- 1Department of Physiology, Faculty of Medicine, Universiti Kebangsaan Malaysia, Kuala Lumpur, Malaysia
- 2Faculty of Medicine and Defence Health, National Defence University of Malaysia, Kuala Lumpur, Malaysia
Hypertension and diabetes mellitus are among the most prevalent diseases affecting people from all walks of life. Medicinal herbs have garnered interest as potential agents for the prevention and treatment of diabetes mellitus and hypertension due to their multiple beneficial effects. Piper sarmentosum Roxb. (PS) is an edible medicinal plant that has been traditionally used in Asia for treating hypertension and diabetes mellitus. This review is aimed to provide comprehensive information from the literature on the effects of PS on hypertension and diabetes mellitus. A computerized database search was performed on Scopus, PubMed and Web of Science databases with the following set of keywords: Piper sarmentosum AND diabetes mellitus OR diabetic OR diabetes OR hyperglyc*emia OR blood glucose OR HbA1c OR glycated h*emoglobin OR h*emoglobin A1c OR hyperten* OR blood pressure. A total of 47 articles were screened and 14 articles published between the years 1998 until 2021 were included for data extraction, comprising of six articles on antihypertensive and eight articles on antidiabetic effects of PS. These studies consist of two in vitro studies and eleven in vivo animal studies. Meta-analysis of three studies on hypertension showed that PS versus no treatment significantly lowered the systolic blood pressure with mean difference (MD) −39.84 mmHg (95% confidence interval (CI) −45.05, −34.62; p < 0.01), diastolic blood pressure with MD −26.68 mmHg (95% CI −31.48, −21.88; p < 0.01), and mean arterial pressure with MD −30.56 mmHg (95% CI −34.49, −26.63; p < 0.01). Most of the studies revealed positive effects of PS against hypertension and diabetes mellitus, suggesting the potential of PS as a natural source of antidiabetic and antihypertensive agents.
1 Introduction
Hypertension and diabetes mellitus are among the most common non-communicable diseases and cardiovascular risk factors worldwide. Diabetic patients have a two to three-fold rise in hypertension prevalence compared to non-diabetics (Wan et al., 2018). Hypertension coexists in about 40–60% of type 2 diabetic patients (Tatsumi and Ohkubo, 2017). Hypertension may also precede the onset of diabetes, with more than 50% of adults having both hypertension and diabetes at the time of diagnosis (Nibouche and Biad, 2016). Diabetes mellitus and hypertension often coexist and share common pathways contributing to metabolic syndrome. Metabolic syndrome is associated with a greater risk for cardiovascular diseases (CVD) including heart attack and stroke (Isomaa et al., 2001; Tune et al., 2017).
The development of hypertension in diabetic patients is contributed by multiple factors such as insulin resistance, hyperglycemia, oxidative stress, and inflammation. Insulin resistance leads to the development of hyperinsulinemia. The anti-natriuretic activity of insulin increases sodium and water reabsorption from the renal tubules, leading to volume overload and elevation of blood pressure. Moreover, the body’s extracellular fluid volume rises because fluid move from the tissues into the vasculature following hyperglycemia-induced hyperosmolarity (Kawasoe et al., 2017). Hyperinsulinemia also activates the sympathetic nervous system and renin-angiotensin system (RAS), leading to an increase in blood pressure (Ohishi, 2018). Besides, hyperinsulinemia leads to vascular smooth muscle cell proliferation and increased vascular stiffness that predispose to hypertension (Shiny et al., 2016; Tsimihodimos et al., 2018). Furthermore, hyperglycemia triggers oxidative stress and inflammatory processes in the vascular wall that cause endothelial dysfunction, impaired vasodilatation and eventually hypertension (Wong et al., 2013; Oguntibeju, 2019; Sun et al., 2020).
On the other hand, hypertensive patients also have an increased risk of developing diabetes mellitus (Zhang et al., 2020). Hypertension is characterized by endothelial dysfunction, which might link hypertension with diabetes (Emdin et al., 2015). Numerous studies have reported that decreased endothelium-dependent vasodilatation in hypertension leads to decreased capillary recruitment that restricts insulin delivery to the metabolically active, insulin-sensitive muscle tissues (Bonadonna et al., 1998; Serné et al., 1999; Meigs et al., 2004). Besides, the altered endothelial permeability impairs insulin delivery to the interstitial space (Meigs et al., 2004). The interstitial insulin level is a rate-limiting step for insulin effectiveness (Miles et al., 1995).
Hypertension and diabetes mellitus share not only common pathophysiologic pathways but also common complications involving the macro- and microvascular disorders. Macrovascular complications include stroke, coronary artery disease and peripheral vascular disease, while microvascular complications include retinopathy, nephropathy and neuropathy (Yamazaki et al., 2018). Since the development of hypertension in patients with diabetes is marked by a significant risk of macro- and microvascular complications, efforts should be made to delay or ideally prevent the increase in blood pressure. Hence, a therapy that can help with glycemic and blood pressure control will be of significant clinical value.
Piper sarmentosum Roxb. (PS) is a herbaceous plant that is widely cultivated in Southeast Asia, Northeast India and China (Mathew et al., 2004). It is a terrestrial creeping herb that belongs to the family of Piperaceae, with an average height of 20 cm and easily grows in shady areas (Mohd Zainudin et al., 2013). PS leaves are light to dark green in colour (Chaveerach et al., 2006), and the fruits are obovoid in shape and sweet to taste (Figure 1). PS are also known as “Kaduk” or “Sirih duduk” in Malaysia; “Cha plu” in Thailand; “Karuk”, “Mengkadak” or “Sirih tanah” in Indonesia; “La lot” in Vietnam; “Phak i leut” in Laos and “Jia ju”, “Xi ye qing wei teng” or “Qing ju” in China (Ismail et al., 2018).
Historically, PS has long been used as a culinary plant as well as a traditional medicine to treat hypertension, joint aches (Subramaniam et al., 2003), cough, pleurisy, fever (Farhana Syed Ab Rahman 2016), and indigestion (Hussain et al., 2012). Pharmacologically, PS possesses antiatherosclerosis (Amran et al., 2010; Amran et al., 2011), anticarcinogenic (Zainal Ariffin et al., 2009), anti-inflammatory (Ridtitid et al., 2007; Zakaria et al., 2010), antiplatelet aggregation (Han, 1995), antiangiogenic (Hussain et al., 2015) and antituberculosis (Hussain et al., 2008) effects. PS also protects against glucocorticoid-induced osteoporosis (Mohamad Asri et al., 2016) and paracetamol-induced oxidative liver injury (Nur Azlina et al., 2014). Several studies have reported that PS has a high antioxidative activity (Subramaniam et al., 2003; Hafizah et al., 2010; Ugusman et al., 2010; Mohd Zainudin et al., 2015). Interestingly, PS also has antidiabetic (Peungvicha et al., 1998; Azlina et al., 2009; Luangpirom et al., 2014) and antihypertensive effects (Hussan et al., 2013; Alwi et al., 2018; Fauzy et al., 2019).
Previous studies demonstrated that different parts of PS contain various phytochemical compounds. The methanolic extract of PS leaves contains carotenes, tannin, vitamin C, vitamin E, xanthophylls, and phenolics (Chanwitheesuk et al., 2005), while the roots and stems of PS contain piplartine, langkamide and 3,4,5-trimethoxycinnamic acid (Bokesch et al., 2011). Besides, the aqueous extract of PS leaves contains flavonoids, phenolic and ascorbic acids (Sumazian et al., 2010). Meanwhile, three amides; 3-(3′,4′,5′-trimethoxyphenylpropanoyl) pyrrolidine, 3-(4′-methoxyphenylpropanoyl) pyrrole and N-(3-phenylpropanoyl) pyrrole, and a sterol; β-sitosterol have been successfully isolated from the hexane and ethyl acetate extracts of aerial parts of PS (Atiax et al., 2011).
The aqueous extract of PS leaves up to 2000 mg/kg/day does not cause subacute toxicity effects; hence it is safe for consumption (Mohd Zainudin et al., 2013). Recently, the consumption of herbal products for complementary and alternative medicine has been rapidly increasing. Previous studies have suggested that antioxidants could be beneficial for managing diabetes mellitus and hypertension (Sabadashka et al., 2021; Tain and Hsu 2022). An earlier systematic review has confirmed the antioxidative effect of PS (Ismail et al., 2018). However, no systematic review and meta-analysis have been conducted to explore the effect of PS on hypertension and diabetes. Thus, we aimed to systemically review the available literature on the effects of PS on diabetes mellitus and hypertension to better understand the herb’s medicinal potential to support its scientific use further.
2 Methodology
2.1 Search strategy
The review was reported according to the Preferred Reporting Items for Systematic Reviews and Meta-Analyses (PRISMA) guideline. The relevant studies were identified from three main databases: Scopus, PubMed and Web of Science (WoS) from their respective inception dates to March 2022. The last search was performed on 26th March 2022. The following set of keywords was used: Piper sarmentosum AND (diabetes mellitus OR diabetic OR diabetes OR hyperglyc*emia OR blood glucose OR HbA1c OR glycated h*emoglobin OR h*emoglobin A1c OR hyperten* OR blood pressure). Articles that could be missing during the database search were searched from the reference list of the review articles retrieved from the initial search and added to the selected articles list (Thent and Das, 2015). The protocol of this review was registered at the International Platform of Registered Systematic Review and Meta-analysis Protocols (INPLASY registration number: 202240020) (Othman et al., 2022).
2.2 Eligibility and exclusion criteria
Only full-length original research articles published in the English language were included. Any clinical (randomized controlled trial) and preclinical (in vitro, in vivo, and ex vivo) studies that reported the effects of PS on hypertension and diabetes models, regardless of the route of administration, formulation, dose and duration of intervention were included. However, any observational studies and studies using combined preparation of PS with other herbs were excluded. Review articles, news, book chapters, conference proceedings, editorial letters, and case studies were also excluded from this review.
2.3 Study selection and data extraction
The literature search and articles screening were performed according to the population, interventions, compare, outcome and study design (PICOS) framework, as follows:
1) Population (P): Adult patients with established hypertension and/or diabetes and preclinical models of hypertension and diabetes, regardless of animal species, were included.
2) Intervention (I): Studies that used PS as an intervention in the experimental group were included.
3) Comparison (C): The comparator groups received either no intervention or were treated with relevant conventional drug.
4) Outcome (O): Changes in blood pressure, blood glucose or glycosylated hemoglobin (HbA1C).
5) Study design (S): Clinical (randomized controlled trial) and preclinical (in vitro, in vivo, ex vivo) studies.
The articles were primarily screened through the articles’ type, language, title and abstracts related to the effect of PS on hypertension and diabetes. Duplicates were removed from each database. The secondary screening involved the removal of articles based on the inclusion and exclusion criteria set for this study. Any similar studies were removed to avoid selection bias. The retrieved articles were reviewed independently by two authors (NO. and AU). Any disagreements were resolved by seeking a third reviewer’s opinion (NR).
Study characteristics including study design, animal model used, plant source, the part of plant used, type of extraction and phytochemical used were extracted. Primary outcomes such as systolic blood pressure (SBP), diastolic blood pressure (DBP), mean arterial pressure (MAP), and blood glucose level were extracted. Other parameters such as nitric oxide (NO), endothelial nitric oxide synthase (eNOS), asymmetric dimethylarginine (ADMA), endothelin-1 (ET-1) and malondialdehyde (MDA) levels, α-glucosidase and α-amylase activities, insulin and urine glucose levels, body and organ weights, and histological analysis of the target organs were also extracted where available. For studies with more than one interventional arms, data from only the relevant arms were considered, e.g. hypertensive rats receiving PS versus hypertensive rats receiving positive control or no treatment. In case of missing or incomplete information, the respective author was contacted by email and the missing data were requested if necessary.
2.4 Risk of bias assessment
Two reviewers analyzed the risk of bias independently (NO and AU). Any disagreement was resolved through discussion with the third reviewer (NR). Cochrane risk of bias (RoB) tool was used to assess the risk of bias in randomized clinical trials (Higgins et al., 2019). Meanwhile, animal studies were assessed using the Systematic Review Center for Laboratory Animal Experimentation (SYRCLE) risk of bias tool. The main components of this item were as follows: 1) Selection bias: random sequence generation, baseline characteristics, allocation concealment; 2) Detection bias: random housing, blinding, random outcome assessment; 3) Attrition bias: incomplete outcome data; 4) Reporting bias: selective reporting; and 5) Other bias (Hooijmans et al., 2014). For in vitro studies, a customized risk of bias tool based on the Joanna Briggs Institute (JBI) checklist for non-randomized experimental studies were used (JBI, 2020). The customized RoB tool comprises of three domains as follows: 1) Reporting quality: source of plant, amount of plant/extract/sample used; 2) Performance bias: reliable tools and/or reagents used to measure outcome; and 3) Detection bias: standard/appropriate control used, multiple measurements of outcome performed. Each domain was evaluated as being a high, moderate, low or unclear risk of bias.
2.5 Statistical analysis
The meta-analysis was performed using the Review Manager (RevMan) 5.4 software (The Cochrane Collaboration, 2020). The mean difference (MD) together with its 95% confidence intervals (CI) was used in reporting the effect size of PS on blood pressure (BP). The heterogeneity between studies was evaluated using 1) the Chi-squared test with a p-value of less than 0.10 denoted statistical significance and 2) the Higgin’s I2 statistic (Higgins et al., 2003). An I2 value of less than 25% was regarded as low heterogeneity, 30%–50% was regarded as moderate heterogeneity, and any value above 75% as high heterogeneity. Due to the small number of studies available for meta-analysis, a fixed-effect (FE) model was used. A p-value of less than 0.05 indicated statistical significance. Sensitivity analysis was conducted by only including studies using a similar PS dose (500 mg/kg) for evaluation of result’s robustness. No subgroup analysis was performed due to the limited number of studies available for meta-analysis. A funnel plot was not reported as less than ten studies were included in the meta-analysis.
3 Results
3.1 Studies selected
Initially, a total of 76 potential articles were identified in Scopus (n = 37), PubMed (n = 12) and Web of Science (n = 27). An additional article was retrieved from the list of references cited in the review articles (n = 1). Subsequently, 30 articles were removed due to duplication. After reviewing the titles and abstracts, 33 articles were excluded. The full text of the remaining 27 articles were read thoroughly and 13 articles were further excluded as they did not fulfil the inclusion criteria. Finally, 14 studies published between the years 1998–2021 were selected for inclusion in this review, comprising of six articles on antihypertensive and eight articles on antidiabetic effects of PS. A flowchart of the article selection process is shown in Figure 2.
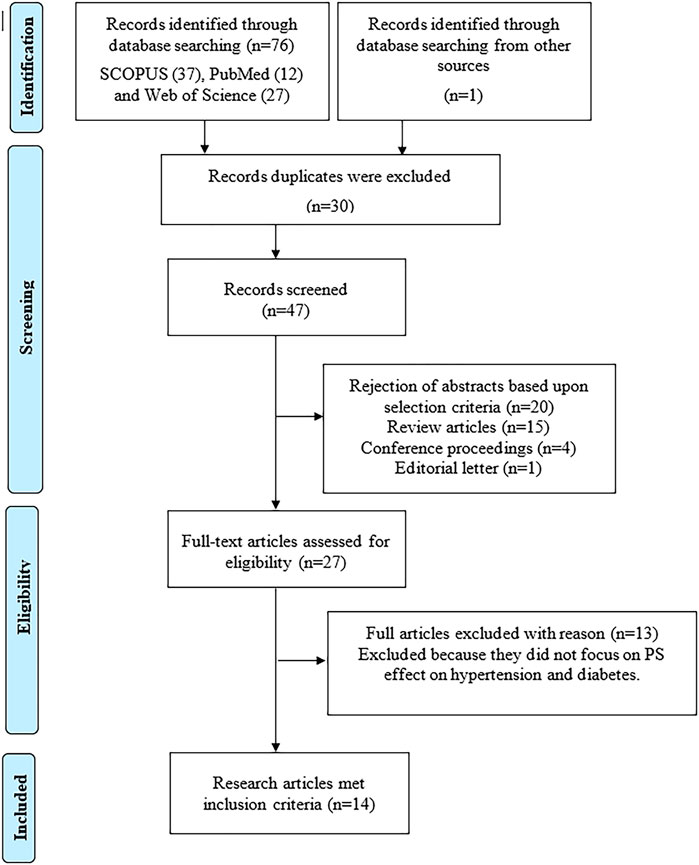
FIGURE 2. The selection process of the articles according to the Preferred Reporting Items for Systematic Reviews and Meta-Analyses (PRISMA) guideline.
3.2 Risk of bias
The risk of bias assessment for the animal studies included in this review is presented in Figure 3. Selection risk and detection of bias was low for all studies. Attrition bias was either low risk (n = 10) or unclear (n = 2) in the included studies. Selection reporting risk of bias was unclear in two of the studies and the remainder (n = 10) was low risk. Other bias was unclear in one of the studies and low for the remainder (n = 11). The JBI critical appraisal checklist summary for reporting in vitro studies is shown in Figure 4. Reporting quality bias was low for all studies. Performance and detection bias was either low risk (n = 1) or unclear (n = 1).
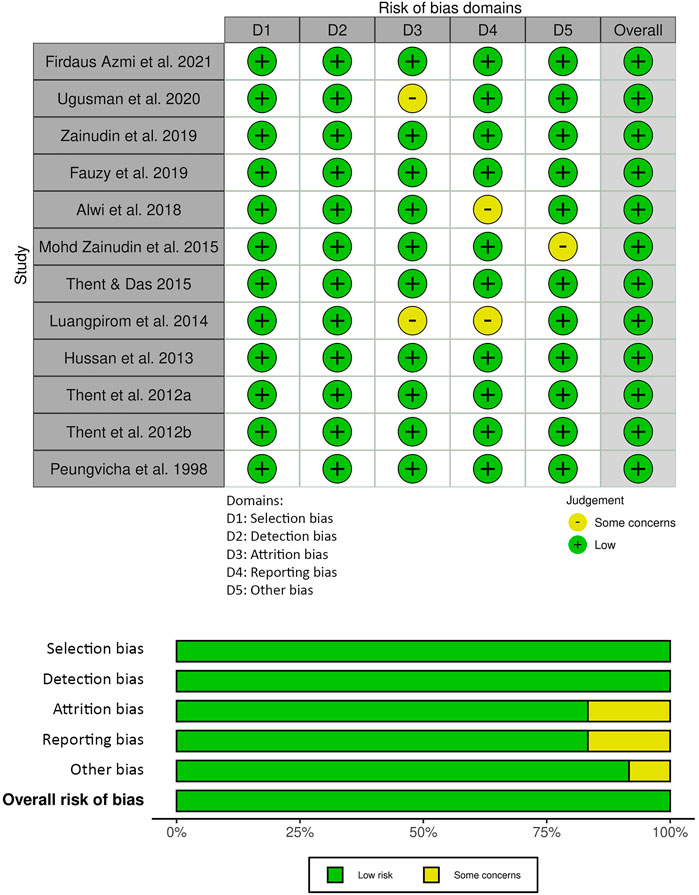
FIGURE 3. Systematic Review Center for Laboratory Animal Experimentation (SYRCLE) risk of bias summary: review authors’ judgements about each risk of bias item for each included study.
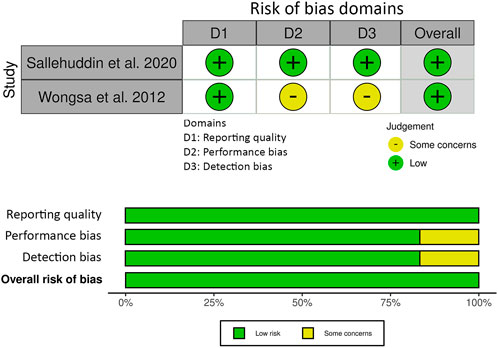
FIGURE 4. The Joanna Briggs Institute (JBI) critical appraisal checklist summary for reporting in vitro studies.
3.3 Study design characteristics
The characteristics of the selected studies are described in Tables 1, 2. All the included studies were preclinical studies, with no clinical trial done previously. As for the preclinical studies, 12 studies involved animal models (Peungvicha et al., 1998; Thent et al., 2012a; Thent et al., 2012b; Hussan et al., 2013; Luangpirom et al., 2014; Mohd Zainudin et al., 2015; Thent and Das, 2015; Alwi et al., 2018; Mohd Zainudin et al., 2019; Fauzy et al., 2019; Ugusman et al., 2020; Firdaus Azmi et al., 2021) and the remaining two studies were in vitro chemical assay studies (Wongsa et al., 2012; Sallehuddin et al., 2020). For studies related to the effect of PS on hypertension, the animal models of hypertension used were spontaneous hypertensive rats (SHR) (Mohd Zainudin et al., 2015; Fauzy et al., 2019; Mohd Zainudin et al., 2019), dexamethasone-induced hypertensive rats (Ugusman et al., 2020; Firdaus Azmi et al., 2021) and Nω-nitro-l-arginine methyl ester hydrochloride (l-NAME)-induced hypertensive rats (Alwi et al., 2018). Meanwhile, induction of diabetes in all the studies were done through streptozotocin (STZ) injection.
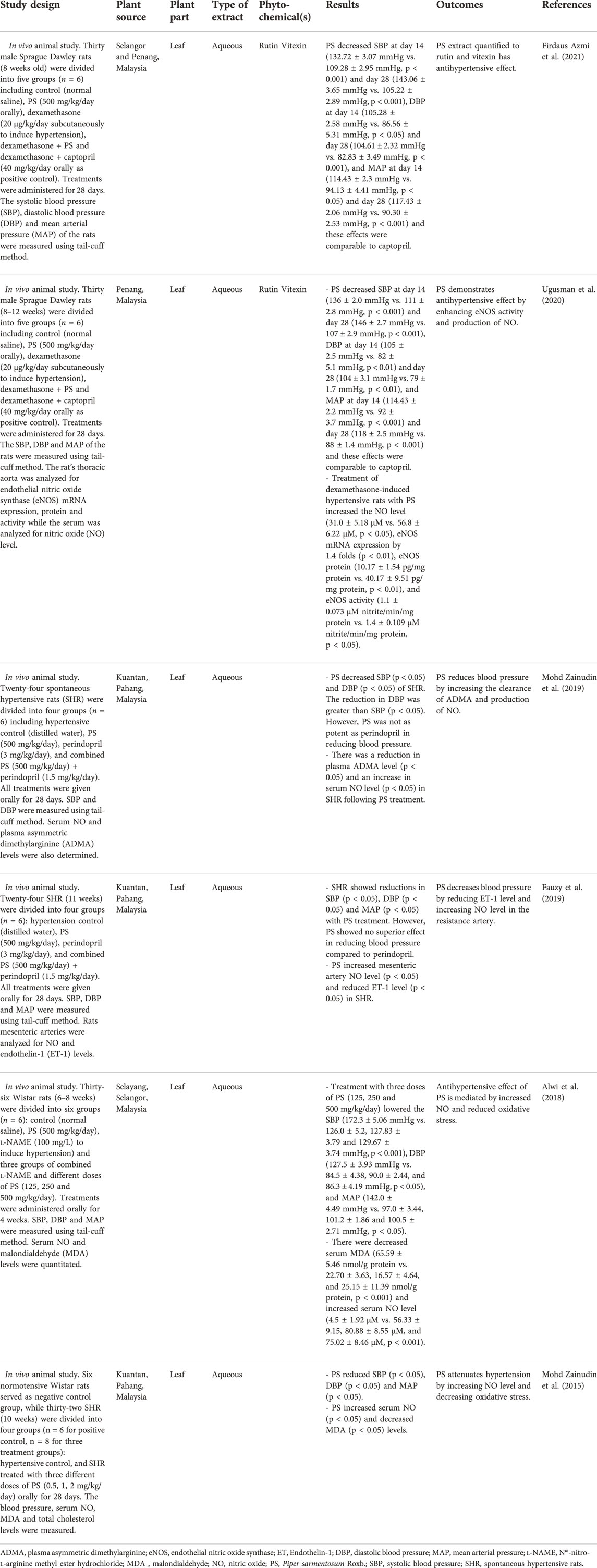
TABLE 1. Characteristics of selected studies on the effects of Piper sarmentosum Roxb. on hypertension.
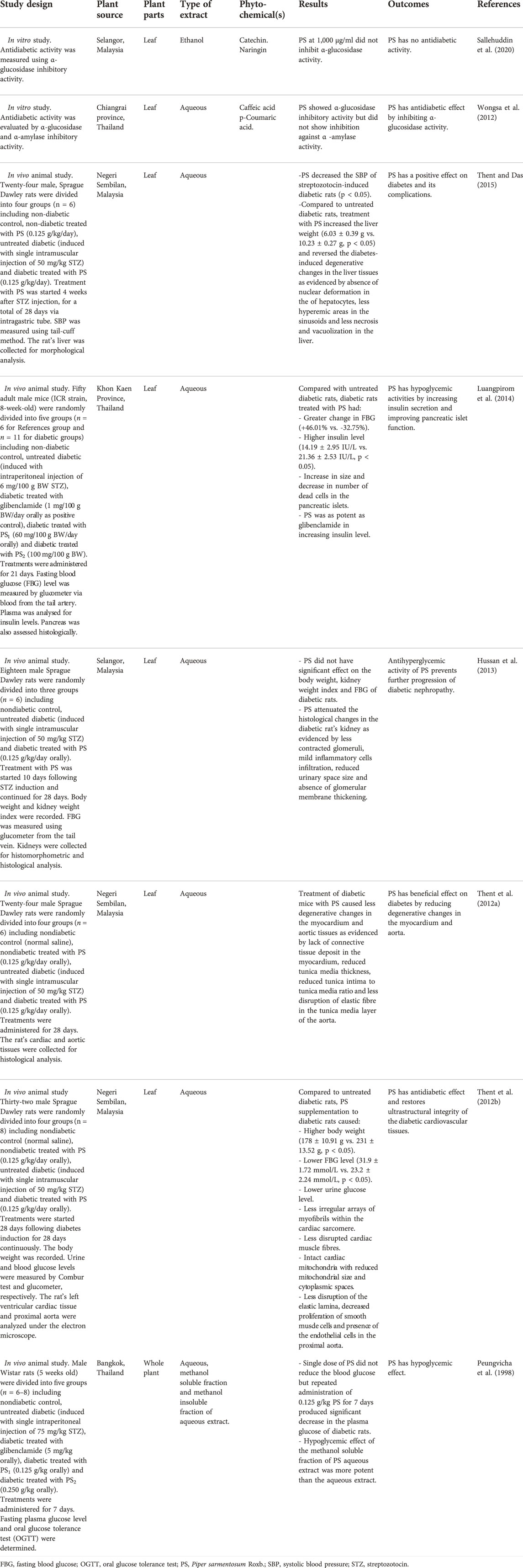
TABLE 2. Characteristics of selected studies on the effects of Piper sarmentosum Roxb. on diabetes mellitus.
The quality control and chemical analysis of PS extracts in the selected studies were summarized in Table 3. The origins of PS were from two main countries: Malaysia (Thent et al., 2012a; Thent et al., 2012b; Hussan et al., 2013; Mohd Zainudin et al., 2015; Thent and Das, 2015; Alwi et al., 2018; Fauzy et al., 2019; Mohd Zainudin et al., 2019; Sallehuddin et al., 2020; Ugusman et al., 2020; Firdaus Azmi et al., 2021) and Thailand (Peungvicha et al., 1998; Wongsa et al., 2012; Luangpirom et al., 2014). Most of the studies used the leaves of PS (Thent et al., 2012a, 2012b; Wongsa et al., 2012; Hussan et al., 2013; Luangpirom et al., 2014; Mohd Zainudin et al., 2015; Alwi et al., 2018; Fauzy et al., 2019; Mohd Zainudin et al., 2019; Sallehuddin et al., 2020; Ugusman et al., 2020; Firdaus Azmi et al., 2021) and only one study used all parts of PS (Peungvicha et al., 1998). Different types of PS extract were used, including ethanol extract (Thent and Das 2015; Sallehuddin et al., 2020), aqueous extract (Peungvicha et al., 1998; Thent et al., 2012a; Thent et al., 2012b; Wongsa et al., 2012; Hussan et al., 2013; Luangpirom et al., 2014; Mohd Zainudin et al., 2015; Alwi et al., 2018; Fauzy et al., 2019; Mohd Zainudin et al., 2019; Ugusman et al., 2020; Firdaus Azmi et al., 2021) and methanol-soluble and -insoluble fractions of aqueous extract of PS (Peungvicha et al., 1998). Among the active compounds found in the phytochemical analyses of the PS extracts were rutin, vitexin (Ugusman et al., 2020; Firdaus Azmi et al., 2021), catechin, naringin (Sallehuddin et al., 2020), caffeic acid and p-coumaric acid (Wongsa et al., 2012).
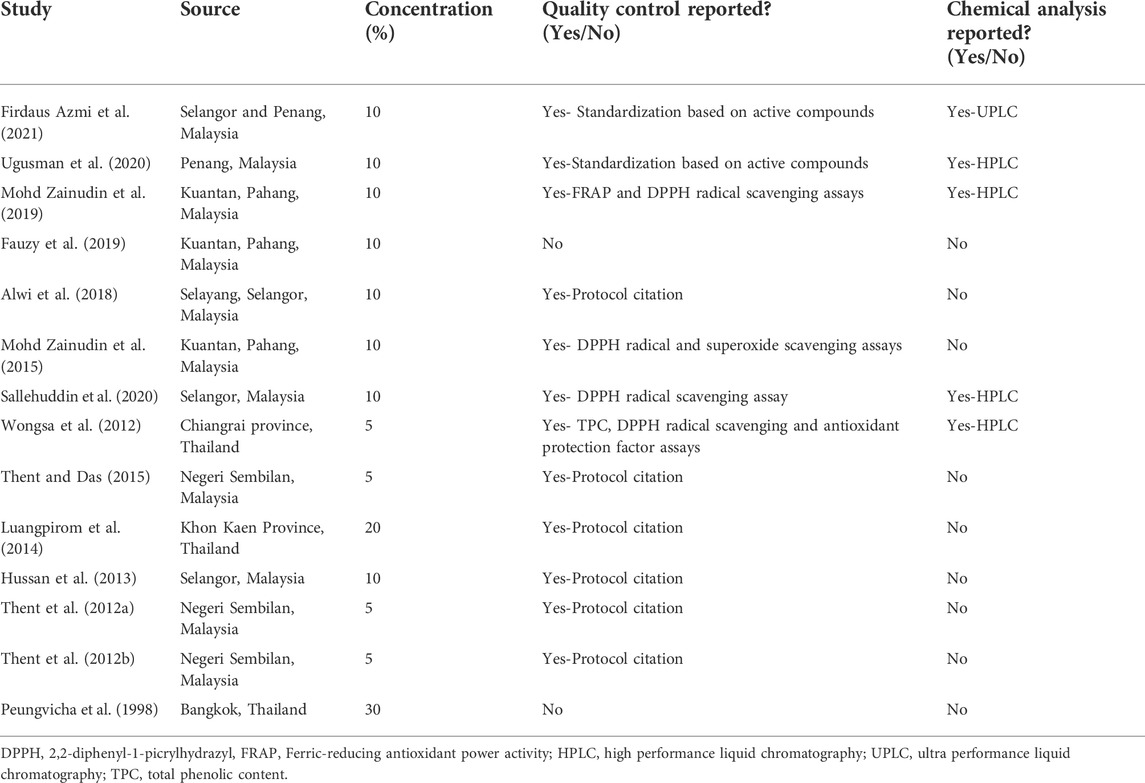
TABLE 3. Quality control and chemical analysis of Piper sarmentosum Roxb. extracts in the selected studies.
3.4 Effect of Piper sarmentosum Roxb. on hypertension
There were six in vivo animal studies that focused on the effect of PS on hypertension (Mohd Zainudin et al., 2015; Alwi et al., 2018; Fauzy et al., 2019; Mohd Zainudin et al., 2019; Ugusman et al., 2020; Firdaus Azmi et al., 2021). Systolic blood pressure (SBP), diastolic blood pressure (DBP), mean arterial pressure (MAP) and nitric oxide (NO) levels were the most common parameters measured. All six studies showed that treatment with PS caused a marked reduction in the SBP, DBP and MAP of hypertensive rat models. A study by Fauzy et al. (2019) demonstrated that PS increased NO and reduced ET-1 levels in the mesenteric artery of SHR. Mohd Zainudin et al. (2015) showed that PS possessed an antihypertensive effect by reducing MDA and increasing serum NO levels in SHR. These findings were further supported by three other studies which demonstrated that the BP-lowering effect of PS was associated with increased NO levels in l-NAME-induced hypertensive rats (Alwi et al., 2018), dexamethasone-induced hypertensive rats (Ugusman et al., 2020; Firdaus Azmi et al., 2021) and SHR (Mohd Zainudin et al., 2019). Ugusman et al. (2020) found that PS increased vascular NO production by increasing eNOS mRNA expression, eNOS protein and eNOS activity in dexamethasone-induced hypertensive rats. Meanwhile, Mohd Zainudin et al. (2015) demonstrated that PS significantly decreased plasma ADMA levels in SHR. However, none of the studies investigated the effect of PS on target organ damage in hypertension.
3.5 Effect of Piper sarmentosum Roxb. on diabetes mellitus
Out of the 14 studies, eight studies assessed the effect of PS on diabetes mellitus. The effects of PS on diabetes were evaluated in two in vitro studies (Wongsa et al., 2012; Sallehuddin et al., 2020) and six in vivo studies (Peungvicha et al., 1998; Thent et al., 2012a; Thent et al., 2012b; Hussan et al., 2013; Luangpirom et al., 2014; Thent and Das, 2015). The in vitro studies involved screening of antidiabetic activity of PS using α-glucosidase and α-amylase inhibition assays. Wongsa et al. (2012) showed that PS had α-glucosidase but not α-amylase inhibitory activity. In contrast, another in vitro study revealed that PS had no α-glucosidase inhibitory activity (Sallehuddin et al., 2020). As for in vivo study, it was first reported that administration of 0.125 g/kg PS for 7 days significantly decreased blood glucose levels in both normal and diabetic rats (Peungvicha et al., 1998). Subsequently, Thent et al. (2012b) showed that PS increased the body weight and reduced fasting blood glucose and urine glucose levels in STZ-induced diabetic rats. Meanwhile, Luangpirom et al. (2014) found that diabetic rats treated with PS improved pancreatic islet function and increased serum insulin, leading to reduced fasting blood glucose.
3.5.1 Piper sarmentosum Roxb.’s effect on diabetic complications/target organ and tissue damage
PS were also beneficial in attenuating the degenerative changes of the target organs in diabetes such as the heart, aorta, kidney, and liver. Light microscopic observation showed that PS reduced the degenerative changes in the myocardium and aortic tissues of diabetic rats as evidenced by lack of connective tissue deposit in the myocardium, reduced tunica media thickness, reduced tunica intima to tunica media ratio and less disruption of elastic fibre in the tunica media layer of the aorta (Thent et al., 2012a). Electron microscopic analysis further supported the findings as PS supplementation restored the ultrastructural integrity of the heart and aorta of diabetic rats (Thent et al., 2012b). As for the liver, PS increased the liver weight and reversed the diabetes-induced degenerative changes in the liver tissues as evidenced by the absence of nuclear deformation in the hepatocytes, less hyperemic areas in the sinusoids and less necrosis and vacuolization in the liver (Thent and Das, 2015). Interestingly, Hussan et al. (2013) demonstrated that PS prevented further progression of diabetic nephropathy in STZ-induced rats as evidenced by less contracted glomeruli, mild inflammatory cells infiltration, reduced urinary space size and absence of glomerular membrane thickening.
3.6 Meta-analysis
Only three studies were included in our meta-analysis, all of which provided data for the pooling of effects of PS on blood pressure parameters. No suitable data on glycemic parameters was available from the included studies, hence no meta-analysis was performed to explore the effects of PS on diabetes. The corresponding author (Luangpirom et al., 2014) was contacted to enquire for more details of the data, but no response was received.
3.6.1 Effects of Piper sarmentosum Roxb. versus no treatment on hypertension
Meta-analysis of three studies (Alwi et al., 2018; Ugusman et al., 2020; Firdaus Azmi et al., 2021) on the effect of PS versus no treatment have shown a statistically significant reduction in SBP with MD −39.84 mmHg (95% CI −45.05, −34.62; p < 0.01; Figure 5A) and no heterogeneity was observed. Similarly, pooling of results from three studies have shown that treatment with PS significantly reduced DBP and MAP with MD values of −26.68 mmHg (95% CI −31.48, −21.88; p < 0.01; Figure 5B), and -30.56 mmHg (95% CI −34.49, − 26.63; p < 0.01; Figure 5C) respectively, albeit the heterogeneity observed were substantial. A sensitivity analysis was performed to assess the robustness of PS effect on BP parameters. When limited to PS dose of 500 mg/kg, a comparable pooled effect size, direction, magnitude and statistical significance were obtained with MD −39.28 mmHg (95% CI −44.61, −33.95; p < 0.01; Figure 6A), −26.84 mmHg (95% CI −31.63, −22.05; p < 0.01; Figure 6B) and −29.74 mmHg (95% CI −33.82, −25.66; p < 0.01; Figure 6C) for SBP, DBP, and MAP respectively.
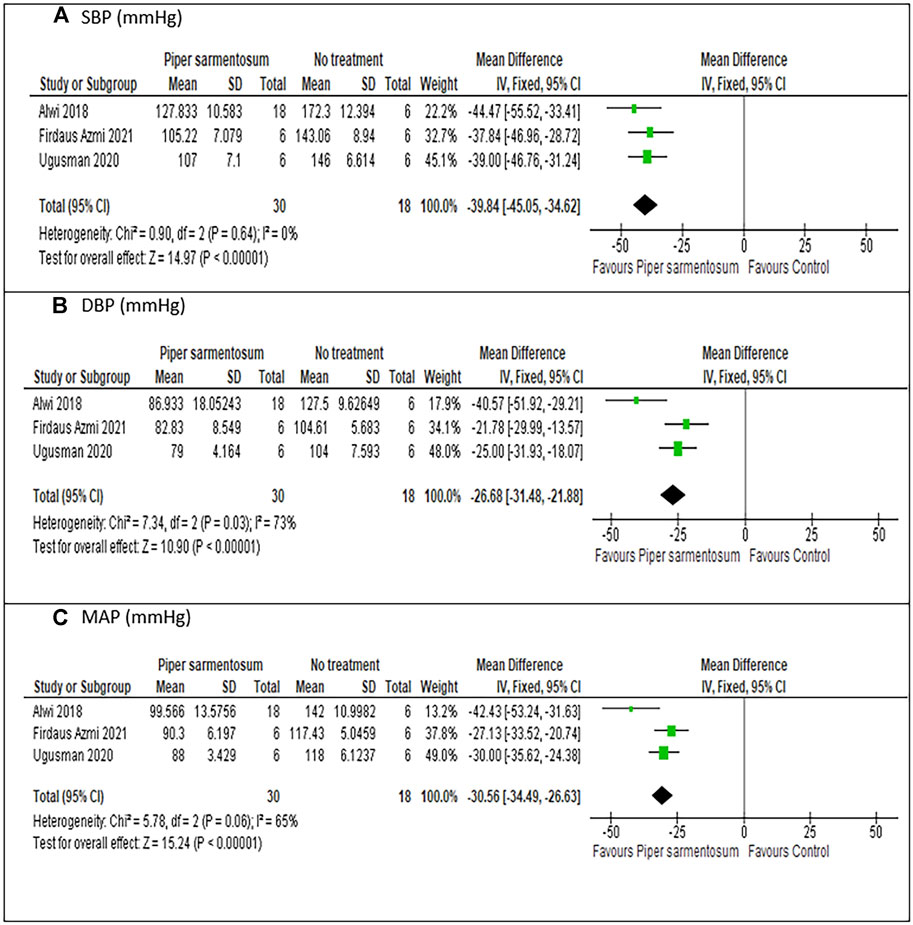
FIGURE 5. Meta-analysis of the effects of Piper sarmentosum Roxb. versus control on blood pressure: (A) SBP, Systolic blood pressure; (B) DBP, diastolic blood pressure, (C) MAP, mean arterial pressure.
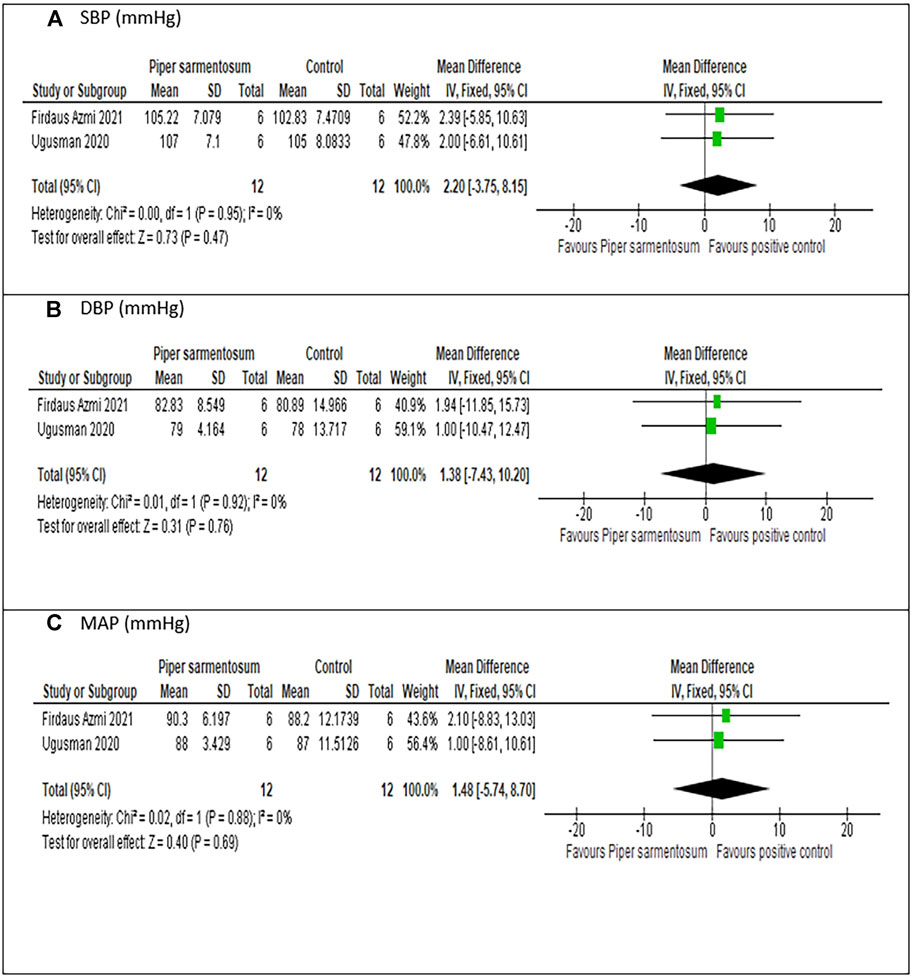
FIGURE 6. Meta-analysis of the effects of Piper sarmentosum Roxb. versus positive control on blood pressure: (A) SBP, Systolic blood pressure; (B) DBP, diastolic blood pressure, (C) MAP, mean arterial pressure.
3.6.2 Effects of Piper sarmentosum Roxb. versus positive control on hypertension
Meta-analysis of three studies (Alwi et al., 2018; Ugusman et al., 2020; Firdaus Azmi et al., 2021) on the effect of PS versus captopril, a positive control, indicated no significant difference in SBP (MD = 2.20 mmHg, 95% CI −3.75, 8.15; p = 0.47; Figure 7A), DBP (MD = 1.38 mmHg, 95% CI −7.43, 10.20; p = 0.76; Figure 7B) and MAP (MD = 1.48 mmHg, 95% CI −5.74, 8.70; p = 0.69; Figure 7C). No heterogeneity was observed.
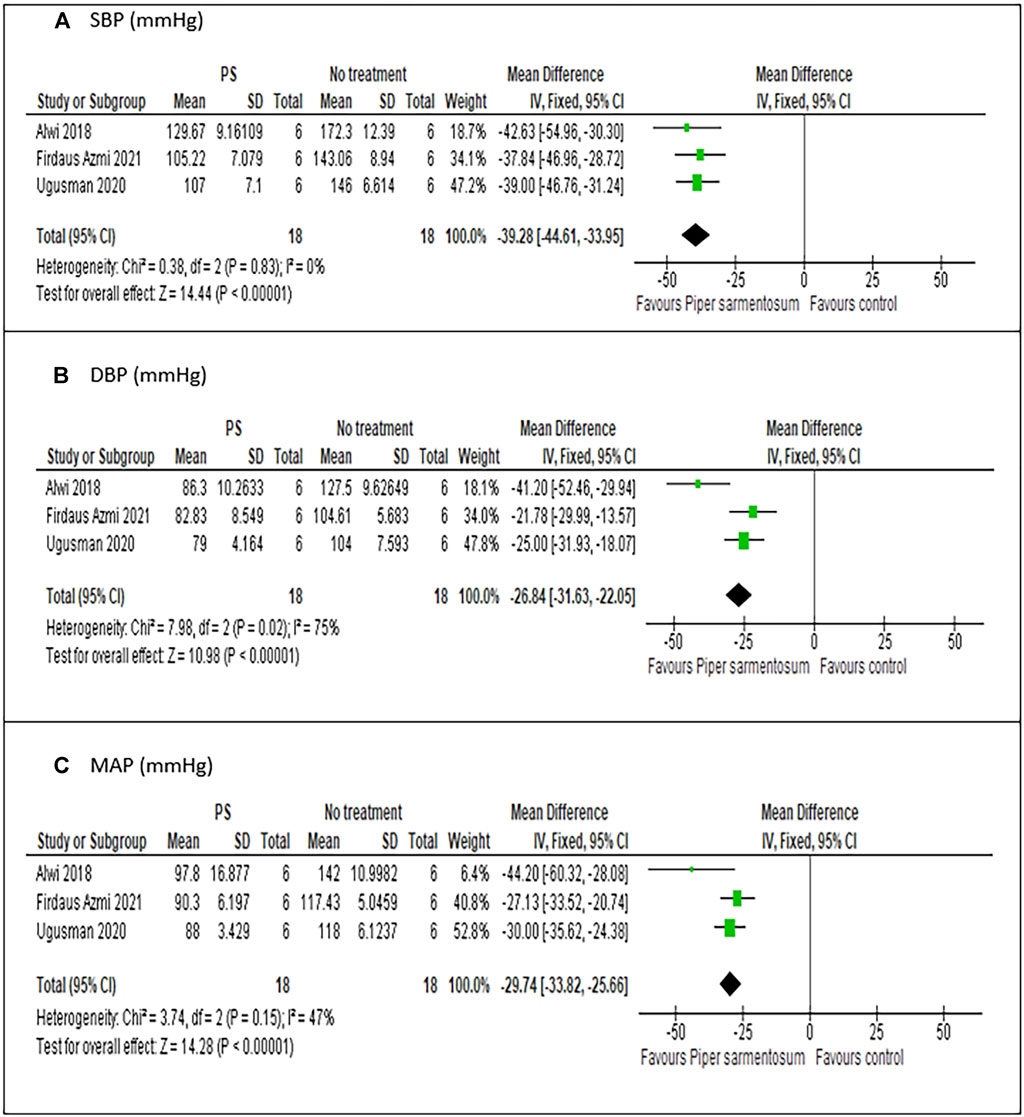
FIGURE 7. Sensitivity analysis of the effects of 500 mg/kg Piper sarmentosum Roxb. versus control on blood pressure: (A) SBP, Systolic blood pressure; (B) DBP, diastolic blood pressure, (C) MAP, mean arterial pressure.
4 Discussion
The present systematic review and meta-analysis suggests that PS has beneficial effects on BP and glycemic control in hypertensive and diabetic animal models.
4.1 Effects of Piper sarmentosum Roxb. on hypertension
PS has demonstrated its antihypertensive effect in various hypertensive animal models including SHR (Mohd Zainudin et al., 2015; Fauzy et al., 2019; Mohd Zainudin et al., 2019), dexamethasone-induced hypertensive rats (Ugusman et al., 2020; Firdaus Azmi et al., 2021) and l-NAME-induced hypertensive rats (Alwi et al., 2018). Our meta-analysis has shown that PS supplementation resulted in significantly reduced SBP, DBP, and MAP by 39.84, 26.688, and 30.56 mmHg, respectively, in hypertensive rat models. The significant reduction is only observed when the groups of hypertensive animals receiving PS were compared to hypertensive rats that do not receive any intervention. No significant difference was observed in BP parameters when the groups of hypertensive animals receiving PS were compared to the groups of animals receiving positive control. A positive control is used in the included studies to control for variability of the experiments as well as for unbiased and objective observation of the effects of studied intervention on BP (Torday and Baluška, 2019). Although the mechanism involved may be different, the analysis has shown evidence that PS has similar effects, if not equal, to captopril in reducing BP parameters.
SHR exhibits many features of human essential hypertension, including increased blood pressure and total peripheral resistance. These features are preceded by oxidative stress, which leads to endothelial dysfunction (Fauzy et al., 2019). MDA, a lipid peroxidation product, is a marker of oxidative stress damage. PS administration to SHR caused a significant reduction in MDA level (Mohd Zainudin et al., 2015), suggesting that the antihypertensive effect of PS was attributed to its antioxidative activity. A previous study also demonstrated that PS reduced oxidative stress by decreasing MDA in endothelial cells (Hafizah et al., 2010). Interestingly, another study showed that PS enhanced the expression of the antioxidant enzymes; superoxide dismutase (SOD), catalase (CAT) and glutathione peroxidase in hydrogen peroxide-induced endothelial cells (Ugusman et al., 2011).
A healthy endothelium releases a plethora of vasoactive factors that contribute to maintaining vascular homeostasis. Endothelial vasoactive mediators such as NO and ET-1 can either decrease or increase the vascular tone, respectively. The imbalance between endothelium-derived vasodilators and vasoconstrictors leads to endothelial dysfunction, which is a precursor of hypertension (Rubanyi, 1993). Endothelial dysfunction occurs when there is reduced NO synthesis, decreased NO bioavailability, or NO antagonism by endothelium-derived vasoconstrictors such as ET-1 (Mordi et al., 2016). The majority of vascular NO is synthesized by eNOS. Meanwhile, decreased bioavailability of NO can be attributed to reactive oxygen species (ROS) that converts NO to peroxynitrite (Mordi et al., 2016).
Dexamethasone-induced hypertensive rats showed reduced eNOS mRNA expression, protein, activity, and serum NO level (Ugusman et al., 2020). Several studies have reported that dexamethasone results in overproduction of ROS that decreases NO bioavailability, which in turn leads to hypertension (de Gennaro Colonna et al., 2002; Rojas et al., 2006; Dubey et al., 2017). Dexamethasone also increases the level of angiotensin-converting enzyme, which is responsible for increasing blood pressure (Fishel et al., 1995). Meanwhile, chronic blockade of NO synthesis by l-NAME is a well-known model of experimental hypertension. It is well established that administration of l-NAME inhibits NO synthesis, causes endothelial dysfunction and vasoconstriction, and thus leads to hypertension (Alwi et al., 2018).
Supplementation of PS successfully reduced the blood pressure of dexamethasone-induced and l-NAME-induced hypertensive rats. This could be attributed to the positive effect of PS on NO production (Alwi et al., 2018; Ugusman et al., 2020; Firdaus Azmi et al., 2021). PS treatment in dexamethasone-induced hypertensive rats increased the eNOS mRNA level, protein level and activity and NO level (Ugusman et al., 2020). Upregulation of eNOS mRNA expression causes more eNOS protein to be synthesized, leading to increased eNOS activity and NO generation. These findings were congruent with a previous study whereby PS increased NO production in hydrogen peroxide-induced endothelial cells by increasing eNOS mRNA expression, protein level and activity (Ugusman et al., 2010). Owing to the antioxidative activity of PS, PS may increase the bioavailability of NO (Mohd Zainudin et al., 2019), as an antioxidant protects NO from the breakdown by ROS (d’Unienville et al., 2021). In SHR, oral administration of a potent antioxidant, lazaroid, improves NO bioavailability and reduces blood pressure (Sorriento et al., 2018).
Competitive inhibitor of eNOS such as ADMA lowers NO synthesis (Hsu and Tain, 2021). Decreased NO also gives rise to unabated ET-1 actions that contribute to vasoconstriction, thereby increasing blood pressure (Böger and Ron, 2005). SHR showed increased ADMA level, which was attenuated by PS supplementation (Mohd Zainudin et al., 2019). Decreased ADMA level is associated with its effective degradation by dimethylarginine dimethylaminohydrolase (DDAH). A previous study reported that PS stimulated DDAH activity in tumor necrosis factor-α-induced endothelial cells, which in turn reduced ADMA level and enhanced NO production (Sundar et al., 2019). Apart from stimulating NO production, PS also reduced ET-1 level in the mesenteric artery of SHR (Fauzy et al., 2019). This observation indicates that PS possesses an anti-vasoconstrictor effect, which contributes to its antihypertensive action.
Even though all the studies reviewed showed positive effects of PS on hypertension, all the studies are animal studies with no clinical trials performed on hypertensive patients. Besides, none of the studies investigated the effect of PS on the renin-angiotensin system, which is an important system that regulates blood pressure. Only two studies on the effect of PS on hypertension that identified the active compounds present in their PS extracts, in which the extracts contain rutin and vitexin (Ugusman et al., 2020; Firdaus Azmi et al., 2021). Oral administration of rutin lowers the blood pressure of l-NAME-induced hypertensive rats (Ganga Raju et al., 2019). Other studies involving phytochemical analysis of PS showed that PS also contains piperine, myricetin and quercetin (Firdaus Azmi et al., 2021). Piperine reduces the blood pressure of l-NAME-induced hypertensive rats (Kumar et al., 2010). Myricetin has also been reported to lower the blood pressure of deoxycorticosterone acetate-salt-hypertensive rats (Borde et al., 2011), whereas quercetin lowers the blood pressure of SHR (Galindo et al., 2012).
4.2 Effects of Piper sarmentosum Roxb. on diabetes mellitus
Hyperglycemia is the hallmark of diabetes secondary to either insufficient insulin secretion, resistance to the action of insulin, or both. Induction of diabetes in laboratory animals using chemical ablation of pancreatic β-cells is the most common experimental model. Alloxan and STZ are the most popular diabetogenic chemicals in diabetes research. However, STZ has notable advantages over alloxan due to its rapid onset, the long half-life, low toxicity and cost-effectiveness (Wszola et al., 2021). STZ is a toxic glucose analogue that is preferentially accumulated in the pancreatic β-cells via the GLUT2 glucose transporters in the plasma membrane.
Following its uptake into beta-cells, STZ triggers oxidative stress, eventually causing the pancreatic β-cells to undergo necrotic cell death, resulting in hypoinsulinemia and hyperglycemia (Choudhari et al., 2017). Additionally, GLUT2 transporters are not only expressed in pancreatic β-cells, but also in the epithelial cells of the kidneys and hepatocytes (Sędzikowska and Szablewski, 2021; Yang et al., 2022). Thus, administration of STZ may result in nephro- and hepatotoxicity along with its potential to damage pancreatic β-cells (Wszola et al., 2021). All six animal studies that investigated the effects of PS on diabetes used a single, high dose of STZ injection (up to 75 mg/kg) to induce pancreatic β-cells damage and diabetes in rats, which mimics type 1 diabetes. None of the studies incorporates the rodent models of type 2 diabetes with underlying insulin resistance, obesity and pancreatic dysfunction, such as the Zucker diabetic fatty rat and db/db mouse (Schwarzer, 2016).
PS supplementation reduced the fasting blood glucose levels (Peungvicha et al., 1998) and urine glucose levels (Thent et al., 2012b) of STZ-induced diabetic rats. One of the mechanisms of the glucose-lowering effect of PS is through improved pancreatic islet function and increased serum insulin (Luangpirom et al., 2014). However, in one study, PS did not cause any significant reduction in the diabetic rats’ fasting blood glucose levels (Hussan et al., 2013). The authors only used fasting blood glucose to measure the rats’ glycemic status without other supporting tests such as the oral glucose tolerance test and HbA1c, thus making the glycemic status assessment less accurate (Bur et al., 2003). Besides, a reduction in body weight was observed in rats following diabetes induction with STZ (Thent et al., 2012b; Hussan et al., 2013). In response to hypoinsulinemia, the body starts burning fat and muscle for energy, causing a reduction in overall body weight (Eleazu et al., 2013). However, the body weights of the diabetic rats were restored with PS supplementation (Thent et al., 2012b). Since PS has been shown to improve pancreatic islet function and increase serum insulin (Thent et al., 2012b; Luangpirom et al., 2014), this might contribute to the improved body weight of the diabetic rats.
PS also exhibits an α-glucosidase inhibitory effect, but not an α-amylase inhibitory effect (Wongsa et al., 2012). In contrast, another in vitro study showed that PS did not possess any α-glucosidase inhibitory effect (Sallehuddin et al., 2020). Alpha-glucosidase and α-amylase inhibitory effects are commonly used to screen the antidiabetic action of natural products (Kittiwisut et al., 2021). Alpha-glucosidase inhibitors act by inhibiting the enzyme α-glucosidase, such as glucoamylase, sucrase, maltase, and isomaltase at the brush border of the intestinal epithelium. This action will block the absorption of carbohydrates in the small intestine, hence reducing postprandial hyperglycemia (Malunga et al., 2016). Meanwhile, α-amylase inhibitors prevent the hydrolysis of α-(1–4)-d-glucosidic linkages in starch, thus reducing carbohydrate digestion and absorption in the gastrointestinal tract and lowering blood glucose (Gong et al., 2020). Most plant-based natural products were effective against either α-amylase or α-glucosidase, with just a few exceptions being effective against both enzymes (Poovitha and Parani, 2016). Besides, α-amylase or α-glucosidase inhibitory assays serve as screening tools for the extract’s antidiabetic activity, in which the results should be further supported by in vivo study findings.
Among the target organs of diabetic complications are the kidney, liver, heart and blood vessels (Wei et al., 2022). PS has been proven to attenuate the damaging effects of diabetes in the kidney, liver, heart and aorta (Thent et al., 2012a; Thent et al., 2012b; Hussan et al., 2013; Thent and Das 2015). Significant alterations in the liver weight, histology and oxidative stress markers are linked to STZ-induced diabetes (Rodríguez et al., 2018; Yazdi et al., 2019). Treatment with PS also increased the liver weight and reversed diabetes-induced degenerative changes in the liver tissues; an effect attributed to its antioxidative action (Thent and Das, 2015). Inflammation in response to intermittent or chronic hyperglycemia is involved in the initiation and progression of diabetic nephropathy (Amorim et al., 2019; Donate-Correa et al., 2021). Diabetic rats have increased kidney weight due to renal hypertrophy, with histological changes of diabetic nephropathy such as contracted glomeruli with widened urinary spaces, marked inflammatory cell infiltration in the renal cortex and medulla, and glomerular membrane thickening (Hussan et al., 2013). Even though treatment with PS did not change the kidney weight, it attenuated the histological changes in the diabetic rat’s kidney as evidenced by less contracted glomeruli, mild inflammatory cells infiltration, reduced urinary space size and absence of glomerular membrane thickening. This could be due to the anti-inflammatory effect of PS (Hussan et al., 2013). Furthermore, PS supplementation reduced the degenerative changes and restored the ultrastructural integrity of the heart and aorta of diabetic rats under light and electron microscopic analyses (Thent et al., 2012a; Thent et al., 2012b). Overall, PS attenuates the complications of diabetes in the kidney, liver, heart and blood vessels through its antioxidative, anti-inflammatory and glucose-lowering effects.
Even though most of the studies reviewed showed positive effects of PS on diabetes, all studies are preclinical studies with no clinical trials performed on diabetic patients. Besides, none of the studies used type 2 diabetes model, which is more common in the society. Only two studies on the effect of PS on diabetes that identified the active compounds present in their PS extracts, in which the extracts contain catechin, naringin (Sallehuddin et al., 2020), caffeic acid and p-coumaric acid (Wongsa et al., 2012). Treatment with catechin lowers blood glucose levels and increases the activity of antioxidant enzymes such as SOD, CAT and glutathione-S-transferase in diabetic rats (Gong et al., 2020). In another study, naringin administration decreased the plasma glucose of diabetic rats as measured using OGTT (Ahmed et al., 2012). Caffeic acid reduces fasting blood glucose when given to alloxan-induced diabetic rats (Oršolić et al., 2021), whereas p-coumaric acid improves glycemic status and increases plasma insulin level in STZ-induced diabetic rats (Amalan and Vijayakumar, 2015).
4.3 Strength and limitation of the study
To the best of our knowledge, this is the first systematic review and meta-analysis investigating the effects of PS on hypertension and diabetes. The systematic literature search ensures all relevant articles were identified whilst at the same time minimizes selection bias. In addition, the meta-analyses performed enables objective evaluation of the effect of PS on BP parameters. However, the current review is not without its limitation. The small number of included studies may have influenced the effect estimates generated in our meta-analysis. Therefore, interpretation of results should be made with caution. Moreover, the various approach used in reporting the results did not allow us to objectively report the effect of PS on glycemic control. Nevertheless, most of the studies reported uniform effects of PS on diabetes mellitus.
5 Conclusion
Overall, PS showed promising antihypertensive and antidiabetic effects. However, all the studies reviewed are preclinical studies with no randomized clinical trials conducted to investigate the antihypertensive and antidiabetic effects of PS in human subjects. Therefore, further clinical studies are recommended to validate the antihypertensive and antidiabetic activities of PS to prepare evidence-based formulations.
Data availability statement
The original contributions presented in the study are included in the article/Supplementary Material, further inquiries can be directed to the corresponding authors.
Author contributions
AU, JM, AA, and AAH. contributed to conception and design; AU, NR, and NO contributed to data acquisition; AU, NC, and NO were involved in analysis, interpretation and drafting the manuscript; AU, NC, JM, AA, and AAH revise the manuscript critically for important intellectual content. The version to be published has received final approval from all authors.
Funding
This study was funded by Faculty of Medicine, Universiti Kebangsaan Malaysia (Grant code: FF-2020-042) and Ministry of Higher Education Malaysia Fundamental Research Grant Scheme (FRGS/1/2019/SKK08/UKM/02/8).
Acknowledgments
We would like to thank Associate Prof. Dr. Siti Fatimah Ibrahim for her technical assistance.
Conflict of interest
The authors declare that the research was conducted in the absence of any commercial or financial relationships that could be construed as a potential conflict of interest.
Publisher’s note
All claims expressed in this article are solely those of the authors and do not necessarily represent those of their affiliated organizations, or those of the publisher, the editors and the reviewers. Any product that may be evaluated in this article, or claim that may be made by its manufacturer, is not guaranteed or endorsed by the publisher.
References
Ahmed, O. M., Mahmoud, A. M., Abdel-Moneim, A., and Ashour, M. B. (2012). Antidiabetic effects of hesperidin and naringin in type 2 diabetic rats. Diabetol. Croat. 41 (2), 53–67.
Alwi, N. A. N. M., Zakaria, Z., Karim, A. A. H., Nordin, N. A. M. M., and Ugusman, A. (2018). Antihypertensive effect of Piper sarmentosum in L-NAME-induced hypertensive rats. Sains Malays. 47 (10), 2421–2428. doi:10.17576/jsm-2018-4710-18
Amalan, V., and Vijayakumar, N. (2015). Antihyperglycemic effect of P-coumaric acid on streptozotocin induced diabetic rats. Indian J. Appl. Res. 5 (1), 1–4.
Amorim, R. G., Guedes, G. D. S., Vasconcelos, S. M. D. L., and Santos, J. C. D. F. (2019). Kidney disease in diabetes mellitus: cross-linking between hyperglycemia, redox imbalance and inflammation. Arq. Bras. Cardiol. 112 (5), 577–587. doi:10.5935/abc.20190077
Amran, A. A., Zakaria, Z., Othman, F., Das, S., Raj, S., and Nordin, N. A. M. M. (2010). Aqueous extract of Piper sarmentosum decreases atherosclerotic lesions in high cholesterolemic experimental rabbits. Lipids Health Dis. 9 (44), 44–46. doi:10.1186/1476-511X-9-44
Amran, A. A., Zakaria, Z., Othman, F., Das, S., Al-Mekhlafi, H. M., and Nordin, N. A. M. (2011). Changes in the vascular cell adhesion molecule-1, intercellular adhesion molecule-1 and C-reactive protein following administration of aqueous extract of Piper sarmentosum on experimental rabbits fed with cholesterol diet. Lipids Health Dis. 10 (2), 2–8. doi:10.1186/1476-511X-10-2
Atiax, E., Ahmad, F., Sirat, H. M., and Arbain, D. (2011). Antibacterial activity and cytotoxicity screening of sumatran kaduk (Piper sarmentosum Roxb). Iran. J. Pharmacol. Ther. 10 (1), 1–5.
Azlina, A. A., Farihah, S., Qodriyah, M. S., and Nur Azlina, M. F. (2009). Effects of Piper sarmentosum (kaduk) water extract on adiponectin and blood glucose levels in ovariectomy-induced obese rats. Res. J. Med. Plant 3 (3), 109–115. doi:10.3923/rjmp.2009.109.115
Böger, R. H., and Ron, E. S. (2005). L-Arginine improves vascular function by overcoming deleterious effects of ADMA, a novel cardiovascular risk factor. Altern. Med. Rev. 10 (1), 14–23.
Bokesch, H. R., Gardella, R. S., Rabe, D. C., Bottaro, D. P., Linehan, W. M., McMahon, J. B., et al. (2011). A new hypoxia inducible factor-2 inhibitory pyrrolinone alkaloid from roots and stems of Piper sarmentosum. Chem. Pharm. Bull. 59 (9), 1178–1179. doi:10.1248/cpb.59.1178
Bonadonna, R. C., Saccomani, M. P., del Prato, S., Bonora, E., DeFronzo, R. A., and Cobelli, C. (1998). Role of tissue-specific blood flow and tissue recruitment in insulin- mediated glucose uptake of human skeletal muscle. Circulation 98, 234–241. doi:10.1161/01.cir.98.3.234
Borde, P., Mohan, M., and Kasture, S. (2011). Effect of myricetin on deoxycorticosterone acetate (DOCA)-salt-hypertensive rats. Nat. Prod. Res. 25 (16), 1549–1559. doi:10.1080/14786410903335190
Bur, A., Herkner, H., Woisetschläger, C., Vlcek, M., Derhaschnig, U., and Hirschl, M. M. (2003). Is fasting blood glucose a reliable parameter for screening for diabetes in hypertension? Am. J. Hypertens. 16 (4), 297–301. doi:10.1016/S0895-7061(02)03273-9
Chanwitheesuk, A., Teerawutgulrag, A., and Rakariyatham, N. (2005). Screening of antioxidant activity and antioxidant compounds of some edible plants of Thailand. Food Chem. 92 (3), 491–497. doi:10.1016/j.foodchem.2004.07.035
Chaveerach, A., Mokkamul, P., Sudmoon, R., and Tanee, T. (2006). Ethnobotany of the genus piper (Piperaceae) in Thailand. Ethnobot. Res. App. 4, 223–231. doi:10.17348/era.4.0.223-231
Choudhari, V. P., Gore, K. P., and Pawar, A. T. (2017). Antidiabetic, antihyperlipidemic activities and herb-drug interaction of a polyherbal formulation in streptozotocin induced diabetic rats. J. Ayurveda Integr. Med. 8 (4), 218–225. doi:10.1016/j.jaim.2016.11.002
d’Unienville, N. M. A., Blake, H. T., Coates, A. M., Hill, A. M., Nelson, M. J., and Buckley, J. D. (2021). Effect of food sources of nitrate, polyphenols, L-arginine and L-citrulline on endurance exercise performance: a systematic review and meta-analysis of randomised controlled trials. J. Int. Soc. Sports Nutr. 18 (76), 76–28. doi:10.1186/s12970-021-00472-y
de Gennaro Colonna, V., Rossoni, G., Rigamonti, A. E., Bonomo, S., Manfredi, B., Berti, F., et al. (2002). Enalapril and quinapril improve endothelial vasodilator function and aortic eNOS gene expression in L-NAME-treated rats. Eur. J. Pharmacol. 450 (1), 61–66. doi:10.1016/S0014-2999(02)02046-0
Donate-Correa, J., Ferri, C. M., Sánchez-Quintana, F., Pérez-Castro, A., González-Luis, A., Martín-Núñez, E., et al. (2021). Inflammatory cytokines in diabetic kidney disease: pathophysiologic and therapeutic implications. Front. Med. 22 (7), 628289–9. doi:10.3389/fmed.2020.628289
Dubey, H., Singh, A., Patole, A. M., and Tenpe, C. R. (2017). Antihypertensive effect of allicin in dexamethasone-induced hypertensive rats. Integr. Med. Res. 6 (1), 60–65. doi:10.1016/j.imr.2016.12.002
Eleazu, C. O., Iroaganachi, M., Okafor, P. N., Ijeh, I. I., and Eleazu, K. C. (2013). Ameliorative potentials of ginger (Z. Officinale roscoe) on relative organ weights in streptozotocin induced diabetic rats. Int. J. Biomed. Sci. 9 (2), 82–90.
Emdin, C. A., Anderson, S. G., Woodward, M., and Rahimi, K. (2015). Usual blood pressure and risk of new-onset diabetes evidence from 4.1 million adults and a meta-analysis of prospective studies. J. Am. Coll. Cardiol. 66 (14), 1552–1562. doi:10.1016/j.jacc.2015.07.059
Farhana Syed Ab Rahman, S. (2016). Piper sarmentosum Roxb: a mini review of ethnobotany, phytochemistry and Pharmacology. J. Anal. Pharm. Res. 2 (5), 12–14. doi:10.15406/japlr.2016.02.00031
Fauzy, F. H., Zainudin, M. M., Ismawi, H. R., and Elshami, T. F. T. (2019). Piper sarmentosum leaves aqueous extract attenuates vascular endothelial dysfunction in spontaneously hypertensive rats. Evid. Based Complement. Altern. Med., 2019, 7198592–7198598. doi:10.1155/2019/7198592
Firdaus Azmi, M., Aminuddin, A., Azdina Jamal, J., Hamid, A. A., and Ugusman, A. (2021). Quantified Piper sarmentosum Roxb. Leaves aqueous leaf extract and its antihypertensive effect in dexamethasone-induced hypertensive rats. Sains Malays. 50 (1), 171–179. doi:10.17576/jsm-2021-5001-17
Fishel, R. S., Eisenberg, S., Shai, S. Y., Redden, R. A., Bernstein, K. E., and Berk, B. C. (1995). Glucocorticoids induce angiotensin-converting enzyme expression in vascular smooth muscle. Hypertension 25 (3), 343–349. doi:10.1161/01.HYP.25.3.343
Galindo, P., Rodriguez-Gómez, I., González-Manzano, S., Dueñas, M., Jiménez, R., Menéndez, C., et al. (2012). Glucuronidated quercetin lowers blood pressure in spontaneously hypertensive rats via deconjugation. PLoS ONE 7 (3), e32673–8. doi:10.1371/journal.pone.0032673
Ganga Raju, M., Prem Prasad, G., and Suvarchala Reddy, N. (2019). Antihypertensive effect of rutin: pharmacological and computational approach. Asian J. Pharm. Clin. Res. 12 (8), 87–92. doi:10.22159/ajpcr.2019.v12i18.34118
Gong, L., Feng, D., Wang, T., Ren, Y., Liu, Y., and Wang, J. (2020). Inhibitors of α-amylase and α-glucosidase: potential linkage for whole cereal foods on prevention of hyperglycemia. Food Sci. Nutr. 8 (12), 6320–6337. doi:10.1002/fsn3.1987
Hafizah, A. H., Zaiton, Z., Zulkhairi, A., Mohd Ilham, A., Nor Anita, M. M. N., and Zaleha, A. M. (2010). Piper sarmentosum as an antioxidant on oxidative stress in human umbilical vein endothelial cells induced by hydrogen peroxide. J. Zhejiang Univ. Sci. B 11 (5), 357–365. doi:10.1631/jzus.B0900397
Han, G. (1995). PAF receptor antagonistic principles from Chinese traditional drugs. Prog. Nat. Sci. 5, 299–306.
Higgins, J. P. T., Thompson, S. G., Deeks, J. J., and Altman, D. G. (2003). Measuring inconsistency in meta-analyses. BMJ 327 (7414), 557–560. doi:10.1136/bmj.327.7414.557
Higgins, J., Savović, J., Page, M. J., and Sterne, J. A. C. (2019). RoB 2: A revised Cochrane risk-of-bias tool for randomized trials - cribsheet. BMJ. Available at: https://methods.cochrane.org/bias/resources/rob-2-revised-cochrane-risk-bias-tool-randomized-trials (Accessed May 10, 2020).
Hooijmans, C. R., Rovers, M. M., de Vries, R. B. M., Leenaars, M., Ritskes-Hoitinga, M., and Langendam, M. W. (2014). SYRCLE’s risk of bias tool for animal studies. BMC Med. Res. Methodol. 14 (1), 43–49. doi:10.1186/1471-2288-14-43
Hsu, C. N., and Tain, Y. L. (2021). Asymmetric dimethylarginine (ADMA) in pediatric renal diseases: from pathophysiological phenomenon to clinical biomarker and beyond. Children 8 (10), 837–914. doi:10.3390/children8100837
Hussain, K., Ismail, Z., Sadikun, A., and Ibrahim, P. (2008). Analysis of proteins, polysaccharides, glycosaponins contents of Piper sarmentosum Roxb and anti-TB evaluation for bio-enhancing/interaction effects of leaf extracts with isoniazid (INH). Nat. Prod. Radiance 7 (5), 402–408.
Hussain, K., Hashmi, F. K., Latif, A., Ismail, Z., and Sadikun, A. (2012). A review of the literature and latest advances in research of Piper sarmentosum. Pharm. Biol. 50 (8), 1045–1052. doi:10.3109/13880209.2011.654229
Hussain, K., Ismail, Z., Sadikun, A., Ibrahim, P., and Malik, A. (2015). In vitro antiangiogenesis activity of standardized extracts of Piper sarmentosum Roxb. J. Ris. Kim. 1 (2), 146–150. doi:10.25077/jrk.v1i2.50
Hussan, F., Bt Mat Zin, N. N., Zullkefli, M. R., Choon, Y. S., Bt Abdullah, N. A., and Lin, T. S. (2013). Piper sarmentosum water extract attenuates diabetic complications in streptozotocin induced sprague-dawley rats. Sains Malays 42 (11), 1605–1612.
Ismail, S. M., Hui, C. K., Aminuddin, A., and Ugusman, A. (2018). Piper sarmentosum as an antioxidant: a systematic review. Sains Malays. 47 (10), 2359–2368. doi:10.17576/jsm-2018-4710-12
Isomaa, B., Almgren, P., Tuomi, T., Forsén, B., Lahti, K., Nissén, M., et al. (2001). Cardiovascular morbidity and mortality associated with the metabolic syndrome. Diabetes Care 2424 (4), 683683–689689. doi:10.2337/diacare.24.4.683
JBI (2020). Critical appraisal tools. Joanna Briggs Institute (JBI). Available at: https://jbi.global/critical-appraisal-tools (Accessed March 15, 2022).
Kawasoe, S., Maruguchi, Y., Kajiya, S., Uenomachi, H., Miyata, M., Kawasoe, M., et al. (2017). Mechanism of the blood pressure-lowering effect of sodium-glucose cotransporter 2 inhibitors in obese patients with type 2 diabetes. BMC Pharmacol. Toxicol. 18 (1), 23–10. doi:10.1186/s40360-017-0125-x
Kittiwisut, S., Amnuoypol, S., Pathompak, P., and Setharaksa, S. (2021). α-Glucosidase and α-amylase inhibitory effects with anti-oxidative activity of tetracera loureiri (finet & gagnep.) pierre ex craib leaf extracts. Pharm. Sci. Asia 48 (2), 175–184. doi:10.29090/psa.2021.02.19.125
Kumar, S., Saravana Kumar, M., and Raja, B. (2010). Efficacy of piperine, an alkaloidal constituent of pepper on nitric oxide, antioxidants and lipid peroxidation markers in L-NAME induced hypertensive rats. Int. J. Res. Pharm. Sci. 1 (3), 300–307. doi:10.1002/cbf.1331
Luangpirom, A., Kourchampa, W., and Somsapt, P. (2014). Evaluation of hypoglycemic properties and fertility effect of Piper sarmentosum Roxb. Aqueous leaf extract in streptozotocin induced diabetic mice. Int. J. Phytomedicine 6 (3). doi:10.5138/ijpm.v6i3.1496
Malunga, L. N., Eck, P., and Beta, T. (2016). Inhibition of intestinal α -glucosidase and glucose absorption by feruloylated arabinoxylan mono- and oligosaccharides from corn bran and wheat aleurone. J. Nutr. Metab. 21, 1932532–1932539. doi:10.1155/2016/1932532
Mathew, S. P., Mohandas, A., and Nair, G. M. (2004). Piper sarmentosum Roxb - an addition to the flora of andaman islands. Curr. Sci. 41, 147–156.
Meigs, J. B., Hu, F. B., Rifai, N., and Manson, J. A. E. (2004). Biomarkers of endothelial dysfunction and risk of type 2 diabetes mellitus. J. Am. Med. Assoc. 291 (16), 1978–1986. doi:10.1001/jama.291.16.1978
Miles, P. D. G., Levisetti, M., Reichart, D., Khoursheed, M., Moossa, A. R., and Olefsky, J. M. (1995). Kinetics of insulin action in vivo: identification of rate-limiting steps. Diabetes 44 (8), 947–953. doi:10.2337/diab.44.8.947
Mohamad Asri, S. F., Mohd Ramli, E. S., Soelaiman, I. N., Mat Noh, M. A., Abdul Rashid, A. H., and Suhaimi, F. (2016). Piper sarmentosum effects on 11β-hydroxysteroid dehydrogenase type 1 enzyme in serum and bone in rat model of glucocorticoid-induced osteoporosis. Molecules 21 (11), 15233–E1610. doi:10.3390/molecules21111523
Mohd Zainudin, M., Zakaria, Z., Megat Mohd Nordin, N. A., and Othman, F. (2013). Does oral ingestion of Piper sarmentosum cause toxicity in experimental animals? Evid. Based Complement. Altern. Med. 2013, 705950–705959. doi:10.1155/2013/705950
Mohd Zainudin, M., Zakaria, Z., and Megat Mohd Nordin, N. A. (2015). The use of Piper sarmentosum leaves aqueous extract (KadukmyTM) as antihypertensive agent in spontaneous hypertensive rats. BMC Complement. Altern. Med. 15 (54), 54–10. doi:10.1186/s12906-015-0565-z
Mohd Zainudin, M., Hashim Fauzy, F., Razak Tariq, A., Elshami, T., Zainudin, M. M., Tft, E., et al. (2019). Factors regulating nitric oxide production in spontaneously hypertensive rats treated with Piper sarmentosum aqueous extract. IMJM 18 (3), 1–7. doi:10.31436/imjm.v18i3.199
Mordi, I., Mordi, N., Delles, C., and Tzemos, N. (2016). Endothelial dysfunction in human essential hypertension. J. Hypertens. 34 (8), 1464–1472. doi:10.1097/HJH.0000000000000965
Nibouche, W. N., and Biad, A. (2016). Arterial hypertension at the time of diagnosis of type 2 diabetes in adults. Ann. Cardiol. Angeiol. 65 (3), 152–158. doi:10.1016/j.ancard.2016.04.017
Nur Azlina, M. F., Qodriyah, H. M. S., Hamizah, A. H., and Kamisah, Y. (2014). Kesan ekstrak metanol Piper sarmentosum terhadap kecederaan oksidatif hepar tikus aruhan parasetamol. Sains Malays 43 (3), 415–421.
Oguntibeju, O. O. (2019). Type 2 diabetes mellitus, oxidative stress and inflammation: examining the links. Int. J. Physiol. Pathophysiol. Pharmacol. 11 (3), 45–63.
Ohishi, M. (2018). Hypertension with diabetes mellitus: physiology and pathology. Hypertens. Res. 41 (6), 389–393. doi:10.1038/s41440-018-0034-4
Oršolić, N., Sirovina, D., Odeh, D., Gajski, G., Balta, V., Šver, L., et al. (2021). Efficacy of caffeic acid on diabetes and its complications in the mouse. Molecules 26 (11), 3262–3325. doi:10.3390/molecules26113262
Othman, N. S., Che Roos, N. A., Hamid, A. A., Aminuddin, A., Kumar, J., and Ugusman, A. (2022). Effects of Piper sarmentosum on diabetes mellitus and hypertension: a systematic review. Inplasy Protoc., 1–3. doi:10.37766/inplasy2022.4.0020
Peungvicha, P., Thirawarapan, S., Temsiririrkkul, R., Watanabe, H., Kumar Prasain, J., and Kadota, S. (1998). Hypoglycemic effect of the water extract of Piper sarmentosum in rats. J. Ethnopharmacol. 60 (1), 27–32. doi:10.1016/S0378-8741(97)00127-X
Poovitha, S., and Parani, M. (2016). In vitro and in vivo α-amylase and α-glucosidase inhibiting activities of the protein extracts from two varieties of bitter gourd. BMC Complement. Altern. Med. 16 (1), 185–189. doi:10.1186/s12906-016-1085-1
Ridtitid, W., Ruangsang, P., Reanmongkol, W., and Wongnawa, M. (2007). Studies of the anti-inflammatory and antipyretic activities of the methanolic extract of Piper sarmentosum Roxb. Leaves in rats. Songklanakarin J. Sci. Technol. 29 (6), 1519–1526.
Rodríguez, V., Plavnik, L., and Tolosa de Talamoni, N. (2018). Naringin attenuates liver damage in streptozotocin-induced diabetic rats. Biomed. Pharmacother. 105, 95–102. doi:10.1016/j.biopha.2018.05.120
Rojas, A., Figueroa, H., Re, L., and Morales, M. A. (2006). Oxidative stress at the vascular wall. Mechanistic and pharmacological aspects. Arch. Med. Res. 37 (4), 436–448. doi:10.1016/j.arcmed.2005.11.012
Rubanyi, G. M. (1993). The role of endothelium in cardiovascular homeostasis and diseases. J. Cardiovasc. Pharmacol. 22 (4), S1–S14. doi:10.1097/00005344-199322004-00002
Sabadashka, M., Hertsyk, D., Strugała-Danak, P., Dudek, A., Kanyuka, O., Kucharska, A. Z., et al. (2021). Anti-diabetic and antioxidant activities of red wine concentrate enriched with polyphenol compounds under experimental diabetes in rats. Antioxidants 10 (9), 1399–1424. doi:10.3390/antiox10091399
Sallehuddin, N. A., Abdul-Hamid, A., Salleh, S. Z., Abdul-Majid, N., Halim, H. H., Ramli, N. S., et al. (2020). Ergogenic, anti-diabetic and antioxidant attributes of selected Malaysian herbs: Characterisation of flavonoids and correlation of functional activities. Int. Food Res. J. 27 (1), 197–207.
Schwarzer, M. (2016). “Models to investigate cardiac metabolism,” in The scientist’s guide to cardiac metabolism (Cambridge: Academic Press), 103–122. doi:10.1016/b978-0-12-802394-5.00008-x
Sędzikowska, A., and Szablewski, L. (2021). Human glucose transporters in renal glucose homeostasis. Int. J. Mol. Sci. 22 (24), 13522–13617. doi:10.3390/ijms222413522
Serné, E. H., Stehouwer, C. D. A., ter Maaten, J. C., ter Wee, P. M., Rauwerda, J. A., Donker, A. J. M., et al. (1999). Microvascular function relates to insulin sensitivity and blood pressure in normal subjects. Circulation 99 (7), 896–902. doi:10.1161/01.CIR.99.7.896
Shiny, A., Regin, B., Mohan, V., and Balasubramanyam, M. (2016). Coordinated augmentation of NFAT and NOD signaling mediates proliferative VSMC phenotype switch under hyperinsulinemia. Atherosclerosis 246, 257–266. doi:10.1016/j.atherosclerosis.2016.01.006
Sorriento, D., De Luca, N., Trimarco, B., and Iaccarino, G. (2018). The antioxidant therapy: new insights in the treatment of hypertension. Front. Physiol. 9, 258–311. doi:10.3389/fphys.2018.00258
Subramaniam, V., Adenan, M. I., Ahmad, A. R., and Sahdan, R. (2003). Natural antioxidants: Piper sarmentosum (kadok) and Morinda elliptica (mengkudu). Malays. J. Nutr. 9 (1), 41–51.
Sumazian, Y., Syahida, A., Hakiman, M., and Maziah, M. (2010). Antioxidant activities, flavonoids, ascorbic acid and phenolic contents of Malaysian vegetables. J. Med. Plant Res. 4 (10), 881–890. doi:10.5897/JMPR10.011
Sun, H. J., Wu, Z. Y., Nie, X. W., and Bian, J. S. (2020). Role of endothelial dysfunction in cardiovascular diseases: the link between inflammation and hydrogen sulfide. Front. Pharmacol. 10, 1568–1615. doi:10.3389/fphar.2019.01568
Sundar, U. M., Ugusman, A., Chua, H. K., Latip, J., and Aminuddin, A. (2019). Piper sarmentosum promotes endothelial nitric oxide production by reducing asymmetric dimethylarginine in tumor necrosis factor-Α-induced human umbilical vein endothelial cells. Front. Pharmacol. 10, 1033–1039. doi:10.3389/fphar.2019.01033
Tain, Y. L., and Hsu, C. N. (2022). Oxidative stress-induced hypertension of developmental origins: preventive aspects of antioxidant therapy. Antioxidants 11 (3), 511–520. doi:10.3390/antiox11030511
Tatsumi, Y., and Ohkubo, T. (2017). Hypertension with diabetes mellitus: significance from an epidemiological perspective for Japanese. Hypertens. Res. 40 (9), 795–806. doi:10.1038/hr.2017.67
The Cochrane Collaboration (2020). Cochrane’s Collaboration Review Manager (RevMan). Version 5.4 [Computer program].
Thent, Z. C., and Das, S. (2015). Piper sarmentosum maintains blood pressure and morphological integrity of liver in type 1 diabetic rats. Int. J. Pharma Med. Biol. Sci. 4 (1), 24–28.
Thent, Z. C., Lin, T. S., Das, S., and Zakaria, Z. (2012a). Histological changes in the heart and the proximal aorta in experimental diabetic rats fed with Piper sarmentosum. Afr. J. Tradit. Complement. Altern. Med. 9 (3), 396–404. doi:10.4314/ajtcam.v9i3.15
Thent, Z. C., Seong Lin, T., Das, S., and Zakaria, Z. (2012b). Effect of Piper sarmentosum extract on the cardiovascular system of diabetic sprague-dawley rats: electron microscopic study. Evid. Based. Complement. Altern. Med. 2012, 628750–628759. doi:10.1155/2012/628750
Torday, J. S., and Baluška, F. (2019). Why control an experiment?: from empiricism, via consciousness, toward implicate order. EMBO Rep. 20 (10), e49110–4. doi:10.15252/embr.201949110
Tsimihodimos, V., Gonzalez-Villalpando, C., Meigs, J. B., and Ferrannini, E. (2018). Hypertension and diabetes mellitus coprediction and time trajectories. Hypertension 71 (3), 422–428. doi:10.1161/HYPERTENSIONAHA.117.10546
Tune, J. D., Goodwill, A. G., Sassoon, D. J., and Mather, K. J. (2017). Cardiovascular consequences of metabolic syndrome. Transl. Res. 183, 57–70. doi:10.1016/j.trsl.2017.01.001
Ugusman, A., Zakaria, Z., Hui, C. K., and Nordin, N. A. M. M. (2010). Piper sarmentosum increases nitric oxide production in oxidative stress: a study on human umbilical vein endothelial cells. Clinics 65 (7), 709–714. doi:10.1590/S1807-59322010000700010
Ugusman, A., Zakaria, Z., Hui, C. K., and Megat Mohd Nordin, N. A. (2011). Piper sarmentosum inhibits ICAM-1 and Nox4 gene expression in oxidative stress-induced human umbilical vein endothelial cells. BMC Complement. Altern. Med. 11 (1), 31–39. doi:10.1186/1472-6882-11-31
Ugusman, A., Md Fadze, N., Hamid, A. A., Asmawi, Z., and Aminuddin, A. (2020). Piper sarmentosum attenuates dexamethasone-induced hypertension by stimulating endothelial nitric oxide synthase. JRP 24 (1), 1–9. doi:10.35333/jrp.2020.122
Wan, E. Y. F., Yu, E. Y. T., Chin, W. Y., Fung, C. S. C., Fong, D. Y. T., Choi, E. P. H., et al. (2018). Effect of achieved systolic blood pressure on cardiovascular outcomes in patients with type 2 diabetes: a population-based retrospective cohort study. Diabetes Care 41 (6), 1134–1141. doi:10.2337/dc17-2443
Wei, J., Tian, J., Tang, C., Fang, X., Miao, R., Wu, H., et al. (2022). The influence of different types of diabetes on vascular complications. J. Diabetes Res. 2022, 3448618–3448712. doi:10.1155/2022/3448618
Wong, W. T., Tian, X. Y., and Huang, Y. (2013). Endothelial dysfunction in diabetes and hypertension: cross talk in RAS, BMP4, and ROS-dependent COX-2-derived prostanoids. J. Cardiovasc. Pharmacol. 61 (3), 204–214. doi:10.1097/FJC.0b013e31827fe46e
Wongsa, P., Chaiwarit, J., and Zamaludien, A. (2012). In vitro screening of phenolic compounds, potential inhibition against α-amylase and α-glucosidase of culinary herbs in Thailand. Food Chem. 131 (3), 964–971. doi:10.1016/j.foodchem.2011.09.088
Wszola, M., Klak, M., Kosowska, A., Tymicki, G., Berman, A., Adamiok-Ostrowska, A., et al. (2021). Streptozotocin-induced diabetes in a mouse model (Balb/c) is not an effective model for research on transplantation procedures in the treatment of type 1 diabetes. Biomedicines 9 (12), 1790–1818. doi:10.3390/biomedicines9121790
Yamazaki, D., Hitomi, H., and Nishiyama, A. (2018). Hypertension with diabetes mellitus complications. Hypertens. Res. 41, 147–156. doi:10.1038/s41440-017-0008-y
Yang, M., Chen, Y., Vagionitis, S., Körtvely, E., Ueffing, M., Schmachtenberg, O., et al. (2022). Expression of glucose transporter-2 in murine retina: Evidence for glucose transport from horizontal cells to photoreceptor synapses. J. Neurochem. 160 (2), 283–296. doi:10.1111/jnc.15533
Yazdi, H. B., Hojati, V., Shiravi, A., Hosseinian, S., Vaezi, G., and Hadjzadeh, M. A. R. (2019). Liver dysfunction and oxidative stress in streptozotocin-induced diabetic rats: Protective role of artemisia turanica. J. Pharmacopuncture 22 (2), 109–114. doi:10.3831/KPI.2019.22.014
Zainal Ariffin, S. H., Wan Omar, W. H. H., Safian, M. F., Ariffin, Z. Z., Senafi, S., and Megat Abdul Wahab, R. (2009). Intrinsic anticarcinogenic effects of Piper sarmentosum ethanolic extract on a human hepatoma cell line. Cancer Cell Int. 9 (6), 6–9. doi:10.1186/1475-2867-9-6
Zakaria, Z. A., Patahuddin, H., Mohamad, A. S., Israf, D. A., and Sulaiman, M. R. (2010). In vivo anti-nociceptive and anti-inflammatory activities of the aqueous extract of the leaves of Piper sarmentosum. J. Ethnopharmacol. 128 (1), 42–48. doi:10.1016/j.jep.2009.12.021
Keywords: antihypertensive, hypertension, Piper sarmentosum, diabetes mellitus, antidiabetic
Citation: Othman NS, Che Roos NA, Aminuddin A, Murthy JK, A. Hamid A and Ugusman A (2022) Effects of Piper sarmentosum Roxb. on hypertension and diabetes mellitus: A systematic review and meta-analysis. Front. Pharmacol. 13:976247. doi: 10.3389/fphar.2022.976247
Received: 23 June 2022; Accepted: 20 July 2022;
Published: 25 August 2022.
Edited by:
Ahmad Nazrun Shuid, MARA University of Technology, MalaysiaReviewed by:
Aminu Mohammed, Ahmadu Bello University, NigeriaMaizura Mohd Zainudin, International Islamic University Malaysia, Malaysia
Nurul Alimah Abdul Nasir, University Teknologi MARA, Malaysia
Ahmad Naqib Shuid, Universiti Sains Malaysia, Malaysia
Copyright © 2022 Othman, Che Roos, Aminuddin, Murthy, A. Hamid and Ugusman. This is an open-access article distributed under the terms of the Creative Commons Attribution License (CC BY). The use, distribution or reproduction in other forums is permitted, provided the original author(s) and the copyright owner(s) are credited and that the original publication in this journal is cited, in accordance with accepted academic practice. No use, distribution or reproduction is permitted which does not comply with these terms.
*Correspondence: Azizah Ugusman, ZHIuYXppemFoQHBwdWttLnVrbS5lZHUubXk=; Adila A. Hamid, YWRpbGFoYW1pZEBwcHVrbS51a20uZWR1Lm15