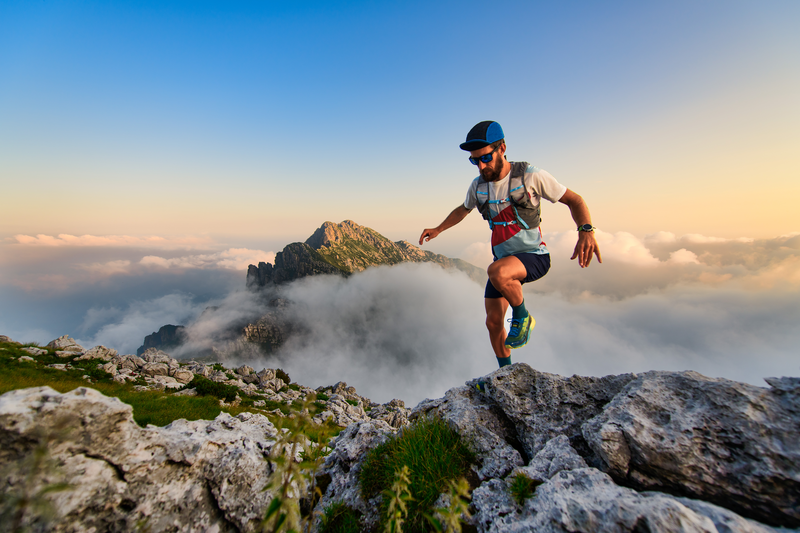
94% of researchers rate our articles as excellent or good
Learn more about the work of our research integrity team to safeguard the quality of each article we publish.
Find out more
MINI REVIEW article
Front. Pediatr. , 07 December 2023
Sec. Pediatric Infectious Diseases
Volume 11 - 2023 | https://doi.org/10.3389/fped.2023.1270564
This article is part of the Research Topic Clinical Challenges in Pediatric Transplant Infectious Diseases View all 11 articles
Introduction: There is increasing recognition of infections due to multidrug-resistant Gram negative (MDRGN) bacterial infections among children undergoing solid organ and hematopoietic cell transplantation, which may be associated with morbidity and mortality.
Methods: We present two vignettes that highlight the clinical challenges of evaluation, management, and prevention of MDRGN bacterial infections in children prior to and after transplantation. The goal of this discussion is to provide a framework to help develop an approach to evaluation and management of these infections.
Results: Source control remains the utmost priority in management of MDR infections and is paired with antibiotic selection guided by in vitro susceptibilities, adverse effect profiles, and clinical response. Identification and confirmation of resistance can be challenging and often requires additional testing for recognition of complex mechanisms. Current antimicrobial approaches to MDRGN infections include use of novel agents, prolonged infusion, and/or combination therapy. We also discuss preventative efforts including infection control, antimicrobial stewardship, targeted pre-emptive or prophylactic treatment, and decolonization.
Discussion: The impact of MDRGN infections on patient and graft survival highlights the need to optimize treatment and prevention strategies.
Bacterial infections are often life-threatening in pediatric patients undergoing solid organ (SOT) or hematopoietic cell transplantation (HCT). Rapid identification and effective management of infections is essential to ensure patients safely undergo transplantation and recover from infections during vulnerable periods of associated immunosuppression. Multidrug-resistant (MDR) bacterial infections are an emerging issue in children and associated with higher mortality rates (1–4). Infections with carbapenem-resistant Enterobacterales (CRE) are of particular concern and especially difficult to treat. Management of MDR infections in children poses many challenges, including the need for extended susceptibility testing, which may lead to a delay in identifying effective therapeutic options, the use of novel antimicrobial agents, and the potential need for combination therapy. Furthermore, many antimicrobials with activity against MDR infections have not been well-studied in children, resulting in limited pharmacokinetics data and uncertain dosing strategies. In addition, the treatment of MDR infections among pediatric SOT and HSCT candidates is additionally complicated by immunosuppression, drug interactions, and challenges in achieving source control.
In this review, we present two clinical vignettes describing MDR Gram-negative (MDRGN) bacterial infections in children undergoing transplantation. We highlight unique considerations regarding evaluation and management of these infections, focusing on the epidemiology of infections most commonly encountered in pediatric transplant recipients, including extended-spectrum β-lactamase (ESBL)-producing Enterobacterales and carbapenem-resistant organisms including Enterobacterales and Pseudomonas aeruginosa, as well as antimicrobial strategies and interventions to optimize outcomes. The goal of this discussion is to present a framework that can enables infectious diseases (ID) trainees and specialists caring for the complex immunocompromised pediatric patient to develop a practical approach to diagnosis and treatment of MDRGN infections.
A 14-year-old male with a history of congenital hepatic fibrosis with severe portal hypertension and recurrent cholangitis presented with a 1 day history of fever, shoulder pain and acute right upper quadrant (RUQ) tenderness. His history was remarkable for recurrent episodes of cholangitis over the prior 2 years, leading to frequent courses of parenteral antibiotics as well as interval biliary stent placement and subsequent cholecystectomy. He was on longstanding fluoroquinolone prophylaxis, and at the time of admission had a marked leukocytosis of 23,000 cells/ml, elevated C-reactive protein of 19.3 mg/dl and an elevated total bilirubin of 3.1 mg/dl. Alanine transferase and aspartame transferase were within normal limits. A computerized tomography (CT) scan of the abdomen/pelvis demonstrated hepatomegaly with multiple cystic regions within the liver, consistent with dilated biliary ducts/bile lakes and the presence of pneumobilia. Piperacillin-tazobactam was started empirically, but antibiotics were escalated to meropenem due to clinical worsening. Blood culture returned positive for extended spectrum β-lactamase (ESBL) producing Escherichia coli, resistant to ceftriaxone, cefepime, ciprofloxacin, gentamicin, and trimethoprim-sulfamethoxazole (TMP-SMX)—but susceptible to meropenem (minimal inhibitory concentration, MIC, 0.25 μg/ml) and ertapenem (MIC 0.25 μg/ml). He received 10 days of meropenem for bacteremia and cholangitis, with rapid interval clinical resolution and was discharged home.
He required re-admission 1 week after completion of antibiotics due to fever, RUQ pain and shoulder pain, consistent with cholangitis. A repeat CT scan demonstrated persistence of biliary lakes, and cultures from a biliary drain placed into the largest collection demonstrated E. coli, now with resistance to meropenem (MIC 4) as well fluoroquinolones. He received a prolonged 4-week course of ceftazidime-avibactam with subsequent clinical resolution, but symptoms of biliary drainage, fever, and right sided abdominal pain recurred within 1 week of completion of antibiotics. He was listed for liver transplant as treatment for recurrent cholangitis.
A 13-month-old female with a history of relapsed acute myeloblastic leukemia (AML) developed new onset left labial erythema, ulceration and fever, 6 days following allogeneic HCT. She had no prior history of bacterial infection and was on voriconazole, levofloxacin, and pentamidine prophylaxis. On workup, she was profoundly neutropenic (absolute neutrophil count of 20 cells/µl) and was started on vancomycin and piperacillin-tazobactam for febrile neutropenia with concern for labial cellulitis. Fevers continued, and she developed progressive erythema extending to the contralateral labia, multiple ulcerations to the perirectal region, and worsening hypotension on day 9 of illness. Piperacillin-tazobactam was discontinued and meropenem started. A MRI of the abdomen/pelvis demonstrated diffuse severe cellulitis of the perineum with bilateral myositis consistent with necrotizing fasciitis. She was taken to the operating room for fasciotomy, and tissue cultures demonstrated carbapenem-resistant Pseudomonas aeruginosa, also resistant to piperacillin/tazobactam, meropenem, imipenem, ceftazidime, aztreonam, cefepime, and fluoroquinolones but susceptible to amikacin and tobramycin.
Extended antimicrobial susceptibility testing (AST) results later demonstrated a high MIC to ceftazidime-avibactam (MIC 16/4, No Interpretation), but susceptibility to colistin (MIC 1) and ceftolozane-tazobactam (MIC 4). She subsequently showed evidence of clinical improvement with neutrophil recovery on day 11 but ultimately required two additional debridement procedures on days 12 and 14 for control of her infection.
Antibiotic resistance can develop via multiple different mechanisms, including inactivation or alteration of the antibiotic molecule, bacterial target site modifications, reduced antibiotic penetration/accumulation, and/or the formation of biofilm (5). For the purpose of this review, the previously published international consensus definition of MDR is utilized, which is defined as nonsusceptibility to at least one agent in three or more antibiotic classes (6). A common resistance mechanism utilized by Gram negative pathogens includes enzymes that inactivate broad-spectrum β-lactam antimicrobial drugs, such as penicillins, cephalosporins, and carbapenems, via hydrolytic cleavage of the β-lactam ring. A wide variety of these unique β-lactamase enzymes exists, and several clinically important β-lactamases have emerged in both the general and transplant populations, including AmpC β-lactamases, extended spectrum β-lactamases (ESBL), and carbapenemases, which are described in further detail in Table 1.
Table 1. Overview of important multidrug resistance mechanisms in gram-negative pathogens (7).
Although this review focuses on β-lactamases, antibiotic resistance in Gram negative organisms is complex. Other mechanisms can also be present individually or simultaneously with β-lactamases to create various resistance patterns. This can be illustrated with an important distinction in the heterogenous mechanisms of CRE. Carbapenem resistance may develop via two mechanisms: (1) production of a carbapenemase encoded by genes usually found on transmissible mobile elements (carbapenemase-producing CRE, CP-CRE) or (2) production of ESBL or AmpC β-lactamases combined with impaired membrane permeability from certain porin mutations or efflux pumps (termed non-CP-CRE). The Centers for Disease Control (CDC) definition of CRE represents both mechanisms in the CRE terminology, including members of the Enterobacterales order resistant to at least one carbapenem antibiotic or those producing a carbapenemase enzyme (9).
While the incidence of MDRGN infections reported among adult SOT and HCT recipients varies by geography and institution, overall rates of infection have steadily increased in recent years, with estimates showing an almost ten-fold rise in incidence between the early 2000's and 2015 (6). ESBL-producing Enterobacterales infections are the most common cause of MDRGN infections among SOT recipients and account for up to 75% of these infections (10–12). The incidence of CRE infection has ranged from 3% to 10% in areas of endemicity and has been best described in abdominal SOT recipients. Adult data also suggests increased mortality among transplant recipients with MDRGN infections, with 30-day mortality ranging from 14% to 26% with ESBL infection and 18%–41% in CRE infection (10–14).
Yet despite the increasing attention on MDR infections among adult transplant recipients, knowledge of the epidemiology of these infections in children remains limited. MDR infections have been increasingly identified in children and adolescents, although data, particularly in immunocompromised children, requires further study (1–4). While the prevalence of CRE infections in US children remains low overall, with the Surveillance Network (TSN) database-USA estimating 0.08% CRE vs. 0.47% ESBL in available isolates from children, rates continue to increase (4). Such infections have been associated with poor outcomes such as ICU admission and death in children (1, 2).
Research directly examining MDRGN infections in pediatric SOT or HCT recipients is scarce, but available data suggests the epidemiology, risk factors, and outcomes in the pediatric population may reflect trends observed among adults, with increased rates of MDRGN infection observed in the pediatric immunocompromised and transplant population. Among pediatric liver transplant recipients, the estimated incidence of MDR in a single-center retrospective study of 118 children in Thailand between 2010 and 2018 found 62.4% (58/93) of culture-proven bacterial infections post-transplant were due to MDRGN isolates, with a predominance of Klebsiella pneumoniae and Escherichia coli (15). These were largely intra-abdominal infections, accounting for 47.9% of infections. Another recent single-center retrospective study of pediatric liver transplant recipients from the United Kingdom found a lower rate, noting 27% of patients had colonization with MDRGN with an infection rate of 16.6% (16). MDR organisms have also been identified as a significant cause of severe sepsis for the pediatric liver transplant population as well, accounting for 47.6% (20/42) of organisms causing bacterial sepsis in a tertiary pediatric intensive care unit in the United States. It should be noted though that MDR organisms in this study were defined by resistance to one or more classes of antibiotics and also included cases of vancomycin resistant Enterococcus (accounting for 9 of the 20 episodes of MDR organisms) (17). Similar to adult studies, ESBL infections accounted for the majority of MDR Gram-negative infections in these pediatric liver transplant recipients, and notably, CRE infection rates were found to range from 3% to 17% (15–17).
Knowledge of the incidence and outcomes of MDRGN infections in children receiving treatment for malignancy or after HCT is also limited. In a multi-national, multicenter retrospective study of 1,291 bloodstream infections in pediatric patients with allogeneic HCT or those treated with chemotherapy, more than 25% of Gram-negative infections were resistant to ceftazidime, cefepime, piperacillin-tazobactam, and ciprofloxacin. CRE represented 9% of infections. This study found that bloodstream infection with MDRGN infection was significantly associated with ICU admission and death (18).
Risk factors for MDR infections in immunocompromised adults have been well described and include prior exposure to antimicrobial drugs, critical illness, prolonged and frequent exposure to health care, residence in long term facilities, pre-transplant colonization, and longer length of stay prior to infection (11). In children, previous exposure to antibiotics, particularly third generation cephalosporins and carbapenems, has been significantly associated with MDRGN bloodstream infection and post-transplant infection in pediatric patients with hematologic malignancy, HCT, and liver transplantation (15, 18). Other noted factors in Phichaphop, et al. included operation duration and length of ICU stay (15).
The molecular and geographic epidemiology of β-lactamase producing organisms, particularly carbapenemases, is an important consideration when approaching diagnostic and empiric treatment strategies. In the United States, the K. pneumoniae carbapenemase (KPC) enzyme is the predominant carbapenemase found in CP-CRE isolates infecting adults and children, while other carbapenemases are infrequently identified (8, 19–21). The class of metallo-β-lactamase (MBL) carbapenemases are unique as they are not inhibited by avibactam, vaborbactam, or relebactam. Given this has a significant impact on the available treatment options, clinicians must utilize caution and have clinical suspicion if carbapenem resistance is detected in isolates from children with epidemiologic links to MBL-endemic regions. Additionally, sporadic domestic acquisition of other MBLs remain possible (8, 22, 23).
Infection due to a MDRGN organism should be suspected in an immunocompromised or transplant recipient patient when there is a lack of clinical response to antimicrobial therapy, risk factors present for possible MDR organism exposure, and/or previous isolation or colonization of such pathogen. Early involvement of pediatric transplant ID specialists in these complex cases is important to assist with additional clinical evaluation, which should be guided by the patient's presentation, signs, and symptoms. Aggressive evaluation should be pursued to identify the source of infection, and relevant imaging studies may be necessary. Sampling with bacterial cultures from blood as well as any other suspected sites of infection should be obtained as clinically feasible to guide therapy. Source control, including drainage of infected fluid collections or removal of infected devices or hardware, are important mainstays of therapy, especially given the limited antimicrobial options for MDR Gram-negative infections.
If a clinical isolate is available, the first step in evaluation of antimicrobial resistance is examining the AST panel results to assess for the possible mechanism(s) of resistance via implementation of interpretive criteria from the Clinical & Laboratory Standards Institute (CLSI) or European Committee on Antimicrobial Susceptibility Testing (EUCAST) (24, 25). Often additional testing may be necessary (such as requesting additional local or send-out susceptibilities to novel agents), and isolates should be evaluated in laboratories experienced with recognition of complex resistance mechanisms.
Ceftriaxone-resistance is used as a common proxy to identify ESBL-producing bacteria. Routine ESBL testing is not performed by most clinical microbiology laboratories as current CLSI and EUCAST breakpoints should detect most ESBL isolates, but testing for ESBL confirmation and characterization may be warranted for infection control or epidemiologic purposes.
For identification of CRE, the current CLSI or EUCAST breakpoints are used to identify nonsusceptibility to imipenem, meropenem, doripenem, or ertapenem. Enterobacterales that are resistant to at least one of the carbapenems listed are considered CRE based on the CDC definition, but this definition could include both CP-CRE and non-CP-CRE as noted previously (9). Both CLSI and EUCAST define Enterobacterales susceptibility to ertapenem and doripenem the same (MICs of ≤0.5 μg/ml and ≤1 μg/ml, respectively), but there is some variation in the breakpoints for Enterobacterales and meropenem/imipenem. CLSI defines susceptibility to meropenem and imipenem with MICs of ≤1 μg/ml, while meropenem and imipenem MICs ≤2 μg/ml are considered susceptible by EUCAST (24, 25).
Although the presence of carbapenemase does not influence the categorization of susceptibility, carbapenemase detection and characterization may be recommended for public health and infection control purposes. Additionally, knowledge of whether a CRE isolate is carbapenemase-producing and the specific carbapenemase is also important to guide clinical decisions (which will be discussed further in the following treatment section). Lab tests are available to identify the presence or absence of a carbapenemase, including the Carba NP assay or the modified carbapenem inactivation method (mCIM). Molecular tests can be used to identify specific carbapenemase genes. It is important to note that “negative” results on molecular carbapenemase testing should not be interpreted as confirmation of the absence of carbapenemase (as other carbapenemase genes may be present that are not included in the assay used) or carbapenem susceptibility (as the isolate may be a non-CP CRE). The CLSI does recommend use of the Carba NP assay, mCIM, and/or a molecular assay when Enterobacterales isolates have an imipenem or meropenem MIC of 2–4 μg/ml or ertapenem MIC of 2 μg/ml.
The recommended diagnostic approach for identification of ESBL and carbapenemases are the focus of this review and are summarized in Table 1. A few other notable MDRGN organisms include Pseudomonas aeruginosa with difficult-to-treat resistance (DTR-P. aeruginosa; defined as non-susceptibility to piperacillin-tazobactam, ceftazidime, cefepime, aztreonam, meropenem, imipenem-cilastatin, ciprofloxacin, and levofloxacin; illustrated in Case 2), carbapenem-resistant Acinetobacter baumannii (CRAB), and Stenotrophomonas maltophilia. CLSI and EUCAST interpretative criteria for antimicrobial susceptibilities are also utilized for these organisms. It is useful to note that carbapenemase production is a rare cause of carbapenem resistance in P. aeruginosa isolates in the US and generally carbapenemase testing of clinical isolates of P. aeruginosa is not as critical as for CRE clinical isolates in US hospitals. That said, carbapenemases have been identified in up to one-fifth of carbapenem-resistant P. aeruginosa in other regions of the world and current IDSA guidance encourages performing additional susceptibility testing for novel β-lactam agents (7).
The treatment of MDRGN infections in the SOT and HCT recipient requires a high index of clinical suspicion with an appreciation of the unique anatomic, immunologic, and environmental risks predisposing individuals to infection. Appropriate empiric antibiotic therapy, coupled with adequate source control and durations tailored to the nature of infection remain the cornerstone of therapy. Among transplant recipients, such consideration should take into account impaired or absent neutrophil function, the risk of anastomotic leakage, and the frequent presence of central venous catheters, foley, peritoneal drains, and hemodialysis catheters (26).
Available antimicrobial treatment regimens for MDRGN infections have rapidly evolved in the past decade, particularly with the addition of novel β-lactam-β-lactamase inhibitor (BLBLI) agents. The optimal treatment of these infections remains complex though given resistance mechanisms and a limited armamentarium, particularly with CRE organisms. Antimicrobial management in children is further complicated by limited pediatric-specific pharmacokinetic and pharmacodynamic data and few clinical trials evaluating novel agents for use in the pediatric population.
Selection of an appropriate empiric antibiotic regimen is essential to reduce morbidity and mortality risk following transplantation, with inappropriate empiric antimicrobial regimens associated with up to a three-fold increased mortality risk in adult HCT recipients (27). Factors that should be considered in choosing an empiric antibiotic regimen include local resistance patterns (particularly ESBL and regional carbapenemases prevalence), prior patient history of infection or colonization, recent antimicrobial exposure, and severity of illness. Empiric antimicrobial regimens following transplantation should demonstrate robust activity against Pseudomonas aeruginosa. Carbapenem agents, such as meropenem or imipenem, should be considered in settings of high local ESBL prevalence, known prior history of ESBL infection or colonization, and/or critical illness. The empiric use of novel BLBLIs (such as ceftazidime-avibactam, ceftolozane-tazobactam, imipenem-cilastatin-relebactam) or other novel agents is generally avoided if possible but should be considered in the setting of known or suspected risk factors for CRE.
For bloodstream and other serious non-urinary tract infections caused by ESBL-E, treatment with carbapenem therapy should be utilized, with meropenem and imipenem preferred over ertapenem in cases of critical illness. Newer BLBLIs and cefiderocol are active against ESBL-producing organisms but are not utilized as first line if other options are available to preferentially reserve these agents for carbapenem resistant organisms.
The results of carbapenem susceptibility testing should guide treatment selection, although antibiotic choice should generally not be stratified based on the presence of carbapenemase production (CP-CRE vs. non-CP-CRE). To start, carbapenem susceptibility testing can initially provide guidance on whether an extended infusion carbapenem can be used vs. a newer BLBLI (22). For isolates that exhibit susceptibility to meropenem and imipenem but are not susceptible to ertapenem, the use of extended-infusion meropenem (or imipenem-cilastatin) is suggested, assuming no carbapenemase has been identified. Extended-infusion β-lactam (typically over 3 h) optimizes killing time and increases the likelihood of achieving target drug levels. This approach is often used in settings of high inoculum or refractory infection as well.
If the CRE isolate has a meropenem MIC ≥4 µg/ml (such as in Case 1), the use of a novel BLBLI (ceftazidime-avibactam, meropenem-vaborbactam, or imipenem-cilastatin-relebactam) is preferred. If specific carbapenemase testing is available and identifies a specific carbapenemase, this can further inform the selection of the optimal BLBLI given differential activity based on carbapenemase type. For example, while these three agents all have a high likelihood of activity against KPC-producing organisms as well as non-CP-CRE, meropenem-vaborbactam and imipenem-cilastatin-relebactam do not retain activity against OXA-48-like carbapenemases. MBL-producing organisms present further challenges in treatment as neither ceftazidime-avibactam, meropenem-vaborbactam, nor imipenem-cilastatin-relebactam have activity.
For patients with suspected or confirmed infection due to MBL-producing organisms or with previous clinical or surveillance cultures for MBL, preferred treatment options include the combination of ceftazidime-avibactam plus aztreonam (used in conjunction to create “aztreonam-avibactam”) or cefiderocol monotherapy. MBLs, such as NDM, hydrolyze penicillins, cephalosporins, and carbapenems—but not aztreonam. Aztreonam is active against MBLs but may not be effective as monotherapy due to co-production of ESBLs, AmpC β-lactamases, or other carbapenemases such as KPC or OXA-48-like. Avibactam does not have an effect on MBL itself but can “protect” aztreonam from hydrolysis due to ability to inhibit these β-lactamases co-produced by most MBL isolates. Of note, avibactam restores activity of aztreonam in MBL-producing Enterobacterales, but not MBL-producing P. aeruginosa due to additional non-β-lactam mechanisms of resistance in P. aeruginosa (28). Specific synergy antimicrobial susceptibility testing of this combination therapy is available in very limited clinical labs, but no specific lab method is endorsed by CLSI. There is clinical experience with combination of ceftazidime-avibactam plus aztreonam but it is not FDA-approved and requires careful attention to the logistics of co-administration (29, 30). Another MBL-producing CRE option is cefiderocol, which exhibits activity against any of the major carbapenemase enzymes as well as non-CP-CRE. Generally cefiderocol is preserved for cases where other β-lactams cannot be used, such as MBL.
If carbapenemase testing is not available or is negative, novel BLBLIs remain the preferred treatment option, unless there is a possible epidemiologic link/exposure to MBL-producing organism or prior clinical or surveillance cultures with an MBL-producing organism.
Other agents to note for management of CRE include aminoglycosides, polymyxins (such as polymyxin B or colistin), plazomicin, tigecycline, eravacycline, and omadacycline. Aminoglycosides and polymyxins were historically the backbone for treatment of CRE, but the newer BLBLIs are favored now due to clinical success, less complex dosing regimens, and less risk of nephrotoxicity. Aminoglycoside monotherapy is generally reserved for urinary tract infections. Tigecycline and eravacycline are alternative options for CRE infections not involving the bloodstream or urinary tract and their activity is independent of type of carbapenemase.
MDR-P. aeruginosa isolates demonstrating carbapenem resistance but susceptibility to traditional anti-pseudomonal agents can usually be treated effectively with traditional beta-lactams via extended infusion. In cases of critical illness or poor source control, novel agents such as ceftolozane-tazobactam, ceftazidime-avibactam, and imipenem-cilastatin-relebactam are viable alternatives, although this is not the otherwise preferred option to preserve effectiveness of these agents for future use in increasingly antibiotic-resistant infections.
DTR-P. aeruginosa should be treated with ceftolozane-tazobactam, ceftazidime-avibactam, or imipenem-cilastatin-relebactam. Ceftolozane and ceftazidime have a similar structure, but a benefit of ceftolozane is the decreased impact by hydrolysis and porin loss compared to ceftazidime. Ceftolozane has independent anti-pseudomonal activity that does not rely on tazobactam and the combination of ceftolozane-tazobactam is relatively stable to all pseudomonal β-lactam resistance mechanisms. The patient in Case 2 was transitioned to ceftolozane-tazobactam for definitive therapy.
Cefiderocol is an alternative treatment option that is reserved for when there is inactivity, intolerance, or unavailability of the newer BLBLIs. In the case of infection with a DTR-P. aeruginosa isolate that is MBL-producing though, cefiderocol would be the drug of choice.
The possible benefit of combination therapy with multiple agents has been explored, but ongoing use once susceptibility testing is available is controversial. Empiric combination antimicrobial therapy, such as use of β-lactams or BLBLIs with aminoglycosides or possibly polymyxins, is often deployed initially to increase the likelihood that at least one agent administered is active against infection in patients at high risk for MDRGN infections, especially CRE and MDR/DTR-P. aeruginosa. However, once a β-lactam agent demonstrates activity, there is no clear established benefit of continued combination therapy over monotherapy in CRE or DTR-P. aeruginosa infections. There is no randomized trial data comparing monotherapy vs. combination therapy, but the current IDSA panel guidance suggests against the use of combination therapy in these cases of CRE or DTR-P. aeruginosa infection given the lack of clear benefit in available studies and risk of antibiotic associated adverse events with multiple antibiotics (7).
In contrast, combination therapy is suggested for infections due to CRAB and MDR Stenotrophomonas maltophilia. While the incidence of CRAB infection remains low among pediatric transplant recipients, the general approach for treatment would include use of high-dose ampicillin-sulbactam (regardless of susceptibility results) in combination with at least one other active agent, given the limited clinical data supporting any single antibiotic. The production of OXA carbapenemases leads to resistance to β-lactams in most CRAB isolates, but these isolates may also produce MBLs and other serine carbapenemases. Sulbactam-durlobactam is a recently FDA-approved agent with activity against CRAB. Durlobactam inhibits class A, C, and D β-lactamase enzymes commonly produced by CRAB, restoring activity of sulbactam. Specific pediatric data on this agent is not available at this time. S. maltophilia infections pose similar challenges to CRAB infections, and treatment selection is hindered by resistance often caused by MBL and other β-lactamases, intrinsic resistance, and accumulation of efflux pumps. These infections are usually addressed with trimethoprim-sulfamethoxazole in combination with another agent. Another possibility is use of the ceftazidime-avibactam and aztreonam combination in cases of critical illness, intolerance, or inactivity of other agents.
A summary of the available antimicrobial agents and general treatment recommendations by organism are also described in Tables 2, 3. Ultimately the final antibiotic regimen will require consideration of susceptibility results, ability to obtain adequate drug levels at site of infection, side effect profile, route of administration, and cost/formulary considerations. Children in need of transplantation may have chronic and prolonged bacterial infections in addition to their underlying organ dysfunction and remain at risk for recurrent and relapsing infections following transplantation (9). As such, in addition to effective antimicrobial regimens, adequate source control, awareness of post-transplant co-morbidities, and consideration of overall host immune defenses are critical in efforts to control bacterial infection following SOT/HCT. Standard recommended antimicrobial durations in SOT and HCT recipients are often confounded by impaired immunologic clearance, high risk of recurrence, and persisting sources of infection, and as such, durations such should be guided by interval clinical improvement, restoration of immune function and demonstrated source control.
The patient in Case 1 required several prolonged courses of antibiotics which would improve his symptoms temporarily, so he was ultimately listed for transplant as treatment for recurrent cholangitis. He underwent successful deceased donor liver transplant 1 month later with subsequent resolution of his infections. The patient in Case 2 underwent successful skin graft procedure on day 21 of illness, and ceftolozane-tazobactam was continued for an additional week post-debridement with subsequent clinical resolution and wound healing.
Prevention of MDR gram-negative infections in transplant candidates and recipients requires consideration of both intrinsic, patient-specific factors, as well as broader hospital-level infection control measures.
Patient-specific factors include prolonged hospitalization in the pre- and peri-transplant setting due to poor functional status or complications related to the underlying diagnosis, indwelling medical devices such as central venous catheters and ventricular-assist devices, and repeated antibiotic treatment courses (11). Such factors may lead to selection for resistant organisms and persistent colonization with these organisms, which may drive increasingly broad antibiotic selection in the setting of fevers and other signs of infection. Antimicrobial stewardship interventions to optimize antibiotic use in both the pre- and post-transplant setting are essential, and the lack of antibiotic de-escalation and use of expansive broad-spectrum empiric antibiotics for prolonged durations represent opportunities for improvement in transplant patients (11). In addition, efforts to remove indwelling medical devices as soon as clinically feasible can reduce risk of infection. Improving the functional status of patients, addressing nutrition and growth needs, and timely progression to transplant to alleviate complications associated with a failing organ or attain immunologic recovery are also critical for preventing infections, but many competing factors can influence the ability to achieve these goals.
Hospital-level infection control and prevention practices can ensure that MDR gram-negative infections are not transmitted within hospital settings. The Centers for Disease Control (CDC) developed a toolkit for healthcare facilities on the prevention of transmission of MDR organisms, specifically for CRE (summarized in Table 4), and the Cystic Fibrosis Foundation Infection Prevention and Control Guidelines should be implemented where transplant recipients with cystic fibrosis access care (32, 33). The benefit of routine surveillance of all patients with MDR organisms, including CRE, in healthcare settings or even on transplant floors is unclear. Only a small proportion of patients colonized with MDR organisms go on to develop clinically significant infections, and optimal infection control measures for transplant candidates or recipients known to be colonized with MDR organisms is uncertain. There is limited evidence of support surveillance outside of the outbreak setting, and intestinal decolonization for patients with known ESBL and CRE carriage is not recommended (11).
Table 4. General infection prevention and control strategies to reduce transmission and acquisition of MDRGN pathogens, such as CRE, in healthcare facilities (32, 33).
Targeted screening of known contacts of patients with MDR infections and active surveillance of patients who meet specific risk criteria for MDR infections may be a more useful approach, particularly to identify the need for contact precautions and patient cohorting (33). For transplant candidates and recipients with known colonization with MDR organisms, pre-emptive treatment or ongoing prophylaxis, in the absence of evidence of clinical infection, is not recommended. Such use of prophylaxis has not demonstrated benefit for clearing colonization and raises concern for development of additional resistance through selective pressure.
Transplant candidates with known colonization with or history of MDR infections, including CRE, should not be excluded from transplantation based on this feature alone, with rare exceptions. SOT donors with MDR infection or colonization should also not be excluded, but communication between donor and recipient healthcare facilities is essential. If a donor is bacteremic or if there is evidence of infection of the allograft being transplanted, recipients will require appropriate post-transplant antibiotic treatment based on susceptibility testing of the identified organism.
Pediatric transplant ID specialists must remain vigilant to ensure rapid evaluation and management for MDR Gram-negative infections in pediatric transplant recipients. Despite limited data in the pediatric populations, risk factors described in these studies and prior adult experiences point to the potential consequences in vulnerable post-transplant recipients exposed to broad-spectrum antibiotics, frequent hospitalizations, and need for invasive procedures and indwelling devices. The approach to MDRGN infections among children following transplantation ultimately requires the optimization of preventive strategies, in conjunction with a high index of suspicion, effective antimicrobial strategies and source identification and control.
SD: Conceptualization, Writing – original draft, Writing – review and editing. TS: Conceptualization, Writing – original draft, Writing – review and editing. PS: Conceptualization, Writing – original draft, Writing – review and editing.
The author(s) declare that no financial support was received for the research, authorship, and/or publication of this article.
The authors declare that the research was conducted in the absence of any commercial or financial relationships that could be construed as a potential conflict of interest.
All claims expressed in this article are solely those of the authors and do not necessarily represent those of their affiliated organizations, or those of the publisher, the editors and the reviewers. Any product that may be evaluated in this article, or claim that may be made by its manufacturer, is not guaranteed or endorsed by the publisher.
1. Chiotos K, Han JH, Tamma PD. Carbapenem-resistant Enterobacteriaceae infections in children. Curr Infect Dis Rep. (2016) 18(1):2. doi: 10.1007/s11908-015-0510-9
2. Chiotos K, Tamma PD, Flett KB, Karandikar MV, Nemati K, Bilker WB, et al. Increased 30-day mortality associated with carbapenem-resistant Enterobacteriaceae in children. Open Forum Infect Dis. (2018) 5(10):ofy222. doi: 10.1093/ofid/ofy222
3. Montagnani C, Prato M, Scolfaro C, Colombo S, Esposito S, Tagliabue C, et al. Carbapenem-resistant Enterobacteriaceae infections in children: an Italian retrospective multicenter study. Pediatr Infect Dis J. (2016) 35(8):862–8. doi: 10.1097/INF.0000000000001188
4. Logan LK, Renschler JP, Gandra S, Weinstein RA, Laxminarayan R; Centers for Disease Control; Prevention Epicenters Program. Carbapenem-resistant Enterobacteriaceae in children, United States, 1999-2012. Emerg Infect Dis. (2015) 21(11):2014–21. doi: 10.3201/eid2111.150548
5. De Oliveira DMP, Forde BM, Kidd TJ, Harris PNA, Schembri MA, Beatson SA, et al. Antimicrobial resistance in ESKAPE pathogens. Clin Microbiol Rev. (2020) 33(3):e00181–19. doi: 10.1128/CMR.00181-19
6. Magiorakos AP, Srinivasan A, Carey RB, Carmeli Y, Falagas ME, Giske CG, et al. Multidrug-resistant, extensively drug-resistant and pandrug-resistant bacteria: an international expert proposal for interim standard definitions for acquired resistance. Clin Microbiol Infect. (2012) 18(3):268–81. doi: 10.1111/j.1469-0691.2011.03570.x
7. Tamma PD, Aitken SL, Bonomo RA, Mathers AJ, van Duin D, Clancy CJ. Infectious Diseases Society of America antimicrobial-resistant treatment guidance: gram-negative bacterial infections. Infectious Diseases Society of America (2023). Available at: https://www.idsociety.org/practice-guideline/amr-guidance/ (Accessed July 16, 2023).
8. Bush K, Bradford PA. Epidemiology of β-lactamase-producing pathogens. Clin Microbiol Rev. (2020) 33(2):e00047–19. doi: 10.1128/CMR.00047-19
9. Centers for Disease Control and Prevention. CRE technical information. Available at: https://www.cdc.gov/hai/organisms/cre/technical-info.html
10. Oriol I, Sabé N, Simonetti AF, Lladó L, Manonelles A, González J, et al. Changing trends in the aetiology, treatment and outcomes of bloodstream infection occurring in the first year after solid organ transplantation: a single-centre prospective cohort study. Transpl Int. (2017) 30(9):903–13. doi: 10.1111/tri.12984
11. Pouch SM, Patel G, AST Infectious Diseases Community of Practice. Multidrug-resistant gram-negative bacterial infections in solid organ transplant recipients-guidelines from the American society of transplantation infectious diseases community of practice. Clin Transplant. (2019) 33(9):e13594. doi: 10.1111/ctr.13594
12. Anesi JA, Lautenbach E, Tamma PD, Thom KA, Blumberg EA, Alby K, et al. Risk factors for extended-spectrum β-lactamase-producing enterobacterales bloodstream infection among solid-organ transplant recipients. Clin Infect Dis. (2021) 72(6):953–60. doi: 10.1093/cid/ciaa190
13. Bartoletti M, Giannella M, Tedeschi S, Viale P. Multidrug-resistant bacterial infections in solid organ transplant candidates and recipients. Infect Dis Clin North Am. (2018) 32(3):551–80. doi: 10.1016/j.idc.2018.04.004
14. Anesi JA, Lautenbach E, Thom KA, Tamma PD, Blumberg EA, Alby K, et al. Clinical outcomes and risk factors for carbapenem-resistant enterobacterales bloodstream infection in solid organ transplant recipients. Transplantation. (2023) 107(1):254–63. doi: 10.1097/TP.0000000000004265
15. Phichaphop C, Apiwattanakul N, Techasaensiri C, Lertudomphonwanit C, Treepongkaruna S, Thirapattaraphan C, et al. High prevalence of multidrug-resistant gram-negative bacterial infection following pediatric liver transplantation. Medicine. (2020) 99(45):e23169. doi: 10.1097/MD.0000000000023169
16. Verma A, Vimalesvaran S, Dhawan A. Epidemiology, risk factors and outcome due to multidrug resistant organisms in paediatric liver transplant patients in the era of antimicrobial stewardship and screening. Antibiot Basel Switz. (2022) 11(3):387. doi: 10.3390/antibiotics11030387
17. Alcamo AM, Alessi LJ, Vehovic SN, Bansal N, Bond GJ, Carcillo JA, et al. Severe sepsis in pediatric liver transplant patients: the emergence of multidrug-resistant organisms. Pediatr Crit Care Med. (2019) 20(7):e326–32. doi: 10.1097/PCC.0000000000001983
18. Castagnola E, Bagnasco F, Mesini A, Agyeman PKA, Ammann RA, Carlesse F, et al. Antibiotic resistant bloodstream infections in pediatric patients receiving chemotherapy or hematopoietic stem cell transplant: factors associated with development of resistance, intensive care admission and mortality. Antibiot Basel Switz. (2021) 10(3):266. doi: 10.3390/antibiotics10030266
19. Woodworth KR. Vital signs: containment of novel multidrug-resistant organisms and resistance mechanisms—United States, 2006–2017. Morb Mortal Wkly Rep. (2018) 67(13):396–401. doi: 10.15585/mmwr.mm6713e1
20. Pannaraj PS, Bard JD, Cerini C, Weissman SJ. Pediatric carbapenem-resistant Enterobacteriaceae in Los Angeles, California, a high-prevalence region in the United States. Pediatr Infect Dis J. (2015) 34(1):11–6. doi: 10.1097/INF.0000000000000471
21. Chiotos K, Tamma PD, Flett KB, Naumann M, Karandikar MV, Bilker WB, et al. Multicenter study of the risk factors for colonization or infection with carbapenem-resistant Enterobacteriaceae in children. Antimicrob Agents Chemother. (2017) 61(12):e01440–17. doi: 10.1128/AAC.01440-17
22. Chiotos K, Hayes M, Gerber JS, Tamma PD. Treatment of carbapenem-resistant Enterobacteriaceae infections in children. J Pediatr Infect Dis Soc. (2020) 9(1):56–66. doi: 10.1093/jpids/piz085
23. Tamma PD, Suwantarat N, Rudin SD, Logan LK, Simner PJ, Rojas LJ, et al. First report of a verona integron-encoded metallo-β-lactamase-producing Klebsiella pneumoniae infection in a child in the United States. J Pediatr Infect Dis Soc. (2016) 5(3):e24–7. doi: 10.1093/jpids/piw025
24. Eucast: clinical breakpoints and dosing of antibiotics. Available at: https://www.eucast.org/clinical_breakpoints (Accessed August 27, 2023).
25. Clinical and Laboratory Standards Institute. M100: Performance standards for antimicrobial susceptibility testing. 33rd ed. Wayne, PA: Clinical and Laboratory Standards Institute (2023).
26. Kritikos A, Manuel O. Bloodstream infections after solid-organ transplantation. Virulence. (2016) 7(3):329–40. doi: 10.1080/21505594.2016.1139279
27. Puerta-Alcalde P, Chumbita M, Charry P, Castaño-DĂez S, Cardozo C, Moreno-GarcĂa E, et al. Risk factors for mortality in hematopoietic stem cell transplantation recipients with bloodstream infection: points to be addressed by future guidelines. Transplant Cell Ther. (2021) 27(6):501.e1–e6. doi: 10.1016/j.jtct.2021.03.017
28. Karlowsky JA, Kazmierczak KM, de Jonge BLM, Hackel MA, Sahm DF, Bradford PA. In vitro activity of aztreonam-avibactam against Enterobacteriaceae and Pseudomonas aeruginosa isolated by clinical laboratories in 40 countries from 2012 to 2015. Antimicrob Agents Chemother. (2017) 61(9):e00472–17. doi: 10.1128/AAC.00472-17
29. Hobson CA, Bonacorsi S, Fahd M, Baruchel A, Cointe A, Poey N, et al. Successful treatment of bacteremia due to NDM-1-producing Morganella morganii with aztreonam and ceftazidime-avibactam combination in a pediatric patient with hematologic malignancy. Antimicrob Agents Chemother. (2019) 63(2):e02463–18. doi: 10.1128/AAC.02463-18
30. Falcone M, Daikos GL, Tiseo G, Bassoulis D, Giordano C, Galfo V, et al. Efficacy of ceftazidime-avibactam plus aztreonam in patients with bloodstream infections caused by metallo-β-lactamase-producing Enterobacterales. Clin Infect Dis. (2021) 72(11):1871–8. doi: 10.1093/cid/ciaa586
31. Harris PNA, Tambyah PA, Lye DC, Mo Y, Lee TH, Yilmaz M, et al. Effect of piperacillin-tazobactam vs meropenem on 30-day mortality for patients with E coli or Klebsiella pneumoniae bloodstream infection and ceftriaxone resistance: a randomized clinical trial. J Am Med Assoc. (2018) 320(10):984–94. doi: 10.1001/jama.2018.12163. Erratum in: J Am Med Assoc. (2019) 321(23):2370. PMID: 30208454; PMCID: PMC6143100
32. Saiman L, Siegel JD, LiPuma JJ, Brown RF, Bryson EA, Chambers MJ, et al. Infection prevention and control guideline for cystic fibrosis: 2013 update. Infect Control Hosp Epidemiol. (2014) 35(S1):S1–S67. doi: 10.1086/676882
33. Centers for Disease Control and Prevention. Facility guidance for control of carbapenem-resistant Enterobacteriaceae (CRE). Available at: https://www.cdc.gov/hai/pdfs/cre/CRE-guidance-508.pdf
Keywords: multidrug-resistant, ESBL, CRE, transplant, pediatric
Citation: Dong SW, Sharma TS and Sue PK (2023) Approach to multidrug resistant infections in pediatric transplant recipients. Front. Pediatr. 11:1270564. doi: 10.3389/fped.2023.1270564
Received: 31 July 2023; Accepted: 7 November 2023;
Published: 7 December 2023.
Edited by:
Gabriela Maron, St. Jude Children’s Research Hospital, United StatesReviewed by:
William Otto, Cincinnati Children’s Hospital Medical Center, United States© 2023 Dong, Sharma and Sue. This is an open-access article distributed under the terms of the Creative Commons Attribution License (CC BY). The use, distribution or reproduction in other forums is permitted, provided the original author(s) and the copyright owner(s) are credited and that the original publication in this journal is cited, in accordance with accepted academic practice. No use, distribution or reproduction is permitted which does not comply with these terms.
*Correspondence: Sara W. Dong sara.winn.dong@emory.edu
Disclaimer: All claims expressed in this article are solely those of the authors and do not necessarily represent those of their affiliated organizations, or those of the publisher, the editors and the reviewers. Any product that may be evaluated in this article or claim that may be made by its manufacturer is not guaranteed or endorsed by the publisher.
Research integrity at Frontiers
Learn more about the work of our research integrity team to safeguard the quality of each article we publish.