- 1School of Medicine, Shanghai University, Shanghai, China
- 2Shanghai Eighth People’s Hospital, Shanghai, China
- 3Department of Pharmaceutical Sciences, College of Pharmacy and Health Sciences, St. John’s University, New York, NY, United States
Ischemia-reperfusion (I/R) injury refers to a new injury caused by reperfusion after the restoration of ischemic tissue or organ blood supply. Salvianic acid A (danshensu) is a primary active ingredient extracted from Salvia miltiorrhiza. It has a protective function against I/R injury in the cardiovascular system, brain, liver, kidney, gastrointestinal tract, and other organs. This article reviews evidence of the protective effects of Salvianic acid A and its potential mechanisms of action in organ I/R injury protection. The aim of this review is to investigate the role of Salvianic acid A in the treatment of I/R injury, providing a reference resource that could facilitate subsequent studies.
1 Introduction
Organ or tissue damage induced by ischemia is the leading cause of fatal diseases, which include myocardial infarction (Heusch and Gersh, 2017), ischemic stroke (Feske, 2021), acute kidney injury (Saat et al., 2016), and organ failure (Jernryd et al., 2020). The timely restoration of blood flow to ischemic tissues using reperfusion therapy is pivotal to the recovery, regeneration, and repair of tissues (Rentrop and Feit, 2015). However, reperfusion can lead to the paradoxical exacerbation of cellular dysfunction, in turn worsening tissue damage; this process is referred to as ischemia-reperfusion (I/R) injury (Wu et al., 2018).
I/R injury is a challenging and life-threatening clinical problem that contributes to the pathology of a wide range of conditions (Anzell et al., 2018). Previous studies have found that the underlying molecular mechanism of I/R injury involves various complex pathological processes, including complement system activation (Gorsuch et al., 2012), platelet–leukocyte aggregation (Al-Mufti et al., 2018), increased free radical production (Baker et al., 1988), calcium overload (Shintani-Ishida et al., 2012), mitochondrial membrane potential destruction (Morciano et al., 2017), reactive oxygen species (ROS) formation (Dambrova et al., 2021), endothelial dysfunction (Bugger and Pfeil, 2020), and endoplasmic reticulum stress (Bai and Lyden, 2015). Severe I/R injury can also cause cell death through the induction of necrosis, apoptosis, autophagy, and other pathways (Kalogeris et al., 2012). Although considerable progress has been made in recent years towards understanding the pathophysiology of I/R injury, safe and effective treatment methods are limited in clinical practice (Bao et al., 2018a). Therefore, it is imperative to explore new drugs to control and treat I/R injury.
Traditional Chinese Medicine (TCM) is thousands of years old and has been used widely to prevent and treat various diseases, particularly cardiovascular diseases (Hao et al., 2017), stroke (Liu et al., 2018), diabetes (Tian et al., 2019), and other chronic diseases (Li et al., 2017). In 2015, Tu Youyou won the Nobel Prize in Physiology or Medicine and delivered a lecture titled “Discovery of Artemisinin: A Gift from Traditional Chinese Medicine to the World,” focusing global attention on TCM (Tu, 2016). Salvia miltiorrhiza, the dried root and rhizome of S. miltiorrhiza Bunge, is one of the most common and versatile herbs in TCM (Xu et al., 2018). It has been utilized extensively in Asian countries to treat cardiovascular diseases (Bi et al., 2021), hyperlipidemia (Sun G. et al., 2021), atherosclerosis (Cho et al., 2013), and cerebrovascular diseases (Lin and Hsieh, 2010). The main active pharmaceutical ingredients of S. miltiorrhiza can be categorized as lipid-soluble tanshinones and water-soluble phenolic acids (Fei et al., 2017). Recently, these phenolic acids have been increasingly attracting attention because they are the chief constituents of aqueous decoctions, which are the most used type of S. miltiorrhiza in clinics (MEIm et al., 2019). Specifically, total salvianolic acid, a group of water-soluble phenolic acids isolated from S. miltiorrhiza, has been granted approval by the State Food and Drug Administration for the treatment of cardiovascular and cerebrovascular diseases in China (Han et al., 2017).
Among these water-soluble phenolic acids, Salvianic acid A [also named danshensu (R)-3- (3, 4-dihydroxyphenyl)-2-hydroxypropanoic acid (Figure 1)] is one of the basic constituent structures of various salvianolic acids, and its pharmacological properties and molecular mechanisms have been studied extensively (Bianconi et al., 2018). Extensive experimental evidence has demonstrated that Salvianic acid A has a broad scope of pharmacological effects that protect against myocardial I/R and cerebral ischemia injury (Choi and Pile-Spellman, 2018), improve microcirculation (Jia et al., 2022), protect endothelial cells (Yang et al., 2010), and exert anti-inflammatory (Xu et al., 2021), anti-oxidant (Guo et al., 2013), antiplatelet, antithrombotic, and antithrombotic properties (Dery et al., 2022). As a result, Salvianic acid A has already been used to alleviate angina pectoris and treat coronary heart disease and is currently undergoing phase II clinical trials in China (drug clinical trial registration number: CTR20201177).
Several studies have demonstrated that Salvianic acid A has a strong protective effect on multiple organs, such as the heart, brain, lungs, kidneys, and liver (Bao X. Y. et al., 2018). Despite several published reviews on the cardiovascular pharmacology of Salvianic acid A, to the best of our knowledge, the mechanism of the protective effect of Salvianic acid A against multiorgan I/R injury has not been summarized. In the present review, we summarize research progress on Salvianic acid A in the treatment of multiple-organ I/R injury, aiming to enhance our understanding of the pharmacological mechanisms and provide novel insights for further in-depth research. The specific mechanisms of Salvianic acid A in the prevention of I/R injury of diverse organs are shown in Supplementary Table S1; Figure 2.
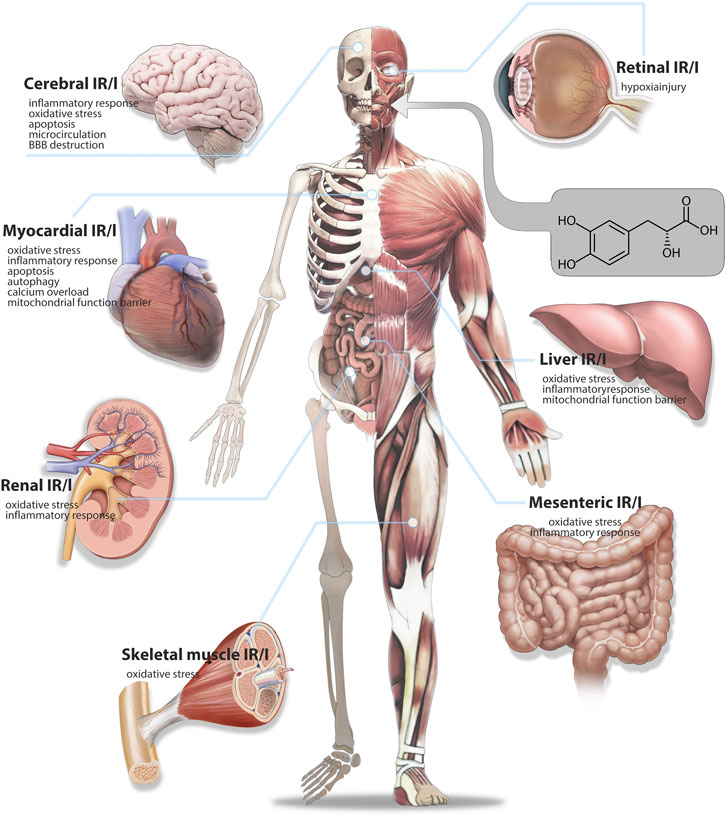
FIGURE 2. Therapeutic mechanism of Salvianic acid A against ischemia-reperfusion injury in various organs.
2 Protective effect of Salvianic acid A against cardiac I/R injury
Myocardial infarction (MI) remains the leading cause of death and morbidity and has become a serious global public health problem (Schirone et al., 2022). Timely and effective restoration of coronary blood flow after MI is a standard therapeutic strategy (Tibaut et al., 2017). Paradoxically, reperfusion itself would result in incremental myocardial damage, which is termed I/R injury (Yellon and Hausenloy, 2007). Research indicates that the mechanism of myocardial I/R injury (MIRI) is complex, with multiple physiological and pathological processes, such as oxidative stress (Guo et al., 2010), inflammatory response (Yao et al., 2022), intracellular Ca2+ overload (Shintani-Ishida et al., 2012), mitochondrial dysfunction (Zhou et al., 2021), apoptosis, and autophagy (Xing et al., 2022). Such factors often work synergistically, leading to the onset and progression of MIRI (Hausenloy and Yellon, 2013).
According to reports, the mechanism by which Salvianic acid A prevents and treats MIRI is not only related to coronary artery regulation and anti-platelet effects to improve blood circulation but also involves antioxidant and anti-inflammatory effects, autophagy regulation, anti-apoptosis mechanisms, calcium overload regulation, and endothelial cell protection, wherein many signaling pathways and related proteins play important roles. For example, regulation of the PI3K/AKT signaling pathway results in antioxidant and anti-apoptotic effects; inhibition of the JNK/NF-KB signaling pathway reduces the expression of TRPC6 and plays an anti-calcium overload role (Gao et al., 2017; Gao et al., 2022; Ge et al., 2021) (Figure 3). Furthermore, administration of Salvianic acid A alone or in combination with other Chinese medicinal products has potential preventive and therapeutic effects on MIRI. In the following sections, we briefly elaborate on the mechanism of action of Salvianic acid A in the treatment of MIRI through different pathways.
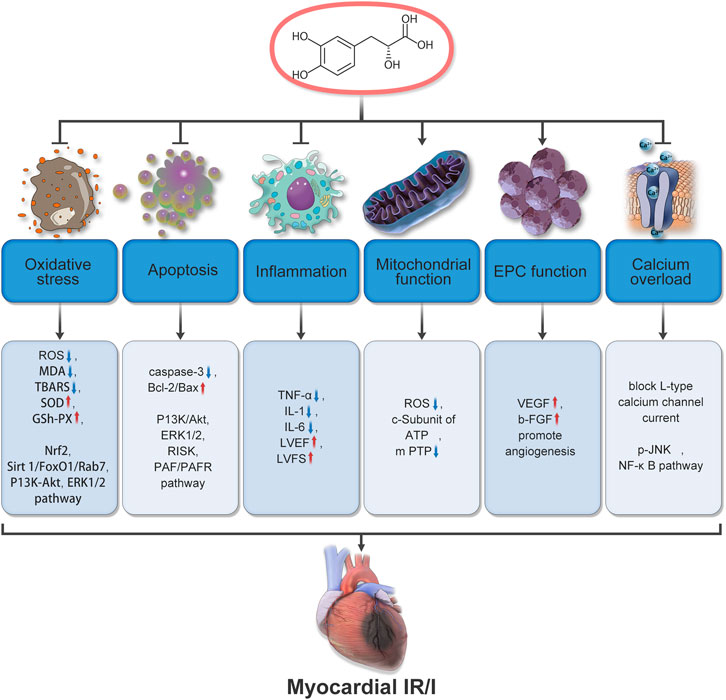
FIGURE 3. Mechanisms underlying the therapeutic effects of Salvianic acid A in cardiac ischemia-reperfusion injury.
2.1 Anti-oxidative effects
Oxidative stress is defined as the imbalance between oxidative and antioxidative processes in vivo (Sies, 2015). The overproduction of ROS and the release of a range of transcription factors that regulate oxidative stress are essential in triggering oxidative responses in ischemic cardiomyocytes (Kurian et al., 2016).
Numerous studies have shown that Salvianic acid A can clear excess ROS (Huang et al., 2019; Ibáñez et al., 2015; Jia et al., 2019a), reduce malondialdehyde (MDA) levels and thiobarbituric acid reactive substances (Kalogeris et al., 2016; Li et al., 2012; Li et al., 2016), and enhance the endogenous antioxidant defense molecules superoxide dismutase (SOD) and glutathione peroxidase (GSH-PX) in a rat MIRI model (Sun D. W. et al., 2019). Therefore, the oxidation of myocardial fatty acids can be inhibited to improve myocardial energy metabolism under pathological conditions and has cardiac protective effects on isolated hearts (Zhang et al., 2010), preventing oxidative stress during I/R injury. The signaling pathways involved include Nrf2 (Li et al., 2018; Pachel et al., 2016; Patel and McMullen, 2017), Sirt1/FoxO1/Rab7 (Sun D. W. et al., 2019), PI3K-Akt, and ERK1/2 (Xu H. et al., 2020). Furthermore, Salvianic acid A reportedly showed antiarrhythmic and cardiac protective effects against hypertrophic heart I/R injury in an I/R model of myocardial hypertrophy by increasing SOD activity and decreasing MDA content (Tang et al., 2011).
Salvianic acid A in combination with other traditional Chinese medicines has also been demonstrated to have a protective effect against myocardial ischemic injury. Studies have shown that paeonol combined with Salvianic acid A can enhance the cardiac antioxidant defense system by regulating the Nrf2/HO-1 and PI3K/Akt pathways and play a cardiac protective role in isoproterenol-induced MI in rats (Qian et al., 2002). The protective effect of salvianolic acid A against MI is mainly reflected in its ability to reduce isoproterenol-induced MI by regulating two signaling pathways against oxidative stress and apoptosis after myocardial cell injury. In another study (Wu et al., 2007), puerarin combined with Salvianic acid A reduced infarct size caused by ischemic injury in a dose-dependent manner through its antioxidant and anti-lipid peroxidation properties and had a significant protective effect against acute ischemic myocardial injury in rats.
2.2 Anti-apoptosis
Apoptosis is the process whereby cells “commit suicide” by activating an intracellular death program (Xu et al., 2019). Previous studies have shown that I/R can lead to cardiomyocyte death through a variety of pathways, including apoptosis (Wang et al., 2016). Salvianic acid A acts against apoptosis in injured cardiomyocytes through the activation of the PI3K/Akt, ERK1/2 (Yin et al., 2013), RISK (Guan et al., 2013), and PAF/PAFR pathways and by weakening caspase-3 activity and increasing the Bcl-2:Bax ratio (Wang et al., 2021), thus reducing the release of downstream apoptotic factors, preventing the loss of cardiomyocytes, and minimizing the cardiac damage caused by MIRI.
Of note, the role of Salvianic acid A in alleviating MIRI appears to be linked to its anti-apoptotic pharmacological activity. Hu et al. (2016) first demonstrated that the combination of Salvianic acid A and hydroxyl safflower yellow A (HSYA) can exert antioxidant and anti-apoptotic effects by activating the Akt/Nrf2/HO-1 signaling pathway to prevent myocardial cell damage. Salvianic acid A performs an important role in anti-apoptosis, and HSYA has an essential anti-oxidation role. Guan et al. (2013) compared the activities of several major active components in danhong injection in terms of antioxidant, anti-inflammatory, and anti-apoptosis properties and found that Salvianic acid A had strong anti-apoptotic effects. The results of such studies enhance our understanding of the mechanisms of function of Salvianic acid A in preventing and treating MIRI.
2.3 Anti-inflammation
During cardiac ischemia, a large number of pro-inflammatory factors are released, causing the adhesion and migration of neutrophils and vascular endothelial cells, which eventually accumulate around myocardial tissue to release lysosomal enzymes, causing damage to myocardial cells and becoming a key factor inducing cardiac I/R injury (Qiu et al., 2018).
The anti-inflammatory effect of Salvianic acid A on myocardial cells has been widely studied and reported. Salvianic acid A can reduce the levels of tumor necrosis factor alpha (TNF-α), interleukin (IL)-1, and IL-6 (Quan et al., 2012); increase left ventricular ejection fraction levels and left ventricular fractional shortening; and reduce cell swelling, inflammatory cell abundance, and MI area (Jia et al., 2019b). Salvianic acid A may have a protective effect on the myocardium by inhibiting the inflammatory response to MIRI.
2.4 Improvement of mitochondrial function
Mitochondria are the most important organelles supplying energy to cardiomyocytes. Damage to mitochondria during MI results in an insufficient energy supply. After reperfusion, mitochondria in ischemic tissue cannot quickly recover their normal form, and ischemic injury persists for a period before improvement occurs, aggravating myocardial cell injury and even causing death (Anzell et al., 2018). The stability of mitochondrial function is closely related to the mitochondrial membrane potential and the mitochondrial permeability transition pore (MPTP) (Griffiths, 2012).
Studies have shown that Salvianic acid A can inhibit opening of the MPTP, stabilize the mitochondrial membrane potential of cardiomyocytes, improve mitochondrial function, and alleviate cardiac I/R injury both in vivo and in vitro by preventing mass ROS production or inhibiting the expression of the c-subunit of adenosine triphosphate (Ren et al., 2019). However, because the relationship between mitochondria and MIRI is not fully understood, further studies exploring the role of mitochondria in the treatment of MIRI using Salvianic acid A are necessary.
2.5 Regulation of autophagy
Autophagy is a key target in the treatment of I/R injury (Aghaei et al., 2019). Excessive autophagy in the myocardial I/R stage leads to the degradation of key proteases in the heart, resulting in increased cell death and aggravated heart injury (Lin et al., 2018). Several studies support the function of Salvianic acid A in the regulation of autophagy-related pathways. Fan et al. (2016) investigated the protective effect of Salvianic acid A on cardiomyocytes and cardiac I/R injury in an isolated rat heart model. Salvianic acid A pretreatment activated the targeted autophagy and apoptosis pathways through mammalian target of rapamycin (mTOR), causing the downregulation of autophagy-related proteins including LC3-II, Beclin-1, and p62, thus alleviating MIRI. Furthermore, Xie et al. (2021) discovered that a novel Salvianic acid A derivative (DT-010) can alleviate oxidative stress-induced autophagy injury in cardiomyocytes via the AMPK–mTOR–Ulk1 signaling pathway and is a potential drug for use in the treatment of MIRI.
2.6 Reduction of calcium overload
During MIRI, calcium overload can cause the generation and release of downstream active factors, induce harmful reactions in cells, and interact with inflammation, oxidative stress, and other mechanisms to cause or aggravate myocardial cell injury (Soares et al., 2019).
Salvianic acid A inhibits the influx of calcium ions at the cellular level. Lam et al. (2007) used a 5-HT precontracted rat model and found that Salvianic acid A had a cardioprotective effect on the heart by dilating coronary vasculature through inhibition of Ca2+ influx in vascular smooth muscle. At the same time, Salvianic acid A has a regulatory effect on calcium channels. Salvianic acid A can block L-type calcium channel current on myocardial cell membranes (Song et al., 2016). It can also reduce the expression of calcium channel protein TRPC6 by inhibiting p-JNK, nuclear factor kappa-B (NF-κB), and other signaling pathways, ultimately reducing Ca2+ influx and the damage caused by calcium overload in myocardial cells (Meng et al., 2016).
2.7 Improvement in endothelial progenitor cell function
Endothelial progenitor cells (EPCs) have a self-proliferation and multi-differentiation capacity (Chopra et al., 2018). In the event of tissue ischemia, EPCs in bone marrow can directly form blood vessels, differentiate into endothelial cells, and integrate with ischemic tissue to engage in the generation of new blood vessels (Sun et al., 2021b; Tani, 1990).
Research on the potential relationship between Salvianic acid A and EPCs could yield novel angiogenesis therapy tools. Yin et al. (2017) found that the cardioprotective effect of Salvianic acid A in an MI rat model may be associated with the promotion of myocardial neovascularization. The potential mechanisms include enhancing EPC survival in a hypoxic environment through Akt phosphorylation and accelerating angiogenesis in EPCs through the SDF-1α–CXCR4 axis. The authors also found that Salvianic acid A increased vascular endothelial growth factor (VEGF) and basic fibroblast growth factor expression in myocardial tissue surrounding the infarcted area after MI, significantly promoting new blood vessel growth in the marginal region of the infarcted zone, which can alleviate MIRI and cardiac insufficiency.
Recently, researchers have conducted many studies on the therapeutic aspects of Salvianic acid A in the treatment of MIRI and have found that Salvianic acid A does not act through a single pathway but rather synergistically modulates multiple mechanisms to achieve a protective effect (Sun DW. et al., 2019). However, how the various pathways work synergistically to achieve this effect needs to be studied further. In addition, more research is necessary to investigate the use of Salvianic acid A in combination with other drugs in MIRI treatment.
3 Protective effects of Salvianic acid A against cerebral I/R injury
Cerebral I/R injury (CIRI) is one of the most common cerebrovascular diseases. It mainly occurs when blood vessels recanalize after cerebral ischemia and blood reperfusion further aggravates the pathological injury of ischemic tissue, causing secondary injury to patients with ischemic stroke and greater damage to the nervous system (Wang et al., 2022; Xia, 2018). The pathological mechanisms underlying CIRI are complex, involving a combination of calcium overload, excitatory amino acid toxicity, mitochondrial dysfunction, oxygen free-radical accumulation, blood–brain barrier (BBB) destruction, autophagy, and inflammation (Xue et al., 2022).
Earlier work suggests that Salvianic acid A exhibits a protective effect against cerebral ischemic disease, diminishing cerebral infarct volume and brain tissue edema, promoting neuronal and vascular regeneration, and improving CIRI (Jia et al., 2022). Various mechanisms underlying CIRI treatment have been reported, including anti-oxidative stress, the promotion of anti-inflammation, endogenous neurogenesis, and anti-apoptotic effects. Further research should be carried out to investigate additional protection mechanisms (Yuan et al., 2010) (Figure 4). However, because of low lipid solubility, the ability of Salvianic acid A to penetrate the BBB alone is limited. Therefore, most existing studies use a synthetic Salvianic acid A carrier system or derivatives to improve the BBB penetration rate of Salvianic acid A and increase the effective concentration of the drug to promote the therapeutic effects of the drug (Li et al., 2023) 18.
3.1 Anti-oxidative effects
After brain tissue ischemia, high amounts of ROS are produced rapidly and cause organelle damage, and the rapid recovery of blood oxygen supply during reperfusion directly leads to the second explosion of ROS generation, thus leading to brain reperfusion injury (Rodrigo et al., 2013). Salvianic acid A, as an oxygen free-radical scavenger, has an inhibitory effect on the overproduction of oxidation factors during CIRI. Yuan et al. (2010) found that in the cerebral cortex of model rats, Salvianic acid A can improve cerebral I/R injury by raising the activities of SOD and GSH-PX while decreasing xanthine oxidase activity and MDA expression to scavenge excessive ROS, reduce the level of cerebral oxidative stress, and alleviate cerebral infarction. Another study synthesized the hybrid of Tanshinol borneol ester (DBZ) with reference to traditional Chinese medicine theory and concluded that DBZ has antioxidant activity in I/R injury mouse models (Yuan et al., 2018). Specifically, DBZ can suppress lipid peroxidation, raise SOD and GSH-PX activities in model mouse brains, and reduce oxidative stress and MDA levels in the brain.
3.2 Promotion of endogenous neurogenesis
Cerebral ischemia can stimulate endogenous neurogenesis in the brain as a defensive response to tissue injury (Koh and Park, 2017). Endogenous neurogenesis contributes to brain tissue repair after ischemic stroke and improves brain neurological function after stroke (Marques et al., 2019). Salvianic acid A can promote cerebral angiogenesis to some extent. Wei et al. (2018) found that in a focal stroke model of mice, chronic Salvianic acid A treatment promoted endogenous neurogenesis and collagen generation by up-regulating the expression of doublecortin-positive cells, VEGF, brain-derived neurotrophic factor, endothelial nitric oxide synthase, and other key nutritional and regenerative factors. This is manifested by increased growth of new neurons, endothelial cell/vascular proliferation, and collateral artery formation, which contribute to the restoration of local blood flow, ultimately leading to long-term sensorimotor recovery in focal ischemic stroke. Jia et al. (2022) demonstrated the role of Salvianic acid A in enhancing angiogenesis in vivo and in vitro by regulating the PI3K/Akt/mTOR signaling pathway and upregulating VEGF expression.
3.3 Anti-inflammatory effects
Severe neuroinflammation during brain ischemia can lead to CIRI, BBB destruction, neuron damage, and vascular aging, further aggravating brain injury (Xu S. et al., 2020). In addition, excessive ROS accumulation can induce inflammation and lead to microcirculation disorders in brain tissue, thus impairing the neurons in the central nervous system (CNS) (Xiang et al., 2021). Research suggests that Salvianic acid A can prevent CIRI through an anti-inflammatory pathway, which involves inhibition of the TLR4–NFκB signal transduction pathway and a decrease in inflammation-associated proteins such as TLR4, NF-κB, NOD-like receptor thermal protein domain associated protein 3, and TNF-α. By decreasing downstream inflammatory factors such as caspase-1, IL-1β, and IL-18, it achieves protection of nerves injured by brain I/R effects (Xv et al., 2018; Yang et al., 2009).
Furthermore, the combination of Salvianic acid A and HSYA has been observed to have stronger anti-inflammatory and antioxidant neuroprotective effects on ischemic tissue, which reflects the unique advantages of compound compatibility in TCM theory (Xu et al., 2017). Owing to its antioxidant and anti-inflammatory properties, Salvianic acid A shows significant protective effects when administered alone; furthermore, the combination of Salvianic acid A and HSYA may have additive or synergistic protective effects, resulting in the enhancement of its neuroprotective effects against CIRI.
3.4 Anti-apoptosis
Apoptosis is one of the crucial elements contributing to cerebral I/R (Tuo et al., 2022). Protecting ischemic core cells or penumbra cells from the interference of apoptosis signals is one of the common therapeutic strategies for cerebral I/R injury (Uzdensky, 2019). Salvianic acid A can also regulate some apoptosis signaling pathways in the brain. Several studies have found that intravenous injection of Salvianic acid A can inhibit caspase-3/7 activity by activating the PI3K/Akt signaling pathway to regulate the expression of apoptotic genes Bax and Bcl-2 (Guo et al., 2015), inhibiting the phosphorylation of AKT1 and mTOR by activating AKT1 to reduce the rate of nerve cell apoptosis or improving the neurological dysfunction and pathological morphology in brain tissues of damaged areas, in turn alleviating cerebral ischemia tissue reperfusion injury (Yu et al., 2014; Yu et al., 2015; Zhang et al., 2019).
3.5 Inhibition of the BBB and drug penetration
As the most selective physiological barrier in the human body (Liao et al., 2023), the BBB protects the CNS from potentially harmful substances, although this also presents considerable challenges for the treatment of CNS disease (Nilles et al., 2022). Many drugs cannot penetrate the BBB, resulting in an insufficient effective concentration of drugs (Yang et al., 2019). P-glycoprotein is an efflux transporter in the brain capillaries that form the BBB that functions as a crucial factor in the entry of therapeutic drugs to the CNS (Chai et al., 2022). Yu et al. (2011) reported that Salvianic acid A can synergize with cerebral I/R to inhibit P-glycoprotein expression, significantly increasing the solubility of Salvianic acid A in brain tissue, with positive effects on the treatment of ischemic cerebrovascular disease. Nevertheless, more research is necessary to clarify the specific mechanisms of its effects.
Although Salvianic acid A, as a small polar molecule, is difficult to accumulate in large quantities in the brain, its multi-pathway protective effects on CIRI are observable. Researchers have developed a number of Salvianic acid A derivatives and delivery platforms to enhance its ability to cross the BBB (Hui et al., 2018). Interestingly, in previous studies, Salvianic acid A seems to promote new neuron generation in CIRI, which has attracted considerable research interest, and further studies are anticipated (Yu et al., 2005).
4 Protective effect of Salvianic acid A against renal I/R injury
With the increasing incidence of hematological malignancies, the frequency of renal I/R injury after kidney resection, kidney transplantation, and complex cardiovascular surgery has increased gradually (Chatauret et al., 2014). Renal I/R injury can also directly or indirectly cause kidney disease, including acute kidney injury, and delayed renal function transplantation, which has adverse postoperative consequences (Saat et al., 2016).
One study found that the anti-inflammatory and antioxidant effects of Salvianic acid A can protect against renal I/R injury to some degree and reduce the occurrence and progression of subsequent kidney disease. Wang reported that Salvianic acid A can reduce MPO activity and inhibit the expression of inflammatory factors such as IL-1β, IL-6, and TNF-α (Wang, 2014). The authors also found that Salvianic acid A increased SOD expression and inhibited MDA expression through anti-inflammatory and antioxidant effects to attenuate the effects of renal I/R injury in mice.
Furthermore, Salvianic acid A can inhibit inflammatory and oxidative reactions in the process of renal injury through the regulation of Nrf2/HO-1, NF-ĸB (Yu et al., 2021), and TGF-β/Smad3 (Guan et al., 2015), and can modulate the expression of factors such as IL-1β, IL-6, TNF-α, MDA, and SOD (Wang, 2014), thus reducing renal injury, particularly renal toxicity induced by chronic renal injury and cisplatin.
As renal I/R injury conditions are increasingly widespread, there is an increased focus on their prevention and treatment, and Salvianic acid A is a good candidate; however, unlike that in the heart and brain, the protective role of Salvianic acid A in renal I/R injury needs to be further validated and researched, and more mechanisms for this role may be unraveled.
5 Protective effect of Salvianic acid A against liver I/R injury
I/R injury in the liver is mostly caused during liver transplantation to treat end-stage liver disease (Gazia et al., 2021). Liver function is significantly affected by I/R injury, which in turn affects the prognosis and survival rate of recipients of liver transplants and also limits the success of transplantation (Quesnelle et al., 2015). Salvianic acid A, as a common protective agent against I/R organ damage, exerts its effects through targets in the liver. Huang et al. (2018) reported that Salvianic acid A pretreatment could modulate the Nrf2–HO-1 axis to inhibit p22phox protein expression to counteract oxidative damage, and could increase TGF-β1 expression and downregulate intercellular cell adhesion molecule-1 expression to inhibit the inflammatory response, thus achieving protective effects on liver I/R injury.
Salvianic acid A has also been identified to have favorable protective effects against acute and chronic liver injury that is triggered by a variety of stimuli, including omethoate poisoning (Ren et al., 2010), iron overload (Zhang et al., 2020), carbon tetrachloride (Wang et al., 2007), acetaminophen (Zhou et al., 2015), and alcohol toxicity in vivo and in vitro (Lan et al., 2020). The protective mechanisms mainly involve antioxidant, anti-inflammatory, and anti-apoptosis effects, along with the maintenance of mitochondrial function and promotion of blood circulation. We postulate that Salvianic acid A exerts a considerable protective effect on liver injury.
Overall, the protective effect of Salvianic acid A on liver I/R injury still has great research potential for the future. Through in-depth research and clinical trials, the mechanism of action and clinical application prospects of Salvianic acid A could be clarified, in addition to providing more effective approaches for the treatment of liver I/R injury.
6 Protective effect of Salvianic acid A against intestinal I/R injury
Intestinal I/R injury mostly occurs during major cardiovascular surgery (Mallick et al., 2004). Increased intestinal permeability after cell injury leads to interstitial edema and increased intestinal endotoxin and bacterial translocation, triggering inflammatory responses, oxidative stress, and other injury mechanisms that ultimately aggravate intestinal ischemia injury (Schoenberg and Beger, 1993). The effects of Salvianic acid A on the microcirculatory system make a significant contribution to intestinal I/R injury treatment as well. Research suggests that Salvianic acid A can reduce peroxide production, leukocyte adhesion, and albumin leakage through the mesenteric vein wall for I/R-induced microcirculation dysfunction irrespective of whether Salvianic acid A is administered as a pre-treatment or after the onset of ischemia, while mast cell granulation can only be inhibited by Salvianic acid A before treatment (Han et al., 2009). The results show that Salvianic acid A exerts its therapeutic action on intestinal I/R injury via antioxidant systems and inhibiting CD11b/CD18, a leukocyte adhesion molecule.
Research on applications of Salvianic acid A in the treatment of intestinal I/R injury is still limited, and the relevant studies are still in the primary stages. Additional studies are required to verify its efficacy and provide novel, effective options for the treatment of intestinal I/R injury.
7 Protective effect of Salvianic acid A against I/R injury in other organs
Salvianic acid A also exerts protective effects on other organs against I/R damage. In retinal I/R injury, VEGF shows an early increase and a late decrease (Muthusamy et al., 2014). A study found that Salvianic acid A sodium did not significantly affect VEGF expression in the initial stages of reperfusion but downregulates VEGF in the late stages of injury, indicating that Salvianic acid A has a certain preventive effect on retinal neovascularization (Ding et al., 2006). I/R injury of skeletal muscle causes a series of physiological and pathological reactions, affecting normal activities of the body (Delay et al., 2017). The specific underlying mechanism may be that after activation, leukocytes migrate and adhere to the surrounding venous endothelial cells, aggravating ischemic injury and preventing microvascular reperfusion by releasing active factors or damaging the microvascular barrier (Gillani et al., 2012). It has been reported that Salvianic acid A can reduce the levels of creatine kinase, aspartate aminotransferase, lactate dehydrogenase, and MDA; raise SOD activity; and attenuate I/R injury in rabbit hindlimb skeletal muscle through its antioxidant effects (Feng et al., 1990).
Current research on Salvianic acid A in I/R injury has indeed focused mainly on the brain and the heart, with fewer studies focusing on other organs. This may be related to the fact that the brain and heart, as important organs in the human body, are more obviously and severely affected by I/R injury. The anti-inflammatory and antioxidant effects of Salvianic acid A have been verified in various organs. However, understanding the specific role of Salvianic acid A in different organs could optimize its therapeutic application for the treatment of I/R injury in specific organs. In future studies, researchers could apply histological techniques to explore the interactions of multiple signaling pathways associated with Salvianic acid A in depth for a comprehensive understanding of its preventive and therapeutic potential in I/R injury.
8 Conclusion
Salvia miltiorrhiza has been used in TCM for thousands of years, and its safety and efficacy have been verified for a long period. Salvianic acid A, as one of many water-soluble phenolic acids contained in S. miltiorrhiza, has attracted considerable research interest because of its protective effect on many organs. Salvianic acid A has a variety of pharmacologic effects, including anti-oxidation, anti-inflammation, and anti-apoptosis properties; reducing calcium overload; inhibiting platelet aggregation: improving microcirculation; and protecting endothelial cells, and has a protective function in I/R injury in the cardiovascular system, brain, liver, kidneys, and gastrointestinal tract. Researchers have identified numerous relevant signaling pathways and targets that are implicated in the protection of Salvianic acid A during organ damage. Understanding such mechanisms may also facilitate the identification of additional diseases that may respond to Salvianic acid A treatment.
However, the structural characteristics of Salvianic acid A limit its research and application to some extent. The molecular weight of Salvianic acid A is not high; however, the contents of polar groups in the entire structure are relatively high. As a result of this structure, Salvianic acid A has poor lipid solubility and is not able to enter tissues and organs through biofilms after being absorbed and distributed in the body via blood. In particular, the concentration of Salvianic acid A entering the brain is very low due to difficulty in penetrating the BBB, which is a key reason why the therapeutic effect of Salvianic acid A has not met expectations. In this review, we identify many studies investigating how to improve the fat solubility of Salvianic acid A and increase the effective concentration of Salvianic acid A in organs. There are two main approaches. The first is to modify the structure of Salvianic acid A. By modifying the groups, structural polarity can be adjusted to balance lipid solubility and water solubility and improve Salvianic acid A bioavailability. The second approach focuses on drug delivery, new materials, or biotechnology to establish a carrier system for Salvianic acid A, which can deliver Salvianic acid A to the target tissue and release it at a fixed point, thereby increasing the effective concentration at a focal site. The studies above propose novel strategies for overcoming the limitations on the therapeutic effect of Salvianic acid A, and satisfactory results have been obtained in studies at the cellular level and in animal models.
Salvianic acid A has considerable potential in the treatment of I/R injury in various organs. However, there are considerable restrictions on Salvianic acid A research and its application in clinical practice. Future developments in science and technology and the pharmaceutical industry will facilitate further research to overcome the limitations and promote the use of Salvianic acid A against ischemic injury.
Author contributions
SY: Conceptualization, Writing–original draft. HC: Writing–original draft. WS: Writing–review and editing. YL: Writing–review and editing. JL: Writing–review and editing. YW: Writing–review and editing. LX: Writing–review and editing. CZ: Writing–review and editing. FL: Writing–review and editing. Z-SC: Writing–review and editing. TW: Investigation, Writing–review and editing.
Funding
The author(s) declare financial support was received for the research, authorship, and/or publication of this article. This work was supported in part by the Natural Science Foundation of Shanghai (Grant No. 20ZR1420000), the Science and Technology Support Project in the Biomedical Field of Science, and the Technology Innovation Action Plan of Shanghai Science and Technology Commission (Grant No. 21S21902100).
Conflict of interest
The authors declare that the research was conducted in the absence of any commercial or financial relationships that could be construed as a potential conflict of interest.
The author(s) declared that they were an editorial board member of Frontiers, at the time of submission. This had no impact on the peer review process and the final decision.
Publisher’s note
All claims expressed in this article are solely those of the authors and do not necessarily represent those of their affiliated organizations, or those of the publisher, the editors and the reviewers. Any product that may be evaluated in this article, or claim that may be made by its manufacturer, is not guaranteed or endorsed by the publisher.
Supplementary material
The Supplementary Material for this article can be found online at: https://www.frontiersin.org/articles/10.3389/fphar.2023.1297124/full#supplementary-material
References
Aghaei, M., Motallebnezhad, M., Ghorghanlu, S., Jabbari, A., Enayati, A., Rajaei, M., et al. (2019). Targeting autophagy in cardiac ischemia/reperfusion injury: a novel therapeutic strategy. J. Cell Physiol. 234 (10), 16768–16778. doi:10.1002/jcp.28345
Al-Mufti, F., Amuluru, K., Roth, W., Nuoman, R., El-Ghanem, M., and Meyers, P. M. (2018). Cerebral ischemic reperfusion injury following recanalization of large vessel occlusions. Neurosurgery 82 (6), 781–789. doi:10.1093/neuros/nyx341
Anzell, A. R., Maizy, R., Przyklenk, K., and Sanderson, T. H. (2018). Mitochondrial quality control and disease: insights into ischemia-reperfusion injury. Mol. Neurobiol. 55 (3), 2547–2564. doi:10.1007/s12035-017-0503-9
Bai, J., and Lyden, P. D. (2015). Revisiting cerebral postischemic reperfusion injury: new insights in understanding reperfusion failure, hemorrhage, and edema. Int. J. Stroke 10 (2), 143–152. doi:10.1111/ijs.12434
Baker, J. E., Felix, C. C., Olinger, G. N., and Kalyanaraman, B. (1988). Myocardial ischemia and reperfusion: direct evidence for free radical generation by electron spin resonance spectroscopy. Proc. Natl. Acad. Sci. U. S. A. 85 (8), 2786–2789. doi:10.1073/pnas.85.8.2786
Bao, X. Y., Zheng, Q., Tong, Q., Zhu, P. C., Zhuang, Z., Zheng, G. Q., et al. (2018b). Danshensu for myocardial ischemic injury: preclinical evidence and novel methodology of quality assessment tool. Front. Pharmacol. 9, 1445. doi:10.3389/fphar.2018.01445
Bao, X. Y., Zheng, Q., Tong, Q., Zhu, P. C., Zhuang, Z., Zheng, G. Q., et al. (2018a). Danshensu for myocardial ischemic injury: preclinical evidence and novel methodology of quality assessment tool. Front. Pharmacol. 9, 1445. doi:10.3389/fphar.2018.01445
Bi, R., Chen, S., Chen, S., Peng, Q., Jin, H., and Hu, B. (2021). The role of leukocytes in acute ischemic stroke-related thrombosis: a notable but neglected topic. Cell Mol. Life Sci. 78 (17-18), 6251–6264. doi:10.1007/s00018-021-03897-5
Bianconi, V., Sahebkar, A., Kovanen, P., Bagaglia, F., Ricciuti, B., Calabrò, P., et al. (2018). Endothelial and cardiac progenitor cells for cardiovascular repair: a controversial paradigm in cell therapy. Pharmacol. Ther. 181, 156–168. doi:10.1016/j.pharmthera.2017.08.004
Bugger, H., and Pfeil, K. (2020). Mitochondrial ROS in myocardial ischemia reperfusion and remodeling. Biochim. Biophys. Acta Mol. Basis Dis. 1866 (7), 165768. doi:10.1016/j.bbadis.2020.165768
Chai, A. B., Callaghan, R., and Gelissen, I. C. (2022). Regulation of P-glycoprotein in the brain. Int. J. Mol. Sci. 23 (23), 14667. Published 2022 Nov 24. doi:10.3390/ijms232314667
Chatauret, N., Badet, L., Barrou, B., and Hauet, T. (2014). Ischemia-reperfusion: from cell biology to acute kidney injury. Prog. Urol. 24 (Suppl. 1), S4–S12. doi:10.1016/S1166-7087(14)70057-0
Cho, Y. H., Ku, C. R., Hong, Z. Y., Heo, J. H., Kim, E. H., Choi, D. H., et al. (2013). Therapeutic effects of water soluble danshen extracts on atherosclerosis. Evid. Based Complement. Altern. Med. 2013, 623639. doi:10.1155/2013/623639
Choi, J. H., and Pile-Spellman, J. (2018). Reperfusion changes after stroke and practical approaches for neuroprotection. Neuroimaging Clin. N. Am. 28 (4), 663–682. doi:10.1016/j.nic.2018.06.008
Chopra, H., Hung, M. K., Kwong, D. L., Zhang, C. F., and Pow, E. H. N. (2018). Insights into endothelial progenitor cells: origin, classification, potentials, and prospects. Stem Cells Int. 2018, 9847015. doi:10.1155/2018/9847015
Dambrova, M., Zuurbier, C. J., Borutaite, V., Liepinsh, E., and Makrecka-Kuka, M. (2021). Energy substrate metabolism and mitochondrial oxidative stress in cardiac ischemia/reperfusion injury. Free Radic. Biol. Med. 165, 24–37. doi:10.1016/j.freeradbiomed.2021.01.036
Delay, C., Paradis, S., Charles, A. L., Thaveau, F., Chenesseau, B., Zoll, J., et al. (2017). Skeletal muscle ischemia-reperfusion and ischemic conditioning pathophysiology-clinical applications for the vascular surgeon. J. Med. Vasc. 42 (1), 29–38. doi:10.1016/j.jmv.2016.11.001
Dery, K. J., and Kupiec-Weglinski, J. W. (2022). New insights into ischemia-reperfusion injury signaling pathways in organ transplantation. Curr. Opin. Organ Transpl. 27 (5), 424–433. doi:10.1097/MOT.0000000000001005
Ding, J. G., Jiang, D. Y., and Wu, R. H. (2006). Effect of danshensu-sodium on VEGF expression after retinal ischemia-reperfusion in rabbits. Recent Adv. Ophthalmol. 2006 (05), 357–360. doi:10.13389/j.cnki.rao.2006.05.012
Fan, G., Yu, J., Asare, P. F., Wang, L., Zhang, H., Zhang, B., et al. (2016). Danshensu alleviates cardiac ischaemia/reperfusion injury by inhibiting autophagy and apoptosis via activation of mTOR signalling. J. Cell Mol. Med. 20, 1908–1919. doi:10.1111/jcmm.12883
Fei, Y. X., Wang, S. Q., Yang, L. J., Qiu, Y. Y., Li, Y. Z., Liu, W. Y., et al. (2017). Salvia miltiorrhiza Bunge (Danshen) extract attenuates permanent cerebral ischemia through inhibiting platelet activation in rats. J. Ethnopharmacol. 207, 57–66. doi:10.1016/j.jep.2017.06.023
Feng, F., Feng, S. C., and Jia, J. Z. (1990). The protection of Salvia miltiorrhia on rabbit skeletal muscle ischemia and reperfusion injury. Chin. J. Traditional Med. Traumatology Orthop. 6 (03), 3–5+2.
Feske, S. K. (2021). Ischemic stroke. Am. J. Med. 134 (12), 1457–1464. doi:10.1016/j.amjmed.2021.07.027
Gao, Q., Deng, H., Yang, Z., Yang, Q., Zhang, Y., Yuan, X., et al. (2022). Sodium danshensu attenuates cerebral ischemia-reperfusion injury by targeting AKT1. Front. Pharmacol. 13, 946668. doi:10.3389/fphar.2022.946668
Gao, Q., Zhao, J., Fan, Z., Bao, J., Sun, D., Li, H., et al. (2017). Cardioprotective effect of danshensu against ischemic/reperfusion injury via c-subunit of ATP synthase inhibition. Evid. Based Complement. Altern. Med. 2017, 7986184. doi:10.1155/2017/7986184
Gazia, C., Lenci, I., Manzia, T. M., Martina, M., Tisone, G., Angelico, R., et al. (2021). Current strategies to minimize ischemia-reperfusion injury in liver transplantation: a systematic review. Rev. Recent Clin. Trials 16 (4), 372–380. doi:10.2174/1574887116666210729112932
Ge, X., Meng, Q., Wei, L., Liu, J., Li, M., Liang, X., et al. (2021). Myocardial ischemia-reperfusion induced cardiac extracellular vesicles harbour proinflammatory features and aggravate heart injury. J. Extracell. Vesicles 10 (4), e12072. doi:10.1002/jev2.12072
Gillani, S., Cao, J., Suzuki, T., and Hak, D. J. (2012). The effect of ischemia reperfusion injury on skeletal muscle. Injury 43 (6), 670–675. doi:10.1016/j.injury.2011.03.008
Gorsuch, W. B., Chrysanthou, E., Schwaeble, W. J., and Stahl, G. L. (2012). The complement system in ischemia-reperfusion injuries. Immunobiology 217 (11), 1026–1033. doi:10.1016/j.imbio.2012.07.024
Griffiths, E. J. (2012). Mitochondria and heart disease. Adv. Exp. Med. Biol. 942, 249–267. doi:10.1007/978-94-007-2869-1_11
Guan, Y., Wu, X. X., Duan, J. L., Yin, Y., Guo, C., Wei, G., et al. (2015). Effects and mechanism of combination of rhein and danshensu in the treatment of chronic kidney disease. Am. J. Chin. Med. 43 (7), 1381–1400. doi:10.1142/S0192415X15500780
Guan, Y., Yin, Y., Zhu, Y. R., Guo, C., Wei, G., Duan, J. L., et al. (2013). Dissection of mechanisms of a Chinese medicinal formula: danhong injection therapy for myocardial ischemia/reperfusion injury in vivo and in vitro. Evid. Based Complement. Altern. Med. 2013, 972370. doi:10.1155/2013/972370
Guo, C., Yin, Y., Duan, J., Zhu, Y., Yan, J., Wei, G., et al. (2015). Neuroprotective effect and underlying mechanism of sodium danshensu [3-(3,4-dihydroxyphenyl) lactic acid from Radix and Rhizoma Salviae miltiorrhizae = Danshen] against cerebral ischemia and reperfusion injury in rats. Phytomedicine 22 (2), 283–289. doi:10.1016/j.phymed.2014.12.001
Guo, J., Zhang, Y., Zeng, L., Liu, J., Liang, J., and Guo, G. (2013). Salvianic acid A protects L-02 cells against γ-irradiation-induced apoptosis via the scavenging of reactive oxygen species. Environ. Toxicol. Pharmacol. 35 (1), 117–130. doi:10.1016/j.etap.2012.11.010
Guo, Y., Xu, X., Li, Q., Li, Z., and Du, F. (2010). Anti-inflammation effects of picroside 2 in cerebral ischemic injury rats. Behav. Brain Funct. 6, 43. doi:10.1186/1744-9081-6-43
Han, J. Y., Horie, Y., Fan, J. Y., Sun, K., Guo, J., Miura, S., et al. (2009). Potential of 3,4-dihydroxy-phenyl lactic acid for ameliorating ischemia-reperfusion-induced microvascular disturbance in rat mesentery. Am. J. Physiol. Gastrointest. Liver Physiol. 296 (1), G36–G44. doi:10.1152/ajpgi.90284.2008
Han, J. Y., Li, Q., Ma, Z. Z., and Fan, J. Y. (2017). Effects and mechanisms of compound Chinese medicine and major ingredients on microcirculatory dysfunction and organ injury induced by ischemia/reperfusion. Pharmacol. Ther. 177, 146–173. doi:10.1016/j.pharmthera.2017.03.005
Hao, P., Jiang, F., Cheng, J., Ma, L., Zhang, Y., and Zhao, Y. (2017). Traditional Chinese medicine for cardiovascular disease: evidence and potential mechanisms. J. Am. Coll. Cardiol. 69 (24), 2952–2966. doi:10.1016/j.jacc.2017.04.041
Hausenloy, D. J., and Yellon, D. M. (2013). Myocardial ischemia-reperfusion injury: a neglected therapeutic target. J. Clin. Invest. 123 (1), 92–100. doi:10.1172/JCI62874
Heusch, G., and Gersh, B. J. (2017). The pathophysiology of acute myocardial infarction and strategies of protection beyond reperfusion: a continual challenge. Eur. Heart J. 38 (11), 774–784. doi:10.1093/eurheartj/ehw224
Hu, T., Wei, G., Xi, M., Yan, J., Wu, X., Wang, Y., et al. (2016). Synergistic cardioprotective effects of Danshensu and hydroxysafflor yellow A against myocardial ischemia-reperfusion injury are mediated through the Akt/Nrf2/HO-1 pathway. Int. J. Mol. Med. 38 (1), 83–94. doi:10.3892/ijmm.2016.2584
Huang, D. D., Wei, X. H., Mu, H. N., Pan, C. S., Li, Q., Hu, B. H., et al. (2019). Total salvianolic acid injection prevents ischemia/reperfusion-induced myocardial injury via antioxidant mechanism involving mitochondrial respiratory chain through the upregulation of Sirtuin1 and Sirtuin3. Shock 51 (6), 745–756. doi:10.1097/SHK.0000000000001185
Huang, Q. X., Yu, Y. Y., Wang, X. F., et al. (2018). Protective role and pathogenesis of Salvianic Acid A on hepatic ischemia reperfusion injury in rats. Electron. J. Liver Tumor 5 (2), 24–27.
Hui, A., Yin, H., Zhang, Z., Zhou, A., Chen, J., Yang, L., et al. (2018). Enhancement of brain-targeting delivery of danshensu in rat through conjugation with pyrazine moiety to form danshensu-pyrazine ester. Drug Deliv. Transl. Res. 8 (3), 787–796. doi:10.1007/s13346-018-0501-0
Ibáñez, B., Heusch, G., Ovize, M., and Van de Werf, F. (2015). Evolving therapies for myocardial ischemia/reperfusion injury. J. Am. Coll. Cardiol. 65 (14), 1454–1471. doi:10.1016/j.jacc.2015.02.032
Jernryd, V., Metzsch, C., Andersson, B., and Nilsson, J. (2020). The influence of ischemia and reperfusion time on outcome in heart transplantation. Clin. Transpl. 34 (5), e13840. doi:10.1111/ctr.13840
Jia, D., Xiong, L., Yu, X., Chen, X., Wang, T., Chen, A. F., et al. (2019b). Cardioprotective mechanism study of salvianic acid A sodium based on a proteome microarray approach and metabolomic profiling of rat serum after myocardial infarction. Mol. Omics 15 (4), 271–279. doi:10.1039/c9mo00005d
Jia, D., Zhang, C. Z., Qiu, Y., Chen, X. F., Jia, L., Chen, A. F., et al. (2019a). Cardioprotective mechanisms of salvianic acid A sodium in rats with myocardial infarction based on proteome and transcriptome analysis. Acta Pharmacol. Sin. 40 (12), 1513–1522. doi:10.1038/s41401-019-0265-1
Jia, H., Qi, X., Wu, H., and Wang, J. (2022). Danshensu enhances cerebral angiogenesis in mice by regulating the PI3K/Akt/Mtor/VEGF signaling Axis. CNS Neurol. Disord. Drug Targets 22, 607–613. doi:10.2174/1871527321666220329144538
Kalogeris, T., Baines, C. P., Krenz, M., and Korthuis, R. J. (2012). Cell biology of ischemia/reperfusion injury. Int. Rev. Cell Mol. Biol. 298, 229–317. doi:10.1016/B978-0-12-394309-5.00006-7
Kalogeris, T., Baines, C. P., Krenz, M., and Korthuis, R. J. (2016). Ischemia/reperfusion. Compr. Physiol. 7 (1), 113–170. doi:10.1002/cphy.c160006
Koh, S. H., and Park, H. H. (2017). Neurogenesis in stroke recovery. Transl. Stroke Res. 8 (1), 3–13. doi:10.1007/s12975-016-0460-z
Kurian, G. A., Rajagopal, R., Vedantham, S., and Rajesh, M. (2016). The role of oxidative stress in myocardial ischemia and reperfusion injury and remodeling: revisited. Oxid. Med. Cell Longev. 2016, 1656450. doi:10.1155/2016/1656450
Lam, F. F., Yeung, J. H., Chan, K. M., and Or, P. M. (2007). Relaxant effects of danshen aqueous extract and its constituent danshensu on rat coronary artery are mediated by inhibition of calcium channels. Vasc. Pharmacol. 46 (4), 271–277. doi:10.1016/j.vph.2006.10.011
Lan, Y., Yan, R., Shan, W., Chu, J., Sun, R., Wang, R., et al. (2020). Salvianic acid A alleviates chronic alcoholic liver disease by inhibiting HMGB1 translocation via down-regulating BRD4. J. Cell Mol. Med. 24 (15), 8518–8531. doi:10.1111/jcmm.15473
Li, H., Song, F., Duan, L. R., Sheng, J. J., Xie, Y. H., Yang, Q., et al. (2016). Paeonol and danshensu combination attenuates apoptosis in myocardial infarcted rats by inhibiting oxidative stress: roles of Nrf2/HO-1 and PI3K/Akt pathway. Sci. Rep. 6, 23693. doi:10.1038/srep23693
Li, H., Xie, Y. H., Yang, Q., Wang, S. W., Zhang, B. L., Wang, J. B., et al. (2012). Cardioprotective effect of paeonol and danshensu combination on isoproterenol-induced myocardial injury in rats. PLoS One 7 (11), e48872. doi:10.1371/journal.pone.0048872
Li, J., Gao, Y. H., Ren, X. M., Wu, L., Yang, X., et al. (2017). The role of biologically active ingredients from Chinese Herbal Medicines in the regulation of autophagy in treating cardiovascular diseases and other chronic diseases. Curr. Pharm. Des. 23 (7), 1060–1069. doi:10.2174/1381612822666161021161850
Li, Y., Luo, Y., Wang, J., Shi, H., Liao, J., Wang, Y., et al. (2023). Discovery of novel danshensu derivatives bearing pyrazolone moiety as potential anti-ischemic stroke agents with antioxidant activity. Bioorg Chem. 131, 106283. doi:10.1016/j.bioorg.2022.106283
Li, Z. M., Xu, S. W., and Liu, P. Q. (2018). Salvia miltiorrhizaBurge (Danshen): a golden herbal medicine in cardiovascular therapeutics. Acta Pharmacol. Sin. 39 (5), 802–824. doi:10.1038/aps.2017.193
Liao, J., Fan, L., Li, Y., Xu, Q. Q., Xiong, L. Y., Zhang, S. S., et al. (2023). Recent advances in biomimetic nanodelivery systems: new brain-targeting strategies. J. Control Release 358, 439–464. doi:10.1016/j.jconrel.2023.05.009
Lin, T. H., and Hsieh, C. L. (2010). Pharmacological effects of Salvia miltiorrhiza (Danshen) on cerebral infarction. Chin. Med. 5, 22. doi:10.1186/1749-8546-5-22
Lin, X. L., Xiao, W. J., Xiao, L. L., and Liu, M. H. (2018). Molecular mechanisms of autophagy in cardiac ischemia/reperfusion injury (Review). Mol. Med. Rep. 18 (1), 675–683. doi:10.3892/mmr.2018.9028
Liu, T., Ding, Y., and Wen, A. (2018). Traditional Chinese medicine for ischaemic stroke. Lancet Neurol. 17 (9), 745. doi:10.1016/S1474-4422(18)30290-4
Mallick, I. H., Yang, W., Winslet, M. C., and Seifalian, A. M. (2004). Ischemia-reperfusion injury of the intestine and protective strategies against injury. Dig. Dis. Sci. 49 (9), 1359–1377. doi:10.1023/b:ddas.0000042232.98927.91
Marques, B. L., Carvalho, G. A., Freitas, E. M. M., Chiareli, R. A., Barbosa, T. G., Di Araújo, A. G. P., et al. (2019). The role of neurogenesis in neurorepair after ischemic stroke. Semin. Cell Dev. Biol. 95, 98–110. doi:10.1016/j.semcdb.2018.12.003
Meim, X. D., Cao, Y. F., Che, Y. Y., Li, J., Shang, Z. P., Zhao, W. J., et al. (2019). Danshen: a phytochemical and pharmacological overview. Chin. J. Nat. Med. 17 (1), 59–80. doi:10.1016/S1875-5364(19)30010-X
Meng, Y., Li, W. Z., Shi, Y. W., Zhou, B. F., Ma, R., and Li, W. P. (2016). Danshensu protects against ischemia/reperfusion injury and inhibits the apoptosis of H9c2 cells by reducing the calcium overload through the p-JNK-NF-κB-TRPC6 pathway. Int. J. Mol. Med. 37 (1), 258–266. doi:10.3892/ijmm.2015.2419
Morciano, G., Bonora, M., Campo, G., Aquila, G., Rizzo, P., Giorgi, C., et al. (2017). Mechanistic role of mPTP in ischemia-reperfusion injury. Adv. Exp. Med. Biol. 982, 169–189. doi:10.1007/978-3-319-55330-6_9
Muthusamy, A., Lin, C. M., Shanmugam, S., Lindner, H. M., Abcouwer, S. F., and Antonetti, D. A. (2014). Ischemia-reperfusion injury induces occludin phosphorylation/ubiquitination and retinal vascular permeability in a VEGFR-2-dependent manner. J. Cereb. Blood Flow. Metab. 34 (3), 522–531. doi:10.1038/jcbfm.2013.230
Nilles, K. L., Williams, E. I., Betterton, R. D., Davis, T. P., and Ronaldson, P. T. (2022). Blood-brain barrier transporters: opportunities for therapeutic development in ischemic stroke. Int. J. Mol. Sci. 23 (3), 1898. doi:10.3390/ijms23031898
Pachel, C., Mathes, D., Arias-Loza, A. P., Heitzmann, W., Nordbeck, P., Deppermann, C., et al. (2016). Inhibition of platelet GPVI protects against myocardial ischemia-reperfusion injury. Arterioscler. Thromb. Vasc. Biol. 36 (4), 629–635. doi:10.1161/ATVBAHA.115.305873
Patel, R. A. G., and McMullen, P. W. (2017). Neuroprotection in the treatment of acute ischemic stroke. Prog. Cardiovasc Dis. 59 (6), 542–548. doi:10.1016/j.pcad.2017.04.005
Qian, W., Deng, C. Y., and Xue, Y. M. (2002). Effects of Danshen on L-type calcium channels in isolated cardiomyocytes of Guinea pig. South China J. Cardiovasc. Dis. 2002 (04), 276–278.
Qiu, Y., Zhang, C., Zhang, G., and Tao, J. (2018). Endothelial progenitor cells in cardiovascular diseases. Aging Med. Milt. 1 (2), 204–208. doi:10.1002/agm2.12041
Quan, W., Zhou, D., Guo, C., Wang, Y., Zhu, Y., Guan, Y., et al. (2012). Protective effect of sodium Danshensu on myocardial ischemia-reperfusion injury by inhibiting inflammation. Cent. South Pharm. 10 (12), 885–888. doi:10.3969/j.issn.1672.2981.2012.12.002
Quesnelle, K. M., Bystrom, P. V., and Toledo-Pereyra, L. H. (2015). Molecular responses to ischemia and reperfusion in the liver. Arch. Toxicol. 89 (5), 651–657. doi:10.1007/s00204-014-1437-x
Ren, J., Fu, L., Nile, S. H., Zhang, J., and Kai, G. (2019). Salvia miltiorrhiza in treating cardiovascular diseases: a review on its pharmacological and clinical applications. Front. Pharmacol. 10, 753. doi:10.3389/fphar.2019.00753
Ren, R., Wang, T., Jiang, N., Liu, T., Du, Y., Li, C., et al. (2010). Protective effects of Danshensu on liver injury induced by omethoate in rats. Toxicol. Mech. Methods 20 (8), 510–514. doi:10.3109/15376516.2010.514963
Rentrop, K. P., and Feit, F. (2015). Reperfusion therapy for acute myocardial infarction: concepts and controversies from inception to acceptance. Am. Heart J. 170 (5), 971–980. doi:10.1016/j.ahj.2015.08.005
Rodrigo, R., Fernández-Gajardo, R., Gutiérrez, R., Matamala, J. M., Carrasco, R., Miranda-Merchak, A., et al. (2013). Oxidative stress and pathophysiology of ischemic stroke: novel therapeutic opportunities. CNS Neurol. Disord. Drug Targets 12 (5), 698–714. doi:10.2174/1871527311312050015
Saat, T. C., van den Akker, E. K., Ijzermans, J. N., Dor, F. J., and de Bruin, R. W. (2016). Improving the outcome of kidney transplantation by ameliorating renal ischemia reperfusion injury: lost in translation? J. Transl. Med. 14, 20. doi:10.1186/s12967-016-0767-2
Schirone, L., Forte, M., D'Ambrosio, L., Valenti, V., Vecchio, D., Schiavon, S., et al. (2022). An overview of the molecular mechanisms associated with myocardial ischemic injury: state of the art and translational perspectives. Cells 11 (7), 1165. doi:10.3390/cells11071165
Schoenberg, M. H., and Beger, H. G. (1993). Reperfusion injury after intestinal ischemia. Crit. Care Med. 21 (9), 1376–1386. doi:10.1097/00003246-199309000-00023
Shintani-Ishida, K., Inui, M., and Yoshida, K. (2012). Ischemia-reperfusion induces myocardial infarction through mitochondrial Ca2⁺ overload. J. Mol. Cell Cardiol. 53 (2), 233–239. doi:10.1016/j.yjmcc.2012.05.012
Sies, H. (2015). Oxidative stress: a concept in redox biology and medicine. Redox Biol. 4, 180–183. doi:10.1016/j.redox.2015.01.002
Soares, R. O. S., Losada, D. M., Jordani, M. C., Évora, P., and Castro-E-Silva, O. (2019). Ischemia/reperfusion injury revisited: an overview of the latest pharmacological strategies. Int. J. Mol. Sci. 20 (20), 5034. doi:10.3390/ijms20205034
Song, Q., Chu, X., Zhang, X., Bao, Y., Zhang, Y., Guo, H., et al. (2016). Mechanisms underlying the cardioprotective effect of Salvianic acid A against isoproterenol-induced myocardial ischemia injury in rats: possible involvement of L-type calcium channels and myocardial contractility. J. Ethnopharmacol. 189, 157–164. doi:10.1016/j.jep.2016.05.038
Sun, D. W., Feng, L. S., Gao, Q., et al. (2019b). Danshensu regulating FoxO1 and stabilizing mitochondral membrane potential of cardiomyocyte in ischemia-reperfusion injury rats. Jilin J. Chin. Med. 39 (2), 237–241. doi:10.13463/j.cnki.jlzyy.2019.02.025
Sun, D. W., Gao, Q., and Qi, X. (2019a). Danshensu ameliorates cardiac ischaemia reperfusion injury through activating sirt1/FoxO1/rab7 signal pathway. Chin. J. Integr. Med. 26, 283–291. doi:10.1007/s11655-019-3165-9
Sun, G., Li, X., Wei, J., Zhang, T., Li, B., Chen, M., et al. (2021a). Pharmacodynamic substances in Salvia miltiorrhiza for prevention and treatment of hyperlipidemia and coronary heart disease based on lipidomics technology and network pharmacology analysis. Biomed. Pharmacother. 141, 111846. doi:10.1016/j.biopha.2021.111846
Sun, K., Li, Y. Y., and Jin, J. (2021b). A double-edged sword of immuno-microenvironment in cardiac homeostasis and injury repair. Signal Transduct. Target Ther. 6 (1), 79. doi:10.1038/s41392-020-00455-6
Tang, Y., Wang, M., Le, X., Meng, J., Huang, L., Yu, P., et al. (2011). Antioxidant and cardioprotective effects of Danshensu (3-(3, 4-dihydroxyphenyl)-2-hydroxy-propanoic acid from Salvia miltiorrhiza) on isoproterenol-induced myocardial hypertrophy in rats. Phytomedicine 18 (12), 1024–1030. doi:10.1016/j.phymed.2011.05.007
Tani, M. (1990). Mechanisms of Ca2+ overload in reperfused ischemic myocardium. Annu. Rev. Physiol. 52, 543–559. doi:10.1146/annurev.ph.52.030190.002551
Tian, J., Jin, D., Bao, Q., Ding, Q., Zhang, H., Gao, Z., et al. (2019). Evidence and potential mechanisms of traditional Chinese medicine for the treatment of type 2 diabetes: a systematic review and meta-analysis. Diabetes Obes. Metab. 21 (8), 1801–1816. doi:10.1111/dom.13760
Tibaut, M., Mekis, D., and Petrovic, D. (2017). Pathophysiology of myocardial infarction and acute management strategies. Cardiovasc Hematol. Agents Med. Chem. 14 (3), 150–159. doi:10.2174/1871525714666161216100553
Tu, Y. (2016). Artemisinin-A Gift from traditional Chinese medicine to the World (Nobel lecture). Angew. Chem. Int. Ed. Engl. 55 (35), 10210–10226. doi:10.1002/anie.201601967
Tuo, Q. Z., Zhang, S. T., and Lei, P. (2022). Mechanisms of neuronal cell death in ischemic stroke and their therapeutic implications. Med. Res. Rev. 42 (1), 259–305. doi:10.1002/med.21817
Uzdensky, A. B. (2019). Apoptosis regulation in the penumbra after ischemic stroke: expression of pro- and antiapoptotic proteins. Apoptosis 24 (9-10), 687–702. doi:10.1007/s10495-019-01556-6
Wang, C. Y., Ma, F. L., Liu, J. T., Tian, J. W., and Fu, F. H. (2007). Protective effect of salvianic acid a on acute liver injury induced by carbon tetrachloride in rats. Biol. Pharm. Bull. 30 (1), 44–47. doi:10.1248/bpb.30.44
Wang, J., Fan, K., He, C., Wang, Q., Zhang, Q., and Huang, W. (2021). A novel danshensu/tetramethylpyrazine protects against myocardial ischemia reperfusion injury in rats. Int. J. Med. Sci. 18 (12), 2716–2724. Published 2021 May 13. doi:10.7150/ijms.59411
Wang, J., Hu, X., and Jiang, H. (2016). ER stress-induced apoptosis: a novel therapeutic target in myocardial ischemia and reperfusion injury. Int. J. Cardiol. 214, 233–234. doi:10.1016/j.ijcard.2016.03.176
Wang, L., Liu, Y., Zhang, X., Ye, Y., Xiong, X., Zhang, S., et al. (2022). Endoplasmic reticulum stress and the unfolded protein response in cerebral ischemia/reperfusion injury. Front. Cell Neurosci. 16, 864426. doi:10.3389/fncel.2022.864426
Wang, L. Y. (2014). The Effect of Salvianic acid A on kidney ischemia-reperfusion injury in mice and its mechanism[D]. Chinese people's liberation army general hospital.
Wei, Z. Z., Chen, D., Liu, L. P., Gu, X., Zhong, W., Zhang, Y. B., et al. (2018). Enhanced neurogenesis and collaterogenesis by sodium danshensu treatment after focal cerebral ischemia in mice. Cell Transpl. 27 (4), 622–636. doi:10.1177/0963689718771889
Wu, L., Qiao, H., Li, Y., and Li, L. (2007). Protective roles of puerarin and Danshensu on acute ischemic myocardial injury in rats. Phytomedicine 14 (10), 652–658. doi:10.1016/j.phymed.2007.07.060
Wu, M. Y., Yiang, G. T., Liao, W. T., Tsai, A. P., Cheng, Y. L., Cheng, P. W., et al. (2018). Current mechanistic concepts in ischemia and reperfusion injury. Cell Physiol. Biochem. 46 (4), 1650–1667. doi:10.1159/000489241
Chen, Y., Wan, H., Zhou, H., Yu, L., He, Y., Li, C., Cheng, L., and Yang, J. (2018). Study on the antiinflammatory effect and mechanism of effective components of Salvia miltiorrhiza safflower on cerebral ischemia-reperfusion injury. Chinese Herbal Medicine 49 (16), 3875–3881. doi:10.7501/j.issn.0253-2670.2018.16.022
Xiang, M., Lu, Y., Xin, L., Gao, J., Shang, C., Jiang, Z., et al. (2021). Role of oxidative stress in reperfusion following myocardial ischemia and its treatments. Oxid. Med. Cell Longev. 2021, 6614009. doi:10.1155/2021/6614009
Xie, C., Luo, J., Hu, H., Wang, L., Yu, P., Xu, L., et al. (2021). A novel danshensu/tetramethypyrazine derivative attenuates oxidative stress-induced autophagy injury via the AMPK-mTOR-Ulk1 signaling pathway in cardiomyocytes. Exp. Ther. Med. 21 (2), 118. doi:10.3892/etm.2020.9550
Xing, Y., Sui, Z., Liu, Y., Wang, M. M., Wei, X., Lu, Q., et al. (2022). Blunting TRPML1 channels protects myocardial ischemia/reperfusion injury by restoring impaired cardiomyocyte autophagy. Basic Res. Cardiol. 117 (1), 20. doi:10.1007/s00395-022-00930-x
Xu, H., He, K., Li, Y., Tao, Y., Xu, C., Hu, Z., et al. (2020a). Cytoprotective effects evaluation of a novel danshensu derivative DEX-018 against oxidative stress injury in HUVECs. Biol. Pharm. Bull. 43 (5), 801–809. doi:10.1248/bpb.b19-00878
Xu, H., Liu, W. X., Liu, T. L., Guo, C., Feng, X., et al. (2017). Synergistic neuroprotective effects of Danshensu and hydroxysafflor yellow A on cerebral ischemia-reperfusion injury in rats. Oncotarget 8 (70), 115434–115443. doi:10.18632/oncotarget.23272
Xu, J., Wei, K., Zhang, G., Lei, L., Yang, D., Wang, W., et al. (2018). Ethnopharmacology, phytochemistry, and pharmacology of Chinese Salvia species: a review. J. Ethnopharmacol. 225, 18–30. doi:10.1016/j.jep.2018.06.029
Xu, S., Lu, J., Shao, A., Zhang, J. H., and Zhang, J. (2020b). Glial cells: role of the immune response in ischemic stroke. Front. Immunol. 11, 294. doi:10.3389/fimmu.2020.00294
Xu, X., Lai, Y., and Hua, Z. C. (2019). Apoptosis and apoptotic body: disease message and therapeutic target potentials. Biosci. Rep. 39 (1), BSR20180992. doi:10.1042/BSR20180992
Xu, Z., Ke, T., Zhang, Y., Guo, L., Chen, F., and He, W. (2021). Danshensu inhibits the IL-1β-induced inflammatory response in chondrocytes and osteoarthritis possibly via suppressing NF-κB signaling pathway. Mol. Med. 27 (1), 80. doi:10.1186/s10020-021-00329-9
Xue, Y., Zhang, L., Zhang, L., Sun, W., Fang, Z., Leng, Y., et al. (2022). Danshensu prevents thrombosis by inhibiting platelet activation via SIRT1/ROS/mtDNA pathways without increasing bleeding risk. Phytomedicine 104, 154271. doi:10.1016/j.phymed.2022.154271
Xv, S., Han, X., and Tian, W. (2018). Research progress on the role of calcium overload in the occurrence and development of myocardial ischemia-reperfusion injury. Med. Innovation China 15 (03), 146–148.
Yang, C., Hawkins, K. E., Doré, S., and Candelario-Jalil, E. (2019). Neuroinflammatory mechanisms of blood-brain barrier damage in ischemic stroke. Am. J. Physiol. Cell Physiol. 316 (2), C135-C153–C153. doi:10.1152/ajpcell.00136.2018
Yang, R. X., Huang, S. Y., Yan, F. F., Lu, X. T., Xing, Y. F., Liu, Y., et al. (2010). Danshensu protects vascular endothelia in a rat model of hyperhomocysteinemia. Acta Pharmacol. Sin. 31 (10), 1395–1400. doi:10.1038/aps.2010.167
Yang, Y., Xue, X., and Liu, Z. P. (2009). Protective effect of danshensu on focal cerebral ischemia-reperfusion injury in rats. Food Drug 11 (01), 24–26.
Yao, Y., Li, F., Zhang, M., Jin, L., Xie, P., Liu, D., et al. (2022). Targeting CaMKII-δ9 ameliorates cardiac ischemia/reperfusion injury by inhibiting myocardial inflammation. Circ. Res. 130 (6), 887–903. doi:10.1161/CIRCRESAHA.121.319478
Yellon, D. M., and Hausenloy, D. J. (2007). Myocardial reperfusion injury. N. Engl. J. Med. 357 (11), 1121–1135. doi:10.1056/NEJMra071667
Yin, Y., Duan, J. L., Guo, C., Wei, G., Wang, Y., Guan, Y., et al. (2017). Danshensu accelerates angiogenesis after myocardial infarction in rats and promotes the functions of endothelial progenitor cells through SDF-1α/CXCR4 axis. Eur. J. Pharmacol. 814, 274–282. doi:10.1016/j.ejphar.2017.08.035
Yin, Y., Guan, Y., Duan, J., Wei, G., Zhu, Y., Quan, W., et al. (2013). Cardioprotective effect of Danshensu against myocardial ischemia/reperfusion injury and inhibits apoptosis of H9c2 cardiomyocytes via Akt and ERK1/2 phosphorylation. Eur. J. Pharmacol. 699, 219–226. doi:10.1016/j.ejphar.2012.11.005
Yu, C., Dong, H., Wang, Q., Bai, J., Li, Y. N., Zhao, J. J., et al. (2021). Danshensu attenuates cisplatin-induced nephrotoxicity through activation of Nrf2 pathway and inhibition of NF-κB. Biomed. Pharmacother. 142, 111995. doi:10.1016/j.biopha.2021.111995
Yu, C., Qi, D., Lian, W., Li, Q. Z., Li, H. J., and Fan, H. Y. (2014). Effects of danshensu on platelet aggregation and thrombosis: in vivo arteriovenous shunt and venous thrombosis models in rats. PLoS One 9 (11), e110124. doi:10.1371/journal.pone.0110124
Yu, J. H., Wang, L. Y., Akinyi, M., Li, Y., Duan, Z., Zhu, Y., et al. (2015). Danshensu protects isolated heart against ischemia reperfusion injury through activation of Akt/ERK1/2/Nrf2 signaling. Int. J. Clin. Exp. Med. 8 (9), 14793–14804.
Yu, P. F., Wang, W. Y., Eerdun, G., Wang, T., Zhang, L. M., Li, C., et al. (2011). The role of P-glycoprotein in transport of Danshensu across the blood-brain barrier. Evid. Based Complement. Altern. Med. 2011, 713523. doi:10.1155/2011/713523
Yu, Q., Luo, Y., Sheng, L. X., Dong, Q., Dong, W., Guo, Y., et al. (2005). Study on effect of danshensu in directional differentiation of mesenchymal stem cells into neuron-like cells. Zhongguo Zhong Xi Yi Jie He Za Zhi 25 (1), 49–53. Chinese.
Yuan, H. J., Chen, Y. H., Ren, Y., Wen, K., Kang, Y., Jiao, J. J., et al. (2010). Study on protection of sodium salvianic acid A against cerebral ischemia/reperfusion injury in rats. Chin. J. Hosp. Pharm. 30 (18), 1545–1549.
Yuan, X., Fei, F., Sun, H., Xiao, C., Zhao, X., Zhang, Y., et al. (2018). Tanshinol borneol ester on nanostructured lipid carriers has longer brain and systemic effector retention and better antioxidant activity in vivo. Int. J. Nanomedicine 13, 2265–2274. doi:10.2147/IJN.S159789
Zhang, J., Zhang, Q., Liu, G., and Zhang, N. (2019). Therapeutic potentials and mechanisms of the Chinese traditional medicine Danshensu. Eur. J. Pharmacol. 864, 172710. doi:10.1016/j.ejphar.2019.172710
Zhang, L., Chang, B. B., Cao, W. W., Cao, Y., Yang, W. L., Liu, X. Q., et al. (2010). Effect of danshensu on myocardial energy metabolism in isolated ischemia-reperfusion rat hearts. J. China Pharm. Univ. 41 (03), 278–282.
Zhang, Y., Zhang, G., Liang, Y., Wang, H., Wang, Q., et al. (2020). Potential mechanisms underlying the hepatic-protective effects of danshensu on iron overload mice. Biol. Pharm. Bull. 43 (6), 968–975. doi:10.1248/bpb.b19-01084
Zhou, M., Yu, Y., Luo, X., Wang, J., Lan, X., Liu, P., et al. (2021). Myocardial ischemia-reperfusion injury: therapeutics from a mitochondria-centric perspective. Cardiology 146 (6), 781–792. doi:10.1159/000518879
Zhou, X., Cheung, C. M., Yang, J. M., Or, P. M., Lee, W. Y., and Yeung, J. H. (2015). Danshen (Salvia miltiorrhiza) water extract inhibits paracetamol-induced toxicity in primary rat hepatocytes via reducing CYP2E1 activity and oxidative stress. J. Pharm. Pharmacol. 67 (7), 980–989. doi:10.1111/jphp.12381
Keywords: Salvianic acid A, ischemia-reperfusion injury, multiple organs, mechanism of action, protective effects
Citation: Yang S, Chen H, Su W, Luo Y, Liao J, Wang Y, Xiong L, Zhang C, Li F, Chen Z-S and Wang T (2023) Protective effects of Salvianic acid A against multiple-organ ischemia-reperfusion injury: a review. Front. Pharmacol. 14:1297124. doi: 10.3389/fphar.2023.1297124
Received: 19 September 2023; Accepted: 06 November 2023;
Published: 27 November 2023.
Edited by:
Wei Zhao, Chengdu Medical College, ChinaReviewed by:
Shridhar Kulkarni, Exelixis Inc., United StatesHe-Hui Xie, Shanghai Jiao Tong University, China
Copyright © 2023 Yang, Chen, Su, Luo, Liao, Wang, Xiong, Zhang, Li, Chen and Wang. This is an open-access article distributed under the terms of the Creative Commons Attribution License (CC BY). The use, distribution or reproduction in other forums is permitted, provided the original author(s) and the copyright owner(s) are credited and that the original publication in this journal is cited, in accordance with accepted academic practice. No use, distribution or reproduction is permitted which does not comply with these terms.
*Correspondence: Fei Li, bGlmZWk4NzA2MDFAMTYzLmNvbQ==; Zhe-Sheng Chen, Y2hlbnpAc3Rqb2hucy5lZHU=; Tingfang Wang, d2FuZ3RmNjZAc2h1LmVkdS5jbg==
†These authors have contributed equally to this work