- 1Tianjin Key Laboratory of Biomedical Material, Institute of Biomedical Engineering, Chinese Academy of Medical Sciences and Peking Union Medical College, Tianjin, China
- 2Department of Gastroenterology, The Second Hospital of Tianjin Medical University, Tianjin, China
Ulcerative colitis (UC), a chronic, nonspecific inflammatory bowel disease characterized by continuous and diffuse inflammatory changes in the colonic mucosa, requires novel treatment method. Photodynamic therapy (PDT), as a promising physico-chemical treatment method, were used to treat UC rats’ model with novel photosensitizer LD4 in this paper, the treatment effect and mechanism was investigated. LD4-PDT could improve the survival rate of 2,4,6-trinitrobenzene sulfonic acid (TNBS)-induced UC model rats, decrease expression of interleukin (IL)-6, IL-1, tumor necrosis factor (TNF)-α, malondialdehyde (MDA), myeloperoxidase (MPO) and increase the expression of glutathione (GSH) and superoxide oxidase (SOD), while protecting the integrity of the intestinal epithelium. LD4-PDT treatment could rebuild the intestinal microflora composition and reprogram the colonic protein profiles in TNBS-induced rats to almost the normal state. Proteomics analysis based upon TNBS-induced UC model rats revealed that Amine oxidase copper-containing 1 (AOC1) was a potential target of LD4-PDT. Novel photosensitizer agent LD4-PDT represents an efficient treatment method for UC, and AOC1 may be a promising target.
Introduction
Ulcerative colitis (UC) is a chronic, nonspecific inflammatory disease of unknown etiology (Shivaji et al., 2020). The lesions are mainly located in the large intestine, mostly in the rectum and sigmoid colon, limited to the colonic mucosa and submucosa, showing continuous non-segmental distribution. The main acute clinical symptoms of UC are recurrent abdominal pain, diarrhea, and hematochezia. UC characterized by a wide range of lesions, complex pathogenesis, frequent recurrent attacks, and easy carcinogenesis, has been included in the list of modern refractory diseases by the World Health Organization (WHO). Its morbidity is related to infection, autoimmunity, heredity, and environment (Costello et al., 2019). With the development of economy and westernization of living habits and dietary composition, the incidence of UC in Asian countries is also increasing annually (Chow et al., 2009; Ng et al., 2017). Finding an effective treatment method of UC, to restore the patients’ physical and mental health and relieve the heavy economic burden brought by the disease to the patients’ families and society in general, has become a key scientific problem in clinical practice.
Currently, the clinical treatment of UC mainly focuses on anti-inflammatory, immune-regulatory or surgical treatments (Qiu et al., 2018; Ward et al., 2018), and pharmaceutical intervention has been the most adopted approach. Conventional therapeutic drugs mainly included amino salicylic acids, glucocorticoids, immunosuppressants, antibiotics and microecological agents. These drugs can only temporarily control and relieve symptoms, but cannot fundamentally cure the disease, and are accompanied by defects such as substantial toxicity and side effects, poor maintenance effect and frequent disease recurrence. Surgical treatment is mainly performed on patients with massive bleeding, intestinal perforation, canceration and toxic intestinal dilatation, and generally carries disadvantages such as large trauma, many complications, slow postoperative recovery and frequent disease recurrence (Hindryckx et al., 2015; Hirono et al., 2018; Ward et al., 2018). Therefore, development of an alternative therapy with good curative effect and fewer adverse reactions become a highly desire in UC research.
With the development of pharmaceutical biotechnology, many novel therapeutic methods have emerged, including biological targeted therapy, traditional Chinese medicine, stem cell therapy and photodynamic therapy (PDT) (Sands et al., 2019). Among them, PDT is a new technology being developed internationally. It can selectively act on target tissues and produce a photodynamic response via a photosensitizer (Mallidi et al., 2015). PDT has the advantages of rapid onset, strong targeting, low toxicity and side effects, and repeatable treatment. Recently, its clinical indications have been extended from malignant tumors to increasing numbers of benign diseases. The rapid development of endoscopy and fiber optics technology makes it possible to use PDT to treat gastrointestinal diseases and provides a new direction for the clinical treatment of UC. However, few reports regarding PDT treatment of UC (or inflammatory bowel disease). Favre and colleagues (Favre et al., 2011), using 5-aminolevulinic acid (5-ALA) as the photosensitizer to treat mice bearing Crohn’s disease, found that low dose PDT can down-regulate the expression of proinflammatory cytokines and induction of T-cell apoptosis to improve T-cell-mediated colitis with no significant side effects. Reinhard et al. (2015) treated dextran sulfate sodium (DSS)-induced inflammatory bowel disease in mice with the photosensitizer temoporfin and found that PDT could effectively reduce the symptoms of colitis and prevent intestinal cancer.
Despite their potential, photosensitizers were crucial in PDT. However 5-ALA itself has no photosensitive activity; instead it is transformed to protoporphyrin Ⅸ upon activation by ALA dehydrase, the concentration remains low, with uneven distribution and low efficiency of photodynamic reaction (Maisch et al., 2011). The poor solubility of temoporfin in water tends to cause adverse reactions such as neuralgia and suppression of the central nervous system (Senge, 2012). Therefore, it is necessary to develop a better photosensitizer for the treatment of UC with good solubility in water, high bioavailability, high photo response efficiency and fewer adverse reactions.
We designed and synthesized a series of alkaline amino acid modified amino tetraphenyl porphyrin compounds, all of which showed good physical and chemical properties (Meng et al., 2015). One of the compounds, 5,10,15,20-tetra{4-[(S)-2,6-diamino-hexamide] phenyl} porphyrin (LD4), with good water solubility, low toxicity and targeting characteristics, showed unique promotion of wound healing and immune regulation, as well inhibition of microbial pathogens in treatment of traumatic infection (Xu et al., 2016; Zhao et al., 2021). On the basis of these results, we sought to determine whether this photosensitizer could regulate the microflora and treat UC.
Amine oxidase copper-containing 1 (AOC1), an amine oxidase containing copper, catalyzes the degradation of compounds such as propylamine and spermine. Some reports have indicated that AOC1 was involved in allergy and immune responses, cell proliferation, tissue differentiation and cell apoptosis (Strolin Benedetti et al., 2007; Shepard and Dooley, 2015; Vakal et al., 2020). Although its mechanism of action in vivo is not fully understood, its role in immune cell transport makes it a target for autoimmune and inflammatory diseases (Peet et al., 2011). AOC1 is mainly distributed in the intestines and kidneys. Clinically, plasma AOC1 activity is used to diagnose intestinal integrity (DiSilvestro et al., 1997). Knockdown of AOC1 could inhibit the activation of protein kinase B (AKT) and the epithelial-mesenchymal transition (EMT) process (Xu et al., 2020). The aim of this study was to investigate the photodynamic therapeutic efficacy and molecular mechanism of LD4 in models of UC.
Materials and Methods
LD4 Synthesis and Characterization
Synthesis and characterization of LD4 was previously reported by our laboratory (Meng et al., 2015). The structure of LD4 is shown in Supplementary Figure S1A. The sample was excited by a 650 nm semiconductor laser (WSLS-650-500m-200M-H4; Wave spectrum Laser; China) through a columnar fiber. The energy density of the spot was measured using a light power meter (LM1; Carl Zeiss).
Experimental Animals
Male Sprague Dawley rats aged 6–8 weeks were purchased from HFK Biosciences Experimental Animals Company (SCXK 2019-0008, China) and raised in specific pathogen-free conditions. All animal experiments procedures were experimented according to the National Institutes of Health Guide for Care and Use of Laboratory Animals, and the protocol was approved by the Laboratory Animal Management Committee/Laboratory Animal Welfare Ethics Committee, Institute of Radiation Medicine, Chinese Academy of Medical Sciences (Approval No. IRM-DWLL-2017092). The ambient temperature was 22 ± 2°C, with relative air humidity of 40–70%, and food and sterile water were fed according to the experimental requirements.
Induction of Colitis
Rats were fasted for 24 h then anesthetized with 10% chloral hydrate before colitis induction. The UC model was induced using the method described by Morris et al. (1989), Wirtz et al. (2007). Briefly, 30 mg of TNBS (A28757; Innochem) in 0.25 ml of 50% ethanol was injected into rat colon through a 3 mm diameter polyethylene rubber catheter (inserted 8 cm into the proximal anal rectum). Rats were then maintained in a head low, tail high position for 1 min. Occult blood test paper was used to detect feces every day.
LD4-Photodynamic Therapy Treatment
The rats were randomly divided into six groups (10 rats per group) and treated as follows: 1, control group (normal saline); 2, TNBS group (TNBS enema); 3, LD4-PDTL group [TNBS and low-dose LD4 (60 μg/kg), both via enema]; 4, LD4-PDTM group [TNBS and medium-dose LD4 (120 μg/kg), both via enema]; 5, LD4-PDTH group [TNBS and high-dose LD4 (240 μg/kg), both via enema]; 6, SASP group [TNBS enema and positive control drug, SASP (500 mg/kg), gavage]. With the day of TNBS enema injection as day 0, treatment was initiated at day 7. LD4 was administered via enema every other day, while SASP was administered via gavage at the same time points, for a total of four treatments. Thirty minutes after each treatment, the colon was irradiated with an intensive 650 nm PDT system at an energy density of 25 J/cm2, excluding the SASP group, which was not irradiated. Body weight and food intake were daily measured. After all treatments were completed, fecal samples from each group were collected and stored at −80°C for further analysis of 16S rRNA. After 24 h of fasting, all rats were sacrificed and whole colons and blood were collected. The colon tissue was weighed, dissected and stored at −80°C. Parts of the tissue were also fixed with 4% paraformaldehyde. All rats in the experiment were treated according to guidelines set out in the National Institutes of Health’s Laboratory Animal Care and Use Guidelines.
FITC-Dextran Fluorescence Intensity Test
Intestinal permeability can be semi-quantitatively measured by detecting fluorescence intensity in serum using fluorescent tracers. Two hundred micrograms of FITC-dextran (FD40S; Sigma-Aldrich) powder were weighed and dissolved in 5 ml rat serum, diluted by doubling dilution method for 10 dilutions, and then fluorescence intensity was detected with a Varioskan Flash 3001 enzyme plate analyzer (Thermo Fisher, Waltham, MA) to obtain a standard curve. The standard curve were shown in Supplementary Figure S1C. Following LD4-PDT treatment, six rats from each group were fasted for 4 h before intragastric administration of FITC-dextran at a dose of 0.6 mg/g. Blood samples were collected before the animals were sacrificed and serum without hemolysis was collected. Sera were added to a 96-well plate, at 100 μl per well. Fluorescence intensity (Ex/Em: 488/520 nm) was measured with a Varioskan Flash 3001 enzyme plate analyzer. The FITC-dextran content in the rat sera was then calculated from the established standard curve.
Biochemical Analysis
Cytokines was correlated tightly with the occurrence of the infection, interleukin-6 (IL-6), tumor necrosis factor-α (TNF-α) and interleukin-1 (IL-1), while myeloperoxidase (MPO), malondialdehyde (MDA), glutathione (GSH) and superoxide dismutase (SOD) were correlated with oxidative stress reaction, which was often occurred in UC. So here we measured this cytokinesis to evaluate the efficacy of the treatment. IL-6 (SEA079Ra; Cloud-Clone Corp) and TNF-α (SEA133Ra; Cloud-Clone Corp) were quantified using commercially available ELISA kits. Standard curves are shown in Supplementary Figure S1D,E. MPO (A044-1-1; Nanjing Jiancheng Bioengineering Institute), GSH (A006-1-1; Nanjing Jiancheng Bioengineering Institute), MDA (A003-1-2; Nanjing Jiancheng Bioengineering Institute) and T-SOD (A001-1-2; Nanjing Jiancheng Bioengineering Institute levels in sera were determined by ELISA assay kits according to the manufacturer’s instructions.
Histopathological Analysis
Harvested colon tissue was dehydrated, embedded and sliced for histopathological analysis. Hematoxylin and eosin staining was performed as previously described (Zhang et al., 2020), and the extent of inflammation was scored according to the literature (Rong et al., 2018).
Bacterial Diversity Analysis
Fresh, uncontaminated feces were collected from six rats in different cages and stored at −80°C until use. DNA was extracted from feces and measured by Qubit Fluorometer. The mass concentration of the DNA library was greater than 1.0 ng/μl, which was of sufficient quality for use in subsequent experiments. Illumina MiSeq technology was used to amplify and sequence the V3–V4 region of the bacterial 16S rRNA gene. The bacterial 16S ribosomal (r) RNA forward primer sequence was 5′-CCTACGGGNGGCWGCAG-3′ and the reverse primer sequence was 5′-GACTACHVGGGTATCTAATCC-3′. USEARCH (http://www.drive5.com/usearch/7.0) was used to analyze the data. Bioinformatics analysis was performed according to the operational taxonomic unit (OTU). Similarity greater than 97% sequence clustering represented an OTU. The ribosomal database project (RDP) classifier was used to systematically classify OTU sequences with reference to the Silva database.
Protein Extraction and Quality Control
The colon tissue samples were lysed by addition of 600 μl of 8 M urea (lysate: protease inhibitor, 50:1), sonicated for 1 s, stopped for 2 s; this procedure was repeated for a total of 120 s. Samples were centrifuged at 14,000 × g for 20 min at 4°C. Protein was quantified by sodium dodecyl sulphate-polyacrylamide gel electrophoresis. The protein solution rapid prototype high performance liquid chromatograph (RP-HPLC) was separated using an RIGOL L-3000 system (Rigol Technologies, inc.; China) according to the manufacturer’s protocol.
Peptide Identification by Liquid Chromatography Mass Spectrometry (LC–MS/MS)
The colon tissue samples were lyophilized and ground into powder before being dissolved in 10 µL of 0.1% formic acid solution, centrifuged at 14,000 × g for 20 min at 4°C, then a 1 µg sample was taken for LC–MS/MS measurement. The label-free mass spectrum was analyzed by MaxQuant software and the protein data were screened by Beijing QLBio Company using the Uniprot database.
Cell Culture
HCoEpiC was cultured in RPMI 1640 (C11875500BT; Gibco) and supplemented with 10% fetal bovine serum (S711-001S; Lonsera) at 37°C in a humidified atmosphere with 5% CO2. The following viability experiment was performed in the same manner in all cell lines, with HCoEpiC cells described here as an example. HCoEpiC cells were divided into six groups randomly, marked as control, LPS (model), LD4-PDTL, LD4-PDTM, LD4-PDTH and Dexamethasone (DXMS) groups. All groups were cultured with 2 ml serum-free 1640 medium. Except for the control group, 10 μg/ml LPS was added to each group, and the cells were cultured for another 24 h. The culture medium was replaced with fresh 1640 medium for the control and model groups, while 2 ml serum-free 1640 medium containing 1.9, 3.8, or 7.5 μM LD4 was added to LD4-PDTL, LD4-PDTM and LD4-PDTH groups, respectively; 2 ml serum-free 1640 medium containing 7.5 μM DXMS was added to the DXMS positive control group. Cells were cultured with LD4 for 30 min. The time was sufficient for bacteria to take up LD4, as demonstrated in the bacterial strain deactivation previously reported (Xu et al., 2016; Zhao et al., 2021), so we chose this time to investigate the cellular damage occurring under the same conditions. Cells were irradiated with an energy density of 6 J/cm2 or kept in the dark for 30 min, following which the cells were cultured for a further 24 h before MTT assay.
Quantitative Real-Time PCR Assay
Total RNA in HCoEpiC cells was extracted using the TRIzol Reagent (15596018; Invitrogen) according to the manufacturer’s protocol. qRT-PCR was performed using a UltraSYBR mixture kit (CW0957H; Kangwei Biotech) according to the manufacturer’s instructions. The IL-1β, IL-6, TNF-α, AOC1, AKT, and NF-κB gene sequences were synthesized by Sangon Biotech (Shanghai, China). Primers are shown in Supplementary Table S1. qRT-PCR was performed using a LightCycler® real-time PCR assay (Roche, China).
Immunofluorescence Staining
HCoEpiC cells were grown on glass bottomed cell culture dishes (801001; NEST) and treated with LPS for 24 h. HCoEpiC cells were then cultured with LD4 for 30 min and irradiated with 650 nm laser light at an energy density of 6 J/cm2. Follow-up experiments were conducted at 24 h. Anti-AOC1(A6249; ABclonal) and fluorescein-conjugated goat anti-rabbit IgG (ZF-0311; ZSGB-BIO) at 1:100 dilution was added as previously described (Liu et al., 2019).
Western Blotting Analysis
RIPA (CW2333; CWBio) protein lysate was added to the culture plates containing treated cells as required to extract proteins. According to the molecular weight of the target protein, the corresponding prefabricated glue (C35502009; GenScript) was used, and the loading volume of the protein sample to be tested was 30–60 μg. Electrophoresis was performed at 150 V constant pressure for 50 min. A constant pressure of 100 V was set using the wet rotation method, and the film was transferred by ice bath for 2 h. The membrane was immersed in western blot blocking solution (232100; BD) and shaken gently at room temperature for 2 h. The following rabbit primary antibodies were diluted with TBST and prepared according to the manufacturer’s instructions; AOC1 (16338-1-AP; Proteintech), AOC1 (A6249; ABclonal), p-NF-κB (3033; CST), NF-κB (8242; CST), p-IκB (2859S; CST), IκB (4812; CST), IKK (2697; CST), AKT (4691; CST), p-AKT (4060; CST), p-IKK (ab178870; Abcam), IL-6 (WL02841; Wanleibio) and TNF-α (WL01581; Wanleibio).
Cell Transfection
HCoEpiC cells were cultured in six-well plates for 24 h and transfected with short hairpin (sh) RNA (Supplementary Table S2) and overexpressing plasmid vector. Sh-AOC1 inserted into the pGPU6/GFP/Neo vector and the total nucleotide sequence of AOC1 inserted into pEX-1 to obtain the plasmid. All plasmid were synthetized by Gene Pharma (Suzhou, China). Cells were cultured in Opti-mem medium (11058021; Gibco). All transfections were performed using lipofectamine 2000 (11668019; Invitrogen) according to the manufacturer’s protocol.
Statistical Analysis
Data analysis were conducted using SPSS 18.0 and GraphPad Prism 6. SPSS 18.0 was data statistical analysis software, used to analyze the differences between data. GraphPad Prism 6 was used to plot the corresponding statistics. All data were expressed as mean ± standard deviation (SD). Significant differences were determined using one-way analysis of variance (ANOVA). Statistical differences in 16S rRNA high-throughput sequencing were assessed using Tukey’s HSD (Li H. et al., 2020), Chen et al., 2020). p < 0.05 was considered statistically significant.
Results
LD4-Photodynamic Therapy Reduces Inflammation in LPS-Induced HCoEpiC Cells
Intestinal epithelial cells were the first protective barrier for the intestinal, its weakness in function would cause the illness in intestinal. So here HCoEpiC cells and LPS-stimulated HCoEpiC cells were chosen as normal and infectious cells in vitro model to evaluate the LD4-PDT in IBD. DXMS a medicine with efficacy like anti-inflammatory, immunosuppressive and other pharmacological effects, is widely used in the treatment of autoimmune diseases, allergies, inflammation and other diseases in clinics. Therefore, DXMS is often selected as a positive control drug in UC in vitro and in vivo. Exposure to less than 30 μM LD4 in either the dark or the light had no effect on the growth of HCoEpiC cells, indicating that LD4-PDT had no obvious toxicity to intestinal tissue cells (Supplementary Figure S1B). While LPS-stimulated HCoEpiC cells were sensitive to LD4-PDT, and their proliferation was inhibited in a LD4 dose-dependent manner (Figure 1A). Proinflammatory cytokine expression is often changed with the progress of UC. We therefore determined the levels of IL-6, TNF-α and IL-1 in HCoEpiC cells by western blotting and qRT-PCR. The protein and gene expressions of IL-1, IL-6 and TNF-α were significantly decreased in the LD4-PDT-treated group compared with control group (Figures 1B–F).
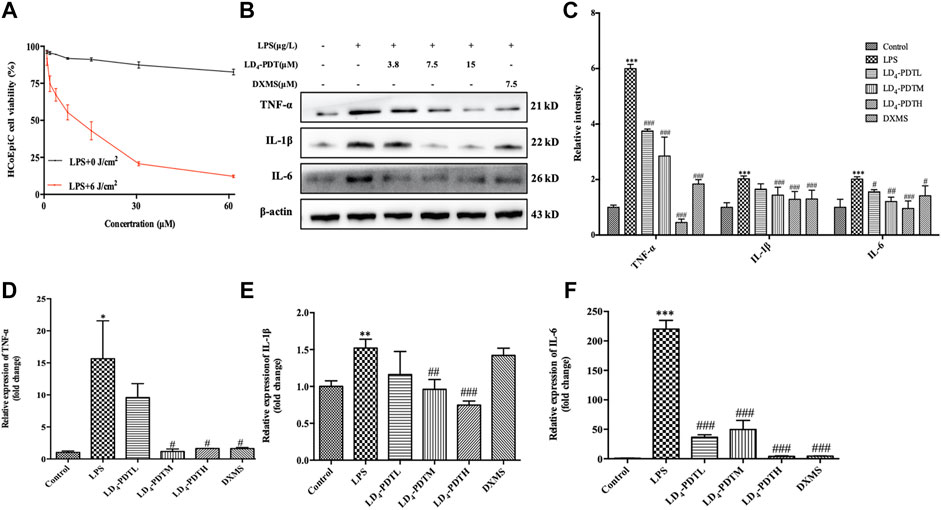
FIGURE 1. LD4-PDT reduced inflammation in LPS-induced HCoEpiC cells. (A) HCoEpiC cells were incubated with LPS for 24 h, LD4 (0–60 μM) for 30 min, then irradiated (6 J/cm2) or untreated. Cell viability was detected by MTT assay. Red line indicated the light reaction; black line indicated the dark reaction. (B,C) Protein expression of TNF-α, IL-6 and IL-1 was determined by western blot. (D–F) Expression levels of TNF-α, IL-6 and IL-1 in HCoEpiC cells were examined by qRT-PCR. **p < 0.01, ***p < 0.001 vs control group; #p < 0.05, ##p < 0.01, ###p < 0.001 vs LPS model group. Data are representative of three independent experiments, expressed as mean ± SD.
LD4-Photodynamic Therapy Treatment of TNBS-Induced UC Model Rats
Our previous research has shown that LD4 could inactivate microbial pathogens in traumatic infection ((Meng et al., 2015; Xu et al., 2016; Zhao et al., 2021). We have proved that the three different doses of LD4 used in this paper have no significant effect on normal rats and PDT alone did not significantly relieve UC (Supplementary Figure S1F,G). The occurrence and development of IBD is also closely related to the change of gut microbes. On the basis of these results, we suggested LD4-PDT could be used to treat UC. UC model was established using TNBS, and the treatment was started from day 7 post-induction, LD4 at doses of 60, 120, and 240 μg/kg was administrated via enema every second day, 30 min later after which the colon was irradiated with an intensive 650 nm PDT system at an energy density of 25 J/cm2, SASP, a standard UC drug as a positive control, was administered to the control group. The treatment was preformed four times. A steady increase in body weight in control group and body weight decrease in TNBS model group was observed, while body weight increasing in LD4-PDT or SASP treated rats (Figure 2A). After LD4-PDT treatment the animals were sacrificed and the colon was harvested. Its inner wall in the normal control group was complete, with regular folds and clear vascular texture, no obvious erosion, ulcers, or granuloma. In the TNBS model group, the colon intestine was shortened, the mucosa was marked with hyperemia and edema, scattered erosion or ulcers with hemorrhage, and large ulcerated areas. While the length of the colon and the thickness of the intestinal wall were improved to varying degrees in LD4-PDT-treated or SASP control groups (Figures 2B,C). Colon histopathology showed that in TNBS group, epithelial cells shedding off, inflammatory cell infiltration in the sub-membrane, crypt abscess and ulcer formation were observed locally, mucosal glands were disorganized, destroyed or absent, goblet cells were reduced or absent, and histological injury score was significantly increased. Compared with the model group, LD4-PDT-treated rats showed significantly alleviated pathological symptoms in the colon, and the colonic mucosal glands were arranged neatly, with few edemas and almost no ulcerative exudate (Figures 2D,E). The intestinal epithelial barrier is an important part of the intestinal innate immunity, and so measuring the permeability of the intestinal wall could determine disease activity. Following administration of FITC-dextran by intragastric gavage, the fluorescence intensity in serum indirectly reflects the intestinal permeability, the higher fluorescent intensity, the more severe the intestinal damage. Results showed that the levels of FITC-dextran in the serum of normal control rats were very low but significantly increased in TNBS groups, indicating that intestinal permeability was increased, and the intestinal wall was damaged following TNBS exposure. The content of FITC in serum of LD4-PDT- and SASP-treated groups was significantly decreased, indicating that these drugs had a protective effect on intestinal integrity (Figure 2F). In accordance with the results above, the expression of IL-1, IL-6 and TNF-α either in serum or in colon tissue were lower in LD4-PDT-treated rats compared with the TNBS group (Figures 2G–J).
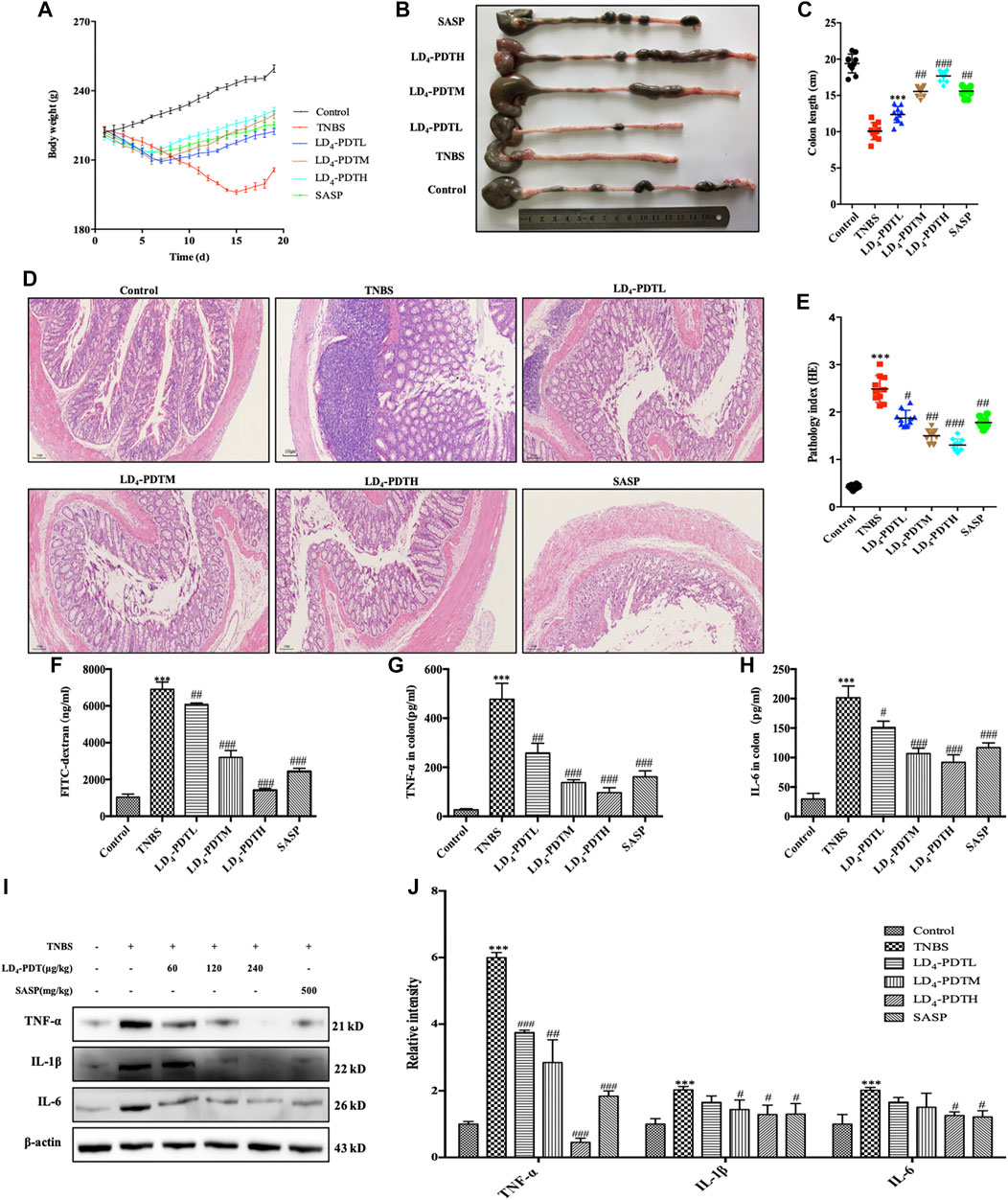
FIGURE 2. LD4-PDT alleviated TNBS-induced UC in model rats. (A) Body weight of UC model rats changed with time. (B,C) Length of colon. (D,E) Hematoxylin and eosin staining of colon tissue and inflammation score. (F) Serum fluorescent intensity of FITC-dextran. (G,H) TNF-α and IL-6 levels determined by ELISA. (I,J) Protein expression of TNF-α, IL-6 and IL-1 were determined by western blot analysis. Each column represents the mean ± SD of 10 rats per group. **p < 0.01, ***p < 0.001 vs control group; #p < 0.05, ##p < 0.01, ###p < 0.001 vs TNBS-treated group.
LD4-Photodynamic Therapy Regulates Oxidative Stress in TNBS-Induced UC Model Rats
Because there is a close relationship between oxidative stress and UC (Morris et al., 1989), the level of MPO and MDA in serum as indicators of oxidative stress were investigated. MPO and MDA were significantly increased in the TNBS group but decreased in LD4-PDT-treated rats in a dose dependent manner (Figures 3A,B), although levels in these animals remained higher than those of the normal control rats. Furthermore, the levels of two antioxidants GSH and SOD in serum were significantly decreased after TNBS exposure but increased significantly after LD4-PDT treatment (Figures 3C,D). These changes all indicated that the LD4-PDT treatment could improve the living state of UC rats.
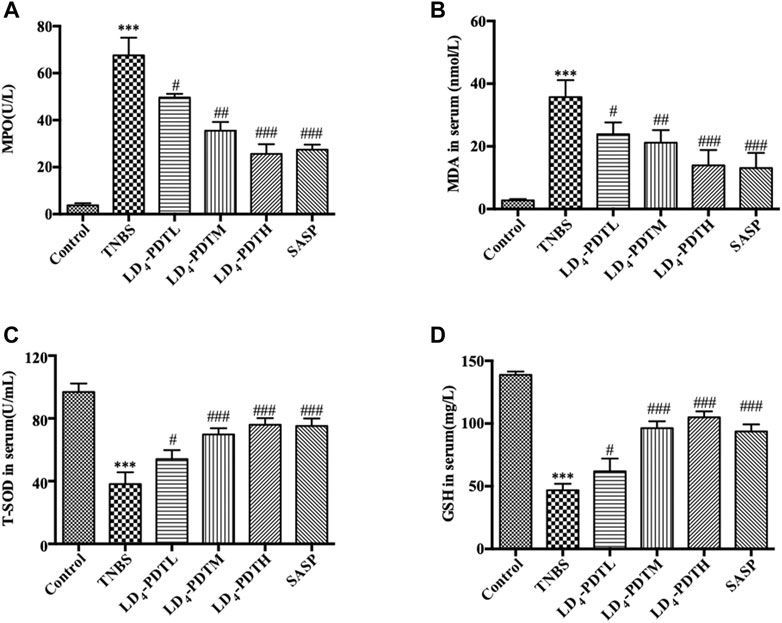
FIGURE 3. LD4-PDT regulated oxidative stress in TNBS-induced UC model rats. Concentrations of (A) MPO, (B) MDA, (C) SOD and (D) GSH in rat’s serum were examined by ELISA. **p < 0.01, ***p < 0.001 vs control group; #p < 0.05, ##p < 0.01, ###p < 0.001 vs TNBS model group. n = 10 rats per group.
LD4-Photodynamic Therapy Reprograms the Protein Profile of Colon Tissues in TNBS-Induced UC Model Rats
In order to investigate the mechanism of LD4-PDT in the treatment of UC, the related protein profile was analyzed based upon proteomics. Label-free proteomics was used to detect the protein profiles in colon tissues of rats in the control, TNBS model and LD4-PDT treatment groups. A t-test was used directly for differential analysis, and the differentially expressed proteins meeting the criteria of p ≤ 0.05 and fold change ≥1.5 times were screened. Scatter plots showed changes in protein expression between the TNBS model and LD4-PDT groups (Figure 4A). Compared with LD4-PDT-treated rats, there were 176 differentially expressed sites in the TNBS model group, among which 116 were highly expressed (Figure 4A, red) and 60 showed low expression (Figure 4A, green). Differentially expressed proteins are shown in Supplementary Figure 2A for comparison with other groups. Gene ontology (GO) is a standard vocabulary to describe the function, location and activity of genes, which covered three aspects of biology, namely biological process, cellular component, and molecular function. GO analysis of biological processes showed that differentially expressed proteins between the control and TNBS model groups were significantly related to collagen fibril organization, collagen biosynthesis, peptide acetyl threonine phosphorylation and negatively regulated biosynthesis by cell adhesion (Supplementary Figure S2B). However, treatment with LD4-PDT changed the colonic protein expression profiles (Figure 4B). In terms of cellular component, the differentially expressed proteins were related to intracellular organelles, collagen and immunoglobulin complexes (Supplementary Figure S2B), indicating that treatment with LD4-PDT resulted in changes in the cell cortex, intracellular organelles, and major histocompatibility complex class II proteins (Figure 4B). In terms of molecular function, the differentially expressed proteins were mainly derived from structural components of the extracellular matrix and the activities of ligase and transferase (Supplementary Figure S2B), while treatment with LD4-PDT changed the activities of oxygenase and hydrolase (Figure 4B). We screened 40 significantly different proteins using heat maps to illustrate the differing protein expression profiles in the colon samples. The results showed that expression of AOC1 in model rats was obviously increased (Supplementary Figure S2C), while this upregulation was eliminated following treatment with LD4-PDT (Figure 4C). The role of AOC1 in immune cell transport made it a target for autoimmune and inflammatory diseases, so we selected AOC1 for further study (Peet et al., 2011).
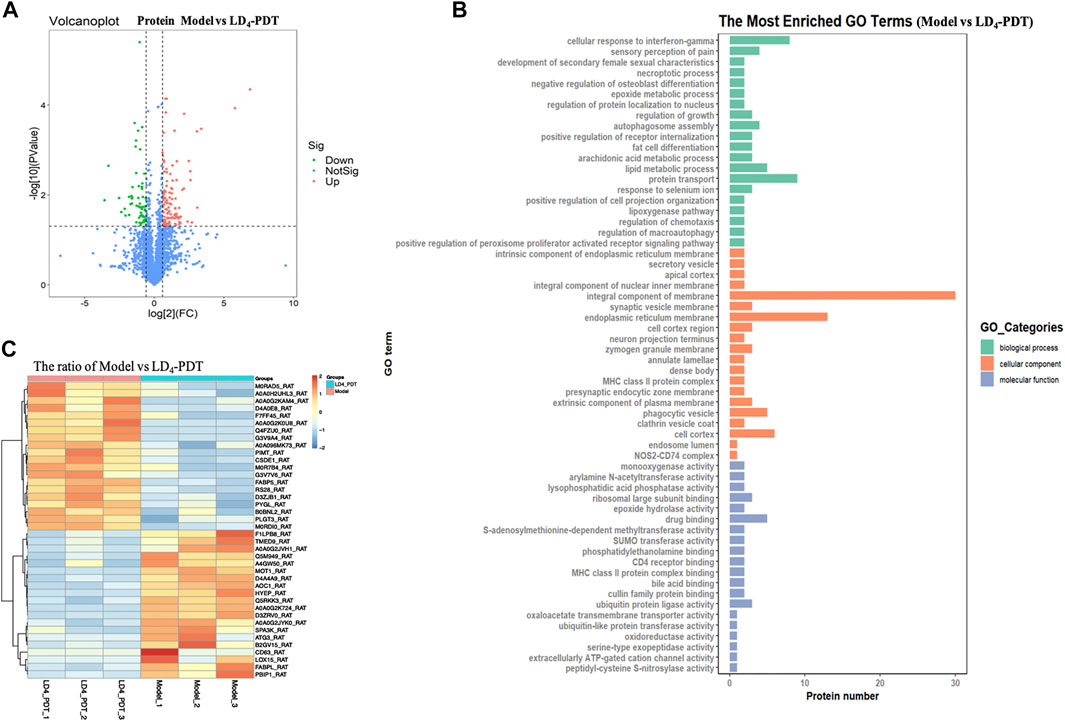
FIGURE 4. LD4-PDT reprogrammed the protein profile of colon tissues in TNBS-induced UC model rats. (A) Volcano plots showing the difference in protein expression among model and LD4-PDT groups. (B) All identified proteins were classified according to the first 20 gene ontology ratios sorted by enrichment degree [−log10 (p-value)] and protein number according to biological process, cell composition and molecular function. Over-representation analysis was used for functional enrichment analysis, and the t-test based on hypergeometric distribution. (C) Heat map of 40 differentially expressed proteins among the model and LD4-PDT groups. Each column represented a sample, and each row represented a factor; red indicated elevated protein expression, green indicated decreased protein expression.
LD4-Photodynamic Therapy Suppresses the Expression of AOC1/AKT/IKK/NF-κB in vitro and in vivo
Western blotting was used to further verify the expression of AOC1 protein. Compared with the control group, the expression of AOC1 was significantly increased in the TNBS model group but was significantly decreased after LD4-PDT treatment (Figure 5A). The expression of AOC1 in HCoEpiC cells was detected by western blot (Figure 5B), qRT-PCR (Figure 5C) and immunofluorescence (Figure 5D). Consistent with the results of the animal experiments, AOC1 protein and gene expression were up-regulated after LPS treatment in HCoEpiC cells but were then reduced upon LD4-PDT treatment. Because AOC1 can activate AKT and downstream pathways (Xu et al., 2020), the key factors interacting with AOC1 were screened. Western blotting showed that compared with the control group, the expression of p-AKT, p-NF-κB, p-IKK, and p-IκB was increased significantly in the TNBS group, while LD4-PDT treatment significantly decreased the expressions of p-AKT, p-NF-κB, p-IKK, and p-IκB compared with the TNBS model group (Figures 6A–D). Similarly, the expressions of p-AKT, p-NF-κB, p-IKK, and p-IκB were significantly decreased after LD4-PDT treatment compared with LPS stimulation alone in HCoEpiC cells (Figures 6E–I). Thus, our findings suggest that treatment with LD4-PDT may decrease or block the expression of AOC1/AKT/IKK/NF-κB in UC.
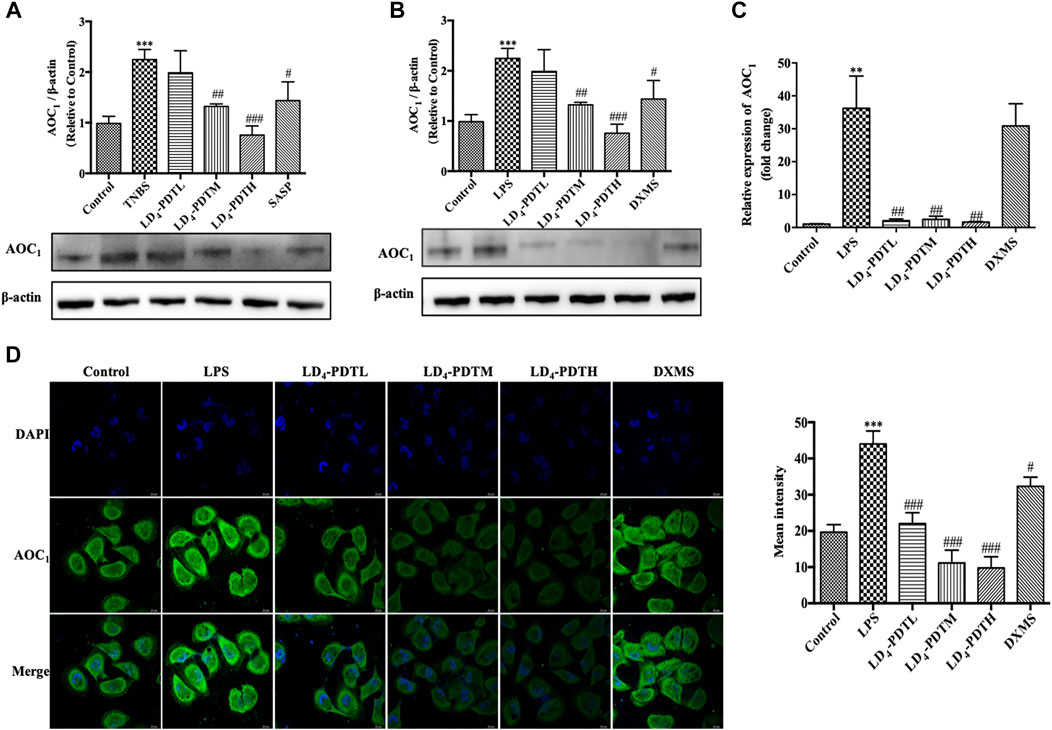
FIGURE 5. LD4-PDT suppressed the expression of AOC1. (A,B) Protein expression of AOC1 in colon tissue and in HCoEpiC cells were determined by western blot analysis. (C) Gene expression of AOC1 in HCoEpiC cells was examined by qRT-PCR. (D) Protein expression of AOC1 was detected by immunofluorescence. Bars, 20 μm **p < 0.01, ***p < 0.001 vs control group; #p < 0.05, ##p < 0.01, ###p < 0.001 vs TNBS model group. Data were representative of three independent experiments, expressed as mean ± SD.
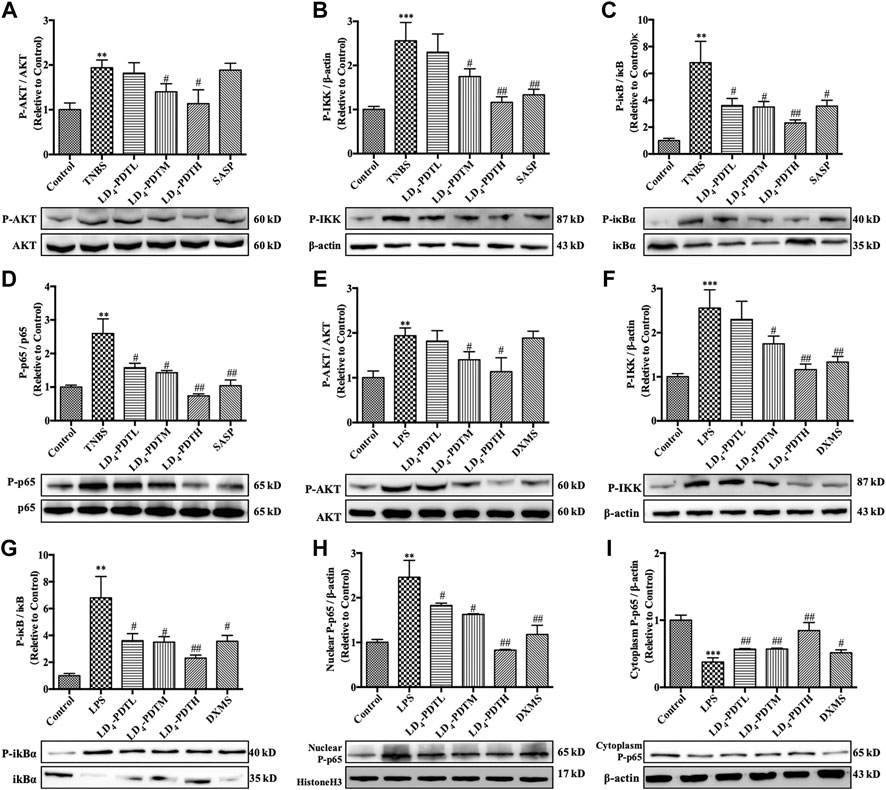
FIGURE 6. LD4-PDT inhibited the expression of AOC1/AKT/IKK/NF-κB. (A–D) Protein expression of (A) p-AKT, (B) p-IKK, (C) p-IκB and (D) p-p65 in colon tissue were determined by western blot. (E–I) Protein expression of (E) p-AKT, (F) p-IKK, (G) p-IκB, (H) p-p65 (in nuclear), and (I) p-p65 (in cytoplasm) in HCoEpiC cells were determined by western blot **p < 0.01, ***p < 0.001 vs control group; #p < 0.05, ##p < 0.01, ###p < 0.001 vs TNBS model or LPS model group. Data are representative of three independent experiments, expressed as mean ± SD.
LD4-Photodynamic Therapy Protects Intestine via Inhibition of AOC1
The above results indicated that AOC1 played an important role in LD4-PDT treatment of UC, so the impact of AOC1 protein in LD4-PDT treatment was further investigated. We knocked down the AOC1 gene in HCoEpiC cells using a specific shRNA then induced the inflammatory model with LPS before treating the cells with LD4-PDT. Western blot verified that more than 70% AOC1 was silenced (Supplementary Figure S3A), and knockdown AOC1 led to reduce the levels of IL-1, IL-6 and TNF-α in the cells to a varying degree compared with mock-transfected cells. Following LD4-PDT treatment, the expression levels of these cytokines in all groups was reduced remarkably, and there was no obvious difference among knockdown AOC1 group and other group (Figures 7A,B). On the contrary, overexpressed AOC1 cells was also built, the same treatment was conducted as that of the down-regulated cells (Supplementary Figure S3B). Western blot assay showed that compared with mock-transfected cells, protein levels of IL-1, IL-6 and TNF-α in the AOC1 overexpression cells were increased, while these cytokines all reduced following LD4-PDT treatment in all cells. Among all LD4-PDT treatment cells, AOC1 overexpression HCoEpiC cells exhibited the higher protein levels of IL-1, IL-6 and TNF-α (Figures 7C,D). We set AOC1-knockdown group and AOC1-overexpression group in HCoEpiC cells without LPS stimulation, as well as control cells group, to examine whether AOC1 is critical for cytokine production at the basal conditions. Our results demonstrated that AOC1 plays an important role in basal condition (Supplementary Figure S3C,D).Summary above, we found that the expression of AOC1 was up-regulated in the model group, while LD4-PDT treatment reduced the expression of AOC1 at the gene and protein levels, indicating that LD4-PDT exerted its effects in UC via AOC1, thus mediating expression of downstream cytokines. These results suggested that LD4-PDT treatment may work via inhibition of AOC1 to mediate AKT/IKK/NF-κB pathways, and thus knockdown of AOC1 protein either by LD4-PDT treatment or other means could alleviate UC symptoms.
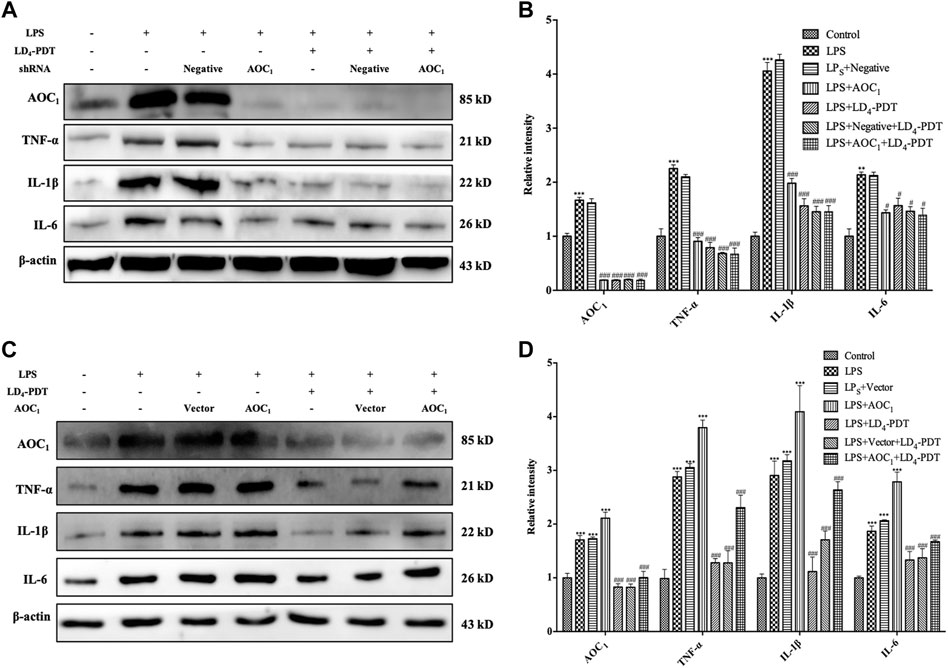
FIGURE 7. LD4-PDT protected intestine via inhibition of AOC1. (A,B) LD4-PDT effect on TNF-α, IL-6 and IL-1 expression in AOC1 knockdown HCoEpiC cells. (C,D) LD4-PDT effect on TNF-α, IL-6 and IL-1 expression in AOC1 over-expression cells. **p < 0.01, ***p < 0.001 vs control group; #p < 0.05, ##p < 0.01, ###p < 0.001 vs LPS groups. Data are representative of three independent experiments, expressed as mean ± SD.
LD4-Photodynamic Therapy Remodels the Gut Bacterial Composition Pattern in TNBS-Induced Ulcerative Colitis Model Rats
Effect of LD4-PDT on the gut microbes among the control, TNBS and LD4-PDT-treated groups were studied using the 16S rRNA method. The Venn diagram (Figure 8A) shows that a total of 2472 OTUs were obtained from all samples. A total of 589 OTUs were appeared in all three groups, while 990 OTUs were shared between the control and LD4-PDT groups, 64 OTUs were shared between the TNBS model and control groups, the TNBS model and LD4-PDT groups shared 60 (Figure 8A). The Shannon curve showed that sequencing depth covered rare new phylotypes, with both diversity and Shannon index in the TNBS model group being lower than those in the control and LD4-PDT groups (Supplementary Figure S4A). Weighted UniFrac-based principal coordinates analysis (PCoA) indicated the unique intestinal microbiota composition clustering of individual groups (Figure 8B). The α-diversity of intestinal bacteria decreased significantly in TNBS model rats, but it was recovered to an almost normal state after LD4-PDT treatment (Figure 8C, Supplementary Figure S4B). Unweighted pair group method with arithmetic mean analysis showed that there was a significant difference in intestinal flora between the TNBS model group and LD4-PDT-treated rats, and LD4-PDT treatment made the overall composition of intestinal flora in UC model rats return to a state similar with the control group (Figure 8D). Taxonomic bins at the phylum level indicated that gut bacterial composition patterns in model animals were obviously different from the control groups (Figure 8E): the proportions of Firmicutes in fecal stool of rats in the TNBS model group were higher than those in the normal control group (Figure 8F), while the abundance of Bacteroidetes (Figure 8G) and Verucomicrobia (Figure 8H) were lower than in control rats, the Firmicutes/Bacteroidetes (F/B) value in the TNBS model group was higher than that of the control group (Figure 8I); however, LD4-PDT treatment reduced Firmicutes, increased Bacteroidetes and Verucomicrobia in fecal samples, the F/B value was reduced after LD4-PDT treatment, and the gut bacterial composition pattern was comparable with that of the control group following LD4-PDT treatment (Figures 8F–I).
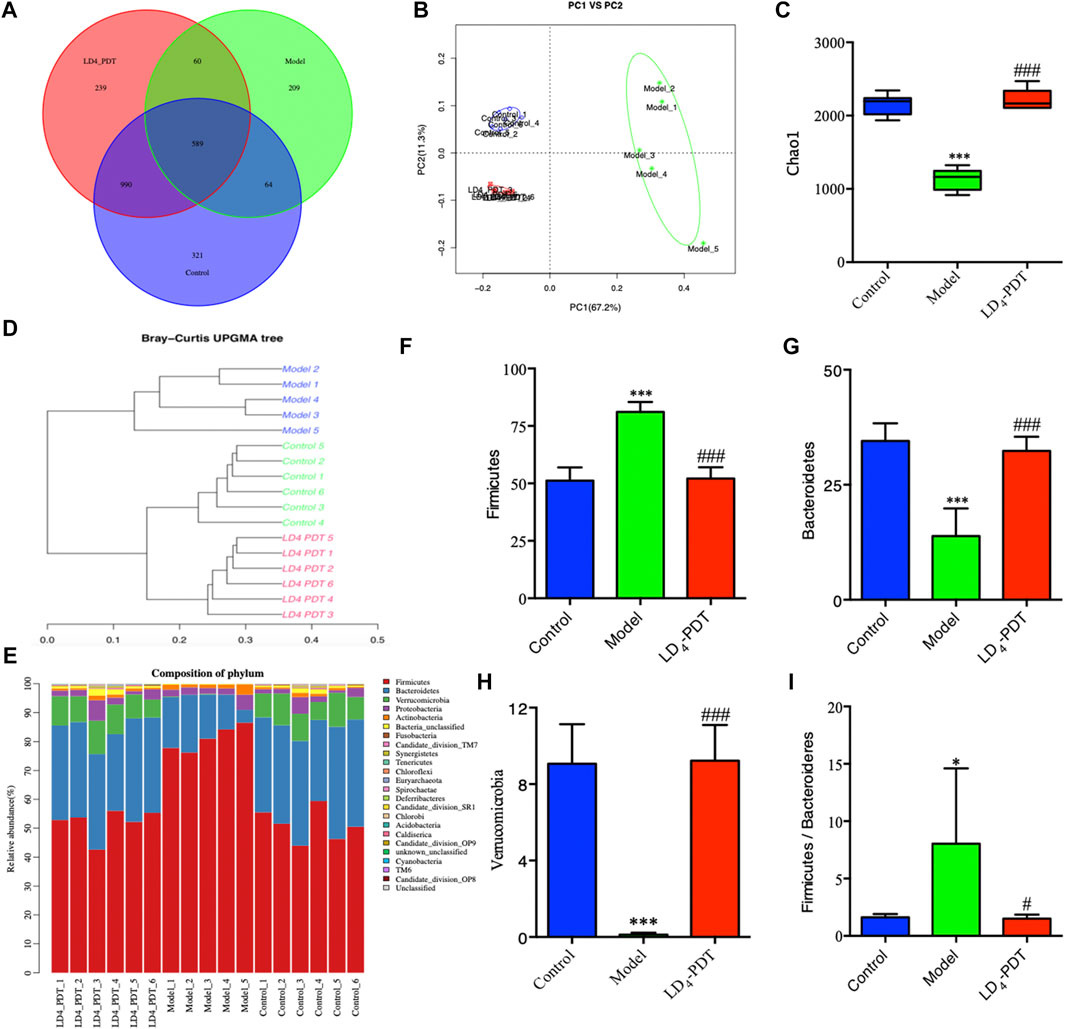
FIGURE 8. LD4-PDT treatment altered the gut microbiota in TNBS-induced UC model rats. (A) Venn diagram indicated the differential numbers of OTUs in each group. (B) PCoA score. (C) Chao1 index represented the α-diversity of the gut microbiota. (D) Hierarchical clustering of fecal microbiota in the control, model and LD4-PDT groups. (E) Phylum-level distribution of fecal microbiota (F–H) Relative abundance of the (F) Firmicutes, (G) Bacteroidetes, (H) Verrucomicrobia (I) The Firmicutes/Bacteroidetes (F/B) value **p < 0.01, ***p < 0.001 vs control group; #p < 0.05, ##p < 0.01, ###p < 0.001 vs model group; n = 6 rats per group.
Discussion
In this study, we found that LD4-PDT can effectively alleviate the inflammation both in vitro and in vivo. TNBS-induced UC model rats showed ruffled fur, loss of appetite, lethargy, blood in the stool, and weight loss, while LD4-PDT treatment recovered their healthy appearance with no significant weight loss. Pathological observations revealed that the colonic mucosal barrier structure of the untreated group was destroyed with infiltration of inflammatory cells and obvious intestinal wall ulcers and adhesions, these illness characteristics was reduced in each LD4-PDT-treated group. Neutrophils are rich in MPO, which can catalyze and oxidize chloride ions to produce hypochlorous acid to kill microorganisms in phagocytes, destroy a variety of target substances, and play a role in production and regulation of inflammation (Gu et al., 2017). MDA levels can reflect the degree of external damage to the body (Wang et al., 2019). Neutrophils in colitis model release MPO, which stimulates oxidative stress in the body, and increases the level of MPO and MDA. However, LD4-PDT treatment significantly reduced both MPO and MDA levels. SOD which can remove the harmful substances produced in the process of metabolism (Gu et al., 2017; Wang et al., 2019), is the only enzyme that decomposes superoxide radicals into H2O2 in antioxidation. Another free radical scavenger, GSH, can also regulate oxidative stress (Gu et al., 2017; Wang et al., 2019). The levels of GSH and SOD were decreased in the colitis model, but were increased significantly after LD4-PDT treatment, indicating that LD4-PDT can regulate the level of oxidative stress during UC.
The inhibitory effect of amino salicylic acid drugs on NF-κB can improve the symptoms of UC (Murray et al., 2020). The role of TNF-α is mainly to regulate the function of immune cells and induce inflammation to produce IL-1 and IL-6. Many studies have shown that the levels of TNF-α, IL-1 and IL-6 were elevated in the UC model (Wang et al., 2019; Zhai et al., 2020), while further studies have confirmed that inhibition of TNF-α, IL-1 and IL-6 could prevent the development of inflammation (Xu et al., 2017; Lee et al., 2020). In this study, LD4-PDT treatment significantly inhibited TNF-α, IL-1 and IL-6 expression, and consequently reduced the severity of colitis in UC model (Huang et al., 2017; Lee et al., 2020; Li et al., 2020). Intestinal barrier function can be assessed by quantification of permeability, so fluorescent tracers can be used to detect the degree of permeability by measuring the fluorescence intensity in serum. Many studies have shown that in UC models, the intestinal mucosal barrier function is impaired and so intestinal permeability increases, allowing harmful substances to invade the body and promoting development of the disease (Wang et al., 2019; Li H. et al., 2020). After LD4-PDT treatment, the intestinal permeability was decreased and intestinal mucosal barrier function was recovered. The above results show that LD4-PDT can not only restore the intestinal mucosal barrier function, but also reduce the damaging effects of mucosal injury factors on the intestinal mucosa, thus playing a key role in the treatment of UC.
The intestinal flora is the largest and most complex microecosystem in the human body. It is a dynamic community composed of bacteria, fungi, and viruses that regulate the homeostasis and physiological functions of the mucous membrane. There is a relatively balanced state between the body’s intestinal microbes and the immune system. Abnormal immune responses to bacteria out of balance may disrupt this homeostasis and are related to human IBD (Le Chatelier et al., 2013). Several studies have shown that intestinal flora affects intestinal mucosal immune function, intestinal cell metabolism and renewal and other processes, which can cause obesity, diabetes, malignant tumors, toxemia and IBD (Chen et al., 2020; Ma et al., 2020; Wang et al., 2020). Therefore, exploring the role of the intestinal flora in the occurrence and development of UC, and restoring the balance of disordered flora is of great significance for the treatment of UC. 16S rRNA sequencing technology has shown that 90% of the dominant human intestinal flora consists of Firmicutes, Bacteroidetes, Proteobacteria and Verrucomicrobia (Nishino et al., 2018; Soltys et al., 2020; Wang et al., 2020; Zhu et al., 2020). Both UC patients and UC mice have serious flora imbalances, and the distribution of UC flora at different stages of disease is also significantly different. In our study, we found that Firmicutes and Bacteroidetes were dominant in the model group, followed by Verrucomicrobia. LD4-PDT treatment increased the diversity of the flora and restored the flora composition towards normal. The F/B value, the ratio of the abundance of Firmicutes and Bacteroides, can effectively reflect the disorder of the intestinal flora and is usually significantly increased in the pathogenesis of UC (Wu et al., 2019; Zhu Y. et al., 2020). In our study, the F/B value in the TNBS model group was higher than that of the control group, and the F/B value was reduced after LD4-PDT treatment, suggesting that LD4-PDT can improve intestinal diseases to a certain extent. Verrucomicrobia, which gain energy from degradation of excess mucin produced in the lining of the gut and produce anti-inflammatory effects, are significantly more abundant in healthy persons than in patients with UC (Shah et al., 2016; Zakerska-Banaszak et al., 2021). In UC patients, the level of mucin is decreased resulting in reduced abundance of Verrucomicrobia (Shah et al., 2016; Zakerska-Banaszak et al., 2021). Consistent with previous reports, we found that at the phylum level, the relative content of Verrucomicrobia was reduced in the UC model compared with control rats, while LD4-PDT treatment increased the abundance of this microbiota and protected the intestinal mucosa to produce an anti-inflammatory effect.
To further explore the biological mechanism of LD4-PDT in UC, colonic protein assays were conducted using proteomics in model, control and LD4-PDT groups. More than 176 proteins were detected and their differential expression were given between model and LD4-PDT group. Although the first two protein changed large, however they were related to basic immunology and energy supply. AOC1, whose abundance change was in third position and was correlated tightly with the inflammation, were identified as a potential target of LD4-PDT. AOC1 is known to be involved in allergic and immune responses, cell proliferation, tissue differentiation, and apoptosis (Strolin Benedetti et al., 2007; Shepard and Dooley, 2015; Vakal et al., 2020). Its role in the transport of immune cells has made it a target for autoimmune and inflammatory diseases (Peet et al., 2011). In addition, AOC1 has the ability to regulate pathophysiological processes, such as cancer and EMT (Xu et al., 2020). Studies have reported that plasma AOC1 activity can be used to diagnose intestinal integrity and play an important role in UC (DiSilvestro et al., 1997). In this study, we found that the expression of AOC1 in the colon was up-regulated in the TNBS model group, while LD4-PDT treatment reduced the expression of AOC1 at the gene and protein levels, indicating that LD4-PDT exerted its effects in UC via AOC1, thus mediating expression of downstream cytokines. To test this hypothesis, AOC1 was specifically knocked down or over-expressed in vitro. Consistent with our hypothesis, silencing of AOC1 reduced the levels of IL-1, IL-6 and TNF-α. These results suggested that AOC1 protein may be a key protein in the treatment of UC, and that reducing its expression, either by LD4-PDT treatment or by other means, could ameliorate UC symptoms. Studies have shown that AOC1 can promote the expression of AKT and further regulate pro-inflammatory factors like NF-κB (Xu et al., 2020). The activated NF-κB signaling pathway can affect various biological processes, including innate and adaptive immunity, inflammation, stress responses, and B-cell development (Liu et al., 2020; Shamekhi et al., 2020). The AOC1-AKT/IKK/ NF-κB pathway plays an important role in immune response and inflammatory response and is involved in the occurrence and development of various inflammatory diseases. Activation of NF-κB can promote the expression of various inflammatory factors such as IL-6, LI-1β and TNF-α, enhance the body’s non-specific and specific immune responses, cause tissue damage and organ dysfunction, and further aggravate the symptoms of UC. Our research shows that when the UC model was established, the AKT/IKK/NF-κB pathway was activated to promote inflammation but following LD4-PDT treatment, the AKT/IKK/NF-κB pathway was inhibited, thereby exerting a therapeutic effect on UC. In summary, our study indicates that LD4-PDT treatment UC was effective, the working mechanism may be LD4-PDT function via AOC1 to mediate AKT/IKK/NF-κB pathways and downstream inflammatory cytokine expression. However, the mechanism of action behind LD4-PDT function and whether AOC1 is the sole target of LD4-PDT in the treatment of UC remain to be fully elucidated.
In conclusion, we have shown that LD4-PDT treatment could promote healing of the colonic mucosa, regulation of intestinal flora, and improvement in the clinical symptoms of UC. LD4-PDT can reduce the mucosal inflammatory response mediated by AOC1, which we identified as a potential target for UC intervention. Novel photosensitizing agent, LD4, was an efficient PDT treatment candidate for UC.
Data Availability Statement
The original contributions presented in the study are publicly available. This data can be found here: http://proteomecentral.proteomexchange.org/cgi/GetDataset, submission number - 1-20210807-77236.
Ethics Statement
The animal study was reviewed and approved by the Laboratory Animal Management Committee/Laboratory Animal Welfare Ethics Committee, Institute of Radiation Medicine, Chinese Academy of Medical Sciences.
Author Contributions
TL and YR designed and performed experiments, analyzed data, and wrote the paper. GH, YJ, NZ, and YL performed experiments. The final manuscript was reviewed and approved by all authors.
Funding
This work was supported by the national major science and technology special project for significant new drugs development under grant (2018ZX09711001-005-018/2019ZX09721001-006-001); The medical and health science and technology innovation project under grant (2017-I2M-3-021/2019-I2M-1-005); The key technologies R & D program of Tianjin under grant (20YFZCSY00570).
Conflict of Interest
The authors declare that the research was conducted in the absence of any commercial or financial relationships that could be construed as a potential conflict of interest.
Publisher’s Note
All claims expressed in this article are solely those of the authors and do not necessarily represent those of their affiliated organizations, or those of the publisher, the editors and the reviewers. Any product that may be evaluated in this article, or claim that may be made by its manufacturer, is not guaranteed or endorsed by the publisher.
Supplementary Material
The Supplementary Material for this article can be found online at: https://www.frontiersin.org/articles/10.3389/fphar.2021.746725/full#supplementary-material
References
Chen, J., Hu, S., Ji, D., Gao, Z., Wang, H., Yang, Y., et al. (2020). Hemolysin BL from Novel Bacillus Toyonensis BV-17 Induces Antitumor Activity Both In Vitro and In Vivo. Gut Microbes 12, 1782158. doi:10.1080/19490976.2020.1782158
Chow, D. K., Leong, R. W., Tsoi, K. K., Ng, S. S., Leung, W. K., Wu, J. C., et al. (2009). Long-Term Follow-Up of Ulcerative Colitis in the Chinese Population. Am. J. Gastroenterol. 104, 647–654. doi:10.1038/ajg.2008.74
Costello, S. P., Conlon, M. A., and Andrews, J. M. (2019). Fecal Microbiota Transplantation for Ulcerative Colitis-Reply. JAMA 321, 2240–2241. doi:10.1001/jama.2019.3950
DiSilvestro, R. A., Jones, A. A., Smith, D., and Wildman, R. (1997). Plasma Diamine Oxidase Activities in Renal Dialysis Patients, a Human with Spontaneous Copper Deficiency and Marginally Copper Deficient Rats. Clin. Biochem. 30, 559–563. doi:10.1016/s0009-9120(97)00102-1
Favre, L., Borle, F., Velin, D., Bachmann, D., Bouzourene, H., Wagnieres, G., et al. (2011). Low Dose Endoluminal Photodynamic Therapy Improves Murine T Cell-Mediated Colitis. Endoscopy 43, 604–616. doi:10.1055/s-0030-1256382
Gu, P., Zhu, L., Liu, Y., Zhang, L., Liu, J., and Shen, H. (2017). Protective Effects of Paeoniflorin on TNBS-Induced Ulcerative Colitis through Inhibiting NF-KappaB Pathway and Apoptosis in Mice. Int. Immunopharmacol. 50, 152–160. doi:10.1016/j.intimp.2017.06.022
Hindryckx, P., Baert, F., Hart, A., Magro, F., Armuzzi, A., Peyrin-Biroulet, L., et al. (2015). Clinical Trials in Ulcerative Colitis: A Historical Perspective. J. Crohns Colitis 9, 580–588. doi:10.1093/ecco-jcc/jjv074
Hirono, H., Watanabe, K., Hasegawa, K., Honma, T., Ajioka, Y., and Ohkoshi, S. (2018). A Case of Right-Sided Ulcerative Colitis with Mesalamine-Induced Hypersensitivity Reactions. Am. J. Case Rep. 19, 623–629. doi:10.12659/AJCR.909644
Huang, Y. F., Zhou, J. T., Qu, C., Dou, Y. X., Huang, Q. H., Lin, Z. X., et al. (2017). Anti-inflammatory Effects of Brucea Javanica Oil Emulsion by Suppressing NF-Κb Activation on Dextran Sulfate Sodium-Induced Ulcerative Colitis in Mice. J. Ethnopharmacol 198, 389–398. doi:10.1016/j.jep.2017.01.042
Le Chatelier, E., Nielsen, T., Qin, J., Prifti, E., Hildebrand, F., Falony, G., et al. (2013). Richness of Human Gut Microbiome Correlates with Metabolic Markers. Nature 500, 541–546. doi:10.1038/nature12506
Lee, C. L., Wang, C. M., Kuo, Y. H., Yen, H. R., Song, Y. C., Chou, Y. L., et al. (2020). IL-17A Inhibitions of Indole Alkaloids from Traditional Chinese Medicine Qing Dai. J. Ethnopharmacol 255, 112772. doi:10.1016/j.jep.2020.112772
Li, H., Fan, C., Lu, H., Feng, C., He, P., Yang, X., et al. (2020a). Protective Role of Berberine on Ulcerative Colitis through Modulating Enteric Glial Cells-Intestinal Epithelial Cells-Immune Cells Interactions. Acta Pharm. Sin B 10, 447–461. doi:10.1016/j.apsb.2019.08.006
Li, Y., Dong, J., Xiao, H., Zhang, S., Wang, B., Cui, M., et al. (2020b). Gut Commensal Derived-Valeric Acid Protects against Radiation Injuries. Gut Microbes 11, 789–806. doi:10.1080/19490976.2019.1709387
Liu, B., Rong, Y., Sun, D., Li, W., Chen, H., Cao, B., et al. (2019). Costunolide Inhibits Pulmonary Fibrosis via Regulating NF-kB and TGF-β1/Smad2/Nrf2-NOX4 Signaling Pathways. Biochem. Biophys. Res. Commun. 510, 329–333. doi:10.1016/j.bbrc.2019.01.104
Liu, Q., Zuo, R., Wang, K., Nong, F. F., Fu, Y. J., Huang, S. W., et al. (2020). Oroxindin Inhibits Macrophage NLRP3 Inflammasome Activation in DSS-Induced Ulcerative Colitis in Mice via Suppressing TXNIP-Dependent NF-Κb Pathway. Acta Pharmacol. Sin. 41, 771–781. doi:10.1038/s41401-019-0335-4
Ma, J., Hong, Y., Zheng, N., Xie, G., Lyu, Y., Gu, Y., et al. (2020). Gut Microbiota Remodeling Reverses Aging-Associated Inflammation and Dysregulation of Systemic Bile Acid Homeostasis in Mice Sex-Specifically. Gut Microbes 11, 1450–1474. doi:10.1080/19490976.2020.1763770
Maisch, T., Moor, A. C., Regensburger, J., Ortland, C., Szeimies, R. M., and Bäumler, W. (2011). Intense Pulse Light and 5-ALA PDT: Phototoxic Effects In Vitro Depend on the Spectral Overlap with Protoporphyrine IX but Do Not Match Cut-Off Filter Notations. Lasers Surg. Med. 43, 176–182. doi:10.1002/lsm.20970
Mallidi, S., Spring, B. Q., and Hasan, T. (2015). Optical Imaging, Photodynamic Therapy and Optically Triggered Combination Treatments. Cancer J. 21, 194–205. doi:10.1097/PPO.0000000000000117
Meng, S., Xu, Z., Hong, G., Zhao, L., Zhao, Z., Guo, J., et al. (2015). Synthesis, Characterization and In Vitro Photodynamic Antimicrobial Activity of Basic Amino Acid-Porphyrin Conjugates. Eur. J. Med. Chem. 92, 35–48. doi:10.1016/j.ejmech.2014.12.029
Morris, G. P., Beck, P. L., Herridge, M. S., Depew, W. T., Szewczuk, M. R., and Wallace, J. L. (1989). Hapten-Induced Model of Chronic Inflammation and Ulceration in the Rat Colon. Gastroenterology 96, 795–803. doi:10.1016/s0016-5085(89)80079-4
Murray, A., Nguyen, T. M., Parker, C. E., Feagan, B. G., and MacDonald, J. K. (2020). Oral 5-Aminosalicylic Acid for Maintenance of Remission in Ulcerative Colitis. Cochrane Database Syst. Rev. 8, CD000544. doi:10.1002/14651858.CD000544.pub5
Ng, S. C., Shi, H. Y., Hamidi, N., Underwood, F. E., Tang, W., Benchimol, E. I., et al. (2017). Worldwide Incidence and Prevalence of Inflammatory Bowel Disease in the 21st Century: A Systematic Review of Population-Based Studies. Lancet 390, 2769–2778. doi:10.1016/S0140-6736(17)32448-0
Nishino, K., Nishida, A., Inoue, R., Kawada, Y., Ohno, M., Sakai, S., et al. (2018). Analysis of Endoscopic Brush Samples Identified Mucosa-Associated Dysbiosis in Inflammatory Bowel Disease. J. Gastroenterol. 53, 95–106. doi:10.1007/s00535-017-1384-4
Peet, G. W., Lukas, S., Hill-Drzewi, M., Martin, L., Rybina, I. V., Roma, T., et al. (2011). Bioluminescent Method for Assaying Multiple Semicarbazide-Sensitive Amine Oxidase (SSAO) Family Members in Both 96- and 384-Well Formats. J. Biomol. Screen. 16, 1106–1111. doi:10.1177/1087057111414897
Qiu, Y., Chen, B. L., Feng, R., Zhang, S. H., He, Y., Zeng, Z. R., et al. (2018). Prolonged Azathioprine Treatment Reduces the Need for Surgery in Early Crohn's Disease. J. Gastroenterol. Hepatol. 33, 664–670. doi:10.1111/jgh.14000
Reinhard, A., Bressenot, A., Dassonneville, R., Loywick, A., Hot, D., Audebert, C., et al. (2015). Photodynamic Therapy Relieves Colitis and Prevents Colitis-Associated Carcinogenesis in Mice. Inflamm. Bowel Dis. 21, 985–995. doi:10.1097/MIB.0000000000000354
Rong, Y., Cao, B., Liu, B., Li, W., Chen, Y., Chen, H., et al. (2018). A Novel Gallic Acid Derivative Attenuates BLM-Induced Pulmonary Fibrosis in Mice. Int. Immunopharmacol. 64, 183–191. doi:10.1016/j.intimp.2018.08.024
Sands, B. E., Peyrin-Biroulet, L., Loftus, E. V., Danese, S., Colombel, J. F., Törüner, M., et al. (2019). Vedolizumab versus Adalimumab for Moderate-To-Severe Ulcerative Colitis. N. Engl. J. Med. 381, 1215–1226. doi:10.1056/NEJMoa1905725
Senge, M. O. (2012). mTHPC--a Drug on its Way from Second to Third Generation Photosensitizer? Photodiagnosis Photodyn Ther. 9, 170–179. doi:10.1016/j.pdpdt.2011.10.001
Shah, R., Cope, J. L., Nagy-Szakal, D., Dowd, S., Versalovic, J., Hollister, E. B., et al. (2016). Composition and Function of the Pediatric Colonic Mucosal Microbiome in Untreated Patients with Ulcerative Colitis. Gut Microbes 7, 384–396. doi:10.1080/19490976.2016.1190073
Shamekhi, S., Abdolalizadeh, J., Ostadrahimi, A., Mohammadi, S. A., Barzegari, A., Lotfi, H., et al. (2020). Apoptotic Effect of Saccharomyces cerevisiae on Human Colon Cancer SW480 Cells by Regulation of Akt/NF-ĸb Signaling Pathway. Probiotics Antimicrob. Proteins 12, 311–319. doi:10.1007/s12602-019-09528-7
Shepard, E. M., and Dooley, D. M. (2015). Inhibition and Oxygen Activation in Copper Amine Oxidases. Acc. Chem. Res. 48, 1218–1226. doi:10.1021/ar500460z
Shivaji, U. N., Nardone, O. M., Cannatelli, R., Smith, S. C., Ghosh, S., and Iacucci, M. (2020). Small Molecule Oral Targeted Therapies in Ulcerative Colitis. Lancet Gastroenterol. Hepatol. 5, 850–861. doi:10.1016/S2468-1253(19)30414-5
Soltys, K., Stuchlikova, M., Hlavaty, T., Gaalova, B., Budis, J., Gazdarica, J., et al. (2020). Seasonal Changes of Circulating 25-Hydroxyvitamin D Correlate with the Lower Gut Microbiome Composition in Inflammatory Bowel Disease Patients. Sci. Rep. 10, 6024. doi:10.1038/s41598-020-62811-4
Strolin Benedetti, M., Tipton, K. F., and Whomsley, R. (2007). Amine Oxidases and Monooxygenases in the In Vivo Metabolism of Xenobiotic Amines in Humans: Has the Involvement of Amine Oxidases Been Neglected? Fundam. Clin. Pharmacol. 21, 467–480. doi:10.1111/j.1472-8206.2007.00498.x
Vakal, S., Jalkanen, S., Dahlström, K. M., and Salminen, T. A. (2020). Human Copper-Containing Amine Oxidases in Drug Design and Development. Molecules 25, 1293. doi:10.3390/molecules25061293
Wang, G., Xu, B., Shi, F., Du, M., Li, Y., Yu, T., et al. (2019). Protective Effect of Methane-Rich Saline on Acetic Acid-Induced Ulcerative Colitis via Blocking the TLR4/NF-Κb/MAPK Pathway and Promoting IL-10/JAK1/STAT3-Mediated Anti-Inflammatory Response. Oxid Med. Cel Longev 2019, 7850324. doi:10.1155/2019/7850324
Wang, Y., Li, J., Chen, C., Lu, J., Yu, J., Xu, X., et al. (2020). Targeting the Gut Microbial Metabolic Pathway with Small Molecules Decreases Uremic Toxin Production. Gut Microbes 12, 1–19. doi:10.1080/19490976.2020.1823800
Ward, M. G., Sparrow, M. P., and Roblin, X. (2018). Therapeutic Drug Monitoring of Vedolizumab in Inflammatory Bowel Disease: Current Data and Future Directions. Therap Adv. Gastroenterol. 11, 1756284818772786. doi:10.1177/1756284818772786
Wirtz, S., Neufert, C., Weigmann, B., and Neurath, M. F. (2007). Chemically Induced Mouse Models of Intestinal Inflammation. Nat. Protoc. 2, 541–546. doi:10.1038/nprot.2007.41
Wu, M., Li, P., An, Y., Ren, J., Yan, D., Cui, J., et al. (2019). Phloretin Ameliorates Dextran Sulfate Sodium-Induced Ulcerative Colitis in Mice by Regulating the Gut Microbiota. Pharmacol. Res. 150, 104489. doi:10.1016/j.phrs.2019.104489
Xu, B., Li, Y. L., Xu, M., Yu, C. C., Lian, M. Q., Tang, Z. Y., et al. (2017). Geniposide Ameliorates TNBS-Induced Experimental Colitis in Rats via Reducing Inflammatory Cytokine Release and Restoring Impaired Intestinal Barrier Function. Acta Pharmacol. Sin. 38, 688–698. doi:10.1038/aps.2016.168
Xu, F., Xu, Y., Xiong, J. H., Zhang, J. H., Wu, J., Luo, J., et al. (2020). AOC1 Contributes to Tumor Progression by Promoting the AKT and EMT Pathways in Gastric Cancer. Cancer Manag. Res. 12, 1789–1798. doi:10.2147/CMAR.S225229
Xu, Z., Gao, Y., Meng, S., Yang, B., Pang, L., Wang, C., et al. (2016). Mechanism and In Vivo Evaluation: Photodynamic Antibacterial Chemotherapy of Lysine-Porphyrin Conjugate. Front. Microbiol. 7, 242. doi:10.3389/fmicb.2016.00242
Zakerska-Banaszak, O., Tomczak, H., Gabryel, M., Baturo, A., Wolko, L., Michalak, M., et al. (2021). Dysbiosis of Gut Microbiota in Polish Patients with Ulcerative Colitis: a Pilot Study. Sci. Rep. 11, 2166. doi:10.1038/s41598-021-81628-3
Zhai, L., Huang, T., Xiao, H. T., Wu, P. G., Lin, C. Y., Ning, Z. W., et al. (2020). Berberine Suppresses Colonic Inflammation in Dextran Sulfate Sodium-Induced Murine Colitis Through Inhibition of Cytosolic Phospholipase A2 Activity. Front. Pharmacol. 11, 576496. doi:10.3389/fphar.2020.576496
Zhang, X. Y., Chen, J., Yi, K., Peng, L., Xie, J., Gou, X., et al. (2020). Phlorizin Ameliorates Obesity-Associated Endotoxemia and Insulin Resistance in High-Fat Diet-Fed Mice by Targeting the Gut Microbiota and Intestinal Barrier Integrity. Gut Microbes 12, 1–18. doi:10.1080/19490976.2020.1842990
Zhao, Z., Ma, J., Wang, Y., Xu, Z., Zhao, L., Zhao, J., et al. (2021). Antimicrobial Photodynamic Therapy Combined with Antibiotic in the Treatment of Rats with Third-Degree Burns. Front. Microbiol. 12, 622410. doi:10.3389/fmicb.2021.622410
Zhu, L., Xu, L. Z., Zhao, S., Shen, Z. F., Shen, H., and Zhan, L. B. (2020a). Protective Effect of Baicalin on the Regulation of Treg/Th17 Balance, Gut Microbiota and Short-Chain Fatty Acids in Rats with Ulcerative Colitis. Appl. Microbiol. Biotechnol. 104, 5449–5460. doi:10.1007/s00253-020-10527-w
Keywords: ulcerative colitis, gut microbiota, photodynamic therapy, novel photosensitizer, AOC1
Citation: Rong Y, Hong G, Zhu N, Liu Y, Jiang Y and Liu T (2021) Photodynamic Therapy of Novel Photosensitizer Ameliorates TNBS-Induced Ulcerative Colitis via Inhibition of AOC1. Front. Pharmacol. 12:746725. doi: 10.3389/fphar.2021.746725
Received: 24 July 2021; Accepted: 01 October 2021;
Published: 21 October 2021.
Edited by:
Andres Trostchansky, Universidad de la República, UruguayReviewed by:
Hao-Sen Chiang, National Taiwan University, TaiwanMohamed Abdo Rizk, Mansoura University, Egypt
Copyright © 2021 Rong, Hong, Zhu, Liu, Jiang and Liu. This is an open-access article distributed under the terms of the Creative Commons Attribution License (CC BY). The use, distribution or reproduction in other forums is permitted, provided the original author(s) and the copyright owner(s) are credited and that the original publication in this journal is cited, in accordance with accepted academic practice. No use, distribution or reproduction is permitted which does not comply with these terms.
*Correspondence: Tianjun Liu, liutianjun@hotmail.com