- 1Department of Pharmacognosy, State Key Laboratory of Natural Medicines, School of Traditional Chinese Pharmacy, China Pharmaceutical University, Nanjing, China
- 2Department of Pharmaceutical Chemistry and Pharmacognosy, School of Pharmacy, Eden University, Lusaka, Zambia
The Annonaceae is one of the plant families with members that are credited with numerous pharmacological functions. Among the group of compounds responsible for these bioactivities are the ent-kaurane diterpenoids. The ent-kauranes are a group of 20-Carbon, tetracyclic diterpenoids that are widely distributed in other plant families including the Annonaceae family. This mini-review focuses mainly on the ent-kaurane diterpenoids isolated from the Annonaceae family, delineates the various biological activities of these compounds, and highlights the research gaps that exist for further scientific scrutiny.
Introduction
A catalog of the Annonaceae family reveals that it comprises shrubs, climbers, aromatic trees and lianas that are almost ubiquitously distributed (i.e., found in almost all seven continents) (Al Kazman et al., 2022). This family is sometimes referred to as a “living fossil” due to the characteristic archaic and primitive features of member plants that have enabled their survival over the years (Attiq et al., 2017; Couvreur et al., 2022). This family comprises at least 120 genera and 2400 species widely distributed in four main subfamilies: Annonoideae, Anaxagoreoideae, Malmeoideae, and Ambavioideae (Attiq et al., 2017).
The classification of the Annonaceae family has undergone systematic evolution after the work of Dunal in 1817 (Attiq et al., 2017; Al Kazman et al., 2022). The work of Dunal was mainly based on the fruit morphology of member plants. Another system of classification was later established by Baillon (1868) and Diels and Alder (1932) on the basis of the floral characteristics of member plants (Attiq et al., 2017; Al Kazman et al., 2022). A more holistic approach that takes into consideration the floral characteristics and fruit morphology was propounded by Fries (1959) and currently serves as the gold standard for the classification of plants in this family (Attiq et al., 2017; Couvreur et al., 2022). Economically, members of this family have often served as a source of food and medicine for traditional uses. Notable members of this family include, Xylopia aethiopica, Xylopia parvifolia, Annona muricata, Annona reticulata, Uvaria grandi, Cananga odorata, Friesodielsia latifolia, Anaxagorea dolichocarpus, etc (Couvreur et al., 2022). Traditionally, these plants have been used for diverse therapeutic purposes such as, pain management and treatment of inflammation-related diseases (Almeida et al., 2012; Woode et al., 2012; Cercato et al., 2015). Phytochemical investigations have found a diversity in the bioactive compounds isolated from this family. The compounds range from alkaloids, flavonoids to acetogenins and ent-kauranes (Chan et al., 1993; Wu, 2006; Liaw et al., 2016; Zhao et al., 2022).
The ent-kauranes which are a group of structurally diverse tetracyclic compounds form an integral part of the bioactive compounds isolated from the Annonaceae family (Wu, 2006; Zhao et al., 2022). Structural diversity within the ent-kauranes is usually the result of changes to the parent skeleton such as bond cleavages, oxidation, intramolecular cyclization or structural rearrangements (Yang et al., 2002; Zhao et al., 2022). They are credited with biological functions including but not limited to antifungal, antibacterial, antitumor and anti-inflammatory activities (Wang et al., 2011; Zhao et al., 2022). This review seeks to throw light on the ent-kauranes diterpenoids with the view to directing the attention of researchers on the need for further research on this group of compounds. The content of this review will include thematic areas such as the biosynthesis, chemistry, and bioactivities of the ent-kaurane diterpenoids and call for further research.
Methodology
Relevant published literature was retrieved from various databases such as Web of Science, Pubmed, google scholar, Elsevier, ACS using the following key words, singly or as combinations: ent-kaurane diterpenoids; Annonaceae; biosynthesis; biological activities. Publications on ent-kaurane diterpenoids from other plant families aside from Annonaceae were excluded. Only relevant publications in the English language were used. Publications in other languages such as Chinese (Mandarin) were also excluded. On the basis of this criterion and relevance to the topic, the articles were scaled down from a total of about 6,342 articles to 98. A flow chart of the methodology used is summarized in Supplementary Figure S1.
Biosynthesis of the ent-kaurane diterpenoids
The term “ent” which stands for “enantiomeric” traces its roots to the earliest identified diterpene from the leaf oil of Agathis, a plant locally known in New Zealand as Kauri pine (Zhao et al., 2022). Due to its negative optical rotation, it was subsequently named “ent-kaurene”. The ent-kauranes, a group of 20-Carbon, tetracyclic diterpenoids are widely distributed in other plant families including the Asteraceae, Lamiaceae, Compositae, Euphorbiaceae, Pteridaceae families aside from the Annonaceae family (Aplin et al., 1963). They are generally accepted as intermediates in the biogenesis of growth hormones in the gibberellin plant (Yamaguchi, 2008; Hedden, 2020). Various strategies have been devised to synthetically produce some ent-kaurane diterpenoids as summarized by Zhao et al. (2022). However, in the parent plants, the ent-kauranes diterpenoids are biosynthesized from geranylgeranyl pyrophosphate (GGPP), a universally accepted precursor for diterpenes. Under the enzymatic action of copalyl diphosphate synthase, CPS (also called kaurene synthase A), GGPP is converted either to ent-copalyl diphosphate (ent-CPP) or syn-CPP based on the specificity of the enzyme (García et al., 2007). The action of ent-CPP synthase produces ent-CPP as the intermediate which is then converted in a series of steps to ent-kaurene by ent-kaurene synthase or kaurene synthase B. Mechanistically, ent-CPP undergoes a cascade of cyclization to produce a tricyclic intermediate PA that possesses a tertiary carbocation at C-8. The saturation at C-14 intramolecularly seeks this carbocation, the result of which generates a tetracyclic beyeranyl-13-cation intermediate, PB (i.e., carbocation is located at C-13). A more stable form of this intermediate (tertiary carbocation) is produced after a 1,2-alkyl migration to generate the ent-kaurenyl-16-cation PE. Alternatively, the tertiary carbocation PE can be directly formed from PA through the joint and coordinated cyclization and alkyl shift processes in a bid to avoid the formation of the less stable secondary carbocations (García et al., 2007; Zhao et al., 2022). Ent-kaurene is finally generated after proton removal from the tertiary carbocation thereby producing the required exocyclic alkene. Various chemical modifications of the parent ent-kaurene carboskeleton such as, C-C bond cleavage, oxidation or structural rearrangements result in the productions of different diterpenoids. For instance, the ent-kaurane carboskeleton is generated when the unsaturation at C-16 and C-17 is lost. These processes have been schematically summarized in Figure 1A.
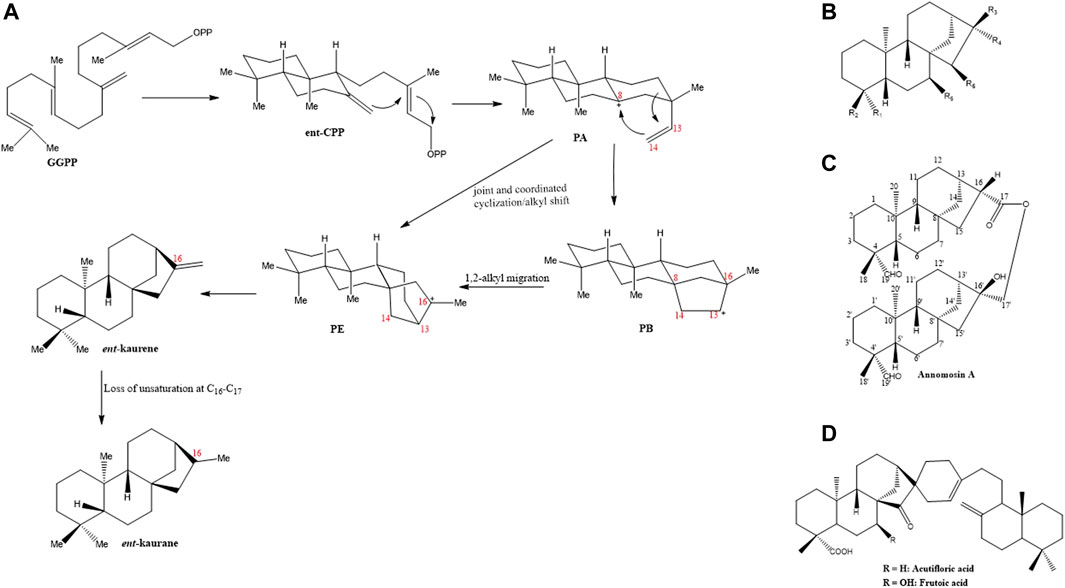
FIGURE 1. (A) Schematic outline of the biosynthetic pathway of ent-kaurene and ent-kaurane (adopted from Zhao et al., 2022). (B) Basic skeleton of the ent-kaurane diterpenoids (C) Chemical structure of dimeric ent-kaurane diterpenoid, Annomosin A (D). Basic skeleton of acutifloric acid and frutoic acid, two dimeric ent-kaurane diterpenoids.
Ent-kaurane diterpenoids isolated from the Annonaceae family
On the basis of their structural characteristics, the ent-kaurane diterpenoids in general can be categorized into the seco-ent-kauranoids, the C-20 oxygenated ent-kauranoids, the C-20 non-oxygenated ent-kauranoids, the nor- or rearranged-ent-kauranoids and grayanes (Sun et al., 2006; Liu et al., 2017). For research on the isolation and structural elucidations of ent-kaurane diterpenoids from plants within the Annonaceae family, the group of Prof. Yang-Chang Wu have contributed enormously. Their series of works on the Formosan Annonaceous plants deserve commendation (Wu, 2006). On the whole, at least 70 ent-kaurane diterpenoids have been isolated and structurally characterized from plants belonging to the Annonaceae family (Eshiet et al., 1971; Etse et al., 1987; Wu, 2006; Nhiem et al., 2015). Table 1 summarizes some of the ent-kaurane diterpenoids and the plants from which they were isolated, Figure 1B illustrates their basic skeletal structure while the chemical structures of all compounds tabulated (Table 1) are shown in Supplementary Figure S2. These compounds range from simple ent-kaurane/ent-kaurene diterpenes and derivatives of same to dimeric diterpenoids. Most of the compounds were isolated from various Annona (Wu et al., 1996; Chang et al., 1998; Chen et al., 1998; Chen et al., 2000; Yang et al., 2002) and Xylopia species (Hasan et al., 1982; 1985; Lajide et al., 1995; Takahashi et al., 1995; Désiré et al., 2013). Of all the compounds highlighted, annomosin A (16β-hydroxy-19-al-ent-kauran-17-yl-16β-hydro-19-al-ent-kauran-17-oate) is dimeric in nature, the first of its kind reported in the Annonaceae family (Wu, 2006). It is composed of two ent-kaurane monomeric units, thus, 19-al-ent-kauran-17-oic acid and 16,17-dihydroxy-ent-kauran-19-al. Other dimeric ent-kaurane diterpenoids which were isolated from the Xylopia acutiflora specie, thus, acutifloric acid and frutoic acid, are composed of a labdane monomer and an ent-kaurane monomer (Hasan et al., 1985; Takahashi et al., 1995) (Figures 1C, D).
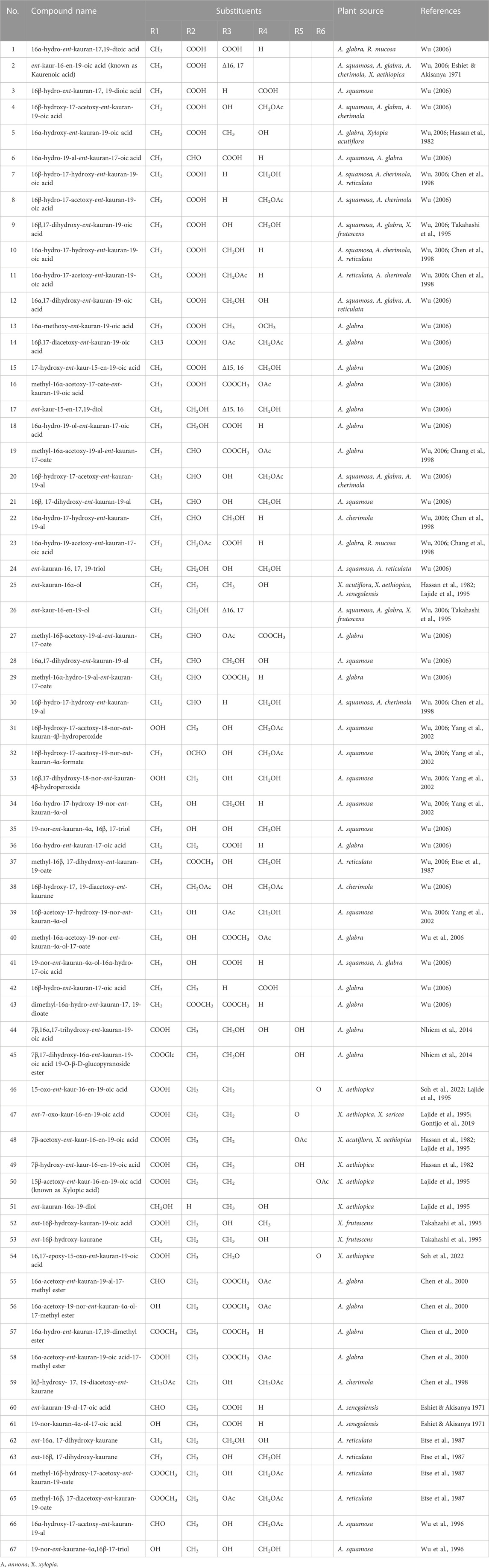
TABLE 1. List of non-dimeric ent-kaurane diterpenoids isolated from plants in the Annonaceae family.
Biological activities
In spite of the fact that a lot of the ent-kaurane diterpenoids have been isolated and reported in literature, not much biological evaluations have been done on them. The few compounds that have been assessed are reported to possess a vast array of biological activities including anti-inflammatory (Yeh et al., 2005; Nhiem et al., 2015), antimicrobial (Boakye-Yiadom et al., 1977), anti-HIV (Wu et al., 1996; Chang et al., 1998), anticancer (Fatope et al., 1996), termite antifeedant (Lajide et al., 1995), hypotensive and coronary vasodilatory (Somova et al., 2001) and anti-platelet aggregation (Yang et al., 2002) effects. Only two of these compounds, thus, kaurenoic acid, KA (Supplementary Figure S3A) and xylopic acid, XA (Supplementary Figure S3B) have received a considerable amount of biological scrutiny by many a researcher. A summary of the biological activities of these two compounds is therefore highlighted herein.
Kaurenoic acid (KA)
KA (ent-kaur-16-en-19-oic acid) has been credited with a plethora of biological activities. It has been reported to attenuate inflammatory processes via diverse mechanisms. Its anti-inflammatory effects have been partly attributed to its ability to activate the transcription factor, nuclear factor erythroid 2-related factor 2, Nrf2 (Paiva et al., 2002; Lyu et al., 2011; Kim et al., 2016), downregulate Th2 and NF-κB/cytokine-related pathways (Borghi et al., 2022) and the transforming growth factor-β (TGF-β) signaling (Kim et al., 2017). It was also reported to dose-dependently inhibit prostaglandin E2 release, nitric oxide (NO) production, inducible nitric oxide synthase (iNOS) and cyclooxygenase-2 (COX-2) expressions (Choi et al., 2011). KA has also been reported to exhibit antinociception in various pain models (Dalenogare et al., 2019; Montiel-Ruiz et al., 2020; Zaninelli et al., 2023). Its analgesic effect has been linked to underlying mechanisms such as the inhibition of cytokine production and NO-cyclic GMP-protein kinase G-ATP-sensitive potassium channel signaling pathway activation (Mizokami et al., 2012).
The potential of KA against diverse microbes has been reported. Together with five of its derivatives, de Andrade et al. (2011) assessed its anticariogenic activity and reported its bactericidal effect against Streptococcus mutans, the primary causative agent of dental caries (Moreira et al., 2016; Moon et al., 2022). Martins et al., who investigated 12-kaurane-type diterpenes for their antibacterial effects against a group of bacteria that cause endodontic infections reported satisfactory activities for KA and its salt (Martins et al., 2018). On the basis of their proteomic data, they inferred that the possible mechanisms that underlie these antibacterial effects could be due to the ability of KA and its salt to hamper bacterial metabolism and virulence factor expression (Martins et al., 2018). KA has been found to be effective against other Gram-positive bacteria such as Bacillus cereus (Wilkens et al., 2002), and Staphylococcus aureus (Okoye et al., 2012; Pereira et al., 2012; Arciniegas et al., 2018). Additionally, KA was found to demonstrate good antifungal activity against Epidermophyton floccosum, Trichophyton rubrum and Trichophyton mentagrophytes (Sartori et al., 2003).
In the search for new and effective anticancer drug leads, the issues of genotoxicity and mutagenicity are of grave concern. To this end, KA was assessed for its possible genotoxic and mutagenic effects using established in vitro and in vivo models (Cavalcanti et al., 2006; Cavalcanti et al., 2010). It was found to exhibit genotoxic and mutagenic effects in human peripheral blood leukocytes, Chinese hamster lung fibroblast (V79) cells, Saccharomyces cerevisiae (baker’s or brewer’s yeast), and mice (Cavalcanti et al., 2006; Cavalcanti et al., 2010). These effects were presumed to be probably the result of either DNA-strand breaks or topoisomerase I inhibition or both (Cavalcanti et al., 2010). It was suggested that the double bond at the C-16 moiety might be active site responsible for the genotoxicity of KA (Cavalcanti et al., 2010). Alongside thirteen other natural isolates, KA was found to exhibit considerable antiproliferative effects in five cell lines, HeLa, A-549, Hep-2, PC-3, and MCF-7 cells in a dose-dependent manner (Cuca et al., 2011). Alves  et al. (2023) in their bid to circumvent the hydrophobicity and thermosensitivity challenges of KA, prepared complexes of ent-kaurenoic acid-enriched Mikania glomerata leaves extract with β-cyclodextrin and assessed the antitumor activity of this formulation in rodents. The formulation displayed low systemic toxicity in mice and its antitumor activity was ascribed to its ability to inhibit LDH activity and NF-κB signaling pathway (Alves  et al., 2023). Antitumor activities have also been reported for microbial-derived KA derivatives against the breast cancer cell lines, MCF-7 (da Costa et al., 2018) and 4 T1 (Ferreira et al., 2022), the human glioblastoma cell line, U87 (Lizarte Neto et al., 2013) and other cell lines (Dutra et al., 2014).
Other reported biological activities of KA include hepatoprotective (Marcondes-Alves et al., 2019), leishmanicidal (Miranda et al., 2015), smooth muscle relaxant (de Alencar Cunha et al., 2003), trypanocidal (Kian et al., 2018), vasorelaxant (Tirapelli et al., 2004), anticonvulsant effect (Okoye et al., 2013), and hypoglycemic (Raga et al., 2010) effects among others.
Xylopic acid (XA)
A perusal of extant scientific literature on XA (15β-acetoxy-ent-kaur-16-en-19-oic acid) reveals reports on the pharmacokinetics and in vitro microsomal liver enzyme metabolism (Alolga, et al., 2023a), forced degradation studies (Alolga, et al., 2023b), quantitative analyses (Adosraku & Oppong Kyekyeku, 2011; Kyekyeku et al., 2020), semi-synthesis (Soh et al., 2022) and evaluations of diverse biological activities. The anti-inflammatory potential of XA has been assessed using various animal models and found to be effective against acute and chronic inflammation (Osafo et al., 2018; Osafo et al., 2019). For instance, Osafo et al. (2018) found XA to be effective against acute inflammation and this effect was due to its ability to modulate the effects of pro-inflammatory markers, prostaglandin E2, serotonin, histamine, and bradykinin. The possible mechanisms that underlie the anti-inflammatory effect of XA according to Boakye et al. (2022) could be due to its ability to regulate the activities of Nrf2 and NF-κB together with increase in HO-1 expression and reduction in VCAM-1 expression. Against chronic inflammatory conditions such as rheumatoid arthritis (RA), XA was found to ameliorate the inflammatory states of adjuvant-induced arthritic rats via reduction of pro-inflammatory cytokines (IL-6 and TNF-α) levels (Osafo et al., 2022). As the principal constituent of a bioinspired reconstituted high-density lipoprotein (rHDL) nanoparticles, the anti-RA potential of XA was assessed via the lens of metabolomics and transcriptomics (Alolga et al., 2021). The anti-RA activity of the rHDL/XA nanoparticles was due mainly to the restoration of perturbed metabolic pathways, thus, amino acids and lipids metabolism (Alolga et al., 2021).
Other bioactivities reported for XA include antimalarial (Boampong et al., 2013; Ameyaw et al., 2018; Osei et al., 2021), antimicrobial (Boakye-Yiadom et al., 1977), antinociceptive (Woode et al., 2015), analgesic (Woode et al., 2013; Ameyaw et al., 2014; Woode et al., 2016), antiproliferative (Soh et al., 2022), antidepressant-like (Biney et al., 2021), and cardiovascular and diuretic (Somova et al., 2001) effects.
Call for further research
The ultimate objective of scientists interested in bioprospection for lead compounds has always been to discover new and more effective drugs for the numerous diseases that have plagued humanity. However, the journey from translation of laboratory findings to clinical application is a herculean task. It involves years of preclinical studies, much of the outcome of which usually fails even before clinical trials. As far as the ent-kaurane diterpenoids are concerned, there is dearth of research on their bioactivities. Much of the research done has been to isolate and structurally elucidate these compounds from their respective plant sources. Isolation and structural elucidation of compounds is merely the first step to a long and winding journey towards possible clinical use. With the advent of computer-simulated combinatorial chemistry and high-throughput screening techniques, there is need for more attention to be devoted to research on the bioactivities of the ent-kaurane diterpenoids. These techniques in combination with established in vitro and in vivo models would aid in the discovery of lead compounds for probable clinical trials. In-depth investigations of the most active compounds would elucidate their exact molecular mechanisms of actions, pharmacokinetic and toxicological profiles. Further research on the most active compounds would also identify and resolve bioavailability and formulation challenges prior to clinical trials. The possible medical solutions to inflammation-related chronic diseases such as diabetes, RA, ulcers, cancers, and even age-long diseases such as HIV/AIDs and malaria, could lie in the ent-kaurane diterpenoids based on the results of available preliminary investigations.
Conclusion
This mini-review provides to a large extent a summary of research progress on the ent-kaurane diterpenoids isolated from various plants in the Annonaceae family, highlights the reported biological activities of these compounds and proffers suggestions for future research on same. In summary, the ent-kaurane diterpenoids are a group of compounds with a probably huge potential as good drug leads but have not had much attention from the scientific community. Available data on preliminary studies conducted on these compounds have credited them with diverse pharmacological properties including but not limited to antimicrobial, anti-inflammatory, anti-HIV, leishmanicidal, trypanocidal, and antimalarial effects among a host of others. There is however a need for further research so as to fully tap into the potential medical benefits of these compounds.
Author contributions
TI and PK collected the relevant information and wrote the draft of the manuscript. MM and RA conceived the study, supervised, and revised the manuscript. RA received funding for the study. All authors contributed to the article and approved the submitted version.
Funding
This study forms part of an ongoing study funded by the National Natural Science Foundation of China (Research Fund for Young International Scientists, No: 82150410450).
Conflict of interest
The authors declare that the research was conducted in the absence of any commercial or financial relationships that could be construed as a potential conflict of interest.
Publisher’s note
All claims expressed in this article are solely those of the authors and do not necessarily represent those of their affiliated organizations, or those of the publisher, the editors and the reviewers. Any product that may be evaluated in this article, or claim that may be made by its manufacturer, is not guaranteed or endorsed by the publisher.
Supplementary material
The Supplementary Material for this article can be found online at: https://www.frontiersin.org/articles/10.3389/fphar.2023.1227574/full#supplementary-material
References
Adosraku, R., and Oppong Kyekyeku, J. (2011). Characterisation and HPLC quantification of xylopic acid in the dried fruits of xylopia aethiopica. Int. J. Pure Appl. Chem. 6 (2), 13–14.
Al Kazman, B. S. M., Harnett, J. E., and Hanrahan, J. R. (2022). Traditional uses, phytochemistry and pharmacological activities of annonacae. Molecules 27 (11), 3462. doi:10.3390/molecules27113462
Almeida, J. R., Araújo, E. C., Ribeiro, L. A., de Lima, J. T., Nunes, X. P., Lúcio, A. S., et al. (2012). Antinociceptive activity of ethanol extract from Duguetia chrysocarpa Maas (Annonaceae). ScientificWorldJournal 2012, 859210. doi:10.1100/2012/859210
Alolga, R. N., Ayensu, I., and Sosu, J. X. (2023a). Forced degradation studies, elucidation of degradation pathways and degradation kinetics of Xylopic acid via LC and LC-MS/MS analyses. J. Pharm. Sci. doi:10.1016/j.xphs.2023.01.004
Alolga, R. N., Opoku-Damoah, Y., Alagpulinsa, D. A., Huang, F. Q., Ma, G., Chavez Leon, M., et al. (2021). Metabolomic and transcriptomic analyses of the anti-rheumatoid arthritis potential of xylopic acid in a bioinspired lipoprotein nanoformulation. Biomaterials 268, 120482. doi:10.1016/j.biomaterials.2020.120482
Alolga, R. N., Wang, S. L., Ayensu, I., and Nebeolisa, C. S. (2023b). Pharmacokinetics and in vitro liver microsomal enzyme metabolism of Xylopic acid. J. Pharm. Biomed. Anal. 224, 115200. doi:10.1016/j.jpba.2022.115200
Alves Â, V. F., Melo, C. R., Chagas-Neto, J. L., Amaral, R. G., Ambrósio, S. R., Moreira, M. R., et al. (2023). Ent-kaurenoic acid-enriched Mikania glomerata leaves-complexed β-cyclodextrin: Pharmaceutical development and in vivo antitumor activity in a sarcoma 180 mouse model. Int. J. Pharm. 631, 122497. doi:10.1016/j.ijpharm.2022.122497
Ameyaw, E. O., Asmah, K. B., Biney, R. P., Henneh, I. T., Owusu-Agyei, P., Prah, J., et al. (2018). Isobolographic analysis of co-administration of two plant-derived antiplasmodial drug candidates, cryptolepine and xylopic acid, in Plasmodium berghei. Malar. J. 17 (1), 153. doi:10.1186/s12936-018-2283-8
Ameyaw, E. O., Woode, E., Boakye-Gyasi, E., Abotsi, W. K., Kyekyeku, J. O., and Adosraku, R. K. (2014). Anti-allodynic and Anti-hyperalgesic effects of an ethanolic extract and xylopic acid from the fruits of Xylopia aethiopica in murine models of neuropathic pain. Pharmacogn. Res. 6 (2), 172–179. doi:10.4103/0974-8490.129041
Aplin, R. T., Cambie, R. C., and Rutledge, P. S. (1963). The taxonomic distribution of some diterpene hydrocarbons. Phytochemistry 2 (3), 205–214. doi:10.1016/S0031-9422(00)85105-5
Arciniegas, A., Pérez-Castorena, A. L., Meléndez-Aguirre, M., Ávila, J. G., García-Bores, A. M., Villaseñor, J. L., et al. (2018). Chemical composition and antimicrobial activity of Ageratina deltoidea. Chem. Biodivers. 15 (3), e1700529. doi:10.1002/cbdv.201700529
Attiq, A., Jalil, J., and Husain, K. (2017). Annonaceae: Breaking the wall of inflammation. Front. Pharmacol. 8, 752. doi:10.3389/fphar.2017.00752
Biney, R. P., Benneh, C. K., Adongo, D. W., Ameyaw, E. O., and Woode, E. (2021). Evidence of an antidepressant-like effect of xylopic acid mediated by serotonergic mechanisms. Psychopharmacol. Berl. 238 (8), 2105–2120. doi:10.1007/s00213-021-05835-6
Boakye, Y. D., Osafo, N., Oppong-Kyekyeku, J., Abotsi, W. K. M., Boakye-Gyasi, E., Heiss, E., et al. (2022). Regulation of Nrf2 and NF-κB activities may contribute to the anti-inflammatory mechanism of xylopic acid. Inflammopharmacology 30 (5), 1835–1841. doi:10.1007/s10787-022-00950-y
Boakye-Yiadom, K., Fiagbe, N. I., and Ayim, J. S. (1977). Antimicrobial properties of some West African medicinal plants iv. Antimicrobial activity of xylopic acid and other constituente of the fruits of Xylopia aethiopica (Annonaceae). Lloydia 40 (6), 543–545.
Boampong, J. N., Ameyaw, E. O., Aboagye, B., Asare, K., Kyei, S., Donfack, J. H., et al. (2013). The curative and prophylactic effects of xylopic acid on plasmodium berghei infection in mice. J. Parasitol. Res. 2013, 356107. doi:10.1155/2013/356107
Borghi, S. M., Domiciano, T. P., Rasquel-Oliveira, F. S., Ferraz, C. R., Bussmann, A. J. C., Vignoli, J. A., et al. (2022). Sphagneticola trilobata (L) Pruski-derived kaurenoic acid prevents ovalbumin-induced asthma in mice: Effect on Th2 cytokines, STAT6/GATA-3 signaling, NFκB/Nrf2 redox sensitive pathways, and regulatory T cell phenotype markers. J. Ethnopharmacol. 283, 114708. doi:10.1016/j.jep.2021.114708
Cavalcanti, B. C., Costa-Lotufo, L. V., Moraes, M. O., Burbano, R. R., Silveira, E. R., Cunha, K. M., et al. (2006). Genotoxicity evaluation of kaurenoic acid, a bioactive diterpenoid present in Copaiba oil. Food Chem. Toxicol. 44 (3), 388–392. doi:10.1016/j.fct.2005.08.011
Cavalcanti, B. C., Ferreira, J. R., Moura, D. J., Rosa, R. M., Furtado, G. V., Burbano, R. R., et al. (2010). Structure-mutagenicity relationship of kaurenoic acid from Xylopia sericeae (Annonaceae). Mutat. Res. 701 (2), 153–163. doi:10.1016/j.mrgentox.2010.06.010
Cercato, L. M., White, P. A., Nampo, F. K., Santos, M. R., and Camargo, E. A. (2015). A systematic review of medicinal plants used for weight loss in Brazil: Is there potential for obesity treatment? J. Ethnopharmacol. 176, 286–296. doi:10.1016/j.jep.2015.10.038
Chan, F.-R., Wu, Y.-C., Duh, C.-Y., and Wang, S.-K. (1993). Studies on the acetogenins of formosan annonaceous plants, II. Cytotoxic acetogenins from Annona reticulata. J. Nat. Prod. 56 (10), 1688–1694. doi:10.1021/np50100a005
Chang, F. R., Yang, P. Y., Lin, J. Y., Lee, K. H., and Wu, Y. C. (1998). Bioactive kaurane diterpenoids from Annona glabra. J. Nat. Prod. 61 (4), 437–439. doi:10.1021/np970497z
Chen, C.-Y., Wu, T.-Y., Chang, F.-R., and Wu, Y.-C. (1998). Lignans and kauranes from the stems of Annona cherimola. J. Chin. Chem. Soc. 45 (5), 629–634. doi:10.1002/jccs.199800095
Chen, C. Y., Chang, F. R., Cho, C. P., and Wu, Y. C. (2000). ent-kaurane diterpenoids from Annona glabra. J. Nat. Prod. 63 (7), 1000–1003. doi:10.1021/np0000320
Choi, R. J., Shin, E. M., Jung, H. A., Choi, J. S., and Kim, Y. S. (2011). Inhibitory effects of kaurenoic acid from Aralia continentalis on LPS-induced inflammatory response in RAW264.7 macrophages. Phytomedicine 18 (8-9), 677–682. doi:10.1016/j.phymed.2010.11.010
Couvreur, T. L. P., Dagallier, L. M. J., Crozier, F., Ghogue, J. P., Hoekstra, P. H., Kamdem, N. G., et al. (2022). Flora of Cameroon - Annonaceae vol 45. PhytoKeys 207, 1–532. doi:10.3897/phytokeys.207.61432
Cuca, L. E., Coy, E. D., Alarcón, M. A., Fernández, A., and Aristizábal, F. A. (2011). Cytotoxic effect of some natural compounds isolated from Lauraceae plants and synthetic derivatives. Biomedica 31 (3), 335–343. doi:10.1590/s0120-41572011000300006
da Costa, R. M., Bastos, J. K., Costa, M. C. A., Ferreira, M. M. C., Mizuno, C. S., Caramori, G. F., et al. (2018). In vitro cytotoxicity and structure-activity relationship approaches of ent-kaurenoic acid derivatives against human breast carcinoma cell line. Phytochemistry 156, 214–223. doi:10.1016/j.phytochem.2018.10.005
Dalenogare, D. P., Ferro, P. R., De Prá, S. D. T., Rigo, F. K., de David Antoniazzi, C. T., de Almeida, A. S., et al. (2019). Antinociceptive activity of Copaifera officinalis Jacq. L oil and kaurenoic acid in mice. Inflammopharmacology 27 (4), 829–844. doi:10.1007/s10787-019-00588-3
de Alencar Cunha, K. M., Paiva, L. A., Santos, F. A., Gramosa, N. V., Silveira, E. R., and Rao, V. S. (2003). Smooth muscle relaxant effect of kaurenoic acid, a diterpene from Copaifera langsdorffii on rat uterus in vitro. Phytother. Res. 17 (4), 320–324. doi:10.1002/ptr.1133
de Andrade, B. B., Moreira, M. R., Ambrosio, S. R., Furtado, N. A., Cunha, W. R., Heleno, V. C., et al. (2011). Evaluation of ent-kaurenoic acid derivatives for their anticariogenic activity. Nat. Prod. Commun. 6 (6), 1934578X1100600–780. doi:10.1177/1934578x1100600608
Désiré, S., Nkwengoua, E., Ngantchou, I., Nyasse, B., Denier, C., Hannaert, V., et al. (2013). Xylopioxyde and other bioactive kaurane-diterpenes from Xylopia aethiopica Dunal (Annonaceae). J. Appl. Pharm. Sci. 3 (12), 013–019. doi:10.7324/JAPS.2013.31203
Dutra, L. M., Bomfim, L. M., Rocha, S. L., Nepel, A., Soares, M. B., Barison, A., et al. (2014). ent-Kaurane diterpenes from the stem bark of Annona vepretorum (Annonaceae) and cytotoxic evaluation. Bioorg Med. Chem. Lett. 24 (15), 3315–3320. doi:10.1016/j.bmcl.2014.06.005
Eshiet, I. T. U., Akisanya, A., and Taylor, D. A. H. (1971). Diterpenes from Annona senegalensis. Phytochemistry 10 (12), 3294–3295. doi:10.1016/S0031-9422(00)97396-5
Etse, J. T., Gray, A. I., and Waterman, P. G. (1987). Chemistry in the Annonaceae, XXIV. Kaurane and kaur-16-ene diterpenes from the stem bark of Annona reticulata. J. Nat. Prod. 50 (5), 979–983. doi:10.1021/np50053a043
Fatope, M. O., Audu, O. T., Takeda, Y., Zeng, L., Shi, G., Shimada, H., et al. (1996). Bioactive ent-kaurene diterpenoids from Annona senegalensis. J. Nat. Prod. 59 (3), 301–303. doi:10.1021/np9601566
Ferreira, K. C. B., Valle, A., Gualberto, A. C. M., Aleixo, D. T., Silva, L. M., Santos, M. M., et al. (2022). Kaurenoic acid nanocarriers regulates cytokine production and inhibit breast cancer cell migration. J. Control Release 352, 712–725. doi:10.1016/j.jconrel.2022.10.048
García, P. A., de Oliveira, A. B., and Batista, R. (2007). Occurrence, biological activities and synthesis of kaurane diterpenes and their glycosides. Molecules 12 (3), 455–483. doi:10.3390/12030455
Hasan, C. M., Healey, T. M., and Waterman, P. G. (1985). Acutifloric acid: A diterpene dimer from the stem bark of xylopia acutiflora. Phytochemistry 24 (1), 192–194. doi:10.1016/S0031-9422(00)80841-9
Hasan, C. M., Healey, T. M., and Waterman, P. G. (1982). Kaurane and kaurene diterpenes from the stem bark of Xylopia acutiflora. Phytochemistry 21 (8), 2134–2135. doi:10.1016/0031-9422(82)83068-9
Hedden, P. (2020). The current status of research on gibberellin biosynthesis. Plant Cell Physiol. 61 (11), 1832–1849. doi:10.1093/pcp/pcaa092
Kian, D., Lancheros, C. A. C., Assolini, J. P., Arakawa, N. S., Veiga-Júnior, V. F., Nakamura, C. V., et al. (2018). Trypanocidal activity of copaiba oil and kaurenoic acid does not depend on macrophage killing machinery. Biomed. Pharmacother. 103, 1294–1301. doi:10.1016/j.biopha.2018.04.164
Kim, K. H., Han, J. W., Jung, S. K., Park, B. J., Han, C. W., and Joo, M. (2017). Kaurenoic acid activates TGF-β signaling. Phytomedicine 32, 8–14. doi:10.1016/j.phymed.2017.04.008
Kim, K. H., Sadikot, R. T., and Joo, M. (2016). Therapeutic effect of ent-kaur-16-en-19-oic acid on neutrophilic lung inflammation and sepsis is mediated by Nrf2. Biochem. Biophys. Res. Commun. 474 (3), 534–540. doi:10.1016/j.bbrc.2016.04.122
Kyekyeku, J. O., Asare-Nkansah, S., Bekoe, S. O., Sezgin, S., Adosraku, R. K., and Spiteller, M. (2020). MALDI-HRMS imaging and HPLC-HRESI-MS(n) characterisation of kaurane diterpenes in the fruits of Xylopia aethiopica (Dunal) A. Rich (Annonaceae). Phytochem. Anal. 31 (3), 349–354. doi:10.1002/pca.2901
Lajide, L., Escoubas, P., and Mizutani, J. (1995). Termite antifeedant activity in Xylopia aethiopica. Phytochemistry 40 (4), 1105–1112. doi:10.1016/0031-9422(95)92653-P
Liaw, C. C., Liou, J. R., Wu, T. Y., Chang, F. R., and Wu, Y. C. (2016). Acetogenins from Annonaceae. Prog. Chem. Org. Nat. Prod. 101, 113–230. doi:10.1007/978-3-319-22692-7_2
Liu, M., Wang, W. G., Sun, H. D., and Pu, J. X. (2017). Diterpenoids from isodon species: An update. Nat. Prod. Rep. 34 (9), 1090–1140. doi:10.1039/c7np00027h
Lizarte Neto, F. S., Tirapelli, D. P., Ambrosio, S. R., Tirapelli, C. R., Oliveira, F. M., Novais, P. C., et al. (2013). Kaurene diterpene induces apoptosis in U87 human malignant glioblastoma cells by suppression of anti-apoptotic signals and activation of cysteine proteases. Braz J. Med. Biol. Res. 46 (1), 71–78. doi:10.1590/1414-431x20121423
Lyu, J. H., Lee, G. S., Kim, K. H., Kim, H. W., Cho, S. I., Jeong, S. I., et al. (2011). ent-kaur-16-en-19-oic Acid, isolated from the roots of Aralia continentalis, induces activation of Nrf2. J. Ethnopharmacol. 137 (3), 1442–1449. doi:10.1016/j.jep.2011.08.024
Marcondes-Alves, L., Fattori, V., Borghi, S. M., Lourenco-Gonzalez, Y., Bussmann, A. J. C., Hirooka, E. Y., et al. (2019). Kaurenoic acid extracted from Sphagneticola trilobata reduces acetaminophen-induced hepatotoxicity through inhibition of oxidative stress and pro-inflammatory cytokine production in mice. Nat. Prod. Res. 33 (6), 921–924. doi:10.1080/14786419.2017.1416372
Martins, C. H., Abrão, F., Moraes, T. S., Oliveira, P. F., Tavares, D. C., Magalhães, L. G., et al. (2018). Kaurenoic acid and its sodium salt derivative: Antibacterial activity against porphyromonas gingivalis and their mechanism of action. Future Microbiol. 13, 1585–1601. doi:10.2217/fmb-2018-0140
Miranda, M. M., Panis, C., da Silva, S. S., Macri, J. A., Kawakami, N. Y., Hayashida, T. H., et al. (2015). Kaurenoic acid possesses leishmanicidal activity by triggering a NLRP12/IL-1β/cNOS/NO pathway. Mediat. Inflamm. 2015, 392918. doi:10.1155/2015/392918
Mizokami, S. S., Arakawa, N. S., Ambrosio, S. R., Zarpelon, A. C., Casagrande, R., Cunha, T. M., et al. (2012). Kaurenoic acid from sphagneticola trilobata inhibits inflammatory pain: Effect on cytokine production and activation of the NO-cyclic GMP-protein kinase G-ATP-sensitive potassium channel signaling pathway. J. Nat. Prod. 75 (5), 896–904. doi:10.1021/np200989t
Montiel-Ruiz, R. M., Córdova-de la Cruz, M., González-Cortázar, M., Zamilpa, A., Gómez-Rivera, A., López-Rodríguez, R., et al. (2020). Antinociceptive effect of hinokinin and kaurenoic acid isolated from aristolochia odoratissima L. Molecules 25 (6), 1454. doi:10.3390/molecules25061454
Moon, K., Hwang, S., Lee, H. J., Jo, E., Kim, J. N., and Cha, J. (2022). Identification of the antibacterial action mechanism of diterpenoids through transcriptome profiling. Front. Microbiol. 13, 945023. doi:10.3389/fmicb.2022.945023
Moreira, M. R., Souza, A. B., Soares, S., Bianchi, T. C., de Souza Eugênio, D., Lemes, D. C., et al. (2016). ent-Kaurenoic acid-rich extract from Mikania glomerata: In vitro activity against bacteria responsible for dental caries. Fitoterapia 112, 211–216. doi:10.1016/j.fitote.2016.06.007
Nhiem, N. X., Hien, N. T., Tai, B. H., Anh Hle, T., Hang, D. T., Quang, T. H., et al. (2015). New ent-kauranes from the fruits of Annona glabra and their inhibitory nitric oxide production in LPS-stimulated RAW264.7 macrophages. Bioorg Med. Chem. Lett. 25 (2), 254–258. doi:10.1016/j.bmcl.2014.11.059
Okoye, T. C., Akah, P. A., Okoli, C. O., Ezike, A. C., Omeje, E. O., and Odoh, U. E. (2012). Antimicrobial effects of a lipophilic fraction and kaurenoic acid isolated from the root bark extracts of Annona senegalensis. Evid. Based Complement. Altern. Med. 2012, 831327. doi:10.1155/2012/831327
Okoye, T. C., Akah, P. A., Omeje, E. O., Okoye, F. B., and Nworu, C. S. (2013). Anticonvulsant effect of kaurenoic acid isolated from the root bark of Annona senegalensis. Pharmacol. Biochem. Behav. 109, 38–43. doi:10.1016/j.pbb.2013.05.001
Osafo, N., Antwi, A. O., and Otu-Boakye, S. (2022). Xylopic acid suppresses adjuvant-induced arthritis in sprague dawley rats via reduction in serum levels of IL-6 and TNF-alpha. Antiinflamm. Antiallergy Agents Med. Chem. 21 (1), 46–61. doi:10.2174/1871523021666220310094218
Osafo, N., Obiri, D. D., Antwi, A. O., and Yeboah, O. K. (2018). The acute anti-inflammatory action of xylopic acid isolated from Xylopia aethiopica. J. Basic Clin. Physiol. Pharmacol. 29 (6), 659–669. doi:10.1515/jbcpp-2018-0019
Osafo, N., Obiri, D. D., Danquah, K. O., Essel, L. B., and Antwi, A. O. (2019). Potential effects of xylopic acid on acetic acid-induced ulcerative colitis in rats. Turk J. Gastroenterol. 30 (8), 732–744. doi:10.5152/tjg.2019.18389
Osei, S. A., Biney, R. P., Obese, E., Agbenyeku, M. A., Attah, I. Y., Ameyaw, E. O., et al. (2021). Xylopic acid-amodiaquine and xylopic acid-artesunate combinations are effective in managing malaria in Plasmodium berghei-infected mice. Malar. J. 20 (1), 113. doi:10.1186/s12936-021-03658-6
Paiva, L. A., Gurgel, L. A., Silva, R. M., Tomé, A. R., Gramosa, N. V., Silveira, E. R., et al. (2002). Anti-inflammatory effect of kaurenoic acid, a diterpene from Copaifera langsdorffi on acetic acid-induced colitis in rats. Vasc. Pharmacol. 39 (6), 303–307. doi:10.1016/s1537-1891(03)00028-4
Pereira, S., Taleb-Contini, S., Coppede, J., Pereira, P., Bertoni, B., França, S., et al. (2012). An ent-kaurane-type diterpene in Croton antisyphiliticus mart. Molecules 17 (8), 8851–8858. doi:10.3390/molecules17088851
Raga, D. D., Alimboyoguen, A. B., del Fierro, R. S., and Ragasa, C. Y. (2010). Hypoglycaemic effects of tea extracts and ent-kaurenoic acid from Smallanthus sonchifolius. Nat. Prod. Res. 24 (18), 1771–1782. doi:10.1080/14786411003594058
Sartori, M. R., Pretto, J. B., Cruz, A. B., Bresciani, L. F., Yunes, R. A., Sortino, M., et al. (2003). Antifungal activity of fractions and two pure compounds of flowers from Wedelia paludosa (Acmela brasiliensis) (Asteraceae). Pharmazie 58 (8), 567–569.
Soh, D., Ernestine, N., Tchana Satchet, E. M., Defokou, U. D., Schneider, B., Giovanni, V., et al. (2022). Antiproliferative activity of semisynthetic xylopic acid derivatives. Nat. Prod. Res. 36 (5), 1288–1295. doi:10.1080/14786419.2021.1876045
Somova, L. I., Shode, F. O., Moodley, K., and Govender, Y. (2001). Cardiovascular and diuretic activity of kaurene derivatives of Xylopia aethiopica and Alepidea amatymbica. J. Ethnopharmacol. 77 (2-3), 165–174. doi:10.1016/s0378-8741(01)00285-9
Sun, H. D., Huang, S. X., and Han, Q. B. (2006). Diterpenoids from Isodon species and their biological activities. Nat. Prod. Rep. 23 (5), 673–698. doi:10.1039/b604174d
Takahashi, J. A., Boaventura, M. A. D., de Carvalho Bayma, J., and Alaíde B, O. (1995). Frutoic acid, a dimeric kaurane diterpene from Xylopia frutescens. Phytochemistry 40 (2), 607–609. doi:10.1016/0031-9422(95)00264-8
Tirapelli, C. R., Ambrosio, S. R., da Costa, F. B., Coutinho, S. T., de Oliveira, D. C., and de Oliveira, A. M. (2004). Analysis of the mechanisms underlying the vasorelaxant action of kaurenoic acid in the isolated rat aorta. Eur. J. Pharmacol. 492 (2-3), 233–241. doi:10.1016/j.ejphar.2004.04.003
Wang, L., Li, D., Wang, C., Zhang, Y., and Xu, J. (2011). Recent progress in the development of natural ent-kaurane diterpenoids with anti-tumor activity. Mini Rev. Med. Chem. 11 (10), 910–919. doi:10.2174/138955711796575416
Wilkens, M., Alarcón, C., Urzúa, A., and Mendoza, L. (2002). Characterization of the bactericidal activity of the natural diterpene kaurenoic acid. Planta Med. 68 (5), 452–454. doi:10.1055/s-2002-32086
Woode, E., Ameyaw, E., Ainooson, G., Abotsi, W., Gyasi, E., and Kyekyeku, J. (2013). Analgesic effects of an ethanol extract of the fruits of Xylopia aethiopica and xylopic acid in murine models of pain: Possible mechanism (s). Pharmacologia 4 (4), 285–300. doi:10.5567/pharmacologia.2013.285.300
Woode, E., Ameyaw, E. O., Abotsi, W. K., and Boakye-Gyasi, E. (2015). An isobolographic analysis of the antinociceptive effect of xylopic acid in combination with morphine or diclofenac. J. Basic Clin. Pharm. 6 (4), 103–108. doi:10.4103/0976-0105.168055
Woode, E., Ameyaw, E. O., Boakye-Gyasi, E., and Abotsi, W. K. (2012). Analgesic effects of an ethanol extract of the fruits of Xylopia aethiopica (Dunal) A. Rich (Annonaceae) and the major constituent, xylopic acid in murine models. J. Pharm. Bioallied Sci. 4 (4), 291–301. doi:10.4103/0975-7406.103251
Woode, E., Ameyaw, E. O., Boakye-Gyasi, E., Abotsi, W. K., Oppong Kyekyeku, J., Adosraku, R., et al. (2016). Effects of an ethanol extract and the diterpene, xylopic acid, of Xylopia aethiopica fruits in murine models of musculoskeletal pain. Pharm. Biol. 54 (12), 2978–2986. doi:10.1080/13880209.2016.1199040
Wu, Y.-C. (2006). “New research and development on the formosan annonaceous plants,” in Studies in natural products chemistry. Editor R. Atta (London, UK: Elsevier).
Wu, Y. C., Hung, Y. C., Chang, F. R., Cosentino, M., Wang, H. K., and Lee, K. H. (1996). Identification of ent-16 beta, 17-dihydroxykauran-19-oic acid as an anti-HIV principle and isolation of the new diterpenoids annosquamosins A and B from Annona squamosa. J. Nat. Prod. 59 (6), 635–637. doi:10.1021/np960416j
Yamaguchi, S. (2008). Gibberellin metabolism and its regulation. Annu. Rev. Plant Biol. 59, 225–251. doi:10.1146/annurev.arplant.59.032607.092804
Yang, Y. L., Chang, F. R., Wu, C. C., Wang, W. Y., and Wu, Y. C. (2002). New ent-kaurane diterpenoids with anti-platelet aggregation activity from Annona squamosa. J. Nat. Prod. 65 (10), 1462–1467. doi:10.1021/np020191e
Yeh, S. H., Chang, F. R., Wu, Y. C., Yang, Y. L., Zhuo, S. K., and Hwang, T. L. (2005). An anti-inflammatory ent-kaurane from the stems of Annona squamosa that inhibits various human neutrophil functions. Planta Med. 71 (10), 904–909. doi:10.1055/s-2005-871234
Zaninelli, T. H., Mizokami, S. S., Bertozzi, M. M., Saraiva-Santos, T., Pinho-Ribeiro, F. A., de Oliveira, G. I., et al. (2023). Kaurenoic acid reduces ongoing chronic constriction injury-induced neuropathic pain: Nitric oxide silencing of dorsal root ganglia neurons. Pharm. (Basel) 16 (3), 343. doi:10.3390/ph16030343
Keywords: Annonaceae, ent-kaurane diterpenoid, biosynthesis, biological activity, isolation
Citation: Ibrahim TS, Khongorzul P, Muyaba M and Alolga RN (2023) Ent-kaurane diterpenoids from the Annonaceae family: a review of research progress and call for further research. Front. Pharmacol. 14:1227574. doi: 10.3389/fphar.2023.1227574
Received: 23 May 2023; Accepted: 22 June 2023;
Published: 29 June 2023.
Edited by:
Adolfo Andrade-Cetto, National Autonomous University of Mexico, MexicoReviewed by:
Valeria P. Sulsen, University of Buenos Aires, ArgentinaCopyright © 2023 Ibrahim, Khongorzul, Muyaba and Alolga. This is an open-access article distributed under the terms of the Creative Commons Attribution License (CC BY). The use, distribution or reproduction in other forums is permitted, provided the original author(s) and the copyright owner(s) are credited and that the original publication in this journal is cited, in accordance with accepted academic practice. No use, distribution or reproduction is permitted which does not comply with these terms.
*Correspondence: Raphael N. Alolga, alolgara@cpu.edu.cn
†These authors share first authorship