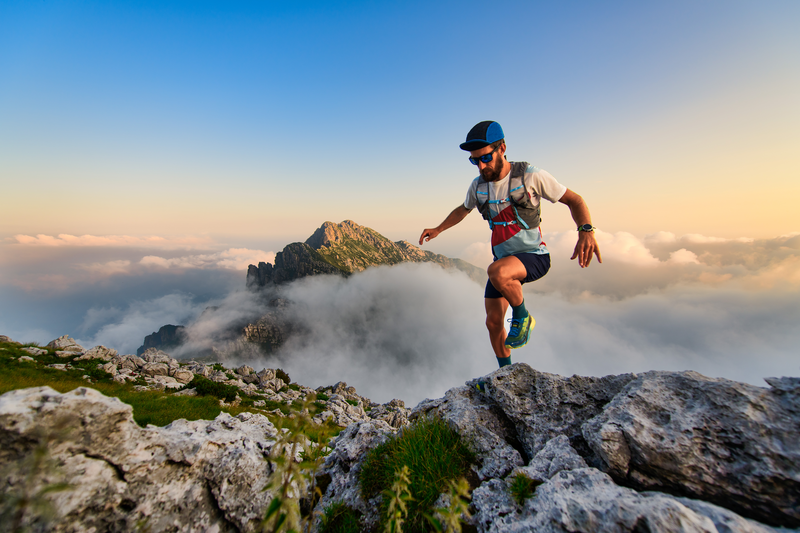
94% of researchers rate our articles as excellent or good
Learn more about the work of our research integrity team to safeguard the quality of each article we publish.
Find out more
MINI REVIEW article
Front. Pharmacol. , 21 October 2022
Sec. Cardiovascular and Smooth Muscle Pharmacology
Volume 13 - 2022 | https://doi.org/10.3389/fphar.2022.1025768
This article is part of the Research Topic Novel and current therapeutic approaches for treatment of cardiovascular disorders View all 9 articles
Vascular calcification (VC) is prevalent in hypertension, diabetes mellitus, chronic kidney disease, and aging and has been identified as an important predictor of adverse cardiovascular events. With the complicated mechanisms involved in VC, there is no effective therapy. Thus, a strategy for attenuating the development of VC is of clinical importance. Recent studies suggest that grape exosome-like nanoparticles (GENs) are involved in cell–cell communication as a means of regulating oxidative stress, inflammation, and apoptosis, which are known to modulate VC development. In this review, we discuss the roles of GENs and their potential mechanisms in the development of VC.
Vascular calcification (VC) is a pathological process characterized by abnormal deposition of hydroxyapatite (HA) crystals in the arterial intima or media of vascular walls, which can result in increased vascular stiffness and decreased vascular compliance (Nicoll and Henein, 2014; Bryan and Simon, 2015; Lanzer et al., 2021). VC commonly occurs in patients with chronic kidney disease (CKD), diabetes, and aging, and it is significantly associated with an increased risk of cardiovascular morbidity and mortality in these populations. The formation of VC is a complex and highly regulated pathological process, similar to bone development and chondrogenesis (Nakahara et al., 2017). Previous research has reported that VC is caused by hyperphosphatemia (Lee et al., 2020) and other risk factors including inflammation, oxidative stress, lipid deposition, and apoptosis. Despite its global clinical burden, no effective therapies are available to deal with VC due to its complicated underlying mechanisms.
Compelling epidemiological evidence suggests that fruit and vegetable consumption can improve lipid metabolism and endothelial function (Luc et al., 2004), lower blood pressure (John et al., 2002), and reduce oxidative stress (John et al., 2002; Zino et al., 1997). Plant-derived exosome-like nanoparticles (PDENs) were isolated and purified from plants that have lipid bilayers and functional cytosolic components such as mRNA, miRNA, proteins, and plant-homologous bioactive small molecules that can protect against vascular disease and cardiovascular-related mortality (Lichtenstein and Russell, 2005; Zhang et al., 2016a). Therefore, PDENs have been widely used to treat a variety of conditions (Mohadeseh et al., 2022) including pneumonia (Yun et al., 2021), intestinal inflammatory disease (Mu et al., 2016), cutaneous wounds (Yağız et al., 2021), and tumors (Meng et al., 2019). The therapeutic utility of PDENs is based on their anti-inflammatory, anti-oxidative, and anti-apoptotic properties. Grape exosome-like nanoparticle (GEN) is one of the most important PDENs. In this context, we consider GENs and discuss their potential role in VC prevention and treatment.
GENs derived from grapes, including proteins, lipids, RNA (mRNAs, miRNAs, and lncRNAs), and natural small molecular compounds, can be transferred to recipient cells and exert biological effects, acting as messengers in intercellular or cross-species communication to treat disease by regulating biological functions (Hadi et al., 2007; Baomei et al., 2014; Doyle and Wang, 2019). Few studies have clarified the compositions and mechanisms of proteins in GENs. A previous study indicated that the proteins in GENs are similar to those found in animal-derived exosomes, with a lower protein content (Songwen et al., 2013). GENs were found to contain proteins that regulate glucose and lipid metabolism (Songwen et al., 2013). Many studies have indicated the presence of phosphatidylethanolamine, phosphatidylcholine, phosphatidic acid, digalactosyl diacylglycerol, monogalactosyl diacylglycerol, phosphatidylinositol, and phosphatidylserine in GENs. However, compared with exosomes in animal cells, GENs contain almost no cholesterol (Songwen et al., 2013; Qilong et al., 2016). MiRNAs in GENs are mostly from the miR169 family, which shares two sequences with human miRNAs (hsa-miR-4480 and hsa-miR-4662a-5p). Further research has revealed that some miRNAs can directly target the expression of inflammatory factor genes such as IL-6, IL-2, IL-5, and IL-1 (Songwen et al., 2013). In vitro data have indicated that miRNAs from GENs can specifically bind to mammalian miRNAs, affecting many important biological processes (Juan et al., 2018). In addition, natural small molecular compounds from grapes were found in GENs, such as procyanidin, polyphenol, and ACH09 (Figure 1; Table 1).
FIGURE 1. Structure of grape exosome-like nanoparticles (GENs) served as a bioactive vesicle for inhibiting the osteogenic differentiation of vascular smooth muscle cells.
Small vesicles derived from plants were first observed with a bilayer membrane structure by electron microscopy in 1968 (Marchant and Robards, 1968). PDENs have been identified and isolated in numerous plants over decades of research and purified and confirmed using characterization techniques such as dynamic light scattering, zeta potential, and Western blotting (Nader et al., 2021). The average diameter of the GEN population was 37.47–380.5 nm. Zeta potential measurements indicated that GENs have a negative zeta potential ranging from -69.6 mV to +2.52 mV, with an average potential of -26.3–8.14 mV (Wenbo et al., 2021). GENs can maintain particle size and surface charge stability at physiological temperatures (37°C). In addition, GENs can exhibit significant stability after being stored in deep-freeze conditions (−80°C) for half a year. Biological molecules’ integrity of GENs can withstand repeated freeze–thaw cycles in the laboratory. GENs are smaller and more homogeneous in simulated gastric fluid and simulated intestinal fluid, with a lower zeta potential (Wenbo et al., 2021). GENs can be taken up by multiple cells including endotheliaocytes, lymphocytes, macrophagocytes, and others via the endocytic pathway, and the findings indicated that GEN uptake by recipient cells is energy-dependent. F4/80 macrophages and stem cells in the intestine consume GENs (Songwen et al., 2013). The cytochalasin D and the macrolide antibiotics, bafilomycin A1 and concanamycin A, which are highly specific V-ATPase inhibitors, significantly inhibit GEN uptake (Songwen et al., 2013). PDEN distribution in vivo varies depending on the route of administration. For example, GENs were found primarily in the distal small intestine, cecum, and colon after passing through the stomach and proximal small intestine after oral administration (Songwen et al., 2013). 1,1-Dioctadecyl-3,3,3,3-tetramethylindotricarbocyaine iodide (DiR) fluorescent signals are predominantly detected in the liver, lungs, kidneys, and splenic tissues 72 h after a tail vein or intraperitoneal injection, whereas intramuscular injections of DiR-labeled grapefruit-derived nanovectors (GNVs) are predominantly localized in the muscle. The majority of DiR-labeled GNVs were found in the lungs and brain after intranasal administration. No signal was found in lung tissue 72 h after intranasal administration (Qilong et al., 2016) (Figure 1).
Similar to artificial liposomes, GENs are capable of delivering drugs, siRNA, DNA, and proteins to various types of cells, and the specificity of GEN is able to be increased by introducing targeting ligands for increasing curative effect (Zhang et al., 2016). Compared with artificial carriers, GENs possess high biocompatibility, low immunogenicity, and easy modifiability, which could cross the blood–brain barrier but could not pass through the placental barrier. Thus, GENs have better security and bright clinical applied prospect as drug carriers.
At present, the common methods of loading nucleic acid therapeutic agents in animal-derived exosomes adopt cell transfection and then collect agent-loaded exosomes (El-Andaloussi et al., 2012). By contrast, there are many methods of loading drugs in PDENs including co-incubation, sonication, repetitive freeze–thawing, and electroporation that provide convenience for drug loading (Cong et al., 2022).
Isolation and purification of GENs are similar to those of exosomes in animal cells or body fluids. A combination of differential centrifugation and sucrose density-gradient ultracentrifugation is the “gold standard” to extract abundant GENs in a short time (Zhang et al., 2016). First, grapes are ground in a blender to extract juice and the juice is strained through a sieve or a piece of gauze. Next, differentially centrifugation (500 g for 10 min, 2,000 g for 20 min, 5,000 g for 30 min, and 10,000 g for 1 h) is used to remove large grape fibers, and then supernatant ultracentrifugation is used to concentrate GENs at 100,000 g for 2 h. Finally, for purification of the GENs, the concentrated solutions of GEN are transferred to a discontinuous sucrose gradient [8%, 30%, 45%, and 60% (g/v)] and ultracentrifuged at 150,000 g for 2 h to remove other vesicles and aggregates of proteins or RNA. The bands in the 30% and 45% layers are purified GENs. In addition, GEN concentration is determined by measuring the protein concentration using a BCA protein quantification assay kit (Songwen et al., 2013; Wenbo et al., 2021). GENs allow for large-scale production as they are plentiful and inexpensive.
The occurrence and development of VC are influenced by oxidative stress for two primary reasons: 1. increased cellular endogenous reactive oxygen species (ROS) levels; 2. an imbalance in ROS generation and ROS scavenging. Excessive ROS causes oxidative damage to DNA (Hongmei et al., 2010; Roberto et al., 2012), lipids, and proteins, inducing phenotypic transformation of vascular smooth muscle cells (VSMCs) by activating the Akt signaling pathways and upregulating Runx2, an essential osteogenic transcription factor (Ting et al., 2021). Excess oxidized low-density lipoprotein (OxLDL) hastens VSMC transdifferentiation into osteoblast-like cells (Zhang et al., 2016b). Diabetes, in particular, has been shown to play a synergistic role in VC via the advanced glycation end product and advanced glycation end product receptor, further increasing the correlation between hyperglycemia and oxidase stress from NADPH oxidase (Hongmei et al., 2010; Mody et al., 2001).
GENs were reported to be functional nanoparticles with the ability to scavenge ROS and protect against non-specific cell damage caused by ROS (Songwen et al., 2013). Zhang et al. synthesized polyphenol nanoparticles from grape seeds with adjustable size, excellent biocompatibility, and ROS scavenging capacity. The role of nanoparticles in preventing cell damage caused by ROS, accelerating wound healing, inhibiting ulcerative colitis, and regulating oxidative stress in dry eye syndrome has been validated (Songwen et al., 2013). Wang et al. created a drug delivery system based on grapeseed extract loaded with solid lipid nanoparticles to improve bioavailability and aqueous solubility compared with the parent compound. They discovered that nanoparticles reduce oxidative stress in respiratory epithelia and are anti-inflammatory and anti-apoptotic (Tianyou et al., 2021). Polygalloyl polyflavan-3-ols were reported to be associated with the cardiovascular disease-protective effects of grapes as they inhibit platelet aggregation and oxidation of OxLDL (Yee-Ling and Shun-Wan, 2014). By inducing nitric oxide production and acting on the insulin signaling pathways, ACH09 (grape skin extract) was found to be extremely effective against obesity, hypertriglyceridemia, hyperglycemia, insulin resistance, and oxidative stress (Shanmuganayagam et al., 2012). According to Angela C et al., grape consumption may lower blood pressure and plasma ROS levels, reduce atherosclerotic plaque formation, and maintain normal serum AST levels (Resende et al., 2013). Several studies have found that grapeseed and grape skin extracts have anti-diabetic effects, improving glucose tolerance and insulin sensitivity in diabetic patients (Chis et al., 2009; Yanni et al., 2015). In the diet-induced obesity mouse models, ACH09 restored decreased plasma and mesenteric artery antioxidant activities of superoxide dismutase, catalase, and glutathione peroxidase (Shanmuganayagam et al., 2012). Several studies have shown that resveratrol slows progression and reduces mortality in CKD rat models, which is attributed to its inhibitory effect on oxidative stress (Byon et al., 2008). Polyphenolic flavonoids derived from grape seeds have been shown to reduce lipid peroxides and carbonylated protein levels in Wistar albino rats. The grape extract increased antioxidant activity in plasma and liver tissues, helping to regulate blood lipids, protect liver cells, and improve blood glucose (Yanni et al., 2015) and suggesting that GENs can improve VC by reducing oxidative stress.
Chronic inflammation is known to contribute to VC. When monocyte-derived macrophages are recruited and activated in mineralized areas, mineral deposition is triggered (Koji et al., 2012). Excess production of proinflammatory factors such as TNF-α, IL-1, and IL-6 can accelerate the formation of VC by increasing the expression of BMP2 and decreasing the expression of MGP (Koji et al., 2012; Kay et al., 2016). Boström Kristina et al. found that TNF-α, L-1β, and TGF-β can induce and promote endothelial-to-mesenchymal transition, sensitizing aortic endothelial cells to BMP-9-induced osteogenic differentiation and enhancing BMP-9-induced mineralization (Kristina, 2005).
GENs were found to inhibit intestinal inflammation in a mouse model of DSS-induced colitis (Songwen et al., 2013). A near-infrared fluorescent dye, DiR, was used to trace the in vivo distribution imaging in mice; it was found that GENs can be absorbed by intestinal stem cells by penetrating the intestinal mucosal barrier through the Wnt/catenin signaling pathway, stimulating Lgr5hi intestinal stem cell proliferation, accelerating the regeneration of small intestinal mucosa, and promoting rapid recovery of intestinal structure. Axin-2, cyclin D1, c-myc, and EGFR expression were found to be significantly upregulated in dextran sulfate sodium (DSS)-induced colitis. It was found that oral administration of GEN protects mice from DSS-induced colitis compared to the PBS control. Orally administered edible plant GENs induce nuclear translocation of macrophage nuclear factor-erythroid-derived 2-related factor-2(Nrf2) and intestinal Wnt/TCF4 activation in mice. Nuclear translocation of Nrf2 and Wnt/TCF4 activation are important in anti-inflammatory responses (Songwen et al., 2013).
Furthermore, by inhibiting nuclear factor kappa-light-chain-enhancer of activated B cell (NF-ҡB) activation and COX-2 expression, resveratrol and other biologically active substances can reduce pro-inflammatory cytokines, PGE2 and PGD2 levels, and neutrophil infiltration (Cianciulli et al., 2012; Panaro et al., 2012; Gonzalo et al., 2019). Proanthocyanidins were loaded into solid lipid nanoparticles and used to treat inflammatory airway diseases (Baomei et al., 2014). Grape phenolic compounds have anti-inflammatory, anti-cancer, and anti-aging properties (Castellani et al., 2018). A previous study found that grape polyphenols may reduce nitric oxide inactivation via oxidant enzymes to prevent inflammation. The main symptoms are lower hypersensitive-c-reactive-protein and IL-6 levels in the blood (Fahimeh et al., 2020). Based on these findings, we hypothesize that GENs lower VC by inhibiting chronic inflammation.
Apoptosis of VSMCs occurs prior to the formation of calcified nodules. Proudfoot D et al. reported that inhibiting apoptosis with the caspase inhibitor z-VAD-FMK reduced calcification in nodules by approximately 40%, but when apoptosis was stimulated in nodular cultures with anti-Fas IgM, calcification was increased 10-fold (Proudfoot et al., 2000). Furthermore, they found that apoptotic VSMCs can produce matrix vesicles and apoptotic bodies, both of which have the ability to concentrate calcium and act as nucleating structures for calcium crystal formation (Proudfoot et al., 2000). They found that apoptosis occurs before calcification and that it activates the early promoter in VC.
Grape proanthocyanidins have been found to inhibit H2O2-induced apoptotic signaling, which is mediated by p53 in osteoblastic MC3T3-E1 cells (Zhang et al., 2014). Grapeseed proanthocyanidins have been shown to reduce stress-induced apoptosis in the endoplasmic reticulum (ER). Another study found that GSP improved long-term neurological outcomes by reducing ischemia–reperfusion-induced neuronal apoptosis and brain injury and inhibiting the expression of ER stress-associated genes. GSP protects mice against ischemic stroke by reducing neuronal apoptosis and ER stress-associated apoptosis by inhibiting GRP78 and caspase-12 (Kun et al., 2019; Yunxia et al., 2020). Thus, it is possible that VC can be improved with GENs by decreasing VSMC apoptosis.
An epidemiological study found that osteoporosis and VC have age-independent associations (Yunxia et al., 2020; Hak et al., 2000). Thandapilly Sijo J et al. discovered CKD patients with dysregulated calcium and phosphate metabolism. However, there are some issues to consider in the relationship between calcium loss from the skeleton in osteoporosis and calcium deposits in VC (Tofani et al., 2005; Demer et al., 2014; Wei S. et al., 2018). Grapeseed proanthocyanidin extract induces anti-osteoporosis effects by increasing bone mineral density and bone strength (Tofani et al., 2005; Wei Z. et al., 2018).
Furthermore, GENs containing some miRNAs can be uptake by VSMCs to participate in immunoregulation. Grape flavonoids can regulate endothelial function and improve endothelial-dependent vasodilation in the aorta (Stein et al., 1999). Numerous molecular targets (silent information regulator 1 (SIRT1), 5′ AMP-activated protein kinase (AMPK), endothelial nitric oxide synthase (eNOS), Nrf2, peroxisome proliferator-activated receptor (PPAR), Kruppel-like factor 2 (KLF2), and NF-kB) (Nader et al., 2021) were found. Several recent studies have found that polyphenol extracts from grapes provide cardiovascular benefits by lowering blood pressure, improving the relaxation of arterial smooth muscle, increasing arterial compliance, and attenuating pathological cardiac hypertrophy (Thandapilly et al., 2012). This approach is expected to be used in the treatment of VC.
VC is a key factor in the development of cardiovascular disease. Although the development of therapeutics for the treatment of VC in experimental treatment has made great progress, clinical first-line medication is still lacking. Unfortunately, current anti-VC drugs are primarily based on phosphate binders and calcimimetic agents, which are restricted by inefficient drug delivery and short residence time (Lanzer et al., 2021; Raggi et al., 2011). GENs are expected to serve as novel drug carriers with synergistic effects for the delivery of anti-VC drugs due to their high biocompatibility, low immunogenicity, and easy modifiability (Songwen et al., 2013).
In this review, we discussed the potential treatment efficacy and various mechanisms of GENs related to affecting the VC (Figure 2). Despite the many benefits of GENs, we still need to consider the following deficiencies in the treatment of VC: 1. particle size distribution is relatively nonuniform; larger particles might not penetrate the intercellular space of vascular endothelium and enter calcified plaques. 2. The complete removal of plant-derived impurities cannot be guaranteed in the process of isolation and purification, causing immune responses. 3. The roles and functions of GENs against VC are unclear, which still is a hypothesis based on existing knowledge of VC and GENs. Therefore, they could have unpredictable effects (Kim et al., 2022). Overall, GENs demonstrate a potential protective effect on VC, and they may constitute the next-generation therapeutics hopefully.
FIGURE 2. Potential regulatory mechanisms of grape exosome-like nanoparticles (GENs) for vascular calcification (VC). Several mechanisms of VC occurrence are modulated by GENs, including oxidative stress, inflammation, apoptosis, and immune response.
YT was in charge of searching all the relative articles and writing this manuscript. JH was in charge of writing. QZ and YZ were in charge of drawing the picture. ZL, TG, YP, and XL helped to revise the manuscript. WF gave valuable and professional suggestions and guidance in organizing and drafting this manuscript. CO was in charge of supervision, writing—review and editing, and funding acquisition.
This work was supported by the National Natural Science Foundation of China (NSFC) (Grant 82200900 to WF; 31671025, 31771099 and 81871504 to CO), the President Foundation of Nanfang Hospital Southern Medical University (Grant 2021B001 to WF), and Medical Scientific Research Foundation of Guangdong Province (A2022295 to WF).
The authors declare that the research was conducted in the absence of any commercial or financial relationships that could be construed as a potential conflict of interest.
All claims expressed in this article are solely those of the authors and do not necessarily represent those of their affiliated organizations, or those of the publisher, the editors, and the reviewers. Any product that may be evaluated in this article, or claim that may be made by its manufacturer, is not guaranteed or endorsed by the publisher.
CKD, chronic kidney disease; DiR, 1,1-dioctadecyl-3,3,3,3-tetramethylindotricarbocyaine iodide; DSS, dextran sulfate sodium; ER, endoplasmic reticulum; GEN, grape exosome-like nanoparticle; GNV, grapefruit-derived nanovector; HA, hydroxyapatite; OxLDL, oxidized low-density lipoprotein; PDEN, plant-derived exosome-like nanoparticle; ROS, reactive oxygen species; VC, vascular calcification; VSMC, vascular smooth muscle cell.
Albers, A. R., Varghese, S., Vitseva, O., Vita, J. A., and Freedman, J. E. (2004). The antiinflammatory effects of purple grape juice consumption in subjects with stable coronary artery disease. Arterioscler. Thromb. Vasc. Biol. 24 (11), e179–e180. doi:10.1161/01.ATV.0000143479.97844.af
Baomei, W., Xiaoying, Z., Zhong-Bin, D., Hong, J., Jingyao, M., Qilong, W., et al. (2014). Targeted drug delivery to intestinal macrophages by bioactive nanovesicles released from grapefruit. Mol. Ther. 22 (3), 522–534. doi:10.1038/mt.2013.190
Borde, P., Mohan, M., and Kasture, S. (2011). Effect of myricetin on deoxycorticosterone acetate (DOCA)-salt-hypertensive rats. Nat. Prod. Res. 25 (16), 1549–1559. doi:10.1080/14786410903335190
Breuss, J. M., Atanasov, A. G., and Uhrin, P. (2019). Resveratrol and its effects on the vascular system. Int. J. Mol. Sci. 20 (7), E1523. doi:10.3390/ijms20071523
Bryan, C., and Simon, W. R. (2015). The relationship between arterial stiffness and heart failure with preserved ejection fraction: a systemic meta-analysis. Heart Fail. Rev. 20 (3), 291. doi:10.1007/s10741-015-9471-1
Byon, C. H., Amjad, J., Qun, D., Kappes, J. C., Clemens, T. L., Victor, M. D-U., et al. (2008). Oxidative stress induces vascular calcification through modulation of the osteogenic transcription factor Runx2 by AKT signaling. J. Biol. Chem. 283 (22), 15319–15327. doi:10.1074/jbc.M800021200
Cai, Q., Li, B. Y., Gao, H. Q., Zhang, J. H., Wang, J. F., Yu, F., et al. (2011). Grape seed procyanidin b2 inhibits human aortic smooth muscle cell proliferation and migration induced by advanced glycation end products. Biosci. Biotechnol. Biochem. 75 (9), 1692–1697. doi:10.1271/bbb.110194
Cao, H., Xie, Y., and Chen, X. (2015). Type 2 diabetes diminishes the benefits of dietary antioxidants: evidence from the different free radical scavenging potential. Food Chem. 186, 106–112. doi:10.1016/j.foodchem.2014.06.027
Castellani, S., Trapani, A., Spagnoletta, A., Toma, Ld, Magrone, T., Gioia, S. D., et al. (2018). Nanoparticle delivery of grape seed-derived proanthocyanidins to airway epithelial cells dampens oxidative stress and inflammation. J. Transl. Med. 16 (1), 140. doi:10.1186/s12967-018-1509-4
Chen, S., and Jiang, H. (2016). Therapeutic effects of quercetin on inflammation, obesity, and type 2 diabetes. Mediat. Inflamm. 2016, 9340637. doi:10.1155/2016/9340637
Chis, I. C., Ungureanu, M. I., Marton, A., Simedrea, R., Muresan, A., Postescu, I. D., et al. (2009). Antioxidant effects of a grape seed extract in a rat model of diabetes mellitus. Diab. Vasc. Dis. Res. 6 (3), 200–204. doi:10.1177/1479164109336692
Cianciulli, A., Calvello, R., Cavallo, P., Dragone, T., Carofiglio, V., and Panaro, M. A. (2012). Modulation of NF-κB activation by resveratrol in LPS treated human intestinal cells results in downregulation of PGE 2 production and COX-2 expression. Toxicol. Vitro 26 (7), 1122–1128. doi:10.1016/j.tiv.2012.06.015
Cong, M., Tan, S., Li, S., Gao, L., Huang, L., Zhang, H. G., et al. (2022). Technology insight: Plant-derived vesicles-How far from the clinical biotherapeutics and therapeutic drug carriers? Adv. Drug Deliv. Rev. 182, 114108. doi:10.1016/j.addr.2021.114108
da Costa, G. F., Ognibene, D. T., da Costa, C. A., Teixeira, M. T., Cordeiro, V., de Bem, G. F., et al. (2020). Vitis vinifera L. Grape skin extract prevents development of hypertension and altered lipid profile in spontaneously hypertensive rats: Role of oxidative stress. Prev. Nutr. Food Sci. 25 (1), 25–31. doi:10.3746/pnf.2020.25.1.25
Demer, L. L., Yin, T., and Tintut, Y. (2014). Inflammatory, metabolic, and genetic mechanisms of vascular calcification. Arterioscler. Thromb. Vasc. Biol. 34 (4), 715–723. doi:10.1161/ATVBAHA.113.302070
Downing, L. E., Edgar, D., Ellison, P. A., and Ricketts, M. L. (2017). Mechanistic insight into nuclear receptor-mediated regulation of bile acid metabolism and lipid homeostasis by grape seed procyanidin extract (GSPE) Cell biochem. Funct. 35 (1), 12–32. doi:10.1002/cbf.3247
Doyle, L. M., and Wang, M. Z. (2019). Overview of extracellular vesicles, their origin, composition, purpose, and methods for exosome isolation and analysis. Cells 8 (7), E727. doi:10.3390/cells8070727
El-Andaloussi, S., Lee, Y., Lakhal-Littleton, S., Li, J., Seow, Y., Gardiner, C., et al. (2012). Exosome-mediated delivery of siRNA in vitro and in vivo. Nat. Protoc. 7 (12), 2112–2126. doi:10.1038/nprot.2012.131
Fahimeh, H., Ali, G., and Mitra, H. (2020). Effect of grape polyphenols on selected inflammatory mediators: A systematic review and meta-analysis randomized clinical trials. EXCLI J. 19, 251–267. doi:10.17179/excli2020-1011
Godse, S., Mohan, M., Kasture, V., and Kasture, S. (2010). Effect of myricetin on blood pressure and metabolic alterations in fructose hypertensive rats. Pharm. Biol. 48 (5), 494–498. doi:10.3109/13880200903188526
Gonzalo, S-D., Amaya, GdV., Vera, vdP., Geerts, M. E., Lindemann, J. H., Gt Johnson, S., et al. (2019). Inflammation induces endothelial-to-mesenchymal transition and promotes vascular calcification through downregulation of BMPR2. J. Pathol. 247 (3), 333–346. doi:10.1002/path.5193
Grau-Bové, C., Sierra-Cruz, M., Miguens-Gomez, A., Rodriguez-Gallego, E., Beltran-Debon, R., Blay, M., et al. (2020). A ten-day grape seed procyanidin treatment prevents certain ageing processes in female rats over the long term. Nutrients 12 (12), E3647. doi:10.3390/nu12123647
Hadi, V., Karin, E., Apostolos, B., Margareta, S., J, L. J., and O, L. J. (2007). Exosome-mediated transfer of mRNAs and microRNAs is a novel mechanism of genetic exchange between cells. Nat. Cell Biol. 9 (6), 654–659. doi:10.1038/ncb1596
Hak, A. E., Pols, H. A., Hofman, A., Witteman, J. C., and van Hemert, A. M. (2000). Progression of aortic calcification is associated with metacarpal bone loss during menopause: a population-based longitudinal study. Arterioscler. Thromb. Vasc. Biol. 20 (8), 1926–1931. doi:10.1161/01.atv.20.8.1926
Hongmei, L., Qian, L., and Kaixun, H. (2010). Selenium suppressed hydrogen peroxide-induced vascular smooth muscle cells calcification through inhibiting oxidative stress and ERK activation. J. Cell. Biochem. 111 (6), 1556–1564. doi:10.1002/jcb.22887
John, J., Ziebland, S., Yudkin, P., Roe, L., and Neil, H. (2002). Effects of fruit and vegetable consumption on plasma antioxidant concentrations and blood pressure: a randomised controlled trial. Lancet 359 (9322), 1969–1974. doi:10.1016/s0140-6736(02)98858-6
Juan, X., Siyuan, F., Xun, W., Keren, L., Yi, L., Yuhao, W., et al. (2018). Identification of exosome-like nanoparticle-derived microRNAs from 11 edible fruits and vegetables. PeerJ 6, e5186. doi:10.7717/peerj.5186
kay, A. M., Simpson, C. L., and Stewart, J. A. (2016). The role of AGE/RAGE signaling in diabetes-mediated vascular calcification. J. Diabetes Res. 2016, 6809703. doi:10.1155/2016/6809703
Keevil, J. G., Osman, H. E., Reed, J. D., and Folts, J. D. (2000). Grape juice, but not orange juice or grapefruit juice, inhibits human platelet aggregation. J. Nutr. 130 (1), 53–56. doi:10.1093/jn/130.1.53
Kim, J., Li, S., Zhang, S., and Wang, J. (2022). Plant-derived exosome-like nanoparticles and their therapeutic activities. Asian J. Pharm. Sci. 17 (1), 53–69. doi:10.1016/j.ajps.2021.05.006
Koji, I., Yuka, S., Yoshiki, A., Youhei, K., Kiyonari, M., Yoshiaki, S., et al. (2012). Macrophages play a unique role in the plaque calcification by enhancing the osteogenic signals exerted by vascular smooth muscle cells. Biochem. Biophys. Res. Commun. 425 (1), 39–44. doi:10.1016/j.bbrc.2012.07.045
Kristina, B. (2005). Proinflammatory vascular calcification. Circ. Res. 96 (12), 1219–1220. doi:10.1161/01.RES.0000172407.20974.e5
Kun, F., Liqiang, C., Shuai, H., Yan, G., Wei, Z., and Yunan, B. (2019). Grape seed proanthocyanidins attenuate apoptosis in ischemic stroke. Acta Neurol. belg. 121, 357–364. (prepublish). doi:10.1007/s13760-019-01111-9
Kundu, J. K., Shin, Y. K., and Surh, Y. J. (2006). Resveratrol modulates phorbol ester-induced pro-inflammatory signal transduction pathways in mouse skin in vivo: NF-kappaB and AP-1 as prime targets. Biochem. Pharmacol. 72 (11), 1506–1515. doi:10.1016/j.bcp.2006.08.005
Lanzer, P., Hannan, F. M., Lanzer, J. D., Janzen, J., Raggi, P., Furniss, D., et al. (2021). Medial arterial calcification: JACC state-of-the-art review. J. Am. Coll. Cardiol. 78 (11), 1145–1165. doi:10.1016/j.jacc.2021.06.049
Lee, S. J., Lee, I-K., and Jeon, J-H. (2020). Vascular calcification-new insights into its mechanism. Sci. Lett. 21 (8), 2685. doi:10.3390/ijms21082685
Leikert, J. F., Räthel, T. R., Wohlfart, P., Cheynier, V., Vollmar, A. M., and Dirsch, V. M. (2002). Red wine polyphenols enhance endothelial nitric oxide synthase expression and subsequent nitric oxide release from endothelial cells. Circulation 106 (13), 1614–1617. doi:10.1161/01.cir.0000034445.31543.43
Li, X. L., Yu, F., Li, B. Y., Fu, C. L., Yu, X., Xu, M., et al. (2019). The protective effects of grape seed procyanidin B2 against asporin mediates glycated low-density lipoprotein induced-cardiomyocyte apoptosis and fibrosis. Cell Biol. Int. 44, 268–277. doi:10.1002/cbin.11229
Lichtenstein, A. H., and Russell, R. M. (2005). Essential nutrients: Food or supplements?: Where should the emphasis Be? JAMAJ. Am. Med. Assoc. 294 (3), 351–358. doi:10.1001/jama.294.3.351
Liu, J., Hu, S., Zhu, B., Shao, S., and Yuan, L. (2020). Grape seed procyanidin suppresses inflammation in cigarette smoke-exposed pulmonary arterial hypertension rats by the PPAR-γ/COX-2 pathway. Nutr. Metab. Cardiovasc. Dis. 30 (2), 347–354. doi:10.1016/j.numecd.2019.09.022
Lu, F., Li, Y., Zhou, B., Guo, Q., and Zhang, Y. (2021). Early-life supplementation of grape polyphenol extract promotes polyphenol absorption and modulates the intestinal microbiota in association with the increase in mRNA expression of the key intestinal barrier genes. Food Funct. 12 (2), 602–613. doi:10.1039/d0fo02231d
Luc, D., Hilary, C., Arnett, D. K., Curtis, E. R., Province, M. A., Moore, L. L., et al. (2004). Fruit and vegetable consumption and LDL cholesterol: the national heart, lung, and blood institute family heart study. Am. J. Clin. Nutr. 79 (2), 213–217. doi:10.1093/ajcn/79.2.213
Magyar, K., Halmosi, R., Palfi, A., Feher, G., Czopf, L., Fulop, A., et al. (2012). Cardioprotection by resveratrol: a human clinical trial in patients with stable coronary artery disease. Clin. Hemorheol. Microcirc. 50 (3), 179–187. doi:10.3233/ch-2011-1424
Marchant, R., and Robards, Aw (1968). Membrane systems associated with the plasmalemma of plant cells. Ann. Bot. 32 (127), 457–471. doi:10.1093/oxfordjournals.aob.a084221
Meng, C., Huaijiang, Y., Xuan, H., Ling, W., Qin, W., Xiaoyan, S., et al. (2019). Ginseng-derived nanoparticles alter macrophage polarization to inhibit melanoma growth. J. Immunother. Cancer 7 (1), 326. doi:10.1186/s40425-019-0817-4
Meng, T., Xiao, D., Muhammed, A., Deng, J., Chen, L., and He, J. (2021). Anti-inflammatory action and mechanisms of resveratrol. Molecules 26 (1), E229. doi:10.3390/molecules26010229
Miyagi, Y., Miwa, K., and Inoue, H. (1997). Inhibition of human low-density lipoprotein oxidation by flavonoids in red wine and grape juice. Am. J. Cardiol. 80 (12), 1627–1631. doi:10.1016/s0002-9149(97)00755-8
Mizutani, K., Ikeda, K., and Yamori, Y. (2000). Resveratrol inhibits AGEs-induced proliferation and collagen synthesis activity in vascular smooth muscle cells from stroke-prone spontaneously hypertensive rats. Biochem. Biophys. Res. Commun. 274 (1), 61–67. doi:10.1006/bbrc.2000.3097
Mody, N., Parhami, F., Sarafian, T. A., and Demer, L. L. (2001). Oxidative stress modulates osteoblastic differentiation of vascular and bone cells. Free Radic. Biol. Med. 31 (4), 509–519. doi:10.1016/s0891-5849(01)00610-4
Mohadeseh, N., Bipin, S., Ahmad, M. R., Mahdieh, N., Azadeh, B., Mahdi, A., et al. (2022). Plant-derived extracellular vesicles: a novel nanomedicine approach with advantages and challenges. Cell Commun. Signal. 20 (1), 69. doi:10.1186/s12964-022-00889-1
Mu, J., Zhuang, X., Wang, Q., Jiang, H., Deng, Z., Wang, B., et al. (2016). Interspecies communication between plant and mouse gut host cells through edible plant derived exosome-like nanoparticles. Mol. Nutr. Food Res. 60 (4), 1561. doi:10.1002/mnfr.201300729
Myers, G. L., Christenson, R. H., Cushman, M., Ballantyne, C. M., Cooper, G. R., Pfeiffer, C. M., et al. (2009). National academy of clinical biochemistry laboratory medicine practice guidelines: emerging biomarkers for primary prevention of cardiovascular disease. Clin. Chem. 55 (2), 378–384. doi:10.1373/clinchem.2008.115899
Nader, K., Anya, D., Paul, S., and Stassen, F. R. (2021). Plant-derived extracellular vesicles: Current findings, challenges, and future applications. Membranes 11 (6), 411. doi:10.3390/membranes11060411
Nakahara, T., Dweck, M. R., Narula, N., Pisapia, D., Narula, J., and Strauss, H. W. (2017). Coronary artery calcification: From mechanism to molecular imaging. JACC. Cardiovasc. Imaging 10 (5), 582–593. doi:10.1016/j.jcmg.2017.03.005
Nassiri-Asl, M., and Hosseinzadeh, H. (2016). Review of the pharmacological effects of Vitis vinifera (grape) and its bioactive constituents: An update. Phytother. Res. 30 (9), 1392–1403. doi:10.1002/ptr.5644
Nicoll, R., and Henein, M. Y. (2014). The predictive value of arterial and valvular calcification for mortality and cardiovascular events. Int J Cardiol Heart & Vessels 3, 1. doi:10.1016/j.ijchv.2014.02.001
Pace-Asciak, C. R., Hahn, S., Diamandis, E. P., Soleas, G., and Goldberg, D. M. (1995). The red wine phenolics trans-resveratrol and quercetin block human platelet aggregation and eicosanoid synthesis: implications for protection against coronary heart disease. Clin. Chim. Acta. 235 (2), 207–219. doi:10.1016/0009-8981(95)06045-1
Pace-Asciak, C. R., Rounova, O., Hahn, S. E., Diamandis, E. P., and Goldberg, D. M. (1996). Wines and grape juices as modulators of platelet aggregation in healthy human subjects. Clin. Chim. Acta. 246 (1-2), 163–182. doi:10.1016/0009-8981(96)06236-5
Panaro, M. A., Carofiglio, V., Acquafredda, A., Cavallo, P., and Cianciulli, A. (2012). Anti-inflammatory effects of resveratrol occur via inhibition of lipopolysaccharide-induced NF-κB activation in Caco-2 and SW480 human colon cancer cells. Br. J. Nutr. 108 (9), 1623–1632. doi:10.1017/S0007114511007227
Proudfoot, D., Skepper, J. N., Hegyi, L., Bennett, M. R., Shanahan, C. M., and Weissberg, P. L. (2000). Apoptosis regulates human vascular calcification in vitro: evidence for initiation of vascular calcification by apoptotic bodies. Circ. Res. 87 (11), 1055–1062. doi:10.1161/01.res.87.11.1055
Qilong, W., Xiaoying, Z., Jingyao, M., Zhong-Bin, D., Hong, J., Lifeng, Z., et al. (2016). Corrigendum: Delivery of therapeutic agents by nanoparticles made of grapefruit-derived lipids. Nat. Commun. 7 (1), 11347. doi:10.1038/ncomms11347
Raggi, P., Chertow, G. M., Torres, P. U., Csiky, B., Naso, A., Nossuli, K., et al. (2011). The ADVANCE study: a randomized study to evaluate the effects of cinacalcet plus low-dose vitamin D on vascular calcification in patients on hemodialysis. Nephrol. dialysis, Transplant. official Publ. Eur. Dialysis Transpl. Assoc. - Eur. Ren. Assoc. 26 (4), 1327–1339. doi:10.1093/ndt/gfq725
Rasines-Perea, Z., and Teissedre, P. L. (2017). Grape polyphenols' effects in human cardiovascular diseases and diabetes. Mol. (Basel, Switz. 22 (1), E68. doi:10.3390/molecules22010068
Resende, A. C., Emiliano, A. F., Cordeiro, V. S., de Bem, G. F., de Cavalho, L. C., de Oliveira, P. R., et al. (2013). Grape skin extract protects against programmed changes in the adult rat offspring caused by maternal high-fat diet during lactation. J. Nutr. Biochem. 24 (12), 2119–2126. doi:10.1016/j.jnutbio.2013.08.003
Roberto, SdM., França, d. C. G., Bello, M. A. S., Ferreira, Q. E., Col, M. D. D., Patrícia, G-S. E., et al. (2012). Vitis vinifera L. grape skin extract activates the insulin-signalling cascade and reduces hyperglycaemia in alloxan-induced diabetic mice. J. Pharm. Pharmacol. 64 (2), 268–276. doi:10.1111/j.2042-7158.2011.01395.x
Rodríguez, R. M., Colom-Pellicer, M., Blanco, J., Calvo, E., Aragones, G., and Mulero, M. (2022). Grape-Seed procyanidin extract (GSPE) seasonal-dependent modulation of glucose and lipid metabolism in the liver of healthy F344 rats. Biomolecules 12 (6), 839. doi:10.3390/biom12060839
Santos, I. B., de Bem, G. F., Cordeiro, V. S. C., da Costa, C. A., de Carvalho, L., da Rocha, A. P. M., et al. (2017). Supplementation with Vitis vinifera L. skin extract improves insulin resistance and prevents hepatic lipid accumulation and steatosis in high-fat diet-fed mice. Nutr. Res. (New York, NY) 43, 69–81. doi:10.1016/j.nutres.2017.05.007
Shanmuganayagam, D., Beahm, M. R., Kuhns, M. A., Krueger, C. G., Reed, J. D., and Folts, J. D. (2012). Differential effects of grape ( Vitis vinifera ) skin polyphenolics on human platelet aggregation and low-density lipoprotein oxidation. J. Agric. Food Chem. 60 (23), 5787–5794. doi:10.1021/jf203487g
Songwen, J., Jingyao, M., Terje, D., Xiaoying, Z., Qilong, W., Hong, J., et al. (2013). Grape exosome-like nanoparticles induce intestinal stem cells and protect mice from DSS-induced colitis. Mol. Ther. 21 (7), 1345–1357. doi:10.1038/mt.2013.64
Stein, J. H., Keevil, J. G., Wiebe, D. A., Aeschlimann, S., and Folts, J. D. (1999). Purple grape juice improves endothelial function and reduces the susceptibility of LDL cholesterol to oxidation in patients with coronary artery disease. Circulation 100 (10), 1050–1055. doi:10.1161/01.cir.100.10.1050
Thandapilly, S. J., LeMaistre, J. L., Xavier, L. L., Anderson, C. M., Thomas, N., and Anderson, H. D. (2012). Vascular and cardiac effects of grape powder in the spontaneously hypertensive rat. Am. J. Hypertens. 25 (10), 1070–1076. doi:10.1038/ajh.2012.98
Tianyou, W., Qianqian, F., Jiaxu, H., Zhan, C., Xujiao, Z., Jianhua, Z., et al. (2021). Therapeutic nanoparticles from grape seed for modulating oxidative stress. Small (Weinheim der Bergstrasse, Ger. 17 (45), e2102485. doi:10.1002/smll.202102485
Ting, H. C., Duo, S. Y., Zhang, L. Y., Xuan, X., Bin, C. Z., Lin, Q. S., et al. (2021). Oxidative stress in vascular calcification. Clin. Chim. Acta 519, 101–110. (prepublish). doi:10.1016/j.cca.2021.04.012
Tiwari, R., Mohan, M., Kasture, S., Maxia, A., and Ballero, M. (2009). Cardioprotective potential of myricetin in isoproterenol-induced myocardial infarction in Wistar rats. Phytother. Res. 23 (10), 1361–1366. doi:10.1002/ptr.2688
Tofani, I., Maki, K., Kojima, K., and Kimura, M. (2005). Beneficial effects of grape seed proanthocyanidins extract on formation of tibia bone in low-calcium feeding rats. Pediatr. Dent. J. 14 (1), 47–53. doi:10.1016/s0917-2394(04)70008-7
Wallerath, T., Deckert, G., Ternes, T., Anderson, H., Li, H., Witte, K., et al. (2002). Resveratrol, a polyphenolic phytoalexin present in red wine, enhances expression and activity of endothelial nitric oxide synthase. Circulation 106 (13), 1652–1658. doi:10.1161/01.cir.0000029925.18593.5c
Wang, Z., Zou, J., Huang, Y., Cao, K., Xu, Y., and Wu, J. M. (2002). Effect of resveratrol on platelet aggregation in vivo and in vitro. Chin. Med. J. 115 (3), 378–380.
Wei, S., Zheng, Y., Zhang, M., Zheng, H., and Yan, P. (2018). Grape seed procyanidin extract inhibits adipogenesis and stimulates lipolysis of porcine adipocytes in vitro. J. Anim. Sci. 96 (7), 2753–2762. doi:10.1093/jas/sky158
Wei, Z., Ziqing, Y., Qiang, Z., Shuangfei, G., Yi, S., Tang, L., et al. (2018). Proanthocyanidins inhibit osteoclast formation and function by inhibiting the NF-κB and JNK signaling pathways during osteoporosis treatment. Biochem. Biophysical Res. Commun. 509 (1), 294. doi:10.1016/j.bbrc.2018.12.125
Wenbo, N., Qian, X., Xuejiao, W., Junqiao, Z., Jinheng, L., Xiaomei, L., et al. (2021). A biomimetic drug delivery system by integrating grapefruit extracellular vesicles and doxorubicin-loaded heparin-based nanoparticles for glioma therapy. Nano Lett. 21 (3), 1484–1492. doi:10.1021/acs.nanolett.0c04753
Xiao, J., and Högger, P. (2014). Influence of diabetes on the pharmacokinetic behavior of natural polyphenols. Curr. Drug Metab. 15 (1), 23–29. doi:10.2174/1389200214666131210142614
Yağız, S., Kaan, K. O., Turhan, B. B., Avşar, A. E., Neslihan, T. P., Fikrettin, Ş., et al. (2021). Grapefruit-derived extracellular vesicles as a promising cell-free therapeutic tool for wound healing. Food Funct. 12, 5144–5156. doi:10.1039/d0fo02953j
Yanni, A. E., Vissarion, E., Pavlos, L., George, A., Nikolaos, K., and KarathanosVT, (2015). Effects of dietary Corinthian currants (Vitis vinifera L., var. Apyrena) on atherosclerosis and plasma phenolic compounds during prolonged hypercholesterolemia in New Zealand White rabbits. Food Funct. 6 (3), 963–971. doi:10.1039/c4fo01106f
Yee-Ling, T., and Shun-Wan, C. (2014). A review of the pharmacological effects of piceatannol on cardiovascular diseases. Phytother. Res. 28 (11), 1581–1588. doi:10.1002/ptr.5185
Yeung, F., Hoberg, J. E., Ramsey, C. S., Keller, M. D., Jones, D. R., Frye, R. A., et al. (2004). Modulation of NF-kappaB-dependent transcription and cell survival by the SIRT1 deacetylase. EMBO J. 23 (12), 2369–2380. doi:10.1038/sj.emboj.7600244
Yin, W., Li, B., Li, X., Yu, F., Cai, Q., Zhang, Z., et al. (2015). Anti-inflammatory effects of grape seed procyanidin B2 on a diabetic pancreas. Food Funct. 6 (9), 3065–3071. doi:10.1039/c5fo00496a
Yun, T., Fangyi, X., Xiangcheng, Z., Jingyao, M., Mohammed, S., Xin, H., et al. (2021). Plant-derived exosomal microRNAs inhibit lung inflammation induced by exosomes SARS-CoV-2 Nsp12. Mol. Ther. 29 (8), 2424–2440. doi:10.1016/j.ymthe.2021.05.005
Yunxia, W., Yunan, W., Wanli, S., Yandi, W., Yini, C., Nuerdida, N., et al. (2020). Grape seed polyphenols ameliorated dextran sulfate sodium-induced colitis via suppression of inflammation and apoptosis. Pharmacology 105 (1-2), 9–18. doi:10.1159/000501897
Zhang, M., Viennois, E., Prasad, M., Zhang, Y., Wang, L., Zhang, Z., et al. (2016a). Edible ginger-derived nanoparticles: A novel therapeutic approach for the prevention and treatment of inflammatory bowel disease and colitis-associated cancer. Biomaterials 101, 321–340. doi:10.1016/j.biomaterials.2016.06.018
Zhang, M., Viennois, E., Xu, C., and Merlin, D. (2016b). Plant derived edible nanoparticles as a new therapeutic approach against diseases. Tissue barriers. 4 (2), e1134415. doi:10.1080/21688370.2015.1134415
Zhang, P., Li, Y., Du, Y., Li, G., Wang, L., and Zhou, F. (2016). Resveratrol ameliorated vascular calcification by regulating sirt-1 and Nrf2. Transpl. Proc. 48 (10), 3378–3386. doi:10.1016/j.transproceed.2016.10.023
Zhang, Z., Zheng, L., Zhao, Z., Shi, J., Wang, X., and Huang, J. (2014). Grape seed proanthocyanidins inhibit H2O2-induced osteoblastic MC3T3-E1 cell apoptosis via ameliorating H2O2-induced mitochondrial dysfunction. J. Toxicol. Sci. 39 (5), 803–813. doi:10.2131/jts.39.803
Keywords: grape exosome-like nanoparticles, vascular calcification, vascular smooth muscle cells, exosomes, osteogenic phenotype differentiation
Citation: Teng Y, He J, Zhong Q, Zhang Y, Lu Z, Guan T, Pan Y, Luo X, Feng W and Ou C (2022) Grape exosome-like nanoparticles: A potential therapeutic strategy for vascular calcification. Front. Pharmacol. 13:1025768. doi: 10.3389/fphar.2022.1025768
Received: 23 August 2022; Accepted: 07 October 2022;
Published: 21 October 2022.
Edited by:
Leonardo Elia, University of Brescia, ItalyReviewed by:
Stephanie Wales Tobin, Trent University, CanadaCopyright © 2022 Teng, He, Zhong, Zhang, Lu, Guan, Pan, Luo, Feng and Ou. This is an open-access article distributed under the terms of the Creative Commons Attribution License (CC BY). The use, distribution or reproduction in other forums is permitted, provided the original author(s) and the copyright owner(s) are credited and that the original publication in this journal is cited, in accordance with accepted academic practice. No use, distribution or reproduction is permitted which does not comply with these terms.
*Correspondence: Caiwen Ou, b3VjYWl3ZW5Ac211LmVkdS5jbg==; Weijing Feng, d2pmZW5nMTk5MEBzbXUuZWR1LmNu
Disclaimer: All claims expressed in this article are solely those of the authors and do not necessarily represent those of their affiliated organizations, or those of the publisher, the editors and the reviewers. Any product that may be evaluated in this article or claim that may be made by its manufacturer is not guaranteed or endorsed by the publisher.
Research integrity at Frontiers
Learn more about the work of our research integrity team to safeguard the quality of each article we publish.