- 1Division of Neonatology, Department of Pediatrics, Faculty of Medicine Siriraj Hospital, Mahidol University, Bangkok, Thailand
- 2Nursing Division, Department of Pediatrics, Faculty of Medicine Siriraj Hospital, Mahidol University, Bangkok, Thailand
- 3Division of Neonatology, Department of Pediatrics, McMaster University, Hamilton, ON, Canada
Objectives: To determine the correlation and agreement between the SenSmart™ and the INVOS™ devices of neonatal cerebral regional oxygen saturation (CrSO2) measurements using neonatal sensors. The secondary objective was to develop a regression model that predicts CrSO2-INVOS values using CrSO2-SenSmart indices and determine whether the values between the devices are interchangeable.
Methods: A prospective, cross-sectional study was conducted in infants during the first 4 weeks of life. Simultaneous, bilateral CrSO2 was measured using the SenSmart™X100 (CrSO2-SenSmart) or INVOS™ 5100C (CrSO2-INVOS) device in each frontoparietal area for 2 h. Five-minute CrSO2 values were extracted for analysis.
Results: Thirty infants were recruited with 720 pairwise measurements and 26 (84%) were evaluated in the first week of life. Mean gestational age of the preterm and term infants was [30.9 ± 2.8 (n = 14) and 38.8 ± 1.1 (n = 16)] weeks, respectively. Overall CrSO2- was 77.08 ± 9.70% and 71.45 ± 12.74% for the SenSmart and INVOS, respectively (p < 0.001). The correlation coefficient (r) between the CrSO2-SenSmart and INVOS was 0.20 (p < 0.001). The mean difference between the CrSO2-SenSmart and INVOS was 5.63 ± 13.87% with −21.6% to 32.8% limits of agreement. The r and mean difference was 0.39 (p < 0.001) and 8.87 ± 12.58% in preterm infants, and 0.06 (p = 0.27) and 2.79 ± 14.34 in term infants.
Conclusion: The CrSO2-SenSmart tended to read higher than the CrSO2-INVOS device. There was no correlation between the CrSO2-SenSmart and the CrSO2-INVOS in term infants and it was weak in preterms. Due to imprecise agreement, the CrSO2-SenSmart values are not interchangeable with those of the CrSO2-INVOS.
Introduction
Near-infrared spectroscopy (NIRS) is an additional non-invasive bedside device to evaluate regional tissue oxygenation. NIRS when combined with other monitoring techniques holds promise for the improved management and outcomes of critically ill neonates, especially extremely preterm infants (1–5), those with hemodynamic instability (6), encephalopathy (7–9), and cardiac disorders (10, 11). NIRS is most commonly utilized to measure cerebral regional oxygen saturation (CrSO2) to gain better understanding of cerebral oxygenation and autoregulation (12).
Commercial NIRS devices employ spatial resolved spectroscopy via a sensor that emits light at a wavelength in the near-infrared spectrum (700–900 nm). Although there are several commercial devices available for use in neonates, each one has specific characteristics including emitter wavelengths, distance between emitter and detectors, artery-to-venous (A:V) ratio, and an operational algorithm (13). This poses a challenge regarding indices generated across devices which impacts clinical interpretation and how best to employ different devices in the same patients (14–16).
The INVOS™ 5100C neonatal device employs the OxyAlert™ sensor that utilizes a single light emitting diode (LED) source that emits wavelengths at 730 and 810 nm and two detectors located 30 and 40 mm from the emitter. The spatial resolved spectroscopy technique excludes light absorption by hair or skull and solely monitors intracranial components. The device integrates the value of absorbed and scattered light in a specific algorithm with an estimated artery-to-venous ratio (AVR) of 25:75 (17).
The SenSmart™ X-100 (Nonin Medical Inc., Plymouth, MN) is another commercial NIRS device that monitors tissue oxygenation via the Equanox™ sensor and is adapted for use in neonates. Two LEDs emit 4 wavelengths of 730, 760, 810 and 880 nm and the 2 detectors are located 12.5 and 25 mm from each emitter, allowing 2 separate sets of a single emission with 2 detectors. Given dual emitters and detector sensor topology, the sensor generates 4 light paths which exclude extracranial contamination providing accurate tissue oxygenation (17). The algorithm AVR is 30:70. The sensor is durable which allows longer use that leads to better cost-effectiveness. However, studies of normative values and the clinical application of the SenSmart™ in neonates is limited.
Hyttel-Sorensen et al. (14) published an in vitro study showing good correlation of the 3 sensors utilized in the INVOS™ and NIRO™ technologies. Similarly in an in vitro study, Kleiser et al. (18) developed a predictive model involving various types and sizes of the sensors and oximeters that included INVOS™ and SenSmart™, but this was not validated in a clinical study. Andresen et al. (16) published a regression formula to predict the values of the SenSmart™ from measurements by the INVOS™. Dix et al. (15) published a moderate to good correlation of CrSO2 values across 3 different NIRS devices without a direct comparison between the SenSmart™ and the INVOS™.
Our primary objective was to explore the correlation between CrSO2 values measured by the SenSmart™ X-100 device that employs the neonatal/pediatric Equanox™ Advance 8004CB NA sensor (CrSO2-SenSmart) and the INVOS™5100C device that employs the neonatal OxyAlert™ sensor (CrSO2-INVOS). We hypothesized that both devices would have a strong correlation. The secondary objective was to develop a regression model that predicts CrSO2-INVOS using CrSO2-SenSmart indices.
Material and methods
This prospective cross-sectional study was conducted in the neonatal intensive care unit (NICU), Siriraj Hospital in Bangkok, Thailand. We generally monitor CrSO2 in extremely low birthweight (ELBW) infants (birthweight; BW <1,000 g) early in the neonatal course, infants with hypoxic-ischemic encephalopathy (HIE), and those who have severe respiratory compromise. Eligible criteria included infants who were admitted to the NICU within the first 4 weeks of life and were allocated to CrSO2 by the responsible physician. Infants who had skin breakdown, inflammation, or birthmarks at the site of sensor placement were excluded. Subjects were enrolled only once in the study. Parental written consent was obtained prior to infant recruitment. The study protocol was approved by the institutional research committee.
Infants were placed supine and were undisturbed throughout the study period. Measurements of CrSO2 were performed with the INVOS™ 5100C device and OxyAlert™ sensor and the SenSmart™ X-100 device with neonatal/pediatric Equanox™ Advance 8004CB NA sensor. For skin protection in ELBW infants, the cover was not removed. Wijbenga et al. (3), reported similar CrSO2 values measured at the left and right frontoparietal area. We, therefore, compared the CrSO2 values between the two devices by simultaneously placing the sensor of each device on the forehead in the right and left frontoparietal position (19). The Equanox™ and OxyAlert™ sensors were first placed on the right and left frontoparietal areas, respectively and were wrapped with Easifix™ cohesive bandage around the head to ensure fixation. Both devices were synchronized for time and simultaneously monitored. After 1-hour, each sensor was transposed to the alternate site and monitoring continued for a further 1 h. Data from both devices were exported and averaged for 5-second values. The SenSmart™ X-100 analyzes CrSO2 every 1.5 s while the INVOS™ 5100C depicts the average 5-second value. Since variation of CrSO2 is common in the neonatal population (20, 21), we chose the value of the last 5-second average of each 5-minute epoch of the CrSO2-INVOS and the correspondent 5-second average of CrSO2-SenSmart values to permit simultaneous and independent comparisons. In view of the fact that the INVOS™ 5100C only displays values between 15% and 95%, values only within this range were included. Time points with poor signal quality as indicated by error codes were excluded.
Statistical analysis
The sample size was derived from a previous clinical study that showed a correlation coefficient (r) of 0.5 between the CrSO2-INVOS and CrSO2-SenSmart (16). We hypothesized that the two devices would have a good positive correlation (r = 0.8) and estimated that 30 comparisons would be required.
Infant demographic characteristics are presented in number (percentage), mean (±standard deviation; SD) or median [25th percentile, 75th percentile; P25, P75] where appropriate. CrSO2 (%) values are presented in mean ± standard deviation (SD) and compared by independent t-test. We used Spearman correlation coefficient (r) to explore the association between CrSO2- SenSmart and CrSO2-INVOS and simple linear regression analysis to generate a regression model to predict CrSO2-INVOS values using CrSO2-SenSmart indices. Since the operational threshold of 55% has been generally used in neonatal practice (22, 23), we further analyzed the correlation and agreement between both devices based on the CrSO2-INVOS values <55% and ≥55%. Bland–Altman analysis was used to identify agreement between both devices and between predicted CrSO2-INVOS from Kleiser et al. [CrSO2-INVOS = 2.67 (CrSO2-SenSmart) −113.8%] (18) to our measured CrSO2-INVOS. Agreements are presented in mean differences and limits of agreement (bias ± 1.96 SD). Degree of bias was explored for the proportional value of CrSO2 by using Pearson correlational coefficient. IBM SPSS statistics 27 software was used for all analyses. A p-value less than 0.05 was considered statistically significant.
Results
From January 1st, 2022, to January 1st, 2023, 30 infants were included in the study. None of the CrSO2-SenSmart measurements were higher than 95%. Therefore, all 720 pairwise readings were included for analysis. Demographic characteristics are shown in Table 1. Twenty-six infants (84%) were monitored during the first week of life. Fourteen (45.2%) and 16 (54.8%) were preterm and term infants with a mean gestational age (GA) and birthweight (BW) of 30.9 ± 2.8 weeks, 1,514.6 ± 590.4 g, and 38.8 ± 1.1 weeks, and 2,898.1 ± 511.1 g, respectively. The use of respiratory support was similar in preterm and term infants. Fourteen infants (46.7%) received inotropic drugs during the study period. The mean hemoglobin level of the preterm infants was significantly lower than term infants (12.2 ± 2.5 and 15.2 ± 2.3 g/dl, p = 0.002).
Overall, the mean CrSO2-SenSmart (77.08 ± 9.70%) was significantly higher than the CrSO2-INVOS (71.45 ± 12.74%), p < 0.001. Figure 1 shows the scatter plot between CrSO2-SenSmart and CrSO2-INVOS. Linear regression analysis showed a low correlation between the two devices (r = 0.20, p < 0.001). The Bland–Altman plot (Figure 2) demonstrates the overall agreement between CrSO2-SenSmart and CrSO2-INVOS with a positive bias of 5.63 ± 13.87% and limits of agreement that ranged from −21.6% to 32.8%. However, the level of bias was proportional to the average degree of CrSO2-SenSmart and CrSO2-INVOS (p < 0.001).
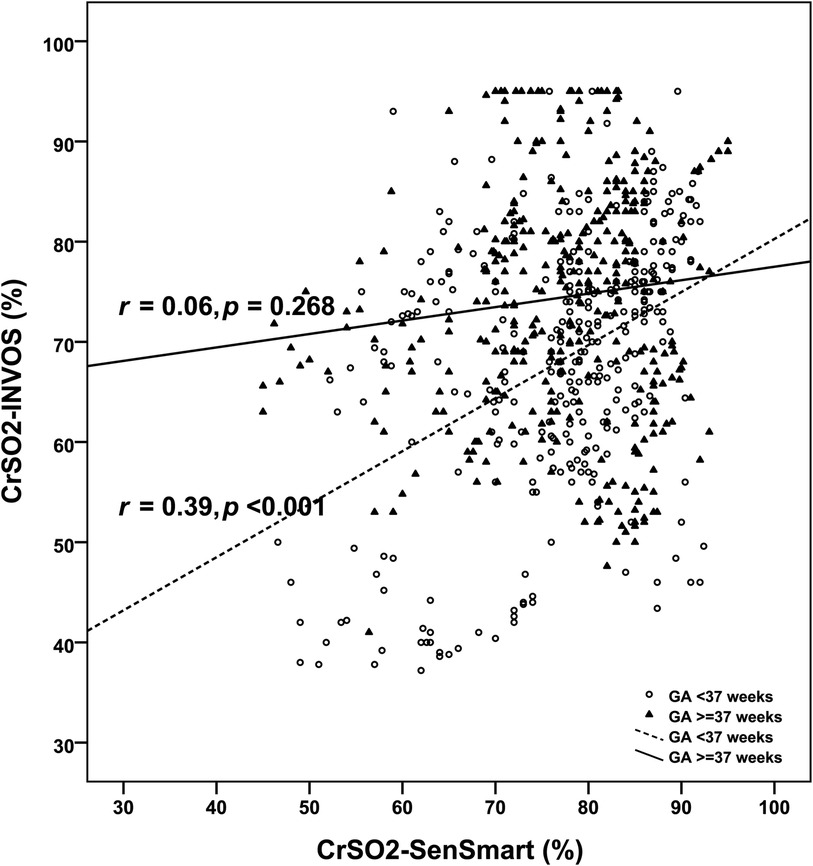
Figure 1. Scatter plots of cerebral regional oxygenation measurements based on the SenSmart™ X100 and the INVOS™ devices (N = 30). The dashed line demonstrates the regression line for preterm infants and the solid line demonstrates the regression line for term infants. r; correlation coefficient.
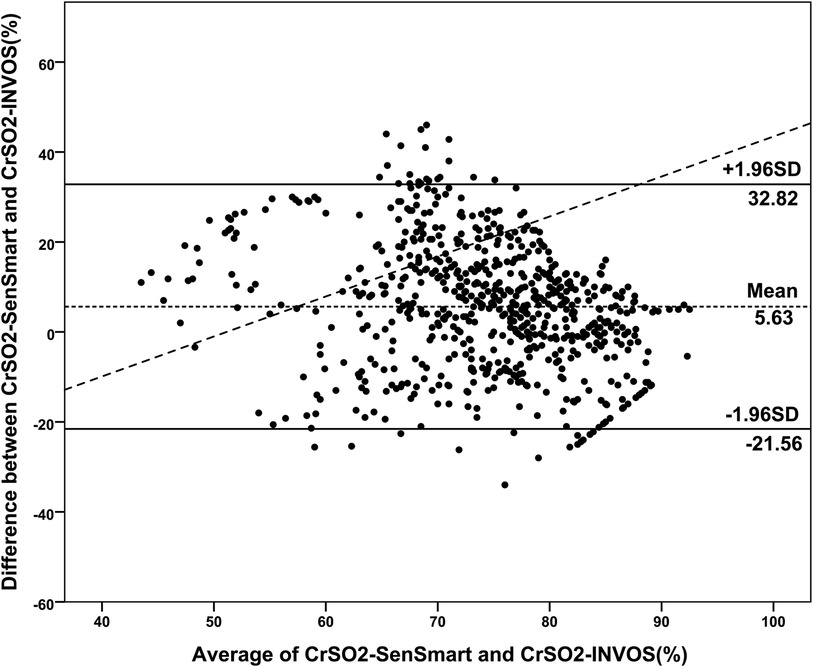
Figure 2. Bland–Altman plots of the differences in cerebral regional oxygenation values measured by the SenSmart™ X100 and the INVOS™ devices (N = 30). The dashed horizontal line indicates the mean of the differences between the two devices, and the solid horizontal lines indicate the upper and lower 95% limits of agreement.
The clinical diagnoses (n) among preterm infants were: Respiratory distress syndrome (4), hydrops fetalis (1), hypoxic-ischemic encephalopathy (HIE) (2), patent ductus arteriosus (1), multiple congenital anomalies (1), persistent pulmonary hypertension of the newborn (PPHN) (1), pulmonary atresia (2), and surgical conditions (2). The mean CrSO2-SenSmart (76.90 ± 10.10%) was significantly higher than the CrSO2-INVOS (68.04 ± 12.82%), p < 0.001 in preterm infants. There was a low correlation between the two devices (r = 0.39, p < 0.001). Bland–Altman analysis revealed a positive bias of 8.87 ± 12.58% with limits of agreement that ranged from −15.8% to 33.5%.
Among the 16 term infants, diagnoses (n) included: HIE with therapeutic hypothermia (7), traumatic brain injury (1), severe anemia with post-cardiac arrest (1), PPHN (2), septic shock (1), dextro-transposition of the great arteries with PPHN (1), tetralogy of fallot (1), gastroschisis (1), and achondroplasia with respiratory failure (1). The mean CrSO2-SenSmart (77.23 ± 9.34%) in term infants was also significantly higher than the CrSO2-INVOS (74.44 ± 11.91%) (p < 0.001). The correlation between the two devices was negligible (r = 0.06, p = 0.268). Bland–Altman analysis revealed a positive bias of 2.79 ± 14.34% with limits of agreement that ranged from −25.3% to 30.9%. Table 2 shows the agreement between the CrSO2-SenSmart and CrSO2-INVOS for each GA group. The CrSO2-SenSmart had a positive bias in all GA groups with wide limits of agreement.
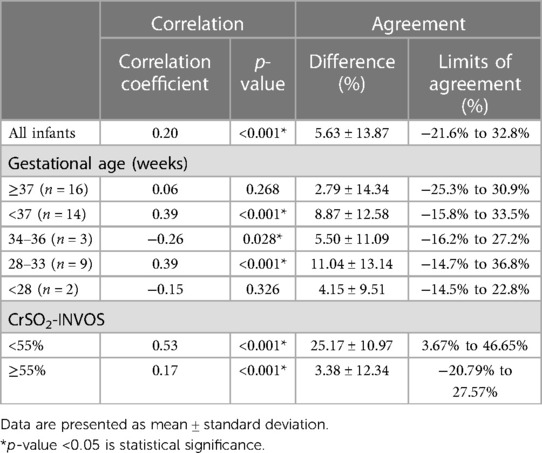
Table 2. Correlation and agreement of cerebral regional oxygen saturation measured by the SenSmart™X-100 (CrSO2-SenSmart) and INVOS™ 5100C (CrSO2-INVOS) (N = 30).
Seventy-four pairwise comparisons (10.3%) had CrSO2-INVOS values <55% while the other 646 pairwise comparisons (89.7%) had CrSO2-INVOS ≥55%. Interestingly, there was a moderate positive correlation in the CrSO2-INVOS <55% (r = 0.53, p < 0.001) while the positive correlation was low in CrSO2-INVOS ≥55% (r = 0.17, p < 0.001).
During the study, 1 infant (3.3%) received dopamine, 11 (36.7%) received dobutamine, 7 (23.3%) received epinephrine, 9 (30%) received norepinephrine, 4 (13.3%) received milrinone, and 6 infants (20%) received hydrocortisone. In total, ten term (62.5%) and 6 preterm infants (42.9%) received inotropic support. We further explored the effect of hemodynamic status on agreement between the devices. Both devices had negligible correlation in infants who received inotropic support (r = 0.13, p = 0.009) and there was a low positive correlation among infants who did not receive inotropic agents (r = 0.31, p < 0.001).
Since term infants had a negligible correlation between the devices, we created regression model to predict CrSO2-INVOS values from CrSO2-SenSmart indices only for preterm infants which is shown in Table 3.
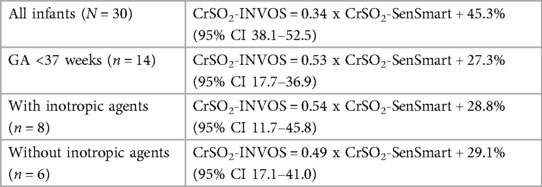
Table 3. Regression model of cerebral regional oxygen saturation measured by the SenSmart™X-100 (CrSO2-SenSmart) to predict the value equivalent to the INVOS™ 5100C (CrSO2-INVOS).
To validate the regression equation from Kleiser et al. (18) we compared predicted CrSO2-INVOS values from CrSO2-SenSmart measurements with our monitored CrSO2-INVOS indices. The correlation coefficient was 0.2 and the mean difference was 20.54 ± 25.74% with limits of agreement that ranged from −29.90% to 70.99%.
Discussion
CrSO2 monitoring is more commonly used in the neonatal setting (24). Several studies demonstrate an association of CrSO2 with occurrences of certain morbidities such as necrotizing enterocolitis (25), respiratory insufficiency, intraventricular hemorrhage (26) and prediction of long-term outcomes (27). However, publications indicate a variation in the choice of NIRS devices and sensors. Currently, there are several devices and neonatal sensors available for use and there is little guidance whether measurements and trends noted in previous studies are uniformly applicable across devices (28).
Several factors need to be considered before accepting NIRS values in neonatal care. These include type of devices, type and size of the sensor, location of sensor placement, and patient's weight. While the INVOS™ 5100C was most commonly used in a large number of studies and generated an operational threshold for clinical intervention (23, 29), newer NIRS devices with updated LED wavelengths, design, and algorithms are now available to improve accuracy of the readings (28). Although values across devices are not interchangeable based on specific topography, they should correlate with each other to facilitate and interpret findings in clinical practice.
Our in vivo results supported by real-world evidence in the NICU, indicates that the CrSO2-SenSmart reads 5.75% higher than the CrSO2-INVOS (p < 0.001) device. The trend of higher readings with Equanox™ sensors compared to other sensors or devices align with the in vitro study (18). We postulate that the unique algorithm and higher AVR of the SenSmart™ contributes to the positive bias that we detected. Disappointingly, we found a low positive correlation between the two devices (r = 0.20) but, more importantly, the imprecision in the mean difference (SD 13.87%) and wider limits of agreement −21.6% to 32.8%) makes it more difficult to predict CrSO2-INVOS values accurately utilizing the SenSmart™ measurements. Although the maximum value of the CrSO2-SenSmart is 100%, compared to 95% in the CrSO2-INVOS, there were no CrSO2-SenSmart values higher than 95%. Therefore this did not impact our results. Interestingly, the correlation between both devices was better in preterm infants (r = 0.39, p < 0.001) which corresponds with the study by Andresen et al. (16) who found a moderate correlation between the INVOS™ and the SenSmart™ (r2 = 0.46) in preterm infants with apnea. The weaker correlation in term infants could be explained by the higher values detected in CrSO2-INVOS ≥55%), which could also contribute to the poor correlation between the devices. Moreover, the heterogenous disorders associated with fluctuating tissue oxygenation in term infants particularly hypoxemia, hypocarbia, and hemodynamic instability related to persistent pulmonary hypertension, severe anemia, or critical congenital heart disease and greater use of inotropic drugs may enhance measurement error across the devices (14, 30). The complexity of abnormal tissue oxygenation in the real-life situation also compliments our finding of the low correlation between our measured values and the predicted value from the in vitro study (18). However, the wide limits of agreement reported by Andresen et al. (16) and this study compromise the ability to accurately predict INVOS™ CrSO2 readings from the SenSmart™. Moreover, the regression model derived from the previous in vitro study (18) when applied to our study was also imprecise and cannot be applied in clinical settings.
We explored the potential of interchanging CrSO2 values between the two NIRS devices in sick neonates. The chosen average CrSO2 of the last 5 s of every 5-minute epoch made each value independent of each other. Along with a large number of comparisons, we analyzed the impact of gestational age on CrSO2 and the effect of inotropic agents. CrSO2 values are potentially interchangeable in preterms but not term infants. Nevertheless, the imprecise agreement jeopardizes accurate prediction of values in any gestational age. Inotropic agents employed to stabilize hemodynamic status could compromise tissue oxygenation and concomitantly have a vasoactive effect. This may have potentially led to a variation of values between the two devices over and above the variation resulting from individual device-related algorithms and A:V ratios. We found low correlations (r = 0.13 and 0.31) whether the subjects received or did not receive inotropic agents, respectively). Therefore, the CrSO2 values from both devices were not interchangeable even if using the predictive model. The operational threshold that was generated from the INVOS™ 5100C device using the in vitro model of Kleiser et al. (18) cannot be properly transferred to the SenSmart™ or other NIRS devices and is likely not valid in a clinical setting. CrSO2 must be monitored using the same device to justify the trend of changes since it is difficult to establish what a clinically relevant threshold should be for a device that is not INVOS™.
There are several limitations of this study that merit consideration. First, the negligible correlation in term infants could have been due to motion artifact (30). Since we compared only the values extracted from the devices, we could not account for the same in the recordings which are more likely to occur in active term infants. However, the large number of paired samples, should have minimized this confounder. Additionally, recognizing this potential limitation, we derived regression equations for only the overall cohort and preterm infants. Second, the number of pairwise comparisons were more than our baseline estimate. We selected our sample size based on a good correlation between devices from a previous study (16) but the same did not hold true in our findings. Therefore, a larger study with a predetermined sample size based on the low correlation from our findings is warranted to expand the generalizability of our results. Last, although the previous study revealed similar CrSO2 values between the left and right frontoparietal areas (19), we accepted that they were non-identical and perhaps contributed to the overall bias. However, this should not have affected the degree of correlation which in part was overcome by the large pairwise samples included in our analysis.
Conclusion
The CrSO2-SenSmart device tends to read higher than the CrSO2-INVOS with a correspondingly low correlation, particularly in in term infants. Both the predictive models derived from a previous study and from this study, cannot accurately predict CrSO2-INVOS from CrSO2-SenSmart values, due to imprecise agreement. Therefore, the values of each device are not interchangeable. The type of device and sensor must be carefully considered when implementing CrSO2 values from previous studies into clinical practice.
Data availability statement
The raw data supporting the conclusions of this article will be made available by the authors, without undue reservation.
Ethics statement
The studies involving humans were approved by Siriraj Institutional Review Board, Mahidol University. The studies were conducted in accordance with the local legislation and institutional requirements. Written informed consent for participation in this study was provided by the participants’ legal guardians/next of kin.
Author contributions
WW performed the data collection and analysis. JA and NY contributed towards the data collection. BP reviewed, edited, and was actively involved in several revisions of the manuscript. RK conceptualized and designed the study, performed the data analysis, drafted, and revised the manuscript. All authors contributed to the article and approved the submitted version.
Acknowledgments
We are grateful to all the parents for allowing their babies to participate in this study. This was an investigator-initiated study, and we thank the local distributors in Thailand for providing both the Equanox™ and OxyAlert™ sensors for this study. However, both companies had no role in the design of the study, interpretation of the data or writing the manuscript.
Conflict of interest
The authors declare that this study did not receive funding from Nonin Medical, Inc. or Medtronic (Thailand) Ltd. The funders had the following involvement with the study: Tech Ace Co., Ltd. (the local distributors of Nonin Medical, Inc in Thailand) supported the Equanox™ sensors and Medtronic (Thailand) Ltd. supported OxyAlert™ sensors for this study.
Publisher's note
All claims expressed in this article are solely those of the authors and do not necessarily represent those of their affiliated organizations, or those of the publisher, the editors and the reviewers. Any product that may be evaluated in this article, or claim that may be made by its manufacturer, is not guaranteed or endorsed by the publisher.
References
1. Tsuji M, Saul JP, du Plessis A, Eichenwald E, Sobh J, Crocker R, et al. Cerebral intravascular oxygenation correlates with mean arterial pressure in critically ill premature infants. Pediatrics. (2000) 106(4):625–32. doi: 10.1542/peds.106.4.625
2. Wong FY, Leung TS, Austin T, Wilkinson M, Meek JH, Wyatt JS, et al. Impaired autoregulation in preterm infants identified by using spatially resolved spectroscopy. Pediatrics. (2008) 121(3):e604–e11. doi: 10.1542/peds.2007-1487
3. Wijbenga RG, Lemmers PM, van Bel F. Cerebral oxygenation during the first days of life in preterm and term neonates: differences between different brain regions. Pediatr Res. (2011) 70(4):389–94. doi: 10.1203/PDR.0b013e31822a36db
4. Pichler G, Baumgartner S, Biermayr M, Dempsey E, Fuchs H, Goos TG, et al. Cerebral regional tissue oxygen saturation to guide oxygen delivery in preterm neonates during immediate transition after birth (cosgod iii): an investigator-initiated, randomized, multi-center, multi-national, clinical trial on additional cerebral tissue oxygen saturation monitoring combined with defined treatment guidelines versus standard monitoring and treatment as usual in premature infants during immediate transition: study protocol for a randomized controlled trial. Trials. (2019) 20(1):178. doi: 10.1186/s13063-019-3258-y
5. Pichler G, Goeral K, Hammerl M, Perme T, Dempsey EM, Springer L, et al. Cerebral regional tissue oxygen saturation to guide oxygen delivery in preterm neonates during immediate transition after birth (COSGOD III): multicentre randomised phase 3 clinical trial. Br Med J. (2023) 381:e072313. doi: 10.1136/bmj-2022-072313
6. Pfurtscheller D, Wolfsberger CH, Höller N, Schwaberger B, Mileder LP, Baik-Schneditz N, et al. Cardiac output and regional-cerebral-oxygen-saturation in preterm neonates during immediate postnatal transition: an observational study. Acta Paediatr. (2023) 112(7):1404–12. doi: 10.1111/apa.16745
7. Szakmar E, Smith J, Yang E, Volpe JJ, Inder T, El-Dib M. Association between cerebral oxygen saturation and brain injury in neonates receiving therapeutic hypothermia for neonatal encephalopathy. J Perinatol. (2021) 41(2):269–77. doi: 10.1038/s41372-020-00910-w
8. Toet MC, Lemmers PM, van Schelven LJ, van Bel F. Cerebral oxygenation and electrical activity after birth asphyxia: their relation to outcome. Pediatrics. (2006) 117(2):333–9. doi: 10.1542/peds.2005-0987
9. Garvey AA, O'Toole JM, Livingstone V, Walsh B, Moore M, Pavel AM, et al. Evolution of early cerebral nirs in hypoxic ischaemic encephalopathy. Acta Paediatr. (2022) 111(10):1870–7. doi: 10.1111/apa.16493
10. Fenton KN, Freeman K, Glogowski K, Fogg S, Duncan KF. The significance of baseline cerebral oxygen saturation in children undergoing congenital heart surgery. Am J Surg. (2005) 190(2):260–3. doi: 10.1016/j.amjsurg.2005.05.023
11. Spaeder MC, Surma VJ. Cerebral regional oxygen saturation variability in neonates following cardiac surgery. Ped Res. (2021) 90(4):815–8. doi: 10.1038/s41390-020-01171-1
12. Mohamed MA, Frasketi MJ, Aly S, El-Dib M, Hoffman HJ, Aly H. Changes in cerebral tissue oxygenation and fractional oxygen extraction with gestational age and postnatal maturation in preterm infants. J Perinatol. (2021) 41(4):836–42. doi: 10.1038/s41372-020-00794-w
13. Vesoulis ZA, Mintzer JP, Chock VY. Neonatal nirs monitoring: recommendations for data capture and review of analytics. J Perinatol. (2021) 41(4):675–88. doi: 10.1038/s41372-021-00946-6
14. Hyttel-Sorensen S, Kleiser S, Wolf M, Greisen G. Calibration of a prototype nirs oximeter against two commercial devices on a blood-lipid phantom. Biomed Opt Express. (2013) 4(9):1662–72. doi: 10.1364/boe.4.001662
15. Dix LM, van Bel F, Baerts W, Lemmers PM. Comparing near-infrared spectroscopy devices and their sensors for monitoring regional cerebral oxygen saturation in the neonate. Pediatr Res. (2013) 74(5):557–63. doi: 10.1038/pr.2013.133
16. Andresen B, Greisen G, Hyttel-Sorensen S. Comparison of invos 5100c and nonin SenSmart x-100 oximeter performance in preterm infants with spontaneous apnea. Pediatr Res. (2020) 87(7):1244–50. doi: 10.1038/s41390-020-0752-6
17. Davie SN, Grocott HP. Impact of extracranial contamination on regional cerebral oxygen saturation: a comparison of three cerebral oximetry technologies. Anesthesiology. (2012) 116(4):834–40. doi: 10.1097/ALN.0b013e31824c00d7
18. Kleiser S, Nasseri N, Andresen B, Greisen G, Wolf M. Comparison of tissue oximeters on a liquid phantom with adjustable optical properties. Biomed Opt Express. (2016) 7(8):2973–92. doi: 10.1364/BOE.7.002973
19. Bruns N, Moosmann J, Münch F, Dohna-Schwake C, Woelfle J, Cesnjevar R, et al. How to administer near-infrared spectroscopy in critically ill neonates, infants, and children. J Vis Exp. (2020) 162:e61533. doi: 10.3791/61533
20. Hyttel-Sorensen S, Hessel TW, la Cour A, Greisen G. A comparison between two nirs oximeters (invos, oxyprem) using measurement on the arm of adults and head of infants after caesarean section. Biomed Opt Express. (2014) 5(10):3671–83. doi: 10.1364/boe.5.003671
21. Hessel TW, Hyttel-Sorensen S, Greisen G. Cerebral oxygenation after birth - a comparison of INVOS(®) and FORE-SIGHT™ near-infrared spectroscopy oximeters. Acta Paediatr. (2014) 103(5):488–93. doi: 10.1111/apa.12567
22. Hyttel-Sorensen S, Pellicer A, Alderliesten T, Austin T, van Bel F, Benders M, et al. Cerebral near infrared spectroscopy oximetry in extremely preterm infants: phase ii randomised clinical trial. Br Med J. (2015) 350:g7635. doi: 10.1136/bmj.g7635
23. Hansen ML, Pellicer A, Hyttel-Sørensen S, Ergenekon E, Szczapa T, Hagmann C, et al. Cerebral oximetry monitoring in extremely preterm infants. N Engl J Med. (2023) 388(16):1501–11. doi: 10.1056/NEJMoa2207554
24. Hunter CL, Oei JL, Suzuki K, Lui K, Schindler T. Patterns of use of near-infrared spectroscopy in neonatal intensive care units: international usage survey. Acta Paediatr. (2018) 107(7):1198–204. doi: 10.1111/apa.14271
25. El-Dib M, Munster C, Sunwoo J, Cherkerzian S, Lee S, Hildrey E, et al. Association of early cerebral oxygen saturation and brain injury in extremely preterm infants. J Perinatol. (2022) 42(10):1385–91. doi: 10.1038/s41372-022-01447-w
26. Katheria AC, Harbert MJ, Nagaraj SB, Arnell K, Poeltler DM, Brown MK, et al. The neu-prem trial: neuromonitoring of brains of infants born preterm during resuscitation-a prospective observational cohort study. J Pediatr. (2018) 198:209–13.e3. doi: 10.1016/j.jpeds.2018.02.065
27. Horne RSC. Can use of cerebral oxygenation predict developmental outcomes in preterm infants with nec? Pediatrics. (2020) 146(3):e2020014407. doi: 10.1542/peds.2020-014407
28. Bruckner M, Wolfsberger CH, Dempsey EM, Liem KD, Lemmers P, Alderliesten T, et al. Normal regional tissue oxygen saturation in neonates: a systematic qualitative review. Pediatr Res. (2021). doi: 10.1038/s41390-021-01786-y [Online ahead of print]34667270
29. Pichler G, Binder C, Avian A, Beckenbach E, Schmolzer GM, Urlesberger B. Reference ranges for regional cerebral tissue oxygen saturation and fractional oxygen extraction in neonates during immediate transition after birth. J Pediatr. (2013) 163(6):1558–63. doi: 10.1016/j.jpeds.2013.07.007
Keywords: cerebral oxygen, INVOS, NIRS, SenSmart, tissue oxygenation
Citation: Wirayannawat W, Amawat J, Yamsiri N, Paes B and Kitsommart R (2023) Comparison of the SenSmart™ and the INVOS™ neonatal cerebral near-infrared spectrometry devices. Front. Pediatr. 11:1243977. doi: 10.3389/fped.2023.1243977
Received: 21 June 2023; Accepted: 15 August 2023;
Published: 25 August 2023.
Edited by:
Kiran More, Bai Jerbai Wadia Hospital for Children, IndiaReviewed by:
Aravanan Anbu Chakkarapani, Sidra Medicine, QatarValerie Chock, Stanford University, United States
© 2023 Wirayannawat, Amawat, Yamsiri, Paes and Kitsommart. This is an open-access article distributed under the terms of the Creative Commons Attribution License (CC BY). The use, distribution or reproduction in other forums is permitted, provided the original author(s) and the copyright owner(s) are credited and that the original publication in this journal is cited, in accordance with accepted academic practice. No use, distribution or reproduction is permitted which does not comply with these terms.
*Correspondence: Ratchada Kitsommart cmF0Y2hhZGEua2l0QG1haGlkb2wuYWMudGg=; cmtpdHNvbW1hcnRAaG90bWFpbC5jb20=