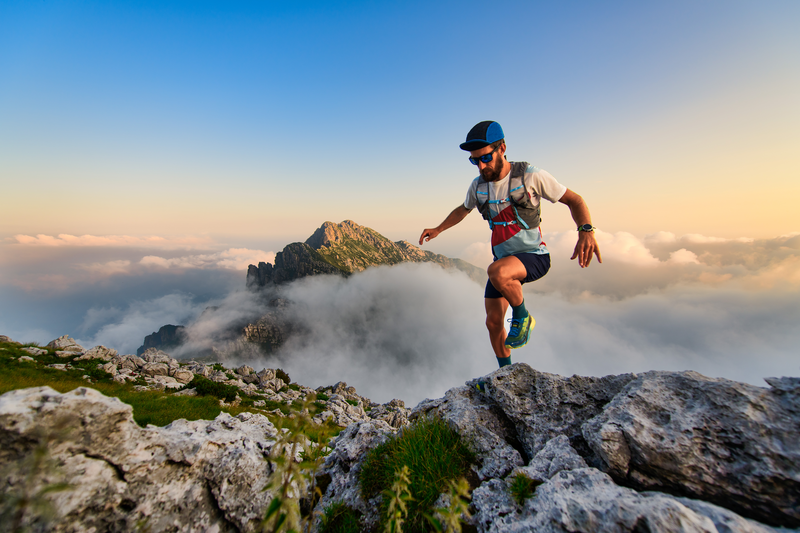
94% of researchers rate our articles as excellent or good
Learn more about the work of our research integrity team to safeguard the quality of each article we publish.
Find out more
MINI REVIEW article
Front. Pharmacol. , 12 September 2022
Sec. Ethnopharmacology
Volume 13 - 2022 | https://doi.org/10.3389/fphar.2022.938395
This article is part of the Research Topic Natural Products for Neuroprotection and Neuroregeneration View all 9 articles
Spinosin, a natural flavone-C-glycoside that is mainly isolated from the seeds of Ziziphus jujuba Mill. var. spinosa. It exerts the effects to ameliorate the neurological disorders, such as hypnosis effects, improvement of cognitive function, sedation effects, and anxiolytic effects, as well as anti-melanogenic effect, cardioprotective effects, and anti-cancer activity. However, the insufficient basic research, unclear mechanisms, and poor bioavailability may limit the prospects of spinosin in clinical utilization. In this review, we comprehensively summarized the latest information on the pharmacology, pharmacokinetics, toxicity, and NMR characteristic of spinosin, to evaluate its potential therapeutic for clinical application, hoping to provide some rational perspective for the innovative agent development and usage of spinosin in future.
Spinosin, (PubChem CID: 24771055, CAS No.: 72,063-39-9, MW: 608.5 g/mol), with the molecular formula of C28H32O15, is a natural flavone-C-glycoside that mainly exists in dried and ripe seeds of Ziziphus jujuba Mill. var. spinosa (ZJS) (Figure 1) (Wu et al., 2011). Recently, spinosin has also been isolated from Cayaponia tayuya (Vell.) Cogn (Aquila et al., 2009), Passiflora edulis Sims (Zucolotto et al., 2009), Leonurus japonicus Houtt. (Liu et al., 2018), and so on.
In traditional Chinese medicine (TCM), ZJS is sweet and sour in flavor, neutral in nature, and belongs to the liver, gallbladder, and heart meridians (Chinese pharmacopoeia, 2020). ZJS possesses the functions of nourishing the heart and liver, calming the mind and nerves, and condensing sweat and producing fluid that has been widely used for hypnosis, palpitations, dreams, sweating, and thirst (Chinese pharmacopoeia, 2020). It also has been widely used as an herb in the preparations of Chinese material medica to treat insomnia and anxiety for its main sedative and hypnotic effects. ZJS contains several groups of bioactive components including flavonoids, saponins, alkaloids, and fatty acids (Jiao et al., 2017), and saponins and flavonoids are the main effective components of sedation and hypnosis. Jujuboside A, jujuboside B, and spinosin are major components of total saponins and total flavones, respectively (Zhu et al., 2015). Spinosin is a C-glycoside flavonoid isolated from the ethanol extract of ZJS’s dried seeds (Huang et al., 2014), as one of the main bioactive components of ZJS, numerous explorations of the pharmacological effects of it have been reported, such as hypnosis effects (Wang et al., 2008, 2010, 2012; Wang et al., 2016a), improvement of cognitive function (Jung et al., 2014; Ko et al., 2015; Xu et al., 2020), anxiolytic effect (Wang and Yan, 2022), inhibition of melanin synthesis (Moon et al., 2019), and antioxidant effects (Zhang et al., 2020a).
Pharmacology research is crucial for the efficient and rational development of drugs, such as elucidating their mechanism, developing new usage of existing drugs, improving their efficacy, and reducing the toxicity of the medicine. The pharmacokinetic (PK) behavior of drugs plays an essential role in their pharmacological actions. The alterations of pharmacokinetic parameters may affect the drug’s therapeutic efficacy (Ma et al., 2012). Detailed toxicological data are the basis for risk assessment. The use of accurate and reliable toxicological data is the first step in hazard identification (Qu and Song, 2021). To better utilize the medicinal resource of spinosin, we discussed the research on spinosin in various research fields in the recent years covering its pharmacology, pharmacokinetics, and toxicology in this review.
The seeds of ZJS are used as a traditional herbal drug for the treatment of insomnia (Tsai et al., 2019). The potentiating sleep effect of spinosin was investigated in pentobarbital-treated (45 mg/kg, i.p.) mice. The results showed that pretreatment with spinosin (10 and 15 mg/kg) enhanced hypnotic effects, while using spinosin alone did not work. Further studies found that co-administration of spinosin (5 mg kg, p.o.) and 5-HTP (2.5 mg kg, i.p.) significantly reduced sleep latency and lengthened the sleep duration time (Wang et al., 2008). The aforementioned research suggested the regulation effect of the 5-HT system in pentobarbital-induced sleep.
The 5-HT1A receptor, a subtype of 5-HT receptors, plays an important role in the modulation of sleep and wakefulness. A deeper study revealed that spinosin (15 mg/kg, i.g.) lengthened the REM sleep time and increased the slow-wave sleep (SWS) mode in rats. A 5-HT1A antagonist, the p-MPPI, reduced sleep latency and increased total sleep time and NREM sleep time. Conversely, a 5-HT1A receptor agonist, the 8-OH-DPAT, reduced the NREM sleep, REM sleep, and SWS time in pentobarbital-treated rats. Spinosin could reverse the 8-OH-DPAT-induced reductions in the aforementioned sleep periods, suggesting spinosin may serve as an antagonist on the postsynaptic 5-HT1A receptors (Wang et al., 2010). Further studies showed spinosin could potentiate pentobarbital-induced loss of righting reflex (LORR) in mice, and verified the antagonist of spinosin on presynaptic 5-HT1A autoreceptor (Wang et al., 2012). Another research indicated that spinosin (20 mg/kg, i.p.) can increase the non-rapid eye movement (NREM) time and shorten the sleep latency time in the active phase of mice by antagonizing the 5-HT1A receptor (Wang et al., 2016a).
Those results indicated that spinosin is an inhibitor on both somatodendritic 5-HT1A autoreceptors and postsynaptic 5-HT1A heteroreceptors, and this ingredient may be used as a potential drug for the treatment of hypnosis.
Alzheimer’s disease (AD) is a neurodegenerative disease with the characteristics of memory deterioration, cognitive function reduction, and behavioral impairments (Chu et al., 2012). The hallmark of AD is hippocampal synaptic dysfunction, which is becoming a potential target for the AD therapy.
Spinosin exerted the neuroprotective effects on cholinergic blockade-induced memory impairment in mice by extending the latency time in the passive avoidance task, lengthening the swimming time, and increasing the expression levels of phosphorylated extracellular signal-regulated kinases and cAMP response element-binding proteins in the hippocampus (Jung et al., 2014). Further research revealed that spinosin increased the number of immature neurons in the dentate gyrus region of the hippocampus and the neuronal cell propagation, and stimulated the neurons’ differentiation by the ERK-CREB-BDNF signaling pathway. The results indicated that spinosin can be used for the treatment of neurological cognitive dysfunction or psychiatric disorders (Lee et al., 2016).
The amyloid-β1-42 (Aβ1-42)–induced mouse model was used to evaluate the activities and mechanisms of spinosin in the treatment of AD. Spinosin improves the memory impairment induced by amyloid Aβ(1–42) oligomer in mice verified by the passive avoidance task and the Y-maze task, decreasing the GFAP or OX-42 in the hippocampus, reducing the number of activated microglia and astrocytes, and enhancing the choline acetyltransferase (ChAT) expression (Ko et al., 2015). Spinosin attenuated the long-term potentiation (LTP), which is the indicator that reflects learning and memory, by the improved plasmin level in hippocampi of 5XFAD mice induced by Aβ (Cai et al., 2020).
Numerous studies have revealed that neuroinflammation play a critical role in the occurrence and development of AD. Anti-inflammation has been considered to be one of the significant ways to improve or even treat AD. Spinosin has been reported to alleviate cognitive impairment by improving the neurotrophic factor (BDNF) and Bcl-2, decreasing the level of MDA, and inhibiting the inflammatory factor IL-6 in the brain (Xu et al., 2019). Another research showed that spinosin inhibited the expression of COX-2 and Bax protein caused by Aβ25-35, and improved the proportion of LTP. The conclusion suggested the repairment of spinosin on the learning and memory impairment induced by Aβ25-35 was mainly by inhibiting the inflammatory response (Du, 2021).
The oxidative stress is often accompanied by the occurrence of AD (Cervellati et al., 2016). Reactive oxygen species (ROS), including hydrogen peroxide (H2O2), hydroxyl radical (OH•), and superoxide anions (O2), is the major source of oxidative stress, which contributes to proteins, lipids, and DNA oxidation in brain tissues. p38MAPK is sensitive to stressful stimuli such as ROS and is related to the development process of AD. Spinosin showed the inhibitory effect on the intracellular ROS production induced by H2O2 in N2a cells. The in-depth mechanism research indicated spinosin inhibited Aβ(1–42) production, decreased Tau phosphorylation, and improved synaptic structural plasticity induced by H2O2 through inhibiting the p38MAPK activation (Xu et al., 2020).
In addition, spinosin inhibited the production and accumulation of Aβ1-42 through influencing the amyloid precursor protein (APP) parade, by activating the antioxidative Nrf2/HO-1 pathway (Zhang et al., 2020c).
The sedative activities of spinosin were evaluated by the climbing test and caffeine-induced hyperactivity model in mice. The results revealed that the number of mice that could not climb the ladder was increased and the frequency of crossing the hole was decreased after spinosin (500 and 1,000 mg/kg) was given (i.p.) (Shin et al., 1978; Shin et al., 1981). Spinosin was injected into mice (15 mg/kg, i.p.), and then 90 min later their brains were isolated and used for immunohistochemical analysis. The results revealed that spinosin markedly decreased c-Fos expression in the lateral hypothalamic area (LHA) and locus coeruleus (LC), suggesting that inactivation of the LHA and LC neuronal was the mechanism of spinosin on sedation (Zhang et al., 2020a).
The anxiety disorder is one of the most common psychiatric disorders that affect the health of the general population. The anxiolytic effects of spinosin were evaluated by a plus maze, light/dark box test, and open field test in mice (Gu et al., 2018). Also, the results showed that spinosin exhibited the anxiolytic effects without influencing the spontaneous activity. Further research illustrated that the anxiolytic-like activity of spinosin can be prevented by flumazenil and WAY-100635, the antagonist of GABAA receptor and 5-HT1A receptor, respectively, which suggested its targets maybe GABAA and 5-HT1A receptors (Liu et al., 2015).
As tyrosinase is the key factor in melanin production, inhibiting the tyrosinase can be used for skin pigmentation. Spinosin showed tyrosinase inhibitory activity (IC50 = 47 μM) and anti-melanogenesis effect (10, 20 μM) in B16F10 cells induced by αMSH- or UVB. The protein docking analysis further demonstrated that spinosin repressed the tyrosinase activity through the hydrogen bonds (Moon et al., 2019). This evidence suggested the potential of spinosin developed as a candidate for skin-lightening cosmetics.
The acute myocardial infarction (AMI) rat model was used to investigate the cardioprotective effects of spinosin and its analog 6‴-feruloyl-spinosin. As a result, pretreatment with spinosin lessened myocardial tissue injury, reduced the serum levels of cTnI and LDH, and promoted autophagy by increasing LC3B-II levels in AMI rats. The mechanism data suggested that spinosin worked by inhibiting the GSK3β, and activating the autophagy and the activity of the PGC-1α/Nrf2/HO-1 pathway (Gu et al., 2019).
The polyphenol extraction from ZJS (ZJSP, 0, 50, 100, 150, and 200 μg/ml) exhibited anti-colorectal cancer (CRC) activity by inhibiting HCT-116 cell growth, increasing cell apoptosis in the HCT-8 and HCT-116 cells, and enhancing the sensitivity of HCT-8FU cells for 5-FU. The AOM/DSS-induced CAC mice were used to evaluate the CRC effect and the results showed the ZJSP (100 and 200 mg/kg) reduced the CAC polyps, promoted the recovery of damaged organs (heart, liver, spleen, lung, kidney, and pancreas), and raised the early CRC markers (COX-II, EMR1, and Ki67) in CAC mice. Further isolation and RP-HPLC-MS/MS results indicated spinosin was the anti-CRC constituent in ZJSP (Shan et al., 2020). This study indicated the potential usage of spinosin as a natural agent against CRC. The details pharmacological activities of spinosin are depicted in Table 1 and Figure 1.
The pharmacokinetic properties are the premise of preclinical and clinical research of drugs. It provides drug toxicity and clinical application information to screen the candidate agents in the process of innovative agent development. Nowadays, HPLC (Li et al., 2014) and HPLC/MS/MS (Liu et al., 2013) were used to investigate the pharmacokinetic parameters of spinosin in vivo with rats (Bao et al., 2013) and dogs (Lee et al., 2020). The rat oral administration of ZJS extract (20 g/kg) containing spinosin revealed the pharmacokinetic parameters with Cmax at 224 ± 82 μg/L, Tmax at 5.5 ± 0.6 h, and T0.5 at 5.8 ± 0.9 h in rat plasma using the HPLC method (Li et al., 2003). The pharmacokinetic parameters of spinosin in ZJSP between the control group (NC) and insomnia model (IM) group were determined using the UPLC-MS method, and the results showed no significant differences between the two groups, but an increasing trend for CL and the decreasing trend for the AUC0-t and AUC0-∞ of spinosin in the IM group, which indicated the faster and poorer absorption of spinosin in the IM group (Du et al., 2020). The Yin-deficiency rats were given ZJS by gavage, spinosin was detectable in the small intestine, stomach, liver, brain, large intestine, spleen, lung, heart, kidney, and blood within 10 min, and it sustained for 240 min (Guo, 2014). The distribution studies revealed that spinosin could be detected in the rat liver, spleen, kidney, lung (Li et al., 2021; Li et al., 2007), gastrointestinal (Zhang et al., 2012), bile (Liu et al., 2013), and testis (Li et al., 2007). Zhang et al. (2015) reported that spinosin could permeate the blood–brain barrier, and reach various areas of the brain such as the corpus striatum, hippocampus, cerebrum, cerebellum, and olfactory region.
Many novel dosage forms, such as solid dispersions, phospholipid complex solid dispersions and its solid lipid nanoparticles, obviously elevated solubility and bioavailability of spinosin, which effectively promoted the oral absorption of spinosin (Zhang et al., 2019; Yang et al., 2019).
The Caco-2 cell model was used to investigate the transport characteristics of spinosin, the results indicated that spinosin was transported through the intestinal mucosa via a passive diffusion at low concentration, while affected by P-glycoprotein (P-gp) at a high concentration with reduced absorption (Huang et al., 2016). Song et al. (2020) found the absorption mechanism of spinosin was energy-dependent monocarboxylate transporter (MCT)–mediated active transport, and the efflux process was mediated by P-gp and multidrug resistance protein (MRP), which may result in a decrease in bioavailability.
The UPLC-MS/MS was carried out to identify metabolites and evaluate the in vivo metabolic profile of spinosin. Three metabolites of I-phase were identified from blood and urine in depression model rats (Wang et al., 2016b). Eight I-phase metabolites of spinosin were detected in the human liver microsome incubation samples, and the cytochrome P450 enzyme was found to be the main metabolic enzyme involved in drug metabolism (Zhang et al., 2020b). Spinosin was degraded by rat intestinal bacteria in vitro and its metabolite was swertisin (Zhang et al., 2013). The main metabolic reactions of spinosin were decarbonylation and demethylation, hydroxylation, hydrolysis-desugar, and RDA.
The serum proteins, such as BSA and HSA, have the ability of binding with drugs, which play an important role in the drug metabolism. The results revealed that spinosin bound with BSA and HSA through Van der Waals force and hydrogen bond and then changed the Tyr and Trp residue microenvironments. The findings may explain the metabolism behaviors of spinosin in oxidation, intestinal hydrolysis, demethylation, and reduction (Wu et al., 2019). The pharmacokinetic studies on spinosin are shown in Table 2 and metabolites of spinosin are shown in Figure 2.
The toxicity of spinosin was studied by intraperitoneally injecting into mice with graded doses ranging from 200 mg/kg to 10 g/kg and there was no mortality of mice even at the highest dosage, which suggested the safety of spinosin (Shin et al., 1978).
Spinosin also did not exhibit cytotoxicity on HaCat, B16F10, and Hs27 cells at 20 μM (Moon et al., 2019). Spinosin had cytotoxicity in N2a/APP695 cells at 200 and 400 μM, but no cytotoxicity in N2a/WT cells at 400 μM (Zhang et al., 2020c). The cytochrome P450 participated in the drug metabolism process and mediated the drug–herb interactions, which have attracted much attention in recent years. The activation effects between CYP450 and drugs may increase the risk of drug application. One report indicated that spinosin exhibited inactive effects on CYP3A4 in human liver microsomes (Bai et al., 2020).
Spinosin, the main active C-glycoside constituent from ZJS, is highly consistent with pharmacological and toxicological properties of ZJS. The ZJS decoction was administered to mice at 15 g/kg by gavage, and no toxicity was observed in 48 h (Shen, 2011). The administration routes affected the drug’s toxicity. ZJS decoction and ethanolic extract of ZJS were injected intravenously into mice and the LD50 values were determined as 14.3 ± 20.0 and 27.5 ± 2.4 g/kg, respectively. The same samples were orally administered to mice at the dosage of 340 g/kg, but no case of mortality was observed (Wang et al., 2009). The chickens were orally administered with ZJS solution at the dosages ranging between 2.5 and 20 g/(kg d) and the maximum tolerable dosage of ZJS solution was calculated as more than 20 g/(kg d), while the LD50 was not determined. The experiments suggested that ZJS solution had no acute toxicity and no long-time toxicity (Li et al., 2010).
The interesting NMR phenomenon of spinosin was observed in 2000 (Gong et al., 2000). Both 1H and 13C NMR data of spinosin exhibited the partial carbons and protons signal splits appearance at room temperature (298 K), and doublet signals disappeared as the temperature rose to coalescence temperature (Tc 363 K). The results of the variable-temperature experiments suggested the presence of two rotational isomerisms at room temperature. Compared to the NMR data with compounds that have similar structures, only the constituents with 7-OCH3 in the flavone-6-C-glycoside skeleton exhibited the aforementioned NMR signal pattern (Gong et al., 2000). The variable-temperature 1H NMR experiments explained that the high energy barrier about the C-6-C-1″ bonds prevents the interchange between rotamers at room temperature. The theoretical (MM2) calculations revealed the minimum energy of two conformations (energy difference ca 0.84 kJ/mol) and the separated energy barrier of ca 67 kJ/mol (Lewis et al., 2000). The finds were approved by many reports in analogs of spinosin, such as 6‴-feruloyl-spinosin, 6‴-acetyl-spinosin, and isovitexin-2″-O-arabinoside (Song et al., 2020; Zhou and Yan, 2021). The aforementioned NMR signal features can be used to quickly distinguish the chemical skeleton and analyze the structure of substituent position. The 1D NMR spectra of spinosin at 298 and 387 K are shown in Figure S1A-B.
This article reviewed the bioactivities and the mechanisms of spinosin (Table 1), its pharmacokinetics parameters (Table 2) and security, as well as characteristic NMR performance. However, many issues need to be further illustrated in further studies. First, a few reports on pharmacological activities and the reported bioactivities of spinosin mainly focused on the phenotypic aspect, but there is lack of an in-depth specific interpretation on the mechanism research. Therefore, it is extremely meaningful to explore the molecular mechanism of its biological activities. Second, the little existing evidence suggested the safety of spinosin in vitro and in vivo, but it is difficult to fully evaluate its security due to lack of research evidence. Hence, it is necessary to systematically evaluate its safety and toxicity in vitro and in vivo for the clinical application. Third, mice are also widely used for pharmacokinetic studies but there have been no relevant reported pharmacokinetic studies on spinosin. So, the comprehensive pharmacokinetic research about spinosin and ZJS in rodent models should be conducted in future.
FX and XK analyzed the data and drafted the manuscript. BL and GS gave valuable advice on manuscript writing and revision. FX, WC, JZ, and TM collected and analyzed many references.
This work was supported by the National Natural Science Foundation of China (No. 82173700); Science and Technology Planning Project of Guangzhou (No. 202102021213); the special foundation of Guangzhou Key Laboratory (No. 202002010004); Special Funds for State Key Laboratory of Dampness Syndrome of Chinese Medicine (No. SZ2021ZZ33).
The authors declare that the research was conducted in the absence of any commercial or financial relationships that could be construed as a potential conflict of interest.
All claims expressed in this article are solely those of the authors and do not necessarily represent those of their affiliated organizations, or those of the publisher, the editors, and the reviewers. Any product that may be evaluated in this article, or claim that may be made by its manufacturer, is not guaranteed or endorsed by the publisher.
The Supplementary Material for this article can be found online at: https://www.frontiersin.org/articles/10.3389/fphar.2022.938395/full#supplementary-material
Aquila, S., Giner, R. M., Recio, M. C., Spegazzini, E. D., and Jose, L. R. (2009). Anti-inflammatory activity of flavonoids from Cayaponia tayuya roots. J. Ethnopharmacol. 121, 333–337. doi:10.1016/j.jep.2008.11.002
Bai, J., Li, L., Zhao, S. Y., Fan, X. Q., Zhang, J., Hu, M. W., et al. (2020). Heterotropic activation of flavonoids on cytochrome P450 3A4: A case example of alleviating dronedarone-induced cytotoxicity. Toxicol. Lett. 319, 187–196. doi:10.1016/j.toxlet.2019.11.016
Bao, K. D., Zhao, J. H., Qi, L. W., Li, H., Yi, J. L., Wang, W., et al. (2013). Determination of spinosin and 6′′′-feruloylspinosin in rat plasma after oral administration of flavonoid extract from ziziphi spinosae semen by SPE-HPLC-DAD. Chin. J. Mod. Appl. Pharm. 30, 707–711. doi:10.13748/j.cnki.issn1007-7693.2013.07.010
Cai, M. D., Jung, I. H., Kwon, H. Y., Cho, E., Jeon, J., Yun, J., et al. (2020). Spinosin attenuates alzheimer's disease-associated synaptic dysfunction via regulation of plasmin activity. Biomol. Ther. 28, 131–136. doi:10.4062/biomolther.2019.076
Cervellati, C., Wood, P. L., Romani, A., Valacchi, G., Squerzanti, M., Sanz, J. M., et al. (2016). Oxidative challenge in alzheimer’s disease: State of knowledge and future needs. J. Investig. Med. 64, 21–32. doi:10.1136/jim-2015-000017
Chinese pharmacopoeia (2020). Pharmacopoeia of China, Part I. Beijing: China Medical Science and Technology Press.
Chu, Y. F., Chang, W. H., Black, R. M., Liu, J. R., Sompol, P., Chen, Y., et al. (2012). Crude caffeine reduces memory impairment and amyloid β1-42 levels in an alzheimer’s mouse model. Food Chem. 135, 2095–2102. doi:10.1016/j.foodchem.2012.04.148
Du, C. H., Yan, Y., Shen, C. X., Cui, X. F., Pei, X. P., and Qin, X. M. (2020). Comparative pharmacokinetics of six major compounds in normal and insomnia rats after oral administration of ziziphi spinosae semen aqueous extract. J. Pharm. Anal. 10, 385–395. doi:10.1016/j.jpha.2020.03.003
Du, H. Z. (2021). Spinosine improves Alzheimer's disease by reducing inflammation. Chin. J. Pharmacol. Toxicol. 5, 658.
Gong, C., Bai, Y., Zhao, Y., Tao, J., Liu, Y., Tu, G. Z., et al. (2000). Flavonoids from Ziziphus jujuba Mill var. spinosa. Tetrahedron 56, 8915–8920. doi:10.1016/S0040-4020(00)00842-5
Gu, C., Zhao, Z., Zhu, X., Wu, T., Lee, B. H., Jiao, Y., et al. (2018). Aqueous extract of semen ziziphi spinosae exerts anxiolytic effects during nicotine withdrawal via improvement of amygdaloid CRF/CRF1R signaling. Evid. Based. Complement. Altern. Med. 2018, 2419183–2419212. doi:10.1155/2018/2419183
Gu, M. H., He, P. K., Lyu, C. F., Liu, X. Y., Xu, Y. L., Cheng, S. B., et al. (2019). Spinosin and 6"'-feruloylspinosin protect the heart against acute myocardial ischemia and reperfusion in rats. Mol. Med. Rep. 20, 4253–4261. doi:10.3892/mmr.2019.10686
Guo, M. J. (2014). Study on the effect of genuine semen ZizyphiSpinosae to the anxiety behavior in the yin-deficiency ratsand the body distribution of major components. Henan, China: Henan College of Traditional Chinese Medicine.
Huang, H. Y., Yu, D. R., Wang, C. G., Shi, S. N., and Shi, J. L. (2014). Determination of apparent oil-water partition coefficient of spinosin. Chin. J. Pharm. Anal. 34 (3), 497–499. doi:10.16155/j.0254-1793.2014.03.030
Huang, H. Y., Guo, Y. L., He, Y. S., Wei, Y. H., and Liu, J. (2016). Transport characteristics of spinosin in caco-2 cell model by UPLC-MS/MS. Chin. J. Pharm. Anal. 36, 980–985. doi:10.16155/j.0254-1793.2016.06.06
Jiao, L., Li, Y., Zhang, Y., Liu, J., Xie, J., Zhang, K., et al. (2017). Degradation kinetics of 6'''-p-Coumaroylspinosin and identification of its metabolites by rat intestinal flora. J. Agric. Food Chem. 65 (22), 4449–4455. doi:10.1021/acs.jafc.7b01486
Jung, I. H., Lee, H. E., Park, S. J., Ahn, Y. J., Kwon, G. Y., Woo, H., et al. (2014). Ameliorating effect of spinosin, a C-glycoside flavonoid, on scopolamine-induced memory impairment in mice. Pharmacol. Biochem. Behav. 120, 88–94. doi:10.1016/j.pbb.2014.02.015
Ko, S. Y., Lee, H. E., Park, S. J., Jeon, S. J., Kim, B., Gao, Q., et al. (2015). Spinosin, a C-glucosylflavone, from Zizyphus jujuba var. Spinosa ameliorates Aβ (1-42) oligomer-induced memory impairment in mice. Biomol. Ther. 23, 156–164. doi:10.4062/biomolther.2014.110
Lee, Y., Jeon, S. J., Lee, H. E., Jung, I. H., Jo, Y. W., Lee, S., et al. (2016). Spinosin, a C-glycoside flavonoid, enhances cognitive performance and adult hippocampal neurogenesis in mice. Pharmacol. Biochem. Behav. 145, 9–16. doi:10.1016/j.pbb.2016.03.007
Lee, C. B., Min, J. S., Chae, S. U., Kim, H. M., Jang, J. H., Jung, I. H., et al. (2020). Simultaneous determination of donepezil, 6-O-desmethyl donepezil and spinosin in beagle dog plasma using liquid chromatography-tandem mass spectrometry and its application to a drug-drug interaction study. J. Pharm. Biomed. Anal. 178, 112919. doi:10.1016/j.jpba.2019.112919
Lewis, K. C., Maxwell, A. R., McLean, S., Reynolds, W. F., and Enriquez, R. G. (2000). Room-temperature (1H, 13C) and variable-temperature (1H) NMR studies on spinosin. Magn. Reson. Chem. 38, 771–774. doi:10.1002/1097-458x(200009)38:9<771:aid-mrc729>3.0.co;2-4
Li, Y. J., Liang, X. M., Xiao, H. B., and Bi, K. S. (2003). Pharmacokinetic study on spinosin in rat plasma after oral administration of suanzaoren extract at a single dose. Acta Pharm. Sin. 38 (2003), 448–450. doi:10.16438/j.0513-4870.2003.06.012
Li, Y. J., Dai, Y. H., Yu, Y. L., Li, Y., and Deng, Y. L. (2007). Pharmacokinetics and tissue distribution of spinosin after intravenous administration in rats. Yakugaku Zasshi 127, 1231–1235. doi:10.1248/yakushi.127.1231
Li, Y. C., Zhang, F. M., He, C., Li, S. M., Chen, X., Chen, H., et al. (2010). Studies on the acute and sub-chronic toxicity of Suanzaoren solution. Chin. Veterinary Sci. 40 (9), 978–983. doi:10.16656/j.issn.1673-4696.2010.09.017
Li, S., Wang, X. F., Dang, Y. F., Tian, J., and Zhao, X. F. (2014). The pharmacokinetics of the main ingredient in zaoren-an-shen granule in rats. J. Northwest U. Nat. Sci. Ed. 44, 252–255. doi:10.16152/j.cnki.xdxbzr.2014.02.026
Li, R., Wang, L., Wang, X., Zhang, D., Zhang, Y. F., Li, Z., et al. (2021). Simultaneous determination of four monoamine neurotransmitters and seven effective components of zaoren anshen prescription in rat tissue using UPLC-MS/MS. Curr. Pharm. Anal. 17, 67–80. doi:10.2174/1573412915666190709095958
Liu, M. Y., Zhao, S. H., Li, S., Wang, H. T., and Tu, P. F. (2013). Determination of three active compounds of shensong yangxin capsules in rat bile by UPLC-MS/MS. Chin. J. Pharm. 44, 1025–1028. doi:10.16522/j.cnki.cjph.2013.10.001
Liu, J., Zhai, W. M., Yang, Y. X., Shi, J. L., Liu, Q. T., Liu, G. L., et al. (2015). GABA and 5-HT systems are implicated in the anxiolytic-like effect of spinosin in mice. Pharmacol. Biochem. Behav. 128, 41–49. doi:10.1016/j.pbb.2014.11.003
Liu, J., Peng, C., Zhou, Q. M., Guo, L., Liu, Z. H., and Xiong, L. (2018). Alkaloids and flavonoid glycosides from the aerial parts of Leonurus japonicus and their opposite effects on uterine smooth muscle. Phytochemistry 145, 128–136. doi:10.1016/j.phytochem.2017.11.003
Ma, R. H., Yang, J., Qi, L. W., Xin, G. Z., Wang, C. Z., Yuan, C. S., et al. (2012). In vivo microdialysis with LC–MS for analysis of spinosin and its interaction with cyclosporin A in rat brain, blood and bile. J. Pharm. Biomed. Anal. 61, 22–29. doi:10.1016/j.jpba.2011.11.014
Moon, K. M., Hwang, Y. H., Yang, J. H., Ma, J. Y., and Lee, B. (2019). Spinosin is a flavonoid in the seed of Ziziphus jujuba that prevents skin pigmentation in a human skin model. J. Funct. Foods 54, 449–456. doi:10.1016/j.jff.2019.01.044
Qu, J. J., and Song, Y. (2021). Development of anthraquinones toxicity. J. Hyg. Res. 50 (05), 868–872. doi:10.19813/j.cnki.weishengyanjiu.2021.05.02
Shan, S. H., Xie, Y., Zhang, C. Y., Jia, B., Li, H. Q., and Li, Z. Y. (2020). Identification of polyphenol from ziziphi spinosae semen against human colon cancer cells and colitis-associated colorectal cancer in mice. Food Funct. 11, 8259–8272. doi:10.1039/d0fo01375g
Shen, Y. J. (2011). Pharmacology of Chinese materia medica. Beijing, China: People's Medical Publishing House, 709.
Shin, K. H., Lee, C. K., Woo, W. S., and Kang, S. S. (1978). Sedative action of spinosin. Arch. Pharm. Res. 1, 7–11. doi:10.1007/BF02856299
Shin, K. H., Woo, W. S., and Lee, C. K. (1981). Sedative action of flavonoids and saponin from the seeds of Zizyphus vulgaris var. spinosus bunge. Soul Taehakkyo Saengyak Yonguso Opjukjip 20, 82–86.
Song, P. P., Xiao, S., Zhang, Y. Q., Xie, J. B., and Cui, X. S. (2020). Mechanism of the intestinal absorption of six flavonoids from zizyphi spinosi semen across caco-2 cell monolayer model. Curr. Drug Metab. 21, 633–645. doi:10.2174/1389200221666200714100455
Tsai, F., Liu, X., Chen, C., Li, T., Chiou, J., Chuang, P., et al. (2019). Chinese herbal medicine therapy and the risk of overall mortality for patients with liver cancer who underwent surgical resection in Taiwan. Complement. Ther. Med. 47, 102213. doi:10.1016/j.ctim.2019.102213
Wang, S., Yan, Y., Yin, X., Chen, B., and Liu, X. (2022). Study on preparation and quality control of total flavonoids in Ziziphi Spinosae Semen. J. Chromatogr. Sci. 53 (02), 232–242. doi:10.1093/chromsci/bmab056
Wang, L. E., Bai, Y. J., Shi, X. R., Cui, X. Y., Cui, S. Y., Zhang, F., et al. (2008). Spinosin, a C-glycoside flavonoid from semen zizhiphi spinozae, potentiated pentobarbital-induced sleep via the serotonergic system. Pharmacol. Biochem. Behav. 90, 399–403. doi:10.1016/j.pbb.2008.03.022
Wang, L. J., Zhang, M. C., and Yan, C. (2009). Study on acute toxicity of alcohol-soluble extract of semen ziziphi spinosae. Lishizhen Med. Mat. Med. Res. 20 (07), 1610–1611. doi:10.3969/j.issn.1008-0805.2009.07.021
Wang, L. E., Cui, X. Y., Cui, S. Y., Cao, J. X., Zhang, J., Zhang, Y. H., et al. (2010). Potentiating effect of spinosin, a C-glycoside flavonoid of semen ziziphi spinosae, on pentobarbital-induced sleep may be related to postsynaptic 5-ht1a receptors. Phytomedicine 17, 404–409. doi:10.1016/j.phymed.2010.01.014
Wang, L. E., Zhang, X. Q., Yin, Y. Q., and Zhang, Y. H. (2012). Augmentative effect of spinosin on pentobarbital-induced loss of righting reflex in mice associated with presynaptic 5-ht1a receptor. J. Pharm. Pharmacol. 64, 277–282. doi:10.1111/j.2042-7158.2011.01400.x
Wang, J., Ni, J., and Qu, W. M. (2016a). Spinosin induces non-rapid eye movement sleep in mice. Chin. J. Pharmacol. Toxicol. 30, 1018. CNKI:SUN:YLBS.0.2016-10-052.
Wang, Y. N., Mu, L. H., Xu, L., Wei, P. P., and Wang, R. (2016b). Analysis on in vivo metabolites of shaozao capsules in rats by UPLC-Q-TOF-MSE. Chin. J. Exp. Tradit. Med. Formula. 22, 77–81. doi:10.13422/j.cnki.syfjx.2016200077
Wu, Y., He, F., Pan, Q., Shi, Y., Min, Z., and Liang, J. Y. (2011). C-glucosyl flavones from the seeds of Ziziphus jujuba var. spinosa. Chem. Nat. Compd. 47, 369–372. doi:10.1007/s10600-011-9936-y
Wu, B. A., Qu, C. H., Wang, Y. X., Zhao, J. F., and Du, H. Z. (2019). Comparison of the quenching effects of two main components of ziziphi spinosae semen on serum Albumin fluorescence. J. Fluoresc. 29, 1113–1123. doi:10.1007/s10895-019-02422-z
Xu, F., He, B. S., Xiao, F., Yan, T. X., Bi, K. S., Jia, Y., et al. (2019). Neuroprotective effects of spinosin on recovery of learning and memory in a mouse model of alzheimer's disease. Biomol. Ther. 27, 71–77. doi:10.4062/biomolther.2018.051
Xu, F. X., Zhang, X. Y., Wang, J. Y., Li, X., He, B. S., Xiao, F., et al. (2020). Spinosin protects N2a cells from H2O2-induced neurotoxicity through inactivation of p38MAPK. J. Pharm. Pharmacol. 72, 1607–1614. doi:10.1111/jphp.13334
Yang, J. Z., Cui, X. G., and Hao, H. J. (2019). Preparation, characterization and pharmacokinetic comparison of spinosin phospholipid complex and its solid lipid nanoparticles. J. Chin. Med. Mat. 42, 1855–1859. doi:10.13863/j.issn1001-4454.2019.08.030
Zhang, T., Xie, J. B., Liu, Z. Y., Zhang, Y. C., and Cheng, D. W. (2012). Absorption kinetics of spinosin in rat gastrointestinal. Chin. J. Exp. Tradit. Med. Formula. 18, 134–139. doi:10.13422/j.cnki.syfjx.2012.13.052
Zhang, T., Xie, J. B., Zhang, Y. Q., and Cheng, D. W. (2013). High-performance liquid chromatography coupled with tandem mass spectrometry applied for metabolic study of spinosin by rat intestinal flora. J. Liq. Chromatogr. Relat. Technol. 36, 1391–1400. doi:10.1080/10826076.2012.691439
Zhang, Y. Q., Zhang, T., Wang, F. L., and Xie, J. B. (2015). Brain tissue distribution of spinosin in rats determined by a new high-performance liquid chromatography-electrospray ionization-mass/mass spectrometry method. J. Chromatogr. Sci. 53, 97–103. doi:10.1093/chromsci/bmu025
Zhang, T. S., Shang, S. Y., Wang, C. Y., and Zhang, Z. C. (2019). Preparation and in vivo pharmacokinetic behavior evaluation for two spinosin solid dispersions. Chin. Tradit. Pat. Med. 41, 2025–2029. doi:10.3969/j.issn.1001-1528.2019.09.001
Zhang, J. P., Liao, D. Q., Li, L., and Chu, L. (2020a). Reduced c-fos expression in orexin neurons of the lateral hypothalamic area and the locus coeruleus following injection of spinosin into mice. Folia Morphol. 79, 429–437. doi:10.5603/FM.a2019.0118
Zhang, Q. Y., Zhang, X., Liu, Y. Y., Wan, C. C., Sun, Y. P., and Zhang, L. T. (2020b). In vitro identification of spinosin metabolites in human liver microsomes using a simple and sensitive UHPLC-Q-TOF-MS/MS method. Curr. Pharm. Anal. 16, 40–46. doi:10.2174/1573412914666181003141210
Zhang, X. Y., Wang, J. Y., Gong, G. W., Ma, R. X., Xu, F. X., Yan, T. X., et al. (2020c). Spinosin inhibits aβ1-42 production and aggregation via activating Nrf2/HO-1 pathway. Biomol. Ther. 28, 259–266. doi:10.4062/biomolther.2019.123
Zhou, G. H., and Yan, H. R. (2021). Variable-temperature 1H-NMR studies on three C-glycosylflavones exhibiting rotational isomerism. Mod. Chem. 9, 8–12. doi:10.11648/j.mc.20210901.12
Zhu, H. Y., Zhang, L. N., Tang, S., Lin, H. C., Wang, G. L., He, Z. M., et al. (2015). Determination of spinosin, jujubosides A and B in Ziziphi Spinosae Semen from three different origins by HPLC. Chin. J. Pharm. Anal. 35 (12), 2099–2104.
Zucolotto, S. M., Goulart, S., Montanher, A. B., Reginatto, F. H., Schenkel, E. P., and Froede, T. S. (2009). Bioassay-guided isolation of anti-inflammatory C-glucosylflavones from Passiflora edulis. Planta Med. 75, 1221–1226. doi:10.1055/s-0029-1185536
5-HTP 5-hydroxytryptophan
AD Alzheimer’s disease
AMI acute myocardial infarction
APP amyloid precursor protein
Aβ1-42 amyloid-β1-42
BDNF brain-derived neurotrophic factor
BSA bovine serum albumin
CAC colitis-associated cancer
ChAT choline acetyltransferase
CL clearance
CRC colorectal cancer
cTnI cardiac troponin I
GABAA γ-aminobutyric acid A
GSK3β glycogen synthase kinase-3β
HPLC high-performance liquid chromatography
HSA human serum albumin
IM insomnia model
LC locus coeruleus
LC3B-II microtubule-associated protein one light chain 3B-Ⅱ
LDH lactate dehydrogenase
LHA lateral hypothalamic area
LORR loss of righting reflex
LTP long-term potentiation
MCT monocarboxylate transporter
MDA malondialdehyde
MRP multidrug resistance protein
NC normal control
NMR nuclear magnetic resonance
NREM non-rapid eye movement
PCPA p-chlorophenylalanine
P-gp P-glycoprotein
PK pharmacokinetic
RDA Retro Diels–Alder reaction
REM rapid eye movement
ROS reactive oxygen species
SWS slow-wave sleep
TCM traditional Chinese medicine
ZJS Ziziphus jujuba Mill. var. spinosa
ZJSP polyphenols of Ziziphus jujuba Mill. var. spinosa
Keywords: spinosin, pharmacology, pharmacokinetics, toxicity, Ziziphus jujuba Mill. var. spinosa
Citation: Kuang X, She G, Ma T, Cai W, Zhao J, Liu B and Xu F (2022) The pharmacology, pharmacokinetics, and toxicity of spinosin: A mini review. Front. Pharmacol. 13:938395. doi: 10.3389/fphar.2022.938395
Received: 07 May 2022; Accepted: 22 August 2022;
Published: 12 September 2022.
Edited by:
Muhamad Noor Alfarizal Kamarudin, Monash University Malaysia, MalaysiaReviewed by:
Jong Hoon Ryu, Kyung Hee University, South KoreaCopyright © 2022 Kuang, She, Ma, Cai, Zhao, Liu and Xu. This is an open-access article distributed under the terms of the Creative Commons Attribution License (CC BY). The use, distribution or reproduction in other forums is permitted, provided the original author(s) and the copyright owner(s) are credited and that the original publication in this journal is cited, in accordance with accepted academic practice. No use, distribution or reproduction is permitted which does not comply with these terms.
*Correspondence: Bo Liu, ZG9jdGxpdUAyNjMubmV0; Fangfang Xu, eHVkdWJmYW5nQDE2My5jb20=
†These authors have contributed equally to this work
Disclaimer: All claims expressed in this article are solely those of the authors and do not necessarily represent those of their affiliated organizations, or those of the publisher, the editors and the reviewers. Any product that may be evaluated in this article or claim that may be made by its manufacturer is not guaranteed or endorsed by the publisher.
Research integrity at Frontiers
Learn more about the work of our research integrity team to safeguard the quality of each article we publish.