- 1Faculty of Agro-Based Industry, Universiti Malaysia Kelantan, Kota Bharu, Malaysia
- 2Faculty of Veterinary Medicine, Universiti Malaysia Kelantan, Kota Bharu, Malaysia
- 3Faculty of Data Science and Information Technology, INTI International University, Nilai, Malaysia
- 4Department of Orthopaedics, School of Medical Sciences, Universiti Sains Malaysia, Kubang Kerian, Malaysia
Background: Beka (Oroxylum indicum (L.) Kurz) has been used as a culinary herb and natural remedy by the local communities in Malaysia. The leaf of O. indicum is traditionally used for the treatment of diarrhea, high blood pressure, and improving digestive health.
Objectives: The present study was conducted to evaluate the phytochemical constituents and wound healing properties (in vitro and in vivo models) of aqueous and ethanol extracts of O. indicum leaves.
Methods: The total phenolic (TPC) and total flavonoid (TFC) contents in the plant extracts were determined by the spectrophotometric methods. Further, the extract was characterized by Liquid Chromatography Time-of-Flight Mass Spectrometry (LC-TOF-MS/MS) and Gas Chromatography-Mass Spectrometry (GC-MS). The wound healing activity was assessed using the in vitro scratch wound-healing assay and in vivo excisional wound model.
Results: The results show the ethanol leaves extract had the higher TPC (164 mg GAE/g) when compared with the aqueous leaves extract (30 mg gallic acid equivalents/g). The ethanol leaves extract was also found to have higher TFC (101 mg Catechin equivalents/g) than the aqueous leaves extract (76 mg Catechin equivalents/g). The ethanol leaves extract was then used for further chemical analysis. The LC–TOF-MS/MS analysis showed that the leaves extracts of O. indicum contains many important compounds such as Orientin, Chrysin, Pinoquercetin, Cupressuflavone, Puerarin xyloside, Forsythiaside and Paederoside. In GC-MS analysis, 19 compounds were identified in ethanolic leaves extract. The wound healing studies shows that O. indicum has promising wound healing activity by increasing the rate of wound contraction significantly (p < 0.05).
Conclusion: In conclusion, the present study showed that O. indicum leaf contains important phytochemicals and the wound healing potential of the O. indicum extract may probably be as a result of the presence of various phytoconstituents.
Introduction
The wound healing process is described as a complex biological process where multiple and interrelated pathways are activated to induce wound repair (Asadi et al., 2013). The wound healing process starts immediately after the injury, generally wound healing process is characterized into three phases: 1) inflammatory phase, consisting of homeostasis and inflammation; 2) proliferative phase, consisting of granulation tissue formation, epithelialisation and contraction; and 3) the remodelling phase (Morales-González et al., 2022). For the wound healing process to occur, it is important to undergo a process that consists of a sequence of molecular and cellular events for the tissue damage to be fixed and restore. These events occur through the integration of dynamic processes involving soluble mediators, blood cells and parenchymal cells (Tottoli et al., 2020). It also involves partially overlapping phases, as some of the factors such as contaminated wound cause prolonged of certain phases, usually the inflammatory phase, which lead to delays in the wound healing process (Tottoli et al., 2020). For wound management or method in treating wounds are debridement, irrigation, antibiotics, tissue grafts and proteolytic enzymes, but these methods have disadvantages and undesirable side effects.
Herbs have been used for both culinary and medicinal purposes for many years (Khanal et al., 2021). Consumption of herbs may help to treat or prevent several diseases such as cancer, diabetes, high cholesterol levels, cardiovascular, and inflammatory diseases (Khanal et al., 2021; Sachdeva et al., 2022). Besides serving as a culinary and medicinal purpose, herbs have been also a valuable source for the production of novel pharmaceutical drugs, food preservative agents and cosmetics products (Gurib-Fakim, 2014; Bailly, 2021; Abdulhafiz, et al., 2022). Recently, the demand for herbal medicine and natural products has increased massively. The increased patronage and interest on herbal medicine has been attributed to several reasons such as safety claims, growing preference for natural therapies, high cost and adverse side effects of modern drugs (Ekor, 2014; Abdulhafiz et al., 2020b; Alsamri et al., 2021; Maher et al., 2021a).
Plants contains various chemicals, also known as phytochemicals, and are responsible for beneficial or biological effects. These chemicals are stored in their various organs such as fruits, seeds, leaves, stems, roots, bark etc. Phytochemical contents in any plant (even within the species) vary from place to place as it is affected by various factors such as environment, rainfall, temperature and growing conditions. Recently, more attention has been focused on identification and quantification techniques to get useful chemical information (fingerprint) in crude extracts from medicinal herbs. This approach has helped to a better understanding of herbs and herbal products (Goodarzi et al., 2013; Chen et al., 2016; Abdulhafiz et al., 2020a; Reduan et al., 2020). Several analytical methods have been developed and used by various researchers around the world (Tistaert et al., 2011; Saini et al., 2016; Rahman et al., 2018; Kharbach et al., 2020; Maqsood et al., 2020; Maher et al., 2021b). One of the most recent technique for generating herbal fingerprints is LC-TOF-MS/MS. This analytical tool has high separation capacity, sensitivity, detects a wide range of chemicals in a short time by its TOF analyzer and provides accurate mass measurement for metabolite identification (Alaerts et al., 2010; Wu et al., 2022) and this method allows the biomarker discovery where the candidate markers are likely to present in smaller quantity (Metz et al., 2007; Lei et al., 2011; Gowda and Djukovic, 2014; Rainville et al., 2014; Want, 2018).
Oroxylum indicum (L.) Kurz belongs to the family Bignoniaceae and is widely distributed throughout Asian counties, including Malaysia, Thailand, Indonesia, India, Vietnam, Philippines, China, Taiwan, and Japan. It is an important culinary herb with several medicinal and culinary purposes (Swargiary and Daimari, 2020). The parts used in traditional medicinal practices are the fruit, seed, leaves, stems, bark and roots. Although, all parts of the plant are being used in traditional medicine, some of them still remain unexplored. Some pharmacological studies reported the medicinal values and phytochemical content of Oroxylum indicum, some of which include antidiabetic (Singh and Kakkar, 2013; Sun et al., 2018), antiadipogenic activity (Hengpratom et al., 2020) and anticancer (Yoganarasimhan, 1996). Therefore, this study was conducted to evaluate the phytochemical constituents and wound healing properties (in vitro and in vivo models) of aqueous and ethanol extracts of O. indicum.
Methods
Chemicals and reagents
Chemicals and solvents such as sodium nitrate, aluminum chloride, sodium carbonate and ethanol (95%) were purchased from local vendor. Folin-Ciocalteu was purchased from Sigma-Aldrich (United States). Distilled water, whatman No.1 filter paper, and aluminum foil were obtained from the laboratory.
Plant sample collection
The fresh leaves of Oroxylum indicum (approximately one month old) were obtained from the local market in Kota Bharu, Kelantan, Malaysia. The plant part was identified by a botanist from Herbarium, Universiti Kebangsaan Malaysia, Selangor, Malaysia. A voucher specimen (ID025/2020) was deposited at the herbarium for reference. The leaves were given cut from leaf axil and separated from the main stem carefully. The leaves sample were then washed with tap water, dried in the open air, and then ground into powder using an electrical blender (Milux MCB-905, Milux Sdn Bhd, Selangor, Malaysia). The powder was stored in air tight plastic container until further use.
Preparation of sample extract
Sample extraction was carried out as described by Abubakar and Haque (2020) with some modifications. Briefly, powdered plant samples about 150 g were macerated for 72 h with 1.5 L of distilled water or 95% ethanol solvent at the room temperature. After 72 h of soaking, the extracts were filtered through Whatman No.1 filter paper. The filtrates were then concentrated to dryness using a rotary evaporator at 40°C. The crude extracts were then stored at 4°C until further use.
Preliminary phytochemical study
Determination of total phenolic content
TPC was determined by using Folin-Ciocalteu reagent spectrophotometrically following the procedure of Abdulhafiz et al. (2020b) with minor modification. Briefly, 100 µL of aqueous or ethanolic extract (500 μg/ml) was mixed with 1 ml of FC reagent (10%). After 10 min, 1 ml of sodium carbonate (7.5%, w/v) was added, mixed and incubated in the dark for 90 min. The absorbance was measured using a spectrophotometer (UV-1900, UV-Vis Spectrophotometer, Shimadzu Co. Japan) at λmax = 750 nm against the blank solution containing 100 µL of 95% ethanol, 1 ml of FC reagent (10%) and 1 ml of sodium carbonate (7.5%). The samples were prepared in triplicate for each analysis and the mean value of absorbance was obtained. TPC was calculated from a calibrated curve, using gallic acid as standard (12.5–100 μg/ml). The concentration of TPC was expressed as mg of gallic acid equivalents per gram of dry weight (mg GA/g extract).
Determination of total flavonoid content
TFC in the plant extracts was determined by the aluminum chloride colorimetric method following the procedure of Fattahi et al. (2014) with a minor modification. One hundred microliters of aqueous or ethanol extract (1 mg/ml) or standard were added to 4 ml of sterile distilled water. Then 0.3 ml of 5% sodium nitrate solution (w/v) was added. After 5 minutes, 0.3 ml of 10% aluminum chloride solution (w/v) was added. In 6 min, 2 ml of 4% (w/v) NaOH was added to the mixture. Then, the mixture was diluted with 1 ml distilled water and mixed thoroughly. The absorbance was measured at λmax = 518 nm immediately. Standard Catechin was used to construct the calibration curve (50–250 μg/ml). The concentration of TFC was expressed as mg of Catechin equivalents per g of dry extract (mg CE/g d.wt extract).
Gas chromatography-mass spectrometry (GC-MS) analysis
The GC-MS analysis of the ethanolic leaves extracts of Beka was carried out by Gas Chromatography-Mass Spectrometry, GC-MS (Perkin-Elmer /Clarus 600T). The Perkin Elmer Clarus 600 GCMS was coupled to TurboMatrix Headspace Sampler 40, run on GC-MS column Elite 5MS (30 m × 250 mm x 0.25 µm). The GC-MS condition was set at the Initial oven temperature of 70°C and raised to 76°C at the rate of 1°C/min and was maintained for 1 min. Then, the oven temperature was raised to 300°C, at the rate of 6 °C/min, and was maintained for 5 min. Injection temperature was ensured at 250°C and helium flow rate 1 μL/min. Ionization voltage 70 eV, split mode as 50:1. The mass spectra range was set at 0–800 (m/z). Compound identification was carried out using the National Institute of Standards and Technology (NIST), version 2.0 reference library and literature data.
Liquid chromatography-time of flight-mass spectrometry analysis
The separation analysis was carried out using Thermo Scientific C18 reversed-phase column of Acclaim™ Polar Advantage II, 3 × 150 mm, 3 µm particle size on a Dionex Ultimate 3,000 ultra-high-performance liquid chromatography (UHPLC) system (Thermo Fisher Scientific, Waltham, MA, United States), maintained at 40°C. The injection volume was 3 µL. The mobile phase consisted of 0.1% formic acid in water (solvent A) and High purity acetonitrile (solvent B), at a flow rate of 0.4 ml/min for a total run time of 22 min. The gradient elution applied was: 5% A (0–3 min); 80% B (3–10 min); 80% B (10–15 min) and 5% B (15–22 min). The extract was analyzed by reverse phase LC-MS for their metabolites contents. The mass spectrometry (MS) analysis was carried out using MicrOTOF-Q III (Bruker Daltonik GmbH, Bremen, Germany) in the electron spray (ESI) negative mode; capillary voltage of 4000 V, nebulizer pressure: 2.0 bar; drying gas: 8 L/min at 300°C. The data acquisition was performed using Compass Data Analysis software version 4.3 and the mass range was set at the mass-to-charge ratio 50–1,000 m/z. The metabolites were identified based on accurate mass and MS/MS fragments by searching MetFrag (https://msbi.ipb-halle.de/MetFragBeta/, accessed 18 January 2022) and PubMed (https://pubmed.ncbi.nlm.nih.gov/, accessed on 18 January 2022) an online public-databases. In addition, previously published literatures were utilized in comparing fragment pattern with their findings. The secondary metabolites in the leaves of O. indicum were analyzed by untargeted analysis.
Wound healing properties of the leaves extracts of oroxylum indicum
In vitro scratch wound-healing assay
HaCaT cells were acquired from the Virology Laboratory, Faculty of Veterinary Medicine, Universiti Malaysia Kelantan. The cell lines were thawed and well maintained. The cells were cultured and maintained in high glucose Dulbecco’s Modified Eagle Medium (DMEM) premixed with 10% fetal bovine serum and antibiotics (Streptomycin 100 μg/ml and Penicillin 100 U/Ml) in a humidified 5% CO2 incubator at 37°C.
The migration rates of HaCaT cells were assessed by the scratch assay method. The cell density of “2 × 105 cells” were spread into each well of a 24-well plate and incubated with complete medium at 37°C and 5% CO2. After 24 h of incubation, the monolayer confluent cells were horizontally scratch with sterile P200 pipette tips. Then, the debris was removed by washing with Phosphate-buffered saline (PBS). After that, the cells were treated with ethanolic and aqueous extracts of O. indicum leaf with various concentration (12.5 μg/ml, 25 μg/ml, and 50 μg/ml) by diluting with serum-free Dulbecco’s modified Eagle’s medium (DMEM). The cells without treatment and treatment with allantoin (Sigma Aldrich, Germany) (50 μg/ml) was used as the control and positive control, respectively. Then, the scratch induced that represented wound was photographed at 0 h by using phase-contrast microscopy at ×40 magnification at 0 h before incubated with the ethanolic and aqueous extracts of O. indicum leaf. After 24 h of incubation, the second set of images were photographed. The images were analyzed and measured using “ImageJ” software to determine the wound closure and compared with the values obtained at 0 h. An increase in the percentage of wound closure indicated the migration of cells. Experiments were performed in a triplicate manner.
Experimental animals
A total of twenty male rats at 8 weeks old age were obtained from Animal Research and Service Centre, Universiti Sains Malaysia (ARASC USM), Health Campus, Kubang Kerian, Kelantan. These animals were randomly selected and caged individually within a polycarbonate cage. All the rats were kept within a standard laboratory condition with a temperature (24 ± 2°C), relative humidity (45 ± 5%) and with a lightning control of 12 h daylight and 12 h dark cycle. Food and water were given ad libitum. The bedding used were wood shaving and changed daily. All rats undergo acclimatization for five days prior to the experiment is started.
In vivo excisional wound model
The study was conducted with the approval from Institutional Animal Care and Use Committee (IACUC) of Faculty of Veterinary Medicine, Universiti Malaysia Kelantan (IACUC number: UMK/FPV/ACUE/FYP/14/2021). The wound was inflicted in all rats at the initial of the experiment (day 0). All the rats were anaesthetized using a combination of xylazine hydrochloride and ketamine with the dosage of 5 and 30 mg/kg respectively through intramuscular injection prior to wound excision. Skin preparation was conducted in an aseptic manner. A full thickness of the squared-shape excision wound with 2.5 cm long and width was excised with the use of toothed forceps and pointed scissor (Thakur et al., 2011).
A total of 20 rats were randomly assigned into 5 groups of 4 rats in each group. The extract at different concentrations (1%, 3% and 5% w/w) was applied topically on the excisional wound of rats at group 1, group 2 and group 3. The positive control group which group 4 received Sudocrem cream while no treatment was given to the negative control group (Group 5). Wound size was measured at days 0, 4, 8, and 14 post-wounding. The initial wound size (day 0) was measured at 3 h post-infliction. The wound size (2 mm) was determined using a calliper. Wound contraction, which contributes to wound closure, was expressed as a reduction in the percentage of the original wound size from the initial day of the experiment until the day of complete wound closure. It is used to calculate the degree of wound healing (Thakur et al., 2011). In each day interval, the wound size was considered as a non-healed area, which was subtracted from the initial wound size to obtain healed area. The rate of wound contraction was determined using the formula; Rate of wound contraction (%): Healed area/Initial wound size x 100%.
Statistical analysis
The data were analyzed by one-way ANOVA to identify significant differences among the treatment/control groups (p < 0.05) using SPSS v. 21.0 statistical software program. All data were presented as mean ± standard deviation.
Results
Total phenolic and flavonoid content in O. indicum extracts
The total phenolic content for aqueous and ethanol leaves extracts of O. indicum were estimated by Folin Ciocalteu’s method using gallic acid as standard. A linear calibration curve of standard gallic acid with r2 value of 0.999 was obtained. The total phenolic content of the leaves extracts were measured using GAE equation of y = 0.0035x - 0.0369. Among the two solvents extract, the ethanol (95%) leaf extract had the higher TPC (164.9 ± 0.1 mg GAE/g) compared with the aqueous leaves extract (30.1 ± 0.1 mg GAE/g) (Figure 1A).
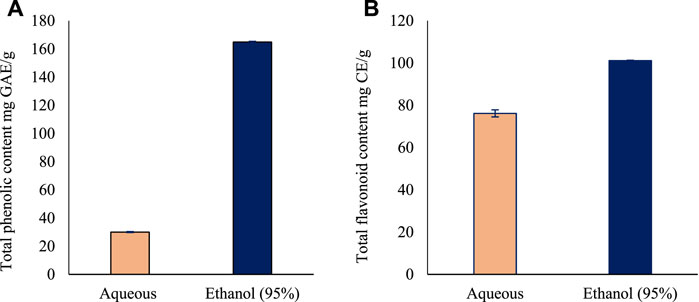
FIGURE 1. The total phenolic and flavonoid content of the leaves extracts of Oroxylum indicum. (A) Total phenolic content in Oroxylum indicum leaf aqueous and ethanolic extracts. (B) Total flavonoid content in Oroxylum indicum leaf aqueous and ethanolic extracts. Results are mean ± SD (n = 3).
The total flavonoid content for aqueous and ethanol leaves extracts of O. indicum were estimated by aluminum chloride colorimetric method using catechin as standard flavonoid compound. A linear calibration curve of standard gallic acid with r2 value of 0.9825 was obtained. The total flavonoid content of the leaves extracts were measured using GAE equation of y = 0.0006x—0.0197. Among the two solvents extract, the ethanol (95%) leaves extract had the higher TFC (101.2 ± 1.6 mg Catechin equivalents/g) followed by the aqueous leaf extract (76.2 ± 0.2 mg Catechin equivalents/g) (Figure 1B).
GC-MS analysis of the ethanolic leaf extract of Oroxylum indicum
Untargeted GC-MS analysis was performed to analyze and tentatively annotate the extracted metabolites. The GC-MS analysis of an ethanolic extract of the O. indicum showed the presence of several compounds. The GC-MS chromatograms of the ethanolic extract are shown in Figure 2. The retention time, name of the phytochemicals annotation, and other GC-MS profiles are presented in Table 1.
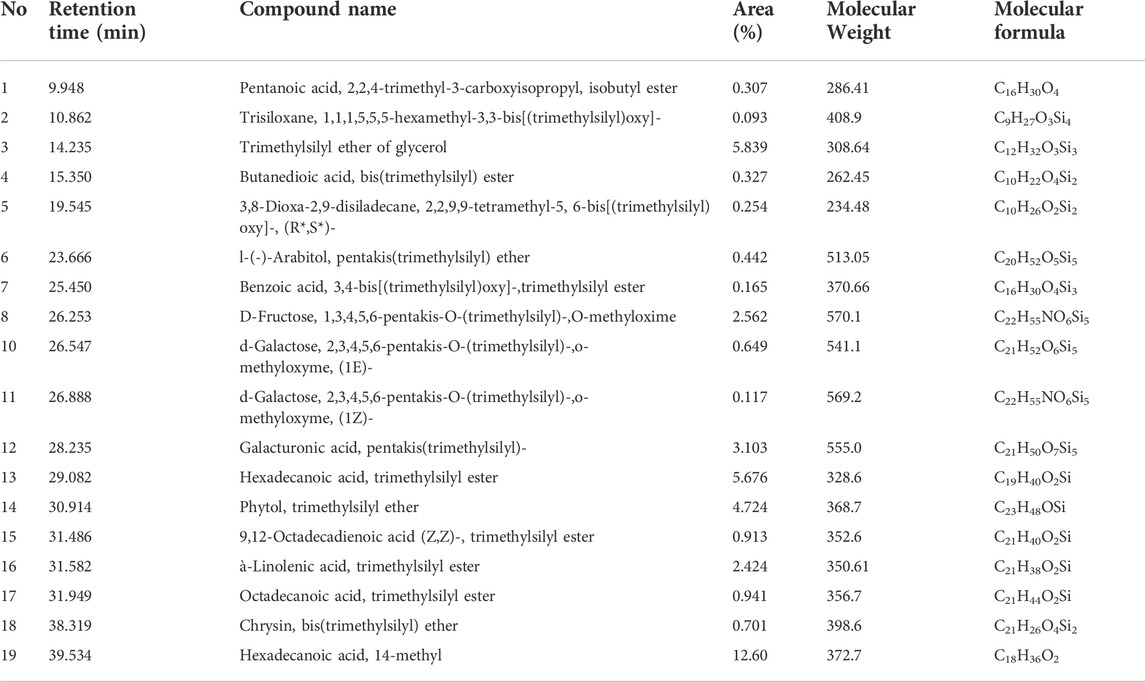
TABLE 1. GC-MS analysis revealed the presence of phytochemical components in ethanol leaf extract of Oroxylum indicum.
LC-MS/MS metabolic profile of the ethanolic leaf extract of Oroxylum indicum
The LC-TOF-MS/MS analysis results from O. indicum crude extract are shown in Table 2. Annotation was carried out based on the outcome of LC-TOF-MS/MS, the information from the online databases and fragmentation in the previous literature. A total of 12 compounds were detected and summarized along with their retention time, molecular ion and fragmentation data. The MS and MS/MS peak chromatograms and chemical structures present in the O. indicum are shown in Table 2.
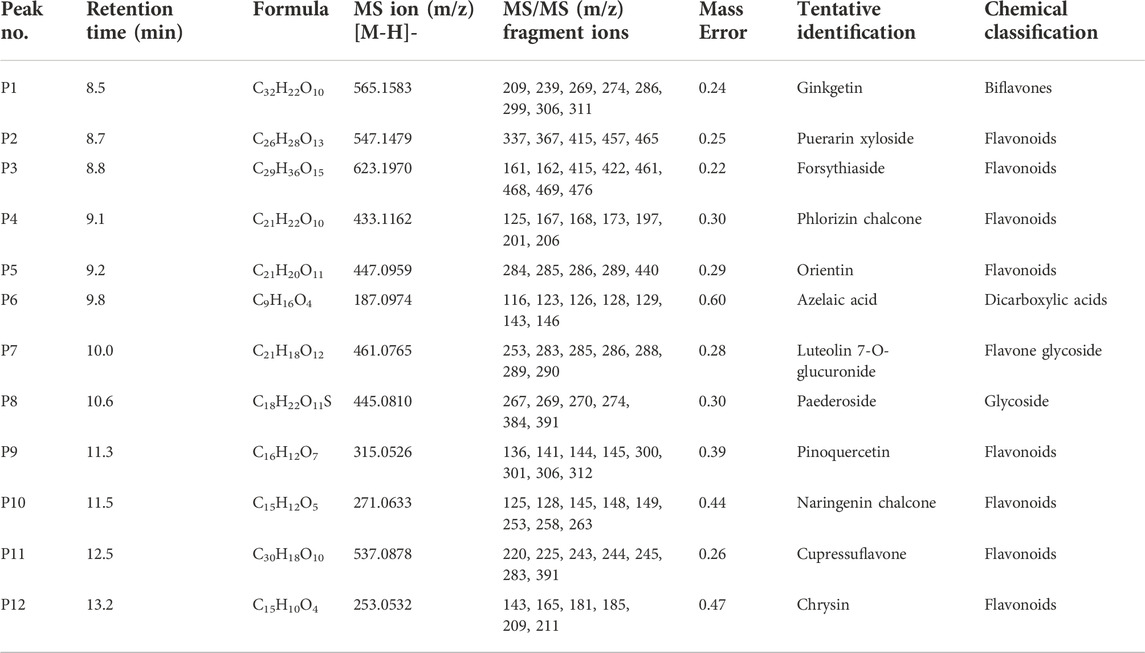
TABLE 2. LC-TOF-MS/MS data-negative ion mode for tentative compounds identification in Oroxylum indicum leaf extract.
According to Table 2, flavonoid account for a large proportion of the components detected in the extract of O. indicum. In this way, Peaks 1–5, 9, 10, 11, and 12 were tentatively identified as flavonoid compounds based on their MS/MS spectra, as shown in Table 2.
Peak 1 presented the [M-H]-ion m/z 565, and its MS/MS fragment ions at m/z 209, 239, 269, 274, 286, 299, 306, and 311 (Table 2). It was identified as Ginkgetin by comparing with those ions recorded in Metfarg. As shown in Table 2, Peak 2 assigned as Puerarin xyloside by comparing with literature reported previously and presented [M-H]—ion at m/z 547 and its typical MS/MS fragment ions at m/z 337, 367, 415, 457, and 465 by the continuous loss of water molecules (Lin et al., 2020). Furthermore, the fragmentation pathways were reported by Zhang and colleagues (Zhang et al., 2005). Peak 3 displayed the parent ion at m/z 623 [M-H]-and its MS/MS fragment ions at m/z 161, 162, 415, 422, 461, 468, 469, 469, and 476. The fragmentation pattern (typical product ions at m/z 461 and m/z 161) confirmed its annotated as Forsythiaside compound (Ye et al., 2010; Varga et al., 2019). Its chemical structure and fragment patterns is presented in Table 2. Peak 4 showed the parent [M-H] - ion m/z 433, and produced daughter ions at m/z 125, 167, 168, 173, 197, 201 and 206. The parent ion at m/z 433 [M-H]– was characterized as Phlorizin providing fragment patterns such as m/z 206 (aglycone), 201, 197, 173, 167 and 125 after a neutral loss of (227 Da) (Balázs et al., 2012). Phlorizin has several beneficial effects on human health. Peak 5 showed the [M-H]– ion at m/z 447 and the fragment ions at m/z 284, 285, 286, 289, and 440. The major fragment of m/z 286 was consistent with the fragmentation pattern of orientin (Balázs et al., 2012; Otify et al., 2015; Sulaiman and Balachandran, 2016).
For peak 6, the parent ion at m/z 187 was annotated as Azelaic acid as it presented major fragments with m/z 116, 123, 143, and 146. This finding agreed with previous reports (Schallreuter and Wood, 1990; Chen et al., 2015; Fialovaa et al., 2015; Zengin et al., 2018). The [M-H]– ion at m/z 461 and its fragments at m/z 253, 283, 285, 286, 288, 289, and 290 has been assigned as Luteolin 7-O-glucuronide (flavone glycoside compound) according to the reference literatures (Fialovaa et al., 2015; Ibrahim et al., 2015; Sulaiman and Balachandran, 2017). The peak with m/z 445 (Peak 8) presented three major fragments with m/z 274, 384, and 391 and it was tentatively assigned as Paederoside. The Peak 9 with m/z 315 showed product ions at m/z 136, 141, 306, and 312. The fragments suggest that the molecule is Pinoquercetin on the basis of online database information. Peak at m/z [M-H]– 271 (Peak 10) was proposed to be Naringenin chalcone as it displayed major fragments with m/z 125, 149, 253, and 258, 263. Peak 11 was tentatively deduced for the m/z 537 and its daughter fragments at m/z 220, 283, and 391 according to the reference literature (Shan et al., 2018; Zhuang et al., 2018). Peak 12 (m/z 253) with its product ions of m/z 143, 165, 185, and 211, was annotated as chrysin according to the reference literature (Chen et al., 2003; Rai et al., 2022).
Effect of O. indicum leaves extracts on scratch wound healing in HaCaT cells
The effect of ethanolic leaves extracts of O. indicum on the migration of keratinocytes (HaCat cells) was determined by the scratch assay method. Scratches were made in confluent HaCaT monolayers. The scratches were treated with various concentrations (12.5–50 μg/ml) of extracts. The progress of the closure of the scratches was recorded after 24 h and the movement of the cells were observed. The O. indicum extract stimulated the migration of keratinocytes into the denuded area and resulted in faster closure of wound area compared with controls groups (Figure 3). The migration rates of HaCat cells that were treated with 50 μg/ml and 25 μg/ml concentrations were significantly higher (p < 0.05) compared to the negative control group. In addition, the migration rate of the HaCat cells that were treated with 50 μg/ml was significantly higher than the positive control. The effect of a range of concentrations of ethanolic leaves extracts on migration rate of HaCat cells are shown in Figure 3.
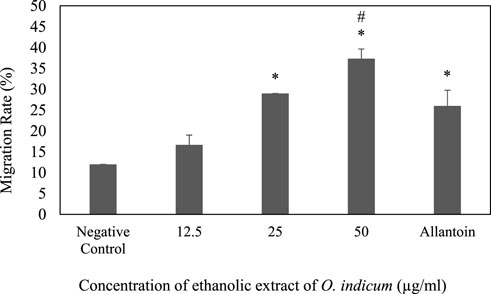
FIGURE 3. Effect of ethanolic extract of Oroxylum indicum on HaCat cell migration. Results are mean ± SD (n = 3). The symbol * denotes the significantly (p < 0.05) difference between the treated and negative controls. The symbol # denotes the values are significantly (p < 0.05) different from the positive control group.
The migration rates of the cells that were treated with 25 μg/ml and 12.5 μg/ml were not significantly (p < 0.05) different from positive control group. The present study indicates that the migration rate for the cell that was treated with the highest concentration of ethanolic extract (50 μg/ml) has highest migration rate compare to other concentrations. The migration of keratinocytes was seen to be more significant in the O. indicum extract-treated cells compared to vehicle-treated and allantoin-treated cells (Figure 4).
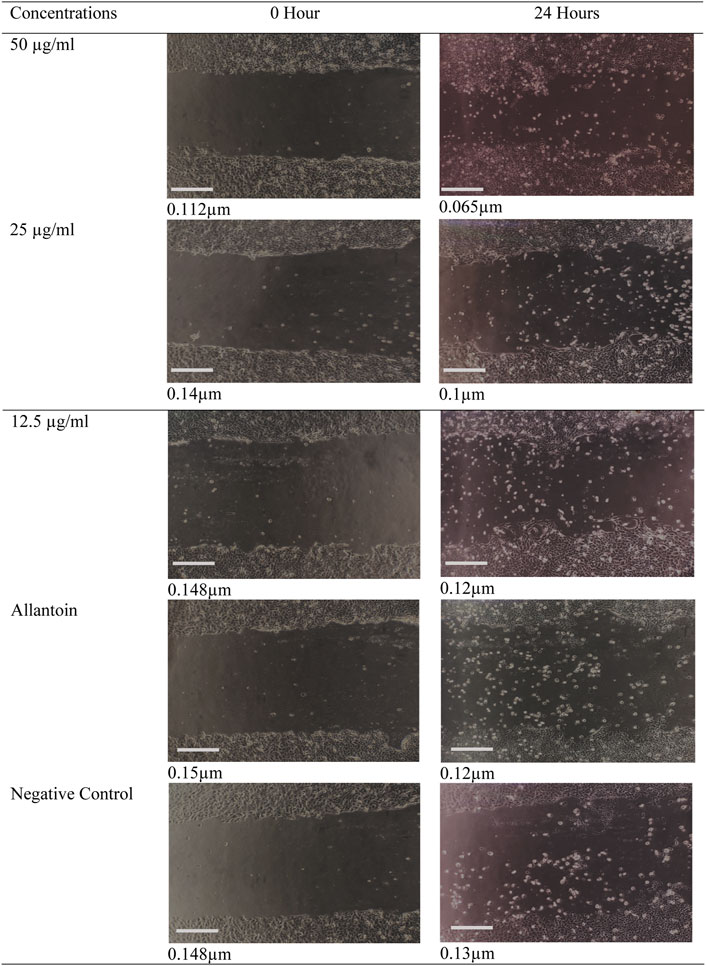
FIGURE 4. Digital image showing the effect of Oroxylum indicum leaves extracts on human keratinocyte cell migration in a scratch assay. Migrations of keratinocytes were photographed using light microscope fitted with digital camera with ×10 magnification. Bar = 1 cm.
The effect of ethanolic extract of Oroxylum indicum on in vivo excision wound model
The effect of O. indicum leaves extracts on excision wound model in albino rats is shown in Figure 5. A significant healing pattern with almost complete wound closure was observed in rats treated with different concentrations of the ethanol extract of O. indicum, whereas untreated animal groups lagged far behind. The increased rate of wound contraction lead to faster healing as confirmed by the increased healed area in the ethanolic extract-treated groups compared to the control ones (Figure 6).
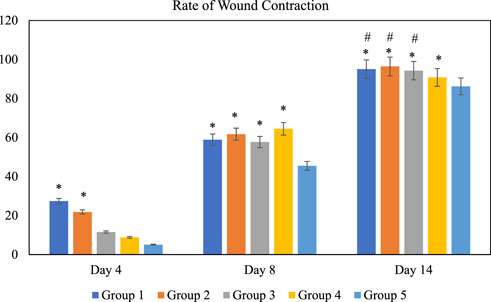
FIGURE 5. Rate of wound contraction for each group at different time intervals, days 4, 8, and 14 of the study. Notes: The symbol * denotes the values are significantly (p < 0.05) different between treated and negative control group and symbol # denotes the values are significantly (p < 0.05) different between treated and positive control group. Results are mean ± SD (n = 4) at (p < 0.05). Group 1: O. indicum (1%), Group 2: O. indicum (3%), Group 3; O. indicum (5%), Group 4: Positive control and Group 5: Negative control.
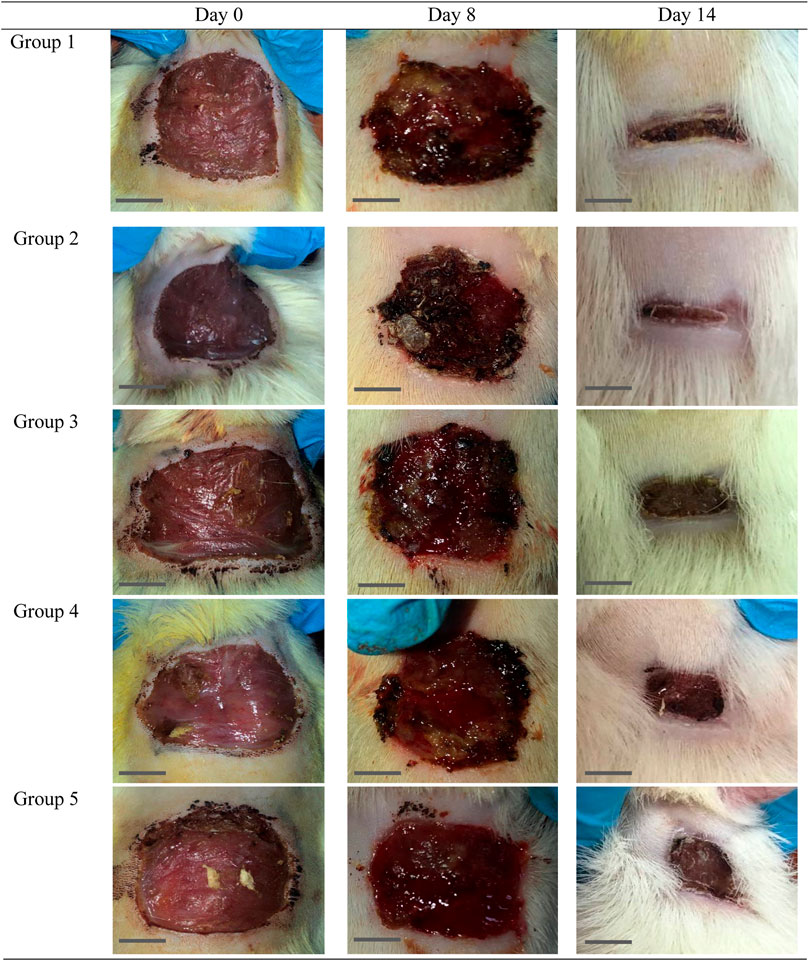
FIGURE 6. Gross morphology of the wound in the control and experimental groups. The wounds appeared dried and the scab was not uniformly on each wound started at day 4 of the study onwards. Bar = 1 cm.
Discussion
Oroxylum indicum has long been used as a medicinal and culinary herb in many Asian countries, including Malaysia. The aerial parts or roots of this plant are used in traditional medicine in Malaysia for treatment of wounds, diarrhea, digestive problems, and control high blood pressure (Reduan et al., 2021). Although O. indicum is widely used in traditional medicines, there has been limited scientific investigation into the biological effects in relation to wound healing activity. Therefore, we evaluated the phytochemical constituents and wound healing properties of aqueous and ethanol extracts of O. indicum using in vitro and in vivo models.
In the present study O. indicum was evaluated for determination of total phenolic and flavonoids contents. The quantification revealed the ethanolic leaves extract of O. indicum showed higher total phenolic (164.9 mg GAE/g) and flavonoids contents (101.2 mg CE/g). The extract with aqueous showed lower quantity of phenols (30.1 mg GAE/g) and flavonoids (76.2 mg CE/g) that showed the ethanol as suitable for preparation of plant extracts with high yield of phenols and flavonoids from O. indicum leaves. Based on rich quantity of phenols and flavonoids observed in the preliminary investigation, O. indicum ethanol extract was subjected to chemical profiling using GC-MS and LC–MS/MS techniques. These analytical techniques have very high sensitivity and resolution, and are suitable for detailed characterization of plant extracts (Heinrich et al., 2020; Heinrich et al., 2022).
The phytochemical analysis of O. indicum leaf extract by GC-MS showed the identification of total 19 compounds. Of them, seven compounds were identified as esters. The Pentanoic acid, 2,2,4-trimethyl-3-carboxyisopropyl, isobutyl ester, Benzoic acid, 3,4-bis[(trimethylsilyl)oxy]-,trimethylsilyl ester, Hexadecanoic acid, trimethylsilyl ester, 9,12-Octadecadienoic acid (Z,Z)-, trimethylsilyl ester, à-Linolenic acid, trimethylsilyl ester, Octadecanoic acid, trimethylsilyl ester and hexadecanoic acid, 14-methyl were detected (Table 1). Pentanoic acid, 2,2,4-trimethyl-3-carboxyisopropyl, isobutyl ester and hexadecanoic acid shows anti-tumor, analgesic, antimicrobial and antioxidants activities (Kumar et al., 2019; Beschi et al., 2021; Madhavan et al., 2021). The following sugars, D-Fructose, 1,3,4,5,6-pentakis-O-(trimethylsilyl)-,O-methyloxime, d-Galactose, 2,3,4,5,6-pentakis-O-(trimethylsilyl)-,o-methyloxyme, (1E)-, Galacturonic acid, pentakis(trimethylsilyl)- and d-Galactose, 2,3,4,5,6-pentakis-O-(trimethylsilyl)-,o-methyloxyme, (1Z)- were detected. Three ether compounds such as Trimethylsilyl ether of glycerol, l-(-)-Arabitol, pentakis(trimethylsilyl) ether and Phytol, trimethylsilyl ether were also detected. Chrysin, bis(trimethylsilyl) ether, a flavone, was also detected in the GC–MS. Chrysin has been reported by others from different parts of O. indicum (Kumar et al., 2019). Chrysin, bis(trimethylsilyl) ether is a natural flavonoid that has been reported to have numerous biological activities such as hepatoprotective and antioxidant (Pushpavalli et al., 2010), cytoprotective and anti-inflammatory (Sulaiman et al., 2014; Deldar et al., 2018; Mani and Natesan, 2018), and cardiovascular protective effects (Farkhondeh et al., 2019).
The LC-TOF-MS/MS profile of O. indicum leaves extract revealed 12 major compounds. Among the identified compounds the number of flavonoids were in abundance. The second highest class of secondary metabolites were glycosides compounds followed by dicarboxylic acids. The current analysis showed the presence of number of phytochemicals which are reported with beneficial biological effects in previous studies. Compounds Orientin, Chrysin, Pinoquercetin, Cupressuflavone, Puerarin xyloside, Forsythiaside and Paederoside have been widely known to have biological activities such as antioxidant, antiviral, antibacterial, anti-inflammatory, and reduced oxidative stress and cardio protective effects (Qiufeng et al., 2004; Praveena et al., 2014; Zhao et al., 2018; Khalil et al., 2022). Chrysin, a natural flavonoid, has also a wide variety of biological activities such as anti-cancer (Ryu et al., 2017), wound-healing (Ansor, 2021; Kaparekar et al., 2021), anti-Inflammatory, and antioxidant activity (Beschi et al., 2021). Other compounds detected in the extract such as Pinoquercetin and Cupressuflavone have been reported to have an antioxidant property, anti-inflammatory, analgesic, and anti-bacteria activities (Quang et al., 2008; Al-Sayed et al., 2018). Together, the LC-TOF-MS/MS analysis of the O. indicum leaves extracts revealed important phytochemicals and this plant could be considered as good source of natural compounds.
Wound healing is a mechanism-based complex process of restoring cellular structures and tissue layers. The wound healing process involve different phases including contraction, the formation of epithelialization and fibrosis. The biological response regulating the body’s own cellular defense mechanisms contributes to the wound and its repair (Morales-González et al., 2022). In the present study, the wound healing effects of ethanolic extract of O. indicum were assessed in both in vitro and in vivo models.
The effects of the O. indicum extracts on wound closure were evaluated by in vitro wound scratch assay through observation of the migration rate of the HaCat cells (i.e. diameter of the wound area). The result of this study shows that the migration rate of cells that were treated with O. indicum extracts showed better migration rate compared with control. Furthermore, the wound closer time of extract-treated cells were faster when compared with other groups. Therefore, the in vitro wound-healing potential of O. indicum can be attributed to the contributions of bioactive compounds in the plant extract such as flavonoids, glycosides, alkaloids, and saponins (Praveena et al., 2014; Tran et al., 2015). These phytochemicals are usually responsible for the biological activity of medicinal plants.
The O. indicum extracts were further evaluated for its wound healing activity using excisional wound model (in vivo). The results of this study revealed that topical application of the ethanolic extract of O. indicum significantly (p < 0.05) increased wound healing effects by increasing the rate of wound contraction compared with control groups. Among the extract-treated groups, the highest contraction was observed in Group 2 (3% of the extract), whereas untreated animal showed lower contraction rate. The O. indicum extracts facilitated wound contraction significantly at all dose levels from 8th day to 14th day as compared to negative control. The phytochemical compounds present in the plant extract may contribute the wound-healing effect by eliminating infection and thus allowing initiation of natural tissue repair processes. These findings are in line with the study conducted by Samarath and Panda (2018) which reported that ethanolic extract has the highest rate of wound contraction in the excision wound and the existence of phytoconstituents such as flavonoids, saponins, phenols, and tannins, either alone or in combination, can have a synergistic impact on the wound healing process. Besides that, wound contraction also works as an indicator of active re-epithelialization, granulation, angiogenesis, fibroblast proliferation, keratinocyte differentiation, and proliferation activities occurred in the wound bed (Boakye et al., 2018). In addition, wounds of the rats in extract-treated groups showed more uniform scabs formation compared to positive and negative controls groups. The scab formation in extract-treated groups formed as early as day two of treatment compared to positive and negative groups. This indicated the presence of active blood clot formation and hemostasis occurred and faster in extract-treated groups.
Throughout the study the extract-treated groups showed no sign of pus formation which indicate there were no bacterial contamination or infection. These findings suggested that the extract may have antimicrobial activity, which fastens the inflammatory phase, and prepared the wound to enter the healing phase. The enhanced wound healing potency of various herbal extracts could be due to the antimicrobial and free radical-scavenging properties of the phytoconstituents present in the extract, and the faster wound healing process could be a individual or synergistic effects of bioactive molecules (Thakur et al., 2011). In our study, higher wound healing properties as exhibited by O. indicum could be due to its high content of phenolics and flavonoids in both the studied models (in vitro and in vivo).
Conclusion
The present study demonstrated that O. indicum leaves extracts contains important phytochemicals. This study also demonstrated that animals treated with ethanol extract of O. indicum exhibited significant wound healing effects (in vitro and in vivo) and its healing potential may probably be as a result of the presence of a mixture of phytochemicals. Thus from this study it can be suggested that the ethanolic extract of O. indicum has wound healing potential and thereby it could be used as a natural wound-healing agent. Future research warrants identification of the individual compounds present in the O. indicum leaves responsible for its wound healing property and subsequent understanding of their mechanisms of bioactivities.
Data availability statement
The original contributions presented in the study are included in the article/supplementary material, further inquiries can be directed to the corresponding authors.
Ethics statement
The animal study was reviewed and approved by Institutional Animal Care and Use Committee (IACUC) of Faculty of Veterinary Medicine, Universiti Malaysia Kelantan (IACUC number: UMK/FPV/ACUE/FYP/14/2021). Written informed consent was obtained from the owners for the participation of their animals in this study.
Author contributions
Conceptualization; MH methodology; MH and FA; laboratory analysis; FA, AH, and IM; Investigation; MH; data curation; FA and MH; writing—original draft preparation; FA and MH; writing—review and editing; FA, MH, IA, FA, AM, ZK, LW, MR, and KG; supervision, MH. All authors have read and agreed to the published version of the manuscript.
Funding
This research was funded by the Universiti Malaysia Kelantan (UMK) under UMK-Rising Star Scheme (Grant code: R/STA/A0600/01391A/003/2021/00936). The Article Processing Charges (APC) was supported by the Universiti Malaysia Kelantan, Universiti Sains Malaysia and INTI International University.
Acknowledgments
The authors would like to acknowledge the Universiti Malaysia Kelantan for the financial support of this research. We would also like to Universiti Malaysia Kelantan, Universiti Sains Malaysia and INTI International University for supporting APC. We would also like to thank Institute of Systems Biology (INBIOSIS), UKM, for providing GC-MS and LC-MS service.
Conflict of interest
The authors declare that the research was conducted in the absence of any commercial or financial relationships that could be construed as a potential conflict of interest.
Publisher’s note
All claims expressed in this article are solely those of the authors and do not necessarily represent those of their affiliated organizations, or those of the publisher, the editors and the reviewers. Any product that may be evaluated in this article, or claim that may be made by its manufacturer, is not guaranteed or endorsed by the publisher.
Abbreviations
CE, Catechin equivalents; HaCaT, Human keratinocyte cells; IACUC, Institutional Animal Care and Use Committee; GAE, Gallic acid equivalents; GC-MS, Gas chromatography–mass spectrometry; LC-MS/MS, Liquid chromatography–mass spectrometry; NIST, National Institute of Standards and Technology; SD, Standard deviation; TFC, Total flavonoids content; TPC, Total phenolic content; UHPLC, Ultra-high-performance liquid chromatography.
References
Abdulhafiz, F., Mohammed, A., Kayat, F., Bhaskar, M., Hamzah, Z., Podapati, S. K., et al. (2020a). Xanthine oxidase inhibitory activity, chemical composition, antioxidant properties and GC-MS Analysis of Keladi Candik (Alocasia longiloba Miq). Molecules 25 (11), 2658. doi:10.3390/molecules25112658
Abdulhafiz, F., Mohammed, A., Kayat, F., Zakaria, S., Hamzah, Z., Reddy Pamuru, R., et al. (2020b). Micropropagation of Alocasia longiloba Miq and comparative antioxidant properties of ethanolic extracts of the field-grown plant, in vitro propagated and in vitro-derived callus. Plants 9 (7), 816. doi:10.3390/plants9070816
Abdulhafiz, F., Reduan, M. F. H., Hamzah, Z., Kari, Z. A., Dawood, M. A., and Mohammed, A. (2022). Acute oral toxicity assessment and anti-hyperuricemic activity of Alocasia longiloba extracts on Sprague-Dawley rats. Saudi J. Biol. Sci. 29 (5), 3184–3193. doi:10.1016/j.sjbs.2022.01.050
Abubakar, A. R., and Haque, M. (2020). Preparation of medicinal plants: Basic extraction and fractionation procedures for experimental purposes. J. Pharm. Bioallied Sci. 12 (1), 1–10. doi:10.4103/jpbs.JPBS_175_19
Alaerts, G., Dejaegher, B., Smeyers-Verbeke, J., and Vander Heyden, Y. (2010). Recent developments in chromatographic fingerprints from herbal products: Set-up and data analysis. Comb. Chem. High. Throughput Screen. 13 (10), 900–922. doi:10.2174/138620710793360284
Alsamri, H., Athamneh, K., Pintus, G., Eid, A. H., and Iratni, R. (2021). Pharmacological and antioxidant activities of Rhus coriaria L. (Sumac). Antioxidants 10 (1), 73. doi:10.3390/antiox10010073
Al‒Sayed, E., Gad, H. A., El‒Shazly, M., Abdel‒Daim, M. M., and Nasser Singab, A. (2018). Anti‒inflammatory and analgesic activities of cupressuflavone from Cupressus macrocarpa: Impact on pro‒inflammatory mediators. Drug Dev. Res. 79 (1), 22–28.
Ansor, N. M. (2021). Wound healing properties of phytoestrogens: In vitro and in vivo studies. Mal. J. Med. Health Sci. 17 (9), 76–84.
Asadi, M. R., Torkaman, G., Hedayati, M., and Mofid, M. (2013). Role of sensory and motor intensity of electrical stimulation on fibroblastic growth factor-2 expression, inflammation, vascularization, and mechanical strength of full-thickness wounds. J. Rehabil. Res. Dev. 50 (4), 489–498. doi:10.1682/jrrd.2012.04.0074
Bailly, C. (2021). The traditional and modern uses of Selaginella tamariscina (P. Beauv.) Spring, in medicine and cosmetic: Applications and bioactive ingredients. J. Ethnopharmacol. 280, 114444. doi:10.1016/j.jep.2021.114444
Balázs, A., Tóth, M., Blazics, B., Héthelyi, É., Szarka, S., Ficsor, E., et al. (2012). Investigation of dietary important components in selected red fleshed apples by GC–MS and LC–MS. Fitoterapia 83 (8), 1356–1363. doi:10.1016/j.fitote.2012.04.017
Beschi, D. A., Appavoo, M. R., and Wilsy, J. I. (2021). GC-MS analysis, collected from kavalkinaru area, tirunelveli district, Tamil nadu, India. Eur. J. Mol. Clin. Med. 8 (11), 2021.
Boakye, Y. D., Agyare, C., Ayande, G. P., Titiloye, N., Asiamah, E. A., and Danquah, K. O. (2018). Assessment of wound-healing properties of medicinal plants: The case of Phyllanthus muellerianus. Front. Pharmacol. 9, 945. doi:10.3389/fphar.2018.00945
Chen, G., Li, X., Saleri, F., and Guo, M. (2016). Analysis of flavonoids in Rhamnus davurica and its antiproliferative activities. Molecules 21 (10), 1275. doi:10.3390/molecules21101275
Chen, L. J., Games, D. E., and Jones, J. (2003). Isolation and identification of four flavonoid constituents from the seeds of Oroxylum indicum by high-speed counter-current chromatography. J. Chromatogr. A 988 (1), 95–105. doi:10.1016/s0021-9673(02)01954-4
Chen, W. C., Tseng, T. S., Hsiao, N. W., Lin, Y. L., Wen, Z. H., Tsai, C. C., et al. (2015). Discovery of highly potent tyrosinase inhibitor, T1, with significant anti-melanogenesis ability by zebrafish in vivo assay and computational molecular modeling. Sci. Rep. 5 (1), 7995–7998. doi:10.1038/srep07995
Deldar, Y., Pilehvar-Soltanahmadi, Y., Dadashpour, M., Montazer Saheb, S., Rahmati-Yamchi, M., and Zarghami, N. (2018). An in vitro examination of the antioxidant, cytoprotective and anti-inflammatory properties of chrysin-loaded nanofibrous mats for potential wound healing applications. Artif. Cells Nanomed. Biotechnol. 46 (4), 706–716. doi:10.1080/21691401.2017.1337022
Ekor, M. (2014). The growing use of herbal medicines: Issues relating to adverse reactions and challenges in monitoring safety. Front. Pharmacol. 4, 177. doi:10.3389/fphar.2013.00177
Farkhondeh, T., Samarghandian, S., and Bafandeh, F. (2019). The cardiovascular protective effects of chrysin: A narrative review on experimental researches. Cardiovasc. Hematol. Agents Med. Chem. 17 (1), 17–27. doi:10.2174/1871525717666190114145137
Fattahi, S., Zabihi, E., Abedian, Z., Pourbagher, R., Ardekani, A. M., Mostafazadeh, A., et al. (2014). Total phenolic and flavonoid contents of aqueous extract of stinging nettle and in vitro antiproliferative effect on hela and BT-474 Cell lines. Int. J. Mol. Cell Med. 3 (2), 102.
Fialovaa, S., Veizerova, L., Nosalova, V., Drabikova, K., Tekelova, D., Grancai, D., et al. (2015). Water extract of mentha x villosa: Phenolic fingerprint and effect on ischemia-reperfusion injury. Nat. Prod. Commun. 10 (6), 937–940.
Goodarzi, M., Russell, P. J., and Vander Heyden, Y. (2013). Similarity analyses of chromatographic herbal fingerprints: A review. Anal. Chim. Acta 804, 16–28. doi:10.1016/j.aca.2013.09.017
Gowda, G. A., and Djukovic, D. (2014). Overview of mass spectrometry-based metabolomics: Opportunities and challenges. Methods Mol. Biol. 1198, 3–12. doi:10.1007/978-1-4939-1258-2_1
Gurib-Fakim, A. (2014). Novel plant bioresources: Applications in food, medicine and cosmetics. West Sussex, United Kingdom: John Wiley & Sons, 395–408. 978-1-118-46061-0.
Heinrich, M., Appendino, G., Efferth, T., Fürst, R., Izzo, A. A., Kayser, O., et al. (2020). Best practice in research–overcoming common challenges in phytopharmacological research. J. Ethnopharmacol. 246, 112230. doi:10.1016/j.jep.2019.112230
Heinrich, M., Jalil, B., Abdel-Tawab, M., Echeverria, J., Kulić, Ž., McGaw, L. J., et al. (2022). Best practice in the chemical characterisation of extracts used in pharmacological and toxicological research—the ConPhyMP—Guidelines. Front. Pharmacol. 13, 953205. doi:10.3389/fphar.2022.953205
Hengpratom, T., Ngernsoungnern, A., Ngernsoungnern, P., Lowe, G. M., and Eumkeb, G. (2020). Antiadipogenesis of Oroxylum indicum (L.) Kurz extract via PPARγ2 in 3T3-L1 adipocytes. London, United Kingdom: Evid. Based Compleme.
Ibrahim, R. M., El-Halawany, A. M., Saleh, D. O., Naggar, E. M. B. E., El-Shabrawy, A. E. R. O., and El-Hawary, S. S. (2015). HPLC-DAD-MS/MS profiling of phenolics from Securigera securidaca flowers and its anti-hyperglycemic and anti-hyperlipidemic activities. Rev. Bras. Farmacogn. 25 (2), 134–141. doi:10.1016/j.bjp.2015.02.008
Kaparekar, P. S., Poddar, N., and Anandasadagopan, S. K. (2021). Fabrication and characterization of Chrysin–a plant polyphenol loaded alginate-chitosan composite for wound healing application. Colloids Surf. B Biointerfaces 206, 111922. doi:10.1016/j.colsurfb.2021.111922
Khalil, H. E., Ibrahim, H. I. M., Ahmed, E. A., Emeka, P. M., and Alhaider, I. A. (2022). Orientin, a bio-flavonoid from trigonella hamosa L., regulates COX-2/PGE-2 in A549 cell lines via miR-26b and miR-146a. Pharmaceuticals 15 (2), 154. doi:10.3390/ph15020154
Khanal, A., Devkota, H. P., Kaundinnyayana, S., Gyawali, P., Ananda, R., and Adhikari, R. (2021). Culinary herbs and spices in Nepal: A review of their traditional uses, chemical constituents, and pharmacological activities. Ethnobot. Res. App. 21, 1–18. doi:10.32859/era.21.40.1-18
Kharbach, M., Marmouzi, I., El Jemli, M., Bouklouze, A., and Vander Heyden, Y. (2020). Recent advances in untargeted and targeted approaches applied in herbal-extracts and essential-oils fingerprinting-A review. J. Pharm. Biomed. Anal. 177, 112849. doi:10.1016/j.jpba.2019.112849
Kumar, R. S., Anburaj, G., Subramanian, A., Vasantha, S., and Selvam, A. P. (2019). Preliminary phytochemical investigation, Antimicrobial activity and GC-MS analysis of leaf extract of Capparis zeylanica Linn. J. Pharm. Phytochem. 8, 1399–1405.
Lei, Z., Huhman, D. V., and Sumner, L. W. (2011). Mass spectrometry strategies in metabolomics. J. Biol. Chem. 286 (29), 25435–25442. doi:10.1074/jbc.R111.238691
Lin, P., Wang, Q., Liu, Y., Qin, Z., Gao, H., Ye, M., et al. (2020). Characterization of chemical profile and quantification of representative components of DanLou tablet, a traditional Chinese medicine prescription, by UHPLC-Q/TOF-MS combined with UHPLC-TQ-MS. J. Pharm. Biomed. Anal. 180, 113070. doi:10.1016/j.jpba.2019.113070
Madhavan Azhagu, S., Sa, V., Ra, S., and Sb, R. (2021). Phytochemical Screening and GC‒MS Analysis Of Bioactive Compounds Present In Ethanolic Leaf Extract Murraya koenigii. Bull. Env. Pharmacol. Life Sci. 10, 158–164.
Maher, T., Ahmad Raus, R., Daddiouaissa, D., Ahmad, F., Adzhar, N. S., Latif, E. S., et al. (2021a). Medicinal plants with anti-leukemic effects: A review. Molecules 26 (9), 2741. doi:10.3390/molecules26092741
Maher, T., Kabbashi, N. A., Mirghani, M. E., Alam, M. Z., Daddiouaissa, D., Abdulhafiz, F., et al. (2021b). Optimization of ultrasound-assisted extraction of bioactive compounds from Acacia seyal gum using response surface methodology and their chemical content identification by Raman, FTIR, and GC-TOFMS. Antioxidants 10 (10), 1612. doi:10.3390/antiox10101612
Mani, R., and Natesan, V. (2018). Chrysin: Sources, beneficial pharmacological activities, and molecular mechanism of action. Phytochemistry 145, 187–196. doi:10.1016/j.phytochem.2017.09.016
Maqsood, S., Adiamo, O., Ahmad, M., and Mudgil, P. (2020). Bioactive compounds from date fruit and seed as potential nutraceutical and functional food ingredients. Food Chem. 308, 125522. doi:10.1016/j.foodchem.2019.125522
Metz, T. O., Zhang, Q., Page, J. S., Shen, Y., Callister, S. J., Jacobs, J. M., et al. (2007). The future of liquid chromatography-mass spectrometry (LC-MS) in metabolic profiling and metabolomic studies for biomarker discovery. Biomark. Med. 1 (1), 159–185. doi:10.2217/17520363.1.1.159
Morales-González, M., Díaz, L. E., Dominguez-Paz, C., and Valero, M. F. (2022). Insights into the design of polyurethane dressings suitable for the stages of skin wound-healing: A systematic review. Polymers 14 (15), 2990. doi:10.3390/polym14152990
Otify, A., George, C., Elsayed, A., and Farag, M. A. (2015). Mechanistic evidence of Passiflora edulis (Passifloraceae) anxiolytic activity in relation to its metabolite fingerprint as revealed via LC-MS and chemometrics. Food Funct. 6 (12), 3807–3817. doi:10.1039/c5fo00875a
Praveena, R., Sadasivam, K., Deepha, V., and Sivakumar, R. (2014). Antioxidant potential of orientin: A combined experimental and DFT approach. J. Mol. Struct. 1061, 114–123. doi:10.1016/j.molstruc.2014.01.002
Pushpavalli, G., Kalaiarasi, P., Veeramani, C., and Pugalendi, K. V. (2010). Effect of chrysin on hepatoprotective and antioxidant status in D-galactosamine-induced hepatitis in rats. Eur. J. Pharmacol. 631 (1-3), 36–41. doi:10.1016/j.ejphar.2009.12.031
Qiufeng, L., Shunqing, F., Yingzhou, C., Yiting, Y., and Lingyun, W. (2004). Study on the antibacterial and antiviral activity compositions of Trollium chinensis Bunge. J. Zhejiang Univ. Sci. 31 (4), 412–415.
Quang, T. H., Cuong, N. X., Van Minh, C., and Van Kiem, P. (2008). New flavonoids from Baeckea frutescens and their antioxidant activity. Nat. Prod. Commun. 3 (5), 1934578X0800300. doi:10.1177/1934578x0800300515
Rahman, M., Satyajit, D., and Sarker, L. N. (2018). “Application of computational methods in isolation of plant secondary metabolites,” in Computational phytochemistry (Amsterdam, Netherlands: Elsevier), 107–139. doi:10.1016/B978-0-12-812364-5.00004-3
Rai, D., Ram, H. A., Patel, K. N., Babu, U. V., Kumar, L. S., and Kannan, R. (2022). In vitro immuno-stimulatory and anticancer activities of Oroxylum indicum (L.) Kurz.: An evidence for substitution of aerial parts for conservation. J. Ayurveda Integr. Med. 13B (2), 100523. doi:10.1016/j.jaim.2021.09.001
Rainville, P. D., Theodoridis, G., Plumb, R. S., and Wilson, I. D. (2014). Advances in liquid chromatography coupled to mass spectrometry for metabolic phenotyping. TrAC Trends Anal. Chem. 61, 181–191. doi:10.1016/j.trac.2014.06.005
Reduan, M. F. H., Hamid, F. F. A., Nordin, M. L., Shaari, R., Hamdan, R. H., Chung, E. L. T., et al. (2020). Acute oral toxicity study of ethanol extract of Oroxylum indicum leaf in mice. Thai J. Vet. Med. 50, 573–581.
Reduan, M. F. H., Mastika, M. R. A., Hamid, F. A., Noralidin, N., Abd Manaf, N. A., Shaari, R., et al. (2021). Sub-acute oral toxicity study of ethanol extract of Oroxylum indicum leafin C57bl/6 mice. Biomed. Pharmacol. J. 14 (3), 1579–1586. doi:10.13005/bpj/2260
Ryu, S., Lim, W., Bazer, F. W., and Song, G. (2017). Chrysin induces death of prostate cancer cells by inducing ROS and ER stress. J. Cell. Physiol. 232 (12), 3786–3797. doi:10.1002/jcp.25861
Sachdeva, H., Khaturia, S., Chahar, M., Behera, K. K., Bist, R., Mohanty, S., et al. (2022). “Nutraceuticals as therapeutic agents for prevention and treatment of diseases,” in Prebiotics, probiotics and nutraceuticals (Singapore, Singapore: Springer). 45-68978-981-16-8990-1.
Saini, R. K., Sivanesan, I., and Keum, Y. S. (2016). Phytochemicals of moringa oleifera: A review of their nutritional, therapeutic and industrial significance. 3 Biotech. 6 (2), 203–214. doi:10.1007/s13205-016-0526-3
Samarath, B. K., and Panda, S. K. (2018). Phytochemical study and evaluation of wound healing potential of crude leaves extracts of Oroxylum indicum (l.) Vent in wistar rats. Chem. Anal. 15, 16.
Schallreuter, K. U., and Wood, J. W. (1990). A possible mechanism of action for azelaic acid in the human epidermis. Arch. Dermatol. Res. 282 (3), 168–171. doi:10.1007/BF00372617
Shan, M., Li, S. F. Y., Yu, S., Qian, Y., Guo, S., Zhang, L., et al. (2018). Chemical fingerprint and quantitative analysis for the quality evaluation of Platycladi cacumen by ultra-performance liquid chromatography coupled with hierarchical cluster analysis. J. Chromatogr. Sci. 56 (1), 41–48. doi:10.1093/chromsci/bmx079
Singh, J., and Kakkar, P. (2013). Modulation of liver function, antioxidant responses, insulin resistance and glucose transport by Oroxylum indicum stem bark in STZ induced diabetic rats. Food Chem. Toxicol. 62, 722–731. doi:10.1016/j.fct.2013.09.035
Sulaiman, C. T., and Balachandran, I. (2016). LC/MS characterization of antioxidant flavonoids from Tragia involucrata L. Beni. Suef. Univ. J. Basic Appl. Sci. 5 (3), 231–235. doi:10.1016/j.bjbas.2016.06.001
Sulaiman, C. T., and Balachandran, I. (2017). LC/MS characterization of phenolic antioxidants of Brindle berry (Garcinia gummi-gutta (L.) Robson). Nat. Prod. Res. 31 (10), 1191–1194. doi:10.1080/14786419.2016.1224871
Sulaiman, G. M., Al-Amiery, A. A., and Bagnati, R. (2014). Theoretical, antioxidant and cytotoxic activities of caffeic acid phenethyl ester and chrysin. Int. J. Food Sci. Nutr. 65 (1), 101–105. doi:10.3109/09637486.2013.832174
Sun, W., Zhang, B., Yu, X., Zhuang, C., Li, X., Sun, J., et al. (2018). Oroxin A from Oroxylum indicum prevents the progression from prediabetes to diabetes in streptozotocin and high-fat diet induced mice. Phytomedicine 38, 24–34. doi:10.1016/j.phymed.2017.10.003
Swargiary, A., and Daimari, M. (2020). Identification of bioactive compounds by GC-MS and α-amylase and α-glucosidase inhibitory activity of rauvolfia tetraphylla L. And Oroxylum indicum (L.) Kurz: An in vitro and in silico approach. Clin. Phytosci. 6 (1), 75–11. doi:10.1186/s40816-020-00219-3
Thakur, R., Jain, N., Pathak, R., and v Sandhu, S. S. (2011). Practices in wound healing studies of plants. Evid. Based. Complement. Altern. Med. 2011 (17), 438056. doi:10.1155/2011/438056
Tistaert, C., Dejaegher, B., and Vander Heyden, Y. (2011). Chromatographic separation techniques and data handling methods for herbal fingerprints: A review. Anal. Chim. Acta 690 (2), 148–161. doi:10.1016/j.aca.2011.02.023
Tottoli, E. M., Dorati, R., Genta, I., Chiesa, E., Pisani, S., and Conti, B. (2020). Skin wound healing process and new emerging technologies for skin wound care and regeneration. Pharmaceutics 12 (8), 735. doi:10.3390/pharmaceutics12080735
Tran, T. V. A., Malainer, C., Schwaiger, S., Hung, T., Atanasov, A. G., Heiss, E. H., et al. (2015). Screening of Vietnamese medicinal plants for NF-κB signaling inhibitors: Assessing the activity of flavonoids from the stem bark of Oroxylum indicum. J. Ethnopharmacol. 159, 36–42. doi:10.1016/j.jep.2014.10.012
Varga, E., Barabás, C., Tóth, A., Boldizsár, I., Noszál, B., and Tóth, G. (2019). Phenolic composition, antioxidant and antinociceptive activities of Syringa vulgaris L. bark and leaf extracts. Nat. Prod. Res. 33 (11), 1664–1669. doi:10.1080/14786419.2018.1425855
Want, E. J. (2018). “LC-MS untargeted analysis,” in I. Metabolic profiling. Methods mol. bio. Editors G. Theodoridis, and H. Gika (New York, USA: WilsonHumana Press), 99–116. 9781493976430.
Wu, C., Wang, H., Liu, Z., Xu, B., Li, Z., Song, P., et al. (2022). Untargeted metabolomics coupled with chemometrics for leaves and stem barks of dioecious morus alba L. Metabolites 12 (2), 106. doi:10.3390/metabo12020106
Ye, J. X., Wei, W., Quan, L. H., Liu, C. Y., Chang, Q., and Liao, Y. H. (2010). An LC–MS/MS method for the simultaneous determination of chlorogenic acid, forsythiaside A and baicalin in rat plasma and its application to pharmacokinetic study of Shuang-huang-lian in rats. J. Pharm. Biomed. Anal. 52 (4), 625–630. doi:10.1016/j.jpba.2010.01.035
Yoganarasimhan, S. N. (1996). Medicinal plants of India: Karnataka. Bangalore: Interline Publishing, 366–367. Vol. 1.
Zengin, G., Uysal, A., Diuzheva, A., Gunes, E., Jekő, J., Cziáky, Z., et al. (2018). Characterization of phytochemical components of Ferula halophila extracts using HPLC-MS/MS and their pharmacological potentials: A multi-functional insight. J. Pharm. Biomed. Anal. 160, 374–382. doi:10.1016/j.jpba.2018.08.020
Zhang, Y., Xu, Q., Zhang, X., Chen, J., Liang, X., and Kettrup, A. (2005). High-performance liquid chromatography–tandem mass spectrometry for identification of isoflavones and description of the biotransformation of kudzu root. Anal. Bioanal. Chem. 383 (5), 787–796. doi:10.1007/s00216-005-0068-8
Zhao, N., Sun, Q., Song, Y., Wang, L., Zhang, T., and Meng, F. (2018). Comparative pharmacokinetics study of orientin in rat plasma by UHPLC-MS/MS after intravenous administration of single orientin and Trollius chinensis Bunge extract. Biomed. Chromatogr. 32 (4), e4142. doi:10.1002/bmc.4142
Keywords: natural products, phytochemicals, beka plant, metabolic profiling, wound healing, herbal medicine, inflammation
Citation: Abdulhafiz F, Reduan MFH, Hisam AH, Mohammad I, Abdul Wahab IR, Abdul Hamid FF, Mohammed A, Nordin ML, Shaari R, Bakar LA, Kari ZA, Wei LS, Goh KW and Ahmad Mohd Zain MR (2022) LC–TOF-MS/MS and GC-MS based phytochemical profiling and evaluation of wound healing activity of Oroxylum Indicum (L.) Kurz (Beka). Front. Pharmacol. 13:1050453. doi: 10.3389/fphar.2022.1050453
Received: 21 September 2022; Accepted: 08 November 2022;
Published: 22 November 2022.
Edited by:
Christian W Gruber, Medical University of Vienna, AustriaReviewed by:
Jen-Tsung Chen, National University of Kaohsiung, TaiwanAjaikumar B Kunnumakkara, Indian Institute of Technology Guwahati, India
Copyright © 2022 Abdulhafiz, Reduan, Hisam, Mohammad, Abdul Wahab, Abdul Hamid, Mohammed, Nordin, Shaari, Bakar, Kari, Wei, Goh and Ahmad Mohd Zain. This is an open-access article distributed under the terms of the Creative Commons Attribution License (CC BY). The use, distribution or reproduction in other forums is permitted, provided the original author(s) and the copyright owner(s) are credited and that the original publication in this journal is cited, in accordance with accepted academic practice. No use, distribution or reproduction is permitted which does not comply with these terms.
*Correspondence: Mohd Farhan Hanif Reduan, farhan.h@umk.edu.my; Khang Wen Goh, khangwen.goh@newinti.edu.my; Muhammad Rajaei Ahmad Mohd Zain, rajaei@usm.my