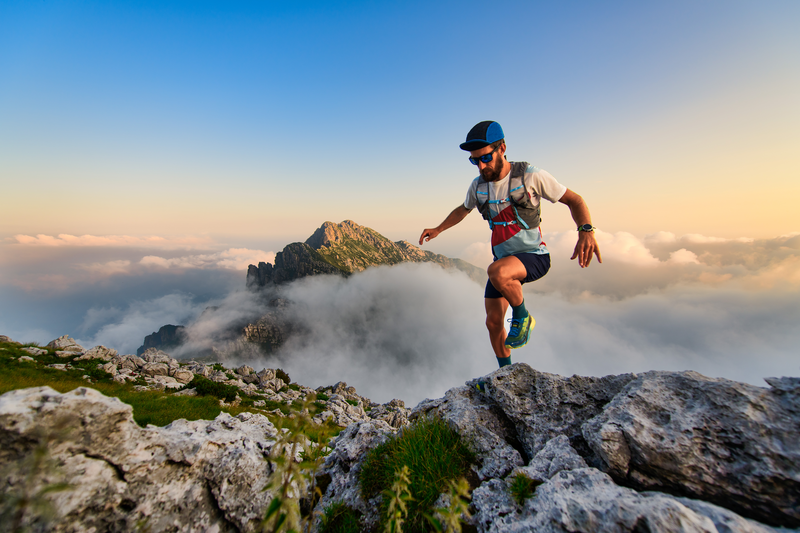
95% of researchers rate our articles as excellent or good
Learn more about the work of our research integrity team to safeguard the quality of each article we publish.
Find out more
SYSTEMATIC REVIEW article
Front. Pharmacol. , 10 August 2022
Sec. Experimental Pharmacology and Drug Discovery
Volume 13 - 2022 | https://doi.org/10.3389/fphar.2022.946554
This article is part of the Research Topic Recent Advances of Minor Saponins in Panax species View all 10 articles
Background: The clinical efficiency of terpenoids in treating human acute lung injury (ALI) is yet to be determined. The lipopolysaccharide-induced rat model of ALI is a well-established and widely used experimental model for studying terpenoids’ effects on ALI. Using a systematic review and meta-analysis, the therapeutic efficiency of terpenoid administration on the lung wet-to-dry weight ratio in rats was investigated.
Methods: Using the Cochrane Library, Embase, and PubMed databases, a comprehensive literature search for studies evaluating the therapeutic efficacy of terpenoids on ALI in rats was conducted. The lung wet-to-dry weight ratio was extracted as the main outcome. The quality of the included studies was assessed using the Systematic Review Center for Laboratory Animal Experimentation’s risk of bias tool.
Results: In total, 16 studies were included in this meta-analysis. In general, terpenoids significantly lowered the lung wet-to-dry weight ratio when compared with the control vehicle (p = 0.0002; standardized mean difference (SMD): −0.16; 95% confidence interval (CI): −0.24, −0.08). Subgroup analysis revealed that low dose (≤10 μmol/kg) (p < 0.0001; SMD: −0.68; 95% CI: −1.02, −0.34), intraperitoneal injection (p = 0.0002; SMD: −0.43; 95% CI: −0.66, −0.20), diterpenoid (p = 0.004; SMD: −0.13; 95% CI: −0.23, −0.04), and triterpenoid (p = 0.04; SMD: −0.28; 95% CI: −0.54, −0.01) significantly lowered the lung wet-to-dry weight ratio when compared with the control vehicle.
Conclusion: A low dose of diterpenoid and triterpenoid administered intraperitoneally is effective in alleviating ALI. This systematic review and meta-analysis provides a valuable mirror for clinical research aiming at the advancement of terpenoids for preventive and therapeutic use.
Systematic Review Registration: CRD42022326779
Acute lung injury (ALI) is an acute inflammatory disease that disrupts the lung’s endothelial and epithelial barriers (Manicone, 2009). It is associated with systemic inflammatory response syndrome and multiple organ dysfunction syndrome (Wu et al., 2021). ALI, which is characterized by pulmonary edema and severe hypoxia, has also been regarded as the leading cause of death in patients with sepsis, imposing an enormous health burden worldwide each year (Schingnitz et al., 2010).
Although the pathophysiology of ALI has been extensively studied, effective clinical treatments for ALI remain limited. Therefore, there is an urgent need for the development of additional medications to treat ALI. Several natural compounds, such as terpenoids, alkaloids, and flavonoids, have been used over the last few years to treat ALI (Ren et al., 2019; Zhang et al., 2019; Zhao et al., 2021). Terpenoids, also known as isoprenoids, are the most abundant and structurally diverse natural compounds found in numerous plant species. They are a diverse and large group of naturally occurring organic chemicals derived from the 5-carbon compound isoprene. In addition, they are known to have diverse pharmacological properties, including antiatherosclerotic, antitumor, anti-inflammatory, antinociceptive, and antimalarial activities (Liu et al., 2019; Yuan et al., 2020; El-Baba et al., 2021; Sankhuan et al., 2022). The majority of research on terpenoids’ anti-ALI effects has focused on diterpenoids; however, there is no consensus on other terpenoids (Yang et al., 2011; Yang et al., 2014; Li et al., 2018a).
Endotoxin can enter humans’ airways through inhalation of contaminated dust or aerosol particles in hospital, occupational, agricultural, and domestic environments. Lipopolysaccharide (LPS), which is a major endotoxin component of Gram-negative bacteria, is regarded as the most important pathogen responsible for the development of ALI in sepsis (Li et al., 2014a; Park et al., 2018). Animal studies allow for the investigation of the efficacy and safety of novel therapies, linking basic research and clinical trials. LPS is thought to be a significant inducer of lung injury. Because of its widespread use and accessibility, LPS-induced lung injury is the most commonly used animal model of ALI for replicating the pathophysiological process (Li et al., 2018a). In this present meta-analysis, we investigated the effects of terpenoid administration on the wet-to-dry weight (W/D) ratio of the lungs in rats with LPS-induced ALI to better understand the preventive and therapeutic potential of terpenoids on ALI.
The systematic review protocol for animal intervention studies was prepared in accordance with the Preferred Reporting Items for Systematic Reviews and Meta-Analyses guideline and the Systematic Review Center for Laboratory Animal Experimentation (SYRCLE) format (Hooijmans and Ritskes-Hoitinga, 2013; De Vries et al., 2015).
A comprehensive search was conducted by a competent information specialist (SW) in the Cochrane Library, Embase, and PubMed databases between January 2000 and March 2022 using the terms “acute lung injury,” “ALI,” “terpenoid,” “lipopolysaccharide,” “LPS,” and “rat.” The search terms are as follows: (acute lung injury or ALI) and (diterpenoid or hemiterpenoid or monoterpenoid or polyterpenoid or sesquiterpenoid or sesterterpenoid or terpenoid or tetraterpenoid or triterpenoid) and (lipopolysaccharide or LPS) and (rat or rats). Following a manual screening, further relevant studies were identified from the datasheet of included and reviewed articles.
The inclusion criteria are as follows: (a) original research, (b) terpenoid intervention, and (c) rat with LPS-induced ALI research model. The exclusion criteria were as follows: (a) reviews, abstracts, case reports, comments, and editorials; (b) missing data; (c) duplicate and/or overlapping datasets; and (d) publications that were not written in English.
To collect qualified studies, the abstracts and titles of the articles identified by the comprehensive search were independently reviewed by three investigators (SW, JJ, and HL). The full text of potentially eligible studies was thereafter reviewed and checked by three investigators (SL, DL, and LS) to determine if the studies met the inclusion and exclusion criteria. Disagreements as regards the study’s selection were resolved through discussion and compromise.
The characteristic data were extracted from qualified studies independently by three investigators (SL, LS, and HL), such as publication year, first author name, sample size of control and terpenoid groups, age, gender, rat strain and weight, diet type, terpenoid dosage, the interval between LPS administration and sacrifice, route and duration of LPS and terpenoid administration, lung wet-to-dry weight ratio, and dryer parameter. The statistics displayed graphically in the original publications were extracted using Adobe Photoshop (Ps v7.0). The main outcome was the lung wet-to-dry weight ratio, which is measured as a numerical value. Disagreements as regards data extraction were resolved through discussion and compromise.
The quality of the included studies was assessed by two investigators (SW and JJ) using SYRCLE’s risk of bias tool. The allocation concealment, incomplete outcome data, randomization, blinding and selective outcome assessments, baseline characteristics, random housing, domains evaluating sequence generation, and other sources of bias were included in the SYRCLE’s risk of bias tool (Hooijmans et al., 2014). Publication bias was assessed through visual inspection of funnel plots. Disagreements about quality assessment were resolved through discussion and compromise.
All statistical analyses were conducted using the Review Manager (RevMan v5.3) software. The effects of control vehicle and terpenoids on lung wet-to-dry weight ratio were assessed using the mean differences with 95% confidence intervals (CI). A fixed effects model was used to pool studies, and the inconsistency index was used to calculate heterogeneity as high (I2 ≥ 50%) or moderate (I2 ≥ 30%).
In one study, datasets with more than three independent groups, namely, a control group, a low terpenoid dosage group, and a high terpenoid dosage group, were defined. A single control group was compared with various groups that investigated different terpenoid dosages in eight studies (Yang et al., 2011; Chen et al., 2014; Yang et al., 2014; Wei and Wang, 2017; Li et al., 2018a; Ren et al., 2019; Wang et al., 2021; Wu et al., 2021). To avoid a redundant expansion in the meta-analysis sample size, the number of samples in the control group in each study was divided by those in the matched groups. Subanalyses of the effects of different terpenoid routes and doses on lung wet-to-dry weight ratio were conducted. A sensitivity analysis was conducted to assess the robustness of the results. A p-value of <0.05 was considered statistically significant.
Through the search strategy, 872 articles were identified. The investigators extracted the abstracts and titles and identified 38 studies that met the inclusion criteria. After reviewing all of the publications, 10 studies were excluded because of missing outcome data (Ehrhart et al., 2000; Tawadros et al., 2007; Nader and Baraka, 2012; An et al., 2014; Wang et al., 2015; Liu and Chen, 2016; Shen et al., 2017; Ni et al., 2019; Wang et al., 2019; Yang et al., 2019), and another 12 studies were excluded because multiple interventions were investigated (Murakami et al., 2000; Lin et al., 2011; Li et al., 2014b; Li et al., 2016a; Li et al., 2016b; Baradaran Rahimi et al., 2019; Ye et al., 2019; Duan et al., 2020; Yue et al., 2020; Choi et al., 2021; Zhang et al., 2021; Liu et al., 2022). In conclusion, 16 studies were included in this meta-analysis, as described below (Shi et al., 2007; Yang et al., 2011; Chen et al., 2014; Yang et al., 2014; Yuan et al., 2014; Wei and Wang, 2017; Li et al., 2018a; Li et al., 2018b; Luo et al., 2019; Ren et al., 2019; Zhang et al., 2020; Ali et al., 2021; Dikmen et al., 2021; Li et al., 2021; Wang et al., 2021; Wu et al., 2021) (Figure 1).
From the 16 included studies, 29 datasets and 499 rats were extracted. The characteristics of these studies are shown in Table 1. Rats ranged in age from 6 to 8 weeks and in weight from 90 to 350 g. In terms of intervention, diterpenoid, monoterpenoid, sesquiterpenoid, and triterpenoid were used in seven studies (Shi et al., 2007; Yang et al., 2011; Yang et al., 2014; Wei and Wang, 2017; Li et al., 2018a; Li et al., 2018b; Luo et al., 2019), three studies (Yuan et al., 2014; Ren et al., 2019; Li et al., 2021), two studies (Ali et al., 2021; Wang et al., 2021), and four studies (Chen et al., 2014; Zhang et al., 2020; Dikmen et al., 2021; Wu et al., 2021), respectively. In addition, 13 studies used male rats (Shi et al., 2007; Yang et al., 2011; Chen et al., 2014; Yang et al., 2014; Yuan et al., 2014; Wei and Wang, 2017; Li et al., 2018a; Luo et al., 2019; Ren et al., 2019; Zhang et al., 2020; Dikmen et al., 2021; Li et al., 2021; Wang et al., 2021), whereas three studies did not report gender (Li et al., 2018b; Ali et al., 2021; Wu et al., 2021). Sprague Dawley rats were used in 11 studies (Shi et al., 2007; Yang et al., 2014; Wei and Wang, 2017; Li et al., 2018a; Li et al., 2018b; Luo et al., 2019; Ren et al., 2019; Ali et al., 2021; Li et al., 2021; Wang et al., 2021; Wu et al., 2021) and Wistar rats in five studies (Yang et al., 2011; Chen et al., 2014; Yuan et al., 2014; Zhang et al., 2020; Dikmen et al., 2021).
LPS was administered intravenously in seven studies (Yang et al., 2011; Yang et al., 2014; Wei and Wang, 2017; Li et al., 2018a; Li et al., 2018b; Luo et al., 2019; Dikmen et al., 2021), intraperitoneally in one study (Shi et al., 2007), and intratracheally in eight studies (Chen et al., 2014; Yuan et al., 2014; Ren et al., 2019; Zhang et al., 2020; Ali et al., 2021; Li et al., 2021; Wang et al., 2021; Wu et al., 2021). The LPS dosage ranged from 0.1 to 10 mg/kg. Terpenoid was administered intragastrically in four studies (Chen et al., 2014; Yang et al., 2014; Yuan et al., 2014; Zhang et al., 2020), intravenously in three studies (Yang et al., 2011; Dikmen et al., 2021; Wang et al., 2021), intraperitoneally in eight studies (Shi et al., 2007; Wei and Wang, 2017; Li et al., 2018a; Li et al., 2018b; Luo et al., 2019; Ren et al., 2019; Ali et al., 2021; Wu et al., 2021), and intratracheally in one study (Li et al., 2021). The terpenoid dosage ranged from 0.08 to 370 μmol/kg. The terpenoid was given prior to LPS in eight studies (Chen et al., 2014; Yang et al., 2014; Yuan et al., 2014; Li et al., 2018a; Luo et al., 2019; Ren et al., 2019; Zhang et al., 2020; Li et al., 2021), after LPS in seven studies (Shi et al., 2007; Yang et al., 2011; Wei and Wang, 2017; Li et al., 2018b; Ali et al., 2021; Dikmen et al., 2021; Wang et al., 2021), and undetermined in one study (Wu et al., 2021). The interval between LPS administration and sacrifice ranged from 3 h to 8 days. The drying time ranged from 20 to 72 h, and the temperature ranged from 60 to 80°C.
The quality assessment of these studies is shown in Figure 2. In total, 12 studies were randomized, with eight studies demonstrating unclear risks of bias in blinding and allocation concealment. All studies’ outcomes were reported, with six studies demonstrating an unclear risk of selective outcome reporting. Overall, the risk of bias from other sources was low. The potential publication bias was evaluated through visual inspection of a funnel plot (Figure 3).
The effect of terpenoids on lung wet-to-dry weight ratio was presented for 29 datasets acquired from 16 studies (rats given terpenoids [n = 317] vs. rats given a control vehicle [n = 182]). Overall, terpenoids significantly reduced the lung wet-to-dry weight ratio when compared with the control vehicle (p = 0.0002; standardized mean difference (SMD): −0.16; 95% CI: −0.24, −0.08), with no evidence of heterogeneity among studies (I2 = 0%) (Figure 4).
FIGURE 4. The forest plot of therapeutic efficiency of terpenoids on lung wet-to-dry weight ratio. Subgroup analyses investigated the therapeutic efficiency of monoterpenoid, sesquiterpene, diterpenoid, and triterpenoid. CI, confidence interval; IV, inverse variance; Std, standard; SD, standard deviation.
Subgroup analyses were conducted to assess the effects of diterpenoid, monoterpenoid, sesquiterpenoid, and triterpenoid on the lung wet-to-dry weight ratio. Monoterpenoid (rats given a monoterpenoid [n = 26] vs. rats given a control vehicle [n = 20]; p = 0.22; SMD: −0.21; 95% CI: −0.55, 0.13) and sesquiterpenoid (rats given a sesquiterpenoid [n = 26] vs. rats given a control vehicle [n = 14]; p = 0.22; SMD: −0.26; 95% CI: −0.69, 0.16) did not significantly lower the lung wet-to-dry weight ratio when compared with the control vehicle, with no evidence of heterogeneity among studies (I2 = 0%). Diterpenoid (rats given a diterpenoid [n = 207] vs. rats given a control vehicle [n = 111]) significantly reduced the lung wet-to-dry weight ratio when compared with the control vehicle (p = 0.004; SMD: −0.13; 95% CI: −0.23, −0.04), with evidence of low heterogeneity among studies (I2 = 20%). Triterpenoid (rats given a triterpenoid [n = 58] vs. rats given a control vehicle [n = 37]) significantly decreased the lung wet-to-dry weight ratio when compared with the control vehicle (p = 0.04; SMD: −0.28; 95% CI: −0.54, −0.01), with no evidence of heterogeneity among studies (I2 = 0%) (Figure 4). The sensitivity analysis, which substituted the random effects model for the fixed effects model, had no effect on the overall outcome (SMD: −0.16, CI: −0.24, −0.08 vs. SMD: −0.36, CI: −0.55, −0.17).
In terms of the administration route, subgroup analyses revealed that intraperitoneal injection of terpenoid (rats given a terpenoid [n = 81] vs. rats given a control vehicle [n = 51]) significantly reduced the lung wet-to-dry weight ratio when compared with the control vehicle (p = 0.0002; SMD: −0.43; 95% CI: −0.66, −0.20), with no evidence of heterogeneity among studies (I2 = 0%). However, intravenous injection of terpenoid (rats given a terpenoid [n = 64] vs. rats given a control vehicle [n = 34]) did not significantly lower the lung wet-to-dry weight ratio when compared with the control vehicle (p = 0.25; SMD: −0.12; 95% CI: −0.32, −0.08), with no evidence of heterogeneity among studies (I2 = 0%). Likewise, intragastric administration of terpenoid (rats given a terpenoid [n = 96] vs. rats given a control vehicle [n = 54]) did not significantly lower the lung wet-to-dry weight ratio when compared with the control vehicle (p = 0.06; SMD: −0.10; 95% CI: −0.20, −0.00), with no evidence of heterogeneity among studies (I2 = 0%) (Figure 5). The sensitivity analysis, which substituted the random effects model for the fixed effects model, had no effect on the overall outcome (SMD: −0.15, CI: −0.24, −0.06 vs. SMD: −0.36, CI: −0.57, −0.15).
FIGURE 5. The forest plot of therapeutic efficiency of the route of terpenoid administration on lung wet-to-dry weight ratio. Subgroup analyses evaluated the therapeutic efficiency of intraperitoneal injection, intravenous injection, and intragastric administration. CI, confidence interval; IV, inverse variance; Std, standard; SD, standard deviation.
In terms of terpenoid dosage, subgroup analyses revealed that low doses of terpenoid administered intraperitoneally (rats given terpenoid at a dose of 10 μmol/kg or lower [n = 32] vs. rats given a control vehicle [n = 20]) significantly lowered the lung wet-to-dry weight ratio when compared with the control vehicle (p < 0.0001; SMD: −0.68; 95% CI: −1.02, −0.34), with no evidence of heterogeneity among studies (I2 = 0%). However, the high dose (rats given terpenoid at a dose greater than 10 μmol/kg [n = 49] vs. rats given a control vehicle [n = 31]) did not significantly lower the lung wet-to-dry weight ratio when compared with the control vehicle (p = 0.16; SMD: −0.22; 95% CI: −0.54, −0.09), with no evidence of heterogeneity among studies (I2 = 0%) (Figure 6). The sensitivity analysis, which substituted the random effects model for the fixed effects model, had no effect on the overall outcome (SMD: −0.43, CI: −0.66, −0.20 vs. SMD: −0.46, CI: −0.83, −0.09).
FIGURE 6. The forest plot of therapeutic efficiency of terpenoid dose on lung wet-to-dry weight ratio. Subgroup analyses investigated therapeutic efficiency of high dose (>10 µmol/kg) and low dose (≤10 µmol/kg). CI, confidence interval; IV, inverse variance; Std, standard; SD, standard deviation.
Despite significant advances in pharmacotherapy agents for ALI, such as antibiotics, N-acetylcysteine, β-agonists, corticosteroids, surfactants, and statins, an efficient approach to lowering ALI morbidity and mortality is yet to be identified (Lewis et al., 2019). Because diterpenoids were found to be promising in treating ALI in some studies (Yang et al., 2011; Yang et al., 2014; Li et al., 2018a), it was necessary to investigate the pharmacological effects of terpenoids on ALI. The use of animal models provides a valuable gateway for preclinical research, identifying novel therapeutic strategies for disease and developing new drugs. An ideal animal model should be able to replicate the consequences and mechanisms of human disease, including pathological and physiological hallmarks. LPS is a component of Gram-negative bacterial cell walls. LPS-induced animal models, by inhalation or systemic (intravenous and intraperitoneal) administration, reproduce acute damage to the lung epithelial and endothelial barriers, as well as acute inflammatory responses, in a short period of time (typically <48 h) (Matute-Bello et al., 2008). LPS-induced injury is a valuable in vivo experimental model that is similar to ALI and acute respiratory distress syndrome in humans. LPS is easy to use, and its outcomes are often replicable in experiments. Although mouse models of human disease are widely used because of the availability of specific reagents and the development of transgenic mice that can be administered to assess the physiological function of specific genes, animal size remains an important consideration when choosing an animal model for ALI. Because there is no difference between rats and mice in ALI animal models (Matute-Bello et al., 2008), rats were used as experimental animals in this study.
Previous animal experiments suggest that natural terpenoids may have therapeutic effects on ALI. However, study parameters such as sample size, animal strain and age, and treatment and follow-up duration were noted to differ among studies. A quantitative and comprehensive analysis of these heterogeneous sources of animal model data can provide insights into the benefits of terpenoids in the treatment of ALI. Therefore, we conducted a systematic review and meta-analysis to investigate the effects of terpenoids on lung wet-to-dry weight ratio in rats with LPS-induced ALI. The findings revealed that the use of terpenoids significantly reduced the lung wet-to-dry weight ratio and alleviated pulmonary edema when compared with the control group.
The terpenoid family includes monoterpenoid, sesquiterpenoid, diterpenoid, triterpenoid, and tetraterpenoid (Christianson, 2017). In a subgroup analysis, the number of isoprene groups, monoterpenoid and sesquiterpenoid, did not drastically lower the lung wet-to-dry weight ratio. By contrast, diterpenoid and triterpenoid attenuated pulmonary edema and ameliorated ALI in LPS-induced ALI rats compared with the control vehicle. We presumed that the differences among the groups might be induced by the sample size gap and underlying publication bias. Of course, more studies are needed to confirm this speculation. Moreover, a low dose (≤10 µmol/kg) of diterpenoid and triterpenoid via intraperitoneal injection showed a significant outcome for decreasing lung wet-to-dry weight ratio. To our knowledge, this meta-analysis is an initial assessment of the therapeutic efficiency of terpenoids on LPS-induced ALI in rats. The total outcomes might offer a valuable reference to the future preventive and therapeutic use of terpenoids in human ALI.
In the overall analysis, there was no heterogeneity among studies. However, among studies in the subgroups of diterpenoid and low-dose terpenoid, it had a low degree of heterogeneity. Possible origin of heterogeneity contained strain of rat, route of LPS, and interval between LPS administration and sacrifice, each of which can influence the progression of ALI (Chen et al., 2010).
The meta-analysis is coupled with some restrictions. First, the correlation between the outcomes and humans is restrained by the differences between species in the development of pulmonary edema. The LPS-induced ALI animal model is well reputable, and the main characteristics and development of pulmonary edema in humans and rats emerge similar, but to a certain extent, it is different in pathogenesis (Tomashefski, 2000). We realize that an LPS-induced ALI animal model duplicates a lot of pathological features, but it is still relatively simple and rapid to reproduce the pathologic processes of humans (Matute-Bello et al., 2008). Second, other animal models of ALI were not included in this meta-analysis, such as pigs and sheep, which are easier to be induced by LPS because they have a more sensitive pulmonary hypertensive response and a higher speed circulation than rats (Warner et al., 1988; Schmidhammer et al., 2006). Third, only the lung wet-to-dry weight ratio was extracted to investigate the effects of terpenoids on ALI. Other parameters, such as proinflammatory cytokines, including tumor necrosis factor and interleukin, lung injury score, and lung-to-body weight ratio, were not considered (Eastwood et al., 2015; Butt et al., 2016). Fourth, hemiterpenoids, sesterterpenoids, and other terpenoids were not investigated in the analysis. In addition, more studies based on a huge number of samples and large-scale animal models are still necessary for determining whether terpenoids are effective for attenuating ALI in humans.
This meta-analysis showed that terpenoid is beneficial for alleviating ALI in rats. In particular, low-dose (≤10 µmol/kg) diterpenoid and triterpenoid significantly decrease lung wet-to-dry weight ratio in rats via intraperitoneal injection. We need more well-designed, prospective, and extensive animal model research to expand our understanding of the mechanisms of terpenoids in ALI treatment. We still need randomized controlled trials in humans to demonstrate the clinical benefit of terpenoids in the prevention and therapy of ALI.
The original contributions presented in the study are included in the article/supplementary material; further inquiries can be directed to the corresponding authors.
SW conducted the analysis, JJ and DL collected and performed a preliminary analysis of references, HL and SL wrote the manuscript, and LS designed and revised the manuscript.
This work was supported by the Office of Science and Technology in Jilin Province (No. 20210204114YY and 20210101325JC).
We would like to thank Enago Academy for the revisions to the manuscript in terms of language and grammar.
The authors declare that the research was conducted in the absence of any commercial or financial relationships that could be construed as potential conflicts of interest.
All claims expressed in this article are solely those of the authors and do not necessarily represent those of their affiliated organizations, or those of the publisher, the editors, and the reviewers. Any product that may be evaluated in this article, or claim that may be made by its manufacturer, is not guaranteed or endorsed by the publisher.
Ali, F. E. M., Ahmed, S. F., Eltrawy, A. H., Yousef, R. S., Ali, H. S., Mahmoud, A. R., et al. (2021). Pretreatment with coenzyme Q10 combined with aescin protects against sepsis-induced acute lung injury. Cells Tissues Organs 210 (3), 195–217. doi:10.1159/000516192
An, J. F., Sun, Y., Zhang, Q. L., Zhang, F. L., and Zhang, J. L. (2014). The effects of germacrone on lipopolysaccharide-induced acute lung injury in neonatal rats. Cell Mol. Biol. (Noisy-le-grand) 60 (4), 8–12.
Baradaran Rahimi, V., Rakhshandeh, H., Raucci, F., Buono, B., Shirazinia, R., Samzadeh Kermani, A., et al. (2019). Anti-inflammatory and anti-oxidant activity of portulaca oleracea extract on LPS-induced rat lung injury. Molecules 24 (1), 139. doi:10.3390/molecules24010139
Butt, Y., Kurdowska, A., and Allen, T. C. (2016). Acute lung injury: A clinical and molecular review. Arch. Pathol. Lab. Med. 140 (4), 345–350. doi:10.5858/arpa.2015-0519-RA
Chen, H., Bai, C., and Wang, X. (2010). The value of the lipopolysaccharide-induced acute lung injury model in respiratory medicine. Expert Rev. Respir. Med. 4 (6), 773–783. doi:10.1586/ers.10.71
Chen, J., Gao, J., Yang, J., Zhang, Y., and Wang, L. (2014). Effect of triptolide on the regulation of ATP-binding cassette transporter A1 expression in lipopolysaccharide-induced acute lung injury of rats. Mol. Med. Rep. 10 (6), 3015–3020. doi:10.3892/mmr.2014.2636
Choi, M., Thuy, L. T., Lee, Y., Piao, C., Choi, J. S., and Lee, M. (2021). Dual-functional dendrimer micelles with glycyrrhizic acid for anti-inflammatory therapy of acute lung injury. ACS Appl. Mater Interfaces 13 (40), 47313–47326. doi:10.1021/acsami.1c08107
Christianson, D. W. (2017). Structural and chemical Biology of terpenoid cyclases. Chem. Rev. 117 (17), 11570–11648. doi:10.1021/acs.chemrev.7b00287
De Vries, R. B. M., Hooijmans, C. R., Langendam, M. W., and Luijk, J. V. (2015). A protocol format for the preparation, registration and publication of systematic reviews of animal intervention studies. Evid. Based Preclin. Med. 2, 1–9. doi:10.1002/ebm2.7
Dikmen, N., Cellat, M., Etyemez, M., İşler, C. T., Uyar, A., Aydın, T., et al. (2021). Ameliorative effects of oleuropein on lipopolysaccharide-induced acute lung injury model in rats. Inflammation 44 (6), 2246–2259. doi:10.1007/s10753-021-01496-x
Duan, Q., Jia, Y., Qin, Y., Jin, Y., Hu, H., and Chen, J. (2020). Narciclasine attenuates LPS-induced acute lung injury in neonatal rats through suppressing inflammation and oxidative stress. Bioengineered 11 (1), 801–810. doi:10.1080/21655979.2020.1795424
Eastwood, M. P., Russo, F. M., Toelen, J., and Deprest, J. (2015). Medical interventions to reverse pulmonary hypoplasia in the animal model of congenital diaphragmatic hernia: A systematic review. Pediatr. Pulmonol. 50 (8), 820–838. doi:10.1002/ppul.23206
Ehrhart, I. C., Zou, L., Theodorakis, M. J., Parkerson, J. B., Gu, X., Caldwell, R. B., et al. (2000). Effect of nitrite on endothelial function in isolated lung. Gen. Pharmacol. 34 (6), 401–408. doi:10.1016/s0306-3623(01)00077-5
El-Baba, C., Baassiri, A., Kiriako, G., Dia, B., Fadlallah, S., Moodad, S., et al. (2021). Terpenoids' anti-cancer effects: Focus on autophagy. Apoptosis 26 (9-10), 491–511. doi:10.1007/s10495-021-01684-y
Hooijmans, C. R., and Ritskes-Hoitinga, M. (2013). Progress in using systematic reviews of animal studies to improve translational research. PLoS Med. 10 (7), e1001482. doi:10.1371/journal.pmed.1001482
Hooijmans, C. R., Rovers, M. M., de Vries, R. B., Leenaars, M., Ritskes-Hoitinga, M., and Langendam, M. W. (2014). SYRCLE's risk of bias tool for animal studies. BMC Med. Res. Methodol. 14, 43. doi:10.1186/1471-2288-14-43
Lewis, S. R., Pritchard, M. W., Thomas, C. M., and Smith, A. F. (2019). Pharmacological agents for adults with acute respiratory distress syndrome. Cochrane Database Syst. Rev. 7 (7), CD004477. doi:10.1002/14651858.CD004477.pub3
Li, G., Zhou, C. L., Zhou, Q. S., and Zou, H. D. (2016). Galantamine protects against lipopolysaccharide-induced acute lung injury in rats. Braz J. Med. Biol. Res. 49 (2), e5008. doi:10.1590/1414-431X20155008
Li, J., Zheng, Y., Li, M. X., Yang, C. W., and Liu, Y. F. (2018). Tanshinone IIA alleviates lipopolysaccharide-induced acute lung injury by downregulating TRPM7 and pro-inflammatory factors. J. Cell Mol. Med. 22 (1), 646–654. doi:10.1111/jcmm.13350
Li, K. C., Ho, Y. L., Chen, C. Y., Hsieh, W. T., Chang, Y. S., and Huang, G. J. (2016). Lobeline improves acute lung injury via nuclear factor-κB-signaling pathway and oxidative stress. Respir. Physiol. Neurobiol. 225, 19–30. doi:10.1016/j.resp.2015.12.003
Li, L., Zhang, Y. G., Tan, Y. F., Zhao, J. J., Zhang, H. R., and Zhao, B. (2018). Tanshinone II is a potent candidate for treatment of lipopolysaccharide-induced acute lung injury in rat model. Oncol. Lett. 15 (2), 2550–2554. doi:10.3892/ol.2017.7581
Li, S., Lei, Y., Lei, J., and Li, H. (2021). All‑trans retinoic acid promotes macrophage phagocytosis and decreases inflammation via inhibiting CD14/TLR4 in acute lung injury. Mol. Med. Rep. 24 (6), 868. doi:10.3892/mmr.2021.12508
Li, T., Liu, Y., Li, G., Wang, X., Zeng, Z., Cai, S., et al. (2014). Polydatin attenuates ipopolysaccharide-induced acute lung injury in rats. Int. J. Clin. Exp. Pathol. 7, 8401–8410.
Li, W., Huang, H., Niu, X., Fan, T., Hu, H., Li, Y., et al. (2014). Tetrahydrocoptisine protects rats from LPS-induced acute lung injury. Inflammation 37 (6), 2106–2115. doi:10.1007/s10753-014-9945-7
Lin, Y., Zhu, X., Yao, W. Z., and Yang, Y. L., (2011). Yohimbine protects against endotoxin-induced acute lung injury by blockade of alpha 2A adrenergic receptor in rats. Chin. Med. J. Engl. 124 (7), 1069–1074.
Liu, H., Zhang, Y., Sun, S., and Wang, S. (2019). Efficacy of terpenoid in attenuating aortic atherosclerosis in apolipoprotein-E deficient mice: A meta-analysis of animal studies. Biomed. Res. Int. 2019, 2931831. doi:10.1155/2019/2931831
Liu, P., Hao, J., Zhao, J., Zou, R., Han, J., Tian, J., et al. (2022). Integrated network Pharmacology and experimental validation approach to investigate the therapeutic effects of capsaicin on lipopolysaccharide-induced acute lung injury. Mediat. Inflamm. 2022, 9272896. doi:10.1155/2022/9272896
Liu, T. Y., and Chen, S. B. (2016). Sarcandra glabra combined with lycopene protect rats from lipopolysaccharide induced acute lung injury via reducing inflammatory response. Biomed. Pharmacother. 84, 34–41. doi:10.1016/j.biopha.2016.09.009
Luo, X., Lin, B., Gao, Y., Lei, X., Wang, X., Li, Y., et al. (2019). Genipin attenuates mitochondrial-dependent apoptosis, endoplasmic reticulum stress, and inflammation via the PI3K/AKT pathway in acute lung injury. Int. Immunopharmacol. 76, 105842. doi:10.1016/j.intimp.2019.105842
Manicone, A. M. (2009). Role of the pulmonary epithelium and inflammatory signals in acute lung injury. Expert Rev. Clin. Immunol. 5 (1), 63–75. doi:10.1586/177666X.5.1.63
Matute-Bello, G., Frevert, C. W., and Martin, T. R. (2008). Animal models of acute lung injury. Am. J. Physiol. Lung Cell. Mol. Physiol. 295 (3), L379–L399. doi:10.1152/ajplung.00010.2008
Murakami, K., Okajima, K., and Uchiba, M. (2000). The prevention of lipopolysaccharide-induced pulmonary vascular injury by pretreatment with cepharanthine in rats. Am. J. Respir. Crit. Care Med. 161 (1), 57–63. doi:10.1164/ajrccm.161.1.9808142
Nader, M. A., and Baraka, H. N. (2012). Effect of betulinic acid on neutrophil recruitment and inflammatory mediator expression in lipopolysaccharide-induced lung inflammation in rats. Eur. J. Pharm. Sci. 46 (1-2), 106–113. doi:10.1016/j.ejps.2012.02.015
Ni, Y. L., Shen, H. T., Su, C. H., Chen, W. Y., Huang-Liu, R., Chen, C. J., et al. (2019). Nerolidol suppresses the inflammatory response during lipopolysaccharide-induced acute lung injury via the modulation of antioxidant enzymes and the AMPK/Nrf-2/HO-1 pathway. Oxid. Med. Cell Longev. 2019, 9605980. doi:10.1155/2019/9605980
Park, J., Chen, Y., Zheng, M., Ryu, J., Cho, G. J., Surh, Y. J., et al. (2018). Pterostilbene 4'-β-Glucoside attenuates LPS-induced acute lung injury via induction of heme oxygenase-1. Oxid. Med. Cell Longev. 2018, 2747018. doi:10.1155/2018/2747018
Ren, J., Lu, Y., Qian, Y., Chen, B., Wu, T., and Ji, G. (2019). Recent progress regarding kaempferol for the treatment of various diseases. Exp. Ther. Med. 18 (4), 2759–2776. doi:10.3892/etm.2019.7886
Sankhuan, D., Niramolyanun, G., Kangwanrangsan, N., Nakano, M., and Supaibulwatana, K. (2022). Variation in terpenoids in leaves of Artemisia annua grown under different LED spectra resulting in diverse antimalarial activities against Plasmodium falciparum. BMC Plant Biol. 22 (1), 128. doi:10.1186/s12870-022-03528-6
Schingnitz, U., Hartmann, K., Macmanus, C. F., Eckle, T., Zug, S., Colgan, S. P., et al. (2010). Signaling through the A2B adenosine receptor dampens endotoxin-induced acute lung injury. J. Immunol. 184 (9), 5271–5279. doi:10.4049/jimmunol.0903035
Schmidhammer, R., Wassermann, E., Germann, P., Redl, H., and Ullrich, R. (2006). Infusion of increasing doses of endotoxin induces progressive acute lung injury but prevents early pulmonary hypertension in pigs. Shock 25 (4), 389–394. doi:10.1097/01.shk.0000209529.43367.00
Shen, B., Zhao, C., Chen, C., Li, Z., Li, Y., Tian, Y., et al. (2017). Picroside II protects rat lung and A549 cell against LPS-induced inflammation by the NF-κB pathway. Inflammation 40 (3), 752–761. doi:10.1007/s10753-017-0519-3
Shi, X. M., Huang, L., Xiong, S. D., and Zhong, X. Y. (2007). Protective effect of tanshinone II A on lipopolysaccharide-induced lung injury in rats. Chin. J. Integr. Med. 13 (2), 137–140. doi:10.1007/s11655-007-0137-2
Tawadros, P. S., Powers, K. A., Yang, I., Becker, D. A., Ginsberg, M. D., Szaszi, K., et al. (2007). Stilbazulenyl nitrone decreases oxidative stress and reduces lung injury after hemorrhagic shock/resuscitation and LPS. Antioxid. Redox Signal 9 (11), 1971–1977. doi:10.1089/ars.2007.1765
Tomashefski, J. F. (2000). Pulmonary pathology of acute respiratory distress syndrome. Clin. Chest Med. 21 (3), 435–466. doi:10.1016/s0272-5231(05)70158-1
Wang, Y. M., Ji, R., Chen, W. W., Huang, S. W., Zheng, Y. J., Yang, Z. T., et al. (2019). Paclitaxel alleviated sepsis-induced acute lung injury by activating MUC1 and suppressing TLR-4/NF-κB pathway. Drug Des. Devel Ther. 13, 3391–3404. doi:10.2147/DDDT.S222296
Wang, Y. Y., Qiu, X. G., and Ren, H. L. (2015). Inhibition of acute lung injury by rubriflordilactone in LPS-induced rat model through suppression of inflammatory factor expression. Int. J. Clin. Exp. Pathol. 8 (12), 15954–15959.
Wang, Y., Zhang, J., Gao, X., Li, Q., and Sun, D. (2021). In vitro and in vivo anti-inflammatory effect of Zaluzanin D isolated from Achillea acuminate. Int. Immunopharmacol. 90, 107130. doi:10.1016/j.intimp.2020.107130
Warner, A. E., DeCamp, M. M., Molina, R. M., and Brain, J. D. (1988). Pulmonary removal of circulating endotoxin results in acute lung injury in sheep. Lab. Invest 59 (2), 219–230.
Wei, Y., and Wang, Y. (2017). Celastrol attenuates impairments associated with lipopolysaccharide-induced acute respiratory distress syndrome (ARDS) in rats. J. Immunotoxicol. 14 (1), 228–234. doi:10.1080/1547691X.2017.1394933
Wu, Y., Huang, D., Wang, X., Pei, C., Xiao, W., Wang, F., et al. (2021). Suppression of NLRP3 inflammasome by Platycodin D via the TLR4/MyD88/NF-κB pathway contributes to attenuation of lipopolysaccharide induced acute lung injury in rats. Int. Immunopharmacol. 96, 107621. doi:10.1016/j.intimp.2021.107621
Yang, H., Lv, H., Li, H., Ci, X., and Peng, L. (2019). Oridonin protects LPS-induced acute lung injury by modulating Nrf2-mediated oxidative stress and Nrf2-independent NLRP3 and NF-κB pathways. Cell Commun. Signal 17 (1), 62. doi:10.1186/s12964-019-0366-y
Yang, N., Liu, Y. Y., Pan, C. S., Sun, K., Wei, X. H., Mao, X. W., et al. (2014). Pretreatment with andrographolide pills(®) attenuates lipopolysaccharide-induced pulmonary microcirculatory disturbance and acute lung injury in rats. Microcirculation 21 (8), 703–716. doi:10.1111/micc.12152
Yang, W., Qiang, D., Zhang, M., Ma, L., Zhang, Y., Qing, C., et al. (2011). Isoforskolin pretreatment attenuates lipopolysaccharide-induced acute lung injury in animal models. Int. Immunopharmacol. 11 (6), 683–692. doi:10.1016/j.intimp.2011.01.011
Ye, J., Guan, M., Lu, Y., Zhang, D., Li, C., and Zhou, C. (2019). Arbutin attenuates LPS-induced lung injury via Sirt1/Nrf2/NF-κBp65 pathway. Pulm. Pharmacol. Ther. 54, 53–59. doi:10.1016/j.pupt.2018.12.001
Yuan, H. L., Zhao, Y. L., Ding, C. F., Zhu, P. F., Jin, Q., Liu, Y. P., et al. (2020). Anti-inflammatory and antinociceptive effects of Curcuma kwangsiensis and its bioactive terpenoids in vivo and in vitro. J. Ethnopharmacol. 259, 112935. doi:10.1016/j.jep.2020.112935
Yuan, Q., Jiang, Y. W., Ma, T. T., Fang, Q. H., and Pan, L. (2014). Attenuating effect of Ginsenoside Rb1 on LPS-induced lung injury in rats. J. Inflamm. (Lond) 11 (1), 40. doi:10.1186/s12950-014-0040-5
Yue, Q., Liu, T., and Cheng, Z. (2020). Protective effect of colchicine on LPS-induced lung injury in rats via inhibition of P-38, ERK1/2, and JNK activation. Pharmacology 105 (11-12), 639–644. doi:10.1159/000504759
Zhang, C., Wang, X., Wang, C., He, C., Ma, Q., Li, J., et al. (2021). Qingwenzhike prescription alleviates acute lung injury induced by LPS via inhibiting TLR4/NF-kB pathway and NLRP3 inflammasome activation. Front. Pharmacol. 12, 790072. doi:10.3389/fphar.2021.790072
Zhang, E., Wang, J., Chen, Q., Wang, Z., Li, D., Jiang, N., et al. (2020). Artesunate ameliorates sepsis-induced acute lung injury by activating the mTOR/AKT/PI3K axis. Gene 759, 144969. doi:10.1016/j.gene.2020.144969
Zhang, Z., Wang, X., Ma, C., Li, Z., Chen, H., Zhang, Z., et al. (2019). Genipin protects rats against lipopolysaccharide-induced acute lung injury by reinforcing autophagy. Int. Immunopharmacol. 72, 21–30. doi:10.1016/j.intimp.2019.03.052
Keywords: acute lung injury, terpenoids, lipopolysaccharide, lung wet-to-dry weight ratio, animal model
Citation: Wang S, Luo SX, Jie J, Li D, Liu H and Song L (2022) Efficacy of terpenoids in attenuating pulmonary edema in acute lung injury: A meta-analysis of animal studies. Front. Pharmacol. 13:946554. doi: 10.3389/fphar.2022.946554
Received: 17 May 2022; Accepted: 27 June 2022;
Published: 10 August 2022.
Edited by:
Bing Chun Yan, Yangzhou University, ChinaReviewed by:
Nasra Ayuob, Damietta University, EgyptCopyright © 2022 Wang, Luo, Jie, Li, Liu and Song. This is an open-access article distributed under the terms of the Creative Commons Attribution License (CC BY). The use, distribution or reproduction in other forums is permitted, provided the original author(s) and the copyright owner(s) are credited and that the original publication in this journal is cited, in accordance with accepted academic practice. No use, distribution or reproduction is permitted which does not comply with these terms.
*Correspondence: Han Liu, bGl1aGFuQGpsdS5lZHUuY24=; Lei Song, bHNvbmdAamx1LmVkdS5jbg==
Disclaimer: All claims expressed in this article are solely those of the authors and do not necessarily represent those of their affiliated organizations, or those of the publisher, the editors and the reviewers. Any product that may be evaluated in this article or claim that may be made by its manufacturer is not guaranteed or endorsed by the publisher.
Research integrity at Frontiers
Learn more about the work of our research integrity team to safeguard the quality of each article we publish.