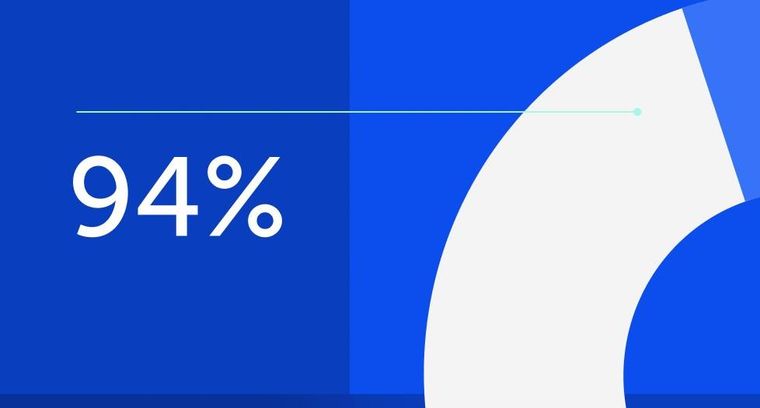
94% of researchers rate our articles as excellent or good
Learn more about the work of our research integrity team to safeguard the quality of each article we publish.
Find out more
REVIEW article
Front. Pharmacol., 12 October 2022
Sec. Experimental Pharmacology and Drug Discovery
Volume 13 - 2022 | https://doi.org/10.3389/fphar.2022.1017538
This article is part of the Research TopicPharmacological Mechanisms of Drugs Affecting Bone Formation and Bone ResorptionView all 16 articles
Bone reconstruction includes a steady state system of bone formation and bone absorption. This tight coupling requires subtle coordination between osteoblasts and osteoclasts. If this balance is broken, it will lead to bone mass loss, bone density reduction, and bone metabolic diseases, such as osteoporosis. Polyphenols in Chinese herbal medicines are active ingredients in plant extracts with high safety and few side effects, and they can play a role in affecting bone formation and bone resorption. Some of these have estrogen-like effects and can better target bone health in postmenopausal women. The purpose of this review is to provide comprehensive information on the mechanisms underlying the relationship between traditional Chinese medicine polyphenols and bone formation or bone resorption.
Bones are essential to the human body, providing structural support, protecting vital organs such as the bone marrow and brain, promoting blood production, and serving as a reservoir of minerals. From birth to death, bones are constantly reshaped to maintain critical functions and maintain constant changes in bone mass.
Bone remodeling is achieved through the tight coupling of bone resorption and bone formation, and this is closely related to the participation of osteoclasts and osteoblasts. Bone marrow-derived osteoclasts are responsible for the absorption of aged bone, and mesenchymal osteoblasts are responsible for the synthesis and mineralization of new bone. If this balance is broken, such as increased bone resorption that is not compensated for by a similar increase in bone formation, this will lead to bone mass loss, bone density reduction, and bone metabolic diseases, such as osteoporosis (Crotti et al., 2015). Bone remodeling is regulated by multiple local cytokines (e.g., platelet-derived growth factor (PDGF), insulin-like growth factor [IGF), beta tumor growth factor (TGF)], and systemic hormones (growth hormones, parathyroid hormone (PTH), insulin, and oxytocin), vitamin d, energy metabolism (Karsenty and Khosla, 2022), and the regulation of multiple signaling pathways. Among these, Wnt, TGFβ, RANK/RANKL, and the M-CSF/C-FMS pathway regulate the differentiation and activity of osteoclasts. The Runt-associated transcription factor 2 (Runx 2), Osterix (Osx), β-catenin, activating transcription factor 4 (Atf 4), and the activating protein 1 (AP-1) family are the primary transcription factors involved in osteoblast differentiation (Chau et al., 2009; Soltanoff et al., 2009; Meyer et al., 2014). However, recent research suggests that positioned bone is also an important organ with paracrine and endocrine functions. Moreover, there is crosstalk between osteoblasts and osteoclasts that allow them to communicate and influence each other. The sympathetic nervous system (SNS) also has an effect on bone balance (Karsenty and Khosla, 2022).
For the past 3 decades, the mainstay of treatment for osteoporosis has been antiresorptive agents (e.g., bisphosphonates) that reduce fracture risk through continuous administration. However, some epidemiological studies have shown an association between long-term bisphosphonate therapy and atypical femoral fractures (AFF) (Shane et al., 2014). Therefore, these drugs are not suitable for long-term use for the treatment of bone-damaging diseases, and they may not be suitable as oral drugs either. In addition, long-term medication can cause problems such as gastrointestinal (GI) toxicity, weight loss, bone pain, low calcium levels (Lu et al., 2016; Gao et al., 2017; Grigg et al., 2017; Lange et al., 2017; Monda et al., 2017). Hence, potential new drugs are urgently needed to replace existing treatment strategies due to clinically adverse effects (Estell and Rosen, 2021).
Chinese herbal medicine has been used for many centuries. Polyphenols are the active ingredients extracted from Chinese herbal medicine. A polyphenol is a type of plant component that widely exists in plants and contains a variety of hydroxyphenols. They are important secondary metabolites in plants, with polyphenol structures. Polyphenols are primarily found in the bark, roots, shells, leaves, and fruits of plants. Polyphenols can be divided into flavonoids, phenolic acids, lignans, and stilbenes according to their structure. As bioactive molecules, polyphenols derived from Chinese herbal medicines have been shown to have many effects on human health by acting on different biological systems. Polyphenols have many physiological activities such as anti-osteoporosis, anti-oxidation, anti-infection, anti-tumor, and anti-atherosclerosis activities. In addition, a large number of studies have shown the effectiveness of polyphenols in the treatment of bone related diseases (Tao et al., 2016; Zou et al., 2016; Chen et al., 2017). Polyphenols can play a role in bone reconstruction by affecting bone formation and bone resorption. Polyphenols act on osteoclasts, osteoblasts and bone marrow mesenchymal stem cells, regulate several important signal pathways, and play a role in bone remodeling. In addition, these polyphenols are low cost and have fewer adverse reactions. Therefore, they are more suitable for long-term use than synthetic drugs. Hence, their therapeutic potential would represent a new approach for future drug discovery and development based on polyphenol extracts from Chinese herbal medicines.
In this paper, the research progress of the specific mechanism of polyphenol compounds on bone formation and bone absorption is reviewed. This paper provides a theoretical basis for the basic research of polyphenol compounds on bone formation and bone absorption (Figures 1–3). In addition to the text of the polyphenols, we have summarized the main traditional Chinese medicine of polyphenols components (Table 1).
FIGURE 1. Chinese herbal polyphenols can play a role in bone reconstruction by affecting bone formation and bone resorption. After being absorbed in the gastrointestinal tract, Chinese medicine polyphenols act on human bones, and improve bone absorption and bone formation, contributing to bone remodeling and health, and reducing and improving adverse events.
TABLE 1. List of Chinese herbal polyphenols connected with the mechanism of bone formation or resorption.
Icariin.
Icariin (ICA) is 8-isopentenyl flavonoid glycoside and is the most abundant flavonoid active ingredient in epimedium. Bone marrow stromal cells (BMSCs) are stem cells isolated from adult bone marrow that have the ability to differentiate into osteoblasts, chondrocytes, adipocytes, and myoblasts. Epimedium has the ability to promote bone formation and can promote the proliferation of bone marrow mesenchymal stem cells and the differentiation of osteoblasts. At the level of the epigenetic regulation mechanism, ICA can regulate the homeostasis between osteogenic and adipogenic differentiation of mesenchymal stem cells (MSCs) through ABCB1 promoter demethylation (Sun et al., 2015). Similarly, it can also conduct epigenetic modification through miRNA. For example, studies have shown that ICA regulates the levels of Mir-23a-3p and Mir-335–5p and regulates the downstream pathway, thus affecting the osteogenic differentiation of BMSCs (Zhang et al., 2021; Teng et al., 2022). In addition, up-regulating the expression of Mir-335–5p and inhibiting phosphatase and tensin homolog deleted on chromosome ten (PTEN) can improve the susceptibility of osteoporosis (OP), thus providing new strategies for the prevention and treatment of OP (Teng et al., 2022). ICA can promote osteogenic differentiation by regulating the BMP/Smads pathway, the BMA1-BMP2 signaling pathway, and the BMP-2/Smad 5/Runx two and WNT/β-catenin pathways in BMSCs (Gao et al., 2021; Zhang et al., 2021; Jiao et al., 2022). Epimedium promotes the migration of bone marrow mesenchymal stem cells in vitro and in vivo through the MAPK signaling pathway (Jiao et al., 2018). In addition, ICA can promote the in-situ proliferation and osteogenic differentiation of bone marrow mesenchymal stem cells, thus improving the curative effect of bone marrow mesenchymal stem cell transplantation in the treatment of OP (Gao et al., 2021).
ICA can promote the differentiation of osteoblasts and increase bone mineral density. Bone formation primarily promotes the synthesis and mineralization of the bone matrix through the proliferation and differentiation of osteoblasts that play important roles in bone formation and osteoporosis. ICA has an estrogen-like pharmacological activity that can stimulate the differentiation and mineralization of osteoblasts, regulate the differentiation of osteoclasts, inhibit the adipogenic trans-differentiation of osteoblasts, and increase the number of osteoblasts differentiated into mature osteoblasts through the ER-mediated pathway (Zhang et al., 2016). In vivo, icariin increases the peak bone mass of rats during the growing period. Osteoblasts respond to icariin through the activation of cAMP/PKA/CREB signals. After the cAMP/PKA/CREB signal was blocked, icariin-induced osteogenesis was inhibited. These results further support that icariin promotes bone formation through the activation of the cAMP/PKA/CREB pathway (Shi et al., 2017). Icariin can also improve OP by regulating the balance of the EphB 4/ephrin-B2 pathway (Huang et al., 2020). Interestingly, ICA can also prevent the iron overload induced reduction of Runx2, alkaline phosphatase, and osteopontin expression, thereby inhibiting iron-induced osteoblast apoptosis and promoting bone formation (Jing et al., 2019). In addition, some studies have shown that icariin might exert an osteoprotective effect by maintaining osteocyte viability and thereby regulating bone remodeling (Feng et al., 2013; Ho et al., 2018; Park et al., 2019)
ICA inhibits the formation of osteoclasts, and ICA inhibits the differentiation of pre-osteoclasts to osteoclasts. It also inhibits the expression of various genes involved in osteoclast formation and bone resorption (Zhang S. et al., 2017). Studies have shown that icariin can block osteoclast formation induced by MCF seven and MDA-MB-231 breast cancer cells by inhibiting the activation of NF-κ B. In addition, icariin inhibits the expression of TRAF-6 stimulated by RANKL and then inhibits ERK phosphorylation, but it has no effect on the activation of p 38, JNK, and Akt (Kim et al., 2018). In addition, ICA can also prevent inflammatory bone loss. ICA inhibits the LPS-induced osteoclast formation process by inhibiting the activation of the p38 and JNK pathways (Hsieh et al., 2011).
In summary, ICA can prevent and treat osteoporosis by improving bone metabolism, promoting the differentiation of bone marrow mesenchymal stem cells, stimulating osteoblasts, and inhibiting osteoclast activity.
Bavachin (BA) is the extract of the Chinese medicine Psoralea corylifolia. BA may stimulate bone formation or have potential anti-osteoporosis activity (Wang et al., 2001). BA can obviously stimulate cell proliferation and promote the differentiation of osteoblasts. This function may be related to its characteristic structure, that is, the isoprene side chains connected in each of its molecular skeletons (Li et al., 2014). It has been shown that BA can reduce bone turnover by decreasing serum alkaline phosphatase, serum carboxy-terminal collagen crosslinks (CTX) levels, and the urine deoxypyridinoline (u-DPD)/creatinine ratio, and preventing OVX-induced urinary calcium and phosphorus excretion. Similarly, BA can reduce the contents of gamma-aminobutyric acid (GABA) and GABABRI in the femur, increase the bone mineral density, and reduce urinary calcium excretion, thus achieving the purpose of preventing and treating osteoporosis in vivo (Zhu et al., 2015).
BA may inhibit osteoclast differentiation through the NF-κB signaling pathway and the MAPK signaling pathway in vitro (Wei et al., 2022). BA treatment can inhibit osteoclast function and promote the up-regulation and down-regulation of the osteoclast marker gene, RANKL, and the osteoblast marker gene, OPG. Serum aminoterminal propeptide of type I procollagen (PINP) is widely considered as a biomarker for evaluating osteoblast activity (Hale et al., 2007). It was found that BA significantly improved the level of serum PINP. These results indicated that BA not only has estrogen-like effects, but also has beneficial effects on the function of osteoblasts. BA can prevent OVX-induced bone loss, but it does not affect uterine estrogen. This type of bone protection makes this a promising alternative to treat postmenopausal osteoporosis (PMOP) safely and effectively (Weng et al., 2015).
BA can achieve the purpose of anti-osteoporosis through a delicate balance of bone formation and bone resorption.
Formononetin (FO) is one of the primary isoflavones extracted from Astragalus membranaceus. Studies have shown that it can stimulate the formation of osteoblasts, thus increasing bone mass and improving the microstructure of bone. FO can regulate the expression of RANKL and OPG at the mRNA level, as well as related markers of osteogenic differentiation, thus promoting the mineralization potential of osteoblasts (Zaklos-Szyda et al., 2020). In addition, FO promoted bone regeneration in a mouse model of cortical bone defect in a manner similar to PTH and upregulated the expression of the predominant runt-related transcription factor 2 and osteocalcin (Singh et al., 2017). The research results showed that FO inhibited fat formation through the AMPK/β-catenin signal transduction pathway, thus improving the inverse relationship between osteoblasts and adipocytes and preventing obesity and bone loss induced by high-fat diets (Gautam et al., 2017).
FO can inhibit the activation of osteoclasts and plays an important role. Studies have shown that FO can inhibit the proliferation and differentiation of primary bone marrow mononuclear macrophages into osteoclasts and down-regulate the expression of proteins and genes related to the bone resorption function of osteoclasts, and this may be one of the mechanisms of FO in preventing and treating destruction and collapse in femoral head necrosis (Hong et al., 2020). FO attenuates osteoclast differentiation and calcium loss by regulating the transcription factor, AP-1, in type I diabetic mice, and it is expected to be a prospective drug for the treatment of osteoporosis (Jing et al., 2022).
The immunomodulatory activity of formononetin can prevent OVX-induced bone loss. In addition, the generation of osteoclasts and apoptosis of osteoblasts induced by IL-17 are inhibited (Mansoori et al., 2016). FO can reduce the production of osteoclasts by inhibiting the activation of NF-κ B, c-fos, and nuclear factors that activate the cytoplasmic one signal pathway induced by RANKL in T cells (Huh et al., 2014). FO also has estrogen-like effects that can inhibit bone loss caused by estrogen deficiency after menopause and improve the activity of alkaline phosphatase in OVX rats in vivo and in vitro (Ha et al., 2010).
In a word, FO can stimulate the formation of osteoblasts and inhibit the activation of osteoclasts.
Pueraria, originating from China, has a long history and is one of the most commonly used Chinese medicines in Asia. Due to its high isoflavone content, it has been widely used as a natural alternative to hormone replacement therapy for postmenopausal symptoms (Lee et al., 2021). Puerarin (PUE) is an isoflavone isolated from the pueraria root that is widely distributed in several organs related to aging, such as the hippocampus, femur, tibia, and mammary gland, after oral administration (Anukunwithaya et al., 2018). In addition to anti-inflammatory, antioxidant, and anti-diabetic effects (Xiao et al., 2011; Chen et al., 2018; Jeon et al., 2020), PUE also plays an important role in bone diseases such as OP. PUE can promote bone formation by influencing the expression of osteogenic related genes and promoting the formation of a mineralized matrix. PUE can significantly enhance alkaline phosphatase activity, mineralized matrix generation, and osteoblast-related protein expression levels. In addition, microCT imaging measurements demonstrated that PUE significantly promoted new bone formation (Yang et al., 2022). At the level of epigenetic modification, PUE regulates transcriptional expression related to bone formation through microRNA (Shan et al., 2018; Zhou et al., 2020). For example, PUE can regulate the up-regulation of Mir-155–3p to promote BMSCs differentiation and bone formation and increase bone mass in bone grafted rats.
Studies have shown that PUE also has a regulatory effect on bone resorption. PUE can down-regulate the mRNA levels of osteoclast marker genes CTR, CATH-K, NFATc1 and c-fos, indicating that PUE inhibits osteoclast cell function in vitro (Yang et al., 2019). In vitro, PUE attenuated bone resorption without impairing osteoclast viability and significantly prevented OVX-induced bone loss by inhibiting bone resorption without altering bone formation (Qiu et al., 2022). Furthermore, PUE inhibites RANKL-induced osteoclast activation, the bone resorption capacity, and F-actin ring formation in vitro with an increase in the PUE concentration (Yang et al., 2019). PUE may play a protective role in osteoclast-related osteolytic diseases. In vitro, PUE prevented RANCL-induced osteoclast differentiation, bone resorption, and F-actin ring formation, reduced phosphorylation of p65, and prevented P65 translocation from the cytoplasm to the nucleus in a concentration-dependent manner. PUE also decreased the expression of osteoclast specific factors (Tang et al., 2020). In vivo experiments, PUE significantly inhibited bone resorption mediated wear particles in a skull bone resorption model (Yang et al., 2019).
PUE can also prevent cell apoptosis through the HDAC1/HDAC3 signaling pathway and regulate the expression of HIF-1α, TIMP-3, and Bcl-2, thus playing an anti-osteoporosis role (Guo et al., 2019; Waqas et al., 2020). For osteoporosis, it also increases bone mass and inhibits osteoclast formation (Yang et al., 2018; Xiao et al., 2020). By enhancing osteogenesis and promoting bone formation, PUE also improves OVX-induced osteoporosis and lipid metabolism by regulating phospholipid metabolism and polyunsaturated fatty acid biosynthesis, thereby reducing adipogenic differentiation. In addition, activation of the Wnt pathway and inhibition of the PPARγ pathway promote adipogenesis in osteogenic differentiation of inactivated rat bone marrow mesenchymal stem cells (Li et al., 2022). For osteoporosis caused by postmenopausal estrogen deficiency, the results of a clinical trial showed that PUE was well tolerated for the short-term treatment of mild to severe menopausal symptoms in women. Kudzu root extract may benefit bone and cartilage health and may be a promising natural alternative to existing treatments for menopausal symptoms (Bihlet et al., 2021).
Recent studies have found that the microbiota plays an important role in regulating the skeletal microenvironment, thereby triggering anti-osteoporosis effects. Furthermore, intestinal microbiota can participate in the process of osteoporosis by inducing inflammatory reactions and changes in the autoimmune system. PUE treatment can improve the bone microenvironment and inhibit OVX-induced osteoporosis by regulating the release of short chain fatty acids (SCFAs) from intestinal flora and repairing the intestinal mucosal integrity (Li B. et al., 2020). In addition, serum pharmacokinetics suggest that pueraria root extract may undergo enterohepatic circulation (Mun et al., 2009).
Genistein is a natural isoflavone compound found in legumes and dentate plants. It is a phytoestrogen that makes up more than 60% of soy isoflavones (Nazari-Khanamiri and Ghasemnejad-Berenji, 2021). Its pharmacological properties make it a potential drug for treating a variety of conditions including postmenopausal symptoms, cancer, and bone, brain, and heart disease (Nazari-Khanamiri and Ghasemnejad-Berenji, 2021).
It is well known that genistein has been shown to stimulate bone formation by osteoblasts and inhibit bone resorption by osteoclasts, thereby increasing bone mass (Yamaguchi, 2012). Genistein improves bone healing by triggering the estrogen receptor α-mediated expression of osteogenesis-related genes and maturation of osteoblasts. The inhibition of ER expression was shown to immediately reduce the genistein-induced enhancement of mitochondrial energy production and osteoblast activation (Wu et al., 2020). Additionally, studies have shown that genistein promotes osteoblast differentiation and maturation by activating the ER, p38MAPK-Runx2, and NO/cGMP pathways and by inducing the osteoclastogenesis inhibitor, osteoprotegerin (OPG), and blocking the NF-κB signaling pathway, inhibiting osteoclast formation and bone resorption (Ming et al., 2013).
At the level of epigenetic modification, genistein counteracts NF-κB-induced osteoclast generation and downstream signaling by directly regulating the transcription of histone methyltransferases EzH2 and EzH1 (Kushwaha et al., 2022). Furthermore, there are clinical trials showing that supplementation with the dietary phytoestrogen genistein may be as effective as hormone replacement therapy in reducing bone loss associated with menopause without the associated bone loss side effects (Cotter and Cashman, 2003; Sansai et al., 2020). In this study, to improve the bioavailability of genistein and reduce its side effects, the nanofied formulation of genistein with Vitamin D was invented to enhance the therapeutic efficacy of the osteoporosis model in vitro and improve alkaline phosphatase activity and multinucleated giant cell formation (Kushwaha et al., 2022). If the bioavailability of genistein is improved, its future market development potential is huge.
Salvia miltiorrhiza Bunge, also known as Salvia miltiorhiza Bunge, is often used in traditional Chinese medicine (TCM) in combination with other traditional Chinese medicines to treat bone diseases. Salvianolic acid B (Sal B) is a water-soluble phenolic compound isolated from Salvia miltiorrhiza Bunge in which the active ingredient in the water-soluble phenolic compound is greater than 50% (Cao et al., 2012). As a polyphenolic acid compound with seven phenolic hydroxyl groups, Sal B is one of the strongest natural antioxidants, and it is metabolized into salvianol in vivo (Chen et al., 2013; Tang et al., 2014).
It is worth noting that Sal B can also act on osteoblasts and induce bone marrow-derived mesenchymal stem cells to become osteoblasts. Studies have shown that Sal B and Salvia miltiorrhiza can induce osteogenic differentiation of rat bone marrow stromal cells by up-regulating the nitric oxide pathway (Zhang X. et al., 2017). In addition, Sal B can protect osteoblasts treated with prednisolone acetate by stimulating the activity of osteoblasts and the expression of genes related to bone formation and differentiation. It can also increase the alkaline phosphatase (ALP) activity in osteoblasts and stimulate the expression of ALP, which is inhibited by prednisolone acetate, and up-regulate the expression of Runx2, Osx, OCN, IGF-I, Col-I, HO-I, mRNA, and protein expression (Qiao et al., 2019). For the first time, studies have shown that Sal B can target TAZ to promote osteogenesis and reduce adipogenesis by activating MEK-ERK signaling pathway, which provides evidence that Sal B can be used as a potential therapeutic agent for the management of bone diseases (Wang et al., 2019). Sal B can also play a cytoprotective role to inhibit the apoptosis of BMSCs by regulating H2O2-mediated ROS/MEK/ERK1/2 pathway (Lu et al., 2010). This indicated that Sal B had a protective effect on osteoblasts by stimulating osteoblast activity and the expression of genes related to bone formation and differentiation.
Flax lignans are phytonutrient extract of Linum usitatissimum L. Chemically, the C6-OH of the glucose of flax lignans is esterified to the carboxylic acid of hydroxymethylglutaric acid (Imran et al., 2015). Flax lignans in combination with low-dose estrogen treatment maximally prevents bone loss induced by oophorectomy (Sacco et al., 2009). However, its use alone has no effect on the bone mineral density content, and a clinical study showed no statistically significant difference in bone turnover markers between the treatment group and the placebo group (Alcorn et al., 2017). Flax lignans have no negative effects on bone strength and bone health in aged rats (Ward et al., 2001a). These studies indicate that supplementation with flaxseed may contribute to improving the bone properties of osteoporosis, and these predominantly protective effects may be attributed to flaxseed oil (predominantly ALA), not to the fractions of flax lignans (Ward et al., 2001b; Lucas et al., 2002; Cohen and Ward, 2005). Flax Lignans are characterized by anti-inflammatory, antioxidant, and neuroprotective properties (Watanabe et al., 2020; Asad et al., 2021; Wu et al., 2021).
Resveratrol (RES), a non-flavonoid polyphenolic organic compound and is a bioactive component in Rhizoma Polygoni Cuspidati. It is easily absorbed after oral administration and is excreted through the urine and feces after metabolism. A large number of experimental studies have shown that RES has antioxidant, anti-inflammatory, anti-cancer, and cardiovascular and cerebrovascular protection effects (Biswas et al., 2020; Thaung Zaw et al., 2021; De Luca et al., 2022; Dzator et al., 2022; Mahjabeen et al., 2022).
Previous studies have shown that RES also plays an important role in protecting and promoting early bone metabolism and differentiation through a mechanism similar to genistein that promotes osteoblast-mediated bone formation and inhibits osteoclast-stimulated bone formation (Tou, 2015). RES increased the serum OPG, femoral SIRTI, and β-catenin expression and significantly decreased the NF-κB ligand receptor activator (RANKL) by stimulating SIRT1 expression and Wnt/β-catenin signaling. Finally, the bone mass of the femur increased and the bone mineral density significantly increased (Wang et al., 2022). In addition, RES can also protect bone cells from some physicochemical damage. For example, studies have shown that RES pretreatment for 30 min can significantly prevent cadmium-induced apoptosis and attenuate ERK1/2 and JNK signaling by regulating ERK1/2 and JNK signaling. It also produces cadmium-induced inhibition of osteogenic differentiation (Mei et al., 2021).
The aging of mesenchymal stem cells (MSCs) and the associated decline of osteogenic function lead to the disruption of the balance between bone formation and resorption, which is the key pathogenesis of osteoporosis during aging. Recent data has shown that RES can improve the osteogenic differentiation of senescent BMMSCs, and long-term intermittent applications can enhance bone formation and compensate for bone loss. The specific mechanism is that RES up-regulates Mitofilin, promotes the transcription of mitochondrial autonomous genes, and restores cell metabolism through mitochondrial function (Lv et al., 2018). Mitofilin, also known as the mitochondrial inner membrane protein (IMT) or Mic60, is a core component of the mitochondrial contact site and crista tissue system (MICOS) (Li et al., 2016; Tarasenko et al., 2017). Mitofilin is indispensable for mitochondrial homeostasis and osteogenesis in bone marrow mesenchymal stem cells (Chen et al., 2008; Pietilä et al., 2010). Mitofilin deficiency leads to aging and bone loss in BMMSC (George et al., 2011; Sahin et al., 2011).
Relevant clinical trials have also proved the efficacy and safety of RES. A clinical study conducted at the Aarhus University Hospital showed that high-dose RSE supplements increased bone mineral density (BMD) and bone alkaline phosphatase in obese men, with positive effects on bones (Ornstrup et al., 2014). A 24-month RES (RESHAW) trial of healthy ageing in women showed that regular 75 mg resveratrol supplementation twice daily had the potential to slow bone loss in the lumbar spine and femoral neck, which is common at fracture sites in postmenopausal women without significant osteoporosis (Wong et al., 2020). However, a systematic review and meta-analysis showed that RES supplements did not show any significant effect on BMD or serum bone markers (Li Q. et al., 2021). Therefore, further research utilizing better organized multicenter randomized trials is necessary so that physicians can provide more advice for clinical decision-making.
The incidence of bone metabolic diseases has been increasing annually. The imbalance of bone formation and absorption is an important mechanism of bone metabolism related diseases. Chinese herbal medicine has been used for thousands of years, among which polyphenols are an important active ingredient. This paper reviewed how polyphenols in Chinese herbal medicine can help bone reconstruction and improve bone metabolism by affecting the balance between bone formation and bone absorption. Generally speaking, regarding the beneficial effects of polyphenols in bone metabolic diseases, due to the lack of multi-center randomized trials in polyphenols in this field, it is considered necessary to conduct human trials, and further research can be conducted in this research field.
All the authors listed in this article made direct and substantial contributions to the work and approved its publication.
Our work was supported by Guangdong Provincial Key Laboratory of Traditional Chinese Medicine Informatization (2021B1212040007), the National Natural Science Foundation of China (81603342), National Key R&D Program of China 2018YFC2002500, Foshan “Summit Plan” of building high-level hospitals, and the Administration of Traditional Chinese Medicine of Guangdong Province (No. 20171074), Guangdong Provincial Bureau of Traditional Chinese Medicine (20221107), Natural Science Foundation of Guangdong Province (2022A1515012641).
The authors declare that the research was conducted in the absence of any commercial or financial relationships that could be construed as a potential conflict of interest.
All claims expressed in this article are solely those of the authors and do not necessarily represent those of their affiliated organizations, or those of the publisher, the editors and the reviewers. Any product that may be evaluated in this article, or claim that may be made by its manufacturer, is not guaranteed or endorsed by the publisher.
Alcorn, J., Whiting, S., Viveky, N., Di, Y., Mansell, K., Fowler, S., et al. (2017). Protocol for a 24-week randomized controlled study of once-daily oral dose of flax lignan to healthy older adults. JMIR Res. Protoc. 6 (2), e14. doi:10.2196/resprot.6817
Algandaby, M. M. (2019). Crocin attenuates metabolic syndrome-induced osteoporosis in rats. J. Food Biochem. 43 (7), e12895. doi:10.1111/jfbc.12895
Anukunwithaya, T., Poo, P., Hunsakunachai, N., Rodsiri, R., Malaivijitnond, S., and Khemawoot, P. (2018). Absolute oral bioavailability and disposition kinetics of puerarin in female rats. BMC Pharmacol. Toxicol. 19 (1), 25. doi:10.1186/s40360-018-0216-3
Asad, B., Khan, T., Gul, F. Z., Ullah, M. A., Drouet, S., Mikac, S., et al. (2021). Scarlet flax Linum grandiflorum (L.) in vitro cultures as a new source of antioxidant and anti-inflammatory lignans. Molecules 26 (15), 4511. doi:10.3390/molecules26154511
Bihlet, A. R., Byrjalsen, I., Andersen, J. R., Simonsen, S. F., Mundbjerg, K., Helmer, B., et al. (2021). The efficacy and safety of multiple dose regimens of kudzu (pueraria lobata) root extract on bone and cartilage turnover and menopausal symptoms. Front. Pharmacol. 12, 760629. doi:10.3389/fphar.2021.760629
Biswas, P., Dellanoce, C., Vezzoli, A., Mrakic-Sposta, S., Malnati, M., Beretta, A., et al. (2020). Antioxidant activity with increased endogenous levels of vitamin C, E and A following dietary supplementation with a combination of glutathione and resveratrol precursors. Nutrients 12 (11), E3224. doi:10.3390/nu12113224
Cao, P. C., Xiao, W. X., Yan, Y. B., Zhao, X., Liu, S., Feng, J., et al. (2014). Preventive effect of crocin on osteoporosis in an ovariectomized rat model. Evid. Based. Complement. Altern. Med. 2014, 825181. doi:10.1155/2014/825181
Cao, W., Guo, X. W., Zheng, H. Z., Li, D. P., Jia, G. B., and Wang, J. (2012). Current progress of research on pharmacologic actions of salvianolic acid B. Chin. J. Integr. Med. 18 (4), 316–320. doi:10.1007/s11655-012-1052-8
Chau, J. F., Leong, W. F., and Li, B. (2009). Signaling pathways governing osteoblast proliferation, differentiation and function. Histol. Histopathol. 24 (12), 1593–1606. doi:10.14670/HH-24.1593
Chen, C. T., Shih, Y. R., Kuo, T. K., Lee, O. K., and Wei, Y. H. (2008). Coordinated changes of mitochondrial biogenesis and antioxidant enzymes during osteogenic differentiation of human mesenchymal stem cells. Stem Cells 26 (4), 960–968. doi:10.1634/stemcells.2007-0509
Chen, C. Y., Li, H., Yuan, Y. N., Dai, H. Q., and Yang, B. (2013). Antioxidant activity and components of a traditional Chinese medicine formula consisting of Crataegus pinnatifida and Salvia miltiorrhiza. BMC Complement. Altern. Med. 13, 99. doi:10.1186/1472-6882-13-99
Chen, S., Liang, H., Ji, Y., Kou, H., Zhang, C., Shang, G., et al. (2021). Curcumin modulates the crosstalk between macrophages and bone mesenchymal stem cells to ameliorate osteogenesis. Front. Cell Dev. Biol. 9, 634650. doi:10.3389/fcell.2021.634650
Chen, X., Ren, S., Zhu, G., Wang, Z., and Wen, X. (2017). Emodin suppresses cadmium-induced osteoporosis by inhibiting osteoclast formation. Environ. Toxicol. Pharmacol. 54, 162–168. doi:10.1016/j.etap.2017.07.007
Chen, X., Yu, J., and Shi, J. (2018). Management of diabetes mellitus with puerarin, a natural isoflavone from pueraria lobata. Am. J. Chin. Med. 46 (8), 1771–1789. doi:10.1142/s0192415x18500891
Cohen, S. L., and Ward, W. E. (2005). Flaxseed oil and bone development in growing male and female mice. J. Toxicol. Environ. Health. A 68 (21), 1861–1870. doi:10.1080/15287390500226516
Cotter, A., and Cashman, K. D. (2003). Genistein appears to prevent early postmenopausal bone loss as effectively as hormone replacement therapy. Nutr. Rev. 61 (10), 346–351. doi:10.1301/nr.2003.oct.346-351
Crotti, T. N., Dharmapatni, A. A., Alias, E., and Haynes, D. R. (2015). Osteoimmunology: Major and costimulatory pathway expression associated with chronic inflammatory induced bone loss. J. Immunol. Res. 2015, 281287. doi:10.1155/2015/281287
De Luca, A., Bellavia, D., Raimondi, L., Carina, V., Costa, V., Fini, M., et al. (2022). Multiple effects of resveratrol on osteosarcoma cell lines. Pharm. (Basel) 15 (3), 342. doi:10.3390/ph15030342
Dzator, J. S. A., Howe, P. R. C., Coupland, K. G., and Wong, R. H. X. (2022). A randomised, double-blind, placebo-controlled crossover trial of resveratrol supplementation for prophylaxis of hormonal migraine. Nutrients 14 (9), 1763. doi:10.3390/nu14091763
Estell, E. G., and Rosen, C. J. (2021). Emerging insights into the comparative effectiveness of anabolic therapies for osteoporosis. Nat. Rev. Endocrinol. 17 (1), 31–46. doi:10.1038/s41574-020-00426-5
Fayed, H. A., Barakat, B. M., Elshaer, S. S., Abdel-Naim, A. B., and Menze, E. T. (2019). Antiosteoporotic activities of isoquercitrin in ovariectomized rats: Role of inhibiting hypoxia inducible factor-1 alpha. Eur. J. Pharmacol. 865, 172785. doi:10.1016/j.ejphar.2019.172785
Feng, R., Feng, L., Yuan, Z., Wang, D., Wang, F., Tan, B., et al. (2013). Icariin protects against glucocorticoid-induced osteoporosis in vitro and prevents glucocorticoid-induced osteocyte apoptosis in vivo. Cell biochem. Biophys. 67 (1), 189–197. doi:10.1007/s12013-013-9533-8
Fu, L., Pan, F., and Jiao, Y. (2017). Crocin inhibits RANKL-induced osteoclast formation and bone resorption by suppressing NF-κB signaling pathway activation. Immunobiology 222 (4), 597–603. doi:10.1016/j.imbio.2016.11.009
Gao, J., Gao, C., Li, H., Wang, G. S., Xu, C., and Ran, J. (2017). Effect of zoledronic acid on reducing femoral bone mineral density loss following total hip arthroplasty: A meta-analysis from randomized controlled trails. Int. J. Surg. 47, 116–126. doi:10.1016/j.ijsu.2017.08.559
Gao, J., Xiang, S., Wei, X., Yadav, R. I., Han, M., Zheng, W., et al. (2021). Icariin promotes the osteogenesis of bone marrow mesenchymal stem cells through regulating sclerostin and activating the wnt/β-catenin signaling pathway. Biomed. Res. Int. 2021, 6666836. doi:10.1155/2021/6666836
Gautam, J., Khedgikar, V., Kushwaha, P., Choudhary, D., Nagar, G. K., Dev, K., et al. (2017). Formononetin, an isoflavone, activates AMP-activated protein kinase/β-catenin signalling to inhibit adipogenesis and rescues C57BL/6 mice from high-fat diet-induced obesity and bone loss. Br. J. Nutr. 117 (5), 645–661. doi:10.1017/S0007114517000149
George, S. K., Jiao, Y., Bishop, C. E., and Lu, B. (2011). Mitochondrial peptidase IMMP2L mutation causes early onset of age-associated disorders and impairs adult stem cell self-renewal. Aging Cell 10 (4), 584–594. doi:10.1111/j.1474-9726.2011.00686.x
Grigg, A., Butcher, B., Khodr, B., Bajel, A., Hertzberg, M., Patil, S., et al. (2017). An individualised risk-adapted protocol of pre- and post transplant zoledronic acid reduces bone loss after allogeneic stem cell transplantation: Results of a phase II prospective trial. Bone Marrow Transpl. 52 (9), 1288–1293. doi:10.1038/bmt.2017.108
Guo, C. J., Xie, J. J., Hong, R. H., Pan, H. S., Zhang, F. G., and Liang, Y. M. (2019). Puerarin alleviates streptozotocin (STZ)-induced osteoporosis in rats through suppressing inflammation and apoptosis via HDAC1/HDAC3 signaling. Biomed. Pharmacother. 115, 108570. doi:10.1016/j.biopha.2019.01.031
Guo, Q., Yang, J., Chen, Y., Jin, X., Li, Z., Wen, X., et al. (2020). Salidroside improves angiogenesis-osteogenesis coupling by regulating the HIF-1α/VEGF signalling pathway in the bone environment. Eur. J. Pharmacol. 884, 173394. doi:10.1016/j.ejphar.2020.173394
Ha, H., Lee, H. Y., Lee, J. H., Jung, D., Choi, J., Song, K. Y., et al. (2010). Formononetin prevents ovariectomy-induced bone loss in rats. Arch. Pharm. Res. 33 (4), 625–632. doi:10.1007/s12272-010-0418-8
Hale, L. V., Galvin, R. J., Risteli, J., Ma, Y. L., Harvey, A. K., Yang, X., et al. (2007). PINP: A serum biomarker of Bone formation in the rat. Bone 40 (4), 1103–1109. doi:10.1016/j.bone.2006.11.027
Ho, M. X., Poon, C. C., Wong, K. C., Qiu, Z. C., and Wong, M. S. (2018). Icariin, but not genistein, exerts osteogenic and anti-apoptotic effects in osteoblastic cells by selective activation of non-genomic ERα signaling. Front. Pharmacol. 9, 474. doi:10.3389/fphar.2018.00474
Hong, Y. B., Jiang, H., Wang, J. W., Yu, P. F., and You, W. L. (2020). Experimental study on the inhibition of Formononetin on the differentiation of osteoclasts induced by RANKL. Zhongguo Gu Shang 33 (1), 64–70. doi:10.3969/j.issn.1003-0034.2020.01.012
Hsieh, T. P., Sheu, S. Y., Sun, J. S., and Chen, M. H. (2011). Icariin inhibits osteoclast differentiation and bone resorption by suppression of MAPKs/NF-κB regulated HIF-1α and PGE(2) synthesis. Phytomedicine 18 (2-3), 176–185. doi:10.1016/j.phymed.2010.04.003
Huang, M., Wang, Y., and Peng, R. (2020). Icariin alleviates glucocorticoid-induced osteoporosis through EphB4/ephrin-B2 Axis. Evid. Based. Complement. Altern. Med. 2020, 2982480. doi:10.1155/2020/2982480
Huh, J. E., Lee, W. I., Kang, J. W., Nam, D., Choi, D. Y., Park, D. S., et al. (2014). Formononetin attenuates osteoclastogenesis via suppressing the RANKL-induced activation of NF-κB, c-Fos, and nuclear factor of activated T-cells cytoplasmic 1 signaling pathway. J. Nat. Prod. 77 (11), 2423–2431. doi:10.1021/np500417d
Hwang, Y. H., Kim, T., Kim, R., and Ha, H. (2018). The natural product 6-gingerol inhibits inflammation-associated osteoclast differentiation via reduction of prostaglandin E₂ levels. Int. J. Mol. Sci. 19 (7), E2068. doi:10.3390/ijms19072068
Imran, M., Ahmad, N., Anjum, F. M., Khan, M. K., Mushtaq, Z., Nadeem, M., et al. (2015). Potential protective properties of flax lignan secoisolariciresinol diglucoside. Nutr. J. 14, 71. doi:10.1186/s12937-015-0059-3
Jeon, Y. D., Lee, J. H., Lee, Y. M., and Kim, D. K. (2020). Puerarin inhibits inflammation and oxidative stress in dextran sulfate sodium-induced colitis mice model. Biomed. Pharmacother. 124, 109847. doi:10.1016/j.biopha.2020.109847
Ji, B., Zhang, Z., Guo, W., Ma, H., Xu, B., Mu, W., et al. (2018). Isoliquiritigenin blunts osteoarthritis by inhibition of bone resorption and angiogenesis in subchondral bone. Sci. Rep. 8 (1), 1721. doi:10.1038/s41598-018-19162-y
Jiao, F., Tang, W., Huang, H., Zhang, Z., Liu, D., Zhang, H., et al. (2018). Icariin promotes the migration of BMSCs in vitro and in vivo via the MAPK signaling pathway. Stem Cells Int. 2018, 2562105. doi:10.1155/2018/2562105
Jiao, F., Tang, W., Wang, J., Liu, D., Zhang, H., and Tang, D. (2022). Icariin promotes the repair of bone marrow mesenchymal stem cells in rabbit knee cartilage defects via the BMP/Smad pathway. Ann. Transl. Med. 10 (12), 691. doi:10.21037/atm-22-2515
Jing, W., Feng, L., Peng, K., Zhang, W., and Wang, B. (2022). Formononetin attenuates osteoclast differentiation and calcium loss by mediating transcription factor AP-1 in type I diabetic mice. J. Biochem. Mol. Toxicol. 36 (6), e23042. doi:10.1002/jbt.23042
Jing, X., Du, T., Chen, K., Guo, J., Xiang, W., Yao, X., et al. (2019). Icariin protects against iron overload-induced bone loss via suppressing oxidative stress. J. Cell. Physiol. 234 (7), 10123–10137. doi:10.1002/jcp.27678
Joyce, K. M., Wong, C. P., Scriven, I. A., Olson, D. A., Doerge, D. R., Branscum, A. J., et al. (2022). Isoliquiritigenin decreases bone resorption and osteoclast differentiation. Mol. Nutr. Food Res. 66 (11), e2100974. doi:10.1002/mnfr.202100974
Karsenty, G., and Khosla, S. (2022). The crosstalk between bone remodeling and energy metabolism: A translational perspective. Cell Metab. 34 (6), 805–817. doi:10.1016/j.cmet.2022.04.010
Karunarathne, W., Molagoda, I. M. N., Lee, K. T., Choi, Y. H., Jin, C. Y., and Kim, G. Y. (2021). Anthocyanins isolated from Hibiscus syriacus L. attenuate lipopolysaccharide-induced inflammation and endotoxic shock by inhibiting the TLR4/MD2-mediated NF-κB signaling pathway. Phytomedicine 91, 153237. doi:10.1016/j.phymed.2020.153237
Khan, K., Singh, A., Mittal, M., Sharan, K., Singh, N., Dixit, P., et al. (2012). [6]-Gingerol induces bone loss in ovary intact adult mice and augments osteoclast function via the transient receptor potential vanilloid 1 channel. Mol. Nutr. Food Res. 56 (12), 1860–1873. doi:10.1002/mnfr.201200200
Kim, B., Lee, K. Y., and Park, B. (2018). Icariin abrogates osteoclast formation through the regulation of the RANKL-mediated TRAF6/NF-κB/ERK signaling pathway in Raw264.7 cells. Phytomedicine 51, 181–190. doi:10.1016/j.phymed.2018.06.020
Kim, H. S., Suh, K. S., Sul, D., Kim, B. J., Lee, S. K., and Jung, W. W. (2012). The inhibitory effect and the molecular mechanism of glabridin on RANKL-induced osteoclastogenesis in RAW264.7 cells. Int. J. Mol. Med. 29 (2), 169–177. doi:10.3892/ijmm.2011.822
Klasik-Ciszewska, S., Kaczmarczyk-Sedlak, I., and Wojnar, W. (2016). Effect of glabridin and glycyrrhizic acid on histomorphometric parameters of bones in ovariectomized rats. Acta Pol. Pharm. 73 (2), 517–527.
Kushwaha, A. C., Mohanbhai, S. J., Sardoiwala, M. N., Jaganathan, M., Karmakar, S., and Roy Choudhury, S. (2022). Nanoemulsified genistein and vitamin D mediated epigenetic regulation to inhibit osteoporosis. ACS Biomater. Sci. Eng. 8 (9), 3810–3818. doi:10.1021/acsbiomaterials.2c00165
Lange, U., Classen, K., Muller-Ladner, U., and Richter, M. (2017). Weekly oral bisphosphonates over 2 years prevent bone loss in cardiac transplant patients. Clin. Transpl. 31 (11), e13122. doi:10.1111/ctr.13122
Lee, J., Noh, A. L., Zheng, T., Kang, J. H., and Yim, M. (2015). Eriodicyol inhibits osteoclast differentiation and ovariectomy-induced bone loss in vivo. Exp. Cell Res. 339 (2), 380–388. doi:10.1016/j.yexcr.2015.10.001
Lee, M. R., Kim, B., Lee, Y., Park, S. Y., Shim, J. H., Chung, B. H., et al. (2021). Ameliorative effects of pueraria lobata extract on postmenopausal symptoms through promoting estrogenic activity and bone markers in ovariectomized rats. Evid. Based. Complement. Altern. Med. 2021, 7924400. doi:10.1155/2021/7924400
Li, B., Liu, M., Wang, Y., Gong, S., Yao, W., Li, W., et al. (2020a). Puerarin improves the bone micro-environment to inhibit OVX-induced osteoporosis via modulating SCFAs released by the gut microbiota and repairing intestinal mucosal integrity. Biomed. Pharmacother. 132, 110923. doi:10.1016/j.biopha.2020.110923
Li, B., Wang, Y., Gong, S., Yao, W., Gao, H., Liu, M., et al. (2022). Puerarin improves OVX-induced osteoporosis by regulating phospholipid metabolism and biosynthesis of unsaturated fatty acids based on serum metabolomics. Phytomedicine. 102, 154198. doi:10.1016/j.phymed.2022.154198
Li, D., Wang, P., Luo, Y., Zhao, M., and Chen, F. (2017). Health benefits of anthocyanins and molecular mechanisms: Update from recent decade. Crit. Rev. Food Sci. Nutr. 57 (8), 1729–1741. doi:10.1080/10408398.2015.1030064
Li, H., Ruan, Y., Zhang, K., Jian, F., Hu, C., Miao, L., et al. (2016). Mic60/Mitofilin determines MICOS assembly essential for mitochondrial dynamics and mtDNA nucleoid organization. Cell Death Differ. 23 (3), 380–392. doi:10.1038/cdd.2015.102
Li, L., Wang, B., Li, Y., Li, L., Dai, Y., Lv, G., et al. (2020b). Celastrol regulates bone marrow mesenchymal stem cell fate and bone-fat balance in osteoporosis and skeletal aging by inducing PGC-1α signaling. Aging (Albany NY) 12 (17), 16887–16898. doi:10.18632/aging.103590
Li, Q., Yang, G., Xu, H., Tang, S., and Lee, W. Y. (2021a). Effects of resveratrol supplementation on bone quality: A systematic review and meta-analysis of randomized controlled trials. BMC Complement. Med. Ther. 21 (1), 214. doi:10.1186/s12906-021-03381-4
Li, W. D., Yan, C. P., Wu, Y., Weng, Z. B., Yin, F. Z., Yang, G. M., et al. (2014). Osteoblasts proliferation and differentiation stimulating activities of the main components of Fructus Psoraleae corylifoliae. Phytomedicine 21 (4), 400–405. doi:10.1016/j.phymed.2013.09.015
Li, X. H., Chen, F. L., and Shen, H. L. (2021b). Salidroside promoted osteogenic differentiation of adipose-derived stromal cells through Wnt/β-catenin signaling pathway. J. Orthop. Surg. Res. 16 (1), 456. doi:10.1186/s13018-021-02598-w
Lin, S. Y., Kang, L., Chen, J. C., Wang, C. Z., Huang, H. H., Lee, M. J., et al. (2019). (-)-Epigallocatechin-3-gallate (EGCG) enhances healing of femoral bone defect. Phytomedicine 55, 165–171. doi:10.1016/j.phymed.2018.07.012
Liu, J., Zhou, H., Song, L., Yang, Z., Qiu, M., Wang, J., et al. (2021). Anthocyanins: Promising natural products with diverse pharmacological activities. Molecules 26 (13), 3807. doi:10.3390/molecules26133807
Lu, B., Ye, Z., Deng, Y., Wu, H., and Feng, J. (2010). MEK/ERK pathway mediates cytoprotection of salvianolic acid B against oxidative stress-induced apoptosis in rat bone marrow stem cells. Cell Biol. Int. 34 (11), 1063–1068. doi:10.1042/CBI20090126
Lu, H., Champlin, R. E., Popat, U., Pundole, X., Escalante, C. P., Wang, X., et al. (2016). Ibandronate for the prevention of bone loss after allogeneic stem cell transplantation for hematologic malignancies: A randomized-controlled trial. Bonekey Rep. 5, 843. doi:10.1038/bonekey.2016.72
Lucas, E. A., Wild, R. D., Hammond, L. J., Khalil, D. A., Juma, S., Daggy, B. P., et al. (2002). Flaxseed improves lipid profile without altering biomarkers of bone metabolism in postmenopausal women. J. Clin. Endocrinol. Metab. 87 (4), 1527–1532. doi:10.1210/jcem.87.4.8374
Lv, Y. J., Yang, Y., Sui, B. D., Hu, C. H., Zhao, P., Liao, L., et al. (2018). Resveratrol counteracts bone loss via mitofilin-mediated osteogenic improvement of mesenchymal stem cells in senescence-accelerated mice. Theranostics 8 (9), 2387–2406. doi:10.7150/thno.23620
Mahjabeen, W., Khan, D. A., and Mirza, S. A. (2022). Role of resveratrol supplementation in regulation of glucose hemostasis, inflammation and oxidative stress in patients with diabetes mellitus type 2: A randomized, placebo-controlled trial. Complement. Ther. Med. 66, 102819. doi:10.1016/j.ctim.2022.102819
Mansoori, M. N., Tyagi, A. M., Shukla, P., Srivastava, K., Dev, K., Chillara, R., et al. (2016). Methoxyisoflavones formononetin and isoformononetin inhibit the differentiation of Th17 cells and B-cell lymphopoesis to promote osteogenesis in estrogen-deficient bone loss conditions. Menopause 23 (5), 565–576. doi:10.1097/GME.0000000000000646
Mariee, A. D., Abd-Allah, G. M., and El-Beshbishy, H. A. (2012). Protective effect of dietary flavonoid quercetin against lipemic-oxidative hepatic injury in hypercholesterolemic rats. Pharm. Biol. 50 (8), 1019–1025. doi:10.3109/13880209.2012.655424
Mei, W., Song, D., Wu, Z., Yang, L., Wang, P., Zhang, R., et al. (2021). Growth responses, accumulation, translocation and distribution of vanadium in tobacco and its potential in phytoremediation. Ecotoxicol. Environ. Saf. 214, 111297. doi:10.1016/j.ecoenv.2020.111297
Melough, M. M., Sun, X., and Chun, O. K. (2017). The role of AOPP in age-related bone loss and the potential benefits of berry anthocyanins. Nutrients 9 (7), E789. doi:10.3390/nu9070789
Meyer, M. B., Benkusky, N. A., and Pike, J. W. (2014). The RUNX2 cistrome in osteoblasts: Characterization, down-regulation following differentiation, and relationship to gene expression. J. Biol. Chem. 289 (23), 16016–16031. doi:10.1074/jbc.M114.552216
Ming, L. G., Chen, K. M., and Xian, C. J. (2013). Functions and action mechanisms of flavonoids genistein and icariin in regulating bone remodeling. J. Cell. Physiol. 228 (3), 513–521. doi:10.1002/jcp.24158
Monda, V., Lupoli, G. A., Messina, G., Peluso, R., Panico, A., Villano, I., et al. (2017). Improvement of bone physiology and life quality due to association of risedronate and anastrozole. Front. Pharmacol. 8, 632. doi:10.3389/fphar.2017.00632
Mun, J. G., Grannan, M. D., Lachcik, P. J., Reppert, A., Yousef, G. G., Rogers, R. B., et al. (2009). In vivo metabolic tracking of 14C-radiolabelled isoflavones in kudzu (Pueraria lobata) and red clover (Trifolium pratense) extracts. Br. J. Nutr. 102 (10), 1523–1530. doi:10.1017/s000711450999047x
Nani, A., Murtaza, B., Sayed Khan, A., Khan, N. A., and Hichami, A. (2021). Antioxidant and anti-inflammatory potential of polyphenols contained in mediterranean diet in obesity: Molecular mechanisms. Molecules 26 (4), 985. doi:10.3390/molecules26040985
Nazari-Khanamiri, F., and Ghasemnejad-Berenji, M. (2021). Cellular and molecular mechanisms of genistein in prevention and treatment of diseases: An overview. J. Food Biochem. 45 (11), e13972. doi:10.1111/jfbc.13972
Ornstrup, M. J., Harsløf, T., Kjær, T. N., Langdahl, B. L., and Pedersen, S. B. (2014). Resveratrol increases bone mineral density and bone alkaline phosphatase in obese men: A randomized placebo-controlled trial. J. Clin. Endocrinol. Metab. 99 (12), 4720–4729. doi:10.1210/jc.2014-2799
Park, B. K., Lee, J. H., Seo, H. W., Oh, K. S., Lee, J. H., and Lee, B. H. (2019). Icariin protects against radiation-induced mortality and damage in vitro and in vivo. Int. J. Radiat. Biol. 95 (8), 1094–1102. doi:10.1080/09553002.2019.1589021
Pietilä, M., Lehtonen, S., Närhi, M., Hassinen, I. E., Leskelä, H. V., Aranko, K., et al. (2010). Mitochondrial function determines the viability and osteogenic potency of human mesenchymal stem cells. Tissue Eng. Part C Methods 16 (3), 435–445. doi:10.1089/ten.tec.2009.0247
Qiao, J., Liu, A., Liu, J., Guan, D., and Chen, T. (2019). Salvianolic acid B (Sal B) alleviates the decreased activity induced by prednisolone acetate on osteoblasts by up-regulation of bone formation and differentiation genes. Food Funct. 10 (9), 6184–6192. doi:10.1039/c9fo01246j
Qiu, Z., Li, L., Huang, Y., Shi, K., Zhang, L., Huang, C., et al. (2022). Puerarin specifically disrupts osteoclast activation via blocking integrin-β3 Pyk2/Src/Cbl signaling pathway. J. Orthop. Transl. 33, 55–69. doi:10.1016/j.jot.2022.01.003
Sacco, S. M., Jiang, J. M., Reza-Lopez, S., Ma, D. W., Thompson, L. U., and Ward, W. E. (2009). Flaxseed combined with low-dose estrogen therapy preserves bone tissue in ovariectomized rats. Menopause 16 (3), 545–554. doi:10.1097/gme.0b013e31818fc00a
Sahin, E., Colla, S., Liesa, M., Moslehi, J., Müller, F. L., Guo, M., et al. (2011). Telomere dysfunction induces metabolic and mitochondrial compromise. Nature 470 (7334), 359–365. doi:10.1038/nature09787
Sansai, K., Na Takuathung, M., Khatsri, R., Teekachunhatean, S., Hanprasertpong, N., and Koonrungsesomboon, N. (2020). Effects of isoflavone interventions on bone mineral density in postmenopausal women: A systematic review and meta-analysis of randomized controlled trials. Osteoporos. Int. 31 (10), 1853–1864. doi:10.1007/s00198-020-05476-z
Shan, Z., Cheng, N., Huang, R., Zhao, B., and Zhou, Y. (2018). Puerarin promotes the proliferation and differentiation of MC3T3-E1 cells via microRNA-106b by targeting receptor activator of nuclear factor-κB ligand. Exp. Ther. Med. 15 (1), 55–60. doi:10.3892/etm.2017.5405
Shane, E., Burr, D., Abrahamsen, B., Adler, R. A., Brown, T. D., Cheung, A. M., et al. (2014). Atypical subtrochanteric and diaphyseal femoral fractures: Second report of a task force of the American society for bone and mineral research. J. Bone Min. Res. 29 (1), 1–23. doi:10.1002/jbmr.1998
Shen, Y. S., Chen, X. J., Wuri, S. N., Yang, F., Pang, F. X., Xu, L. L., et al. (2020). Polydatin improves osteogenic differentiation of human bone mesenchymal stem cells by stimulating TAZ expression via BMP2-Wnt/β-catenin signaling pathway. Stem Cell Res. Ther. 11 (1), 204. doi:10.1186/s13287-020-01705-8
Shi, W., Gao, Y., Wang, Y., Zhou, J., Wei, Z., Ma, X., et al. (2017). The flavonol glycoside icariin promotes bone formation in growing rats by activating the cAMP signaling pathway in primary cilia of osteoblasts. J. Biol. Chem. 292 (51), 20883–20896. doi:10.1074/jbc.M117.809517
Singh, K. B., Dixit, M., Dev, K., Maurya, R., and Singh, D. (2017). Formononetin, a methoxy isoflavone, enhances bone regeneration in a mouse model of cortical bone defect. Br. J. Nutr. 117 (11), 1511–1522. doi:10.1017/S0007114517001556
Soltanoff, C. S., Yang, S., Chen, W., and Li, Y. P. (2009). Signaling networks that control the lineage commitment and differentiation of bone cells. Crit. Rev. Eukaryot. Gene Expr. 19 (1), 1–46. doi:10.1615/critreveukargeneexpr.v19.i1.10
Song, F., Zhou, L., Zhao, J., Liu, Q., Yang, M., Tan, R., et al. (2016). Eriodictyol inhibits RANKL-induced osteoclast formation and function via inhibition of NFATc1 activity. J. Cell. Physiol. 231 (9), 1983–1993. doi:10.1002/jcp.25304
Sun, Z. B., Wang, J. W., Xiao, H., Zhang, Q. S., Kan, W. S., Mo, F. B., et al. (2015). Icariin may benefit the mesenchymal stem cells of patients with steroid-associated osteonecrosis by ABCB1-promoter demethylation: A preliminary study. Osteoporos. Int. 26 (1), 187–197. doi:10.1007/s00198-014-2809-z
Tan, L., Cao, Z., Chen, H., Xie, Y., Yu, L., Fu, C., et al. (2021). Curcumin reduces apoptosis and promotes osteogenesis of human periodontal ligament stem cells under oxidative stress in vitro and in vivo. Life Sci. 270, 119125. doi:10.1016/j.lfs.2021.119125
Tang, W., Xiao, L., Ge, G., Zhong, M., Zhu, J., Qin, J., et al. (2020). Puerarin inhibits titanium particle-induced osteolysis and RANKL-induced osteoclastogenesis via suppression of the NF-κB signaling pathway. J. Cell. Mol. Med. 24 (20), 11972–11983. doi:10.1111/jcmm.15821
Tang, Y., Jacobi, A., Vater, C., Zou, X., and Stiehler, M. (2014). Salvianolic acid B protects human endothelial progenitor cells against oxidative stress-mediated dysfunction by modulating Akt/mTOR/4EBP1, p38 MAPK/ATF2, and ERK1/2 signaling pathways. Biochem. Pharmacol. 90 (1), 34–49. doi:10.1016/j.bcp.2014.04.008
Tao, K., Xiao, D., Weng, J., Xiong, A., Kang, B., and Zeng, H. (2016). Berberine promotes bone marrow-derived mesenchymal stem cells osteogenic differentiation via canonical Wnt/β-catenin signaling pathway. Toxicol. Lett. 240 (1), 68–80. doi:10.1016/j.toxlet.2015.10.007
Tarasenko, D., Barbot, M., Jans, D. C., Kroppen, B., Sadowski, B., Heim, G., et al. (2017). The MICOS component Mic60 displays a conserved membrane-bending activity that is necessary for normal cristae morphology. J. Cell Biol. 216 (4), 889–899. doi:10.1083/jcb.201609046
Teng, J. W., Bian, S. S., Kong, P., and Chen, Y. G. (2022). Icariin triggers osteogenic differentiation of bone marrow stem cells by up-regulating miR-335-5p. Exp. Cell Res. 414 (2), 113085. doi:10.1016/j.yexcr.2022.113085
Thaung Zaw, J. J., Howe, P. R., and Wong, R. H. (2021). Long-term effects of resveratrol on cognition, cerebrovascular function and cardio-metabolic markers in postmenopausal women: A 24-month randomised, double-blind, placebo-controlled, crossover study. Clin. Nutr. 40 (3), 820–829. doi:10.1016/j.clnu.2020.08.025
Tou, J. C. (2015). Evaluating resveratrol as a therapeutic bone agent: Preclinical evidence from rat models of osteoporosis. Ann. N. Y. Acad. Sci. 1348 (1), 75–85. doi:10.1111/nyas.12840
Tsuji, M., Yamamoto, H., Sato, T., Mizuha, Y., Kawai, Y., Taketani, Y., et al. (2009). Dietary quercetin inhibits bone loss without effect on the uterus in ovariectomized mice. J. Bone Min. Metab. 27 (6), 673–681. doi:10.1007/s00774-009-0088-0
Wang, D., Li, F., and Jiang, Z. (2001). Osteoblastic proliferation stimulating activity of Psoralea corylifolia extracts and two of its flavonoids. Planta Med. 67 (8), 748–749. doi:10.1055/s-2001-18343
Wang, N., Li, Y., Li, Z., Liu, C., and Xue, P. (2019). Sal B targets TAZ to facilitate osteogenesis and reduce adipogenesis through MEK-ERK pathway. J. Cell. Mol. Med. 23 (5), 3683–3695. doi:10.1111/jcmm.14272
Wang, X., Lu, C., Chen, Y., Wang, Q., Bao, X., Zhang, Z., et al. (2022). Resveratrol promotes bone mass in ovariectomized rats and the SIRT1 rs7896005 SNP is associated with bone mass in women during perimenopause and early postmenopause. Climacteric 20 (7), 1–9. doi:10.1080/13697137.2022.2073809
Wang, Y., Bai, S., Cheng, Q., Zeng, Y., Xu, X., and Guan, G. (2021). Naringenin promotes SDF-1/CXCR4 signaling pathway in BMSCs osteogenic differentiation. Folia histochem. Cytobiol. 59 (1), 66–73. doi:10.5603/FHC.a2021.0008
Waqas, M., Qamar, H., Zhang, J., Yao, W., Li, A., Wang, Y., et al. (2020). Puerarin enhance vascular proliferation and halt apoptosis in thiram-induced avian tibial dyschondroplasia by regulating HIF-1α, TIMP-3 and BCL-2 expressions. Ecotoxicol. Environ. Saf. 190, 110126. doi:10.1016/j.ecoenv.2019.110126
Ward, W. E., Yuan, Y. V., Cheung, A. M., and Thompson, L. U. (2001a). Exposure to flaxseed and its purified lignan reduces bone strength in young but not older male rats. J. Toxicol. Environ. Health. A 63 (1), 53–65. doi:10.1080/152873901750128362
Ward, W. E., Yuan, Y. V., Cheung, A. M., and Thompson, L. U. (2001b). Exposure to purified lignan from flaxseed (Linum usitatissimum) alters bone development in female rats. Br. J. Nutr. 86 (4), 499–505. doi:10.1079/bjn2001429
Watanabe, Y., Ohata, K., Fukanoki, A., Fujimoto, N., Matsumoto, M., Nessa, N., et al. (2020). Antihypertensive and renoprotective effects of dietary flaxseed and its mechanism of action in deoxycorticosterone acetate-salt hypertensive rats. Pharmacology 105 (1-2), 54–62. doi:10.1159/000502789
Wei, C., Zhu, X., Lu, J., Xia, C., and Li, W. (2022). Effects and mechanism of bavachin on osteoclast differentiation. J Chin. J. Osteoporos. 28 (07), 942–947.
Weng, Z. B., Gao, Q. Q., Wang, F., Zhao, G. H., Yin, F. Z., Cai, B. C., et al. (2015). Positive skeletal effect of two ingredients of Psoralea corylifolia L. on estrogen deficiency-induced osteoporosis and the possible mechanisms of action. Mol. Cell. Endocrinol. 417, 103–113. doi:10.1016/j.mce.2015.09.025
Wong, R. H., Thaung Zaw, J. J., Xian, C. J., and Howe, P. R. (2020). Regular supplementation with resveratrol improves bone mineral density in postmenopausal women: A randomized, placebo-controlled trial. J. Bone Min. Res. 35 (11), 2121–2131. doi:10.1002/jbmr.4115
Wu, G. J., Chen, J. T., Cherng, Y. G., Chang, C. C., Liu, S. H., and Chen, R. M. (2020). Genistein improves bone healing via triggering estrogen receptor alpha-mediated expressions of osteogenesis-associated genes and consequent maturation of osteoblasts. J. Agric. Food Chem. 68 (39), 10639–10650. doi:10.1021/acs.jafc.0c02830
Wu, Z., Wu, B., Lv, X., Xie, Y., Xu, S., Ma, C., et al. (2021). Serumal lipidomics reveals the anti-inflammatory effect of flax lignans and sinapic acid in high-fat-diet-fed mice. J. Agric. Food Chem. 69 (32), 9111–9123. doi:10.1021/acs.jafc.0c07291
Xi, J., Li, Q., Luo, X., Wang, Y., Li, J., Guo, L., et al. (2018). Celastrol inhibits glucocorticoidinduced osteoporosis in rat via the PI3K/AKT and Wnt signaling pathways. Mol. Med. Rep. 18 (5), 4753–4759. doi:10.3892/mmr.2018.9436
Xiao, C., Li, J., Dong, X., He, X., Niu, X., Liu, C., et al. (2011). Anti-oxidative and TNF-α suppressive activities of puerarin derivative (4AC) in RAW264.7 cells and collagen-induced arthritic rats. Eur. J. Pharmacol. 666 (1-3), 242–250. doi:10.1016/j.ejphar.2011.05.061
Xiao, L., Zhong, M., Huang, Y., Zhu, J., Tang, W., Li, D., et al. (2020). Puerarin alleviates osteoporosis in the ovariectomy-induced mice by suppressing osteoclastogenesis via inhibition of TRAF6/ROS-dependent MAPK/NF-κB signaling pathways. Aging (Albany NY) 12 (21), 21706–21729. doi:10.18632/aging.103976
Xing, L. Z., Ni, H. J., and Wang, Y. L. (2017). Quercitrin attenuates osteoporosis in ovariectomized rats by regulating mitogen-activated protein kinase (MAPK) signaling pathways. Biomed. Pharmacother. 89, 1136–1141. doi:10.1016/j.biopha.2017.02.073
Xu, D., Hu, M. J., Wang, Y. Q., and Cui, Y. L. (2019). Antioxidant activities of quercetin and its complexes for medicinal application. Molecules 24 (6), E1123. doi:10.3390/molecules24061123
Xu, Q., Chen, G., Xu, H., Xia, G., Zhu, M., Zhan, H., et al. (2021). Celastrol attenuates RANKL-induced osteoclastogenesis in vitro and reduces titanium particle-induced osteolysis and ovariectomy-induced bone loss in vivo. Front. Pharmacol. 12, 682541. doi:10.3389/fphar.2021.682541
Yamaguchi, M. (2012). Nutritional factors and bone homeostasis: Synergistic effect with zinc and genistein in osteogenesis. Mol. Cell. Biochem. 366 (1-2), 201–221. doi:10.1007/s11010-012-1298-7
Yang, C., Li, J., Zhu, K., Yuan, X., Cheng, T., Qian, Y., et al. (2019). Puerarin exerts protective effects on wear particle-induced inflammatory osteolysis. Front. Pharmacol. 10, 1113. doi:10.3389/fphar.2019.01113
Yang, C., Zhu, K., Yuan, X., Zhang, X., Qian, Y., and Cheng, T. (2020). Curcumin has immunomodulatory effects on RANKL-stimulated osteoclastogenesis in vitro and titanium nanoparticle-induced bone loss in vivo. J. Cell. Mol. Med. 24 (2), 1553–1567. doi:10.1111/jcmm.14842
Yang, X., Yang, Y., Zhou, S., Gong, X., Dai, Q., Zhang, P., et al. (2018). Puerarin stimulates osteogenic differentiation and bone formation through the ERK1/2 and p38-MAPK signaling pathways. Curr. Mol. Med. 17 (7), 488–496. doi:10.2174/1566524018666171219101142
Yang, Y., Chen, D., Li, Y., Zou, J., Han, R., Li, H., et al. (2022). Effect of puerarin on osteogenic differentiation in vitro and on new bone formation in vivo. Drug Des. devel. Ther. 16, 2885–2900. doi:10.2147/dddt.S379794
Yuan, Y., Feng, G., Yang, J., Yang, C., and Tu, Y. H. (2022). Polydatin alleviates osteoporosis by enhancing the osteogenic differentiation of osteoblasts. Eur. Rev. Med. Pharmacol. Sci. 26 (12), 4392–4402. doi:10.26355/eurrev_202206_29078
Zaklos-Szyda, M., Budryn, G., Grzelczyk, J., Perez-Sanchez, H., and Zyzelewicz, D. (2020). Evaluation of isoflavones as bone resorption inhibitors upon interactions with receptor activator of nuclear factor-κb ligand (RANKL). Molecules 25 (1), 206. doi:10.3390/molecules25010206
Zang, L., Kagotani, K., Nakayama, H., Bhagat, J., Fujimoto, Y., Hayashi, A., et al. (2021). 10-Gingerol suppresses osteoclastogenesis in RAW264.7 cells and zebrafish osteoporotic scales. Front. Cell Dev. Biol. 9, 588093. doi:10.3389/fcell.2021.588093
Zhang, D., Fong, C., Jia, Z., Cui, L., Yao, X., and Yang, M. (2016). Icariin stimulates differentiation and suppresses adipocytic transdifferentiation of primary osteoblasts through estrogen receptor-mediated pathway. Calcif. Tissue Int. 99 (2), 187–198. doi:10.1007/s00223-016-0138-2
Zhang, R., Zhang, Q., Zou, Z., Li, Z., Jin, M., An, J., et al. (2021a). Curcumin supplementation enhances bone marrow mesenchymal stem cells to promote the anabolism of articular chondrocytes and cartilage repair. Cell Transpl. 30, 963689721993776. doi:10.1177/0963689721993776
Zhang, S., Feng, P., Mo, G., Li, D., Li, Y., Mo, L., et al. (2017a). Icariin influences adipogenic differentiation of stem cells affected by osteoblast-osteoclast co-culture and clinical research adipogenic. Biomed. Pharmacother. 88, 436–442. doi:10.1016/j.biopha.2017.01.050
Zhang, S. L., Chen, Z. H., Lin, D. T., Yan, Q., Gao, F., and Lin, H. (2021b). Epigallocatechin gallate regulates inflammatory responses and new bone formation through Wnt/β-Catenin/COX-2 pathway in spondyloarthritis. Int. Immunopharmacol. 98, 107869. doi:10.1016/j.intimp.2021.107869
Zhang, X. Y., Li, H. N., Chen, F., Chen, Y. P., Chai, Y., Liao, J. Z., et al. (2021). Icariin regulates miR-23a-3p-mediated osteogenic differentiation of BMSCs via BMP-2/Smad5/Runx2 and WNT/β-catenin pathways in osteonecrosis of the femoral head. Saudi Pharm. J. 29 (12), 1405–1415. doi:10.1016/j.jsps.2021.10.009
Zhang, X., Zou, L., Li, J., Xu, B., Wu, T., Fan, H., et al. (2017b). Salvianolic acid B and danshensu induce osteogenic differentiation of rat bone marrow stromal stem cells by upregulating the nitric oxide pathway. Exp. Ther. Med. 14 (4), 2779–2788. doi:10.3892/etm.2017.4914
Zheng, H., Qi, S., and Chen, C. (2018). Salidroside improves bone histomorphology and prevents bone loss in ovariectomized diabetic rats by upregulating the OPG/RANKL ratio. Molecules 23 (9), 2398. doi:10.3390/molecules23092398
Zhou, X., Zhang, Z., Jiang, W., Hu, M., Meng, Y., Li, W., et al. (2022). Naringenin is a potential anabolic treatment for bone loss by modulating osteogenesis, osteoclastogenesis, and macrophage polarization. Front. Pharmacol. 13, 872188. doi:10.3389/fphar.2022.872188
Zhou, Y., Lian, H., Liu, K., Wang, D., Xiu, X., and Sun, Z. (2020). Puerarin improves graft bone defect through microRNA‑155‑3p‑mediated p53/TNF‑α/STAT1 signaling pathway. Int. J. Mol. Med. 46 (1), 239–251. doi:10.3892/ijmm.2020.4595
Zhu, L., Wei, H., Wu, Y., Yang, S., Xiao, L., Zhang, J., et al. (2012). Licorice isoliquiritigenin suppresses RANKL-induced osteoclastogenesis in vitro and prevents inflammatory bone loss in vivo. Int. J. Biochem. Cell Biol. 44 (7), 1139–1152. doi:10.1016/j.biocel.2012.04.003
Zhu, Z., Sheng, H., Lin, Z., Ding, X., and liu, Y. (2015). Experimental study on the effect of bavachin on osteoporosis in ovariectomized rats. J. Shaanxi Tradit. Chin. Med. 36 (08), 1083–1084.
Keywords: Chinese herbal medicine, polyphenols, bone resorption, bone formation, osteoblast, osteoclast
Citation: Li Y, Li L, Li X, Luo B, Ye Q, Wang H, Yang L, Zhu X, Han L, Zhang R, Tian H and Wang P (2022) A mechanistic review of chinese medicine polyphenols on bone formation and resorption. Front. Pharmacol. 13:1017538. doi: 10.3389/fphar.2022.1017538
Received: 12 August 2022; Accepted: 03 October 2022;
Published: 12 October 2022.
Edited by:
Dongwei Zhang, Beijing University of Chinese Medicine, ChinaReviewed by:
Liming Xue, Shanghai Municipal Center for Disease Control and Prevention, ChinaCopyright © 2022 Li, Li, Li, Luo, Ye, Wang, Yang, Zhu, Han, Zhang, Tian and Wang. This is an open-access article distributed under the terms of the Creative Commons Attribution License (CC BY). The use, distribution or reproduction in other forums is permitted, provided the original author(s) and the copyright owner(s) are credited and that the original publication in this journal is cited, in accordance with accepted academic practice. No use, distribution or reproduction is permitted which does not comply with these terms.
*Correspondence: Ronghua Zhang, dHpyaEBqbnUuZWR1LmNu; Huaqin Tian, MTM5Mjk5NjkyNjJAMTM5LmNvbQ==; Panpan Wang, d2FuZ3BwQGpudS5lZHUuY24=
†These authors have contributed equally to this work and share first authorship
Disclaimer: All claims expressed in this article are solely those of the authors and do not necessarily represent those of their affiliated organizations, or those of the publisher, the editors and the reviewers. Any product that may be evaluated in this article or claim that may be made by its manufacturer is not guaranteed or endorsed by the publisher.
Research integrity at Frontiers
Learn more about the work of our research integrity team to safeguard the quality of each article we publish.