurobehavioral deficits in mice: involvement of glutamic acid decarboxylase isoform-67, oxido-nitrergic stress, and neuroinflammatory mechanisms. J. Mol. Neurosci. 71, 431–445. doi:10.1007/s12031-020-01664-y
Perez-Caballero, L., Torres-Sanchez, S., Romero-LóPEZ-Alberca, C., GonzáLEZ-Saiz, F., Mico, J. A., and Berrocoso, E. (2019). Monoaminergic system and depression. Cell Tissue Res. 377, 107–113. doi:10.1007/s00441-018-2978-8
Pozzo, E. D., Tremolanti, C., Costa, B., Giacomelli, C., Milenkovic, V. M., Bader, S., et al. (2019). Microglial pro-inflammatory and anti-inflammatory phenotypes are modulated by translocator protein activation. Int. J. Mol. Sci. 20, 4467. doi:10.3390/ijms20184467
Qian, M., Peng, R., Yue, C., Yang, Z., Zhu, H., Liu, B., et al. (2020). A comparison study of chaihu shugan san and fluoxetine on antidepression and regulating blood rheology effects with chronic restrained stress rats. Evidence-Based Complementary Altern. Med. 2020, 1–18. doi:10.1155/2020/6426383
Qiu, J. (2014). To investigate the effects of Chaihu Shukan powder and its decoction on the behavior and the expression of p38MAPK and ERK5 in the brain tissue of depression model rats. Central South University.
Qi, X., Wang, S., Huang, Q., Chen, X., Qiu, L., Ouyang, K., et al. (2023). The association between non-high-density lipoprotein cholesterol to high-density lipoprotein cholesterol ratio (NHHR) and risk of depression among US adults: a cross-sectional NHANES study. J. Affect Disord. 344, 451–457. doi:10.1016/j.jad.2023.10.064
Reyes-Lizaola, S., Luna-Zarate, U., Tendilla-BeltráN, H., Morales-Medina, J. C., and Flores, G. (2023). Structural and biochemical alterations in dendritic spines as key mechanisms for severe mental illnesses. Prog. Neuropsychopharmacol. Biol. Psychiatry 129, 110876. doi:10.1016/j.pnpbp.2023.110876
Sasaki, K., Iwata, N., Ferdousi, F., and Isoda, H. (2019). Antidepressant-like effect of ferulic acid via promotion of energy metabolism activity. Mol. Nutr. Food Res. 63, e1900327. doi:10.1002/mnfr.201900327
Sehgal, M., Song, C., Ehlers, V. L., and Moyer, J. R. (2013). Learning to learn - intrinsic plasticity as a metaplasticity mechanism for memory formation. Neurobiol. Learn Mem. 105, 186–199. doi:10.1016/j.nlm.2013.07.008
Shengqiang, L., Wenna, L., Yachan, L., Yunlong, C., Shujiao, C., and Candong, L. (2015). Effect of Chaihushugan San on expression of the Raf/mitogen-activated protein kinase/extracellular signal-regulated kinase pathway in the hippocampi of perimenopausal rats induced by immobilization stress. J. Tradit. Chin. Med. 35, 445–452. doi:10.1016/s0254-6272(15)30123-0
Singh, S., and Singh, T. G. (2020). Role of nuclear factor kappa B (NF-κB) signalling in neurodegenerative diseases: an mechanistic approach. Curr. Neuropharmacol. 18, 918–935. doi:10.2174/1570159X18666200207120949
Smith, J. A., Das, A., Ray, S. K., and Banik, N. L. (2012). Role of pro-inflammatory cytokines released from microglia in neurodegenerative diseases. Brain Res. Bull. 87, 10–20. doi:10.1016/j.brainresbull.2011.10.004
Soares, C. L. R., Wilairatana, P., Silva, L. R., Moreira, P. S., Vilar Barbosa, N. M. M., Da Silva, P. R., et al. (2023). Biochemical aspects of the inflammatory process: a narrative review. Biomed. Pharmacother. 168, 115764. doi:10.1016/j.biopha.2023.115764
Su, Z. H., Jia, H. M., Zhang, H. W., Feng, Y. F., An, L., and Zou, Z. M. (2014). Hippocampus and serum metabolomic studies to explore the regulation of Chaihu-Shu-Gan-San on metabolic network disturbances of rats exposed to chronic variable stress. Mol. Biosyst. 10, 549–561. doi:10.1039/c3mb70377k
Su, J., Pan, Y. W., Wang, S. Q., Li, X. Z., Huang, F., and Ma, S. P. (2020). Saikosaponin-d attenuated lipopolysaccharide-induced depressive-like behaviors via inhibiting microglia activation and neuroinflammation. Int. Immunopharmacol. 80, 106181. doi:10.1016/j.intimp.2019.106181
Sun, K., Wu, A., Li, X., and Cui, P. (2020). Efficacy and safety of Chaihu Shugan powder combined with antidepressants in the treatment of depression: a systematic review and Meta-analysis. Shenzhen J. Integr. Traditional Chin. West. Med. 30, 4–8.
Suvrathan, A., Payne, H. L., and Raymond, J. L. (2016). Timing rules for synaptic plasticity matched to behavioral function. Neuron 92, 959–967. doi:10.1016/j.neuron.2016.10.022
Sweatt, J. D. (2016). Neural plasticity and behavior - sixty years of conceptual advances. J. Neurochem. 139 (2), 179–199. doi:10.1111/jnc.13580
Tao, X. U., Sheng, Y. E., and Li, Y. (2023). Clinical efficacy of Chaihu Shuohe San plus reduction combined with haloperidol melittin tablets in the treatment of post-stroke depression. Clin. Ration. use Medicat. 16, 15–18.
Tashiro, A., Sandler, V. M., Toni, N., Zhao, C., and Gage, F. H. (2006). NMDA-receptor-mediated, cell-specific integration of new neurons in adult dentate gyrus. Nature 442, 929–933. doi:10.1038/nature05028
Tong, Y., Zhao, G., Shuang, R., Wang, H., and Zeng, N. (2023). Saikosaponin a activates tet1/dll3/notch1 signalling and promotes hippocampal neurogenesis to improve depression-like behavior in mice. J. Ethnopharmacol. 319, 117289. doi:10.1016/j.jep.2023.117289
Trivedi, M. H., Hollander, E., Nutt, D., and Blier, P. (2008). Clinical evidence and potential neurobiological underpinnings of unresolved symptoms of depression. J. Clin. Psychiatry 69, 246–258. doi:10.4088/jcp.v69n0211
Trivedi, M. H., Rush, A. J., Wisniewski, S. R., Nierenberg, A. A., Warden, D., Ritz, L., et al. (2006). Evaluation of outcomes with citalopram for depression using measurement-based care in STAR* D: implications for clinical practice. Am. J. Psychiatry 163, 28–40. doi:10.1176/appi.ajp.163.1.28
Tsuchimine, S., Saito, M., Kaneko, S., and Yasui-Furukori, N. (2015). Decreased serum levels of polyunsaturated fatty acids and folate, but not brain-derived neurotrophic factor, in childhood and adolescent females with depression. Psychiatry Res. 225, 187–190. doi:10.1016/j.psychres.2014.11.018
Undurraga, J., and Baldessarini, R. J. (2012). Randomized, placebo-controlled trials of antidepressants for acute major depression: thirty-year meta-analytic review. Neuropsychopharmacology 37, 851–864. doi:10.1038/npp.2011.306
Wang, A. R., Mi, L. F., Zhang, Z. L., Hu, M. Z., Zhao, Z. Y., Liu, B., et al. (2021a). Saikosaponin A improved depression-like behavior and inhibited hippocampal neuronal apoptosis after cerebral ischemia through p-CREB/BDNF pathway. Behav. Brain Res. 403, 113138. doi:10.1016/j.bbr.2021.113138
Wang, KAIXIN, Wang, SANWANG, Qingling, ZHAI, Zhang, D. I., Jing, L. I. U., Fantao, MENG, et al. (2022). Effect of nocardione on depressive-like behavior and PKA/CREB/BDNF signaling in the hippocampus. J. Anhui Med. Univ. 57, 725–730.
Wang, YONGZHI, Yi, D. U., Yu, HANG, Li, L., and Li, Y. (2014). Antidepressant-like activity of Chaihu-Shugan-San aqueous extract in rats and its possible mechanism. Beijing Tradit. Chin. Med. 33, 50–53. doi:10.4103/0973-1296.127342
Wang, H., Yang, Y., Pei, G., Wang, Z., and Chen, N. (2023a). Neurotrophic basis to the pathogenesis of depression and phytotherapy. Front. Pharmacol. 14, 1182666. doi:10.3389/fphar.2023.1182666
Wang, K., Li, K., Chen, Y., Wei, G., Yu, H., Li, Y., et al. (2021b). Computational network pharmacology-based strategy to capture key functional components and decode the mechanism of chai-hu-shu-Gan-san in treating depression. Front. Pharmacol. 12, 782060. doi:10.3389/fphar.2021.782060
Wang, T., Liu, J., Luo, X., Hu, L., and Lu, H. (2021c). Functional metabolomics innovates therapeutic discovery of traditional Chinese medicine derived functional compounds. Pharmacol. Ther. 224, 107824. doi:10.1016/j.pharmthera.2021.107824
Wang, X., Li, S., Yu, J., Wang, W., Du, Z., Gao, S., et al. (2023b). Saikosaponin B2 ameliorates depression-induced microglia activation by inhibiting ferroptosis-mediated neuroinflammation and ER stress. J. Ethnopharmacol. 316, 116729. doi:10.1016/j.jep.2023.116729
Wang, Y., Fan, R., and Huang, X. (2012). Meta-analysis of the clinical effectiveness of traditional Chinese medicine formula Chaihu-Shugan-San in depression. J. Ethnopharmacol. 141, 571–577. doi:10.1016/j.jep.2011.08.079
Wang, Y., Wang, M., Xu, M., Li, T., Fan, K., Yan, T., et al. (2018). Nootkatone, a neuroprotective agent from Alpiniae Oxyphyllae Fructus, improves cognitive impairment in lipopolysaccharide-induced mouse model of Alzheimer's disease. Int. Immunopharmacol. 62, 77–85. doi:10.1016/j.intimp.2018.06.042
Wei, Y., Gao, H., Luo, Y., Feng, J., Li, G., Wang, T., et al. (2023). Systemic inflammation and oxidative stress markers in patients with unipolar and bipolar depression: a large-scale study. J. Affect Disord. 346, 154–166. doi:10.1016/j.jad.2023.10.156
Wilson, G. C., Keitsch, S., Soddemann, M., Wilker, B., Edwards, M. J., and Gulbins, E. (2023). Role of tyrosine nitrosylation in stress-induced major depressive disorder: mechanisms and implications. Int. J. Mol. Sci. 24, 14626. doi:10.3390/ijms241914626
Xiang, Y., Xin, J., Le, W., and Yang, Y. (2020). Neurogranin: a potential biomarker of neurological and mental diseases. Front. Aging Neurosci. 12, 584743. doi:10.3389/fnagi.2020.584743
Xie, K., Jin, B., Zhu, H., Zhou, P., Du, L., and Jin, X. (2020a). Ferulic acid (FA) protects human retinal pigment epithelial cells from H(2) O(2) -induced oxidative injuries. J. Cell Mol. Med. 24, 13454–13462. doi:10.1111/jcmm.15970
Xie, L., Gu, Z., Liu, H., Jia, B., Wang, Y., Cao, M., et al. (2020b). The anti-depressive effects of hesperidin and the relative mechanisms based on the NLRP3 inflammatory signaling pathway. Front. Pharmacol. 11, 1251. doi:10.3389/fphar.2020.01251
Xiong, Y. (2021). Clinical observation of Chaihu Shugan powder combined with escitalopram oxalate in the treatment of senile depression. Beijing: Beijing University of Chinese Medicine.
Xiong, Y., Guo, R., Song, D., Sun, Y., Wang, P., and Ying, L. (2019). To investigate the effect of Chaihu Shugan powder on mood and quality of life in elderly patients with depression. World J. Integr. Chin. West. Med. 14, 1291–1294.
Xu, Q., Yao, Y., Liu, Y., Zhang, J., and Mao, L. (2023). The mechanism of traditional medicine in alleviating ulcerative colitis: regulating intestinal barrier function. Front. Pharmacol. 14, 1228969. doi:10.3389/fphar.2023.1228969
Xu, Y. H., Wang, X. X., Wang, M. J., Liu, Y. Y., Xue, Z., and Chen, J. X. (2021). Influence of progestational stress on BDNF and NMDARs in the hippocampus of male offspring and amelioration by Chaihu Shugan San. Biomed. Pharmacother. 135, 111204. doi:10.1016/j.biopha.2020.111204
Xuesong, G. A. O., Li, L. I., Wang, ANNA, Wang, YONGZHI, Yi, D. U., and Zhao, J. (2023). Effects of Chaihu Shugan powder on behavior and tryptophan metabolism in raphe nucleus in depression rats. Laboratory Animal Sci. 40, 16–21.
Xu, Q., Ji, X. F., Chi, T. Y., Liu, P., Jin, G., Gu, S. L., et al. (2015). Sigma 1 receptor activation regulates brain-derived neurotrophic factor through NR2A-CaMKIV-TORC1 pathway to rescue the impairment of learning and memory induced by brain ischaemia/reperfusion. Psychopharmacol. Berl. 232, 1779–1791. doi:10.1007/s00213-014-3809-6
Yan, L., Xu, X., He, Z., Wang, S., Zhao, L., Qiu, J., et al. (2020). Antidepressant-like effects and cognitive enhancement of coadministration of chaihu shugan san and fluoxetine: dependent on the BDNF-ERK-CREB signaling pathway in the Hippocampus and frontal cortex. Biomed. Res. Int. 2020, 2794263. doi:10.1155/2020/2794263
Yang, J., Jin, W., Liu, D., Zhong, Q., and Zhou, T. (2020). Enhanced pseudotargeted analysis using a segment data dependent acquisition strategy by liquid chromatography-tandem mass spectrometry for a metabolomics study of liquiritin in the treatment of depression. J. Sep. Sci. 43, 2088–2096. doi:10.1002/jssc.202000107
Yang, Y., Gu, K., Meng, C., Li, J., Lu, Q., Zhou, X., et al. (2023). Relationship between sleep and serum inflammatory factors in patients with major depressive disorder. Psychiatry Res. 329, 115528. doi:10.1016/j.psychres.2023.115528
Yan, T., Li, F., Xiong, W., Wu, B., Xiao, F., He, B., et al. (2021). Nootkatone improves anxiety- and depression-like behavior by targeting hyperammonemia-induced oxidative stress in D-galactosamine model of liver injury. Environ. Toxicol. 36, 694–706. doi:10.1002/tox.23073
Ying, DENG, Zhang, CHUNHU, Zhang, HAINAN, Suiyu, H. U., Huang, X. I., Yang, WANG, et al. (2011). To investigate the effects of Chaihu Shugan powder and its decoction on behavior and BDNF and its receptor TrkB in hippocampus, amygdala and frontal lobe of depression model rats. Chin. J. Integr. Traditional Chin. West. Med. 31, 1373–1378.
Yufeng, M. A., Wang, JIALIN, Jia, XING, Xiaochen, Z. H. U., Guangxin, Y. U. E., Wang, YULAI, et al. (2016). Effects of Chaihu Shuohe San on monoamine neurotransmitters in the hippocampus and hypothalamus of rats with liver qi stagnation syndrome. J. Integr. Cardiovasc. Cerebrovasc. Dis. 14, 2494–2497.
Yunhui, L. I., Zhang, CHUNHU, Wang, S. U. E., Juan, Q. I. U., Suiyu, H. U., and Xiao, G. (2009). Effects of Chai Hu Shu Hepatosan on behaviour and plasma adrenocorticotropic hormone-releasing hormone and adrenocorticotropic hormone in rats with chronic stress depression model. J. Integr. Chin. West. Med. 7, 1073–1077.
Yu, Y., Zhang, G., Han, T., Liu, H., and Huang, H. (2022). A network meta-analysis of the clinical efficacy and safety of commonly used Chinese patent medicines in the auxiliary treatment of poststroke depression. Evid. Based Complement. Altern. Med. 2022, 7265769. doi:10.1155/2022/7265769
ZáDORI, D., Veres, G., SzaláRDY, L., KlivéNYI, P., and VéCSEI, L. (2018). Alzheimer's disease: recent concepts on the relation of mitochondrial disturbances, excitotoxicity, neuroinflammation, and kynurenines. J. Alzheimers Dis. 62, 523–547. doi:10.3233/JAD-170929
Zeng, L. F., Cao, Y., Wang, L., Dai, Y. K., Hu, L., Wang, Q., et al. (2017). Role of medicinal plants for liver-qi regulation adjuvant therapy in post-stroke depression: a systematic review of literature. Phytother. Res. 31, 40–52. doi:10.1002/ptr.5740
Zhang, FUMIN, and Liu, J. (2020). To study the antidepressant effect of Chaihu Shugan powder by regulating the cyclic adenosine monophosphate/cyclic adenosine monophosphate response element-binding protein/brain-derived neurotrophic factor signaling pathway in the frontal lobe of rats. Anhui Med. 24, 646–650.
Zhang, XIAOJIE, Haiying, DONG, Sun, YURONG, Chunrong, L. I. N., and Li, X. (2011a). Effects of Chaihu Shugan powder on hippocampal CA3 neurons in depression model rats. Shi Zhen Natl. Med. Natl. Med. 22, 43–45.
Zhang, Y. J., Huang, X., Wang, Y., Xie, Y., Qiu, X. J., Ren, P., et al. (2011b). Ferulic acid-induced anti-depression and prokinetics similar to Chaihu-Shugan-San via polypharmacology. Brain Res. Bull. 86, 222–228. doi:10.1016/j.brainresbull.2011.07.002
Zhang, ZHEN, Zhao, B. O., Zhijian, L. I., Guo, YONGSHENG, Ran, DING, and Zhang, J. (2021b). Protective mechanism of Chaihu Shugan powder on orbitofrontal cortex in CUMS rats. Tradit. Chin. Med. Mater. 44, 1713–1718.
Zhang, H., Kang, E., Wang, Y., Yang, C., Yu, H., Wang, Q., et al. (2018a). Publisher Correction: brain-specific Crmp2 deletion leads to neuronal development deficits and behavioural impairments in mice. Nat. Commun. 9, 16229. doi:10.1038/ncomms16229
Zhang, L., Lu, R. R., Xu, R. H., Wang, H. H., Feng, W. S., and Zheng, X. K. (2023). Naringenin and apigenin ameliorates corticosterone-induced depressive behaviors. Heliyon 9, e15618. doi:10.1016/j.heliyon.2023.e15618
Zhang, S., Lu, Y., Chen, W., Shi, W., Zhao, Q., Zhao, J., et al. (2021a). Network pharmacology and experimental evidence: PI3K/AKT signaling pathway is involved in the antidepressive roles of chaihu shugan san. Drug Des. Devel Ther. 15, 3425–3441. doi:10.2147/DDDT.S315060
Zhang, X., Han, B., Feng, Z. M., Yang, Y. N., Jiang, J. S., and Zhang, P. C. (2018b). Ferulic acid derivatives from Ligusticum chuanxiong. Fitoterapia 125, 147–154. doi:10.1016/j.fitote.2018.01.005
Zhang, Y. (2016). Treatment of 43 cases of liver-qi stagnation-type depression by Chaihu Shuohe San. Mod. Distance Educ. Chin. Traditional Med. 14, 85–86.
Zhao, X. H., An, N., Xia, M. H., Liu, W. P., Wang, Q. Q., and Bao, J. Z. (2023a). Nootkatone improves chronic unpredictable mild stress-induced depressive-like behaviors by repressing NF-κB/NLRP3-Mediated neuroinflammation. Chin. J. Integr. Med. 29, 37–43. doi:10.1007/s11655-022-3725-2
Zhao, Y., Xu, D., Wang, J., Zhou, D., Liu, A., Sun, Y., et al. (2023b). The pharmacological mechanism of chaihu-jia-longgu-muli-tang for treating depression: integrated meta-analysis and network pharmacology analysis. Front. Pharmacol. 14, 1257617. doi:10.3389/fphar.2023.1257617
Zheng, X., Cheng, Y., Chen, Y., Yue, Y., Li, Y., Xia, S., et al. (2019). Ferulic acid improves depressive-like behavior in prenatally-stressed offspring rats via anti-inflammatory activity and HPA Axis. Int. J. Mol. Sci. 20 (3), 493. doi:10.3390/ijms20030493
Zheng, P., Zeng, B., Zhou, C., Liu, M., Fang, Z., Xu, X., et al. (2016). Gut microbiome remodeling induces depressive-like behaviors through a pathway mediated by the host's metabolism. Mol. Psychiatry 21, 786–796. doi:10.1038/mp.2016.44
Zhong, X., Li, G., Qiu, F., and Huang, Z. (2018). Paeoniflorin ameliorates chronic stress-induced depression-like behaviors and neuronal damages in rats via activation of the ERK-CREB pathway. Front. Psychiatry 9, 772. doi:10.3389/fpsyt.2018.00772
Zhu, Y. L., Wang, H. J., Xue, H., Zhang, Y., Cheng, Q. S., Chen, L. Y., et al. (2020b). Simultaneous determination of five components of chaihu-shugan-san in beagle plasma by HPLC-MS/MS and its application to a pharmacokinetic study after a single dose of chaihu-shugan-san. J. Anal. Methods Chem. 2020, 8831938. doi:10.1155/2020/8831938
Zhu, H., Guan, A., Liu, J., Peng, L., Zhang, Z., and Wang, S. (2023). Noteworthy perspectives on microglia in neuropsychiatric disorders. J. Neuroinflammation 20, 223. doi:10.1186/s12974-023-02901-y
Zhu, X., Li, T., Hu, E., Duan, L., Zhang, C., Wang, Y., et al. (2021). Proteomics study reveals the anti-depressive mechanisms and the compatibility advantage of chaihu-shugan-san in a rat model of chronic unpredictable mild stress. Front. Pharmacol. 12, 791097. doi:10.3389/fphar.2021.791097
Keywords: Chaihu-Shugan-San, depression, active ingredients, antidepressant, systematic review
Citation: Guo Z, Long T, Yao J, Li Y, Xiao L and Chen M (2024) Potential antidepressant effects of Traditional Chinese botanical drug formula Chaihu-Shugan-San and its active ingredients. Front. Pharmacol. 15:1337876. doi: 10.3389/fphar.2024.1337876
Received: 13 November 2023; Accepted: 13 March 2024;
Published: 02 April 2024.
Edited by:
Michael Heinrich, University College London, United KingdomReviewed by:
Ying Guo, Central South University, ChinaCen Li, Chinese Academy of Sciences (CAS), China
Copyright © 2024 Guo, Long, Yao, Li, Xiao and Chen. This is an open-access article distributed under the terms of the Creative Commons Attribution License (CC BY). The use, distribution or reproduction in other forums is permitted, provided the original author(s) and the copyright owner(s) are credited and that the original publication in this journal is cited, in accordance with accepted academic practice. No use, distribution or reproduction is permitted which does not comply with these terms.
*Correspondence: Lu Xiao, hbxl1527@163.com; Min Chen, mchen@must.edu.mo
†These authors share first authorship