- 1Department of Biotherapy, Cancer Center, and National Clinical Research Center for Geriatrics, West China Hospital of Sichuan University, Chengdu, China
- 2Department of Dermatovenerology, West China Hospital, Sichuan University, Chengdu, China
- 3Department of Neurosurgery, West China Hospital, Sichuan University, Chengdu, China
- 4West China School of Medicine, Sichuan University, Chengdu, China
Immune checkpoint inhibitors (ICIs) that target cytotoxic T lymphocyte antigen 4, programmed cell death-1, and PD-ligand 1 have revolutionized cancer treatment, achieving unprecedented efficacy in multiple malignancies. ICIs are increasingly being used in early cancer settings and in combination with various other types of therapies, including targeted therapy, radiotherapy, and chemotherapy. However, despite the excellent therapeutic effect of ICIs, these medications typically result in a broad spectrum of toxicity reactions, termed immune-related adverse events (irAEs). Of all irAEs, cardiotoxicity, uncommon but with high mortality, has not been well recognized. Herein, based on previous published reports and current evidence, we summarize the incidence, diagnosis, clinical manifestations, underlying mechanisms, treatments, and outcomes of ICI-associated cardiotoxicity and discuss possible management strategies. A better understanding of these characteristics is critical to managing patients with ICI-associated cardiotoxicity.
Introduction
The immune system employs several suppressive molecules and pathways to maintain T lymphocyte cell tolerance and prevent autoimmunity (Boussiotis, 2016). Cytotoxic T lymphocyte-associated antigen-4 (CTLA-4), a coinhibitory molecule, is expressed on stimulated CD4+/CD8+ T cells to attenuate T cell activation. Of note, CTLA-4 also constitutively resides on Foxp3+ regulatory CD4+ T cells and directly facilitates the inhibitory function of regulatory T cells (Peggs et al., 2009; Walker and Sansom, 2011). Moreover, the programmed cell death protein 1 (PD-1)/programmed cell death-ligand 1 (PD-L1) pathway plays a predominant role in regulating T-cell-driven immune response. PD-1 exists inherently on the surface of T cells and is expressed on antigen-presenting cells (APCs), such as on macrophages and dendritic cells. The binding of these two molecules (PD1/PD/L1) can inhibit the immune response by reducing cytokine production and suppressing T-cell proliferation (Swaika et al., 2015). Intriguingly, numerous cancer cells overexpress PD-L1 on their surface, which contributes to their immune evasion by enhancing immune escape ability, resulting in a poor prognosis (Hino et al., 2010).
Based on the inhibitory roles of these checkpoint molecules or pathways, several immune checkpoint inhibitors (ICIs), including PD-1 inhibitors (nivolumab and pembrolizumab), PD-L1 inhibitors (atezolizumab, avelumab, and durvalumab), and CTLA-4 inhibitors (ipilimumab and tremelimumab) have been developed to restore the T cell-mediated immune response and improve the efficacy of anti-tumor treatments (Wolchok, 2015; Ribas and Wolchok, 2018). Encouragingly, these agents have revolutionized the treatment of various hematological and solid tumors (Powles et al., 2014; Tumeh et al., 2014; Pi et al., 2016).
The combination of ICIs, either use of multiple ICIs or ICIs combined with other therapies, such as chemotherapy, radiation, and anti-angiogenic drugs, has been associated with a significantly better prognosis than monotherapy (Larkin et al., 2015a). However, these reagents, both alone and in combination, also produce a wide spectrum of immune-related adverse events (irAEs), mainly due to aberrant autoreactive T cell activation (Weber et al., 2012; Nishino et al., 2015; Postow et al., 2018). Immune-mediated toxicities can affect any organ or tissue involving the skin, gastrointestinal system, endocrine system, lung, or liver (Champiat et al., 2016; Michot et al., 2016) and can largely be controlled by glucocorticoid therapy (Friedman et al., 2016). Among these toxicities, cardiotoxicity, a potentially fatal irAE, has rarely been reported in early clinical trials of ICI therapy because of its low incidence and nonspecific symptomatology (Mahmood et al., 2018a). Over the years, although increasing cases and case series of ICI-associated cardiotoxicity have been reported, it has not been fully recognized (Varricchi et al., 2017). Herein, to strengthen understanding of cardiotoxicity induced by ICIs and reduce deaths, we elaborate on the incidence, clinical manifestations, diagnosis, mechanisms, and outcomes of cardiotoxicity associated with ICIs. We will also discuss prophylactic strategies, potential treatments, and management of ICI-associated cardiotoxicity based on relevant literatures and current knowledge.
Incidence and Clinical Manifestations of Immune Checkpoint Inhibitor-Associated Cardiotoxicity
Since the first specific case of ICI-associated cardiotoxicity was reported in 2014 (Heery et al., 2014), cardiotoxicity during ICI treatment has been reported in a gradually increasing number of patients (Geisler et al., 2015; Laubli et al., 2015; Heinzerling et al., 2016; Johnson et al., 2016a; Koelzer et al., 2016; Tadokoro et al., 2016; Behling et al., 2017; Norwood et al., 2017; Reuben et al., 2017; Tajmir-Riahi et al., 2018). Cardiotoxicity attributed to ICIs spreads to almost all parts of the heart (Figure 1) and involves both inflammatory cardiotoxicity and non-inflammation-mediated cardiotoxicity. The former includes myocarditis, perimyocarditis, pericarditis, left ventricular dysfunction without myocarditis, and others (Lyon et al., 2018). The latter includes asymptomatic noninflammatory left ventricular dysfunction (Roth et al., 2016), Takotsubo-like syndrome with both basal (Ederhy et al., 2018) and apical (Geisler et al., 2015; Anderson and Brooks, 2016) variants, coronary vasospasm (Nykl et al., 2017), arrhythmias (Salem et al., 2018), and myocardial infarction (Weinstock et al., 2017). Of all the cardiotoxicity-associated ICIs, myocarditis is the most common cardiotoxic reaction. Pericardial diseases and conduction diseases were reported in 15 and 12% of patients with ICI-related cardiotoxicity, respectively (Mir et al., 2018). One published study (Mahmood et al., 2018a) on 964 patients between 2013 and 2017 indicated that 1.14% of patients developed myocarditis and 0.52% developed a major adverse cardiovascular event (MACE), such as complete heart block, cardiogenic shock, and cardiac arrest, during treatment with ICIs. As for the presentation of myocarditis, it is highly variable and nonspecific (Table 1). Based on previously published studies, the manifestations of cardiotoxicity range from subclinical disease with asymptomatic cardiac biomarker elevation, fatigue, and general malaise to chest pain, dyspnea, palpitations, multiorgan failure, cardiogenic shock, and cardiac arrest (Laubli et al., 2015; Yun et al., 2015; Gibson et al., 2016; Escudier et al., 2017; Reuben et al., 2017; Mahmood et al., 2018a; Neilan et al., 2018; Wang et al., 2018; Samara et al., 2019).
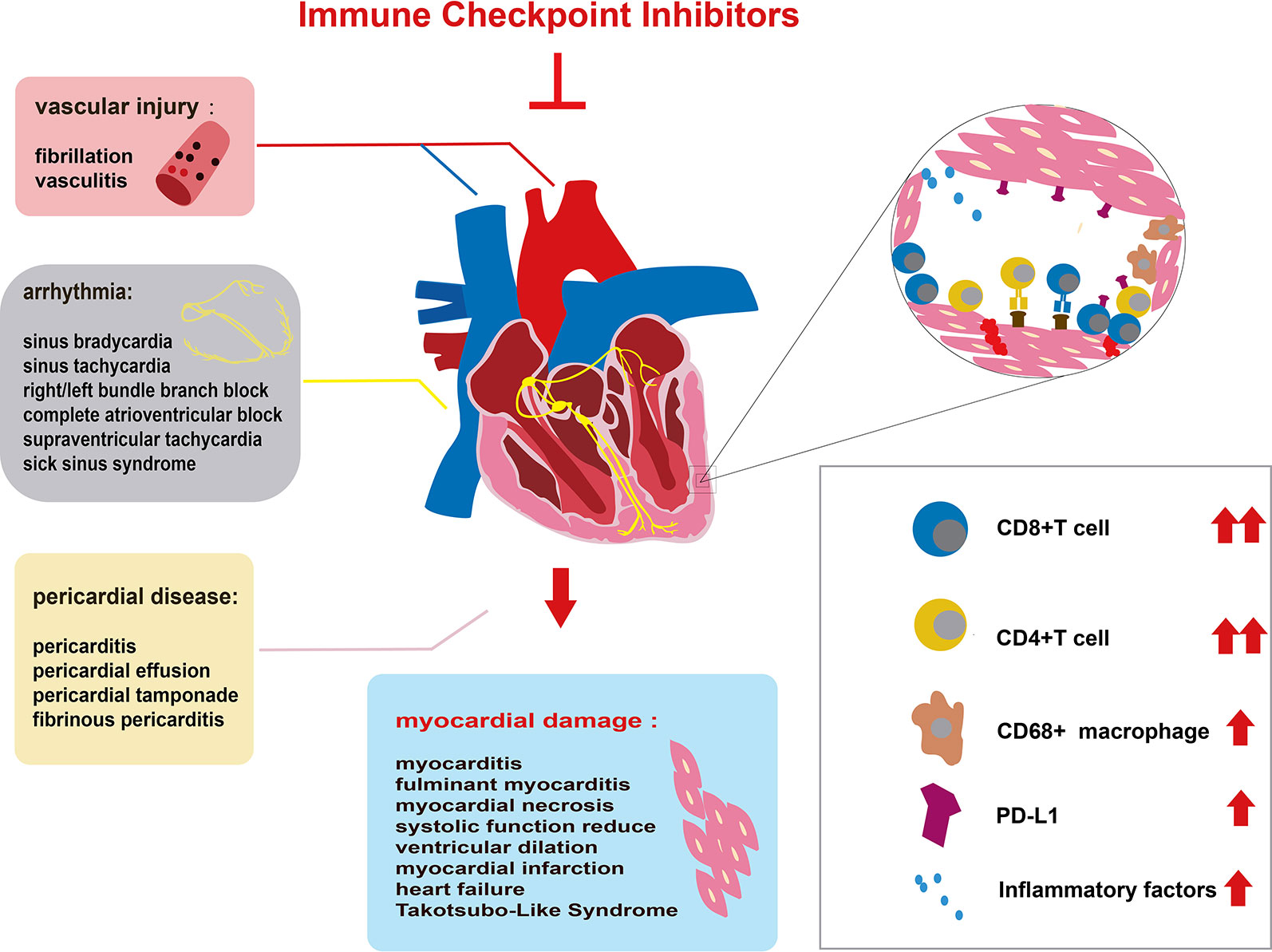
Figure 1 Immune checkpoint inhibitor (ICI)-related cardiac toxicity and its underlying mechanism. Anatomically, ICI-related cardiac toxicity involves almost all parts of the heart. The myocardium is most sensitive to ICI toxicity, showing impaired heart function. The cardiac conduction system, vascular system, and pericardium are also influenced by ICIs. Many infiltrating cells including hyperactivated CD4+/CD8+ T lymphocytes and a few macrophages (CD68+ cells) can microscopically be found in the heart tissue of patients with ICI-related cardiac toxicity. The infiltrating T cells are regarded as the main cause of ICI-related cardiac toxicity. The production of inflammatory factors promotes T cell activity. Elevated PD-L1 expression on cardiac muscle cells and T cells also contributes to the ICI-related cardiac toxicity.
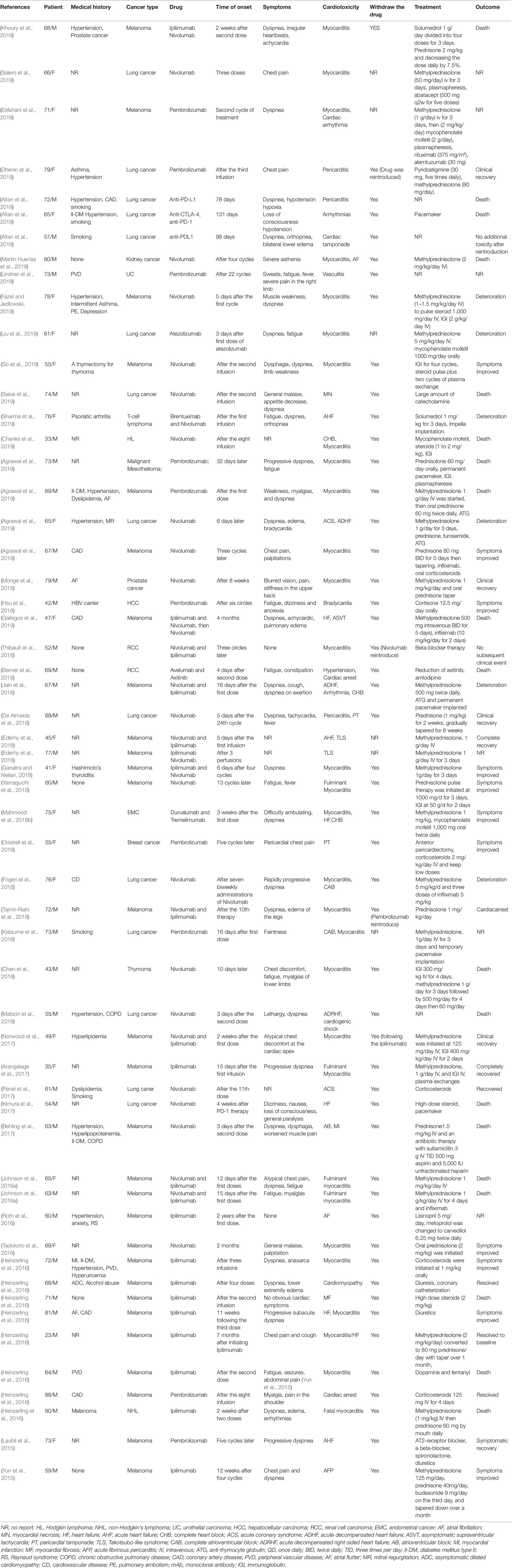
Table 1 Published case reports and case series of immune checkpoint inhibitor-associated cardiotoxicity.
As for the incidence and severity of cardiotoxicity, it is still not well recognized due to limited small-sample retrospective analyses and case reports regarding cardiotoxicity induced by ICIs. A multicenter retrospective study of 752 patients with melanoma treated with ipilimumab had shown that only one case of myocardial fibrosis had occurred (Voskens et al., 2013). Despite the lack of prospective randomized controlled trials to assess myocarditis, retrospective evaluation literature has estimated that the incidence of ICI-related myocarditis ranges from 0.09 (Johnson et al., 2016a) to 1.14% (Mahmood et al., 2018a). Although cardiotoxicity is rare, a high case fatality rate (35%) of myocarditis from ICIs had been reported in a systematic review by Hassan Mir (Mir et al., 2018). A systematic review by Wang et al. of fatal toxic effects associated with ICIs had found that ICI-related myocarditis appeared to present the highest (39.7%) death rate, with 52 deaths among 131 cases (Wang et al., 2018).
In patients treated with the combination of two ICIs, the incidence and death rate of cardiotoxicity is higher than with immunotherapy alone (Larkin et al., 2015b). The largest study of ICI-associated cardiotoxicity to date, using the global database (VigiBase) of the World Health Organization, has revealed that the incidence of myocarditis in patients treated with ICIs is 11 times greater than those without ICI treatment (Salem et al., 2018). A significantly higher case fatality rate (46%) in combination therapy was reported in this study. In addition, myocarditis was found to be more frequent (0.27 vs. 0.06%) and severe (60 vs. 10%) in patients prescribed a combination of nivolumab and ipilimumab than in those prescribed nivolumab alone (Johnson et al., 2016a). For the adverse events induced by the combination of nivolumab and ipilimumab, the rates of 1.3% for tachycardia, 1.1% for hypertension, 0.4% for arrhythmias, and 0.2% for atrial fibrillation were reported by the European Medicines Agency’s European public assessment report, Opdivo (Hassel et al., 2017). Nevertheless, when ICIs are combined with other non-ICI therapies, it remains unknown whether ICI-related myocarditis is more frequent. In a phase 1b trial of 55 patients treated with avelumab (anti-PD-L1 monoclonal antibody) plus axitinib (a vascular endothelial growth factor [VEGF] inhibitor), only one (1.8%) case developed lethal myocarditis (Choueiri et al., 2018).
The time to onset of cardiotoxicity presentation varies depending on the medical history, type of medication, duration of usage, and double or single medication (Table 1). Approximately 80% of ICI-associated myocarditis occurs within the first 3 months of starting ICI therapy (Larkin et al., 2015a; Postow et al., 2015). Approximately 62–64% of patients received only one or two doses of ICIs before the onset of myocarditis (Moslehi et al., 2018; Atallah-Yunes et al., 2019). Cardiac disorders, including myocarditis, pericarditis, and cardiomyopathy are reported to occur between 2 and 17 weeks after ICI treatment onset (Wang et al., 2017; Oristrell et al., 2018). An analysis of an eight-center institutional registry indicated the median time for myocarditis was 34–65 days after initiation of treatment (Mahmood et al., 2018a). In contrast, a patient with melanoma had been reported to develop pericarditis 3 months after four cycles of ipilimumab (Yun et al., 2015). Interestingly, patients without any obvious symptoms had been found to have fulminant myocarditis after 1 year of ICI treatment (Yamaguchi et al., 2018). We reviewed previously published cases of adverse cardiac reactions and found that the onset time of cardiotoxicity was earlier in the combination of two ICIs. In the combination of two ICIs, in more than half (53%) of patients, cardiac toxicity occurred within 4 weeks after ICI initiation, whereas in ICIs alone, cardiac toxicity occurred in 17% of patients around the first ICI dose, and it occurred in 34% of patients 4 months later (Figure 2).
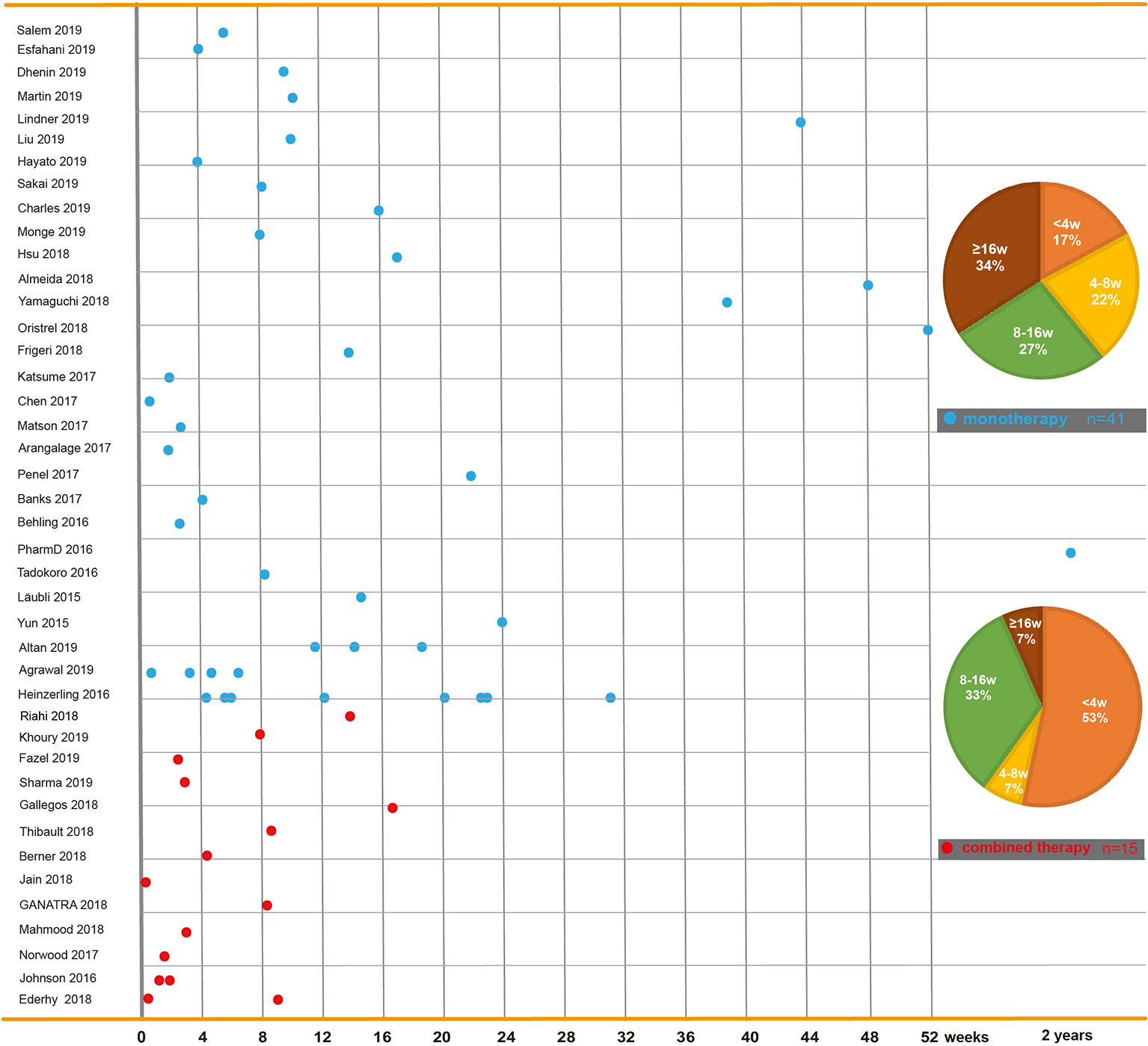
Figure 2 Time to onset of immune checkpoint inhibitor-related cardiac toxicity. In reported cases or case series, reagents including PD-1 inhibitors (nivolumab and pembrolizumab), PD-L1 inhibitors (atezolizumab, avelumab, and durvalumab), and CTLA-4 inhibitors (ipilimumab and tremelimumab) are used solely or in combination. This figure is built based on the cases’ therapy types (monotherapy and combined therapy). The time when the ICI-related cardiac toxicity occurred since the first dose of each case is recorded as a dot. The time to onset of monotherapy (the blue dot) and combined therapy (the red dot) are illustrated. The onset time trend of ICI-related cardiac toxicity in each group is shown. On the right, two pie charts reveal case percentages of different onset time periods in each group.
Potential Mechanism of Immune Checkpoint Inhibitor-Related Cardiac Toxicity
The mechanism of ICI-related cardiac toxicity is not yet fully understood. Histological analyses of patients and monkey models with ICI-associated myocarditis have revealed that the infiltration of predominant CD4+/CD8+ T lymphocytes and a few macrophages (CD68+ cells) are the main cause of ICI-associated myocarditis (Johnson et al., 2016a; Ganatra and Neilan, 2018; Ji et al., 2019) (Figure 1). In addition, the expression change of multiple chemokine receptors further proves the enhancement of T cells. CXCR3–CXCL9/CXCL10 and CCR5/CCL5 are required for T cell activities to upregulate (Tokunaga et al., 2018; Ji et al., 2019). Tumor necrosis factor-α, granzyme B, and interferon-γ are produced by activated T cells, inducing cell death. These inflammatory molecules are overexpressed, which might contribute to cardiac injury (Varricchi et al., 2017; Tocchetti et al., 2018).
The most likely explanation is the “shared antigen” between the tumor and cardiac muscle, with muscle-specific antigens (desmin and troponin) detected in the tumor. Moreover, similar clonal T cell populations have been found infiltrating tumors and cardiac muscle. In this case, hyperproliferative T lymphocytes and macrophages aberrantly infiltrate the cardiac muscle after treatment with ICIs, thereby inducing fatal myocarditis (Johnson et al., 2016a). This theory is also supported by the shared epitope between myeloma cells and cardiomyocytes (Martinez-Calle et al., 2018).
Like tumor cells, cardiomyocytes might also employ the PD-1/PD-L1 and CTLA-4 pathways to prevent T cells from hyper-activation in physiological condition. ICIs, a promising anti-cancer agent, liberate the T-cell inhibition by tumor cells, may also relieve the same type of suppression by cardiomyocytes, which leads to T-cell hyperactivation in the heart. Subsequently, T-cell hyperactivation may result in ICI associated cardiotoxicity. CD28 binds to CD80 (B7-2)/CD86 (B7-2) on antigen-presenting cells (APCs) as a second activation signal to stimulate TCR signaling. CTLA-4 can complete with CD28 for binding with CD80/86 to inhibit the immune response. In contrast, silencing the genes that encode CTLA-4 promotes the proliferation and infiltration of CD8+ T cells in the heart, contributing to the development of myocarditis (Love et al., 2007). In many preclinical models, PD-1 has also been demonstrated to limit the T cell response in the heart as a negative immunoregulatory receptor. The disruption of PD-1 induces CD8+ T cell-mediated autoimmune dilated cardiomyopathy and myocarditis (Nishimura, 2001; Wang et al., 2010), suggesting PD-1 is protective against inflammation and cardiac damage (Tarrio et al., 2012).Similarly, the knockdown of PD-L1 also leads to mortal autoimmune myocarditis in a preclinical model of Murphy Roths Large mice (Lucas et al., 2008). Moreover, in patients with injured myocardium, PD-L1 is overexpressed on injured cardiomyocytes and infiltrating CD8+ T cells (Johnson et al., 2016a). Consistently, in preclinical studies of T cell-mediated myocarditis, the expression of PD-L1 is also upregulated (Grabie et al., 2007).The upregulation of PD-L1 might protect the myocardium from damage; however, this upregulation can be neutralized by ICIs (Grabie et al., 2007). In summary, these limited findings indicate that PD-1/PD-L1 and CTLA-4 play crucial roles in the emergence and development of ICI-related cardiac toxicity. Further studies are needed to elucidate the underlying mechanisms of these effects.
The Diagnosis of Immune Checkpoint Inhibitor-Associated Cardiotoxicity
The detection of ICI-associated cardiotoxicity is currently challenging due to the lack of consistency of its clinical manifestation (Table 1). If ICI-related cardiotoxicity is observed in a patient, a detailed history and physical exams are required to exclude alternative cardiomyopathy etiologies, such as viral and autoimmune cardiac disease, infectious myocarditis or myocardial infarction. This comprehensive diagnostic information is helpful to correct circulation in a timely manner, to provide patients with specific treatment, and to help improve their symptoms (Catena et al., 2009).
For early diagnosis of subclinical myocarditis, serial laboratory tests, electrocardiograms (ECGs) and transthoracic echocardiograms (TTEs) can be beneficial for patients treated with ICIs. Laboratory tests typically include troponin (cardiac troponin I [cTnI] or troponin T [cTnT]), creatine phosphokinase (CPK), creatine kinase (CK), creatine kinase-myocardial band (CK-MB), brain natriuretic peptide (BNP), and N-terminal pro-brain natriuretic peptide (NT-proBNP) (Table 2). Of all the markers, troponin is generally the most sensitive marker for confirming or excluding the diagnosis of myocarditis (Asnani, 2018). Mahmood et al. have indicated that almost all (94%) myocarditis cases had elevated troponin at the time of manifestation (Mahmood et al., 2018a). We reviewed the previous cases/case series and found that 84 and 89% of patients with ICI-associated cardiotoxicity had elevated cTnI and abnormal ECG, respectively (Figure 3). Even very low serum concentrations of troponin can provide important diagnostic and/or prognostic information for oncologists (Jaffe, 2011; Mahajan and Jarolim, 2011; Eggers and Lindahl, 2017). Notably, in patients with myositis, cTnT and CPK can also be elevated; thus, cTnI is the preferred marker for cardiac injury (Hughes et al., 2015). Interestingly, Mahmood et al. have also indicated that higher levels of serum cTnT might be associated with a greater risk of MACE (Mahmood et al., 2018a). BNP or NT-proBNP, the sensitive indicators (Figure 3), were reported to increase in most patients (Lyon et al., 2018); these are also helpful for the diagnosis of ICI-associated cardiotoxicity (Norwood et al., 2017). In cynomolgus monkeys with moderate mononuclear cell infiltration or with myocardial degeneration, an NT-proBNP or cTnI increase was observed at multiple time points after the first dose of ICIs; thus, they might serve as valuable biomarkers for ICI-induced myocarditis (Ji et al., 2019). However, BNP is a poorly specific marker for the diagnosis of ICI-associated cardiotoxicity, because it not only can be elevated in noninflammatory left ventricular dysfunction or other causes of acute cardiac injury, but also in many patients with cancer who have cardiotoxicity (Bando et al., 2017). The elevation of CK can also be observed in ICI-induced myocarditis (Figure 3) (Chen et al., 2018; Agrawal et al., 2019; So et al., 2019). Moreover, several reports have indicated that a complete atrioventricular block is usually associated with ICI-related myocarditis, with a considerably elevated CK level (Heinzerling et al., 2016; Johnson et al., 2016a). Of note, mild to massive elevation of serum CK was found in patients diagnosed with ICI-related myositis or myasthenia gravis (Kimura et al., 2016; Chang et al., 2017; Suzuki et al., 2017; March et al., 2018); therefore, the specificity of elevated CK was relatively poor.
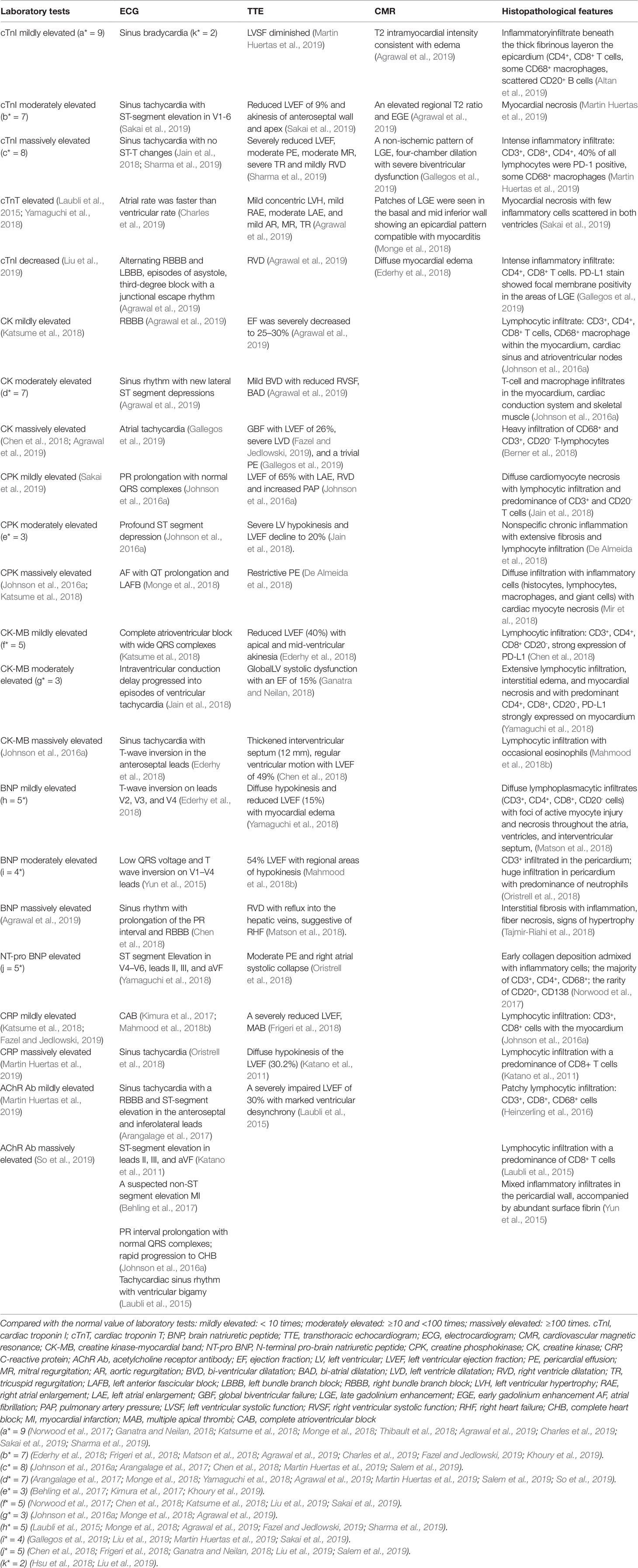
Table 2 Laboratorial, radiological, and histopathological features of immune checkpoint inhibitor-associated cardiotoxicity.
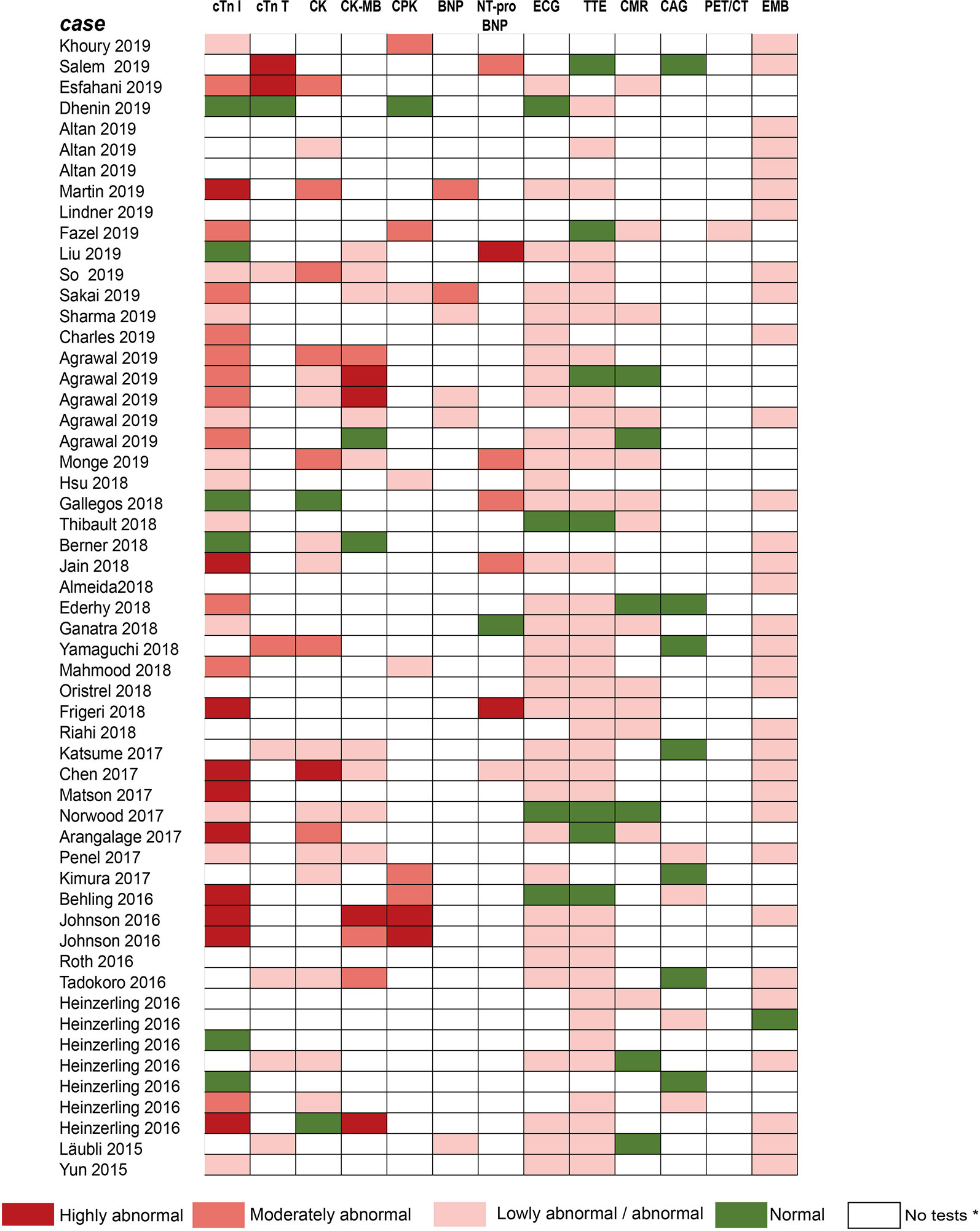
Figure 3 Summarized results of laboratory tests and other examinations for diagnosis in published cases reports/case series. The laboratory tests include cTnI, cTnT, CK, CK-MB, CPK, BNP, NT-pro-BNP. Other examinations include ECG, TTE, CMR, CAG, PET/CT, and EMB. No tests* means no laboratory testing or examination is performed in cases. Compared with the normal value of laboratory tests: Lowly abnormal: 10 times; Moderately abnormal: ≥10 and 100 times; Highly abnormal: ≥100 times. cTnI, cardiac troponin I; cTnT, cardiac troponin T; CPK, creatine phosphokinase; CK, creatine kinase; CK-MB, creatine kinase-myocardial band; BNP, brain natriuretic peptide; NT pro BNP, N-terminal pro-brain natriuretic peptide; ECG, electrocardiograph; TTE, transthoracic echocardiogram; CMR, cardiovascular magnetic resonance; CAG, coronary angiography; PET/CT, positron emission tomography/computed tomography; EMB, endomyocardial biopsy.
Given ECG has widespread availability and is easy to perform, it is considered a first-line test to identify patients with suspected ICI-associated myocarditis (Ganatra and Neilan, 2018). In our review of previous case reports, most patients had had an ECG performed (Figure 3). Abnormal ECGs have been reported in 40–89% of patients with ICI-related cardiotoxicity; however, these changes are often nonspecific (Table 2) (Escudier et al., 2017; Mahmood et al., 2018a). TTE has also been applied to provide further insight into left ventricular ejection fraction (LVEF) impairment, pericardial effusions, and wall motion abnormalities. However, a normal TTE report does not rule out ICI-associated myocarditis (Neilan et al., 2018; Tocchetti et al., 2018). Patients presenting with cardiac marker elevation, ST elevation, and ischemic symptoms should receive emergency coronary angiography to eliminate acute coronary syndrome (Tajiri et al., 2018).
To accurately diagnose myocarditis, further diagnostic technologies, such as cardiac magnetic resonance (CMR) imaging or an endomyocardial biopsy (EMB) are also necessary (Guglin and Nallamshetty, 2012; Bami et al., 2016). Gadolinium contrast-enhanced CMR imaging, a meaningful noninvasive diagnostic tool superior to echocardiography, can identify tissue characterization and offer accurate diagnosis of fibrosis and inflammation for hemodynamically stable patients in the early course of the disease (Friedrich et al., 2009; Aquaro et al., 2017). The CMR features of myocarditis, including edema, necrosis, and scar formation, were previously defined as the Lake Louise Criteria (Table 2) (Friedrich et al., 2009). For instance, an enhanced T2 signal on CMR could be indicative of underlying myocardial edema or myocarditis (Sharma et al., 2019). However, for patients who require invasive hemodynamic or respiratory and/or circulatory support, CMR imaging might not be feasible. Furthermore, although CMR imaging to detect myocardial edema and late gadolinium enhancement is accurate, its sensitivity is relatively poor (Laubli et al., 2015; Escudier et al., 2017; Norwood et al., 2017; Mahmood et al., 2018a) and an absence of positive findings on CMR does not rule out myocarditis (Abdel-Aty et al., 2005).
EMB, a gold standard for the diagnosis of myocarditis (Kindermann et al., 2012; Leone et al., 2012; Caforio et al., 2013), should be conducted when the treatment course is affected by suspected cardiotoxicity. This is especially true in unclear situations in which the oncologist does not know whether to continue or terminate ICI treatment. An EMB can reveal various features of interstitial inflammation suggested by interstitial fibrosis and lymphocyte infiltration (Sharma et al., 2019). Previous reports on ICI-related myocarditis have shown that significant T cells (CD4+, CD8+) and macrophage infiltration were observed in the myocardium (Laubli et al., 2015; Heinzerling et al., 2016; Johnson et al., 2016a; Koelzer et al., 2016; Tadokoro et al., 2016), cardiac conduction system (Johnson et al., 2016a), interventricular septum (Matson et al., 2018) and pericardium (Oristrell et al., 2018) (Table 2). Similar T cell populations were also observed in cardiomyocytes, according to a postmortem report of a patient who died from ICI-induced myocarditis (Johnson et al., 2016a), suggesting hyperactivated cytotoxic T cells directly injuring themyocardium as the probable mechanism of myocarditis induced by ICIs. B lymphocytes and/or plasma cells are usually rare or absent (Altan et al., 2019). Inflammatory infiltration can be transient and focal, however, and can sometimes be inaccessible to pathological puncture. Therefore, a biopsy sampling error from patients with myocarditis can result in a false negative diagnosis (Leone et al., 2012). In this case, it is suggested that EMB should be reattempted in cases with unexplained progressive heart failure (Caforio et al., 2013).
Treatment and Outcome of Immune Checkpoint Inhibitor-Associated Cardiotoxicity
The treatment regimens for ICI-associated cardiotoxicity vary depending on the case (Table 1); however, the principal strategy concentrates on targeting the hyperactive T-cell response. High-dose steroids have constituted the first-line treatment for ICI-related myocarditis (Heinzerling et al., 2016; Johnson et al., 2016a; Kimura et al., 2016; Semper et al., 2016; Tadokoro et al., 2016; Haanen et al., 2017; Brahmer et al., 2018). Prompt initiation of high-dose intravenous methylprednisolone and immediate ICI discontinuation are associated with improved symptoms (Escudier et al., 2017; Mahmood et al., 2018a). A higher starting dose of steroids (intravenous methylprednisolone 1 g) was related to a lower rate of MACE according to a recent study by Mahmood et al. (Mahmood et al., 2018a). Although data regarding treatment for irAEs from rigorous studies are lacking, rapidly initiating intravenous or oral prednisone (1–2 mg/kg) for most patients and intravenous methylprednisolone (0.5–1.0g) for refractory cases with progressive tapering are recommended according to consensus guidelines (Brahmer et al., 2018). However, multiple studies have indicated that corticosteroids alone might not be sufficient to improve immune-mediated cardiac adverse reactions, and patients with ICI-associated cardiac events might even progress to malignant arrhythmias and severe heart failure symptoms during steroid treatment (Heinzerling et al., 2016; Johnson et al., 2016a). Many patients had received corticosteroids early in their cardiotoxicity management, tapering them over 1 month; however, no significant effect was observed (Norwood et al., 2017; Agrawal et al., 2019; Martin Huertas et al., 2019).
For patients with a poor response to corticosteroids, other immunosuppressive drugs should be administered, including immunoglobulin (Caforio et al., 2013), plasmapheresis, mycophenolate mofetil, tacrolimus, and infliximab (Haanen et al., 2017; Jain et al., 2017; Norwood et al., 2017; Reddy et al., 2017; Brahmer et al., 2018; Frigeri et al., 2018; Lyon et al., 2018). Infliximab, a chimeric immunoglobulin G1 monoclonal antibody blocking tumor necrosis factor-α, is used to treat patients with steroid-refractory ICI-associated colitis (Pages et al., 2013). The use of infliximab has been documented in the context of severe steroid-refractory myocarditis (Heinzerling et al., 2016; Johnson et al., 2016a; Tay et al., 2017; Frigeri et al., 2018) and has demonstrated significant clinical recovery and biochemical normalization (Agrawal et al., 2019). It is cautioned that infliximab could be potentially associated with deteriorating heart failure and is prohibited for patients with moderate to severe heart failure (Kwon et al., 2003). Considering the histological similarity between ICI-associated myocarditis and cardiac transplantation rejection, anti-transplant rejection medications (e.g., anti-thymocyte globulin [ATG]) have also been used for treating patients with ICI-related myocarditis (Tay et al., 2017). One case series has indicated that two patients treated with ATG after their clinical course worsened during steroid treatment responded well to ATG therapy, with remission of cardiogenic shock and malignant arrhythmias (Agrawal et al., 2019). The underlying mechanism could be associated with ATG leading to a rapid reduction in lymphocyte infiltration and T cell superactivation, thereby resulting in myocardial conduction improvement (Tay et al., 2017).
Recently, two reports (Esfahani et al., 2019; Salem et al., 2019) have shown that the new therapeutic agents alemtuzumab and CTLA-4 agonists (abatacept and belatacept), could be associated with significant relief of symptoms of cardiotoxic reactions caused by ICIs. Alemtuzumab, a monoclonal antibody that binds to CD52, can result in destruction of complement-mediated peripheral immune cells (monocytes, lymphocytes, macrophages, natural killer cells, and dendritic cells). Although the use of alemtuzumab in the context of cardiac allograft rejection has previously been evaluated, data on its use in patients with irAEs is limited (Cahoon et al., 2012). A recent report has indicated that 30 mg of alemtuzumab led to rapid T-cell depletion and was associated with the resolution of cardiac immunotoxic effects (Esfahani et al., 2019). CTLA-4 agonists, either abatacept or belatacept, can inhibit T cell costimulation mediated by CD28/B7 at the dendritic cell level, thereby abrogating the costimulation of T cells upstream of PD-1/PD-L1 and the CTLA-4 pathways. Abatacept can rapidly cause global T cell anergy (the inactivation of normal immune response) with specific reverse pathways activated by ICIs (Ingelfinger and Schwartz, 2005). When high-dose methylprednisolone injection and sustained plasmapheresis did not work, abatacept resulted in a rapid reduction in cTnI levels and recovery of LVEF (Salem et al., 2019). However, given the potential risks of infectious complications and tumor growth, it is necessary to further evaluate of the risk–benefit balance of abatacept in ICI-induced myocarditis (Ingelfinger and Schwartz, 2005).
Apart from the immunosuppressive therapies above, when necessary, beta-blockers, angiotensin converting enzyme inhibitors (ACEIs), and high-dose aspirin could be required as auxiliary therapies for patients with heart failure and in the context of raised troponin and the indication of cardiac ischemia (Berner et al., 2018). Extracorporeal membrane oxygenation is also required for a patient with severe myocarditis induced by the combination therapy of nivolumab and ipilimumab (Arangalage et al., 2017).
Possible Management of Immune Checkpoint Inhibitor-Associated Cardiotoxicity and Future Directions
Cardiotoxicity, a rare but fatal irAE, is particularly difficult to supervise and manage (Puzanov et al., 2017; Rapoport et al., 2017; Brahmer et al., 2018). Currently, no standard management guidelines on ICI-related cardiotoxicity have been established, due to its low incidence and the limited data on its manifestation, diagnosis, therapy, and outcomes. Based on previous studies and evidence, we summarized the possible management of ICI-related cardiotoxic reactions (Figure 4) as follows.
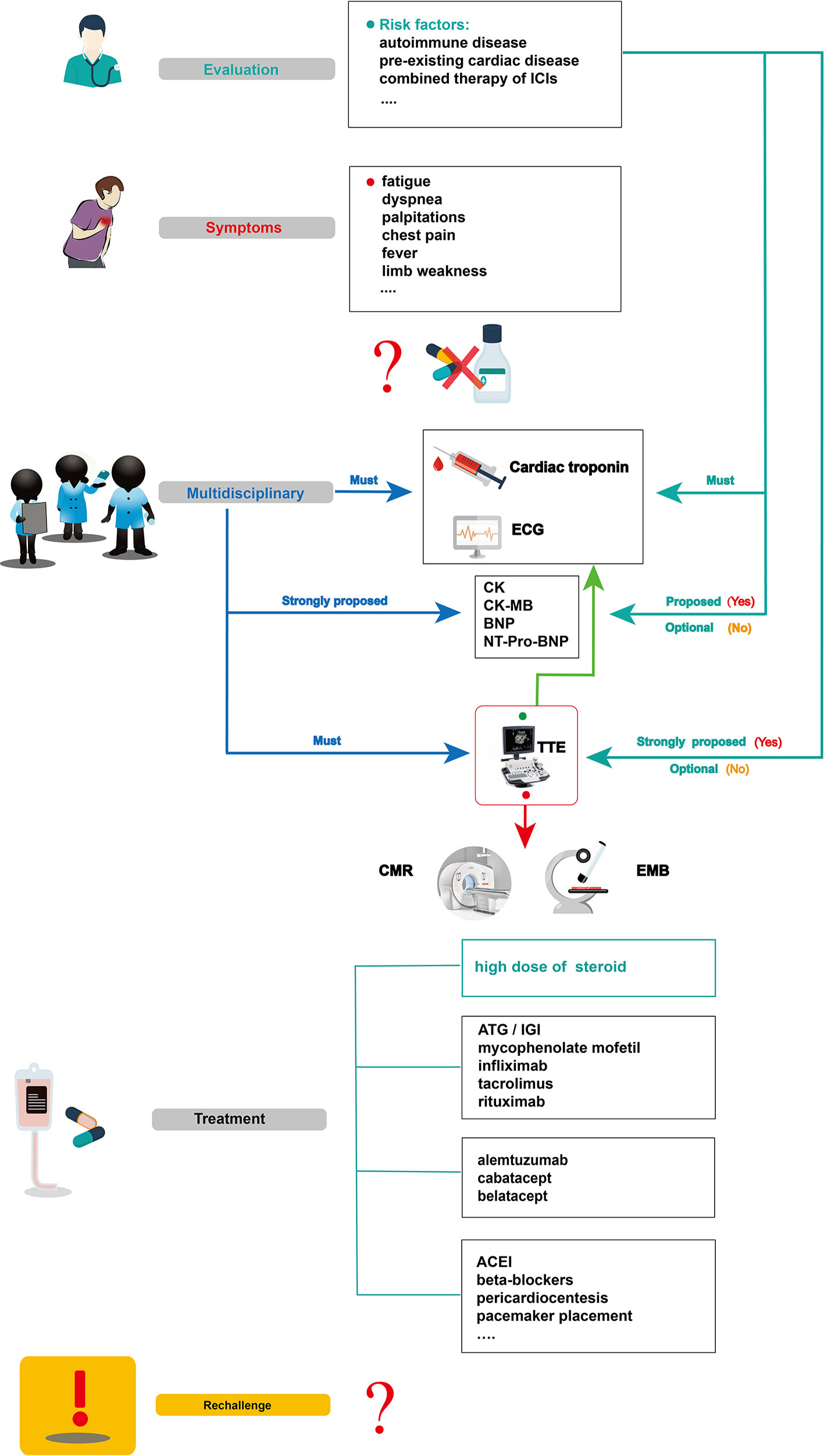
Figure 4 Summarized overview on potential monitoring and management of ICI-related cardiotoxicity. CK, creatine kinase; CK-MB, creatine kinase-myocardial band; BNP, brain natriuretic peptide; NT pro BNP, N-terminal pro-brain natriuretic peptide; TTE, transthoracic echocardiogram; CMR, cardiovascular magnetic resonance; EMB, endomyocardial biopsy; ATG, anti-thymocyte globulin; IGI, immunoglobulin; ACEI, angiotensin converting enzyme inhibitor.
Before initiating ICI therapy, a comprehensive assessment of cardiovascular risk factors and a detailed cardiac history should be obtained for all patients, given the risk factors for ICI-associated cardiotoxicity remain unclear to date. According to previous reports, potential risk factors such as autoimmune disease, pre-existing cardiac disease, the combination of ICIs, and age should be evaluated before ICI treatment. In 50% of patients treated with ipilimumab, pre-existing autoimmune diseases worsened (Bowyer et al., 2016). Several studies have also revealed that patients with underlying autoimmune disease might be at high risk of irAEs, including cardiotoxicity (Johnson et al., 2016b; Johnson et al., 2017; Postow et al., 2018). For instance, patients with systemic autoimmune disorders are more likely to have subclinical myocarditis than those without autoimmune diseases. These results show that clinical and subclinical autoimmune diseases are an important consideration before initiation of ICI therapy (Varricchi et al., 2017). Physicians should be aware of potentially cardiotoxic events during ICI treatment, especially for those with pre-existing cardiac conditions (Hsu et al., 2018). In Heinzerling’s report, pre-existing cardiac disease or peripheral arterial disease had been present in most patients (5 of 8) who developed autoimmune cardiotoxicity (Heinzerling et al., 2016). The combination therapy of ICIs might also be a risk factor for ICI-associated myocarditis (Varricchi et al., 2017). Some VEGF inhibitors can increase the risk of thrombosis and coronary ischemia (Jain et al., 2018). They are also known to be related to cardiotoxicity and left ventricular dysfunction (Shah and Morganroth, 2015). Therefore, when ICIs are combined with anti-VEGF therapies, we should be vigilant for cardiac adverse effects. Given that the common adverse reaction to anti-VEGF therapies, such as axitinib, is hypertension (Hamnvik et al., 2015), it is not a likely causal agent in case of myocarditis; however, it can result in poor heart function in reaction to the physiological challenge. In addition, age can be associated with the incidence of ICI-associated cardiotoxicity. In Wang’s retrospective cohort, fatal irAEs involving cardiovascular toxicities were more common in the elderly than in the young (median,70 vs 62 years, P = .009) (Wang et al., 2018). Other risk factors, such as diabetes mellitus, a history of smoking, and dyslipidemia, might be included (Behling et al., 2017; Norwood et al., 2017; Tomita et al., 2017; Katsume et al., 2018; Agrawal et al., 2019; Altan et al., 2019), however, large sample studies are needed for confirmation.
To accurately diagnose ICI cardiotoxicity, several appropriate steps can be taken into consideration: First, serum troponin tests, baseline ECG, and serial surveillance are necessary. TTE and other laboratory tests, such as CK, CK-MB, BNP, or NT-proBNP can also be performed. Notably, for some special cases with a high risk of cardiotoxicity, TTE is strongly recommended to record the patient’s baseline cardiac function before the initiation of ICIs, and other laboratory inspections should be performed to record their baseline cardiac status. When a cardiotoxic reaction is suspected during treatment with ICIs alone and in combination—for example, the patient’s symptoms might include chest pain, dyspnea, fatigue, palpitations, fever, and limb weakness—one major challenge for oncologists is to identify whether these symptoms are clinical manifestations of cardiovascular irAEs. In a highly suspected ICI-associated cardiotoxic event, temporary discontinuation of ICIs is suggested; it is then necessary for the oncologists, cardiologists, and immunologists to together discuss further diagnosis and treatment. Second, apart from cardiac troponin and ECG, other laboratory biomarker tests, such as serum CK, CK-MB, BNP, and NT-proBNP tests, are strongly proposed. Although a TTE must be performed to record the heart function, normal cardiac function does not rule out ICI-induced myocarditis. CMR is also advisable to further evaluate abnormal cardiac structure. When CMR is not available or contraindicated, cardiac positron emission tomography/computed tomography is beneficial to diagnose myocardial inflammation. Third, in uncertain cases, EMB should be performed and samples from multiple sites should be collected to optimize the diagnostic accuracy of focal myocarditis and reduce sampling errors (Leone et al., 2012). After a comprehensive diagnosis, if there is no evidence of cardiac dysfunction, myocarditis, or other cardiotoxic events, ICI therapy can be slowly reintroduced under close troponin and ECG monitoring.
For patients with confirmed ICI-associated myocarditis, permanent discontinuation of ICIs after cardiac adverse events (G1) has been recommended by the American Society of Clinical Oncology guidelines, though the recommendation is based on anecdotal evidence (Brahmer et al., 2018). Then, prompt administration of oral prednisone (1–2 mg/kg/day) (Jain et al., 2017) or intravenous methylprednisolone (1–2 mg/kg/day) (Puzanov et al., 2017; Wang et al., 2017; Brahmer et al., 2018; De Almeida et al., 2018) should be initiated. If improved signs are observed, a slow tapering dose of glucocorticoid over at least 4 weeks has been recommended (Hu et al., 2019). In patients with pericarditis, despite the resolution of pericardial effusion via pericardial window, prednisone (1 mg/kg) initiation approximately 2 weeks later can prevent constrictive pericarditis (De Almeida et al., 2018). For patients with sick sinus syndrome, a low dose of cortisone (12.5 mg/day) taken orally might help relieve symptoms (Hsu et al., 2018). Nonetheless, if a patient shows a poor response to glucocorticoids, secondary drugs, including ATG, immunoglobulin, infliximab, tacrolimus, mycophenolate mofetil, rituximab, CTLA-4 agonists, and alemtuzumab can be considered (Jain et al., 2017; Norwood et al., 2017; Reddy et al., 2017; Frigeri et al., 2018; Esfahani et al., 2019; Salem et al., 2019). What should be emphasized is that infliximab is generally contraindicated because it can induce congestive heart failure (Kwon et al., 2003). Although the safety and efficacy of these immunosuppressive agents (e.g., ATG, alemtuzumab, abatacept, belatacept) need further confirmation, these drugs could be a viable choice, especially for a critically ill patient with rapidly deteriorating cardiovascular function when high-dose glucocorticoid therapy is not possible.
In parallel with the immunosuppressive agents above, guideline-based therapy and supportive care is recommended for patients with ICI-associated cardiotoxicity. Patients with congestive heart failure should be treated with tolerable medications, including renin-angiotensin system inhibitors and beta-blockers (Yancy et al., 2017). Those with progressive life-threatening arrhythmias should be treated with appropriate antiarrhythmic drugs, or a patient with advanced conduction disease should be considered for temporary/permanent pacemaker placement. When necessary, invasive therapies such as pericardial window placement or pericardiocentesis might also be needed (Yang and Asnani, 2018).
It is controversial whether immunotherapy should be reintroduced after recovery from cardiac toxicity (Brahmer et al., 2018). Although cardiac dysfunction can be significantly improved by high-dose glucocorticoid therapy, an anti-PD1 antibody rechallenge might aggravate immune-related toxicity (Tajmir-Riahi et al., 2018). Given the potential for fulminant or fatal ICI-related myocarditis, ICIs are not recommended for reintroduction in patients (Champiat et al., 2016). However, in a retrospective analysis of 30 patients who were diagnosed with cardiotoxic irAEs, four patients resumed ICIs safely, without cardiotoxic event recurrence (Escudier et al., 2017).Therefore, the clinical oncologist, cardiologist, and immunologist collaboration should give discreet consideration to patients according to their manifestations, outcome, and alternative cancer treatment options to determine the safety of reintroducing ICI therapy.
In summary, the detection and management of ICI-associated cardiotoxic reactions are challenging, and more efforts are needed in future. First and foremost, one of the most important challenges is to improve preventive measures and increase early detection of cardiac toxicity via monitoring of cardiac damage. Second, a multidisciplinary team constituting of oncologists, cardiologists, radiologists, immunologists, and pathologists should be organized to achieve optimal management of ICI-induced cardiotoxicity and to decrease its lethal capacity. Further, developing cardiac protectants that can be used in conjunction with ICIs will be critical in preventing ICI cardiotoxicity. Last but not least, research into new immunotherapeutic agents with unknown cardiotoxicity incidence, such as anti-T cell Ig or anti-lymphocyte-activated gene-3 and mucin-containing protein 3, as well as V-domain Ig suppressor of T cell activation or B and T lymphocyte attenuator blockade, would be a new challenge for physicians. In addition, more clinical trials should focus on the effects of T cell costimulation blockers on cardiovascular disease. For example, CD40–TRAF6 inhibitors have already been well examined. Blocking OX40 and anti-4-1BB costimulation could be a promising strategy in the future (Simons et al., 2019).
Conclusion
Immune checkpoint inhibitors, either alone or in combination, can result in cardiotoxic adverse reactions, such as myocarditis, pericarditis, conduction abnormalities, cardiomyopathy, acute coronary syndrome, and others. Of all ICI-related cardiotoxic events, myocarditis is the most common cardiotoxic reaction. Though the incidence of ICI-associated cardiotoxicity remains relatively low, clinicians must be aware of these adverse events due to their high fatality rate. It mainly occurs in the early stage after ICI initiation, with nonspecific symptoms ranging from asymptomatic cardiac biomarker elevation, fatigue, and general malaise to chest pain, dyspnea, palpitations, multiorgan failure, cardiogenic shock, and cardiac arrest. A high level of clinical suspicion and early diagnosis indicators are required due to the rapid progress and fulminant course of the disease. The assessment of clinical features in combination with laboratory examinations (cTnI, cTnT, CK, CK-MB, BNP, and NT-proBNP), ECG, TTE, CMR, and EMB contribute to the diagnosis of ICI-associated cardiotoxicity. Among these diagnostic methods, troponin is generally the most sensitive marker, ECG has widespread availability and is easily performed, and EMB is a gold standard diagnosis. Before initiating ICIs, a comprehensive assessment of cardiovascular risk factors and a detailed cardiac history should be obtained, especially for patients with autoimmune disease or pre-existing cardiac disease, and when ICIs are combined with other treatments. For patients with confirmed cardiotoxic events, prompt high-dose steroids and other immunosuppressors, such as ATG, immunoglobulin, infliximab, tacrolimus, mycophenolate mofetil, rituximab, CTLA-4 agonists, and alemtuzumab can result in clinical recovery and increased survival. Auxiliary therapies, such as ACEIs, beta-blockers, aspirin, diuretics, antiarrhythmic drugs, pacemaker placement, and pericardiocentesis can also help. In addition, cardiac function assessment and frequent monitoring are necessary. To better understand the pathogenesis of this disease and provide effective treatment strategies, larger studies are needed.
Author Contributions
YZhou collected and reviewed the literature and wrote the manuscript. YZhu wrote and revised the manuscript. MW and YX rechecked the manuscript and put forward meaningful comments on it. CC, TZ, and FX assisted in drawing. JL and ZD contributed equally to writing the design and revised the manuscript. All authors read and approved the final manuscript.
Funding
This study was supported by the National Clinical Research Center for Geriatrics (West China Hospital, Z2018B12),1.3.5 Project for Disciplines of Excellence, West China Hospital, Sichuan University (ZYJC18022), and Sichuan Science and Technology Department Key Research and Development Project (2019YFS0539).
Conflict of Interest
The authors declare that the research was conducted in the absence of any commercial or financial relationships that could be construed as a potential conflict of interest.
References
Abdel-Aty, H., Boye, P., Zagrosek, A., Wassmuth, R., Kumar, A., Messroghli, D., et al. (2005). Diagnostic performance of cardiovascular magnetic resonance in patients with suspected acute myocarditis: comparison of different approaches. J. Am. Coll. Cardiol. 45, 1815–1822. doi: 10.1016/j.jacc.2004.11.069
Agrawal, N., Khunger, A., Vachhani, P., Colvin, T. A., Hattoum, A., Spangenthal, E., et al. (2019). Cardiac toxicity associated with immune checkpoint inhibitors: case series and review of the literature. Case Rep. Oncol. 12, 260–276. doi: 10.1159/000498985
Altan, M., Toki, M. I., Gettinger, S. N., Carvajal-Hausdorf, D. E., Zugazagoitia, J., Sinard, J. H., et al. (2019). Immune checkpoint inhibitor–associated pericarditis. J. Thoracic Oncol. 14, 1102–1108. doi: 10.1016/j.jtho.2019.02.026
Anderson, R. D., Brooks, M. (2016). Apical takotsubo syndrome in a patient with metastatic breast carcinoma on novel immunotherapy. Int. J. Cardiol. 222, 760–761. doi: 10.1016/j.ijcard.2016.07.291
Aquaro, G. D., Perfetti, M., Camastra, G., Monti, L., Dellegrottaglie, S., Moro, C., et al. (2017). Cardiac MR with late gadolinium enhancement in acute myocarditis with preserved systolic function: ITAMY Study. J. Am. Coll. Cardiol. 70, 1977–1987. doi: 10.1016/j.jacc.2017.08.044
Arangalage, D., Delyon, J., Lermuzeaux, M., Ekpe, K., Ederhy, S., Pages, C., et al. (2017). Survival after fulminant myocarditis induced by immune-checkpoint inhibitors. Ann. Intern. Med. 167, 683–684. doi: 10.7326/L17-0396
Asnani, A. (2018). Cardiotoxicity of Immunotherapy: Incidence, Diagnosis, and Management. Curr. Oncol. Rep. 20, 44. doi: 10.1007/s11912-018-0690-1
Atallah-Yunes, S. A., Kadado, A. J., Kaufman, G. P., Hernandez-Montfort, J. (2019). Immune checkpoint inhibitor therapy and myocarditis: a systematic review of reported cases. J. Cancer Res. Clin. Oncol. 145, 1527–1557. doi: 10.1007/s00432-019-02927-x
Bami, K., Haddad, T., Dick, A., Dennie, C., Dwivedi, G. (2016). Noninvasive imaging in acute myocarditis. Curr. Opin. Cardiol. 31, 217–223. doi: 10.1097/hco.0000000000000265
Bando, S., Soeki, T., Matsuura, T., Tobiume, T., Ise, T., Kusunose, K., et al. (2017). Plasma brain natriuretic peptide levels are elevated in patients with cancer. PloS One 12, e0178607. doi: 10.1371/journal.pone.0178607
Behling, J., Kaes, J., Munzel, T., Grabbe, S., Loquai, C. (2017). New-onset third-degree atrioventricular block because of autoimmune-induced myositis under treatment with anti-programmed cell death-1 (nivolumab) for metastatic melanoma. Melanoma Res. 27, 155–158. doi: 10.1097/cmr.0000000000000314
Berner, A. M., Sharma, A., Agarwal, S., Al-Sam, S., Nathan, P. (2018). Fatal autoimmune myocarditis with anti-PD-L1 and tyrosine kinase inhibitor therapy for renal cell cancer. Eur. J. Cancer 101, 287–290. doi: 10.1016/j.ejca.2018.06.021
Boussiotis, V. A. (2016). Molecular and Biochemical Aspects of the PD-1 Checkpoint Pathway. N. Engl. J. Med. 375, 1767–1778. doi: 10.1056/NEJMra1514296
Bowyer, S., Prithviraj, P., Lorigan, P., Larkin, J., Mcarthur, G., Atkinson, V., et al. (2016). Efficacy and toxicity of treatment with the anti-CTLA-4 antibody ipilimumab in patients with metastatic melanoma after prior anti-PD-1 therapy. Br. J. Cancer 114, 1084–1089. doi: 10.1038/bjc.2016.107
Brahmer, J. R., Lacchetti, C., Schneider, B. J., Atkins, M. B., Brassil, K. J., Caterino, J. M., et al. (2018). Management of immune-related adverse events in patients treated with immune checkpoint inhibitor therapy: American Society of Clinical Oncology Clinical Practice Guideline. J. Clin. Oncol. 36, 1714–1768. doi: 10.1200/jco.2017.77.6385
Caforio, A. L., Pankuweit, S., Arbustini, E., Basso, C., Gimeno-Blanes, J., Felix, S. B., et al. (2013). Current state of knowledge on aetiology, diagnosis, management, and therapy of myocarditis: a position statement of the European Society of Cardiology Working Group on Myocardial and Pericardial Diseases. Eur. Heart J. 34, 2636–2648, 2648a-2648d. doi: 10.1093/eurheartj/eht210
Cahoon, W. D., Ensor, C. R., Shullo, M. A. (2012). Alemtuzumab for cytolytic induction of immunosuppression in heart transplant recipients. Prog. Transplant. 22, 344–349; quiz 350.
Catena, E., Paino, R., Milazzo, F., Colombo, T., Marianeschi, S., Lanfranconi, M., et al. (2009). Mechanical circulatory support for patients with fulminant myocarditis: the role of echocardiography to address diagnosis, choice of device, management, and recovery. J. Cardiothorac. Vasc. Anesth. 23, 87–94. doi: 10.1053/j.jvca.2008.03.008
Champiat, S., Lambotte, O., Barreau, E., Belkhir, R., Berdelou, A., Carbonnel, F., et al. (2016). Management of immune checkpoint blockade dysimmune toxicities: a collaborative position paper. Ann. Oncol. 27, 559–574. doi: 10.1093/annonc/mdv623
Chang, E., Sabichi, A. L., Sada, Y. H. (2017). Myasthenia gravis after nivolumab therapy for squamous cell carcinoma of the bladder. J. Immunother. 40, 114–116. doi: 10.1097/cji.0000000000000161
Charles, J., Giovannini, D., Terzi, N., Schwebel, C., Sturm, N., Masson, D., et al. (2019). Multi-organ failure induced by Nivolumab in the context of allo-stem cell transplantation. Exp. Hematol. Oncol. 8, 8. doi: 10.1186/s40164-019-0132-2
Chen, Q., Huang, D. S., Zhang, L. W., Li, Y. Q., Wang, H. W., Liu, H. B. (2018). Fatal myocarditis and rhabdomyolysis induced by nivolumab during the treatment of type B3 thymoma. Clin. Toxicol. (Phila) 56, 667–671. doi: 10.1080/15563650.2017.1401079
Choueiri, T. K., Larkin, J., Oya, M., Thistlethwaite, F., Martignoni, M., Nathan, P., et al. (2018). Preliminary results for avelumab plus axitinib as first-line therapy in patients with advanced clear-cell renal-cell carcinoma (JAVELIN Renal 100): an open-label, dose-finding and dose-expansion, phase 1b trial. Lancet Oncol. 19, 451–460. doi: 10.1016/s1470-2045(18)30107-4
De Almeida, D. V. P., Gomes, J. R., Haddad, F. J., Buzaid, A. C. (2018). Immune-mediated pericarditis with pericardial tamponade during nivolumab therapy. J. Immunother. 41, 329–331. doi: 10.1097/cji.0000000000000217
Dhenin, A., Samartzi, V., Lejeune, S., Seront, E. (2019). Cascade of immunologic adverse events related to pembrolizumab treatment. BMJ Case Rep. 12, 6. doi: 10.1136/bcr-2018-229149
Ederhy, S., Cautela, J., Ancedy, Y., Escudier, M., Thuny, F., Cohen, A. (2018). Takotsubo-like syndrome in cancer patients treated with immune checkpoint inhibitors. JACC Cardiovasc. Imaging 11, 1187–1190. doi: 10.1016/j.jcmg.2017.11.036
Eggers, K. M., Lindahl, B. (2017). Application of cardiac troponin in cardiovascular diseases other than acute coronary syndrome. Clin. Chem. 63, 223–235. doi: 10.1373/clinchem.2016.261495
Escudier, M., Cautela, J., Malissen, N., Ancedy, Y., Orabona, M., Pinto, J., et al. (2017). Clinical features, management, and outcomes of immune checkpoint inhibitor-related cardiotoxicity. Circulation 136, 2085–2087. doi: 10.1161/circulationaha.117.030571
Esfahani, K., Buhlaiga, N., Thébault, P., Lapointe, R., Johnson, N. A., Miller, W. H. (2019). Alemtuzumab for immune-related myocarditis due to PD-1 therapy. New Engl. J. Med. 380, 2375–2376. doi: 10.1056/NEJMc1903064
Fazel, M., Jedlowski, P. M. (2019). Severe myositis, myocarditis, and myasthenia gravis with elevated anti-striated muscle antibody following single dose of ipilimumab-nivolumab therapy in a patient with metastatic melanoma. Case Rep. Immunol. 2019, 2539493. doi: 10.1155/2019/2539493
Friedman, C. F., Proverbs-Singh, T. A., Postow, M. A. (2016). Treatment of the immune-related adverse effects of immune checkpoint inhibitors: a review. JAMA Oncol. 2, 1346–1353. doi: 10.1001/jamaoncol.2016.1051
Friedrich, M. G., Sechtem, U., Schulz-Menger, J., Holmvang, G., Alakija, P., Cooper, L. T., et al. (2009). Cardiovascular magnetic resonance in myocarditis: A JACC White Paper. J. Am. Coll. Cardiol. 53, 1475–1487. doi: 10.1016/j.jacc.2009.02.007
Frigeri, M., Meyer, P., Banfi, C., Giraud, R., Hachulla, A. L., Spoerl, D., et al. (2018). Immune checkpoint inhibitor-associated myocarditis: a new challenge for cardiologists. Can. J. Cardiol. 34, 92 e91–92 e93. doi: 10.1016/j.cjca.2017.09.025
Gallegos, C., Rottmann, D., Nguyen, V. Q., Baldassarre, L. A. (2019). Myocarditis with checkpoint inhibitor immunotherapy: case report of late gadolinium enhancement on cardiac magnetic resonance with pathology correlate. Eur. Heart J. Case Rep. 3, yty149. doi: 10.1093/ehjcr/yty149
Ganatra, S., Neilan, T. G. (2018). Immune checkpoint inhibitor-associated myocarditis. Oncologist 23, 879–886. doi: 10.1634/theoncologist.2018-0130
Geisler, B. P., Raad, R. A., Esaian, D., Sharon, E., Schwartz, D. R. (2015). Apical ballooning and cardiomyopathy in a melanoma patient treated with ipilimumab: a case of takotsubo-like syndrome. J. Immunother. Cancer 3, 4. doi: 10.1186/s40425-015-0048-2
Gibson, R., Delaune, J., Szady, A., Markham, M. (2016). Suspected autoimmune myocarditis and cardiac conduction abnormalities with nivolumab therapy for non-small cell lung cancer. BMJ Case Rep. doi: 10.1136/bcr-2016-216228
Grabie, N., Gotsman, I., Dacosta, R., Pang, H., Stavrakis, G., Butte, M. J., et al. (2007). Endothelial programmed death-1 ligand 1 (PD-L1) regulates CD8+ T-cell mediated injury in the heart. Circulation 116, 2062–2071. doi: 10.1161/CIRCULATIONAHA.107.709360
Guglin, M., Nallamshetty, L. (2012). Myocarditis: diagnosis and treatment. Curr. Treat Options Cardiovasc. Med. 14, 637–651. doi: 10.1007/s11936-012-0204-7
Haanen, J., Carbonnel, F., Robert, C., Kerr, K. M., Peters, S., Larkin, J., et al. (2017). Management of toxicities from immunotherapy: ESMO Clinical Practice Guidelines for diagnosis, treatment and follow-up. Ann. Oncol. 28, iv119–iv142. doi: 10.1093/annonc/mdx225
Hamnvik, O. P., Choueiri, T. K., Turchin, A., Mckay, R. R., Goyal, L., Davis, M., et al. (2015). Clinical risk factors for the development of hypertension in patients treated with inhibitors of the VEGF signaling pathway. Cancer 121, 311–319. doi: 10.1002/cncr.28972
Hassel, J. C., Heinzerling, L., Aberle, J., Bahr, O., Eigentler, T. K., Grimm, M. O., et al. (2017). Combined immune checkpoint blockade (anti-PD-1/anti-CTLA-4): Evaluation and management of adverse drug reactions. Cancer Treat Rev. 57, 36–49. doi: 10.1016/j.ctrv.2017.05.003
Heery, C. R., Coyne, G. H. O. S., Madan, R. A. (2014). Phase I open-label, multiple ascending dose trial of MSB0010718C, an anti-PD-L1 monoclonal antibody, in advanced solid malignancies. J Clin Oncol. 32, 15 suppl:3064a.
Heinzerling, L., Ott, P. A., Hodi, F. S., Husain, A. N., Tajmir-Riahi, A., Tawbi, H., et al. (2016). Cardiotoxicity associated with CTLA4 and PD1 blocking immunotherapy. J. Immunother. Cancer 4, 50. doi: 10.1186/s40425-016-0152-y
Hino, R., Kabashima, K., Kato, Y., Yagi, H., Nakamura, M., Honjo, T., et al. (2010). Tumor cell expression of programmed cell death-1 ligand 1 is a prognostic factor for malignant melanoma. Cancer 116, 1757–1766. doi: 10.1002/cncr.24899
Hsu, C. Y., Su, Y. W., Chen, S. C. (2018). Sick sinus syndrome associated with anti-programmed cell death-1. J. Immunother. Cancer 6, 72. doi: 10.1186/s40425-018-0388-9
Hu, J. R., Florido, R., Lipson, E. J., Naidoo, J., Ardehali, R., Tocchetti, C. G., et al. (2019). Cardiovascular toxicities associated with immune checkpoint inhibitors. Cardiovasc. Res. 115, 854–868. doi: 10.1093/cvr/cvz026
Hughes, M., Lilleker, J. B., Herrick, A. L., Chinoy, H. (2015). Cardiac troponin testing in idiopathic inflammatory myopathies and systemic sclerosis-spectrum disorders: biomarkers to distinguish between primary cardiac involvement and low-grade skeletal muscle disease activity. Ann. Rheumatol. Dis. 74, 795–798. doi: 10.1136/annrheumdis-2014-206812
Ingelfinger, J. R., Schwartz, R. S. (2005). Immunosuppression–the promise of specificity. N. Engl. J. Med. 353, 836–839. doi: 10.1056/NEJMe058166
Jaffe, A. S. (2011). The 10 commandments of troponin, with special reference to high sensitivity assays. Heart 97, 940–946. doi: 10.1136/hrt.2009.185751
Jain, V., Bahia, J., Mohebtash, M., Barac, A. (2017). Cardiovascular complications associated with novel cancer immunotherapies. Curr. Treat Options Cardiovasc. Med. 19, 36. doi: 10.1007/s11936-017-0532-8
Jain, V., Mohebtash, M., Rodrigo, M. E., Ruiz, G., Atkins, M. B., Barac, A. (2018). Autoimmune myocarditis caused by immune checkpoint inhibitors treated with antithymocyte globulin. J. Immunother. 41, 332–335. doi: 10.1097/cji.0000000000000239
Ji, C., Roy, M. D., Golas, J., Vitsky, A., Ram, S., Kumpf, S. W., et al. (2019). Myocarditis in cynomolgus monkeys following treatment with immune checkpoint inhibitors. Clin. Cancer Res. 25, 4735–4748. doi: 10.1158/1078-0432.CCR-18-4083
Johnson, D. B., Balko, J. M., Compton, M. L., Chalkias, S., Gorham, J., Xu, Y., et al. (2016a). Fulminant myocarditis with combination immune checkpoint blockade. N. Engl. J. Med. 375, 1749–1755. doi: 10.1056/NEJMoa1609214
Johnson, D. B., Sullivan, R. J., Menzies, A. M. (2017). Immune checkpoint inhibitors in challenging populations. Cancer 123, 1904–1911. doi: 10.1002/cncr.30642
Johnson, D. B., Sullivan, R. J., Ott, P. A., Carlino, M. S., Khushalani, N. I., Ye, F., et al. (2016b). Ipilimumab therapy in patients with advanced melanoma and preexisting autoimmune disorders. JAMA Oncol. 2, 234–240. doi: 10.1001/jamaoncol.2015.4368
Katano, H., Kano, M., Nakamura, T., Kanno, T., Asanuma, H., Sata, T. (2011). A novel real-time PCR system for simultaneous detection of human viruses in clinical samples from patients with uncertain diagnoses. J. Med. Virol. 83, 322–330. doi: 10.1002/jmv.21962
Katsume, Y., Isawa, T., Toi, Y., Fukuda, R., Kondo, Y., Sugawara, S., et al. (2018). Complete atrioventricular block associated with pembrolizumab-induced acute myocarditis: the need for close cardiac monitoring. Intern. Med. 57, 3157–3162. doi: 10.2169/internalmedicine.0255-17
Khoury, Z. H., Hausner, P. F., Idzik-Starr, C. L., Frykenberg, M. R. A., Brooks, J. K., Dyalram, D., et al. (2019). Combination nivolumab/ipilimumab immunotherapy for melanoma with subsequent unexpected cardiac arrest: a case report and review of literature. J. Immunother. 42, 313–317. doi: 10.1097/cji.0000000000000282
Kimura, H., Tounai, Y., Nagato, K., Morimoto, J. I., Tounai, Y. (2017). A case of heart failure after treatment with anti-PD-1 antibody followed by adoptive transfer of cytokine-activated killer cells in a recurrent lung cancer patient. J. Thorac. Oncol. 12, e128–e130. doi: 10.1016/j.jtho.2017.04.024
Kimura, T., Fukushima, S., Miyashita, A., Aoi, J., Jinnin, M., Kosaka, T., et al. (2016). Myasthenic crisis and polymyositis induced by one dose of nivolumab. Cancer Sci. 107, 1055–1058. doi: 10.1111/cas.12961
Kindermann, I., Barth, C., Mahfoud, F., Ukena, C., Lenski, M., Yilmaz, A., et al. (2012). Update on myocarditis. J. Am. Coll. Cardiol. 59, 779–792. doi: 10.1016/j.jacc.2011.09.074
Koelzer, V. H., Rothschild, S. I., Zihler, D., Wicki, A., Willi, B., Willi, N., et al. (2016). Systemic inflammation in a melanoma patient treated with immune checkpoint inhibitors-an autopsy study. J. Immunother. Cancer 4, 13. doi: 10.1186/s40425-016-0117-1
Kwon, H. J., Cote, T. R., Cuffe, M. S., Kramer, J. M., Braun, M. M. (2003). Case reports of heart failure after therapy with a tumor necrosis factor antagonist. Ann. Intern. Med. 138, 807–811. doi: 10.7326/0003-4819-138-10-200305200-00008
Larkin, J., Chiarion-Sileni, V., Gonzalez, R., Grob, J. J., Cowey, C. L., Lao, C. D., et al. (2015a). Combined Nivolumab and Ipilimumab or Monotherapy in Untreated Melanoma. N. Engl. J. Med. 373, 23–34. doi: 10.1056/NEJMoa1504030
Larkin, J., Hodi, F. S., Wolchok, J. D. (2015b). Combined Nivolumab and Ipilimumab or Monotherapy in Untreated Melanoma. N. Engl. J. Med. 373, 1270–1271. doi: 10.1056/NEJMc1509660
Laubli, H., Balmelli, C., Bossard, M., Pfister, O., Glatz, K., Zippelius, A. (2015). Acute heart failure due to autoimmune myocarditis under pembrolizumab treatment for metastatic melanoma. J. Immunother. Cancer 3, 11. doi: 10.1186/s40425-015-0057-1
Leone, O., Veinot, J. P., Angelini, A., Baandrup, U. T., Basso, C., Berry, G., et al. (2012). 2011 consensus statement on endomyocardial biopsy from the Association for European Cardiovascular Pathology and the Society for Cardiovascular Pathology. Cardiovasc. Pathol. 21, 245–274. doi: 10.1016/j.carpath.2011.10.001
Lindner, A. K., Gruenbacher, G., Schachtner, G., Thurnher, M., Pichler, R. (2019). Rare, but severe: vasculitis and checkpoint inhibitors. Eur. Urol. Focus. doi: 10.1016/j.euf.2019.04.014
Liu, S. Y., Huang, W. C., Yeh, H. I., Ko, C. C., Shieh, H. R., Hung, C. L., et al. (2019). Sequential blockade of PD-1 and PD-L1 causes fulminant cardiotoxicity-from case report to mouse model validation. Cancers (Basel). 11, 4. doi: 10.3390/cancers11040580
Love, V. A., Grabie, N., Duramad, P., Stavrakis, G., Sharpe, A., Lichtman, A. (2007). CTLA-4 ablation and interleukin-12 driven differentiation synergistically augment cardiac pathogenicity of cytotoxic T lymphocytes. Circ. Res. 101, 248–257. doi: 10.1161/CIRCRESAHA.106.147124
Lucas, J. A., Menke, J., Rabacal, W. A., Schoen, F. J., Sharpe, A. H., Kelley, V. R. (2008). Programmed death ligand 1 regulates a critical checkpoint for autoimmune myocarditis and pneumonitis in MRL mice. J. Immunol. 181, 2513–2521. doi: 10.4049/jimmunol.181.4.2513
Lyon, A. R., Yousaf, N., Battisti, N. M. L., Moslehi, J., Larkin, J. (2018). Immune checkpoint inhibitors and cardiovascular toxicity. Lancet Oncol. 19, e447–e458. doi: 10.1016/s1470-2045(18)30457-1
Mahajan, V. S., Jarolim, P. (2011). How to interpret elevated cardiac troponin levels. Circulation 124, 2350–2354. doi: 10.1161/circulationaha.111.023697
Mahmood, S. S., Fradley, M. G., Cohen, J. V., Nohria, A., Reynolds, K. L., Heinzerling, L. M., et al. (2018a). Myocarditis in patients treated with immune checkpoint inhibitors. J. Am. Coll. Cardiol. 71, 1755–1764. doi: 10.1016/j.jacc.2018.02.037
Mahmood, S. S., Chen, C. L., Shapnik, N., Krishnan, U., Singh, H. S., Makker, V. (2018b). Myocarditis with tremelimumab plus durvalumab combination therapy for endometrial cancer: a case report. Gynecol. Oncol. Rep. 25, 74–77. doi: 10.1016/j.gore.2018.05.014
March, K. L., Samarin, M. J., Sodhi, A., Owens, R. E. (2018). Pembrolizumab-induced myasthenia gravis: A fatal case report. J. Oncol. Pharm. Pract. 24, 146–149. doi: 10.1177/1078155216687389
Martin Huertas, R., Saavedra Serrano, C., Perna, C., Ferrer Gomez, A., Alonso Gordoa, T. (2019). Cardiac toxicity of immune-checkpoint inhibitors: a clinical case of nivolumab-induced myocarditis and review of the evidence and new challenges. Cancer Manage. Res. 11, 4541–4548. doi: 10.2147/CMAR.S185202
Martinez-Calle, N., Rodriguez-Otero, P., Villar, S., Mejias, L., Melero, I., Prosper, F., et al. (2018). Anti-PD1 associated fulminant myocarditis after a single pembrolizumab dose: the role of occult pre-existing autoimmunity. Haematologica 103, e318–e321. doi: 10.3324/haematol.2017.185777
Matson, D. R., Accola, M. A., Rehrauer, W. M., Corliss, R. F. (2018). Fatal Myocarditis Following Treatment with the PD-1 Inhibitor Nivolumab. J. Forensic. Sci. 63, 954–957. doi: 10.1111/1556-4029.13633
Michot, J. M., Bigenwald, C., Champiat, S., Collins, M., Carbonnel, F., Postel-Vinay, S., et al. (2016). Immune-related adverse events with immune checkpoint blockade: a comprehensive review. Eur. J. Cancer 54, 139–148. doi: 10.1016/j.ejca.2015.11.016
Mir, H., Alhussein, M., Alrashidi, S., Alzayer, H., Alshatti, A., Valettas, N., et al. (2018). Cardiac complications associated with checkpoint inhibition: a systematic review of the literature in an important emerging area. Can. J. Cardiol. 34, 1059–1068. doi: 10.1016/j.cjca.2018.03.012
Monge, C., Maeng, H., Brofferio, A., Apolo, A. B., Sathya, B., Arai, A. E., et al. (2018). Myocarditis in a patient treated with Nivolumab and PROSTVAC: a case report. J. Immunother. Cancer 6, 150. doi: 10.1186/s40425-018-0473-0
Moslehi, J. J., Salem, J. E., Sosman, J. A., Lebrun-Vignes, B., Johnson, D. B. (2018). Increased reporting of fatal immune checkpoint inhibitor-associated myocarditis. Lancet 391, 933. doi: 10.1016/s0140-6736(18)30533-6
Neilan, T. G., Rothenberg, M. L., Amiri-Kordestani, L., Sullivan, R. J., Steingart, R. M., Gregory, W., et al. (2018). Myocarditis associated with immune checkpoint inhibitors: an expert consensus on data gaps and a call to action. Oncologist 23, 874–878. doi: 10.1634/theoncologist.2018-0157
Nishimura, H. (2001). Autoimmune Dilated Cardiomyopathy in PD-1 Receptor-Deficient Mice. Science 291, 319–322. doi: 10.1126/science.291.5502.319
Nishino, M., Sholl, L. M., Hodi, F. S., Hatabu, H., Ramaiya, N. H. (2015). Anti-PD-1-related pneumonitis during cancer immunotherapy. N. Engl. J. Med. 373, 288–290. doi: 10.1056/NEJMc1505197
Norwood, T. G., Westbrook, B. C., Johnson, D. B., Litovsky, S. H., Terry, N. L., Mckee, S. B., et al. (2017). Smoldering myocarditis following immune checkpoint blockade. J. Immunother. Cancer 5, 91. doi: 10.1186/s40425-017-0296-4
Nykl, R., Fischer, O., Vykoupil, K., Taborsky, M. (2017). A unique reason for coronary spasm causing temporary ST elevation myocardial infarction (inferior STEMI) - systemic inflammatory response syndrome after use of pembrolizumab. Arch. Med. Sci. Atheroscler. Dis. 2, e100–e102. doi: 10.5114/amsad.2017.72531
Oristrell, G., Baneras, J., Ros, J., Munoz, E. (2018). Cardiac tamponade and adrenal insufficiency due to pembrolizumab: a case report. Eur. Heart J. Case Rep. 2, yty038. doi: 10.1093/ehjcr/yty038
Pages, C., Gornet, J. M., Monsel, G., Allez, M., Bertheau, P., Bagot, M., et al. (2013). Ipilimumab-induced acute severe colitis treated by infliximab. Melanoma Res. 23, 227–230. doi: 10.1097/CMR.0b013e32835fb524
Peggs, K. S., Quezada, S. A., Chambers, C. A., Korman, A. J., Allison, J. P. (2009). Blockade of CTLA-4 on both effector and regulatory T cell compartments contributes to the antitumor activity of anti-CTLA-4 antibodies. J. Exp. Med. 206, 1717–1725. doi: 10.1084/jem.20082492
Penel, N., Cordoba, A., Mathoulin-Pelissier, S. (2017). Lessons from three phase III trials assessing neoadjuvant treatment in sarcoma patients. Ann. Oncol. 28, 2891–2893. doi: 10.1093/annonc/mdx454
Pi, G., He, H., Bi, J., Li, Y., Li, Y., Zhang, Y., et al. (2016). Efficacy of short-term nivolumab treatment in a Chinese patient with relapsed advanced-stage lung squamous cell carcinoma: A case report. Med. (Baltimore) 95, e5077. doi: 10.1097/md.0000000000005077
Postow, M. A., Chesney, J., Pavlick, A. C., Robert, C., Grossmann, K., Mcdermott, D., et al. (2015). Nivolumab and ipilimumab versus ipilimumab in untreated melanoma. N. Engl. J. Med. 372, 2006–2017. doi: 10.1056/NEJMoa1414428
Postow, M. A., Sidlow, R., Hellmann, M. D. (2018). Immune-related adverse events associated with immune checkpoint blockade. N. Engl. J. Med. 378, 158–168. doi: 10.1056/NEJMra1703481
Powles, T., Eder, J. P., Fine, G. D., Braiteh, F. S., Loriot, Y., Cruz, C., et al. (2014). MPDL3280A (anti-PD-L1) treatment leads to clinical activity in metastatic bladder cancer. Nature 515, 558–562. doi: 10.1038/nature13904
Puzanov, I., Diab, A., Abdallah, K., Bingham, C. O., Brogdon, C., Dadu, R., et al. (2017). Managing toxicities associated with immune checkpoint inhibitors: consensus recommendations from the Society for Immunotherapy of Cancer (SITC) Toxicity Management Working Group. J. Immunother. Cancer 5, 95. doi: 10.1186/s40425-017-0300-z
Rapoport, B. L., Van Eeden, R., Sibaud, V., Epstein, J. B., Klastersky, J., Aapro, M., et al. (2017). Supportive care for patients undergoing immunotherapy. Support Care Cancer 25, 3017–3030. doi: 10.1007/s00520-017-3802-9
Reddy, N., Moudgil, R., Lopez-Mattei, J. C., Karimzad, K., Mouhayar, E. N., Somaiah, N., et al. (2017). Progressive and Reversible Conduction Disease With Checkpoint Inhibitors. Can. J. Cardiol. 33, 1335.e1313–1335.e1315. doi: 10.1016/j.cjca.2017.05.026
Reuben, A., Petaccia De Macedo, M., Mcquade, J., Joon, A., Ren, Z., Calderone, T., et al. (2017). Comparative immunologic characterization of autoimmune giant cell myocarditis with ipilimumab. Oncoimmunology 6, e1361097. doi: 10.1080/2162402x.2017.1361097
Ribas, A., Wolchok, J. D. (2018). Cancer immunotherapy using checkpoint blockade. Science 359, 1350–1355. doi: 10.1126/science.aar4060
Roth, M. E., Muluneh, B., Jensen, B. C., Madamanchi, C., Lee, C. B. (2016). Left ventricular dysfunction after treatment with ipilimumab for metastatic Melanoma. Am. J. Ther. 23, e1925–e1928. doi: 10.1097/mjt.0000000000000430
Sakai, T., Yahagi, K., Hoshino, T., Yokota, T., Tanabe, K., Mori, M., et al. (2019). Nivolumab-induced myocardial necrosis in a patient with lung cancer: A case report. Respir. Med. Case Rep. 27, 100839. doi: 10.1016/j.rmcr.2019.100839
Salem, J. E., Allenbach, Y., Vozy, A., Brechot, N., Johnson, D. B., Moslehi, J. J., et al. (2019). Abatacept for severe immune checkpoint inhibitor-associated myocarditis. N. Engl. J. Med. 380, 2377–2379. doi: 10.1056/NEJMc1901677
Salem, J. E., Manouchehri, A., Moey, M., Lebrun-Vignes, B., Bastarache, L., Pariente, A., et al. (2018). Cardiovascular toxicities associated with immune checkpoint inhibitors: an observational, retrospective, pharmacovigilance study. Lancet Oncol. 19, 1579–1589. doi: 10.1016/s1470-2045(18)30608-9
Samara, Y., Yu, C. L., Dasanu, C. A. (2019). Acute autoimmune myocarditis and hepatitis due to ipilimumab monotherapy for malignant melanoma. J. Oncol. Pharm. Pract. 25, 966–968. doi: 10.1177/1078155218755868
Semper, H., Muehlberg, F., Schulz-Menger, J., Allewelt, M., Grohe, C. (2016). Drug-induced myocarditis after nivolumab treatment in a patient with PDL1- negative squamous cell carcinoma of the lung. Lung Cancer 99, 117–119. doi: 10.1016/j.lungcan.2016.06.025
Shah, R. R., Morganroth, J. (2015). Update on cardiovascular safety of tyrosine kinase inhibitors: with a special focus on QT interval, left ventricular dysfunction and overall risk/benefit. Drug Saf. 38, 693–710. doi: 10.1007/s40264-015-0300-1
Sharma, M., Suero-Abreu, G. A., Kim, B. (2019). A Case of Acute Heart Failure due to Immune Checkpoint Blocker Nivolumab. Cardiol. Res. 10, 120–123. doi: 10.14740/cr838
Simons, K. H., De Jong, A., Jukema, J. W., De Vries, M. R., Arens, R., Quax, P. H. A. (2019). T cell co-stimulation and co-inhibition in cardiovascular disease: a double-edged sword. Nat. Rev. Cardiol. 16, 325–343. doi: 10.1038/s41569-019-0164-7
So, H., Ikeguchi, R., Kobayashi, M., Suzuki, M., Shimizu, Y., Kitagawa, K. (2019). PD-1 inhibitor-associated severe myasthenia gravis with necrotizing myopathy and myocarditis. J. Neurol. Sci. 399, 97–100. doi: 10.1016/j.jns.2019.02.023
Suzuki, S., Ishikawa, N., Konoeda, F., Seki, N., Fukushima, S., Takahashi, K., et al. (2017). Nivolumab-related myasthenia gravis with myositis and myocarditis in Japan. Neurology 89, 1127–1134. doi: 10.1212/wnl.0000000000004359
Swaika, A., Hammond, W. A., Joseph, R. W. (2015). Current state of anti-PD-L1 and anti-PD-1 agents in cancer therapy. Mol. Immunol. 67, 4–17. doi: 10.1016/j.molimm.2015.02.009
Tadokoro, T., Keshino, E., Makiyama, A., Sasaguri, T., Ohshima, K., Katano, H., et al. (2016). Acute lymphocytic myocarditis with anti-PD-1 antibody nivolumab. Circ. Heart Fail. 9, 10. doi: 10.1161/circheartfailure.116.003514
Tajiri, K., Aonuma, K., Sekine, I. (2018). Immune checkpoint inhibitor-related myocarditis. Jpn. J. Clin. Oncol. 48, 7–12. doi: 10.1093/jjco/hyx154
Tajmir-Riahi, A., Bergmann, T., Schmid, M., Agaimy, A., Schuler, G., Heinzerling, L. (2018). Life-threatening autoimmune cardiomyopathy reproducibly induced in a patient by checkpoint inhibitor therapy. J. Immunother. 41, 35–38. doi: 10.1097/cji.0000000000000190
Tarrio, M. L., Grabie, N., Bu, D. X., Sharpe, A. H., Lichtman, A. H. (2012). PD-1 protects against inflammation and myocyte damage in T cell-mediated myocarditis. J. Immunol. 188, 4876–4884. doi: 10.4049/jimmunol.1200389
Tay, R. Y., Blackley, E., Mclean, C., Moore, M., Bergin, P., Gill, S., et al. (2017). Successful use of equine anti-thymocyte globulin (ATGAM) for fulminant myocarditis secondary to nivolumab therapy. Br. J. Cancer 117, 921–924. doi: 10.1038/bjc.2017.253
Thibault, C., Vano, Y., Soulat, G., Mirabel, M. (2018). Immune checkpoint inhibitors myocarditis: not all cases are clinically patent. Eur. Heart J. 39, 3553. doi: 10.1093/eurheartj/ehy485
Tocchetti, C. G., Galdiero, M. R., Varricchi, G. (2018). Cardiac toxicity in patients treated with immune checkpoint inhibitors: it is now time for cardio-immuno-oncology. J. Am. Coll. Cardiol. 71, 1765–1767. doi: 10.1016/j.jacc.2018.02.038
Tokunaga, R., Zhang, W., Naseem, M., Puccini, A., Berger, M. D., Soni, S., et al. (2018). CXCL9, CXCL10, CXCL11/CXCR3 axis for immune activation - A target for novel cancer therapy. Cancer Treat. Rev. 63, 40–47. doi: 10.1016/j.ctrv.2017.11.007
Tomita, Y., Sueta, D., Kakiuchi, Y., Saeki, S., Saruwatari, K., Sakata, S., et al. (2017). Acute coronary syndrome as a possible immune-related adverse event in a lung cancer patient achieving a complete response to anti-PD-1 immune checkpoint antibody. Ann. Oncol. 28, 2893–2895. doi: 10.1093/annonc/mdx326
Tumeh, P. C., Harview, C. L., Yearley, J. H., Shintaku, I. P., Taylor, E. J., Robert, L., et al. (2014). PD-1 blockade induces responses by inhibiting adaptive immune resistance. Nature 515, 568–571. doi: 10.1038/nature13954
Varricchi, G., Galdiero, M. R., Marone, G., Criscuolo, G., Triassi, M., Bonaduce, D., et al. (2017). Cardiotoxicity of immune checkpoint inhibitors. ESMO Open 2, e000247. doi: 10.1136/esmoopen-2017-000247
Voskens, C. J., Goldinger, S. M., Loquai, C., Robert, C., Kaehler, K. C., Berking, C., et al. (2013). The price of tumor control: an analysis of rare side effects of anti-CTLA-4 therapy in metastatic melanoma from the ipilimumab network. PloS One 8, e53745. doi: 10.1371/journal.pone.0053745
Walker, L. S., Sansom, D. M. (2011). The emerging role of CTLA4 as a cell-extrinsic regulator of T cell responses. Nat. Rev. Immunol. 11, 852–863. doi: 10.1038/nri3108
Wang, D. Y., Okoye, G. D., Neilan, T. G., Johnson, D. B., Moslehi, J. J. (2017). Cardiovascular toxicities associated with cancer immunotherapies. Curr. Cardiol. Rep. 19, 21. doi: 10.1007/s11886-017-0835-0
Wang, D. Y., Salem, J. E., Cohen, J. V., Chandra, S., Menzer, C., Ye, F., et al. (2018). Fatal toxic effects associated with immune checkpoint inhibitors: a systematic review and meta-analysis. JAMA Oncol. 4, 1721–1728. doi: 10.1001/jamaoncol.2018.3923
Wang, J., Okazaki, I. M., Yoshida, T., Chikuma, S., Kato, Y., Nakaki, F., et al. (2010). PD-1 deficiency results in the development of fatal myocarditis in MRL mice. Int. Immunol. 22, 443–452. doi: 10.1093/intimm/dxq026
Weber, J. S., Kahler, K. C., Hauschild, A. (2012). Management of immune-related adverse events and kinetics of response with ipilimumab. J. Clin. Oncol. 30, 2691–2697. doi: 10.1200/jco.2012.41.6750
Weinstock, C., Khozin, S., Suzman, D., Zhang, L., Tang, S., Wahby, S., et al. (2017). U.S. Food and Drug Administration Approval Summary: atezolizumab for metastatic non-small cell lung cancer. Clin. Cancer Res. 23, 4534–4539. doi: 10.1158/1078-0432.Ccr-17-0540
Yamaguchi, S., Morimoto, R., Okumura, T., Yamashita, Y., Haga, T., Kuwayama, T., et al. (2018). Late-onset fulminant myocarditis with immune checkpoint inhibitor nivolumab. Can. J. Cardiol. 34, 812 e811–812 e813. doi: 10.1016/j.cjca.2018.03.007
Yancy, C. W., Jessup, M., Bozkurt, B., Butler, J., Casey, D. E., Colvin, M. M., et al. (2017). ACC/AHA/HFSA Focused Update of the 2013 ACCF/AHA Guideline for the Management of Heart Failure: A Report of the American College of Cardiology/American Heart Association Task Force on Clinical Practice Guidelines and the Heart Failure Society of America. J. Card. Fail. 23, 628–651. doi: 10.1016/j.cardfail.2017.04.014
Yang, S., Asnani, A. (2018). Cardiotoxicities associated with immune checkpoint inhibitors. Curr. Probl. Cancer 42, 422–432. doi: 10.1016/j.currproblcancer.2018.07.002
Keywords: immune checkpoint inhibitors, cardiotoxicity, myocarditis, pericarditis, cytotoxic T lymphocyte-associated antigen-4, programmed cell death protein 1, programmed cell death-ligand 1
Citation: Zhou Y-W, Zhu Y-J, Wang M-N, Xie Y, Chen C-Y, Zhang T, Xia F, Ding Z-Y and Liu J-Y (2019) Immune Checkpoint Inhibitor-Associated Cardiotoxicity: Current Understanding on Its Mechanism, Diagnosis and Management. Front. Pharmacol. 10:1350. doi: 10.3389/fphar.2019.01350
Received: 11 August 2019; Accepted: 24 October 2019;
Published: 29 November 2019.
Edited by:
Shuang Zhou, University of Houston, United StatesReviewed by:
Zhejian Ji, Harvard Medical School, United StatesFeng Tian, Harvard Medical School, United States
Copyright © 2019 Zhou, Zhu, Wang, Xie, Chen, Zhang, Xia, Ding and Liu. This is an open-access article distributed under the terms of the Creative Commons Attribution License (CC BY). The use, distribution or reproduction in other forums is permitted, provided the original author(s) and the copyright owner(s) are credited and that the original publication in this journal is cited, in accordance with accepted academic practice. No use, distribution or reproduction is permitted which does not comply with these terms.
*Correspondence: Zhen-Yu Ding, ZGluZ3poZW55dUBzY3UuZWR1LmNu; Ji-Yan Liu, bGl1aml5YW4xOTcyQDE2My5jb20=