- 1School of Medicine, Kashan University of Medical Sciences, Kashan, Iran
- 2Student Research Committee, Kashan University of Medical Sciences, Kashan, Iran
- 3Faculty of Medicine, Mashhad University of Medical Sciences, Mashhad, Iran
- 4Department of Medicine, Mashhad Medical Sciences Branch, Islamic Azad University, Mashhad, Iran
- 5Research Center for Biochemistry and Nutrition in Metabolic Diseases, Institute for Basic Sciences, Kashan University of Medical Sciences, Kashan, Iran
- 6Laser Research Centre, Faculty of Health Science, University of Johannesburg, Doornfontein, South Africa
- 7Infectious Disease Research Center, School of Nursing and Midwifery, Kashan University of Medical Sciences, Kashan, Iran
- 8Clinical Research Development Center, Mahdiyeh Educational Hospital, Shahid Beheshti University of Medical Sciences, Tehran, Iran
Many cellular signaling pathways contribute to the regulation of cell proliferation, division, motility, and apoptosis. Deregulation of these pathways contributes to tumor cell initiation and tumor progression. Lately, significant attention has been focused on the use of natural products as a promising strategy in cancer treatment. Quercetin is a natural flavonol compound widely present in commonly consumed foods. Quercetin has shown significant inhibitory effects on tumor progression via various mechanisms of action. These include stimulating cell cycle arrest or/and apoptosis as well as its antioxidant properties. Herein, we summarize the therapeutic effects of quercetin in gastrointestinal cancers (pancreatic, gastric, colorectal, esophageal, hepatocellular, and oral).
1 Introduction
Nowadays, cancer is the major cause of death worldwide. Nearly 17 million people from 185 countries are affected by 36 different kinds of cancer, according to a report by the International Agency for Research on Cancer (IARC) and the American Cancer Society (ACS) (Miller et al., 2020; Schiller and Lowy 2021). Cancer occurs because the normal control of physiologic cell multiplication has failed, and the cells undergo mitosis many times to form a tumor mass. Moreover, cancer cells can migrate to different parts of the body to form secondary tumors or metastases. Benign tumors mostly remain limited to their site of origin and do not spread to other parts of the body, while malignant tumors, on the other hand, have a strong tendency to spread. There are three routes for metastasis: lymphatic, hematogenous, and by invasion into adjacent tissues and secondary organs (Patel 2020). The best way to improve treatment of different kinds of cancer is to diagnose the cancer at an early stage and to select the most suitable treatment option for the tumor type and stage, including surgery, radiotherapy, chemotherapy, radiochemotherapy, hormonal therapy, targeted therapy, or immunotherapy. Although there are many different kinds of treatment, the best approach is to use a combination of several types. Moreover, the physician should consider the side effects of each approach and choose an overall low-risk, high-benefit treatment (Colli et al., 2017; Schirrmacher 2019). In the past, doctors have investigated natural drugs, especially plant products, along with limited surgical interventions. There are many historical documents describing traditional medicine, such as Indian Ayurvedic practice and traditional Chinese medicine, which used plant components to treat patients. One advantage of natural products is that we have enormous resources of plant, fungal, and microbial species that can be used against cancer if they have tolerable toxicity. Natural products can be considered an opposite point of view to the present pharmaceutical-centered culture and are often considered preferable by holistic and complementary physicians. Nowadays, there are many anticancer medicines which have been obtained from plants, animals, microorganisms, and the marine environment (Chamberlin et al., 2019). McCulloch et al. and Firenzuol et al. both emphasized the need of more studies to evaluate the efficacy of natural products in cancer therapy and to compare them to standard pharmacological treatment. Moreover, it is likely that natural products will be combined with pharmaceutical drugs in the future (Firenzuoli et al., 2004; McCulloch et al., 2011). Many medicinal herbs, such as Curcuma species, Cinnamomum species, and Artemisia species, and their components have been used in cancer therapy (Banikazemi et al., 2021; Davoodvandi et al., 2021). Medicinal herbs can inhibit tumor cell growth, induce apoptosis, and inhibit angiogenesis (Bevara et al., 2018; Jung et al., 2018; Mayzlish-Gati et al., 2018; Zhou et al., 2019a). Flavonoids are a diverse class of phytonutrients found in almost all fruits and vegetables. They include isoflavones, anthocyanidins, flavanones, and flavonols. Although their molecular structures differ, the presence of flavonoids significantly increases the bioavailability of other compounds (Böhm 1994; Gu et al., 2004; Ding et al., 2016). Many studies have emphasized the beneficial effects of flavonoids in the daily diet and suggested that the consumption of flavonoids could be effective in reducing the risk of chronic heart and brain disorders and cancer (Kozłowska and Szostak-Wegierek 2014; Panche et al., 2016; Honari et al., 2019; Hoseini et al., 2019; Maleki Dana et al., 2021). One prominent member of the flavonoid family is quercetin (3,3′,4′,5,7-pentahydroxyflavone), which is present in different kinds of fruits and vegetables, such as buckwheat, broccoli, and onions. Nowadays, quercetin is used as a dietary supplement and could be used as an anticancer agent in the daily diet (Vargas and Burd 2010; Vafadar et al., 2020). There are many studies which demonstrate that quercetin consumption at a tolerable dosage could have beneficial biological effects, including antioxidant, anticancer, and anti-inflammatory effects (Li et al., 2016). In this article, we summarize the anticancer properties of quercetin against gastrointestinal (GI) cancers in vivo and in vitro and also assess its possible inhibitory effect on human cancers based on its cellular and molecular mechanisms.
2 Quercetin: Potential, Bioavailability, and Mechanisms of Action in Cancer Therapy
Quercetin is a member of the flavonoid family with a flavone nucleus, composed of a heterocyclic pyrone ring that links the two benzene rings to form the central nucleus (Russo et al., 2014). The main form of quercetin found in herbs is composed of hydrophilic glycosides (sugar conjugates), which are not easily and directly absorbed by enterocytes. The daily dosage of quercetin for human usage is 10–100 mg, which could be obtained from 500–1,000 mg of purified extract (Brito et al., 2015). Within enterocytes, quercetin undergoes a variety of enzymatic reactions, including methylation, hydrolysis, sulfonylation, and glucuronidation, by specific transferase enzymes (Brito et al., 2015). Following transportation into the intestinal lumen and then the liver, quercetin metabolites (the major quercetin-derived circulating compounds in plasma, quercetin-3-glucuronide and quercetin-3ʹ-sulfate) are formed via other conjugation reactions (Alrawaiq and Abdullah 2014). There are two forms of quercetin: conjugated and non-conjugated; the plasma concentration of each is 3.5–5.0 μmol/L and <0.33 μmol/L, respectively. The form of quercetin which is absorbed by enterocytes is the conjugated form (Alrawaiq and Abdullah 2014). Recently, a new study revealed that the microbes of the gut play an important role in quercetin absorption because they have enzymes which render the quercetin molecules smaller and more absorbable (Xu et al., 2017; Santangelo et al., 2019). Quercetin can be metabolized to glucuronidated, methylated, or sulfated derivatives (D'Andrea 2015). As previously stated, quercetin has several anticancer effects that have been demonstrated in numerous in vitro and in vivo studies. Its antitumor effects include its antioxidant activity, inhibition of angiogenesis, inhibition of the cell cycle and proliferation, and prevention of tumor metastasis. In addition, quercetin has many other beneficial properties that make it an effective supplement in the daily diet, including anti-inflammatory effects, antihypertensive effects, antithrombotic effects, anti-atherosclerosis properties, and anti-arrhythmia activity. Therefore quercetin has attracted attention from researchers as an adjuvant agent to take advantage of its antitumor, antioxidant, cytoprotective, and anti-proliferative properties (Lugli et al., 2009). Many studies have been conducted to evaluate the beneficial antitumor effects of quercetin against the kidney, breast, prostate, lung, ovarian, colorectal, pancreatic, and nasopharyngeal cancer (Lee et al., 2015a; Liu et al., 2017a; Baby et al., 2018; Huang et al., 2018; Li et al., 2018; Polukonova et al., 2018; Yang et al., 2019a). One of the most important features of quercetin is its pro-apoptotic effect, which is caused by increasing pro-apoptotic molecules such as P53, BAX, caspase-3, and caspase-9 or stimulating the mitochondrial apoptosis pathway or decreasing antiapoptotic proteins (Roos and Kaina, 2006; Zhang et al., 2008a; Zhang et al., 2009a; Tan et al., 2009). Quercetin could regulate and inhibit the cell cycle by activating p21 and decreasing D1/Cd4 and E/Cdk2 ratios. Quercetin can arrest the cell cycle at the G1 phase as well as inhibit microtubule polymerization which also affects the cell cycle (Gupta and Panda, 2002; Moon et al., 2003). There are various mechanisms and pathways by which quercetin can regulate the cell cycle, some of which can increase the cell cycle, such as the PI3K/AKT/PKB pathway. Moreover, quercetin can inhibit carcinogenesis and metastasis along with apoptosis induction (JÁF, 2004; Gulati et al., 2006). p53 is a key molecule in the regulation of cell death and cell survival pathways and plays a role in cancer therapy. p53 can act as an antioxidant by protecting the cellular DNA from oxidative damage and by regulating genes for endogenous antioxidants, such as catalase, Gpx1, microsomal GSH homologous transferase PIG12, Mn-SOD2, and aldehyde dehydrogenase ALDH4A1. Many research works have confirmed that quercetin can stabilize p53 levels and increase its phosphorylation (Polyak et al., 1997; Hussain et al., 2004; Yoon et al., 2004; Tanigawa et al., 2008). In malignant cells, p53 genes can be blocked or mutated, causing loss of functions (Gibellini et al., 2011). Another antioxidant function of quercetin is to quench reactive oxygen species (ROS), thereby preventing ROS-mediated DNA damage (Metodiewa et al., 1999; Awad et al., 2000). Therefore, quercetin has the potential to be used in cancer treatment because of its ability to regulate the cell cycle, antioxidant effects, p53 stabilization, and apoptosis induction. The potent anticancer activity and structure of quercetin are illustrated in Figure 1.
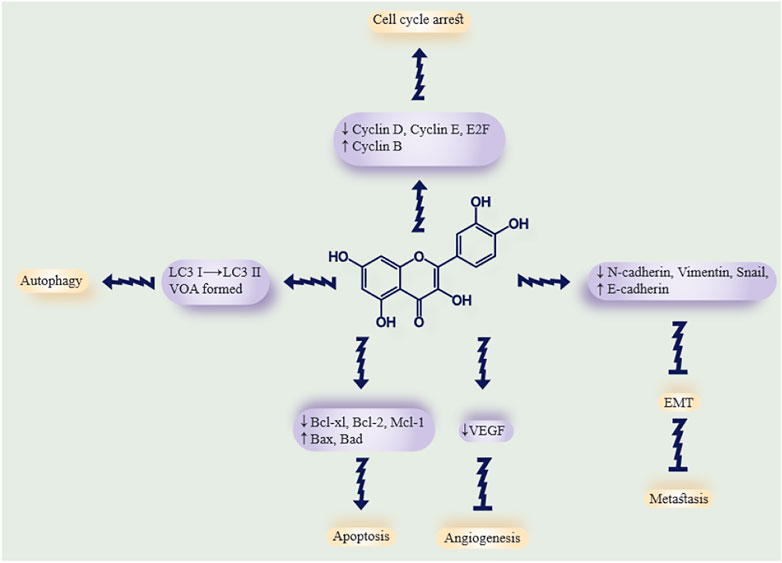
FIGURE 1. Summary of the antitumor properties of curcumin. The effects of quercetin on tumor cells and the possible molecular targets for each effect. The arrows show quercetin antitumor mechanisms, while the blunt lines show tumor-suppressive effects. Downwards or upwards arrows indicate downregulation or upregulation of molecular targets.
3 Quercetin and Gastrointestinal Cancer
3.1 Quercetin and Pancreatic Cancer
Yangyang Guo and others evaluated the effects of quercetin on pancreatic ductal adenocarcinoma (PDA) using different techniques, including a real-time cell analysis, migration, proliferation, and invasion, and used a nude mouse tumor formation assay. They evaluated tumor growth and metastasis as well as colony formation, cell migration, and flow cytometry analysis of SHH signaling in pancreatic cancer cells (PCCs), in vivo and in vitro (Guo et al., 2021a). Quercetin showed an antitumor activity by downregulating c-Myc, leading to inhibition of PCC proliferation. Quercetin reduced the level of TGF-β1 and suppressed the epithelial-mesenchymal transition, thereby blocking migration and invasion. Quercetin induced apoptosis via death receptor pathways and the mitochondrial pathway. In an animal study in nude mice, treatment with quercetin reduced metastasis. The therapeutic effects of quercetin on PDA involve the regulation of SHH activity and are related to Gli2 but not Gli1. The quercetin-mediated suppression of PCC proliferation, migration, and invasion was abrogated when the SHH activity was increased using recombinant Shh protein. Moreover, by promoting the expression of Zeb2 and Snail1, Shh could activate TGF-β1/Smad2/3 signaling and promote EMT, resulting in a partial reversal of the quercetin-mediated suppression of PCC migration and invasion. These findings suggest that quercetin could be used to treat PDA by inhibiting PCC migration, metastasis, and invasion and by inducing apoptosis via antagonizing the TGF-β1/Smad and SHH signaling pathways (55).
Sarah Hassan and her colleagues evaluated the efficacy of a combination of quercetin with the known drugs gemcitabine (GEM) and doxorubicin (DOX) against human pancreatic cancer cells and hepatic cancer cells, respectively. They also evaluated the effects of quercetin on the activity of drug efflux pumps as well as the effects related to hypoxia. Moreover, they investigated the role of multidrug transporters (including MDR) and HIF-1α (Hassan et al., 2020). In their research, they combined quercetin with other anticancer drugs and showed that combination therapy (anticancer drug plus quercetin) had better results than single therapy. In 2D and 3D cultures, the percentage of dead cells increased to 60%. Deeper evaluation revealed that quercetin induced p53-mediated apoptosis via increased levels of the p53 regulator and also downregulated HIF-1α. Moreover quercetin could inhibit the MDR-1 efflux activity. In conclusion, they found quercetin could be administered in combination with GEM or DOX in multidrug resistant pancreatic and hepatic cancers, respectively (Hassan et al., 2020).
Liu et al. (2020) evaluated the effects of quercetin on GEM-resistant PC cells and its mechanism. They evaluated two cell lines from each of pancreatic and hepatocellular carcinoma. The PCC lines were BxPC-3 and PANC-1, and the HCC cell lines were HepG2 and Huh-7. Using a proliferation assay, it was found that quercetin had a cytotoxic effect on HepG2 and PANC-1 (GEM-resistant), and a flow cytometry analysis revealed a pro-apoptotic effect on HepG2 and PANC-1. Quercetin could induce apoptosis via upregulation of antitumor protein p53 and also cyclin-D1 downregulation, as seen by Western blotting. Moreover, it caused cell cycle arrest in the S phase. In conclusion, their data revealed that quercetin could be used in combination with known anticancer drugs against GEM-resistant hepatic and pancreatic cancers (Liu et al., 2020).
Hoca et al. (2020a) evaluated the effect of quercetin and resveratrol on the epithelial-mesenchymal transition (EMT) in pancreatic cancer cells (CD133-negative and CD133-positive). The CD133+ cancer stem cells were purified from PANC-1 cells using the MiniMACS system, and then the three cell variants (CD133+, CD133-, and PANC-1) received different doses of quercetin and resveratrol. Immunocytochemistry with antibodies against vimentin, TNF-α, ACTA-2, N-cadherin, IL-1β, and the MTT assay were employed. In the CD133 + cells that were treated with quercetin, the intensity of N-cadherin, ACTA-2, and IL-1β staining was reduced, compared to CD133 + cells treated with resveratrol. In conclusion, quercetin could reduce N-cadherin expression and prevent EMT and metastasis more than resveratrol in PCC stem cells (Hoca et al., 2020a). Table 1 lists some studies on the therapeutic effects of quercetin in pancreatic cancer.
3.2 Quercetin and Gastric Cancer
Cing-Syuan Lei et al. tested whether a combination of quercetin and irinotecan might be effective for decreasing the metastasis of gastric cancer (GC) by measuring gene and protein expression (Lei et al., 2018). Their study compared the effect of low-dose SN-38 (irinotecan metabolite) in combination with quercetin with a high dose of SN-38 alone on β-catenin expression, cell viability, and apoptosis. In vivo xenograft animal models and in vitro studies looked at the effects of quercetin and low-dose irinotecan on GC metastasis. The β-catenin protein levels were lower in AGS cells treated with quercetin and a low dose of SN-38 than the single therapy using quercetin. ITG-β6 and Twist-1 gene expression (two EMT markers) as well as cyclooxygenase-2 gene expression were higher in high-dose irinotecan-treated cells than the combination therapy. In the AGS mouse model, VEGF-A (vascular endothelial growth factor), VEGF-receptor 2, and the percentage of Tie2-expressing monocytes were significantly lower after combined therapy. The data suggested that the treatment of GC with irinotecan could be improved by combining it with quercetin (Lei et al., 2018).
Hemati et al. evaluated the effect of si-RNA targeted against CDC20 (cell division cycle protein 20 homolog) and antiproliferative drugs (quercetin and DOX) against GC. They investigated niosome-encapsulated delivery vehicles for si-RNA and drugs. They found that si-RNA delivery in combination with anticancer drugs led to downregulation of CDC20, and therefore improved GC treatment (Hemati et al., 2019). To optimize the si-RNA loading capacity and physicochemical properties, they varied the cationic lipid content of cationic PEGylated niosomes. Quercetin and DOX as well as anti-CDC20 si-RNA were loaded into the co-delivery system, and physicochemical properties, controlled release, thermosensitivity, rates of apoptosis, and gene silencing efficacy were measured. Intriguingly, the data revealed that the co-delivery system, which was designed for loading si-RNA, had an appropriate high positive charge for drug delivery. They also showed a thermosensitive drug release behavior that successfully silenced CDC20 expression when compared with the single delivery of either si-RNA or the drug. Furthermore, their system effectively inhibited GC cell growth. Their data suggested that PEGylated niosomes co-loaded with CDC20 si-RNA plus anticancer drugs might be used as a novel system for GC treatment (Hemati et al., 2019).
Hai Li et al. evaluated the mechanism and effects of quercetin on metastasis of GC and also searched whether urokinase plasminogen activator and urokinase plasminogen receptor (uPA/uPAR) were involved in the mechanism or not. The uPA/uPAR system plays a key role in GC metastasis, so they planned the study to test whether quercetin could affect this system. In their study, they measured the amount of uPA and uPAR activity in precancerous tissues and compared them with different GC cell lines in terms of migration and invasion (Li and Chen 2018a). The data revealed that in precancerous tissues, uPA and uPAR activities were lower than that in GC cells, and the migration and invasion of GC cell lines were correlated with uPAR expression. uPA and uPAR protein expression levels were reduced along with migration and invasion after GC BGC823 and AGS cells were treated with quercetin (10 µM for 72 h). Quercetin combined with uPAR knockdown decreased matrix metalloproteinase-9 and metalloproteinase-2 activities, thereby inhibiting Pak1-Limk1-cofilin signaling. Quercetin treatment inhibited AMPKα activator, NF-κB, ERK1/2, and PKC-δ, which caused downregulation of uPA and uPAR expression. In conclusion, quercetin could be a novel component in GC therapy for reducing metastasis and invasion (Li and Chen 2018a).
Zeng et al. (2018) evaluated the effects of quercetin on GC. They treated human GC cells (NCI-N87) with 15 μM quercetin for 48 h along with dimethyl sulfoxide as a control. The HiSeq 2500 DNA sequence data were used to compare differentially expressed genes (DEGs) between groups. An advanced analysis was used to assess the protein–protein interaction (PPI) network. The regulatory network of transcription factors (TFs-DEGs) was elucidated using Cytoscape. The DEGS found were Fos proto-oncogene (FOS, degree = 12), aryl hydrocarbon receptor (AHR, degree = 12), Jun proto-oncogene (JUN, degree = 11), and cytochrome P450 family 1 subfamily A member 1 (CYP1A1, degree = 11), which were significantly associated with other proteins in the PPI network with higher degrees. Early growth response 1 (EGR1), FOS like 1 (FOSL1), FOS, and JUN were higher among the five TF-DEGs, whereas AHR was downregulated. The Wnt signaling pathway was also enriched for FOSL1, JUN, and Wnt family member 7B (WNT7B). In the PPI network, CYP1A1 was closely linked to AHR. Therefore, quercetin may have targeted FOS, AHR, JUN, CYP1A1, EGR1, FOSL1, and WNT7B in GC (Zeng et al., 2018).
Shang et al. (2018a) and his colleagues carried out in vitro studies on the potential of quercetin to induce human GC cell death, apoptosis, and alter gene expression. Their data revealed that quercetin could induce GC cell apoptosis and also change gene expression. Flow cytometry revealed that quercetin increased the level of reactive oxygen species (ROS) and led to the destruction of the mitochondrial membrane by reducing certain protein levels, and finally it caused apoptosis in AGS cells. The Western blotting showed that quercetin decreased the level of antiapoptotic proteins, including Bcl-x, Bcl-2, and Mcl-1, while increasing pro-apoptotic proteins, including Bid, Bax, and Bad. Quercetin induced various effects on gene expression. For instance, quercetin decreased the expression of KDELC2F (KDEL [Lys-Asp-Glu-Leu] containing 2), VEGF-B (vascular endothelial growth factor B), and CDK10 (cyclin-dependent kinase 10) but increased the expression of TP53INP1 (tumor protein p53 inducible nuclear protein 1), TNFRSF10D (tumor necrosis factor receptor superfamily 10D, decoy with truncated death domain), JUN-B (jun B proto-oncogene), and TP53INP1. In conclusion, their data revealed the molecular mechanism, gene expression, and signaling pathway involved in quercetin’s ability to inducing apoptosis in human GC cells (Shang et al., 2018a). Table 2 lists some studies on the therapeutic effects of quercetin in GC.
3.3 Quercetin and Colorectal Cancer
Mostafa et al. carried out a study to design and synthesize multi-target anticancer drugs for CRC treatment. Their agents targeted some essential enzymes for CRC survival and proliferation, including PIM-1, 5-LOX, and COX-2 (117). Compounds 5a-d and 5g inhibited the COX-2 enzyme similar to celecoxib with a high selectivity index. Compound 5b-e inhibited the 5-LOX enzyme to the same extent as quercetin, while 5g, 5f, and 5a showed slightly lower inhibitory activity than quercetin. They concluded that 5e-g and 5a-c were better than Celecoxib, while 5g, 5f, and 5a were better than diclofenac sodium, in an in vivo model. Compounds 5e-g and 5a-c showed a better safety profile than celecoxib in fasted rats. Compounds 5g, 5f, and 5d had the highest potency against CRC cell lines (HCT-116 and Caco-2) at a much lower dosage than against normal human cells. Moreover, compounds 5g and 5e induced apoptosis in CRC cell lines and induced caspase activation. Compounds 5d, 5g, and 5e inhibited PIM1 and PIM2 kinase, which was comparable to the reference staurosporine (El-Miligy et al., 2021).
Ghamedi et al. evaluated the mechanism and effects of a 200 mg dose of quercetin and a 150 mg dose of EGCG (epigallocatechin gallate) at varying proportions on the induction of apoptosis and inhibition of proliferation of human CRC cell line HCT-116 (Al-Ghamdi et al., 2021). The phytomolecules inhibited cell growth, arrested cell cycle, annexin V, and reduced clonogenicity. Colony formation was inhibited by the lowest dose of the tested drugs. Furthermore, a significant increase in annexin V was found at 150 mg dose of quercetin and 100 mg dose of EGCG. The combination therapy induced cell cycle arrest at the G1 phase. In conclusion, the combination of EGCG plus quercetin could be used as effective combination chemotherapy in the future, but more studies are required to establish a suitable dose and any side effects (Al-Ghamdi et al., 2021).
Erdoğan et al. (2021) evaluated the antiangiogenic effects of quercetin and luteolin on colon cancer cells (HT29) and also their anticancer effects compared to the traditional chemotherapy drug 5FU, as well as a combination of 5FU with luteolin or quercetin. They used Western blotting, qRT-PCR, human VEGF ELISA, fluorescence microscopy, and an MTT assay. They used Western blotting to assess the effects on the genes p53, Bax, Bcl-2, P38, MAPK, PTEN, Akt protein, and mTOR. ELISA was used to determine the effects of treatment on angiogenesis and the MTT assay to measure cell viability. Fluorescence microscopy was used to detect apoptosis in HT29 cells. The induction of apoptosis in cells treated with quercetin and 5FU was eight times higher than the control, and it was 10 times higher for luteolin and 5FU. The VEGF level was significantly lower in cells treated with a combination of quercetin or luteolin plus 5FU. They found that quercetin and luteolin could regulate apoptosis in HT-29 cells and also that combination therapy reduced antiapoptotic factors, including Bcl-2, mTOR, and Akt gene expression compared to the control group. P53, P38, MPK, and PTEN gene expression increased faster in groups treated with 5FU and quercetin than in cells treated with 5FU and luteolin. In conclusion, luteolin and quercetin could synergistically increase the anticancer effect of 5FU and could also reduce the toxic effects of 5FU in colorectal cancer (Erdoğan et al., 2021).
In a study by Wenhui Liu et al., they induced constipation (a known colorectal risk factor) by administering loperamid in a rat model, and then evaluated the effects of quercetin on loperamid-induced constipation (Liu and Zhi 2021). The data revealed that in rats that were treated with 25 mg/kg and 50 mg/kg of quercetin, the intestinal transit rate was increased, as well as the concentration of short-chain fatty acids and levels of gastrin, motilin, and substance P. Moreover, quercetin improved intestinal peristalsis movement and reduced somatostatin levels. The expression of aquaporin 3, transient receptor potential vanilloid 1, glial cell line-derived neurotrophic factor, enteric nerve-related factors, nitric oxide synthase, stem cell factor, and c-Kit was evaluated by Western blotting and RT-qPCR. They found that quercetin treatment reduced loperamid-induced constipation by increasing the markers of interstitial cells of Cajal, including stem cell factor, its receptor c-Kit, and AQP3. In conclusion, their data showed that quercetin had protective effects against loperamide-induced constipation, so it could be used to reduce the risk of colorectal cancer (Liu and Zhi 2021).
Iván Benito et al. evaluated the effects of daily supplementation with microencapsulated probiotics alone or in combination with microencapsulated quercetin, for the prevention of colorectal cancer. They used ApcMin/+ mice which spontaneously develop intestinal adenomas and carcinoma. They assessed histological alterations, intestinal bleeding, fat depots, respiratory quotient, body weight, and energy expenditure. Furthermore, they evaluated gene expression involved in the Wnt signaling pathway (Benito et al., 2021). ApcMin/+ mice were administered with Bf and Lg probiotic strains, 10 (7) CFU/100 g of food, or both probiotic strains plus microencapsulated quercetin (15 mg/100 g of food) for 73 days. Then they evaluated the energy metabolism, changes in organ and body weight, colon tissue histology, intestinal microbiota, and Wnt signaling pathway gene expression. The data revealed that the microencapsulated supplement (probiotics plus quercetin) could prevent colorectal cancer progression in ApcMin/+ mice (Benito et al., 2021).
Shree et al. (2021) evaluated the role of quercetin in apoptosis, hyperproliferation, and inflammation and also its mechanism in 1,2-dimethylhydrazine (DMH)-induced carcinogenicity and tumor multiplicity. They administered the rats with quercetin at 25 or 50 mg/kg body weight orally and 20 mg/kg bodyweight of DMH subcutaneously for 15 weeks and then sacrificed them. The DMH produces reactive oxygen species (superoxide) by membrane lipid peroxidation and causes an imbalance in redox homeostasis. DMH also decreases tissue antioxidant levels. Proliferative and inflammatory factors were increased in DMH-induced intestinal carcinogenicity as a result of low Bax/Bcl-2 ratio and apoptosis dysregulation. Quercetin pretreatment reduced the harmful effects of DMH, including preserving the detoxifying enzyme activity and reducing proliferation and early markers (mucin depletion and goblet cell disintegration) in colonic tissue. Quercetin regulated the expression of β-catenin and APC and lowered the incidence and multiplicity of tumors. The histological results further confirmed the beneficial role of quercetin in reducing DMH-induced pathological alterations (Shree et al., 2021). Table 3 lists some studies on the therapeutic effect of quercetin in colorectal cancer.
In a study by Antara Banerjee et al., the effects of a combination of Lycopodium clavatum and quercetin on CRC cells (Colo-320) were evaluated by measuring expression of extracellular matrix proteins, cytotoxicity assay, morphological alterations, and expression of apoptotic genes. Furthermore, the anti-inflammatory, apoptotic, and proliferative responses of cancer cells treated with Lycopodium and quercetin alone or in combination, were evaluated to assess potential synergistic effects and expression of tumor suppressor genes (Banerjee et al., 2020). Their study was mainly conducted to identify whether L. clavatum extract or quercetin or a combination could produce significant anti-inflammatory effects in Colo-320 cells. Gelatin zymmography, toxicity biomarkers, and apoptotic gene expression were measured. The combination of quercetin (50 μm) and L. clavatum extract (10 μL) significantly attenuated cell growth and decreased the proliferation potential and colony formation compared to their separate administration. Antimicrobial assays showed that Lycopodium exerted antimicrobial activity against Pseudomonas aeruginosa and Escherichia coli. The quercetin and L. clavatum extracts contain flavonoids and alkaloids. Gelatin zymography showed a prominent decrease in MMP9 and MMP2 activity following administration of the quercetin and Lycopodium combination. This regimen altered the expression of catalase, cyclin D1, Bcl2, caspase-3, Bax, and Wnt1 genes in colon cancer cells. Quercetin and Lycopodium showed synergistic effects which may effectively attenuate progression of CRC with few adverse effects and no drug resistance (Banerjee et al., 2020).
Yana Li et al. evaluated whether quercetin could sensitize colon cancer cells to radiotherapy, considering the fact that quercetin can inhibit the Notch-1 signaling pathway and thus reduce proliferation of colon cancer cells and CSCs (colon cancer stem cells) (Li et al., 2020). Their study showed that a combination of quercetin with ionizing radiation (IR) had more significant antitumor effects than either IR or quercetin alone by suppressing the Notch-1 signaling pathway in CSCs. These findings were further validated using an in vivo xenograft of human colon cancer in nude mice, which showed a significant reduction in CSC markers and Notch-1 signaling proteins. Combined treatment with low-dose IR and quercetin remarkably decreased the expression of all gamma-secretase complex proteins in DLD-1 and HT-29 cells. Moreover, the combination therapy was partly abrogated by altering the expression of NICD (Notch intracellular domain). Finally, their study suggested that a combination of IR (5 Gy) and quercetin (20 mM) could be a novel therapeutic approach in colon cancer by suppressing the Notch-1 signaling pathway in CSCs. Further studies are required to confirm the therapeutic potential of combined quercetin–radiotherapy in clinical trials (Li et al., 2020).
3.4 Quercetin and Esophageal Cancer
In a study by Xin et al., it was shown that NF-κB plays a major role in resistance to chemotherapy in esophageal cancer cells treated with 5-FU. Their study mainly focused on the potential benefits of quercetin on the chemosensitivity of human esophageal cancer and identifying the underlying antitumor mechanism (Chuang-Xin et al., 2012a). Their study evaluated the effect of combination of quercetin and traditional chemotherapeutic drugs on esophageal carcinoma. The MMT assay was employed to measure the effects of quercetin on Eca109 and EC9706 cell proliferation. Western blotting was employed to evaluate protein levels, while apoptosis was investigated using FACS (Annexin V-FITC/propidium iodide). Their study showed that the combination of 5-FU and quercetin considerably reduced cell growth and provoked apoptosis in esophageal cancer cell lines (Eca109 and EC9706) in comparison with either 5-FU or quercetin alone. These effects were correlated with the downregulation of phosphorylated inhibitor of NF-κB (pIκBα) in response to treatment with 5-FU alone. In conclusion, the addition of quercetin to the conventional chemotherapeutic drug 5-FU may improve treatment of esophageal cancer (Chuang-Xin et al., 2012a).
Zhao et al. investigated whether quercetin-3-methyl ether (Q3ME), which is a flavonoid frequently found in tea, vegetables, fruits, wine, and tea, could inhibit the formation and progression of preneoplastic esophageal lesions produced by N-nitrosomethylbenzylamine. They studied inflammation and proliferation of esophageal cells in vivo. Q3ME suppressed esophageal cancer cell proliferation as well as the malignant transformation of healthy esophageal cells by inhibiting the MAPK and AKT/mTOR/p70S6K signaling pathways. Q3ME inhibited esophageal tumorigenesis by targeting ERKs and AKT (Zhao et al., 2018a). They found that phosphorylated ERKs (p-ERKs) and p-AKT were overexpressed in esophageal cancer cell lines and in tissue samples from patients with esophageal cancer. Using a human pull-down assay and a phosphokinase array, they showed that Q3ME could interact with ERKs and AKT and inhibit their kinase activity. Mechanistically, Q3ME reduced proliferation of esophageal cancer cells and anchorage-independent growth. According to Western blotting, Q3ME could inhibit the activity of ERK and AKT downstream signaling pathways and subsequently suppress AP-1 (activating protein-1) transcription factor. Interestingly, Q3ME also suppressed the development of preneoplastic lesions caused by NMBA. This inhibitory effect was correlated with attenuated proliferation of esophageal cancer cells and lower inflammation in vivo (Zhao et al., 2018a).
Yue Liu et al. evaluated the effects of quercetin on the angiogenesis and migration of esophageal cancer cells, in addition to the underlying mechanism (Liu et al., 2021). In their study, human esophageal cancer cells (Eca109) received 5 or 10 μg/ml of quercetin. A scratch wound healing assay evaluated cell migration, invasion was examined using a transwell assay, and a colony formation assay was conducted. Human umbilical vein vascular endothelial cells (CLR-1730) were inoculated in Eca109 conditioned medium, and the effects of quercetin were measured by tube formation and wound healing assays. Western blotting measured the levels of MMP2, MMP9, and VEGF-A protein expression. Quercetin (10 μg/ml) decreased colony formation in Eca109 cells; however, no difference was observed between the control group and the 5 μg/ml quercetin group. The group treated with 10 μg/ml quercetin showed reduced cell migration and invasion, while 5 μg/ml only suppressed invasion. Tube formation ability and migration of endothelial cells was inhibited in cells incubated in Eca109 conditioned medium. The group treated with 10 μg/ml quercetin showed reduced levels of MMP2, MMP9, and VEGF-A (Liu et al., 2021). Table 4 lists some studies on the therapeutic effects of quercetin in esophageal cancer.
3.5 Quercetin and Hepatocellular Carcinoma
Yamada et al., reported that myricetin and quercetin could suppress the AKT signaling axis, which may subsequently inhibit TGF-α- and HGF-mediated HuH7 cell migration (Yamada et al., 2020). In their study, the effects of quercetin on the migration of HuH7 cancer cells induced by TGF-α or HGF were investigated. Quercetin prominently inhibited the migration of HuH7 cells mediated by both TGF-α and HGF in a dose-dependent manner. Furthermore, myricetin (another flavonol compound) also prominently suppressed the migration of cancer cells. Neither myricetin nor quercetin affected the autophosphorylation of receptors mediated by TGF-α and HGF. Moreover, quercetin did not affect the phosphorylation of p38 MAPK induced by TGF-α or HGF. On the other hand, both myricetin and quercetin suppressed AKT phosphorylation mediated by the growth factors. Their study showed that quercetin could inhibit HCC cell migration mediated by growth factors by blocking the AKT signaling pathway but not p38 MAPK (Yamada et al., 2020).
Zhao et al., conducted a study to evaluate whether a combination of quercetin plus cisplatin could produce synergistic effects in HCC cells (Zhao et al., 2014). The hepatocellular carcinoma cell line HepG2 was treated with either cisplatin (10 μM) or quercetin (50 μM) or their combination, and apoptosis and cell proliferation were measured. The cisplatin and quercetin combination triggered apoptosis and inhibited proliferation in HepG2 cells compared with either agent alone. The combination of cisplatin and quercetin affected the expression of genes involved in the cell cycle and apoptosis. Administration of quercetin alone led to a significant increase in p16 expression in HepG2 cells. G1 phase cell cycle arrest induced by quercetin and apoptosis in HepG2 cells were partly abrogated by p16 targeted knockdown using RNA interference technology. Therefore, the cell cycle arrest and apoptosis caused by quercetin plus cisplatin likely involved p16 and provided a more prominent antiproliferative and apoptotic effect (Zhao et al., 2014).
Ding and colleagues showed that quercetin could inhibit the proteasome activity and decrease the phosphorylation of ERK1/2. The increased activity of ERK1/2 led to an increase in the proteasome chymotrypsin-like activity, whereas the increased activity of MEK1 resulted in a lower proteasome chymotrypsin-like activity. Administration of quercetin mitigated the expression of proteasome β subunits. Their study showed that the MEK1/ERK1/2 signaling pathway could modulate the expression of proteasome β subunits, leading to a lower proteasome chymotrypsin-like activity (Ding et al., 2018). Caspase and trypsin-like protease activities remained constant in HepG2 cells along with an increased activity of JNK and p38 MAPK and attenuated phosphorylation of ERK1/2. The decreased proteasome activity induced by quercetin may not be reversed following suppression of the JNK and p38 MAPK signaling pathways. Upregulation or downregulation of MEK1 enhanced or attenuated proteasome chymotrypsin-like activity, respectively. The expression of β subunits of proteasome was reduced by both MEK1/ERK1/2 inhibition and by quercetin administration (Ding et al., 2018).
Srisa-Nga et al. designed a study to prepare quercetin magnetic nanocarriers using polymeric micelles to allow monitoring and treatment of hepatocellular carcinoma. These polymeric micelles were characterized based on their morphology, size, and magnetic properties. They evaluated the cellular uptake, cytotoxicity, cell cycle analysis, and potential magnetic targeting in HCC cells transfected with hepatitis B virus (HepG2.2.15) (Srisa-Nga et al., 2019). They co-encapsulated superparamagnetic iron oxide nanoparticles (SPIONs) and quercetin (QCT) into mPEG750-b-OCL-Bz micelles (QCT-SPION micelles) and added methoxy-poly (ethylene glycol)-b-oligo-3-caprolactone in order to inhibit the growth of HepG2.2.15 cells. The QCT-SPION micelles were prepared using the film hydration technique. They had a spherical morphology with an average size of 22–55 nm. The optimized micelles showed a quercetin loading capacity and entrapment efficiency of 3.5 and 70%, respectively. The SPIONs alone had a T2 relaxivity of 137 mM−1 s−1, while the cluster of SPIONs inside the SPION-QCT micelles had a T2 relaxivity value of about 246 mM−1 s−1, implying a higher sensitivity for magnetic resonance imaging. The SPION-QCT micelles were taken up by HepG2.2.15 cells and showed more cytotoxicity than quercetin, along with cell cycle arrest at the G0/G1 phase. The SPION-QCT micelles accumulated in the vicinity of neodymium–iron–boron (NdFeB) magnetic discs, and the application of a strong magnetic field further inhibited tumor cell growth. These mPEG750-b-OCL-Bz micelles acted as a novel multifunctional co-delivery vehicle for SPIONs and quercetin for monitoring and treatment of HCC (Srisa-Nga et al., 2019).
Pasachan et al. designed a study to evaluate the antidiabetic effects of TTE (Tiliacora triandra Colebr) on the gluconeogenesis of liver cells and HCC cells (HepG2). The principal constituents of TTE include quercetin and epicatechin. They investigated the underlying mechanism and the in vivo effects in a type 2 diabetes mellitus (T2DM) rat model. They asked whether TTE could act as a nutritional agent in the management of T2DM and prevent complications such as non-alcoholic steatohepatitis, non-alcoholic fatty liver disease, and eventual cirrhosis (Pasachan et al., 2021a). They used a Diels aqueous extract of TTE to affect glucose synthesis in cells with T2DM and HepG2 cells. The HepG2 cells received TTE and purified quercetin and epicatechin. Hepatic gluconeogenesis was evaluated in rats with T2DM, which received either daily TTE (1,000 mg/kg body weight), metformin (30 mg/kg), or the combination of metformin plus TTE over a period of 12 weeks. Similar to quercetin and epicatechin, TTE stimulated gene expression of catalase, glutathione peroxidase, and copper–zinc superoxide dismutase. TTE lowered the synthesis of new glucose by suppressing phosphoenolpyruvate carboxykinase and glucose-6-phosphatase and stimulating AMP-induced protein kinase phosphorylation in HepG2 cells. Similar to metformin, TTE showed antidiabetic, anti-triglyceridemic, anti-hyperglycemic, and antioxidant effects in rats with T2DM. TTE may be used as a nutraceutical agent in individuals with insulin resistance, obesity, or those receiving antidiabetic medication (Pasachan et al., 2021a). Table 5 lists some studies on the therapeutic effects of quercetin in HCC.
3.6 Quercetin and Oral Cancer
Singh et al. (2020) investigated the effects of a combination of quercetin and resveratrol (at minimum non-toxic concentrations) on oral cancer. In their study, the combination caused cell death in Cal-33 oral cancer cells but not in HEK-293 normal cells. They found that stimulation of cyclin E (a cell cycle regulatory protein) and reduced expression of cyclin A led to cell cycle arrest at the S phase in Cal-33 cells. The comet assay in association with gamma-H2AX foci showed DNA injury, and the overexpression of Bax and PARP1 cleavage showed cellular apoptosis after the combination treatment. Resveratrol treatment, both alone and in combination, could downregulate the expression of histone deacetylase 1 (HDAC), HDAC8, and HDAC3. Collectively, the combined quercetin and resveratrol regimen suppressed cell growth and caused DNA damage and cell cycle arrest in oral cancer cells but not in non-cancer cells (Singh et al., 2020).
Yuan and others designed a study to identify the effects of quercetin on proliferation, migration, apoptosis, and invasion in the vincristine (VCR)-resistant P glycoprotein (P-gp) overexpressing oral cancer cell line, KB/VCR. The VCR sensitivity of KB/VCR cells was increased by inhibiting P-gp expression with quercetin. They found that the proliferation, invasion, and migration of KB/VCR cells were suppressed by quercetin, in addition to enhanced VCR sensitivity and apoptosis (Yuan et al., 2015). The anticancer activity of these agents was assessed in KB/VCR oral cancer cells in vitro. According to their study, 25–100 µM concentrations of quercetin could efficiently block proliferation, invasion, and migration of KB/VCR cells, while at concentration above 50 µM, it resulted in the G1 phase cell cycle arrest. According to apoptosis analysis, 50 or 100 µM of quercetin stimulated apoptosis in KB/VCR cells by inhibiting Bax expression and upregulating Bcl-2 and caspase-3. Dose ranges of 25–100 µM quercetin reduced drug efflux mediated by decreasing the expression of P-gp in KB/VCR cells. A combination of quercetin (50 µM) and vincristin (0.375 µM) was even more effective in inducing apoptosis. Consequently, quercetin may reduce drug resistance as a promising approach for treatment of oral cancer and other tumor types (Yuan et al., 2015).
In another study by Ma et al., the effects of quercetin on the human oral cancer SAS cell line were evaluated. They found that quercetin stimulated apoptosis by mitochondrial and endoplasmic reticulum stress signaling pathways in SAS cells (Ma et al., 2018). The effect of quercetin on cell death was investigated using flow cytometry, Western blotting, confocal laser microscopy, and Annexin V/propidium iodide (PI) double staining, at 6–48 h following quercetin treatment. They found that quercetin reduced cell viability and increased production of Ca2+ and reactive oxygen species. Annexin V/PI staining showed increased apoptosis, with a reduction in mitochondrial membrane potential (ΔΨm), in addition to modified expression of apoptosis-related proteins. Western blotting showed that quercetin increased Fas, Fas ligand, Fas-related protein with death domain, and caspase-8, which were correlated with death receptors on the surface of the cells. Quercetin upregulated ATF-6α (activating transcription factor), gastrin-releasing peptide-78, and ATF-6β, showing endoplasmic reticulum stress. The levels of pro-apoptotic protein BH3-interacting death-domain antagonist in conjunction with reduced expression of antiapoptotic proteins Bcl-2 and Bcl-extra-large protein, all of which reduced mitochondrial membrane potential. In addition, confocal microscopy showed that quercetin upregulated the expression of apoptosis-inducing factor, cytochrome c, and endonuclease G, all of which can stimulate apoptosis. Therefore, quercetin may be a promising anticancer drug in the treatment of oral cancer (Ma et al., 2018).
Huang et al. (2013) explored the antitumor effects of quercetin in human oral cancer cell lines with EGFR upregulation and assessed the role of FOXO1 transcription factor in growth inhibition induced by quercetin. They showed that FOXO1 played a critical role in suppressing cellular growth mediated by quercetin in oral cancer cells with high EGFR expression. Quercetin results caused cell cycle arrest at the G2 phase and stimulated apoptosis in TW206 and HSC-3 oral tumor cells with high EGFR expression. Quercetin activated FOXO1 and blocked EGFR/Akt activation. Silencing of FOXO1 decreased the expression of FasL and p21 mediated by quercetin and further increased apoptosis and G2 cell cycle arrest. Similarly, quercetin inhibited cancer growth in the mouse HSC-3 xenograft model (Huang et al., 2013).
Cheng et al. (2012) focused on the effects of quercetin on overcoming drug resistance and the underlying mechanisms in oral squamous cell carcinoma. They used a non-adhesive cell culture system, called the DRSP (drug-resistant sphere) model, in order to generate drug-resistant cells from oral tumor cell line SCC25. A comparative analysis was conducted between DSRPs and the parental control cells based on the measurement of EMT (epithelial-mesenchymal transition)-related markers, CSC features, and genes related to drug resistance in vitro. They also looked at carcinogenesis and tumor treatment in vivo. Their study on DRSPs showed overexpression of genes involved in drug resistance, including CSC-related markers and ABCG2, such that DRSPs had higher cisplatin (Cis) resistance and more CSC features than those in the control group. Furthermore, upregulation of p-Hsp27 (phosphorylated heat-shock protein 27) due to activation of the p38 MAPK signaling pathway was observed in DRSPs. Hsp27 silencing reduced the resistance to cisplatin and triggered apoptosis in DRSPs. Moreover, quercetin reduced the expression of p-Hsp27 and acted as an Hsp27 inhibitor to reduce the EMT status, resulting in the activation of apoptosis in DRSPs. Enhanced tumorigenesis was verified in DRSPs using a nude mouse xenograft model. Combined treatment by cisplatin and quercetin could reduce tumor growth and decrease drug resistance in OSCC. The p38 MAPK–Hsp27 signaling axis plays a major function in drug resistance induced by CSCs in oral cancer, and this could be inhibited by quercetin and cisplatin treatment (Cheng et al., 2012). Table 6 lists some studies on the therapeutic effects of quercetin in oral cancer.
4 Delivery of Quercetin
Quercetin and flavonoids have been used in the treatment of several human diseases, and they may be found in a wide range of foods and dietary supplements. Quercetin and its related compounds can have a wide range of beneficial effects, and numerous studies have been designed to overcome the drawbacks, such as low oral absorption and poor water solubility. Quercetin has low skin permeability, and some drug formulations have been produced to increase its permeability for topical application, such as nanocapsules, nanoemulsion, microemulsions, and solid–lipid nanoparticles (Nagula and Wairkar 2019).
Different delivery systems for flavonoids have been designed based on nanoformulations, which may enhance the efficacy of these molecules (Hariharan et al., 2006; Vicentini et al., 2008; Yen et al., 2009). For instance, nanoparticles, microemulsions, solid lipid nanoparticles, and liposomes have been widely used for different applications, particularly for neurodegenerative diseases and aging (Priprem et al., 2008; Wu et al., 2008).
When hydrophobic drugs are encapsulated within nanoparticles, they become completely dispersed in an aqueous environment and can then be administered intravenously. Antitumor drugs can be delivered to the tumor microenvironment when they are incorporated inside biodegradable polymeric nanoparticles, which have been explored as a novel drug delivery system in clinical trials (Park et al., 2009). For instance, PCL/PEG (poly-3-caprolactone)-polyethylene glycol) is a readily obtained biodegradable agent with amphiphilic properties that can be used as a drug delivery system (Gou et al., 2011). The use of PCL/PEG nanoparticles to increase the water solubility of hydrophobic compounds has been widely explored in recent studies. In a study by Gao et al. (2012) the effects of a quercetin nanoformulation on colon carcinogenesis and the proliferation of ovarian cells were investigated. Quercetin was encapsulated into biodegradable micelles made of monomethoxy-poly (ethylene glycol)-poly (ε-caprolactone) (MPEG-PCL) for the treatment of ovarian cancer. These MPEG-PCL micelles were loaded with quercetin (QU/MPEG-PCL) with a drug loading capacity of 6.9% and an average particle size of 36 nm and allowed the complete dispersion of quercetin in water.
Lipid nanocapsules (LNCs) can also be used for quercetin encapsulation because their lipid-content will dissolve quercetin. In a study by Hatahet et al., they prepared spherical lipid nanocapsules containing Cremophor EL ranging in size from 26 ± 3 nm to 54 ± 3 nm (Hatahet et al., 2017). The introduction of Cremophor El allowed better encapsulation of quercetin because this compound contains OH groups that interact with quercetin polar groups. The water solubility of quercetin increased up to 5,000 times, with adequate thermal stability at 4 and 25°C. Less than 15% of the quercetin loaded into LNC was released after 1 day, which suggests a long-term controlled delivery into the skin. Quercetin LNC’s anti-inflammatory activity was assessed in THP-1 cells (leukemic monocytes), which showed that there was no cytotoxicity, and quercetin may exert its anti-inflammatory effects by removing free radicals from the skin, thus protecting epidermal keratinocytes. Moreover, LNCs loaded with quercetin reduced reactive oxygen species and decreased inflammation (Hatahet et al., 2017).
MOFs (metal organic frameworks) have been studied recently as drug delivery vehicles as they possess a regular pore structure, significant pore volume, adequate chemical and thermal stability, and easy surface functionalization (Horcajada et al., 2008; Sun et al., 2011; Chen et al., 2014). Among these compounds, ZIF-8 (zeolitic imidazolate framework-8) is a biocompatible metal organic framework made up of 2-methylimidazolate and zinc ions. ZIF-8 has suitable stability in various physiological conditions but will disintegrate in the tumor tissue due to the lower extracellular pH. Therefore, ZIF-8 may serve as a novel drug delivery vehicle (Venna et al., 2010; Sun et al., 2012; Zhang et al., 2017b). PTT (photothermal therapy) employs light-induced hyperthermia to destroy the target tumor cells, as a new approach in cancer therapy (Liang et al., 2016; Li et al., 2017; Sheng et al., 2018). Much effort has been made to synthesize nanoparticles (NPs) as chemo-PTT platforms to improve the therapeutic efficacy and to overcome drug resistance in tumor cells (Yao et al., 2017a; Yao et al., 2017b; Tian et al., 2017). For instance, CuS NPs have been used as PTT agents and chemo-PTT nanocomposites (Gao et al., 2016; Liu et al., 2017b; Ren et al., 2017). These CuS NPs could be encapsulated in the ZIF-8 framework without changing the drug loading capacity or altering the pH sensitivity (Wang et al., 2016b). Jiang et al. (2018) asked whether quercetin could be loaded into ZIF-8, considering the opposite charges and the coordination effect between ZIF-8 and quercetin. In their study, a combined platform with quercetin as an antitumor agent and CuS NPs as a PTT agent was synthesized based on folic acid–bovine serum albumin (FA–BSA)-modified ZIF-8. Their study showed that the low water solubility, poor bioavailability, and chemical instability of quercetin could be improved by using FA–BSA/CuS@ZIF-8 as a drug delivery vehicle. According to in vivo NIRF imaging results, the tumor uptake was significantly increased by using the FA–BSA-modified MOF. Moreover, FA–BSA/CuS@ZIF-8-QT had minimal hemolysis and only minor toxic effects to normal organs, suggesting it could be safe for intravenous administration. Furthermore, the in vivo studies showed that the FA–BSA/CuS@ZIF-8-QT combined with laser irradiation showed a higher anticancer effect than PTT or chemotherapy alone, leading to significant inhibition of tumor growth. They concluded that FA–BSA/CuS@ZIF-8-QT could be a novel multimodal nano-platform for the administration of quercetin in cancer therapy (Jiang et al., 2018).
5 Conclusion
There are a wide range of treatment options for GI tumors. These treatment strategies may be used alone or combined with adjuvant or other therapies. Up to now, the available treatment strategies for GI tumors include: (Miller et al., 2020) surgical resection, the removal of the solid mass in patients without advanced disease; (Schiller and Lowy 2021) chemotherapy, the administration of cytotoxic drugs to destroy malignant cells; (Patel 2020) radiotherapy, used in the treatment of localized solid lesions; and (Colli et al., 2017) hormonal therapy, a systemic therapeutic approach targeting tumor cells all over the body. Nevertheless, effective tumor treatment often necessitates highly coordinated combinations of different therapeutic strategies. This approach is known as multi-modality treatment, including surgical resection, chemotherapy, radiotherapy, and hormone therapy. Since quercetin is able to exert major anticancer effects on GI tumors, this agent can be considered to be a promising antitumor natural compound. However, some questions regarding its application require further study. Some of its limitations comprise inactive metabolic products, poor aqueous solubility, insufficient intrinsic activity, poor absorption, and excessive metabolism. On the other hand, metabolic conversion of quercetin can significantly alter its efficacy and bioavailability. Similar to other xenobiotics, the reactivity of quercetin may be attenuated by conjugation. A number of quercetin-conjugated metabolites have a biological function. Aglycone-conjugated quercetin metabolites are synthesized by the site-specific action of certain enzymes, and several biological targets have been identified. As a result, more detailed studies need to be performed to improve our understanding of quercetin and its active metabolites, and their underlying mechanism of action. Moreover, nanostructured carriers including, liposomes, polymers, chitosan, and other nanoparticles may be effectively used to deliver quercetin and active metabolites to various tissues. These nanocarriers can increase the solubility of polyphenols in water, prolong the circulation time, improve the absorption rate, and the target-specificity. However, although quercetin delivery via these carriers has not been much investigated, it may be a useful approach to improve the activity of polyphenols in different types of cancer, especially in colorectal cancer. Additionally, further investigations are needed to identify any synergistic effects of quercetin when combined with other antitumor drugs. Moreover, clinical trials investigating the preventive and therapeutic activity of quercetin in GI cancers have high priority. More data for future epidemiological studies may be obtained by updating of the dietary flavonoids databases. Finally, although quercetin has shown some promising results in the prevention and treatment GI tumors, further studies are still needed to thoroughly understand the underlying mechanisms and any possible adverse effects.
Author Contributions
HM involved in conception, design, statistical analysis, and drafting of the manuscript. SM, FD, MT, PE, AS, AA, FE, FM and MH contributed in data collection and manuscript drafting.
Conflict of Interest
The authors declare that the research was conducted in the absence of any commercial or financial relationships that could be construed as a potential conflict of interest.
Publisher’s Note
All claims expressed in this article are solely those of the authors and do not necessarily represent those of their affiliated organizations, or those of the publisher, the editors, and the reviewers. Any product that may be evaluated in this article, or claim that may be made by its manufacturer, is not guaranteed or endorsed by the publisher.
References
Abd-Rabou, A. A., and Ahmed, H. H. (2017). CS-PEG Decorated PLGA Nano-Prototype for Delivery of Bioactive Compounds: A Novel Approach for Induction of Apoptosis in HepG2 Cell Line. Adv. Med. Sci. 62 (2), 357–367. doi:10.1016/j.advms.2017.01.003
Abdelmoneem, M. A., Elnaggar, M. A., Hammady, R. S., Kamel, S. M., Helmy, M. W., Abdulkader, M. A., et al. (2019). Dual-Targeted Lactoferrin Shell-Oily Core Nanocapsules for Synergistic Targeted/Herbal Therapy of Hepatocellular Carcinoma. ACS Appl. Mater. Inter. 11 (30), 26731–26744. doi:10.1021/acsami.9b10164
AbouAitah, K., Swiderska-Sroda, A., Farghali, A. A., Wojnarowicz, J., Stefanek, A., Gierlotka, S., et al. (2018). Folic Acid-Conjugated Mesoporous Silica Particles as Nanocarriers of Natural Prodrugs for Cancer Targeting and Antioxidant Action. Oncotarget 9 (41), 26466–26490. doi:10.18632/oncotarget.25470
Ackland, M. L., van de Waarsenburg, S., and Jones, R. (2005). Synergistic Antiproliferative Action of the Flavonols Quercetin and Kaempferol in Cultured Human Cancer Cell Lines. In Vivo 19 (1), 69–76.
Adikrisna, R., Tanaka, S., Muramatsu, S., Aihara, A., Ban, D., Ochiai, T., et al. (2012). Identification of Pancreatic Cancer Stem Cells and Selective Toxicity of Chemotherapeutic Agents. Gastroenterology 143 (1), 234–e7. doi:10.1053/j.gastro.2012.03.054
Aghdassi, A., Phillips, P., Dudeja, V., Dhaulakhandi, D., Sharif, R., Dawra, R., et al. (2007). Heat Shock Protein 70 Increases Tumorigenicity and Inhibits Apoptosis in Pancreatic Adenocarcinoma. Cancer Res. 67 (2), 616–625. doi:10.1158/0008-5472.CAN-06-1567
Agullo, G., Gamet, L., Besson, C., Demigné, C., and Rémésy, C. (1994). Quercetin Exerts a Preferential Cytotoxic Effect on Active Dividing colon Carcinoma HT29 and Caco-2 Cells. Cancer Lett. 87 (1), 55–63. doi:10.1016/0304-3835(94)90409-x
Agullo, G., Gamet-Payrastre, L., Fernandez, Y., Anciaux, N., Demigné, C., and Rémésy, C. (1996). Comparative Effects of Flavonoids on the Growth, Viability and Metabolism of a Colonic Adenocarcinoma Cell Line (HT29 Cells). Cancer Lett. 105 (1), 61–70. doi:10.1016/0304-3835(96)04262-0
Ahmed, H. H., Aglan, H. A., Zaazaa, A. M., Shalby, A. B., and El Toumy, S. A. (2016). Quercetin Confers Tumoricidal Activity through Multipathway Mechanisms in A N-Methylnitrosourea Rat Model of Colon Cancer. Asian Pac. J. Cancer Prev. 17 (11), 4991–4998. doi:10.22034/APJCP.2016.17.11.4991
Al-Ghamdi, M. A., Al-Enazy, A., Huwait, E. A., Albukhari, A., Harakeh, S., and Moselhy, S. S. (2021). Enhancement of Annexin V in Response to Combination of Epigallocatechin Gallate and Quercetin as a Potent Arrest the Cell Cycle of Colorectal Cancer. Braz. J. Biol. 83, e248746. doi:10.1590/1519-6984.248746
Alrawaiq, N., and Abdullah, A. (2014). A Review of Flavonoid Quercetin: Metabolism, Bioactivity and Antioxidant Properties. Int. J. PharmTech Res. 6, 933–941.
Angst, E., Park, J. L., Moro, A., Lu, Q. Y., Lu, X., Li, G., et al. (2013). The Flavonoid Quercetin Inhibits Pancreatic Cancer Growth In Vitro and In Vivo. Pancreas 42 (2), 223–229. doi:10.1097/MPA.0b013e318264ccae
Appari, M., Babu, K. R., Kaczorowski, A., Gross, W., and Herr, I. (2014). Sulforaphane, Quercetin and Catechins Complement Each Other in Elimination of Advanced Pancreatic Cancer by miR-Let-7 Induction and K-Ras Inhibition. Int. J. Oncol. 45 (4), 1391–1400. doi:10.3892/ijo.2014.2539
Arabski, M., Kazmierczak, P., Wiśniewska-Jarosińska, M., Morawiec, Z., Morawiec-Bajda, A., Klupińska, G., et al. (2006). Helicobacter pylori Infection Can Modulate the Susceptibility of Gastric Mucosa Cells to MNNG. Cell Mol Biol Lett 11 (4), 570–578. doi:10.2478/s11658-006-0045-z
Atashpour, S., Fouladdel, S., Movahhed, T. K., Barzegar, E., Ghahremani, M. H., Ostad, S. N., et al. (2015). Quercetin Induces Cell Cycle Arrest and Apoptosis in CD133(+) Cancer Stem Cells of Human Colorectal HT29 Cancer Cell Line and Enhances Anticancer Effects of Doxorubicin. Iran J. Basic Med. Sci. 18 (7), 635–643.
Awad, H. M., Boersma, M. G., Vervoort, J., and Rietjens, I. M. (2000). Peroxidase-catalyzed Formation of Quercetin Quinone Methide-Glutathione Adducts. Arch. Biochem. Biophys. 378 (2), 224–233. doi:10.1006/abbi.2000.1832
Baby, B., Antony, P., and Vijayan, R. (2018). Interactions of Quercetin with Receptor Tyrosine Kinases Associated with Human Lung Carcinoma. Nat. Prod. Res. 32 (24), 2928–2931. doi:10.1080/14786419.2017.1385015
Bahman, A. A., Abaza, M. S. I., Khoushiash, S. I., and Al-Attiyah, R. J. (2018). Sequence-dependent E-ffect of S-orafenib in C-ombination with N-atural P-henolic C-ompounds on H-epatic C-ancer C-ells and the P-ossible M-echanism of A-ction. Int. J. Mol. Med. 42 (3), 1695–1715. doi:10.3892/ijmm.2018.3725
Banerjee, A., Pathak, S., Jothimani, G., and Roy, S. (2020). Antiproliferative Effects of Combinational Therapy of Lycopodium Clavatum and Quercetin in colon Cancer Cells. J. Basic Clin. Physiol. Pharmacol. 31, 31. doi:10.1515/jbcpp-2019-0193
Banikazemi, Z., Mirazimi, S. M., Dashti, F., Mazandaranian, M. R., Akbari, M., Morshedi, K., et al. (2021). Coumarins and Gastrointestinal Cancer: A New Therapeutic Option? Front. Oncol. 11, 752784. doi:10.3389/fonc.2021.752784
Benito, I., Encío, I. J., Milagro, F. I., Alfaro, M., Martínez-Peñuela, A., Barajas, M., et al. (2021). Microencapsulated Bifidobacterium Bifidum and Lactobacillus Gasseri in Combination with Quercetin Inhibit Colorectal Cancer Development in ApcMin/+ Mice. Ijms 22 (9), 4906. doi:10.3390/ijms22094906
Bevara, G. B., Naveen Kumar, A. D., Koteshwaramma, K. L., Badana, A., Kumari, S., and Malla, R. R. (2018). C-glycosyl Flavone from Urginea Indica Inhibits Proliferation & Angiogenesis & Induces Apoptosis via Cyclin-dependent Kinase 6 in Human Breast, Hepatic & colon Cancer Cell Lines. Indian J. Med. Res. 147 (2), 158–168. doi:10.4103/ijmr.IJMR_51_16
Bianchi, M., Canavesi, R., Aprile, S., Grosa, G., and Del Grosso, E. (2018). Troxerutin, a Mixture of O-Hydroxyethyl Derivatives of the Natural Flavonoid Rutin: Chemical Stability and Analytical Aspects. J. Pharm. Biomed. Anal. 150, 248–257. doi:10.1016/j.jpba.2017.12.018
Biswas, S., and Reddy, N. D. (2018). Eval. Novel 3-Hydroxyflavone Analogues as HDAC Inhibitors against Colorectal Cancer 2018, 4751806. doi:10.1155/2018/4751806
Böhm, H. G. (1994). Mazza und E. Miniati: Anthocyanins in Fruits, Vegetables and Grains. 362 Seiten, zahlr. Abb. und Tab. CRC Press, Boca Raton, Ann Arbor, London, Tokyo 1993. Preis: 144.— £. Food/Nahrung 38 (3), 343. doi:10.1002/food.19940380317
Borska, S., Chmielewska, M., Wysocka, T., Drag-Zalesinska, M., Zabel, M., and Dziegiel, P. (2012). In Vitro effect of Quercetin on Human Gastric Carcinoma: Targeting Cancer Cells Death and MDR. Food Chem. Toxicol. 50 (9), 3375–3383. doi:10.1016/j.fct.2012.06.035
Brito, A. F., Ribeiro, M., Abrantes, A. M., Mamede, A. C., Laranjo, M., Casalta-Lopes, J. E., et al. (2016). New Approach for Treatment of Primary Liver Tumors: The Role of Quercetin. Nutr. Cancer 68 (2), 250–266. doi:10.1080/01635581.2016.1145245
Brito, A. F., Ribeiro, M., Abrantes, A. M., Pires, A. S., Teixo, R. J., Tralhão, J. G., et al. (2015). Quercetin in Cancer Treatment, Alone or in Combination with Conventional Therapeutics? Curr. Med. Chem. 22 (26), 3025–3039. doi:10.2174/0929867322666150812145435
Browning, A. M., Walle, U. K., and Walle, T. (2005). Flavonoid Glycosides Inhibit Oral Cancer Cell Proliferation-Rrole of Cellular Uptake and Hydrolysis to the Aglycones. J. Pharm. Pharmacol. 57 (8), 1037–1042. doi:10.1211/0022357056514
Bulzomi, P., Galluzzo, P., Bolli, A., Leone, S., Acconcia, F., and Marino, M. (2012). The Pro-apoptotic Effect of Quercetin in Cancer Cell Lines Requires ERβ-dependent Signals. J. Cel Physiol 227 (5), 1891–1898. doi:10.1002/jcp.22917
Cao, C., Sun, L., Mo, W., Sun, L., Luo, J., Yang, Z., et al. (2015). Quercetin Mediates β-Catenin in Pancreatic Cancer Stem-like Cells. Pancreas 44 (8), 1334–1339. doi:10.1097/MPA.0000000000000400
Carrasco-Torres, G., Baltierrez-Hoyos, R., Andrade-Jorge, E., Villa-Trevino, S., Cytotoxicity, Trujillo-Ferrara. J. G., Stress, Oxidative., et al. (2017). after Combined Treat. Hepatocarcinoma Cell Maleic Anhydride Derivatives Quercetin 2017, 2734976. doi:10.1155/2017/2734976
Chamberlin, S. R., Blucher, A., Wu, G., Shinto, L., Choonoo, G., Kulesz-Martin, M., et al. (2019). Natural Product Target Network Reveals Potential for Cancer Combination Therapies. Front. Pharmacol. 10, 557. doi:10.3389/fphar.2019.00557
Chang, Y. F., Chi, C. W., and Wang, J. J. (2006). Reactive Oxygen Species Production Is Involved in Quercetin-Induced Apoptosis in Human Hepatoma Cells. Nutr. Cancer 55 (2), 201–209. doi:10.1207/s15327914nc5502_12
Chang, Y. F., Hsu, Y. C., Hung, H. F., Lee, H. J., Lui, W. Y., Chi, C. W., et al. (2009). Quercetin Induces Oxidative Stress and Potentiates the Apoptotic Action of 2-methoxyestradiol in Human Hepatoma Cells. Nutr. Cancer 61 (5), 735–745. doi:10.1080/01635580902825571
Chen, B., Yang, Z., Zhu, Y., and Xia, Y. (2014). Zeolitic Imidazolate Framework Materials: Recent Progress in Synthesis and Applications. J. Mater. Chem. A. 2 (40), 16811–16831. doi:10.1039/c4ta02984d
Chen, S. F., Nieh, S., Jao, S. W., Liu, C. L., Wu, C. H., Chang, Y. C., et al. (2012). Quercetin Suppresses Drug-Resistant Spheres via the P38 MAPK-Hsp27 Apoptotic Pathway in Oral Cancer Cells. PloS one 7 (11), e49275. doi:10.1371/journal.pone.0049275
Chen, S. F., Nien, S., Wu, C. H., Liu, C. L., Chang, Y. C., and Lin, Y. S. (2013). Reappraisal of the Anticancer Efficacy of Quercetin in Oral Cancer Cells. J. Chin. Med. Assoc. 76 (3), 146–152. doi:10.1016/j.jcma.2012.11.008
Chen, Z., Huang, C., Ma, T., Jiang, L., Tang, L., Shi, T., et al. (2018). Reversal Effect of Quercetin on Multidrug Resistance via FZD7/β-Catenin Pathway in Hepatocellular Carcinoma Cells. Phytomedicine 43, 37–45. doi:10.1016/j.phymed.2018.03.040
Cheng, M. R., Li, Q., Wan, T., He, B., Han, J., Chen, H. X., et al. (2012). Galactosylated Chitosan/5-Fluorouracil Nanoparticles Inhibit Mouse Hepatic Cancer Growth and its Side Effects. World J. Gastroenterol. 18 (42), 6076–6087. doi:10.3748/wjg.v18.i42.6076
Cheong, E., Ivory, K., Doleman, J., Parker, M. L., Rhodes, M., and Johnson, I. T. (2004). Synthetic and Naturally Occurring COX-2 Inhibitors Suppress Proliferation in a Human Oesophageal Adenocarcinoma Cell Line (OE33) by Inducing Apoptosis and Cell Cycle Arrest. Carcinogenesis 25 (10), 1945–1952. doi:10.1093/carcin/bgh184
Chuang-Xin, L., Wen-Yu, W., Yao, C., Xiao-Yan, L., and Yun, Z. (2012). Quercetin Enhances the Effects of 5-Fluorouracil-Mediated Growth Inhibition and Apoptosis of Esophageal Cancer Cells by Inhibiting NF-Κb. Oncol. Lett. 4 (4), 775–778. doi:10.3892/ol.2012.829
Chuang-Xin, L., Wen-Yu, W., Yao, C., Xiao-Yan, L., and Yun, Z. (2012). Quercetin Enhances the Effects of 5-Fluorouracil-Mediated Growth Inhibition and Apoptosis of Esophageal Cancer Cells by Inhibiting NF-Κb. Oncol. Lett. 4 (4), 775–778. doi:10.3892/ol.2012.829
C. Maiyo, F., Moodley, R., SinghAntioxidant, M., and Studies, A. (2016). Cytotoxicity, Antioxidant and Apoptosis Studies of Quercetin-3-O Glucoside and 4-(?-D-Glucopyranosyl-1?4-?-L-Rhamnopyranosyloxy)-Benzyl Isothiocyanate from Moringa Oleifera. Acamc 16 (5), 648–656. doi:10.2174/1871520615666151002110424
Colli, L. M., Machiela, M. J., Zhang, H., Myers, T. A., Jessop, L., Delattre, O., et al. (2017). Landscape of Combination Immunotherapy and Targeted Therapy to Improve Cancer Management. Cancer Res. 77 (13), 3666–3671. doi:10.1158/0008-5472.CAN-16-3338
D'Andrea, G. (2015). Quercetin: A Flavonol with Multifaceted Therapeutic Applications? Fitoterapia 106, 256–271. doi:10.1016/j.fitote.2015.09.018
Dai, W., Gao, Q., Qiu, J., Yuan, J., Wu, G., and Shen, G. (2016). Quercetin Induces Apoptosis and Enhances 5-FU Therapeutic Efficacy in Hepatocellular Carcinoma. Tumour Biol. 37 (5), 6307–6313. doi:10.1007/s13277-015-4501-0
David, K. I., Jaidev, L. R., Sethuraman, S., and Krishnan, U. M. (2015). Dual Drug Loaded Chitosan Nanoparticles-Sugar-Ccoated Arsenal against Pancreatic Cancer. Colloids Surf. B Biointerfaces 135, 689–698. doi:10.1016/j.colsurfb.2015.08.038
Davoodvandi, A., Farshadi, M., Zare, N., Akhlagh, S. A., Alipour Nosrani, E., Mahjoubin-Tehran, M., et al. (2021). Antimetastatic Effects of Curcumin in Oral and Gastrointestinal Cancers. Front. Pharmacol. 12, 668567. doi:10.3389/fphar.2021.668567
Dihal, A. A., de Boer, V. C., van der Woude, H., Tilburgs, C., Bruijntjes, J. P., Alink, G. M., et al. (2006). Quercetin, but Not its Glycosidated Conjugate Rutin, Inhibits Azoxymethane-Induced Colorectal Carcinogenesis in F344 Rats. J. Nutr. 136 (11), 2862–2867. doi:10.1093/jn/136.11.2862
Ding, M., Pan, A., Manson, J. E., Willett, W. C., Malik, V., Rosner, B., et al. (2016). Consumption of Soy Foods and Isoflavones and Risk of Type 2 Diabetes: a Pooled Analysis of Three US Cohorts. Eur. J. Clin. Nutr. 70 (12), 1381–1387. doi:10.1038/ejcn.2016.117
Ding, Y., Chen, X., Wang, B., Yu, B., Ge, J., and Shi, X. (2018). Quercetin Suppresses the Chymotrypsin-like Activity of Proteasome via Inhibition of MEK1/ERK1/2 Signaling Pathway in Hepatocellular Carcinoma HepG2 Cells. Can. J. Physiol. Pharmacol. 96 (5), 521–526. doi:10.1139/cjpp-2017-0655
Du, F., Feng, Y., Fang, J., and Yang, M. (2015). MicroRNA-143 Enhances Chemosensitivity of Quercetin through Autophagy Inhibition via Target GABARAPL1 in Gastric Cancer Cells. Biomed. Pharmacother. 74, 169–177. doi:10.1016/j.biopha.2015.08.005
Dudeja, V., Mujumdar, N., Phillips, P., Chugh, R., Borja-Cacho, D., Dawra, R. K., et al. (2009). Heat Shock Protein 70 Inhibits Apoptosis in Cancer Cells through Simultaneous and Independent Mechanisms. Gastroenterology 136 (5), 1772–1782. doi:10.1053/j.gastro.2009.01.070
El-Din, M. S., Taha, A. M., Sayed, A. A., and Salem, A. M. (2019). Ziziphus Spina-Christi Leaves Methanolic Extract Alleviates Diethylnitrosamine-Induced Hepatocellular Carcinoma in Rats. Biochem. Cel Biol 97 (4), 437–445. doi:10.1139/bcb-2018-0318
El-Miligy, M. M. M., Al-Kubeisi, A. K., El-Zemity, S. R., Nassra, R. A., Abu-Serie, M. M., and Hazzaa, A. A. (2021). Discovery of Small Molecule Acting as Multitarget Inhibitor of Colorectal Cancer by Simultaneous Blocking of the Key COX-2, 5-LOX and PIM-1 Kinase Enzymes. Bioorg. Chem. 115, 105171. doi:10.1016/j.bioorg.2021.105171
Erdoğan, M. K., Ağca, C. A., Quercetin, A. H., and Improve, L. (2021). “The Anticancer Effects of 5-Fluorouracil in Human Colorectal Adenocarcinoma,” in A Mechanistic Insight. Editor V. Model, 1–17.
Fan, P., Zhang, Y., Liu, L., Zhao, Z., Yin, Y., Xiao, X., et al. (2016). Continuous Exposure of Pancreatic Cancer Cells to Dietary Bioactive Agents Does Not Induce Drug Resistance unlike Chemotherapy. Cell Death Dis 7 (6), e2246. doi:10.1038/cddis.2016.157
Firenzuoli, F., Gori, L., Crupi, A., and Neri, D. (2004). Flavonoids: Risks or Therapeutic Opportunities? Recenti Prog. Med. 95 (7-8), 345–351.
Gao, X., Wang, B., Wei, X., Men, K., Zheng, F., Zhou, Y., et al. (2012). Anticancer Effect and Mechanism of Polymer Micelle-Encapsulated Quercetin on Ovarian Cancer. Nanoscale 4 (22), 7021–7030. doi:10.1039/c2nr32181e
Gao, Z., Liu, X., Deng, G., Zhou, F., Zhang, L., Wang, Q., et al. (2016). Fe3O4@mSiO2-FA-CuS-PEG Nanocomposites for Magnetic Resonance Imaging and Targeted Chemo-Photothermal Synergistic Therapy of Cancer Cells. Dalton Trans. 45 (34), 13456–13465. doi:10.1039/c6dt01714b
Gee, J. M., Hara, H., and Johnson, I. T. (2002). Suppression of Intestinal Crypt Cell Proliferation and Aberrant Crypt Foci by Dietary Quercetin in Rats. Nutr. Cancer 43 (2), 193–201. doi:10.1207/S15327914NC432_10
Ghosh, A., Ghosh, D., Sarkar, S., Mandal, A. K., Thakur Choudhury, S., and Das, N. (2012). Anticarcinogenic Activity of Nanoencapsulated Quercetin in Combating Diethylnitrosamine-Induced Hepatocarcinoma in Rats. Eur. J. Cancer Prev. 21 (1), 32–41. doi:10.1097/CEJ.0b013e32834a7e2b
Gibellini, L., Pinti, M., Nasi, M., Montagna, J. P., De Biasi, S., Roat, E., et al. (2011). Quercetin and Cancer Chemoprevention. Evid. Based Complement. Alternat Med. 2011, 591356. doi:10.1093/ecam/neq053
Gou, M., Men, K., Shi, H., Xiang, M., Zhang, J., Song, J., et al. (2011). Curcumin-loaded Biodegradable Polymeric Micelles for colon Cancer Therapy In Vitro and In Vivo. Nanoscale 3 (4), 1558–1567. doi:10.1039/c0nr00758g
Gu, L., Kelm, M. A., Hammerstone, J. F., Beecher, G., Holden, J., Haytowitz, D., et al. (2004). Concentrations of Proanthocyanidins in Common Foods and Estimations of normal Consumption. J. Nutr. 134 (3), 613–617. doi:10.1093/jn/134.3.613
Gulati, N., Laudet, B., Zohrabian, V. M., Murali, R., and Jhanwar-Uniyal, M. (2006). The Antiproliferative Effect of Quercetin in Cancer Cells Is Mediated via Inhibition of the PI3K-Akt/PKB Pathway. Anticancer Res. 26 (2A), 1177–1181.
Guo, D., Wu, C., Li, J., Guo, A., Li, Q., Jiang, H., et al. (2009). Synergistic Effect of Functionalized Nickel Nanoparticles and Quercetin on Inhibition of the SMMC-7721 Cells Proliferation. Nanoscale Res. Lett. 4 (12), 1395–1402. doi:10.1007/s11671-009-9411-x
Guo, X. F., Liu, J. P., Ma, S. Q., Zhang, P., and Sun, W. D. (2018). Avicularin Reversed Multidrug-Resistance in Human Gastric Cancer through Enhancing Bax and BOK Expressions. Biomed. Pharmacother. 103, 67–74. doi:10.1016/j.biopha.2018.03.110
Guo, Y., Tong, Y., Zhu, H., Xiao, Y., Guo, H., Shang, L., et al. (2021). Quercetin Suppresses Pancreatic Ductal Adenocarcinoma Progression via Inhibition of SHH and TGF-β/Smad Signaling Pathways. Cell Biol Toxicol 37 (3), 479–496. doi:10.1007/s10565-020-09562-0
Guo, Y., Tong, Y., Zhu, H., Xiao, Y., Guo, H., Shang, L., et al. (2021). Quercetin Suppresses Pancreatic Ductal Adenocarcinoma Progression via Inhibition of SHH and TGF-β/Smad Signaling Pathways. Cel Biol Toxicol 37 (3), 479–496. doi:10.1007/s10565-020-09562-0
Gupta, K., and Panda, D. (2002). Perturbation of Microtubule Polymerization by Quercetin through Tubulin Binding: a Novel Mechanism of its Antiproliferative Activity. Biochemistry 41 (43), 13029–13038. doi:10.1021/bi025952r
Haghiac, M., and Walle, T. (2005). Quercetin Induces Necrosis and Apoptosis in SCC-9 Oral Cancer Cells. Nutr. Cancer 53 (2), 220–231. doi:10.1207/s15327914nc5302_11
Han, M., Song, Y., and Zhang, X. (2016). Quercetin Suppresses the Migration and Invasion in Human Colon Cancer Caco-2 Cells through Regulating Toll-like Receptor 4/Nuclear Factor-Kappa B Pathway. Pharmacogn Mag. 12 (Suppl. 2), S237–S244. doi:10.4103/0973-1296.182154
Hariharan, S., Bhardwaj, V., Bala, I., Sitterberg, J., Bakowsky, U., and Ravi Kumar, M. N. (2006). Design of Estradiol Loaded PLGA Nanoparticulate Formulations: a Potential Oral Delivery System for Hormone Therapy. Pharm. Res. 23 (1), 184–195. doi:10.1007/s11095-005-8418-y
Harris, D. M., Li, L., Chen, M., Lagunero, F. T., Go, V. L., and Boros, L. G. (2012). Diverse Mechanisms of Growth Inhibition by Luteolin, Resveratrol, and Quercetin in MIA PaCa-2 Cells: a Comparative Glucose Tracer Study with the Fatty Acid Synthase Inhibitor C75. Metabolomics 8 (2), 201–210. doi:10.1007/s11306-011-0300-9
Hassan, S., Peluso, J., Chalhoub, S., Idoux Gillet, Y., Benkirane-Jessel, N., Rochel, N., et al. (2020). Quercetin Potentializes the Respective Cytotoxic Activity of Gemcitabine or Doxorubicin on 3D Culture of AsPC-1 or HepG2 Cells, through the Inhibition of HIF-1α and MDR1. PLoS One 15, e0240676. doi:10.1371/journal.pone.0240676
Hatahet, T., Morille, M., Shamseddin, A., Aubert-Pouëssel, A., Devoisselle, J. M., and Bégu, S. (2017). Dermal Quercetin Lipid Nanocapsules: Influence of the Formulation on Antioxidant Activity and Cellular protection against Hydrogen Peroxide. Int. J. Pharm. 518 (1-2), 167–176. doi:10.1016/j.ijpharm.2016.12.043
Hemati, M., Haghiralsadat, F., Jafary, F., Moosavizadeh, S., and Moradi, A. (2019). Targeting Cell Cycle Protein in Gastric Cancer with CDC20siRNA and Anticancer Drugs (Doxorubicin and Quercetin) Co-loaded Cationic PEGylated Nanoniosomes. Int. J. Nanomedicine 14, 6575–6585. doi:10.2147/IJN.S211844
Hisaka, T., Sakai, H., Sato, T., Goto, Y., Nomura, Y., Fukutomi, S., et al. (2020). Quercetin Suppresses Proliferation of Liver Cancer Cell Lines In Vitro. Anticancer Res. 40 (8), 4695–4700. doi:10.21873/anticanres.14469
Hoca, M., Becer, E., Kabadayi, H., Yucecan, S., and Vatansever, H. S. (2019). The Effect of Resveratrol and Quercetin on Epithelial-Mesenchymal Transition in Pancreatic Cancer Stem Cell. Nutr. Cancer, 1–12. doi:10.1080/01635581.2019.1670853
Hoca, M., Becer, E., Kabadayı, H., Yücecan, S., and Vatansever, H. S. (2020). The Effect of Resveratrol and Quercetin on Epithelial-Mesenchymal Transition in Pancreatic Cancer Stem Cell. Nutr. Cancer 72 (7), 1231–1242. doi:10.1080/01635581.2019.1670853
Hoca, M., Becer, E., Kabadayı, H., Yücecan, S., and Vatansever, H. S. (2020). The Effect of Resveratrol and Quercetin on Epithelial-Mesenchymal Transition in Pancreatic Cancer Stem Cell. Nutr. Cancer 72 (7), 1231–1242. doi:10.1080/01635581.2019.1670853
Honari, M., Shafabakhsh, R., Reiter, R. J., Mirzaei, H., and Asemi, Z. (2019). Resveratrol Is a Promising Agent for Colorectal Cancer Prevention and Treatment: Focus on Molecular Mechanisms. Cancer Cel Int 19, 180. doi:10.1186/s12935-019-0906-y
Horcajada, P., Serre, C., Maurin, G., Ramsahye, N. A., Balas, F., Vallet-Regí, M., et al. (2008). Flexible Porous Metal-Organic Frameworks for a Controlled Drug Delivery. J. Am. Chem. Soc. 130 (21), 6774–6780. doi:10.1021/ja710973k
Hoseini, A., Namazi, G., Farrokhian, A., Reiner, Ž., Aghadavod, E., Bahmani, F., et al. (2019). The Effects of Resveratrol on Metabolic Status in Patients with Type 2 Diabetes Mellitus and Coronary Heart Disease. Food Funct. 10 (9), 6042–6051. doi:10.1039/c9fo01075k
Hosokawa, N., Hosokawa, Y., Sakai, T., Yoshida, M., Marui, N., Nishino, H., et al. (1990). Inhibitory Effect of Quercetin on the Synthesis of a Possibly Cell-Cycle-Related 17-kDa Protein, in Human colon Cancer Cells. Int. J. Cancer 45 (6), 1119–1124. doi:10.1002/ijc.2910450622
Huang, C. Y., Chan, C. Y., Chou, I. T., Lien, C. H., Hung, H. C., and Lee, M. F. (2013). Quercetin Induces Growth Arrest through Activation of FOXO1 Transcription Factor in EGFR-Overexpressing Oral Cancer Cells. J. Nutr. Biochem. 24 (9), 1596–1603. doi:10.1016/j.jnutbio.2013.01.010
Huang, D. Y., Dai, Z. R., Li, W. M., Wang, R. G., and Yang, S. M. (2018). Inhibition of EGF Expression and NF-Κb Activity by Treatment with Quercetin Leads to Suppression of Angiogenesis in Nasopharyngeal Carcinoma. Saudi J. Biol. Sci. 25 (4), 826–831. doi:10.1016/j.sjbs.2016.11.011
Hussain, S. P., Amstad, P., He, P., Robles, A., Lupold, S., Kaneko, I., et al. (2004). p53-induced Up-Regulation of MnSOD and GPx but Not Catalase Increases Oxidative Stress and Apoptosis. Cancer Res. 64 (7), 2350–2356. doi:10.1158/0008-5472.can-2287-2
Hyun, J. J., Lee, H. S., Keum, B., Seo, Y. S., Jeen, Y. T., Chun, H. J., et al. (2013). Expression of Heat Shock Protein 70 Modulates the Chemoresponsiveness of Pancreatic Cancer. Gut Liver 7 (6), 739–746. doi:10.5009/gnl.2013.7.6.739
Igbe, I., Shen, X. F., Jiao, W., Qiang, Z., Deng, T., Li, S., et al. (2017). Dietary Quercetin Potentiates the Antiproliferative Effect of Interferon-α in Hepatocellular Carcinoma Cells through Activation of JAK/STAT Pathway Signaling by Inhibition of SHP2 Phosphatase. Oncotarget 8 (69), 113734–113748. doi:10.18632/oncotarget.22556
Isa, N. M., Abdelwahab, S. I., Mohan, S., Abdul, A. B., Sukari, M. A., Taha, M. M., et al. (2012). In Vitro anti-inflammatory, Cytotoxic and Antioxidant Activities of Boesenbergin A, a Chalcone Isolated from Boesenbergia Rotunda (L.) (Fingerroot). Braz. J. Med. Biol. Res. 45 (6), 524–530. doi:10.1590/s0100-879x2012007500022
Jáf, Vara. (2004). Casado E, de Castro J, Cejas P, Belda-Iniesta C, González-Barón M. PI3K/Akt signalling pathway and cancer. Cancer Treat. Rev. 30 (2), 193–204.
Jeon, J. S., Kwon, S., Ban, K., Kwon Hong, Y., Ahn, C., Sung, J. S., et al. (2019). Regulation of the Intracellular ROS Level Is Critical for the Antiproliferative Effect of Quercetin in the Hepatocellular Carcinoma Cell Line HepG2. Nutr. Cancer 71 (5), 861–869. doi:10.1080/01635581.2018.1559929
Ji, Y., Li, L., Ma, Y. X., Li, W. T., Li, L., Zhu, H. Z., et al. (2019). Quercetin Inhibits Growth of Hepatocellular Carcinoma by Apoptosis Induction in Part via Autophagy Stimulation in Mice. J. Nutr. Biochem. 69, 108–119. doi:10.1016/j.jnutbio.2019.03.018
Jiang, W., Zhang, H., Wu, J., Zhai, G., Li, Z., Luan, Y., et al. (2018). CuS@MOF-Based Well-Designed Quercetin Delivery System for Chemo-Photothermal Therapy. ACS Appl. Mater. Inter. 10 (40), 34513–34523. doi:10.1021/acsami.8b13487
Jin, W., Han, H., Ma, L., Zhou, H., and Zhao, C. (2016). The Chemosensitization Effect of Quercetin on Cisplatin Induces the Apoptosis of Human colon Cancer Ht-29 Cell Line. Int. J. Clin. Exp. Med. 9, 2285–2292.
Johnson, J. L., Rupasinghe, S. G., Stefani, F., Schuler, M. A., and Gonzalez de Mejia, E. (2011). Citrus Flavonoids Luteolin, Apigenin, and Quercetin Inhibit Glycogen Synthase Kinase-3β Enzymatic Activity by Lowering the Interaction Energy within the Binding Cavity. J. Med. Food 14 (4), 325–333. doi:10.1089/jmf.2010.0310
Jung, K. H., Rumman, M., Yan, H., Cheon, M. J., Choi, J. G., Jin, X., et al. (2018). An Ethyl Acetate Fraction of Artemisia Capillaris (ACE-63) Induced Apoptosis and Anti-angiogenesis via Inhibition of PI3K/AKT Signaling in Hepatocellular Carcinoma. Phytother Res. 32 (10), 2034–2046. doi:10.1002/ptr.6135
Kang, J. W., Kim, J. H., Song, K., Kim, S. H., Yoon, J. H., and Kim, K. S. (2010). Kaempferol and Quercetin, Components of Ginkgo Biloba Extract (EGb 761), Induce Caspase-3-dependent Apoptosis in Oral Cavity Cancer Cells. Phytother ResSuppl 24 Suppl 1, S77–S82. doi:10.1002/ptr.2913
Kim, G. T., Lee, S. H., Kim, J. I., and Kim, Y. M. (2014). Quercetin Regulates the Sestrin 2-AMPK-P38 MAPK Signaling Pathway and Induces Apoptosis by Increasing the Generation of Intracellular ROS in a P53-independent Manner. Int. J. Mol. Med. 33 (4), 863–869. doi:10.3892/ijmm.2014.1658
Kim, G. T., Lee, S. H., and Kim, Y. M. (2013). Quercetin Regulates Sestrin 2-AMPK-mTOR Signaling Pathway and Induces Apoptosis via Increased Intracellular ROS in HCT116 Colon Cancer Cells. J. Cancer Prev. 18 (3), 264–270. doi:10.15430/jcp.2013.18.3.264
Kim, H. J., Kim, S. K., Kim, B. S., Lee, S. H., Park, Y. S., Park, B. K., et al. (2010). Apoptotic Effect of Quercetin on HT-29 colon Cancer Cells via the AMPK Signaling Pathway. J. Agric. Food Chem. 58 (15), 8643–8650. doi:10.1021/jf101510z
Kim, H. S., Wannatung, T., Lee, S., Yang, W. K., Chung, S. H., Lim, J. S., et al. (2012). Quercetin Enhances Hypoxia-Mediated Apoptosis via Direct Inhibition of AMPK Activity in HCT116 colon Cancer. Apoptosis 17 (9), 938–949. doi:10.1007/s10495-012-0719-0
Kim, J. H., Kim, M. J., Choi, K. C., and Son, J. (2016). Quercetin Sensitizes Pancreatic Cancer Cells to TRAIL-Induced Apoptosis through JNK-Mediated cFLIP Turnover. Int. J. Biochem. Cel Biol. 78, 327–334. doi:10.1016/j.biocel.2016.07.033
Kim, J. H., Nam, G. S., Kim, S. H., Ryu, D. S., and Lee, D. S. (2019). Orostachys Japonicus Exerts Antipancreatic Cancer Activity through Induction of Apoptosis and Cell Cycle Arrest in PANC-1 Cells. Food Sci. Nutr. 7 (11), 3549–3559. doi:10.1002/fsn3.1207
Kim, J. Y., Kim, E. H., Park, S. S., Lim, J. H., Kwon, T. K., and Choi, K. S. (2008). Quercetin Sensitizes Human Hepatoma Cells to TRAIL-Induced Apoptosis via Sp1-Mediated DR5 Up-Regulation and Proteasome-Mediated C-FLIPS Down-Regulation. J. Cel Biochem 105 (6), 1386–1398. doi:10.1002/jcb.21958
Kim, M. C., Lee, H. J., Lim, B., Ha, K. T., Kim, S. Y., So, I., et al. (2014). Quercetin Induces Apoptosis by Inhibiting MAPKs and TRPM7 Channels in AGS Cells. Int. J. Mol. Med. 33 (6), 1657–1663. doi:10.3892/ijmm.2014.1704
Kim, W. K., Bang, M. H., Kim, E. S., Kang, N. E., Jung, K. C., Cho, H. J., et al. (2005). Quercetin Decreases the Expression of ErbB2 and ErbB3 Proteins in HT-29 Human colon Cancer Cells. J. Nutr. Biochem. 16 (3), 155–162. doi:10.1016/j.jnutbio.2004.10.010
Kovacovicova, K., Skolnaja, M., Heinmaa, M., Mistrik, M., Pata, P., Pata, I., et al. (2018). Senolytic Cocktail Dasatinib+Quercetin (D+Q) Does Not Enhance the Efficacy of Senescence-Inducing Chemotherapy in Liver Cancer. Front. Oncol. 8, 459. doi:10.3389/fonc.2018.00459
Kozics, K., Valovicova, Z., and Slamenova, D. (2011). Structure of Flavonoids Influences the Degree Inhibition of Benzo(a)pyrene - Induced DNA Damage and Micronuclei in HepG2 Cells. Neoplasma 58 (6), 516–524. doi:10.4149/neo_2011_06_516
Kozłowska, A., and Szostak-Wegierek, D. (2014). Flavonoids--food Sources and Health Benefits. Rocz Panstw Zakl Hig 65 (2), 79–85.
Kuntz, S., Wenzel, U., and Daniel, H. (1999). Comparative Analysis of the Effects of Flavonoids on Proliferation, Cytotoxicity, and Apoptosis in Human colon Cancer Cell Lines. Eur. J. Nutr. 38 (3), 133–142. doi:10.1007/s003940050054
Kuo, S. M. (1996). Antiproliferative Potency of Structurally Distinct Dietary Flavonoids on Human colon Cancer Cells. Cancer Lett. 110 (1-2), 41–48. doi:10.1016/s0304-3835(96)04458-8
Lai, W. W., Hsu, S. C., Chueh, F. S., Chen, Y. Y., Yang, J. S., Lin, J. P., et al. (2013). Quercetin Inhibits Migration and Invasion of SAS Human Oral Cancer Cells through Inhibition of NF-Κb and Matrix Metalloproteinase-2/-9 Signaling Pathways. Anticancer Res. 33 (5), 1941–1950.
Lan, C. Y., Chen, S. Y., Kuo, C. W., Lu, C. C., and Yen, G. C. (2019). Quercetin Facilitates Cell Death and Chemosensitivity through RAGE/PI3K/AKT/mTOR axis in Human Pancreatic Cancer Cells. J. Food Drug Anal. 27 (4), 887–896. doi:10.1016/j.jfda.2019.07.001
Lee, J., Han, S. I., Yun, J. H., and Kim, J. H. (2015). Quercetin 3-O-Glucoside Suppresses Epidermal Growth Factor-Induced Migration by Inhibiting EGFR Signaling in Pancreatic Cancer Cells. Tumour Biol. 36 (12), 9385–9393. doi:10.1007/s13277-015-3682-x
Lee, J., Han, S. I., Yun, J. H., and Kim, J. H. (2015). Quercetin 3-O-Glucoside Suppresses Epidermal Growth Factor-Induced Migration by Inhibiting EGFR Signaling in Pancreatic Cancer Cells. Tumour Biol. 36 (12), 9385–9393. doi:10.1007/s13277-015-3682-x
Lee, J. H., Lee, H. B., Jung, G. O., Oh, J. T., Park, D. E., and Chae, K. M. (2013). Effect of Quercetin on Apoptosis of PANC-1 Cells. J. Korean Surg. Soc. 85 (6), 249–260. doi:10.4174/jkss.2013.85.6.249
Lee, R. H., Cho, J. H., Jeon, Y. J., Bang, W., Cho, J. J., Choi, N. J., et al. (2015). Quercetin Induces Antiproliferative Activity against Human Hepatocellular Carcinoma (HepG2) Cells by Suppressing Specificity Protein 1 (Sp1). Drug Dev. Res. 76 (1), 9–16. doi:10.1002/ddr.21235
Lee, S. J., Jung, Y. S., Lee, S. H., Chung, H. Y., and Park, B. J. (2009). Isolation of a Chemical Inhibitor against K-Ras-Induced P53 Suppression through Natural Compound Screening. Int. J. Oncol. 34 (6), 1637–1643. doi:10.3892/ijo_00000294
Lee, Y. K., Hwang, J. T., Kwon, D. Y., Surh, Y. J., and Park, O. J. (2010). Induction of Apoptosis by Quercetin Is Mediated through AMPKalpha1/ASK1/p38 Pathway. Cancer Lett. 292 (2), 228–236. doi:10.1016/j.canlet.2009.12.005
Lei, C. S., Hou, Y. C., Pai, M. H., Lin, M. T., and Yeh, S. L. (2018). Effects of Quercetin Combined with Anticancer Drugs on Metastasis-Associated Factors of Gastric Cancer Cells: In Vitro and In Vivo Studies. J. Nutr. Biochem. 51, 105–113. doi:10.1016/j.jnutbio.2017.09.011
Li, H., and Chen, C. (2018). Quercetin Has Antimetastatic Effects on Gastric Cancer Cells via the Interruption of uPA/uPAR Function by Modulating NF-Κb, PKC-δ, ERK1/2, and AMPKα. Integr. Cancer Ther. 17 (2), 511–523. doi:10.1177/1534735417696702
Li, H., and Chen, C. (2018). Quercetin Has Antimetastatic Effects on Gastric Cancer Cells via the Interruption of uPA/uPAR Function by Modulating NF-Κb, PKC-δ, ERK1/2, and AMPKα. Integr. Cancer Ther. 17 (2), 511–523. doi:10.1177/1534735417696702
Li, X., Zhou, N., Wang, J., Liu, Z., Wang, X., Zhang, Q., et al. (2018). Quercetin Suppresses Breast Cancer Stem Cells (CD44+/CD24-) by Inhibiting the PI3K/Akt/mTOR-Signaling Pathway. Life Sci. 196, 56–62. doi:10.1016/j.lfs.2018.01.014
Li, X., Shan, J., Zhang, W., Su, S., Yuwen, L., and Wang, L. (2017). Recent Advances in Synthesis and Biomedical Applications of Two-Dimensional Transition Metal Dichalcogenide Nanosheets. Small 13 (5), 1602660. doi:10.1002/smll.201602660
Li, Y., Wang, Z., Jin, J., Zhu, S. X., He, G. Q., Li, S. H., et al. (2020). Quercetin Pretreatment Enhances the Radiosensitivity of colon Cancer Cells by Targeting Notch-1 Pathway. Biochem. Biophys. Res. Commun. 523 (4), 947–953. doi:10.1016/j.bbrc.2020.01.048
Li, Y., Yao, J., Han, C., Yang, J., Chaudhry, M. T., Wang, S., et al. (2016). Quercetin, Inflammation and Immunity. Nutrients 8 (3), 167. doi:10.3390/nu8030167
Liang, C., Xu, L., Song, G., and Liu, Z. (2016). Emerging Nanomedicine Approaches Fighting Tumor Metastasis: Animal Models, Metastasis-Targeted Drug Delivery, Phototherapy, and Immunotherapy. Chem. Soc. Rev. 45 (22), 6250–6269. doi:10.1039/c6cs00458j
Lim, J. H., Park, J. W., Min, D. S., Chang, J. S., Lee, Y. H., Park, Y. B., et al. (2007). NAG-1 Up-Regulation Mediated by EGR-1 and P53 Is Critical for Quercetin-Induced Apoptosis in HCT116 colon Carcinoma Cells. Apoptosis 12 (2), 411–421. doi:10.1007/s10495-006-0576-9
Linsalata, M., Orlando, A., Messa, C., Refolo, M. G., and Russo, F. (2010). Quercetin Inhibits Human DLD-1 colon Cancer Cell Growth and Polyamine Biosynthesis. Anticancer Res. 30 (9), 3501–3507.
Liu, W., and Zhi, A. (2021). The Potential of Quercetin to Protect against Loperamide-Induced Constipation in Rats. Food Sci. Nutr. 9 (6), 3297–3307. doi:10.1002/fsn3.2296
Liu, X., Deng, G., Wang, Q., Zhang, L., Wang, Q., and Lu, J.Multifunctional PS@ CS@ Au–Fe₃O₄–FA Nanocomposites for CT, MR and Fluorescence Imaging Guided Targeted-Photothermal Therapy of Cancer Cells. Journal of Materials Chemistry B. 2017.
Liu, Y., Gong, W., Yang, Z. Y., Zhou, X. S., Gong, C., Zhang, T. R., et al. (2017). Quercetin Induces Protective Autophagy and Apoptosis through ER Stress via the P-STAT3/Bcl-2 axis in Ovarian Cancer. Apoptosis 22 (4), 544–557. doi:10.1007/s10495-016-1334-2
Liu, Y., Li, C.-L., Xu, Q.-Q., Cheng, D., Liu, K.-D., and Sun, Z.-Q. (2021). Quercetin Inhibits Invasion and Angiogenesis of Esophageal Cancer Cells. Pathol. - Res. Pract. 222, 153455. doi:10.1016/j.prp.2021.153455
Liu, Z. J., Xu, W., Han, J., Liu, Q. Y., Gao, L. F., Wang, X. H., et al. (2020). Quercetin Induces Apoptosis and Enhances Gemcitabine Therapeutic Efficacy against Gemcitabine-Resistant Cancer Cells. Anticancer Drugs 31 (7), 684–692. doi:10.1097/CAD.0000000000000933
Lugli, E., Ferraresi, R., Roat, E., Troiano, L., Pinti, M., Nasi, M., et al. (2009). Quercetin Inhibits Lymphocyte Activation and Proliferation without Inducing Apoptosis in Peripheral Mononuclear Cells. Leuk. Res. 33 (1), 140–150. doi:10.1016/j.leukres.2008.07.025
Ma, X., Yang, J., Deng, S., Huang, M., Zheng, S., Xu, S., et al. (2017). Two New Megastigmanes from Chinese Traditional Medicinal Plant Sedum Sarmentosum. Nat. Prod. Res. 31 (13), 1473–1477. doi:10.1080/14786419.2017.1280493
Ma, Y. S., Yao, C. N., Liu, H. C., Yu, F. S., Lin, J. J., Lu, K. W., et al. (2018). Quercetin Induced Apoptosis of Human Oral Cancer SAS Cells through Mitochondria and Endoplasmic Reticulum Mediated Signaling Pathways. Oncol. Lett. 15 (6), 9663–9672. doi:10.3892/ol.2018.8584
MacKenzie, T. N., Mujumdar, N., Banerjee, S., Sangwan, V., Sarver, A., Vickers, S., et al. (2013). Triptolide Induces the Expression of miR-142-3p: a Negative Regulator of Heat Shock Protein 70 and Pancreatic Cancer Cell Proliferation. Mol. Cancer Ther. 12 (7), 1266–1275. doi:10.1158/1535-7163.MCT-12-1231
Maleki Dana, P., Sadoughi, F., Asemi, Z., and Yousefi, B. (2021). Anti-cancer Properties of Quercetin in Osteosarcoma. Cancer Cel Int 21 (1), 349. doi:10.1186/s12935-021-02067-8
Mandal, A. K., Ghosh, D., Sarkar, S., Ghosh, A., Swarnakar, S., and Das, N. (2014). Nanocapsulated Quercetin Downregulates Rat Hepatic MMP-13 and Controls Diethylnitrosamine-Induced Carcinoma. Nanomedicine (Lond) 9 (15), 2323–2337. doi:10.2217/nnm.14.11
Manu, K. A., Shanmugam, M. K., Ramachandran, L., Li, F., Siveen, K. S., Chinnathambi, A., et al. (2015). Isorhamnetin Augments the Anti-tumor Effect of Capecitabine through the Negative Regulation of NF-Κb Signaling cascade in Gastric Cancer. Cancer Lett. 363 (1), 28–36. doi:10.1016/j.canlet.2015.03.033
Maurya, A. K., and Vinayak, M. (2015). Anticarcinogenic Action of Quercetin by Downregulation of Phosphatidylinositol 3-kinase (PI3K) and Protein Kinase C (PKC) via Induction of P53 in Hepatocellular Carcinoma (HepG2) Cell Line. Mol. Biol. Rep. 42 (9), 1419–1429. doi:10.1007/s11033-015-3921-7
Mayzlish-Gati, E., Fridlender, M., Nallathambi, R., Selvaraj, G., Nadarajan, S., and Koltai, H. (2018). Review on Anti-cancer Activity in Wild Plants of the Middle East. Curr. Med. Chem. 25 (36), 4656–4670. doi:10.2174/0929867324666170705113129
McCulloch, M., Broffman, M., van der Laan, M., Hubbard, A., Kushi, L., Abrams, D. I., et al. (2011). Colon Cancer Survival with Herbal Medicine and Vitamins Combined with Standard Therapy in a Whole-Systems Approach: Ten-Year Follow-Up Data Analyzed with Marginal Structural Models and Propensity Score Methods. Integr. Cancer Ther. 10 (3), 240–259. doi:10.1177/1534735411406539
Metodiewa, D., Jaiswal, A. K., Cenas, N., Dickancaité, E., and Segura-Aguilar, J. (1999). Quercetin May Act as a Cytotoxic Prooxidant after its Metabolic Activation to Semiquinone and Quinoidal Product. Free Radic. Biol. Med. 26 (1-2), 107–116. doi:10.1016/s0891-5849(98)00167-1
Miller, K. D., Fidler-Benaoudia, M., Keegan, T. H., Hipp, H. S., Jemal, A., and Siegel, R. L. (2020). Cancer Statistics for Adolescents and Young Adults, 2020a Cancer Journal for Clinicians. CA Cancer J. Clin. 70 (6), 443–459. doi:10.3322/caac.21637
Mira, A., and Shimizu, K. (2015). In Vitro Cytotoxic Activities and Molecular Mechanisms of Angelica Shikokiana Extract and its Isolated Compounds. Pharmacogn Mag. 11 (Suppl. 4), S564–S569. doi:10.4103/0973-1296.172962
Mohamed Al-Taweel, A., Ahmed Fawzy, G., and Perveen, S. (2012). Cytotoxic Flavonoid Glycosides from Rapistrum rugosum L. Iran J. Pharm. Res. 11 (3), 839–844.
Mohammed, H. A., and Al-Omar, M. S. (2021). Chemical Profile, K RA., Antioxidant, Antimicrobial, and Anticancer Activities of the Water-Ethanol Extract of Pulicaria Undulata Growing in the Oasis of Central Saudi Arabian Desert. 10.
Moon, S. K., Cho, G. O., Jung, S. Y., Gal, S. W., Kwon, T. K., Lee, Y. C., et al. (2003). Quercetin Exerts Multiple Inhibitory Effects on Vascular Smooth Muscle Cells: Role of ERK1/2, Cell-Cycle Regulation, and Matrix Metalloproteinase-9. Biochem. Biophys. Res. Commun. 301 (4), 1069–1078. doi:10.1016/s0006-291x(03)00091-3
Morales, A. M., Mukai, R., Murota, K., and Terao, J. (2018). Inhibitory Effect of Catecholic Colonic Metabolites of Rutin on Fatty Acid Hydroperoxide and Hemoglobin Dependent Lipid Peroxidation in Caco-2 Cells. J. Clin. Biochem. Nutr. 63 (3), 175–180. doi:10.3164/jcbn.18-38
Mouria, M., Gukovskaya, A. S., Jung, Y., Buechler, P., Hines, O. J., Reber, H. A., et al. (2002). Food-derived Polyphenols Inhibit Pancreatic Cancer Growth through Mitochondrial Cytochrome C Release and Apoptosis. Int. J. Cancer 98 (5), 761–769. doi:10.1002/ijc.10202
Murtaza, I., Marra, G., Schlapbach, R., Patrignani, A., Künzli, M., Wagner, U., et al. (2006). A Preliminary Investigation Demonstrating the Effect of Quercetin on the Expression of Genes Related to Cell-Cycle Arrest, Apoptosis and Xenobiotic Metabolism in Human CO115 colon-adenocarcinoma Cells Using DNA Microarray. Biotechnol. Appl. Biochem. 45 (Pt 1), 29–36. doi:10.1042/BA20060044
Nagula, R. L., and Wairkar, S. (2019). Recent Advances in Topical Delivery of Flavonoids: A Review. J. Control. Release 296, 190–201. doi:10.1016/j.jconrel.2019.01.029
Naowaratwattana, W., De-Eknamkul, W., and De Mejia, E. G. (2010). Phenolic-containing Organic Extracts of mulberry (Morus alba L.) Leaves Inhibit HepG2 Hepatoma Cells through G2/M Phase Arrest, Induction of Apoptosis, and Inhibition of Topoisomerase IIα Activity. J. Med. Food 13 (5), 1045–1056. doi:10.1089/jmf.2010.1021
Narayansingh, R., and Hurta, R. A. (2009). Cranberry Extract and Quercetin Modulate the Expression of Cyclooxygenase-2 (COX-2) and IκBα in Human colon Cancer Cells. J. Sci. Food Agric. 89 (3), 542–547. doi:10.1002/jsfa.3471
Nwaeburu, C. C., Abukiwan, A., Zhao, Z., and Herr, I. (2017). Quercetin-induced miR-200b-3p Regulates the Mode of Self-Renewing Divisions in Pancreatic Cancer. Mol. Cancer 16 (1), 23. doi:10.1186/s12943-017-0589-8
Nwaeburu, C. C., Bauer, N., Zhao, Z., Abukiwan, A., Gladkich, J., Benner, A., et al. (2016). Up-regulation of microRNA Let-7c by Quercetin Inhibits Pancreatic Cancer Progression by Activation of Numbl. Oncotarget 7 (36), 58367–58380. doi:10.18632/oncotarget.11122
Pan, B., and Pan, W. (2021). Pharmacol. Mech. Underlying Hepatoprotective Effects Ecliptae herba Hepatocellular Carcinoma 2021, 5591402. doi:10.1155/2021/5591402
Panche, A. N., Diwan, A. D., and Chandra, S. R. (2016). Flavonoids: an Overview. J. Nutr. Sci. 5, e47. doi:10.1017/jns.2016.41
Pandey, A., Vishnoi, K., Mahata, S., Tripathi, S. C., Misra, S. P., Misra, V., et al. (2015). Berberine and Curcumin Target Survivin and STAT3 in Gastric Cancer Cells and Synergize Actions of Standard Chemotherapeutic 5-Fluorouracil. Nutr. Cancer 67 (8), 1293–1304. doi:10.1080/01635581.2015.1085581
Park, C. H., Chang, J. Y., Hahm, E. R., Park, S., Kim, H. K., and Yang, C. H. (2005). Quercetin, a Potent Inhibitor against Beta-catenin/Tcf Signaling in SW480 colon Cancer Cells. Biochem. Biophys. Res. Commun. 328 (1), 227–234. doi:10.1016/j.bbrc.2004.12.151
Park, J. H., Gu, L., Von Maltzahn, G., Ruoslahti, E., Bhatia, S. N., and Sailor, M. J. (2009). Biodegradable Luminescent Porous Silicon Nanoparticles for In Vivo Applications. Nat. Mater. 8 (4), 331–336. doi:10.1038/nmat2398
Pasachan, T., Duangjai, A., Ontawong, A., Amornlerdpison, D., Jinakote, M., Phatsara, M., et al. (2021). Tiliacora Triandra (Colebr.) Diels Leaf Aqueous Extract Inhibits Hepatic Glucose Production in HepG2 Cells and Type 2 Diabetic Rats. Molecules (Basel, Switzerland). 2021;26.
Pasachan, T., Duangjai, A., Ontawong, A., and Amornlerdpison, D. (2021). Tiliacora Triandra (Colebr.) Diels Leaf Aqueous Extract Inhibits Hepatic Glucose Production in HepG2 Cells and Type 2 Diabetic Rats, 26. doi:10.3390/molecules26051239
Patel, A. (2020). Benign vs Malignant Tumors. JAMA Oncol. 6 (9), 1488. doi:10.1001/jamaoncol.2020.2592
Pham, T. N. D., Stempel, S., Shields, M. A., Spaulding, C., Kumar, K., Bentrem, D. J., et al. (2019). Quercetin Enhances the Anti-tumor Effects of BET Inhibitors by Suppressing hnRNPA1. Int. J. Mol. Sci. 20, 20. doi:10.3390/ijms20174293
Pi, J., Li, B., Tu, L., Zhu, H., Jin, H., Yang, F., et al. (2016). Investigation of Quercetin-Induced HepG2 Cell Apoptosis-Associated Cellular Biophysical Alterations by Atomic Force Microscopy. Scanning 38 (2), 100–112. doi:10.1002/sca.21245
Polukonova, N. V., Navolokin, N. A., Bucharskaya, A. B., Mudrak, D. A., Baryshnikova, M. A., Stepanova, E. V., et al. (2018). The Apoptotic Activity of Flavonoid-Containing Gratiola Officinalis Extract in Cell Cultures of Human Kidney Cancer. Russ. Open Med. J., 7. doi:10.15275/rusomj.2018.0402
Polyak, K., Xia, Y., Zweier, J. L., Kinzler, K. W., and Vogelstein, B. (1997). A Model for P53-Induced Apoptosis. Nature 389 (6648), 300–305. doi:10.1038/38525
Priprem, A., Watanatorn, J., Sutthiparinyanont, S., Phachonpai, W., and Muchimapura, S. (2008). Anxiety and Cognitive Effects of Quercetin Liposomes in Rats. Nanomedicine 4 (1), 70–78. doi:10.1016/j.nano.2007.12.001
Psahoulia, F. H., Drosopoulos, K. G., Doubravska, L., Andera, L., and Pintzas, A. (2007). Quercetin Enhances TRAIL-Mediated Apoptosis in colon Cancer Cells by Inducing the Accumulation of Death Receptors in Lipid Rafts. Mol. Cancer Ther. 6 (9), 2591–2599. doi:10.1158/1535-7163.MCT-07-0001
Psahoulia, F. H., Moumtzi, S., Roberts, M. L., Sasazuki, T., Shirasawa, S., and Pintzas, A. (2007). Quercetin Mediates Preferential Degradation of Oncogenic Ras and Causes Autophagy in Ha-RAS-Transformed Human colon Cells. Carcinogenesis 28 (5), 1021–1031. doi:10.1093/carcin/bgl232
Qi, J., Yu, J., Li, Y., Luo, J., Zhang, C., Ou, S., et al. (2019). Alternating Consumption of β‐glucan and Quercetin Reduces Mortality in Mice with Colorectal Cancer. Food Sci. Nutr. 7 (10), 3273–3285. doi:10.1002/fsn3.1187
Raffaele, M., Kovacovicova, K., Frohlich, J., Lo Re, O., Giallongo, S., Oben, J. A., et al. (2021). Mild Exacerbation of Obesity- and Age-dependent Liver Disease Progression by Senolytic Cocktail Dasatinib + Quercetin. Cell Commun Signal 19 (1), 44. doi:10.1186/s12964-021-00731-0
Ramachandran, L., Manu, K. A., Shanmugam, M. K., Li, F., Siveen, K. S., Vali, S., et al. (2012). Isorhamnetin Inhibits Proliferation and Invasion and Induces Apoptosis through the Modulation of Peroxisome Proliferator-Activated Receptor γ Activation Pathway in Gastric Cancer. J. Biol. Chem. 287 (45), 38028–38040. doi:10.1074/jbc.M112.388702
Ranelletti, F. O., Ricci, R., Larocca, L. M., Maggiano, N., Capelli, A., Scambia, G., et al. (1992). Growth-inhibitory Effect of Quercetin and Presence of Type-II Estrogen-Binding Sites in Human colon-cancer Cell Lines and Primary Colorectal Tumors. Int. J. Cancer 50 (3), 486–492. doi:10.1002/ijc.2910500326
Rashedi, J., Ghorbani Haghjo, A., Mesgari Abbasi, M., Dastranj Tabrizi, A., Yaqoubi, S., Sanajou, D., et al. (2019). Anti-tumor Effect of Quercetin Loaded Chitosan Nanoparticles on Induced Colon Cancer in Wistar Rats. Adv. Pharm. Bull. 9 (3), 409–415. doi:10.15171/apb.2019.048
Refolo, M. G., D'Alessandro, R., Malerba, N., Laezza, C., Bifulco, M., Messa, C., et al. (2015). Anti Proliferative and Pro Apoptotic Effects of Flavonoid Quercetin Are Mediated by CB1 Receptor in Human Colon Cancer Cell Lines. J. Cel Physiol 230 (12), 2973–2980. doi:10.1002/jcp.25026
Ren, L., Liu, X., Wang, Q., Zhang, L., Deng, G., Zhou, F., et al. (2017). Facile Fabrication of a Magnetically Smart PTX-Loaded Cys-Fe3O4/CuS@BSA Nano-Drug for Imaging-Guided Chemo-Photothermal Therapy. Dalton Trans. 46 (7), 2204–2213. doi:10.1039/c6dt04308a
Roos, W. P., and Kaina, B. (2006). DNA Damage-Induced Cell Death by Apoptosis. Trends Mol. Med. 12 (9), 440–450. doi:10.1016/j.molmed.2006.07.007
Russo, G. L., Russo, M., Spagnuolo, C., Tedesco, I., Bilotto, S., Iannitti, R., et al. (2014). Quercetin: a Pleiotropic Kinase Inhibitor against Cancer. Cancer Treat. Res. 159, 185–205. doi:10.1007/978-3-642-38007-5_11
Salama, Y. A., El-Karef, A., El Gayyar, A. M., and Abdel-Rahman, N. (2019). Beyond its Antioxidant Properties: Quercetin Targets Multiple Signalling Pathways in Hepatocellular Carcinoma in Rats. Life Sci. 236, 116933. doi:10.1016/j.lfs.2019.116933
Santangelo, R., Silvestrini, A., and Mancuso, C. (2019). Ginsenosides, Catechins, Quercetin and Gut Microbiota: Current Evidence of Challenging Interactions. Food Chem. Toxicol. 123, 42–49. doi:10.1016/j.fct.2018.10.042
Schiller, J. T., and Lowy, D. R. (2021). Viruses and Human Cancer: Springer, 1–11. doi:10.1007/978-3-030-57362-1_1An Introduction to Virus Infections and Human Cancer
Schirrmacher, V. (2019). From Chemotherapy to Biological Therapy: A Review of Novel Concepts to Reduce the Side Effects of Systemic Cancer Treatment (Review). Int. J. Oncol. 54 (2), 407–419. doi:10.3892/ijo.2018.4661
Sekiguchi, H., Washida, K., and Murakami, A. (2008). Suppressive Effects of Selected Food Phytochemicals on CD74 Expression in NCI-N87 Gastric Carcinoma Cells. J. Clin. Biochem. Nutr. 43 (2), 109–117. doi:10.3164/jcbn.2008054
Serri, C., Quagliariello, V., Iaffaioli, R. V., Fusco, S., Botti, G., Mayol, L., et al. (2019). Combination Therapy for the Treatment of Pancreatic Cancer through Hyaluronic Acid-Decorated Nanoparticles Loaded with Quercetin and Gemcitabine: A Preliminary In Vitro Study. J. Cel Physiol 234 (4), 4959–4969. doi:10.1002/jcp.27297
Seufi, A. M., Ibrahim, S. S., Elmaghraby, T. K., and Hafez, E. E. (2009). Preventive Effect of the Flavonoid, Quercetin, on Hepatic Cancer in Rats via Oxidant/antioxidant Activity: Molecular and Histological Evidences. J. Exp. Clin. Cancer Res. 28, 80. doi:10.1186/1756-9966-28-80
Sezer, E. D., Oktay, L. M., Karadadaş, E., Memmedov, H., Selvi Gunel, N., and Sözmen, E. (2019). Assessing Anticancer Potential of Blueberry Flavonoids, Quercetin, Kaempferol, and Gentisic Acid, through Oxidative Stress and Apoptosis Parameters on HCT-116 Cells. J. Med. Food 22 (11), 1118–1126. doi:10.1089/jmf.2019.0098
Shaalan, Y. M., Handoussa, H., Youness, R. A., Assal, R. A., El-Khatib, A. H., Linscheid, M. W., et al. (2018). Destabilizing the Interplay between miR-1275 and IGF2BPs by Tamarix Articulata and Quercetin in Hepatocellular Carcinoma. Nat. Prod. Res. 32 (18), 2217–2220. doi:10.1080/14786419.2017.1366478
Shan, B. E., Wang, M. X., and Li, R. Q. (2009). Quercetin Inhibit Human SW480 colon Cancer Growth in Association with Inhibition of Cyclin D1 and Survivin Expression through Wnt/beta-Catenin Signaling Pathway. Cancer Invest. 27 (6), 604–612. doi:10.1080/07357900802337191
Shang, H.-S., Lu, H.-F., Lee, C.-H., Chiang, H.-S., Chu, Y.-L., Chen, A., et al. (2018). Quercetin Induced Cell Apoptosis and Altered Gene Expression in AGS Human Gastric Cancer Cells. Environ. Toxicol. 33 (11), 1168–1181. doi:10.1002/tox.22623
Shang, H. S., Lu, H. F., Lee, C. H., Chiang, H. S., Chu, Y. L., Chen, A., et al. (2018). Quercetin Induced Cell Apoptosis and Altered Gene Expression in AGS Human Gastric Cancer Cells. Environ. Toxicol. 33 (11), 1168–1181. doi:10.1002/tox.22623
Sharma, A., and Bhat, M. K. (2011). Enhancement of Carboplatin- and Quercetin-Induced Cell Death by Roscovitine Is Akt Dependent and P53 Independent in Hepatoma Cells. Integr. Cancer Ther. 10, NP4–14. doi:10.1177/1534735411423922
Shen, X., Si, Y., Wang, Z., Wang, J., Guo, Y., and Zhang, X. (2016). Quercetin Inhibits the Growth of Human Gastric Cancer Stem Cells by Inducing Mitochondrial-dependent Apoptosis through the Inhibition of PI3K/Akt Signaling. Int. J. Mol. Med. 38 (2), 619–626. doi:10.3892/ijmm.2016.2625
Sheng, J., Wang, L., Han, Y., Chen, W., Liu, H., Zhang, M., et al. (2018). Dual Roles of Protein as a Template and a Sulfur Provider: a General Approach to Metal Sulfides for Efficient Photothermal Therapy of Cancer. Small 14 (1), 1702529. doi:10.1002/smll.201702529
Shi, M., Wang, F. S., and Wu, Z. Z. (2003). Synergetic Anticancer Effect of Combined Quercetin and Recombinant Adenoviral Vector Expressing Human Wild-type P53, GM-CSF and B7-1 Genes on Hepatocellular Carcinoma Cells In Vitro. World J. Gastroenterol. 9 (1), 73–78. doi:10.3748/wjg.v9.i1.73
Shi, X., Liu, D., Zhang, J., Hu, P., Shen, W., Fan, B., et al. (2016). Extraction and Purification of Total Flavonoids from pine needles of Cedrus Deodara Contribute to Anti-tumor In Vitro. BMC Complement. Altern. Med. 16, 245. doi:10.1186/s12906-016-1249-z
Shree, A., Islam, J., and Sultana, S. (2021). Quercetin Ameliorates Reactive Oxygen Species Generation, Inflammation, Mucus Depletion, Goblet Disintegration, and Tumor Multiplicity in colon Cancer: Probable Role of Adenomatous Polyposis Coli, β-catenin. Phytother Res. 35 (4), 2171–2184. doi:10.1002/ptr.6969
Singh, B. N., Singh, B. R., Sarma, B. K., and Singh, H. B. (2009). Potential Chemoprevention of N-Nitrosodiethylamine-Induced Hepatocarcinogenesis by Polyphenolics from Acacia Nilotica Bark. Chem. Biol. Interact 181 (1), 20–28. doi:10.1016/j.cbi.2009.05.007
Singh, V., Singh, R., Kujur, P. K., and Singh, R. P. (2020). Combination of Resveratrol and Quercetin Causes Cell Growth Inhibition, DNA Damage, Cell Cycle Arrest, and Apoptosis in Oral Cancer Cells. Assay Drug Dev. Technol. 18 (5), 226–238. doi:10.1089/adt.2020.972
Sobral, F., Calhelha, R. C., Barros, L., Dueñas, M., Tomás, A., Santos-Buelga, C., et al. (2017). Flavonoid Composition and Antitumor Activity of Bee Bread Collected in Northeast Portugal. Molecules 22, 22. doi:10.3390/molecules22020248
Srisa-Nga, K., Mankhetkorn, S., Okonogi, S., and Khonkarn, R. (2019). Delivery of Superparamagnetic Polymeric Micelles Loaded with Quercetin to Hepatocellular Carcinoma Cells. J. Pharm. Sci. 108 (2), 996–1006. doi:10.1016/j.xphs.2018.08.008
Srivastava, R. K., Tang, S. N., Zhu, W., Meeker, D., and Shankar, S. (2011). “Sulforaphane Synergizes with Quercetin to Inhibit Self-Renewal Capacity of Pancreatic Cancer Stem Cells,”In Front. Biosci. Editor F. Biosci, E3, 515–528. doi:10.2741/e266
Sudan, S., and Rupasinghe, H. P. (2014). Quercetin-3-O-glucoside Induces Human DNA Topoisomerase II Inhibition, Cell Cycle Arrest and Apoptosis in Hepatocellular Carcinoma Cells. Anticancer Res. 34 (4), 1691–1699.
Sudan, S., and Rupasinghe, H. V. (2015). Antiproliferative Activity of Long Chain Acylated Esters of Quercetin-3-O-Glucoside in Hepatocellular Carcinoma HepG2 Cells. Exp. Biol. Med. (Maywood) 240 (11), 1452–1464. doi:10.1177/1535370215570828
Sun, C. Y., Qin, C., Wang, C. G., Su, Z. M., Wang, S., Wang, X. L., et al. (2011). Chiral Nanoporous Metal-Organic Frameworks with High Porosity as Materials for Drug Delivery. Adv. Mater. 23 (47), 5629–5632. doi:10.1002/adma.201102538
Sun, C. Y., Qin, C., Wang, X. L., Yang, G. S., Shao, K. Z., Lan, Y. Q., et al. (2012). Zeolitic Imidazolate Framework-8 as Efficient pH-Sensitive Drug Delivery Vehicle. Dalton Trans. 41 (23), 6906–6909. doi:10.1039/c2dt30357d
Tan, J., Wang, B., and Zhu, L. (2009). Regulation of Survivin and Bcl-2 in HepG2 Cell Apoptosis Induced by Quercetin. Chem. Biodivers 6 (7), 1101–1110. doi:10.1002/cbdv.200800141
Tang, S. N., Fu, J., Nall, D., Rodova, M., Shankar, S., and Srivastava, R. K. (2012). Inhibition of Sonic Hedgehog Pathway and Pluripotency Maintaining Factors Regulate Human Pancreatic Cancer Stem Cell Characteristics. Int. J. Cancer 131 (1), 30–40. doi:10.1002/ijc.26323
Tanigawa, S., Fujii, M., and Hou, D. X. (2008). Stabilization of P53 Is Involved in Quercetin-Induced Cell Cycle Arrest and Apoptosis in HepG2 Cells. Biosci. Biotechnol. Biochem. 72 (3), 797–804. doi:10.1271/bbb.70680
Tao, X., Chen, Q., Li, N., Xiang, H., Pan, Y., Qu, Y., et al. (2020). Serotonin-RhoA/ROCK axis Promotes Acinar-To-Ductal Metaplasia in Caerulein-Induced Chronic Pancreatitis. Biomed. Pharmacother. 125, 109999. doi:10.1016/j.biopha.2020.109999
Tian, Z., Yao, X., and Zhu, Y. (2017). Simple Synthesis of Multifunctional Zeolitic Imidazolate Frameworks-8/graphene Oxide Nanocrystals with Controlled Drug Release and Photothermal Effect. Microporous Mesoporous Mater. 237, 160–167. doi:10.1016/j.micromeso.2016.09.036
Vafadar, A., Shabaninejad, Z., Movahedpour, A., Fallahi, F., Taghavipour, M., Ghasemi, Y., et al. (2020). Quercetin and Cancer: New Insights into its Therapeutic Effects on Ovarian Cancer Cells. Cell Biosci 10, 32. doi:10.1186/s13578-020-00397-0
van der Woude, H., Gliszczyńska-Swigło, A., Struijs, K., Smeets, A., Alink, G. M., and Rietjens, I. M. (2003). Biphasic Modulation of Cell Proliferation by Quercetin at Concentrations Physiologically Relevant in Humans. Cancer Lett. 200 (1), 41–47. doi:10.1016/s0304-3835(03)00412-9
van Erk, M. J., Roepman, P., van der Lende, T. R., Stierum, R. H., Aarts, J. M., van Bladeren, P. J., et al. (2005). Integrated Assessment by Multiple Gene Expression Analysis of Quercetin Bioactivity on Anticancer-Related Mechanisms in colon Cancer Cells In Vitro. Eur. J. Nutr. 44 (3), 143–156. doi:10.1007/s00394-004-0503-1
Vargas, A. J., and Burd, R. (2010). Hormesis and Synergy: Pathways and Mechanisms of Quercetin in Cancer Prevention and Management. Nutr. Rev. 68 (7), 418–428. doi:10.1111/j.1753-4887.2010.00301.x
Varshosaz, J., Jafarian, A., Salehi, G., and Zolfaghari, B. (2014). Comparing Different Sterol Containing Solid Lipid Nanoparticles for Targeted Delivery of Quercetin in Hepatocellular Carcinoma. J. Liposome Res. 24 (3), 191–203. doi:10.3109/08982104.2013.868476
Velázquez, K. T., Enos, R. T., Narsale, A. A., Puppa, M. J., Davis, J. M., Murphy, E. A., et al. (2014). Quercetin Supplementation Attenuates the Progression of Cancer Cachexia in ApcMin/+ Mice. J. Nutr. 144 (6), 868–875. doi:10.3945/jn.113.188367
Venna, S. R., Jasinski, J. B., and Carreon, M. A. (2010). Structural Evolution of Zeolitic Imidazolate Framework-8. J. Am. Chem. Soc. 132 (51), 18030–18033. doi:10.1021/ja109268m
Vicentini, F. T., Simi, T. R., Del Ciampo, J. O., Wolga, N. O., Pitol, D. L., Iyomasa, M. M., et al. (2008). Quercetin in W/o Microemulsion: In Vitro and In Vivo Skin Penetration and Efficacy against UVB-Induced Skin Damages Evaluated In Vivo. Eur. J. Pharm. Biopharm. 69 (3), 948–957. doi:10.1016/j.ejpb.2008.01.012
Vijayakumar, K., Rengarajan, R. L., Radhakrishnan, R., Mathew, S., Qadri, I., and Vijaya Anand, A. (2019). Psidium Guajava Leaf Extracts and Their Quercetin Protect HepG2 Cell Lines against CCL4 Induced Cytotoxicity. Indian J. Clin. Biochem. 34 (3), 324–329. doi:10.1007/s12291-018-0752-z
Walle, T., and Walle, U. K. (2007). Novel Methoxylated Flavone Inhibitors of Cytochrome P450 1B1 in SCC-9 Human Oral Cancer Cells. J. Pharm. Pharmacol. 59 (6), 857–862. doi:10.1211/jpp.59.6.0012
Wang, C., Su, L., Wu, C., Wu, J., Zhu, C., and Yuan, G. (2016). RGD Peptide Targeted Lipid-Coated Nanoparticles for Combinatorial Delivery of Sorafenib and Quercetin against Hepatocellular Carcinoma. Drug Dev. Ind. Pharm. 42 (12), 1938–1944. doi:10.1080/03639045.2016.1185435
Wang, K., Liu, R., Li, J., Mao, J., Lei, Y., Wu, J., et al. (2011). Quercetin Induces Protective Autophagy in Gastric Cancer Cells: Involvement of Akt-mTOR- and Hypoxia-Induced Factor 1α-Mediated Signaling. Autophagy 7 (9), 966–978. doi:10.4161/auto.7.9.15863
Wang, P., Zhang, K., Zhang, Q., Mei, J., Chen, C. J., Feng, Z. Z., et al. (2012). Effects of Quercetin on the Apoptosis of the Human Gastric Carcinoma Cells. Toxicol. Vitro 26 (2), 221–228. doi:10.1016/j.tiv.2011.11.015
Wang, Z., Tang, X., Wang, X., Yang, D., Yang, C., Lou, Y., et al. (2016). Near-infrared Light-Induced Dissociation of Zeolitic Imidazole Framework-8 (ZIF-8) with Encapsulated CuS Nanoparticles and Their Application as a Therapeutic Nanoplatform. Chem. Commun. (Camb) 52 (82), 12210–12213. doi:10.1039/c6cc06616j
Warren, C. A., Paulhill, K. J., Davidson, L. A., Lupton, J. R., Taddeo, S. S., Hong, M. Y., et al. (2009). Quercetin May Suppress Rat Aberrant Crypt Foci Formation by Suppressing Inflammatory Mediators that Influence Proliferation and Apoptosis. J. Nutr. 139 (1), 101–105. doi:10.3945/jn.108.096271
Wenzel, U., Herzog, A., Kuntz, S., and Daniel, H. (2004). Protein Expression Profiling Identifies Molecular Targets of Quercetin as a Major Dietary Flavonoid in Human colon Cancer Cells. Proteomics 4 (7), 2160–2174. doi:10.1002/pmic.200300726
Wu, H., Pan, L., Gao, C., Xu, H., Li, Y., Zhang, L., et al. (2019). Quercetin Inhibits the Proliferation of Glycolysis-Addicted HCC Cells by Reducing Hexokinase 2 and Akt-mTOR Pathway. Molecules 24, 24. doi:10.3390/molecules24101993
Wu, L., Li, J., Liu, T., Li, S., Feng, J., Yu, Q., et al. (2019). Quercetin Shows Anti‐tumor Effect in Hepatocellular Carcinoma LM3 Cells by Abrogating JAK2/STAT3 Signaling Pathway. Cancer Med. 8 (10), 4806–4820. doi:10.1002/cam4.2388
Wu, T. H., Yen, F. L., Lin, L. T., Tsai, T. R., Lin, C. C., and Cham, T. M. (2008). Preparation, Physicochemical Characterization, and Antioxidant Effects of Quercetin Nanoparticles. Int. J. Pharm. 346 (1-2), 160–168. doi:10.1016/j.ijpharm.2007.06.036
Xavier, C. P., Lima, C. F., Preto, A., Seruca, R., Fernandes-Ferreira, M., and Pereira-Wilson, C. (2009). Luteolin, Quercetin and Ursolic Acid Are Potent Inhibitors of Proliferation and Inducers of Apoptosis in Both KRAS and BRAF Mutated Human Colorectal Cancer Cells. Cancer Lett. 281 (2), 162–170. doi:10.1016/j.canlet.2009.02.041
Xavier, C. P., Lima, C. F., Rohde, M., and Pereira-Wilson, C. (2011). Quercetin Enhances 5-Fluorouracil-Induced Apoptosis in MSI Colorectal Cancer Cells through P53 Modulation. Cancer Chemother. Pharmacol. 68 (6), 1449–1457. doi:10.1007/s00280-011-1641-9
Xia, J., Rong, L., Sawakami, T., Inagaki, Y., Song, P., Hasegawa, K., et al. (2018). Shufeng Jiedu Capsule and its Active Ingredients Induce Apoptosis, Inhibit Migration and Invasion, and Enhances Doxorubicin Therapeutic Efficacy in Hepatocellular Carcinoma. Biomed. Pharmacother. 99, 921–930. doi:10.1016/j.biopha.2018.01.163
Xu, J., Chen, H. B., and Li, S. L. (2017). Understanding the Molecular Mechanisms of the Interplay between Herbal Medicines and Gut Microbiota. Med. Res. Rev. 37 (5), 1140–1185. doi:10.1002/med.21431
Yamada, N., Matsushima-Nishiwaki, R., and Kozawa, O. (2020). Quercetin Suppresses the Migration of Hepatocellular Carcinoma Cells Stimulated by Hepatocyte Growth Factor or Transforming Growth Factor-α: Attenuation of AKT Signaling Pathway. Arch. Biochem. Biophys. 682, 108296. doi:10.1016/j.abb.2020.108296
Yang, L., Liu, Y., Wang, M., Qian, Y., Dong, X., Gu, H., et al. (2016). Quercetin-induced Apoptosis of HT-29 colon Cancer Cells via Inhibition of the Akt-CSN6-Myc Signaling axis. Mol. Med. Rep. 14 (5), 4559–4566. doi:10.3892/mmr.2016.5818
Yang, Y., Wang, T., Chen, D., Ma, Q., Zheng, Y., Liao, S., et al. (2019). Quercetin Preferentially Induces Apoptosis in KRAS-Mutant Colorectal Cancer Cells via JNK Signaling Pathways. Cell Biol Int 43 (2), 117–124. doi:10.1002/cbin.11055
Yang, Y., Wang, T., Chen, D., Ma, Q., Zheng, Y., Liao, S., et al. (2019). Quercetin Preferentially Induces Apoptosis in KRAS-Mutant Colorectal Cancer Cells via JNK Signaling Pathways. Cel Biol Int 43 (2), 117–124. doi:10.1002/cbin.11055
Yao, X., Niu, X., Ma, K., Huang, P., Grothe, J., Kaskel, S., et al. (2017). Graphene Quantum Dots-Capped Magnetic Mesoporous Silica Nanoparticles as a Multifunctional Platform for Controlled Drug Delivery, Magnetic Hyperthermia, and Photothermal Therapy. Small 13 (2), 1602225. doi:10.1002/smll.201602225
Yao, X., Tian, Z., Liu, J., Zhu, Y., and Hanagata, N. (2017). Mesoporous Silica Nanoparticles Capped with Graphene Quantum Dots for Potential Chemo-Photothermal Synergistic Cancer Therapy. Langmuir 33 (2), 591–599. doi:10.1021/acs.langmuir.6b04189
Yen, F. L., Wu, T. H., Lin, L. T., Cham, T. M., and Lin, C. C. (2009). Naringenin-loaded Nanoparticles Improve the Physicochemical Properties and the Hepatoprotective Effects of Naringenin in Orally-Administered Rats with CCl(4)-Induced Acute Liver Failure. Pharm. Res. 26 (4), 893–902. doi:10.1007/s11095-008-9791-0
Yoon, K. A., Nakamura, Y., and Arakawa, H. (2004). Identification of ALDH4 as a P53-Inducible Gene and its Protective Role in Cellular Stresses. J. Hum. Genet. 49 (3), 134–140. doi:10.1007/s10038-003-0122-3
Yoshida, M., Sakai, T., Hosokawa, N., Marui, N., Matsumoto, K., Fujioka, A., et al. (1990). The Effect of Quercetin on Cell Cycle Progression and Growth of Human Gastric Cancer Cells. FEBS Lett. 260 (1), 10–13. doi:10.1016/0014-5793(90)80053-l
Yu, D., Ye, T., Xiang, Y., Shi, Z., Zhang, J., Lou, B., et al. (2017). Quercetin Inhibits Epithelial-Mesenchymal Transition, Decreases Invasiveness and Metastasis, and Reverses IL-6 Induced Epithelial-Mesenchymal Transition, Expression of MMP by Inhibiting STAT3 Signaling in Pancreatic Cancer Cells. Onco Targets Ther. 10, 4719–4729. doi:10.2147/OTT.S136840
Yuan, Z., Wang, H., Hu, Z., Huang, Y., Yao, F., Sun, S., et al. (2015). Quercetin Inhibits Proliferation and Drug Resistance in KB/VCR Oral Cancer Cells and Enhances its Sensitivity to Vincristine. Nutr. Cancer 67 (1), 126–136. doi:10.1080/01635581.2015.965334
Yuan, Z. P., Chen, L. J., Wei, Y. Q., Fan, L. Y., Tang, M. H., and Yang, G. L. (2006). Nanoliposomal Quercetin Inhibits Formation of Malignant Ascites of Hepatocellular Carcinoma. Ai Zheng 25 (8), 941–945.
Zeng, Y., Shen, Z., Gu, W., and Wu, M. (2018). Bioinformatics Analysis to Identify Action Targets in NCI-N87 Gastric Cancer Cells Exposed to Quercetin. Pharm. Biol. 56 (1), 393–398. doi:10.1080/13880209.2018.1493610
Zhang, B., Yu, D., Luo, N., Yang, C., and Zhu, Y. (2020). Four Active Monomers from Moutan Cortex Exert Inhibitory Effects against Oxidative Stress by Activating Nrf2/Keap1 Signaling Pathway. Korean J. Physiol. Pharmacol. 24 (5), 373–384. doi:10.4196/kjpp.2020.24.5.373
Zhang, H., Zhang, M., Yu, L., Zhao, Y., He, N., and Yang, X. (2012). Antitumor Activities of Quercetin and Quercetin-5',8-Disulfonate in Human colon and Breast Cancer Cell Lines. Food Chem. Toxicol. 50 (5), 1589–1599. doi:10.1016/j.fct.2012.01.025
Zhang, H., Jiang, W., Liu, R., Zhang, J., Zhang, D., Li, Z., et al. (2017). Rational Design of Metal Organic Framework Nanocarrier-Based Codelivery System of Doxorubicin Hydrochloride/verapamil Hydrochloride for Overcoming Multidrug Resistance with Efficient Targeted Cancer Therapy. ACS Appl. Mater. Inter. 9 (23), 19687–19697. doi:10.1021/acsami.7b05142
Zhang, J. Y., Lin, M. T., Zhou, M. J., Yi, T., Tang, Y. N., Tang, S. L., et al. (2015). Combinational Treatment of Curcumin and Quercetin against Gastric Cancer MGC-803 Cells In Vitro. Molecules 20 (6), 11524–11534. doi:10.3390/molecules200611524
Zhang, L., Angst, E., Park, J. L., Moro, A., Dawson, D. W., Reber, H. A., et al. (2010). Quercetin Aglycone Is Bioavailable in Murine Pancreas and Pancreatic Xenografts. J. Agric. Food Chem. 58 (12), 7252–7257. doi:10.1021/jf101192k
Zhang, Q., Zhao, X. H., and Wang, Z. J. (2009). Cytotoxicity of Flavones and Flavonols to a Human Esophageal Squamous Cell Carcinoma Cell Line (KYSE-510) by Induction of G2/M Arrest and Apoptosis. Toxicol. Vitro 23 (5), 797–807. doi:10.1016/j.tiv.2009.04.007
Zhang, Q., Zhao, X. H., and Wang, Z. J. (2009). Cytotoxicity of Flavones and Flavonols to a Human Esophageal Squamous Cell Carcinoma Cell Line (KYSE-510) by Induction of G2/M Arrest and Apoptosis. Toxicol. Vitro 23 (5), 797–807. doi:10.1016/j.tiv.2009.04.007
Zhang, Q., Zhao, X. H., and Wang, Z. J. (2008). Flavones and Flavonols Exert Cytotoxic Effects on a Human Oesophageal Adenocarcinoma Cell Line (OE33) by Causing G2/M Arrest and Inducing Apoptosis. Food Chem. Toxicol. 46 (6), 2042–2053. doi:10.1016/j.fct.2008.01.049
Zhang, Q., Zhao, X. H., and Wang, Z. J. (2008). Flavones and Flavonols Exert Cytotoxic Effects on a Human Oesophageal Adenocarcinoma Cell Line (OE33) by Causing G2/M Arrest and Inducing Apoptosis. Food Chem. Toxicol. 46 (6), 2042–2053. doi:10.1016/j.fct.2008.01.049
Zhang, S., Huang, J., Xie, X., He, Y., Mo, F., and Luo, Z. (2017). Quercetin from Polygonum Capitatum Protects against Gastric Inflammation and Apoptosis Associated with Helicobacter pylori Infection by Affecting the Levels of p38MAPK, BCL-2 and BAX. Molecules 22, 22. doi:10.3390/molecules22050744
Zhang, X. A., Zhang, S., Yin, Q., and Zhang, J. (2015). Quercetin Induces Human colon Cancer Cells Apoptosis by Inhibiting the Nuclear Factor-Kappa B Pathway. Pharmacogn Mag. 11 (42), 404–409. doi:10.4103/0973-1296.153096
Zhao, J., Fang, Z., Zha, Z., Sun, Q., Wang, H., Sun, M., et al. (2019). Quercetin Inhibits Cell Viability, Migration and Invasion by Regulating miR-16/HOXA10 axis in Oral Cancer. Eur. J. Pharmacol. 847, 11–18. doi:10.1016/j.ejphar.2019.01.006
Zhao, J. L., Zhao, J., and Jiao, H. J. (2014). Synergistic Growth-Suppressive Effects of Quercetin and Cisplatin on HepG2 Human Hepatocellular Carcinoma Cells. Appl. Biochem. Biotechnol. 172 (2), 784–791. doi:10.1007/s12010-013-0561-z
Zhao, S., Jiang, Y., Zhao, J., Li, H., Yin, X., Wang, Y., et al. (2018). Quercetin-3-methyl Ether Inhibits Esophageal Carcinogenesis by Targeting the AKT/mTOR/p70S6K and MAPK Pathways. Mol. Carcinog 57 (11), 1540–1552. doi:10.1002/mc.22876
Zhao, S., Jiang, Y., Zhao, J., Li, H., Yin, X., Wang, Y., et al. (2018). Quercetin‐3‐methyl Ether Inhibits Esophageal Carcinogenesis by Targeting the AKT/mTOR/p70S6K and MAPK Pathways. Mol. Carcinogenesis 57 (11), 1540–1552. doi:10.1002/mc.22876
Zhao, Y., Fan, D., Zheng, Z. P., Li, E. T., Chen, F., Cheng, K. W., et al. (2017). 8-C-(E-phenylethenyl)quercetin from Onion/beef Soup Induces Autophagic Cell Death in colon Cancer Cells through ERK Activation. Mol. Nutr. Food Res. 61, 61. doi:10.1002/mnfr.201600437
Zheng, N. G., Mo, S. J., Li, J. P., and Wu, J. L. (2014). Anti-CSC Effects in Human Esophageal Squamous Cell Carcinomas and Eca109/9706 Cells Induced by Nanoliposomal Quercetin Alone or Combined with CD 133 Antiserum. Asian Pac. J. Cancer Prev. 15 (20), 8679–8684. doi:10.7314/apjcp.2014.15.20.8679
Zheng, N. G., Wang, J. L., Yang, S. L., and Wu, J. L. (2014). Aberrant Epigenetic Alteration in Eca9706 Cells Modulated by Nanoliposomal Quercetin Combined with Butyrate Mediated via Epigenetic-NF-Κb Signaling. Asian Pac. J. Cancer Prev. 15 (11), 4539–4543. doi:10.7314/apjcp.2014.15.11.4539
Zhou, J., Fang, L., Yao, W. X., Zhao, X., Wei, Y., Zhou, H., et al. (2011). Effect of Quercetin on Heat Shock Protein Expression in HepG2 Cells Determined by SILAC. Zhonghua Zhong Liu Za Zhi 33 (10), 737–741.
Zhou, P., Cheng, Y., Liu, F., Wu, K., Qiu, W., and Wang, S. (2019). Tanshindiol-C Suppresses In Vitro and In Vivo Hepatocellular Cancer Cell Growth by Inducing Mitochondrial-Mediated Apoptosis, Cell Cycle Arrest, Inhibition of Angiogenesis and Modulation of Key Tumor-Suppressive miRNAs. J. Buon 24 (2), 622–627.
Zhou, W., Kallifatidis, G., Baumann, B., Rausch, V., Mattern, J., Gladkich, J., et al. (2010). Dietary Polyphenol Quercetin Targets Pancreatic Cancer Stem Cells. Int. J. Oncol. 37 (3), 551–561. doi:10.3892/ijo_00000704
Zhou, W., Liang, X., Dai, P., Chen, Y., Zhang, Y., Zhang, M., et al. (2019). Alteration of Phenolic Composition in Lettuce (Lactuca sativa L.) by Reducing Nitrogen Supply Enhances its Anti-proliferative Effects on Colorectal Cancer Cells. Int. J. Mol. Sci. 20, 20. doi:10.3390/ijms20174205
Zhou, X. L., Chen, Z. D., Zhou, Y. M., Shi, R. H., and Li, Z. J. (2019). The Effect of Tartary Buckwheat Flavonoids in Inhibiting the Proliferation of MGC80-3 Cells during Seed Germination. Molecules 24, 24. doi:10.3390/molecules24173092
Zhu, Y., Jiang, Y., Shi, L., Du, L., Xu, X., Wang, E., et al. (2017). 7-O-Geranylquercetin Induces Apoptosis in Gastric Cancer Cells via ROS-MAPK Mediated Mitochondrial Signaling Pathway Activation. Biomed. Pharmacother. 87, 527–538. doi:10.1016/j.biopha.2016.12.095
Keywords: gastrointestinal cancers, quercetin, therapy, natural compounds, bioactive compounds
Citation: Mirazimi SMA, Dashti F, Tobeiha M, Shahini A, Jafari R, Khoddami M, Sheida AH, EsnaAshari P, Aflatoonian AH, Elikaii F, Zakeri MS, Hamblin MR, Aghajani M, Bavarsadkarimi M and Mirzaei H (2022) Application of Quercetin in the Treatment of Gastrointestinal Cancers. Front. Pharmacol. 13:860209. doi: 10.3389/fphar.2022.860209
Received: 22 January 2022; Accepted: 02 March 2022;
Published: 06 April 2022.
Edited by:
Husain Yar Khan, Wayne State University, United StatesReviewed by:
Ashish Ranjan Sharma, Hallym University, South KoreaMohd Farhan, King Faisal University, Saudi Arabia
Copyright © 2022 Mirazimi, Dashti, Tobeiha, Shahini, Jafari, Khoddami, Sheida, EsnaAshari, Aflatoonian, Elikaii, Zakeri, Hamblin, Aghajani, Bavarsadkarimi and Mirzaei. This is an open-access article distributed under the terms of the Creative Commons Attribution License (CC BY). The use, distribution or reproduction in other forums is permitted, provided the original author(s) and the copyright owner(s) are credited and that the original publication in this journal is cited, in accordance with accepted academic practice. No use, distribution or reproduction is permitted which does not comply with these terms.
*Correspondence: Mohammad Aghajani, YWdoYWphbmkxMzYyQHlhaG9vLmNvbQ==; Hamed Mirzaei, bWlyemFlaS1oQGthdW1zLmFjLmly
†These authors have equally contributed to this work