- 1Faculty of Pharmacy, Research Institute for Medicines (iMed.ULisboa), Universidade de Lisboa, Lisbon, Portugal
- 2Institute of Pathology, University Hospital Charité, Berlin, Germany
- 3Science for Life Laboratory, Department of Cell and Molecular Biology, Uppsala University, Uppsala, Sweden
Background: Multidrug resistance (MDR) has been regarded as one of the major hurdles for the successful outcome of cancer chemotherapy. The collateral sensitivity (CS) effect is one the most auspicious anti-MDR strategies. Epoxylathyrane derivatives 1–16 were obtained by derivatization of the macrocyclic diterpene epoxyboetirane A (17), a lathyrane-type macrocyclic diterpene isolated from Euphorbia boetica. Some of these compounds were found to strongly modulate P-glycoprotein (P-gp/ABCB1) efflux.
Purpose: The main goal was to develop lathyrane-type macrocyclic diterpenes with improved MDR-modifying activity, by targeting more than one anti-MDR mechanism.
Study design/methods: In this study, the potential CS effect of compounds 1–16 was evaluated against gastric (EPG85-257), pancreatic (EPP85-181), and colon (HT-29) human cancer cells and their drug-resistant counterparts, respectively selected against mitoxantrone (EPG85-257RNOV; EPP85-181RNOV; HT-RNOV) or daunorubicin (EPG85-257RDB; EPP85-181RDB; HT-RDB). The most promising compounds (8, 15, and 16) were investigated as apoptosis inducers, using the assays annexin V/PI and active caspase-3.
Results: The compounds were more effective against the resistant gastric cell lines, being the CS effect more significant in EPG85-257RDB cells. Taking together the IC50 values and the CS effect, compounds 8, 15, and 16 exhibited the best results. Epoxyboetirane P (8), with the strongest MDR-selective antiproliferative activity against gastric carcinoma EPG85-257RDB cells (IC50 of 0.72 µM), being 10-fold more active against this resistant subline than in sensitive gastric carcinoma cells. The CS effect elicited by compounds 15 and 16 appeared to be by inducing apoptosis via caspase-3 activation. Structure-activity relationships of the compounds were additionally obtained through regression models to clarify the structural determinants associated to the CS effect.
Conclusions: This study reinforces the importance of lathyrane-type diterpenes as lead molecules for the research of MDR-modifying agents.
Introduction
Multidrug resistance (MDR) is among the main clinical hurdles to successful cancer chemotherapy. It is defined by the development of cell resistance to a large variety of structurally unrelated drugs with diverse mechanisms of action. There is a great consensus that cancer cells might become resistant to anticancer drugs by several mechanisms that are still not completely understood and could occur simultaneously. Some of the most common cellular factors attributed to MDR include: changes in membrane transport through reduced drug uptake or augmented drug efflux; changes in drug targets and metabolism; increased DNA damage repair; and failure of apoptotic events. However, the most known and characterized MDR mechanism is owing to an increased efflux of the anticancer drugs as a result of the overexpression of ATP-binding cassette (ABC) transporter proteins, namely, P-glycoprotein (P-gp/ABCB1), multidrug resistance protein 1 (MRP1/ABCC1), and breast cancer resistance protein (BCRP/ABCG2) that act as extrusion pumps. From these mechanisms, P-gp still constitutes one of the biggest challenges for medicinal chemistry (Gottesman et al., 2002; Gottesman and Ling, 2006; Gottesman et al., 2016).
Several approaches have been developed to eradicate MDR in cancer. The most general has been the development of P-gp inhibitors to co-administer with anticancer drugs. However, despite great in vitro success, there is no P-gp inhibitor currently available for clinical use. The development of collateral sensitizing compounds is also included on the set of the most encouraging approaches to tackle MDR (Callaghan et al., 2014; Szakács et al., 2014). The collateral sensitivity (CS) effect is characterized by an increased sensitivity or hypersensitivity of resistant cells to certain compounds. The phenomenon was firstly recognized in the early 1950s, when it was observed that resistant Escherichia coli was hypersensitive to several drugs at the same time (Szybalski and Bryson, 1952). Based on this concept, which presently is considered a strong anti-MDR strategy, alterations of cancer cells that confer resistance to certain agents might simultaneously generate weaknesses that may rend drug-resistant cells more sensitive to alternative drugs than the corresponding parental cells. CS is thought to be highly correlated with the overexpression of one of the three major efflux proteins (P-gp, MRP1 or BCRP) in resistant cancer cells, therefore representing a new strategy to circumvent ABC transporters-mediated MDR (Gottesman et al., 2016). Thus, these vulnerabilities developed by cancer cells can be targeted for improving chemotherapy by developing compounds that are selective against resistant-phenotypes (MDR-selective compounds) and thus able to re-sensitize resistant tumors and reestablish drugs effectiveness. Several compounds have been reported to exert CS. However, although many hypotheses have been proposed, the mechanism of collateral sensitizing compounds remains unclear (Callaghan et al., 2014; Szakács et al., 2014). In this way, while in P-gp-overexpressing cancer cells, CS agents seem to be related to diverse biochemical mechanisms, in MRP1-overexpressing cancer cells, CS agents appeared to behave as stimulators of glutathione efflux, altering redox balance and thus triggering apoptosis of multi-resistant cells (Klukovits and Krajcsi, 2015).
Natural products have been of crucial importance for drug research and development. Concerning cancer, since the beginning of chemotherapy in the 1940s to date, about 75% of anticancer drugs approved world-wide are natural products or their synthetic derivatives (Newman and Cragg, 2012). In an effort to find out anticancer compounds from plants, for targeting MDR cancer cells, our group has been given particular attention on the development of MDR reversal compounds (Reis et al., 2015; Paterna et al., 2016; Ramalhete et al., 2016; Reis et al., 2016; Reis et al., 2017; Ferreira et al., 2018a; Ferreira et al., 2018b; Paterna et al., 2018; Ramalhete et al., 2018). Duo to the high and unusual chemical diversity of their metabolites, many of which coupled with strong biological properties, we have given particular attention to Euphorbia species (Euphorbiaceae), which are well known since ancient times for their use in folk medicine worldwide, namely, to cure cancer, tumors, and warts (Hartwell, 1969; Ernst et al., 2015). Other significant reported uses included treatment of respiratory and digestive disorders and inflammation (Ernst et al., 2015). Most importantly, in 2012, Food and Drug Administration (FDA) and European Medicines Agency (EMA) approved ingenol 3-angelate (Picato®), isolated from Euphorbia peplus, for the treatment of actinic keratosis. This diterpene ester, with a dual and unique mechanism of action embracing a rapid cellular necrosis and a specific immune response (Rosen et al., 2012), is a valuable example of the strong bioactivity and pharmacological importance of some Euphorbia genus metabolites.
Jatrophane and lathyrane-type diterpenes, from Euphorbia species, have revealed a significant MDR modulatory activity through reversion of the ABCB1 MDR phenotype (Ferreira et al., 2014). Aiming at optimizing their structures for improving their MDR reversal activity, in silico and structure-activity relationship studies were performed (Ferreira et al., 2011; Reis et al., 2012; Sousa et al., 2012; Ferreira et al., 2013; Reis et al., 2013; Baptista et al., 2016). In this regard, Euphorbia boetica Boiss. (Euphorbiacae) was a fruitful source of novel compounds and prototypes for the design of MDR reversers (Vieira et al., 2014; Matos et al., 2015). Macrocyclic diterpene derivatives with the lathyrane skeleton, obtained from this species, were found promising ABCB1 efflux modulators (Vieira et al., 2014; Matos et al., 2015).
Therefore, the present work aimed at assessing the ability of the macrocyclic diterpene derivatives 1–16 (Figure 1) for their potential as collateral sensitizing compounds, using the human tumor gastric (EPG85-257), pancreatic (EPP85-181), and colon (HT-29) cell models (drug-sensitive and drug-resistant sublines), well described for MDR (Table S2; Lage et al., 2010; Hilgeroth et al., 2013; Reis et al., 2014). Additionally, the MDR-selective antiproliferative activity mode of action of compounds 8, 15, and 16 was assessed towards apoptosis and caspase-3 activation, using the same cell lines. From the obtained results, and to better understand which structural features are correlated with the observed CS effect, regression models were further obtained from molecular descriptors calculated for a small library of macrocyclic diterpenes.
Materials and Methods
Tested Compounds
Epoxylathyrane derivatives (1–16), namely, epoxylathyrol (1), epoxyboetirane E (2), epoxyboetirane L (4), epoxyboetirane M (5), epoxyboetirane N (6), epoxyboetirane O (7), epoxyboetirane P (8), epoxyboetirane Q (9), epoxyboetirane R (10), epoxycarbamoylboetirane B (11), epoxycarbamoylboetirane C (12), methoxyboetirane B (15), and methoxyboetirane C (16), were obtained by derivatization of the macrocyclic diterpene epoxyboetirane A (17), isolated from Euphorbia boetica (Vieira et al., 2014; Matos et al., 2015). Briefly, epoxyboetirane A, was firstly hydrolyzed, yielding mostly epoxylathyrol (1). Methoxyboetirol (13), resulting from a Payne-rearranged Michael adduct, was also obtained as minor product. Compound 2–12 and 14–16 were obtained by acylation reactions of epoxylathyrol (1) and methoxyboetirol (13), respectively (Vieira et al., 2014; Matos et al., 2015).
Cell Lines, Cell Culture
The establishment and characterization of the cell lines EPG85-257P (gastric); EPP85-181P (pancreatic); and HT-29P (colon) and their drug-resistant sublines (EPG85-257RNOV, EPG85-257RDB, EPP85-181RNOV, EPP85-181RDB, HT-29RNOV, HT-29RDB used have been described previously (Reis et al., 2014). More experimental details at supplementary material and Table S2.
Cell Proliferation Assay, Annexin V/PI Staining, and Active Caspase-3 Assay
The antiproliferative activity of compounds was evaluated using a proliferation assay based on sulforhodamine B (SRB) staining, as previously described (Reis et al., 2014). More experimental details at supplementary material.
For detection of cytotoxic drug-induced apoptosis, a FITC Annexin V apoptosis detection kit (BD Pharmingen, BD Biosciences) was used. Detection of intracellular presence of active caspase-3 was also performed using FITC active Caspase-3 Apoptosis Kit (BD Pharmingen, BD Biosciences). Both assays followed the same experimental design, described at supplementary material.
Regression Models
For a given set of molecules obtained in the present and previous works (N = 42, Table S1) (Reis et al., 2014; Reis et al., 2016; Reis et al., 2017), a comprehensive database of molecular descriptors (constitutional, topological, and geometrical) was obtained from E-DRAGON, PaDEL, and MOE as in a previous work (Baptista et al., 2016). Afterward, relative resistant (RR) values obtained in the EPG-257RDB cell line were added to the dataset and transformed into a binary classification of 1 (RR ≤ 0.5) or 0 (RR > 0.5) for the presence or absence of CS, respectively. All QSAR models were built using WEKA v3.8.3 (Hall et al., 2009) software as previously reported (Baptista et al., 2016), with only minor modifications. The CfsSubsetEval algorithm with the BestFirst search method was applied as default prior to model classification. All models were generated using WEKA’s default options. The robustness of the generated models (NB and RT) were assessed by a 10-fold cross-validation and their predictive power by splitting the dataset into training and test sets (66:34). All models were analysed using several parameters, among which true positive rate (TP), false positive rate (FP), precision, Matthews Correlation Coefficient (MCC), and Receiver Operating Characteristics (ROC) area under the curve. Other parameters as the mean absolute error (MAE), root mean squared error (RMSE), and Kappa statistic (k) were also used to assess the reliability of the model.
Results and Discussion
Collateral Sensitivity Effect
Aiming at optimizing macrocyclic diterpenes with lathyrane scaffold as active MDR reversal agents, the phytochemical investigation of Euphorbia boetica aerial parts (methanolic extract) was conducted, yielding several compounds with the lathyrane scaffold (Vieira et al., 2014). Epoxylathyrane A (17), a lathyrane polyester isolated in large amount, was hydrolyzed, in an alkaline methanolic solution, giving rise to epoxylathyrol (1), as main product, and a Payne-rearranged Michael adduct named methoxyboetirol (13) (Matos et al., 2015). In this previous work, compounds 1 and 13 were acylated, yielding the derivatives 2–12 and 14–16, respectively. The potential of epoxylathyrane derivatives 1–16 as P-gp-mediated MDR reversers, was evaluated at non-cytotoxic doses in L5178Y ABCB1-transfected mouse T-lymphoma cells, by the accumulation rhodamine-123 assay. Most of the tested derivatives exhibited strong P-gp modulating activities and, in addition, they were able to enhance the cytotoxicity of doxorubicin in a synergistic mode, restoring its sensitivity by reversion of the ABCB1-MDR phenotype. By structure-activity relationships studies, it was concluded that the presence of an aromatic moiety on those structures improved significantly the inhibition of rhodamine-123 efflux (Matos et al., 2015).
Taking into account that targeting more than one MDR mechanism could be a way to provide a good therapeutic outcome, in the course of the development of epoxylathyrane derivatives as MDR reversal agents, it is also important to consider other potential anti-MDR mechanisms of action (Fojo, 2008; Gottesman et al., 2016). Therefore, the epoxylathyrane derivatives 1–16 were investigated for their potential CS effect against gastric (EPG85-257), pancreatic (EPP85-181), and colon (HT-29) human cancer cells and their drug-resistant counterparts, respectively selected against mitoxantrone (RNOV) or daunorubicin (RDB), using a proliferation assay (Reis et al., 2014). The MDR-selective activity was assessed by the relative resistance ratio (RR = IC50(resistant)/IC50(parental)). Values of RR < 1 indicate that the compound kills MDR cells more effectively than parental cells, but if RR ≤ 0.5, then a CS effect is taking place (Hall et al., 2009). The cytotoxic agents etoposide and cisplatin were used as positive controls. The antiproliferative activity and CS effects of compounds 1–16 are presented in Tables 1–4. The heat map represented in Table 1 allows the recognition of compounds that exhibit MDR-selective activity at a specific IC50 level.
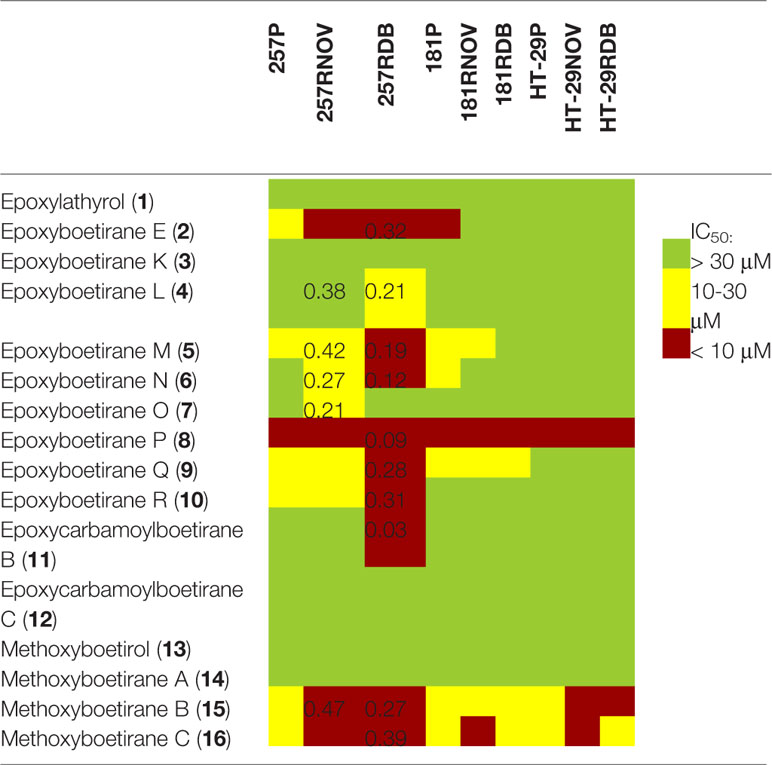
Table 1 Heat map table summarizing the antiproliferative and collateral sensitivity results against gastric carcinoma cells (EPG85-257P, EPG85-257RNOV, and EPG85-257RDB), and pancreatic carcinoma cells (EPP85-181P, EPP85-181RNOV, and EPP85-181RDB). This representation allows finding compounds that present MDR-selective activity at a determined IC50 level. CS values (RR ≤ 0.5) are presented.
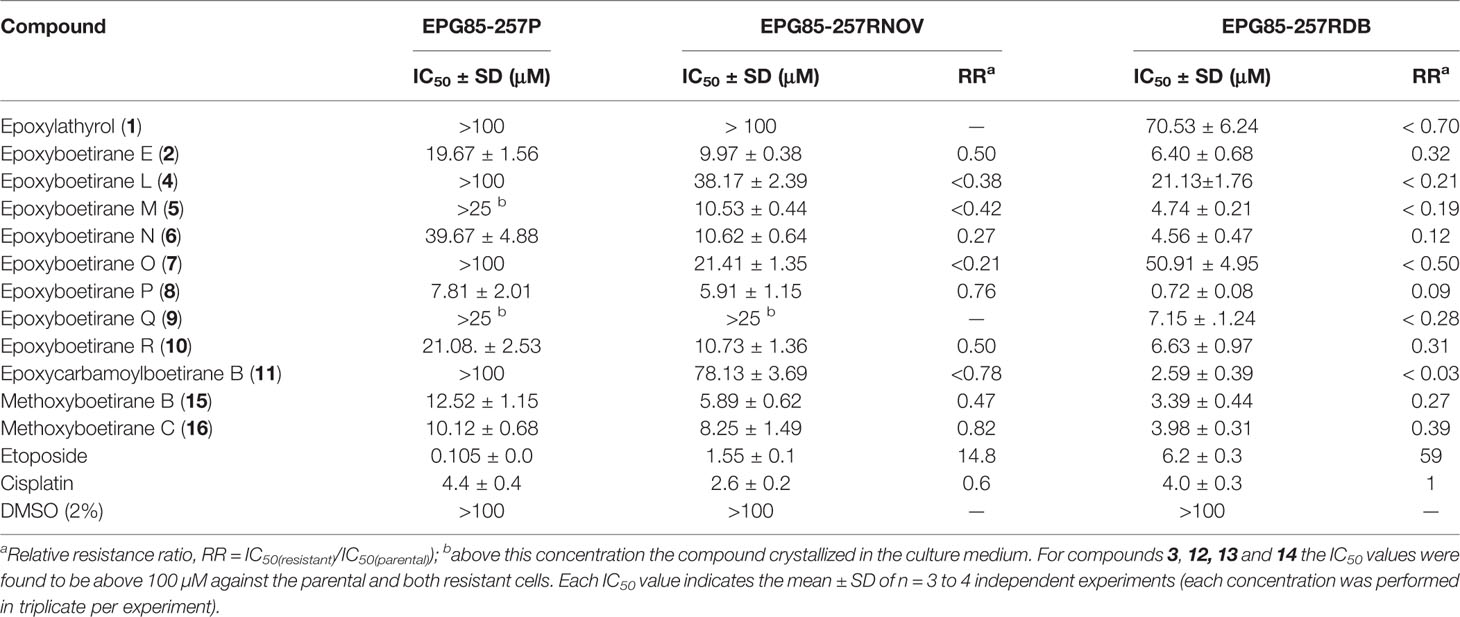
Table 2 Antiproliferative activity of compounds 1–16 in gastric carcinoma cells: EPG85-257P (parental), EPG85-257RNOV (multidrug resistance [MDR] phenotype), and EPG85-257RDB (multidrug resistance [MDR] phenotype).
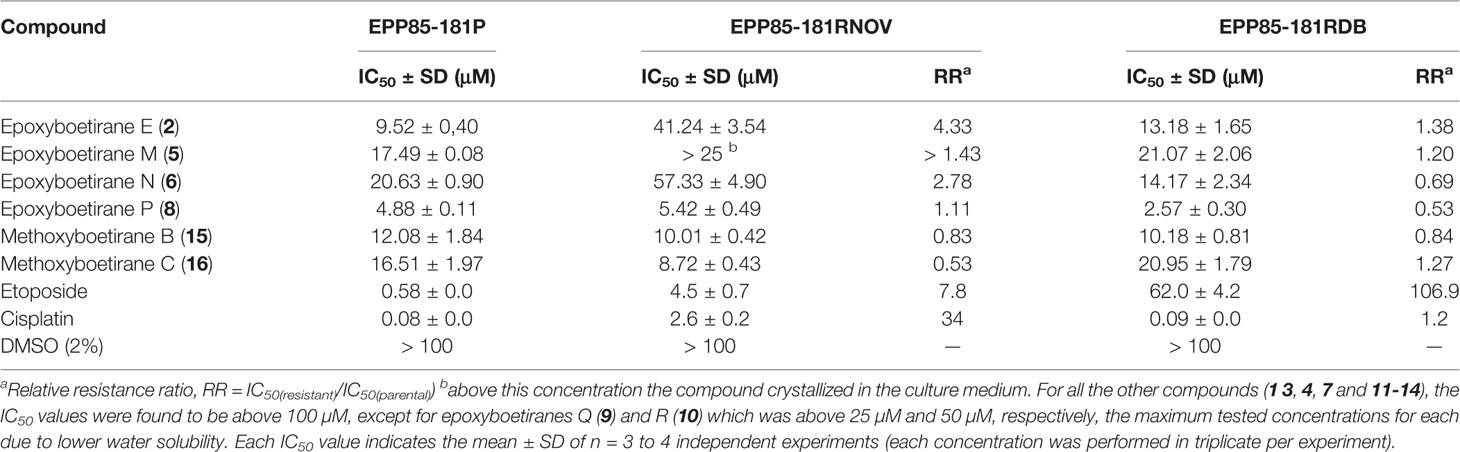
Table 3 Antiproliferative activity of 1–16 in in pancreatic carcinoma cells: EPP85-181P (parental), EPP85-181RNOV (multidrug resistance [MDR] phenotype), and EPP85-181RBD (multidrug resistance [MDR] phenotype).
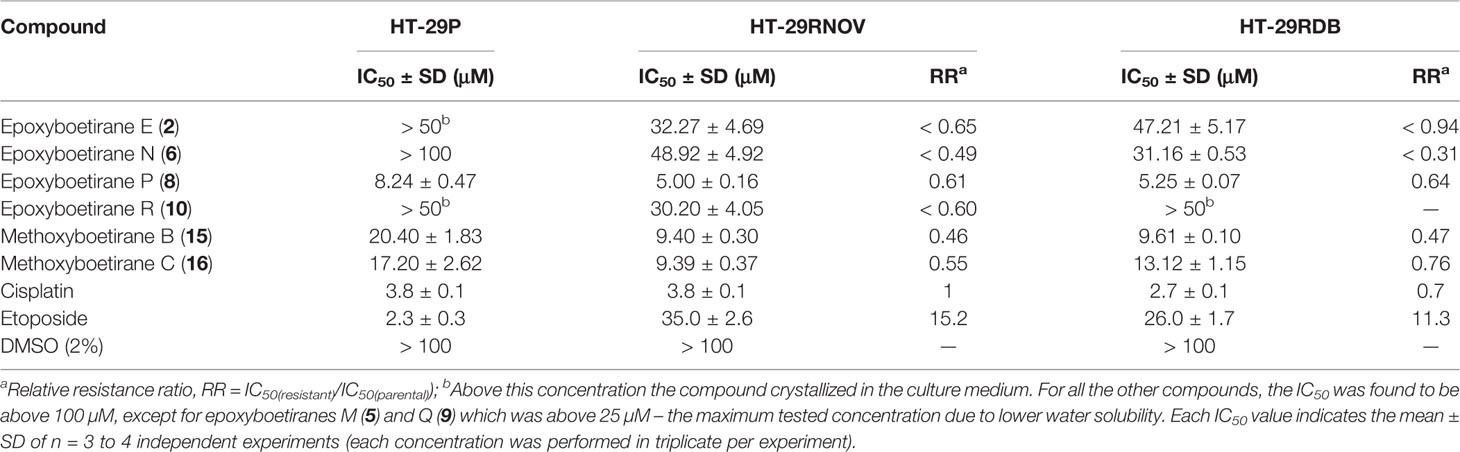
Table 4 Antiproliferative activity of compounds 1–16 in colon carcinoma cells HT-29P (parental), HT-29RNOV (multidrug resistance [MDR] phenotype), and HT-29RBD (multidrug resistance [MDR] phenotype).
As showed in Tables 2–4, the strongest antiproliferative effect in drug-sensitive cell lines was observed for epoxyboetirane P (8), bearing p-(dimethylamino)benzoyl acyl groups, that exhibited an IC50 < 10 µM in the three cell lines tested (EPG85-257P, EPP85-181P, and HT-29P, IC50 = 7.81 ± 2.01, 4.88 ± 0.11, and 8.24 ± 0.47, respectively). An IC50 < 10 µM was also found for epoxyboetirane E (2) but only in pancreatic parental cells (EPP85-181P, IC50 = 9.52 ± 0.40). Significant IC50 values were also observed for methoxyboetiranes B (15) and C (16), in gastric parental cells (EPG85-257P, IC50 = 12.52 ± 1.15 and 10.12 ± 0.68, respectively), exhibiting the former (15) also an IC50 = 12.08 ± 1.84, against pancreatic cells. The other compounds were inactive or barely active, displaying a moderate/weak antiproliferative activity in parental drug-sensitive cell lines (Tables 2–4).
When analyzing the results against MDR sublines, several compounds were found to be more active against multidrug-resistant cells than in parental cells (Tables 2–4), exhibiting relative resistance values lower than 1.0. Moreover, as it can be observed, some compounds showed CS effect (RR ≤ 0.5), being more effective toward the resistant gastric cell lines, and more pronounced in EPG85-257RDB cells (Tables 1 and 2), which are characterized by ABCB1 overexpression (Dietel et al., 1990). Taking together the IC50 and RR values, the best results were found for epoxyboetirane P (8), which showed an antiproliferative effect 10-fold higher against the MDR subline of gastric carcinoma EPG85-257RDB than in parental drug-sensitive cells (IC50 = 0.72 ± 0.08; RR = 0.09). A selective IC50 value was also obtained with this compound against the resistant EPG85-257 RNOV subline (IC50 = 5.91 ± 1.15), although without CS effect (RR = 0.76). CS effect, associated with potent antiproliferative activity, was also registered for methoxyboetiranes B (15), (IC50 = 3.39 ± 0.44; RR = 0.27), and C (16) (IC50 = 3.98 ± 0.31; RR = 0.39), against the gastric EPG85-257 RDB subline, whose IC50 values were comparable to those of the positive controls etoposide and cisplatin and (IC50 = 6.2 ± 0.3 and 4.0 ± 0.3, respectively). Compound 15, having unsubstituted benzoyl moieties, showed also CS effect in EPG85-257 RNOV subline (IC50 = 5.89 ± 0.62; RR = 0.47), whereas compound 16, having trifluoromethyl substituents at para-position, exhibited slightly higher relative resistance and IC50 values (IC50 = 8.25 ± 1.49; RR = 0.82). Furthermore, epoxyboetyranes E, M, N, O, R (2, 5, 6, 9, and 10) and epoxycarbamoylboetirane B (11) also exhibited CS effect (RR ≤ 0.5) coupled with high selective antiproliferative activity (IC50 values ranging from 2.59 ± 0.39 to 7.15 ± 1.24) against the gastric EPG85-257 RDB subline. Excepting for compound 11, comparable results were also observed for this set of compounds in the EPG85-257 RNOV subline, although associated with a lower antiproliferative effect (Table 2).
The tested compounds were less active and showed no CS effect in pancreatic cancer cell lines (Tables 1 and 3). Epoxyboetirane P (8) was once more the most active (IC50 < 10 µM) in parental and both resistant sublines, exhibiting MDR-selective antiproliferative effects (RR < 1) in resistant EPP85-181RRDB subline (EPP85-181P, IC50 = 4.88 ± 0.11; EPP85-181RDB, IC50 = 2.57 ± 0.30, RR = 0.53; EPP85-181RNOV, IC50 = 5.42 ± 0.49, RR = 1.11). When comparing with the positive controls, methoxyboetirane B (15) also showed significant antiproliferative activity in both resistant sublines associated with RR < 1 (EPP85-181RDB, IC50 = 10.18 ± 0.81, RR = 0.84; EPP85-181RNOV, IC50 = 10.01 ± 0.42, RR = 0.83).
In turn, the best results revealed by methoxyboetirane C (16) were in EPP85-181RNOV subline, showing an IC50 = 8.72 ± 0.43. (RR = 0.53).
Epoxyboetirane P (8) also showed the lowest IC50 values in colon cancer cell lines (Table 4), (HT-29RDB, IC50 = 5.25 ± 0.07 μM, RR = 0.64; HT-29RNOV; IC50 = 5.00 ± 0.16 μM, RR = 0.61). However, in colon cancer cells, CS effect (RR ≤ 5), associated with significant antiproliferative activity (IC50≤ 10), was only observed for methoxyboetirane B (15), with similar results in both resistant variants (HT-29RDB, IC50 = 9.61 ± 0.10, RR = 0.47; HT-29RNOV IC50 = 9.40 ± 0.30, RR = 0.46). Methoxyboetirane C (16) showed comparable IC50 values against HT-29RNOV variant (9.39 ± 0.37, RR = 0.55). Indeed, it exhibited RR< 1 in HT-29RDB subline although with lower antiproliferative activity (IC50 = 13.12 ± 1.15, RR = 0.76).
When analyzing the results, it could be concluded that the antiproliferative activity depends on the acylation patterns. Thus, in both epoxylathyrol (1) and methoxyboetirol (13) derivatives, (2–12 and 13–16, respectively), acyl moieties bearing simple aromatic moieties, including benzoyl (2, 5, 6–8, 15, 16) furoyl (9), and thiophenecarbonyl (10) groups, were generally favorable for the antiproliferative activity. Conversely, compounds with biphenylcarbonyl (3) and naphthoyl substituents (4) were inactive or barely active in the three human cancer parental cells and corresponding MDR-sublines. No significant activity was observed for the parent compounds epoxylathyrol (1) and methoxyboetirol (13), without ester moieties, and for compounds 11, 12, and 14, bearing aliphatic acyl moieties.
As already mentioned, this set of macrocyclic diterpenes (1–16) was previously evaluated for their ability to reverse P-gp-mediated MDR, using a functional assay (Vieira et al., 2014; Matos et al., 2015). In this study, it is noteworthy that compounds with significant MDR-selective antiproliferative activities (2, 5, 6, 8–11, 15, and 16), mostly in drug-resistant gastric sublines, were also found to be strong P-gp modulators in a concentration-dependent manner.
Apoptosis Induction Activity
The ability of compounds 8, 15, and 16 as apoptosis inducers was evaluated using as models gastric and pancreatic cancer cells. The apoptotic process usually occurs through the extrinsic or intrinsic pathways. Despite their mechanistic differences, both converge on the same execution pathway, which is initiated by the activation of caspase-3. This cysteine protease takes a fundamental part in apoptosis, being pro-caspase-3, the penultimate enzyme for accomplishment of the apoptotic process (Tan et al., 2009). Therefore, the active caspase-3 was quantified by flow cytometry after 48 h of exposure at 20 µM of the compounds 8, 15, 16 (Figure 2). The results were expressed as fold increase (ratio between treated samples and untreated samples).
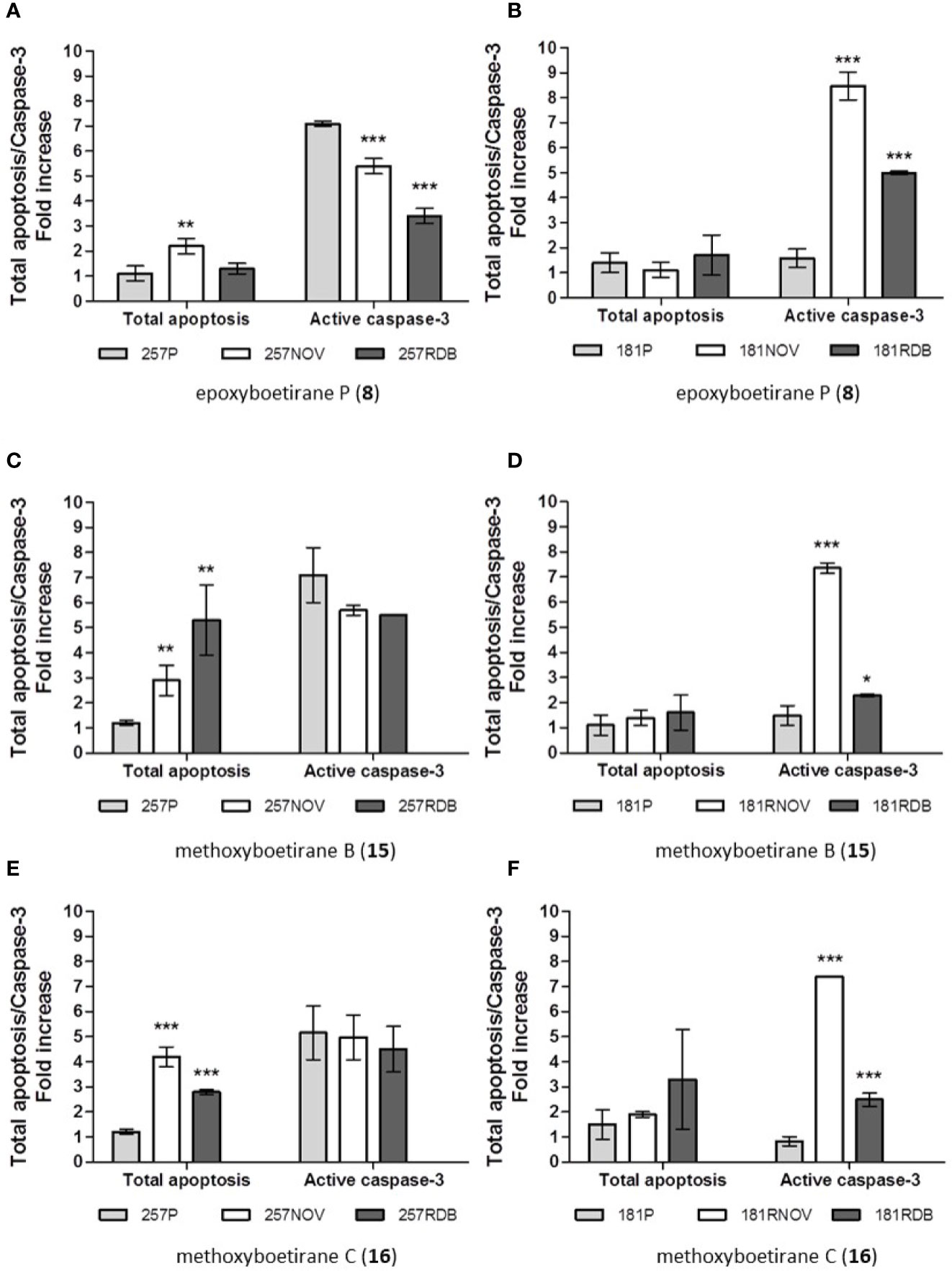
Figure 2 Cell death mechanism measurements: apoptosis induction and active caspase-3 activation in gastric (A, C, E) and pancreatic (B, D, F) cancer cell lines after 72 h incubation with epoxyboetirane P (8), methoxyboetirane B (15), and methoxyboetirane C (16) (30 μM). Apoptosis induction: representative flow cytometry analysis after annexin V-FITC/PI staining. The FL1 and FL2 axis represent the fluorescence intensities of Annexin V-FITC and PI, respectively. Camptothecin (1 μM) was used as internal positive control. Total apoptosis was considered the sum of early and late apoptotic events (cells annexin V-FITC positive/PI negative plus cells annexin V-FITC positive/PI positive). The results were expressed as the ratio between treated samples with untreated. Each column represents the mean ± SD of three independent experiments. Statistical significance was calculated for the difference between treated resistant cell lines and treated parental cells using a two-tailed unpaired Student’s t test. Level of significance *, p < 0.05. **, p < 0.01. ***, p < 0.001. Active caspase-3: the results were expressed as the ratio between treated samples with untreated. Each column represents the mean ± SD (n = 3). Statistical significance was calculated for the difference between treated resistant cell lines and treated parental cells using a two-tailed unpaired Student’s t test. Level of significance *, p < 0.05. **, p < 0.01. ***, p < 0.001.
The subsequent apoptotic events include exposure of phosphatidylserine on the external surface of the plasma membrane, which is used as marker of apoptosis. As this phospholipid is shifted from the inner to the outer leaflet of the plasma membrane in early apoptosis, the annexin V/propidium iodide (PI) staining permits to identify both early and late apoptotic cells (PI negative, annexin V positive, and PI positive, annexin V positive, respectively) (Chen, 2009). Hence, the annexin V/PI assay was used to evaluate the induction of apoptosis by compounds 8, 15, and 16 (20 µM) (Figure 2). The results were presented as total apoptosis (early and late apoptotic events) and the effects were expressed as fold increase (ratio between treated samples and untreated samples).
As described above, epoxyboetirane P (8) not only presented a highly antiproliferative profile against EPG85-257 gastric cells, but also produced a CS effect for EPG85-257RDB (Table 1). After 48 h incubation, compound 8 elicited the activation of caspase-3 in EPG85-257P, EPG85-257RNOV and EPG85-257RDB (Figure 2A). Contrary to what would be expected this effect was significantly more pronounced (7-fold) on the parental cell line. With such results, it was expected to observe early and late apoptotic events, after 72 h incubation; however significant results were only obtained for the EPG85-257RNOV cells (Figure 2A). Furthermore, epoxyboetirane P (8) induced a different response in EPP85-181 pancreatic cells in respect to active caspase-3 (Figure 2B). Statistically significant discrimination between resistant pancreatic cell lines and EPP85-181P cells was observed (Figure 2B). Compound 8 produced an 8- and 5-fold increase of active caspase-3 in EPP85-181RNOV and EPP85-181RDB cells, respectively. Nonetheless, this compound was not able to induce early or late apoptotic events that would be quantifiable by the annexin V/PI assay, after 72 h incubation.
On our previous studies, methoxyboetirane B (15) was highlighted as a promising lead compound for MDR-reversal, since it showed a remarkable ABCB1 modulatory activity potential through a competitive mechanism of action (Matos et al., 2015). Herein, it presented an interesting CS effect on both gastric MDR phenotypes (RR = 0.47 and 0.27, respectively, to EPG85-257RNOV and EPG85-257RDB. These observations were also reflected in the results obtained from the annexin V/PI assays, where a significant stimulation of apoptotic events was recorded in the MDR phenotypes but not in the parental (Figure 2C). Compound 15 caused a 3- and 5-fold increase of total apoptosis in EPG85-257RNOV and EPG85-257RDB, respectively. Moreover, the active caspase-3 assays quantified induction of these proteins on about 5.5 to 7-fold, but without significant phenotypic discrimination (Figure 2C). In light of these experimental data it might be inferred that the selective antiproliferative activity of methoxyboetirane B (15) proceeded through caspase-dependent apoptosis. Regarding the pancreatic cancer cells, compound 15 did not presented CS effect despite the strong antiproliferative effect. Nevertheless, methoxyboetirane B (15) showed to be able to provoke differential activation of capase-3 in the MDR phenotypes, but not in the parental (Figure 2D). Though, no significant apoptotic events were recorded.
Methoxyboetirane C (16) differs from methoxyboetirane B (15) on the benzoyl ester substituent, where compound 16 bears a p-trifluoromethyl group. This structural variance had impact on reducing the ABCB1 modulatory efficacy of methoxyboetirane C (16), when compared with compound 15 (Matos et al., 2015). However, in the present study, structure activity effects were not observed. Both compounds presented comparable antiproliferative profiles against the tested cell lines. Likewise, in the apoptosis induction assays similar effects can be observed (Figures 2E, F versus Figures 2C, D). Therefore, on gastric EPG85-257RNOV and EPG85-257RDB cells methoxyboetirane C (16) modulated a MDR-selective cell death through capase-3-dependent apoptosis (Figure 2E). Besides, compound 16 triggered differential activation of capase-3 on pancreatic EPP85-181RNOV and EPP85-181RDB cells, but not in the parental (Figure 2F), without significant phosphatidylserine translocation and/or membrane damage.
Regression Models
In an attempt to identify which molecular descriptors explain the CS effect by the compounds, a computational approach was undertaken by developing regression models, which are powerful tools that allow further insights on the molecular determinants underlying any observed biological activity. They were calculated for a small library of macrocyclic diterpenes, including compounds 1–16 along with compounds 17–42 (Supporting Information, Table S1). For classification purposes, a binary classification identifying the presence (RR ≤ 0.5) or absence (RR > 0.5) of CS was used. Two models were built: the first using a Näive Bayes (NB) classification scheme, a simple probabilistic classifier that examines all samples independently and calculates the individual probability of each particular compound to belong to a distinct cluster (John and Langley, 1995); and a Random Tree (RT) model in which an algorithm constructs trees with K randomly chosen attributes at each node to estimate class probabilities (Breiman, 2001). Herein, both models performed well in predicting which compounds trigger CS in EPG85-257RDB cells, with RT correctly classifying 100% of the compounds against 92.86% with the NB classifier. However, from both the 10-fold cross-validation and internal test (66% training, remaining test set) validations, the NB model provides increased robustness when compared with the RT approach, correctly classifying 85.71% in both validations (MAE, 0.1574 and 0.1106; RMSE, 0.3769 and 0.2685, respectively) against 80.95% (MAE, 0.1905; RMSE, 0.4364) and 85.71% (MAE 0.1429; RMSE, 0.3780) for the RT classifier, respectively (Table 5). At the end, we concluded that both models are reliable in predicting which compounds are able to induce CS in the tested P-gp-expressing resistant cancer cell line.
To further improve the ability of the compounds in inducing such a biological response, the identification of the underlying molecular features is of the utmost importance. As in the Naïve Bayes all attributes are assumed to be equally important a priori (Lee et al., 2011), from the RT model it is possible to infer which molecular descriptors have the greatest weight in the decision tree. The first and most important descriptor was R4p (R autocorrelation of lag 4/weighted by polarizabilities), followed by MATS8m (Moran autocorrelation of lag 8/weighted by mass) at its first branch and X1A (Average connectivity index of order 1) on the second branch. Together, they are responsible for the classification of 24 compounds with RR ≤ 0.5 (Figure S1). For the classification of the remaining compounds, the second branch is further divided by using E2m (2nd component accessibility directional WHIM index/weighted by mass), MDEC-22 (Molecular distance edge between all secondary carbons) and Mor03m (signal 03/weighted by mass) (Todeschini and Consonni, 2009; Devinyak et al., 2014). Herein, it is worth noticing that the E2m descriptor alone is responsible for the classification of 13 compounds (Figure S1). All these are geometrical descriptors encode information about the molecular size, shape, geometry, and symmetry. Therefore, it appears that the spatial position of the structural fragments and atom distribution within the molecular scaffold, together with its axial symmetry, are the most important structural determinants ruling CS effects.
Therefore, the aromaticity of the substituent increases the polarizability within the scaffold while promoting CS, in opposition to non-aromatic substituents as in compounds 12–14 and 39 (Table S1). However, substituents with higher mass ratios, obtained through the quotient between the substituents’ and the molecules molecular weight (as in compounds 3, 4, 20, 21, 37, and 38) or with a meta substitution pattern most (particularly in mono-substituted compounds 18 and 32) (Table S1) are expected to perturb the axial symmetry of the molecular scaffold and thus impairing its biological activity. Yet, from the data it is also inferred that π-π stacking (9, 10, and 15) or the presence of additional hydrogen-bond acceptor moieties (as in compounds 8, 11, and 16) are also important for the observed activity.
Overall, the achieved results hints that the substitution pattern and the presence of heteroatoms in an aromatic substituent are the strongest determinants relating to the observed CS effect. Furthermore, as the presence of substituents at positions C-15 and C-17 also impairs CS in a greater extent, the data suggests that future derivatives will have improved CS activities if i) the hydroxyl (or acetyl) group remains unchanged at position C-15 and ii) if only positions C-3 and/or C-5 are substituted but not C-17.
Conclusion
Targeting more than one anti-MDR mechanism has been considered a realistic strategy for overcoming the complex and multifactorial phenomenon of MDR. In a previous work, some epoxylathyrane derivatives were identified as strong P-glycoprotein efflux modulators. Consequently, aiming at improving their MDR-modifying activity, in this work the CS effect, one of the most hopeful anti-MDR strategies, was addressed. Derivatives 8, 15, and 16 were found to be very promising compounds, being many-fold more effective against MDR sublines, mainly in relation to gastric carcinoma cells. In these drug resistant counterparts compounds 15 and 16 significantly induced cell death through apoptosis.
The development of regression models emphasized axial symmetry of the overall molecular scaffold and, more particularly, its substitution pattern as the most determinant features related to the observed CS effect. Furthermore, while the hydroxyl function at position C-15 (or a small acetyl moiety) seems to be determinant, the aromaticity of the substituent together with the presence of heteroatoms was also inferred to be relevant for the observed activity.
All in all, this study reinforces the potential of macrocyclic diterpenes as leads for the development of MDR-modifying agents.
Data Availability Statement
All datasets generated for this study are included in the article/Supplementary Material.
Author Contributions
All authors have participated and contributed substantially to this manuscript. HL and M-JF designed and supervised the study. MR, AM, and OA carried out the experiments. RF performed the regression models. M-JF, MR, ND, and RF wrote the manuscript. M-JF, MR, and ND revised the manuscript.
Funding
This study was financially supported by the FCT (Fundação para a Ciência e a Tecnologia) under the project PTDC/MED-QUI/30591/2017 and SAICTPAC/0019/2015.
Conflict of Interest
The authors declare that the research was conducted in the absence of any commercial or financial relationships that could be construed as a potential conflict of interest.
Supplementary Material
The Supplementary Material for this article can be found online at: https://www.frontiersin.org/articles/10.3389/fphar.2020.00599/full#supplementary-material
Abbreviations
ABC, ATP binding cassette; ABCB1, ATP-binding cassette, sub-family B; CS, collateral sensitivity; EPG85- 257P, parental gastric cancer cells; EPG85- 257RDB, gastric cancer cells selected against daunorubicin; EPG85- 257RNOV, gastric cancer cells selected against mitoxantrone; EPP-181 RDB, pancreatic cancer cells selected against daunorubicin; EPP-181P, parental pancreatic cancer cells; EPP-181RNOV, pancreatic cancer cells selected against mitoxantrone; MDR, multidrug resistance; MDR1, multidrug resistance gene 1; RR, relative resistance; NB, Naïve Bayes; RT, random trees.
References
Baptista, R., Ferreira, R. J., dos Santos, D. J., Fernandes, M. X., Ferreira, M. J. U. (2016). Optimizing the macrocyclic diterpenic core toward the reversal of multidrug resistance in cancer. Future Med. Chem. 8, 629–645. doi: 10.4155/fmc.16.11
Callaghan, R., Luk, F., Bebawy, M., Cuperus, F. J. C., Claudel, T., Gautherot, J., et al. (2014). Inhibition of the multidrug resistance P-glycoprotein: time for a change of strategy? Drug Metab. Dispos. 42, 623–631. doi: 10.1124/dmd.113.056176
Chen, T. (2009). A Practical Guide to Assay Development and High-Throughput Screening in Drug Discovery (Boca Raton, Florida: CRC Press).
Devinyak, O., Havrylyuk, D., Lesyk, R. (2014). 3D-MoRSE descriptors explained. J. Mol. Graph. Model. 54, 194–203. doi: 10.1016/j.jmgm.2014.10.006
Dietel, M., Arps, H., Lage, H., Niendorf, A. (1990). Membrane vesicle formation due to acquired mitoxantrone resistance in human gastric carcinoma cell line EPG85-257. Cancer Res. 50, 6100–6106.
Ernst, M., Grace, O. M., Saslis-Lagoudakis, C. H., Nilsson, N., Simonsen, H. T., Rønsted, N. (2015). Global medicinal uses of Euphorbia L. (Euphorbiaceae). J. Ethnopharmacol. 176, 90–101. doi: 10.1016/j.jep.2015.10.025
Ferreira, R. J., dos Santos, D. J. V., Ferreira, M. J. U., Guedes, R. C. (2011). Toward a better pharmacophore description of P-glycoprotein modulators, based on macrocyclic diterpenes from Euphorbia species. J. Chem. Inf. Model. 51, 1315–1324. doi: 10.1021/ci200145p
Ferreira, R. J., Ferreira, M. J. U., dos Santos, D. J. V. (2013). Molecular docking characterizes substrate-binding sites and efflux modulation mechanisms within P-glycoprotein. J. Chem. Inf. Model. 53, 1747–1760. doi: 10.1021/ci400195v
Ferreira, M. J. U., Duarte, N., Reis, M., Madureira, A. M., Molnár, J. (2014). Euphorbia and Momordica metabolites for overcoming multidrug resistance. Phytochem. Rev. 13, 915–935. doi: 10.1007/s11101-014-9342-8
Ferreira, R. J., Baptista, R., Moreno, A., Madeira, P. G., Khonkarn, R., Baubichon-Cortay, H., et al. (2018a). Optimizing the flavanone core toward new selective nitrogen-containing modulators of ABC transporters. Future Med. Chem. 10, 725–741. doi: 10.4155/fmc-2017-0228
Ferreira, R. J., Kincses, A., Gajdács, M., Spengler, G., Dos Santos, D. J., Molnár, J., et al. (2018b). Terpenoids from Euphorbia pedroi as multidrug-resistance reversers. J. Nat. Prod. 81, 2032–2040. doi: 10.1021/acs.jnatprod.8b00326
Fojo, T. (2008). Commentary: Novel therapies for cancer: why dirty might be better. Oncologist 13, 277–283. doi: 10.1634/theoncologist.2007-0090
Gottesman, M. M., Ling, V. (2006). The molecular basis of multidrug resistance in cancer: the early years of P-glycoprotein research. FEBS Lett. 580, 998–1009. doi: 10.1016/j.febslet.2005.12.060
Gottesman, M. M., Fojo, T., Bates, S. E. (2002). Multidrug resistance in cancer: role of ATP-dependent transporters. Nat. Rev. Cancer 2, 48–58. doi: 10.1038/nrc706
Gottesman, M. M., Lavi, O., Hall, M. D., Gillet, J. P. (2016). Toward a Better Understanding of the Complexity of Cancer Drug Resistance. Annu. Rev. Pharmacol. Toxicol. 56, 85–102. doi: 10.1146/annurev-pharmtox-010715-103111
Hall, M. D., Handley, M. D., Gottesman, M. M. (2009). Is resistance useless? Multidrug resistance and collateral sensitivity. Trends Pharmacol. Sci. 30, 546–556. doi: 10.1016/j.tips.2009.07.003
Hall, M., Frank, E., Holmes, G., Pfahringer, B., Reutemann, P., Witten, I. H. (2009). The WEKA data mining software: an update. ACM SIGKDD Explor. 11, 10–18. doi: 10.1145/1656274.1656278
Hilgeroth, A., Baumert, C., Coburger, C., Seifert, M., Krawczyk, S., Hempel, C., et al. (2013). Novel structurally varied N-alkyl 1,4-dihydropyridines as ABCB1 inhibitors: structure-activity relationships, biological activity and first bioanalytical evaluation. Med. Chem. 9, 487–493. doi: 10.2174/1573406411309040002
John, G. H., Langley, P. (1995).Estimating continuous distributions in Bayesian classifiers, in: Proceedings of the UAI’95 Eleventh Conference on Uncertainty in Artificial Intelligence, Montréal, QC, Canada, 18–20 August. pp. 338–345.
Klukovits, A., Krajcsi, P. (2015). Mechanisms and therapeutic potential of inhibiting drug efflux transporters. Expert Opin. Drug Metab. Toxicol. 11, 907–920. doi: 10.1517/17425255.2015.1028917
Lage, H., Duarte, N., Coburger, C., Hilgeroth, A., Ferreira, M. J. U. (2010). Antitumor activity of terpenoids against classical and atypical multidrug resistant cancer cells. Phytomedicine 17, 441–448. doi: 10.1016/j.phymed.2009.07.009
Lee, C., Gutierrez, F., Dou, D. (2011).Calculating feature weights in Naive Bayes with Kullback-Leibler measure, in: 2011 IEEE 11th International Conference on Data Mining, Vancouver, BC. pp. 1146–1151.
Matos, A. M., Reis, M., Duarte, N., Spengler, G., Molnár, J., Ferreira, M. J. U. (2015). Epoxylathyrol derivatives: modulation of ABCB1-mediated multidrug resistance in human colon adenocarcinoma and mouse T-Lymphoma cells. J. Nat. Prod. 78, 2215–2228. doi: 10.1021/acs.jnatprod.5b00370
Newman, D. J., Cragg, G. M. (2012). Natural products as sources of new drugs over the 30 years from 1981 to 2010. J. Nat. Prod. 75, 311–335. doi: 10.1021/np200906s
Paterna, A., Kincses, A., Spengler, G., Mulhovo, S., Molnár, J., Ferreira, M. J. U. (2016). Dregamine and tabernaemontanine derivatives as ABCB1 modulators on resistant cancer cells. Eur. J. Med. Chem. 128, 247–257. doi: 10.1016/j.ejmech.2017.01.044
Paterna, A., Khonkarn, R., Mulhovo, S., Moreno, A., Madeira, G., Baubichon-Cortay, H., et al. (2018). Monoterpene indole alkaloid azine derivatives as MDR reversal agents. Bioorg. Med. Chem. 26, 421–443. doi: 10.1016/j.bmc.2017.11.052
Ramalhete, C., Mulhovo, S., Molnar, J., Ferreira, M. J. U. (2016). Triterpenoids from Momordica balsamina: Reversal of ABCB1-mediated multidrug resistance. Bioorg. Med. Chem. 24, 5061–5067. doi: 10.1016/j.bmc.2016.08.022
Ramalhete, C., Mulhovo, S., Molnar, J., Ferreira, M. J. U. (2018). Triterpenoids from Momordica balsamina with a collateral sensitivity effect for tackling multidrug resistance in cancer cells. Planta Med. 84, 1372–1379. doi: 10.1055/a-0651-8141
Reis, M., Ferreira, R., Serly, J., Duarte, N., Madureira, A., Santos, D. J., et al. (2012). Colon adenocarcinoma multidrug resistance reverted by Euphorbia diterpenes: structure-activity relationships and pharmacophore modeling. Anticancer Agents Med. Chem. 12, 1015–1024. doi: 10.2174/187152012803529655
Reis, M., Ferreira, R. J., Santos, M. M. M., dos Santos, D. J. V., Molnár, J., Ferreira, M. J. U. (2013). Enhancing macrocyclic diterpenes as multidrug-resistance reversers: structure-activity studies on jolkinol D derivatives. J. Med. Chem. 56, 748–760. doi: 10.1021/jm301441w
Reis, M. A., Paterna, A., Ferreira, R. J., Lage, H., Ferreira, M. J. U. (2014). Macrocyclic diterpenes resensitizing multidrug resistant phenotypes. Bioorg. Med. Chem. 22, 3696–3702. doi: 10.1016/j.bmc.2014.05.006
Reis, M. A., André, V., Duarte, M. T., Lage, H., Ferreira, M. J. U. (2015). 12,17-Cyclojatrophane and Jatrophane Constituents of Euphorbia welwitschii. J. Nat. Prod. 78, 2684–2690. doi: 10.1021/acs.jnatprod.5b00631
Reis, M. A., Ahmed, O. B., Spengler, G., Molnár, J., Lage, H., Ferreira, M. J. U. (2016). Jatrophane diterpenes and cancer multidrug resistance - ABCB1 efflux modulation and selective cell death induction. Phytomedicine 23, 968–978. doi: 10.1016/j.phymed.2016.05.007
Reis, M. A., Ahmed, O. B., Spengler, G., Molnár, J., Lage, H., Ferreira, M. J. U. (2017). Exploring Jolkinol D derivatives to overcome multidrug resistance in cancer. J. Nat. Prod. 80, 1411–1420. doi: 10.1021/acs.jnatprod.6b01084
Rosen, R. H., Gupta, A. K., Tyring, S. K. (2012). Dual mechanism of action of ingenol mebutate gel for topical treatment of actinic keratoses: Rapid lesion necrosis followed by lesion-specific immune response. J. Am. Acad. Dermatol. 66, 486–493. doi: 10.1016/j.jaad.2010.12.038
Sousa, I. J., Ferreira, M. J. U., Molnár, J., Fernandes, M. X. (2012). QSAR studies of macrocyclic diterpenes with P-glycoprotein inhibitory activity. Eur. J. Pharm. Sci. 48, 542–553. doi: 10.1016/j.ejps.2012.11.012
Szakács, G., Hall, M. D., Gottesman, M. M., Boumendjel, A., Kachadourian, R., Day, B. J., et al. (2014). Targeting the achilles heel of multidrug-resistant Cancer by exploiting the fitness cost of resistance. Chem. Rev. 114, 5753–5774. doi: 10.1021/cr4006236
Szybalski, W., Bryson, V. (1952). Genetic studies on microbial cross resistance to toxic agents I, cross resistance of Escherichia coli to fifteen antibiotics. J. Bacteriol. 64, 489–499. doi: 10.1128/JB.64.4.489-499.1952
Tan, M. L., Ooi, J. P., Ismail, N., Moad, A. I. H., Muhammad, T. S. T. (2009). Programmed cell death pathways and current antitumor targets. Pharm. Res. 26, 1547–1560. doi: 10.1007/s11095-009-9895-1
Todeschini, R., Consonni, V. (2009). “Molecular Descriptors for Chemoinformatics,” in Methods and Principles in Medicinal Chemistry (Weinheim: Wiley-VCH Verlag GmbH & Co. KGaA).
Keywords: multidrug resistance, collateral sensitivity, apoptosis, Euphorbia, macrocyclic diterpenes, lathyrane, regression models
Citation: Reis MA, Matos AM, Duarte N, Ahmed OB, Ferreira RJ, Lage H and Ferreira M-JU (2020) Epoxylathyrane Derivatives as MDR-Selective Compounds for Disabling Multidrug Resistance in Cancer. Front. Pharmacol. 11:599. doi: 10.3389/fphar.2020.00599
Received: 31 January 2020; Accepted: 17 April 2020;
Published: 08 May 2020.
Edited by:
Cyril Corbet, Fonds National de la Recherche Scientifique (FNRS), BelgiumReviewed by:
Krystyna Małgorzata Michalak, Wroclaw Medical University, PolandChin-Chuan Hung, China Medical University, Taiwan
Copyright © 2020 Reis, Matos, Duarte, Ahmed, Ferreira, Lage and Ferreira. This is an open-access article distributed under the terms of the Creative Commons Attribution License (CC BY). The use, distribution or reproduction in other forums is permitted, provided the original author(s) and the copyright owner(s) are credited and that the original publication in this journal is cited, in accordance with accepted academic practice. No use, distribution or reproduction is permitted which does not comply with these terms.
*Correspondence: Maria-José U. Ferreira, mjuferreira@ff.ulisboa.pt
†Present address: Mariana Alves Reis, Interdisciplinary Centre of Marine and Environmental Research (CIIMAR/CIMAR), University of Porto, Matosinhos, Portugal
‡These authors share first authorship