- 1Department of Paediatrics and Child Health, Faculty of Medicine and Health Sciences, Stellenbosch University, Cape Town, South Africa
- 2Infection Prevention and Control Service, Mowbray Maternity Hospital, Cape Town, South Africa
- 3Center for Neonatal and Pediatric Infection, St George's University of London, London, United Kingdom
- 4Infection, Immunity and Inflammation, UCL Great Ormond Street Institute of Child Health, University College London, London, United Kingdom
- 5Department of Medical Microbiology, University of Ghana Medical School, Accra, Ghana
- 6Department of Paediatric and Adolescent Health, Princess Marina Hospital, Gaborone, Botswana
- 7Department of Global Medicine, Children's Hospital of Philadelphia, Philadelphia, PA, United States
- 8Division of Medical Microbiology, Faculty of Medicine and Health Sciences, Stellenbosch University, Stellenbosch, South Africa
- 9National Health Laboratory Service, Tygerberg Hospital, Cape Town, South Africa
- 10Division of Infectious Diseases, Department of Pediatrics, Children's Hospital of Philadelphia, Philadelphia, PA, United States
Healthcare-associated infections (HAIs) and antimicrobial-resistant (AMR) infections are leading causes of neonatal morbidity and mortality, contributing to an extended hospital stay and increased healthcare costs. Although the burden and impact of HAI/AMR in resource-limited neonatal units are substantial, there are few HAI/AMR prevention studies in these settings. We reviewed the mechanism of action and evidence supporting HAI/AMR prevention interventions, including care bundles, for hospitalized neonates in low- and middle-income countries (LMIC).
Introduction
Epidemiology of Neonatal Deaths in Low- and Middle-Income Countries
The neonatal period, defined as the first 28 days from birth, is the most vulnerable period in the life of a child, accounting for 47% of deaths in children under 5 years of age (1, 2). For neonates in LMIC, the risk of death is up to 11-fold greater than in high-income countries (2). Reducing neonatal mortality to below 12 per 1,000 live births globally by 2030 is a key target of the sustainable development goals (SDGs) (2). Despite substantial progress in reducing the annual number of global neonatal deaths from 5 to 2.5 million between 1990 and 2019, there has been no reduction in neonatal mortality in sub-Saharan Africa. Over 95% of the 2.5 million neonatal deaths each year now occur in LMICs, with the sub-Saharan African and South-East Asian regions contributing approximately 1 million deaths each, with 27 and 23 deaths per 1,000 live births, respectively (2).
Contribution of Severe Bacterial Infection to Neonatal Mortality
Approximately, one-third of neonatal deaths annually (680,000) are caused by infections (3), notably severe bacterial infections (bloodstream infection, pneumonia, and meningitis). In LMIC, the proportion of neonatal infections that are healthcare-associated infections (HAIs) to total neonatal infections is uncertain, as few neonatal units in resource-limited settings conduct systematic surveillance (4). There is also evidence that many deaths in preterm neonates are attributable to sepsis, but are mislabeled as prematurity-related deaths due to a lack of access to accurate diagnostic tests (5). The burden and impact of neonatal HAI are substantial and are likely to increase due to growth in population, in-hospital births, and preterm delivery rates in sub-Saharan Africa and South-East Asia (6).
Factors Contributing to Bacterial Colonization in LMIC Neonatal Units
In resource-limited settings, early and heavy neonatal bacterial pathogen colonization occurs through exposure to suboptimal water, sanitation, hygiene, infection prevention and control (IPC) practices (4, 7), and high rates of maternal colonization with multidrug-resistant organisms (MDROs) (8) There are numerous challenges to the implementation of IPC best practices in LMIC obstetric and neonatal units. These include overcrowding, shared equipment with inadequate reprocessing, inadequate environmental cleaning, suboptimal hand hygiene compliance, limited laboratory and capacity for HAI surveillance with delayed recognition of outbreaks, and high rates of antibiotic prescribing (4, 7, 9, 10). In such settings, sustained bacterial transmission pressure leads to MDRO colonization, with many neonates subsequently developing HAI after bacterial translocation, invasion, and dissemination (11).
We reviewed the literature to identify, categorize, and prioritize HAI prevention interventions for hospitalized neonates in LMIC, including care bundles and multimodal infection prevention programs. Below, we present our findings, categorized into four main domains of interventions: (a) promotion of colonization with normal flora, (b) prevention of colonization with pathogens, (c) maintenance of skin integrity, and (d) HAI surveillance, education, and advocacy (Table 1).
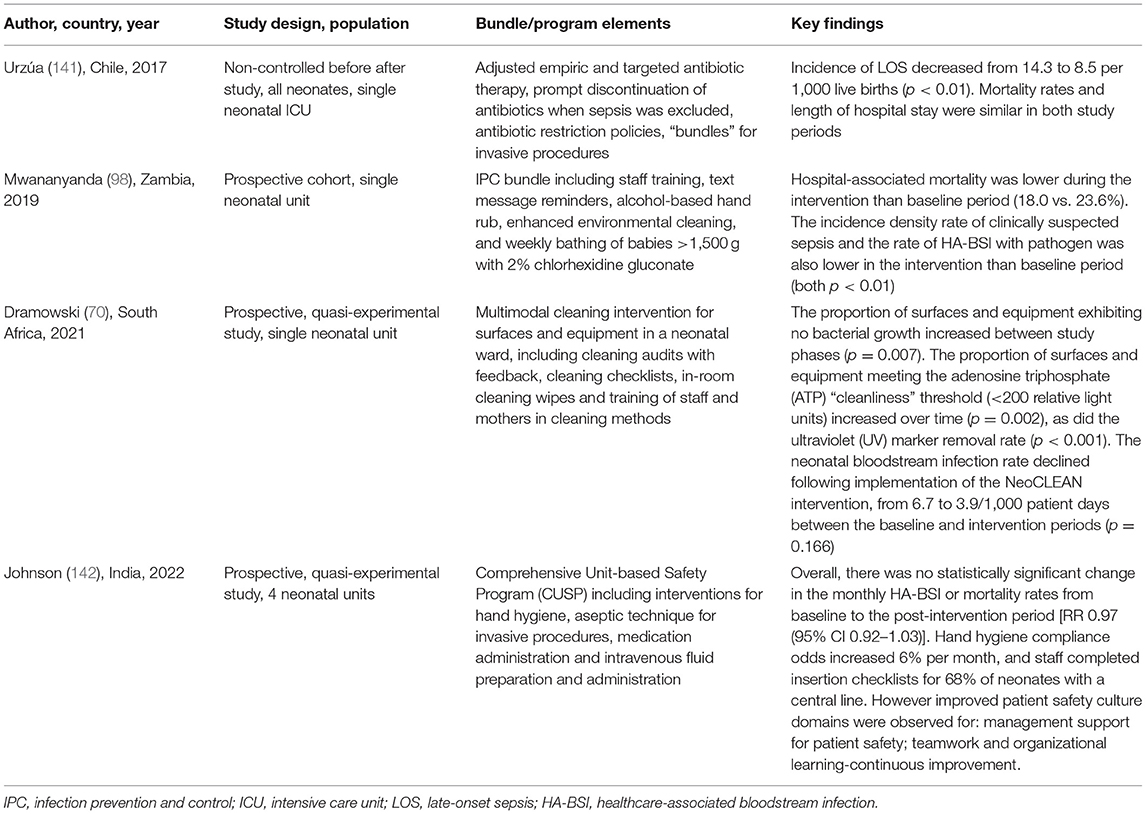
Table 1. Selected healthcare-associated infection (HAI) prevention bundles and programs implemented in low- and middle-income country (LMIC) neonatal units.
Promoting Colonization With Normal Flora
Kangaroo Mother Care and Breastmilk
Neonates are extremely vulnerable to HAIs owing to their immune system immaturity and poorly developed skin and gastrointestinal barriers. Both kangaroo mother care (KMC) and breast feeding are evidence-based interventions that significantly reduce HAI risk and length of hospital stay, promote growth, and enhance neonatal survival (12–15). Several meta-analyses concluded that KMC reduces in-hospital neonatal mortality by up to 40% in babies with birth weight <2,000 g, and is highly effective in reducing severe morbidity, particularly from infection (12, 13). A recent randomized trial provides new evidence that a further 25% reduction in preterm neonatal mortality can be achieved by initiating KMC immediately after birth, instead of after clinical stability is attained. Despite its major benefits, KMC is not widely adopted in many LMICs. Inadequate facilities and/or space, limited hospital budgets, inadequate staffing, lack of KMC policies, and the reluctance of families to accept extended hospitalization are the main barriers to KMC uptake in Africa (16–18).
Mother's breastmilk is critical to seeding the neonatal gut microbiota, providing passive immunity and accelerating mucosal immune development and maturation of the intestinal epithelium, contributing to intestinal barrier integrity and decreasing infection risk. Bioactive substances in breastmilk, such as immunoglobulins, immune cells, microbes, mucins, cytokines, and human milk oligosaccharides, promote the development of the neonate's immune system and reduce infection risk (15). Both KMC and exclusive breastmilk feeding are low-cost, feasible, and evidence-based interventions, but require the continuous, onsite presence of mothers at the hospital. To enable the full benefits of these interventions to be realized, neonatal units in LMIC must include “in-unit” beds and KMC facilities.
Probiotics
Despite extremely promising, neonatal probiotic interventions are not yet well-established options for IPC care bundles. Probiotics, such as Bifidobacterium spp. and Lactobacillus spp., are live microorganisms, which support neonatal gut microbiota colonization (19). Preterm neonates with immature gut development may benefit from early probiotic exposure to improve intestinal barrier integrity and modulate immune system development (20). A systematic review of 23 randomized controlled trials in preterm neonates in LMICs compared probiotics to placebo and found that probiotics-supplemented neonates experienced a reduced risk of necrotizing enterocolitis (NEC), late-onset sepsis, and all-cause mortality (21). Access to probiotics remains limited in LMIC neonatal units and more research is needed to identify the optimal combination of strains for HAI prevention. A similar product of interest is prebiotics, which can be produced and administered exogenously, resembling prebiotics that occurs naturally in breast milk. Prebiotics can promote colonization by commensal organisms, inhibit pathogen binding, and strengthen the intestinal barrier. Prebiotics and symbiotics (a combination of prebiotics and probiotics) have very little efficacy data in the neonatal population, and further trials are required (22).
Antibiotic Stewardship and Diagnostic Microbiology Laboratory Support to Neonatal Units
Neonates are at high risk of bacterial infection with high empiric antibiotic prescription rates in LMICs attributable to diagnostic uncertainty, low blood culture yields, and concerns for infection-related deaths. The adverse long-term effects of neonatal antibiotic exposure are increasingly recognized, including shifts in gastrointestinal microbiome composition and selection for resistance genes (23). This phenomenon occurs both in individual patients and in the neonatal unit environment resulting in multiple foci of antibiotic-resistant bacteria (24–26). For this reason, antimicrobial stewardship programs (ASP) are of particular importance in neonatal units, although data on program implementation in LMIC neonatal units are very limited (27–29).
Up to 16 times more neonates receive antibiotics for culture-negative sepsis than for blood culture-confirmed infection, with suspected early onset sepsis contributing the largest proportion (30). Prompt cessation of empiric antibiotics is therefore a priority for antimicrobial stewardship in LMIC neonatal units. This could be facilitated by aseptically collected blood cultures, adequate blood culture bottle inoculum volume (minimum 1 ml), and prompt antibiotic discontinuation in the absence of clear clinical, biochemical, or microbiological indicators of infection (30). A barrier to this is the time-to-detection of bacterial growth. Current estimates of 48 h are based on automated blood culture systems, which show better performance in yield and speed, but pose financial and logistic challenges compared to manual blood culture systems, which are largely used in LMICs (31). The time-to-detection of growth is not clearly defined for manual blood cultures, and further research and innovation are needed in manual blood culture methods (32). For culture-positive cases, antibiotic treatment should be tailored to the pathogen's susceptibility using the narrowest spectrum antibiotic available. Antibiotic stewardship committees to advise on difficult cases and provide antibiotic authorization may also curb unnecessary antibiotic use.
An additional and growing challenge in LMIC neonatal units is antimicrobial resistance (AMR), which contributes to an estimated 140,000 neonatal deaths annually (33). Inappropriate and unnecessary use of antimicrobials in neonatal units exacerbates the development of AMR and further limits options for the treatment of AMR infections. Acquisition of colonization with AMR pathogens and subsequent development of HAI is a particular problem in neonatal intensive care units (NICU), owing to higher use of invasive devices and higher rates of exposure to antibiotics. Strategies to slow the development of AMR in neonatal units must include both considerate antibiotic use (through ASP) and the adoption of strict infection prevention practices. However, the evidence base for effective ASP in LMIC neonatal units remains limited (27).
The infrastructure, services, and expertise of diagnostic microbiology laboratories are critical contributors to IPC and ASP (10). LMIC neonatal units, in particular, benefit from access to on-site or closely situated microbiology laboratories, owing to their high burden of HAI and frequent outbreaks (34). Additional important contributions of clinical microbiology services include rapid pathogen detection, identification of resistance patterns, development of antibiograms, outbreak investigation, diagnostic stewardship, and HAI surveillance (10).
Prevention of Colonization With Pathogens
Prevention of Vertical Pathogen Transmission in Labor
Many interventions to reduce vertical bacterial transmission and neonatal sepsis do not specifically target MDROs and reduce the transmission of antibiotic-resistant and susceptible pathogens. Two such interventions are prepartum chlorhexidine gluconate (CHG) vaginal cleaning and neonatal whole-body CHG bathing. To date, the results have been mixed, with one South African study of intrapartum 0.5% CHG vaginal wipes showing no change in early onset neonatal sepsis or transmission of Group B Streptococcus (35), while another study in Zimbabwe using 1% CHG wipes showed a 50% reduction in vaginal pathogen colonization compared to standard care (36). An ongoing clinical trial in Malawi (NeoVT-AMR), comparing CHG and octenidine, should provide further insights into the impact of vaginal wipes on pathogen transmission (37).
The World Health Organization (WHO) promotes the use of clean birth kits in LMICs, containing soap, razor blade, cord ties, alcohol swabs, plastic sheets, and gauze. A recent review showed that birth kits were associated with reductions in puerperal sepsis, neonatal tetanus, perinatal, neonatal, and infant mortality (38). Barriers to the widespread use of clean birth kits and antiseptics include the lack of awareness, cost, and availability.
Mode of delivery and intrapartum antibiotic prophylaxis (IAP) influence neonatal MDRO acquisition. Metagenomic profiling showed that neonates born by Cesarean section, as compared to vaginal birth, had greater gut colonization with potential pathogens (including Klebsiella species, Enterobacter species, and Enterococcus species). The authors speculated that pathogen colonization was driven by extended exposure to the hospital environment (i.e., prolonged in-hospital stay following Cesarean section) (39). In this and other studies, babies delivered vaginally who received IAP, also showed microbiota perturbation. Further research is needed to identify ways to modulate the impact of mode of delivery and IAP on neonatal bacterial colonization (40).
Decolonization Interventions
Mupirocin for methicillin-resistant Staphylococcus aureus (MRSA) decolonization is an intervention aimed at reducing transmission of a specific MDRO although it is worth remembering that this intervention will also reduce methicillin-susceptible S. aureus colonization. There is still debate about the role of nasal mupirocin to reduce MRSA carriage, including who to screen and when, and how to manage recolonization, which is seen in up to 50% of neonates. A Society for Healthcare Epidemiology of America (SHEA) white paper in 2020 suggested that neonatal strategies for bacterial decolonization target specific contexts (e.g., outbreaks) or populations (e.g., patients at high risk of infection) (41). The evidence supporting nasal mupirocin is mixed, with some reports of successful reduction and elimination of MRSA colonization, infection, or both (42). Others argue that alternative approaches such as cleaning (43) or adoption of a multimodal approach, including strict cohorting, colonization screening, improved hand hygiene, and environmental cleaning compliance can reduce MRSA transmission (44). Whole-body CHG bathing is an example of an intervention aimed at reducing bacterial transmission and colonization in general (45–47), although the evidence for reducing HAI and MDRO infection is conflicting (48, 49).
Hand Hygiene
The hands of healthcare workers are frequently contaminated in the course of healthcare delivery and are often implicated in pathogen transmission (50, 51). Hand hygiene is identified as a key HAI prevention strategy by the WHO and most national IPC guidelines, with demonstrated success in improving hand hygiene compliance in resource-limited settings. However, there is limited evidence to improve hand hygiene compliance and prevent HAI in resource-limited neonatal units (52–55). As parents provide a large proportion of daily neonatal care and contribute a high number of hand hygiene opportunities, any hand hygiene intervention should involve these important caregivers (55).
Screening for MDROs
Multi-drug-resistant Gram-negative bacteria, such as Klebsiella pneumoniae and Acinetobacter baumannii, are the predominant HAI pathogens in LMIC neonatal units (56–59). In sub-Saharan Africa, neonatal rectal MDRO colonization rates have been shown to exceed 50% in some settings, with carriage rates rising rapidly the following hospitalization (47, 60, 61). Active patient screening for MDRO colonization at admission or at regular intervals during hospitalization may facilitate early identification, isolation, and application of contact precautions to reduce potential cross-infection within neonatal units (62). However, most resource-limited neonatal units have few or no isolation facilities, complicating the ability to separate MDRO-colonized neonates from non-colonized neonates. Given the widespread and hyperendemic nature of MDRO colonization in hospitalized LMIC neonates, active screening may be particularly useful during outbreaks or for limited surveillance approaches [e.g., monthly point prevalence surveys (PPS)].
The Hospital Environment: Built, Aqueous, and Equipment
Overcrowding is often cited as a contributing factor to pathogen transmission in neonatal units (63–65), but efforts to redesign hospital environments to reduce crowding and facilitate other IPC measures have not been rigorously studied in LMICs. In high-income countries, single family rooms/units (vs. cohort wards/cubicles) have lowered HAI rates (66, 67). However, single patient rooms are neither feasible nor safe in under-resourced neonatal units due to a lack of healthcare personnel and monitoring devices. Modular walls and movable barriers allow flexible cohorting by MDRO colonization or infection status, with evidence of a modest reduction in horizontal infection transmission (68, 69). In resource-limited neonatal units, incubators have been used successfully for patient “isolation” in the absence of formal isolation rooms or areas.
Sink drains, washbasins, and other moist surfaces (e.g., humidifiers and ventilator tubing) are sources of nosocomial outbreaks in neonatal units (70–73). Potential interventions to prevent colonization with waterborne pathogens include waterless hand hygiene using CHG or alcohol-based hand sanitizer (74), water-free patient care including sink removal from patient care areas (75, 76), and acetic acid (vinegar) as a low-cost method to decontaminate sink drains and multiple-use medical equipment (77–79).
Prevention of horizontal MDRO transmission in neonatal units relies on the implementation of robust IPC practices, including cleaning and disinfection of high-touch surfaces and equipment (80). While cleaning and disinfection can reduce the burden of pathogens in the neonatal environment (70), implementing and sustaining adequate environmental cleaning remains a challenge. High-touch surfaces may become contaminated between cleaning times, undermining hand hygiene initiatives by causing hand recontamination in the neonatal care unit. The use of low-cost tools, such as fluorescent markers or gel dots, to assess cleaning quality and provide regular feedback is relatively easy to implement in many LMIC settings. This strategy, within a multimodal bundle, has been demonstrated to improve cleaning practices (70, 81, 82). Commonly used neonatal medical and feeding equipment, such as suction catheters (83–85) and breast pumps (86, 87), are also prone to contamination, especially when shared or reused. Potential interventions include multimodal cleaning interventions (70), nurse training in disinfection methods, (79) and using surface materials with antimicrobial properties (88).
Skin Antisepsis
Interventions to improve skin integrity and antisepsis represent a crucial opportunity to prevent HAI and require greater clinical focus and further research. As the largest organ in the greatest contact with the external environment, neonatal skin is an important area of vulnerability, particularly in preterm neonates. However, evidence to guide the best interventions for skin antisepsis and integrity remains limited. Skin antisepsis prior to invasive procedures is well established, but there is a significant variation in the practice of the use of antiseptics for the prevention of neonatal sepsis. There is no consensus on the best antiseptic and concentration to use. Considerations must include balancing efficacy and safety concerns, particularly in extremely preterm neonates who are at risk of severe skin reactions (89–93).
Chlorhexidine gluconate is widely used for skin preparation prior to invasive procedures (94), but in addition to uncertainties about the optimal concentration, the question of whether to combine with alcohol (as recommended in older children/adults) is the subject of ongoing research (95). Other less commonly used antiseptics such as octenidine and povidone-iodine are also being studied, although the latter is associated with a risk of thyroid dysfunction (96). Importantly, increasing documentation of tolerance of Enterobacterales (including Klebsiella spp.) and Staphylococcus spp. to antiseptics, including CHG and octenidine, may decrease their effectiveness and necessitate the use of higher antiseptic concentrations (92). In addition, the efficacy of CHG and other antiseptics for the prevention of neonatal sepsis in most LMICs where Gram-negative pathogens predominate is unclear (47). Aside from antisepsis for invasive procedures, there is strong evidence for prophylactic CHG (4%) application to the umbilical cord in the community in high mortality settings (97), and for reduction of infection and mortality with CHG bathing (2%) within the multiple bundled IPC interventions in hospitalized neonates (98). There is a clear need for more evidence on whole-body prophylactic antiseptic use in high-risk hospitalized neonates, including optimal type, frequency, and concentration.
Maintenance of Skin Integrity
The promotion of neonatal skin integrity requires multifaceted approaches that include rationalizing medical procedures, minimizing harm from devices and medical adhesives (99), positioning to prevent pressure sores, careful diapering, using barrier creams, providing skin-to-skin and KMC, as well as optimizing humidity, temperature, and nutrition (100, 101). Beyond this, generalized emollient application, which improves neonatal skin condition (102), is also receiving increased research focus using either synthetic or natural products. Emollient-impregnated wipes have been demonstrated to improve skin conditions, but may be prohibitively expensive (103). Importantly, some studies have suggested that the use of certain emollients may be associated with increased colonization by Staphylococcus spp. and increased rates of candidaemia (100). Alternatively, natural oils have been in longstanding traditional use, but some oils may be harmful (104, 105) while others have advantages with particular fatty acid profiles (e.g., higher linoleic acid content) (106–108). The use of sunflower oil as an emollient demonstrated a reduction in infections in neonates in a hospital-based study (109), and encouraging trends in a subgroup analysis of very low birth weight infants in a community-based study (106); however, more evidence is required (110). The strategy of combining emollients with antiseptics has also been the subject of recent pilot studies (47) and warrants further research.
HAI Surveillance, IPC Education, and Advocacy
Advocacy for Infection Prevention and Surveillance Programs in LMIC Neonatal Units
Low- and middle-income countries' neonatal units face many challenges, such as inadequate financing, understaffing, aging infrastructure, and the lack of effective leadership (8). IPC is the foundation of safe healthcare service provision for vulnerable populations. Prioritization of IPC programs is likely one of the most effective in-hospital public health interventions for LMICs striving to reduce neonatal mortality. Support and guidance on developing and sustaining neonatal unit IPC programs are needed from national and provincial ministries of health, institutional managers, neonatal nurses, and physician leaders alike. Medical and nursing societies are an often untapped area of support for clinicians and infection control practitioners. Benefits involve sharing expertise, networking beyond one's own place of work, developing guidelines, and advocacy. A recent example is a supplement on antimicrobial stewardship and IPC for LMICs (111).
Enhanced HAI Surveillance and Feedback
Institution of infection surveillance in neonatal units is a priority for patient safety and quality of care (112, 113). Coordinated infection surveillance programs and regular IPC practice audits are key activities to assist with the identification of neonatal units with high HAI burden. Surveillance on its own can contribute to the reduction of HAIs (114) and can be an effective mechanism for early detection and control of HAIs including outbreaks (113, 115). HAI surveillance establishes infection rates and trends, detects outbreaks, and measures the risk of infection. Any HAI surveillance plan should yield accurate and comprehensive information within the limits of available resources (116). Surveillance data should ideally include stratification by infection timing (early onset sepsis vs. HAI), infection type, or site (bloodstream, urinary tract infection or meningitis; laboratory-confirmed vs. clinically suspected), and perinatal risks of infection (birth weight, gestation, comorbidities, anatomical anomalies, invasive procedures, and indwelling devices) and causative pathogens with their antimicrobial susceptibility profile (when available).
Although prospective HAI surveillance is the gold standard, it is neither feasible nor sustainable in many LMICs. Also, many low-resource settings lack diagnostic microbiology services, which are key to implementing prospective surveillance (32). An alternative method of surveillance, PPS using clinical definitions of HAI, although less robust, is less expensive and easier to conduct in resource-limited settings (117, 118). Ideally, PPS should focus on a limited spectrum of high-impact neonatal HAI (such as bloodstream infections and meningitis) and be coupled with ongoing improvement efforts. Also, PPS protocols using clinical definitions should be considered for settings that lack access to diagnostic microbiology services but may overestimate the HAI burden.
Regular audits of compliance with IPC standard operating procedures should also be conducted. The audit tool must be customized so that observations align with the unique practices and risks particular to neonatal care (119). Other performance data that provide valuable information on IPC risk are hand hygiene compliance audits, compliance with IPC interventions (e.g., care bundles), and adverse events in relation to IPC. All of these data sources must be evaluated in conjunction so that priorities for performance improvement can be determined and targeted interventions can be implemented. Regular feedback to staff about performance and HAI outcomes helps to reinforce IPC education, maintain staff engagement, create a culture of compliance, and modify behavior (120, 121).
Staff and Parent Education
Healthcare staff training should be based on up-to-date IPC standard operating procedures for the healthcare institution, ensuring that all staff follows expected standards of care. To ensure compliance with IPC standards, regular in-service training accompanied by the assessment of knowledge and performance is essential. Teaching methods should be varied and aimed at achieving active participation, promoting insights, and eventually effecting behavior modification. IPC education is a key element in the success of multimodal HAI performance improvement initiatives (122). As mothers are constantly present in the neonatal unit, their importance in IPC should be recognized and incorporated into standards of care, IPC education, and monitoring programs. Topics should include hand hygiene, infection transmission between babies, the need for clean equipment and clean surfaces around the baby, maintaining good personal hygiene, handling of expressed breast milk and formula feeds, and reporting to medical staff if they become ill.
Infection Prevention Bundles and Programs for LMIC Neonatal Units
Bundling care interventions to prevent neonatal sepsis in LMIC has great potential. Care bundles simplify and enable the reliable application of evidence-based best practices, typically applying four–six evidence-based interventions simultaneously (123). Ideally, bundle elements should be supported by data from randomized controlled trials, although evidence from peer-reviewed, indexed research papers is acceptable where trial data are lacking (124). To date, most bundles have targeted patients in intensive care settings and the prevention of device-associated infections (124). In neonates, bundles have been used successfully to prevent ventilator-associated pneumonia (VAP) and central line-associated bloodstream infections (CLABSIs) (125).
Adaptation of care bundle methodology, disease, and device targets is needed to ensure broad applicability in LMIC neonatal units. In these settings, programs to prevent device-associated infections (VAP and CLABSI) may be less important than bundles targeting more commonly used devices [e.g., peripheral intravenous cannulas (PIVCs)] and procedures (e.g., noninvasive ventilation). PIVC insertion is the most frequently performed procedure in neonatal units (126, 127). This procedure, which increases the risk of infection, commonly requires multiple insertion attempts before successful cannulation (126, 128, 129), and PIVCs are often replaced due to a short lifespan (129–131). Therefore, the need for a PIVC should be critically evaluated for each neonate and these devices should be removed as soon as feasible. Additionally, measures to prolong functional duration, such as effective dressing and securement, may be suitable targets for interventions.
Individual components of neonatal HAI prevention care bundle in LMICs should be low-cost or highly cost-effective, simple to implement, measure and evaluate, and scalable. Further important considerations are the cultural acceptability of any intervention and the involvement of mothers who perform a substantial amount of in-hospital neonatal care in many resource-limited facilities. Lastly, any neonatal HAI prevention bundle should include interventions targeting multiple mechanisms of sepsis prevention (Figure 1). Possible bundle elements could include the promotion of a clean birthing and neonatal unit environment, CHG body washing, emollient application, PIVC access care, and interventions to improve hand hygiene compliance in neonatal caregivers, among others. The development of HAI prevention bundles and programs suitable for use in LMIC neonatal units should be prioritized as the evidence base supporting these interventions is currently extremely limited (Table 1).
A systematic review describing interventions to reduce hospital-acquired bloodstream infections in neonates in LMIC was recently published, including both single and bundled interventions (132). Overall, there was limited evidence, with no studies from low-income settings, only one from sub-Saharan Africa (98) and just two based in multiple countries (133, 134). Among the reports of studies using a single intervention, the strongest evidence was for sunflower oil emollient, which reduced neonatal bloodstream infections in Egypt and Bangladesh (108, 135). There was limited evidence for KMC in reducing bloodstream infection, of which only one demonstrated an impact on infection (136, 137), in contrast to a previous Cochrane review (13).
Of the nine studies that evaluated bundled interventions (of which seven are LMIC), the strongest evidence was for the prevention of device-associated infection, namely, VAP and CLABSIs. VAP rates were reduced in a multicountry study in 10 NICUs [relative risk (RR) 0.67; 95% confidence interval (CI) 0.50–0.91], after the implementation of a multimodal strategy including hand hygiene, oral antiseptics, ventilator circuit management, and enhanced infection surveillance (133), with similar reductions seen in smaller single-site studies in China and Egypt (138, 139) CLABSIs were also reduced in a four-country study using staff feedback, education, and surveillance (134), and in one single-site study using hand hygiene, CHG skin preparation, and education (140). Encouraging evidence from a single-site study in Zambia evaluated a bundle of training, hand hygiene promotion, text message reminders on IPC practices, enhanced cleaning, and weekly bathing of neonates >1.5 kg with 2% CHG (49, 98). Overall, the bundle significantly reduced neonatal mortality risk by 21% (RR 0.79; 95% CI 0.76–0.83), culture-proven, and clinically suspected bloodstream infection in all but the smallest babies (<1 kg).
Conclusion
To address the high morbidity and mortality of neonatal HAI in LMIC, IPC programs and evidence-based specific HAI prevention interventions should be identified and prioritized by institutions and national ministries of health. For the greatest impact, these interventions should be implemented simultaneously in care bundles or multimodal programs and target multiple mechanisms of sepsis prevention.
Author Contributions
AD drafted the manuscript outline, solicited contributions from each co-author, and compiled the edited first draft of this manuscript. All authors discussed and planned the manuscript content, provided substantive feedback on the first draft and coedited this manuscript, and approved the final version and agree to be accountable for the content of this work.
Funding
AD is supported by a National Institutes of Health Emerging Global Leader Award (NIH K43 TW010682). FF is supported by the Academy of Medical Sciences and by funders of the Starter Grants for Clinical Lecturers scheme and the National Institute for Health Research Great Ormond Street Hospital Biomedical Research Centre.
Conflict of Interest
The authors declare that the research was conducted in the absence of any commercial or financial relationships that could be construed as a potential conflict of interest.
Publisher's Note
All claims expressed in this article are solely those of the authors and do not necessarily represent those of their affiliated organizations, or those of the publisher, the editors and the reviewers. Any product that may be evaluated in this article, or claim that may be made by its manufacturer, is not guaranteed or endorsed by the publisher.
Acknowledgments
The authors acknowledge all the infection prevention staff at their respective institutions whose expertise and experience have contributed to the development of guidelines for the prevention of HAI/AMR.
References
1. Perin J, Mulick A, Yeung D, Villavicencio F, Lopez G, Strong KL, et al. Global, regional, and national causes of under-5 mortality in 2000–19: an updated systematic analysis with implications for the Sustainable Development Goals. Lancet Child Adolesc Health. (2022) 6:106–15. doi: 10.1016/S2352-4642(21)00311-4
2. United Nations Inter-agency Group for Child Mortality Estimation, Levels & Trends in Child Mortality: Report 2021. New York, NY (2021). Available online at: https://data.unicef.org/topic/child-survival/neonatal-mortality/ (accessed 1 February 2021).
3. Seale AC, Blencowe H, Manu AA, Nair H, Bahl R, Qazi SA, et al. Estimates of possible severe bacterial infection in neonates in sub-Saharan Africa, south Asia, and Latin America for 2012: a systematic review and meta-analysis. Lancet Infect Dis. (2014) 14:731–41. doi: 10.1016/S1473-3099(14)70804-7
4. Zaidi AKM, Huskins WC, Thaver D, Bhutta ZA, Abbas Z, Goldmann DA. Hospital-acquired neonatal infections in developing countries. Lancet. (2005) 365:1175–88. doi: 10.1016/S0140-6736(05)71881-X
5. Madhi SA, Pathirana J, Baillie V, Izu A, Bassat Q, Blau DM, et al. Unraveling specific causes of neonatal mortality using minimally invasive tissue sampling: an observational study. Clin Infect Dis. (2019) 69:S351–60. doi: 10.1093/cid/ciz574
6. Chawanpaiboon S, Vogel JP, Moller AB, Lumbiganon P, Petzold M, Hogan D, et al. Global, regional, and national estimates of levels of preterm birth in 2014: a systematic review and modelling analysis. Lancet Glob Health. (2019) 7:e37–46. doi: 10.1016/S2214-109X(18)30451-0
7. Flynn E, Dreibelbis R, Cumming O, Shiras T, Olutunde O, Om'Iniabohs A, et al. Phase I Report: WASH for Neonatal and Maternal Sepsis Reduction Study. (2017), p. 1–17. Available online at: https://www.healthynewbornnetwork.org/resource/phase-report-wash-neonatal-maternal-sepsis-reduction-study/ (accessed February 3, 2022).
8. Bulabula ANH, Dramowski A, Mehtar S. Maternal colonization or infection with extended-spectrum beta-lactamase-producing Enterobacteriaceae in Africa: a systematic review and meta-analysis. Int J Infect Dis. (2017) 64:58–66. doi: 10.1016/j.ijid.2017.08.015
9. Dramowski A, Velaphi S, Reubenson G, Bekker A, Perovic O, Finlayson H, et al. National Neonatal Sepsis Task Force launch: supporting infection prevention and surveillance, outbreak investigation and antimicrobial stewardship in neonatal units in South Africa. S Afr Med J. (2020) 110:360–3. doi: 10.7196/SAMJ.2020.v110i5.14564
10. Jacobs J, Hardy L, Semret M, Lunguya O, Phe T, Affolabi D, et al. Diagnostic bacteriology in district hospitals in Sub-Saharan Africa: at the forefront of the containment of antimicrobial resistance. Front Med. (2019) 6:205. doi: 10.3389/fmed.2019.00205
11. Folgori L, Tersigni C, Hsia Y, Kortsalioudaki C, Heath P, Sharland M, et al. The relationship between Gram-negative colonization and bloodstream infections in neonates: a systematic review and meta-analysis. Clin Microbiol Infect. (2018) 24:251–7. doi: 10.1016/j.cmi.2017.08.008
12. Lawn JE, Mwansa-Kambafwile J, Horta BL, Barros FC, Cousens S. “Kangaroo mother care” to prevent neonatal deaths due to preterm birth complications. Int J Epidemiol. (2010) 39:144–54. doi: 10.1093/ije/dyq031
13. Conde-Agudelo A, Díaz-Rossello JL. Kangaroo mother care to reduce morbidity and mortality in low birthweight infants. Cochrane Database Syst Rev. (2016) CD002771. doi: 10.1002/14651858.CD002771.pub4
14. Charpak N, Montealegre-Pomar A, Bohorquez A. Systematic review and meta-analysis suggest that the duration of Kangaroo mother care has a direct impact on neonatal growth. Acta Paediatr. (2021) 110:45–59. doi: 10.1111/apa.15489
15. Dawod B, Marshall JS, Azad MB. Breastfeeding and the developmental origins of mucosal immunity: how human milk shapes the innate and adaptive mucosal immune systems. Curr Opin Gastroenterol. (2021) 37:547–56. doi: 10.1097/MOG.0000000000000778
16. WHO Immediate KMC Study Group, Arya S, Naburi H, Kawaza K, Newton S, Anyabolu CH, et al. Immediate “kangaroo mother care” and survival of infants with low birth weight. N Engl J Med. (2021) 384:2028–38. doi: 10.1056/NEJMoa2026486
17. Kinshella MLW, Hiwa T, Pickerill K, Vidler M, Dube Q, Goldfarb D, et al. Barriers and facilitators of facility-based kangaroo mother care in sub-Saharan Africa: a systematic review. BMC Pregnancy Childbirth. (2021) 21:176. doi: 10.1186/s12884-021-03646-3
18. Gill VR, Liley HG, Erdei C, Sen S, Davidge R, Wright AL, et al. Improving the uptake of Kangaroo Mother Care in neonatal units: a narrative review and conceptual framework. Acta Paediatr. (2021) 110:1407–16. doi: 10.1111/apa.15705
19. Durack J, Kimes NE, Lin DL, Rauch M, McKean M, McCauley K, et al. Delayed gut microbiota development in high-risk for asthma infants is temporarily modifiable by Lactobacillus supplementation. Nat Commun. (2018) 9:707. doi: 10.1038/s41467-018-03157-4
20. Di Mauro A, Neu J, Riezzo G, Raimondi F, Martinelli D, Francavilla R, et al. Gastrointestinal function development and microbiota. Ital J Pediatr. (2013) 39:15. doi: 10.1186/1824-7288-39-15
21. Deshpande G, Jape G, Rao S, Patole S. Benefits of probiotics in preterm neonates in low-income and medium-income countries: a systematic review of randomised controlled trials. BMJ Open. (2017) 7:e017638. doi: 10.1136/bmjopen-2017-017638
22. Murphy K, Ross RP, Ryan CA, Dempsey EM, Stanton C. Probiotics, prebiotics, and synbiotics for the prevention of necrotizing enterocolitis. Front Nutr. (2021) 8:667188. doi: 10.3389/fnut.2021.667188
23. Gasparrini AJ, Crofts TS, Gibson MK, Tarr PI, Warner BB, Dantas G. Antibiotic perturbation of the preterm infant gut microbiome and resistome. Gut Microbes. (2016) 7:443–9. doi: 10.1080/19490976.2016.1218584
24. Li X, Xu X, Yang X, Luo M, Liu P, Su K, et al. Risk factors for infection and/or colonisation with extended-spectrum β-lactamase-producing bacteria in the neonatal intensive care unit: a meta-analysis. Int J Antimicrob Agents. (2017) 50:622–8. doi: 10.1016/j.ijantimicag.2017.06.027
25. Roberts T, Limmathurotsakul D, Turner P, Day NPJ, Vandepitte WP, Cooper BS. Antimicrobial-resistant Gram-negative colonization in infants from a neonatal intensive care unit in Thailand. J Hosp Infect. (2019) 103:151–5. doi: 10.1016/j.jhin.2019.04.004
26. Turner P, Pol S, Soeng S, Sar P, Neou L, Chea P, et al. High prevalence of antimicrobial-resistant gram-negative colonization in hospitalized Cambodian infants. Pediatr Infect Dis J. (2016) 35:856–61. doi: 10.1097/INF.0000000000001187
27. Araujo da Silva AR, Marques A, Di Biase C, Faitanin M, Murni I, Dramowski A, et al. Effectiveness of antimicrobial stewardship programmes in neonatology: a systematic review. Arch Dis Child. (2020) 105:563–8. doi: 10.1136/archdischild-2019-318026
28. Mukhopadhyay S, Sengupta S, Puopolo KM. Challenges and opportunities for antibiotic stewardship among preterm infants. Arch Dis Child Fetal Neonatal Ed. (2019) 104:F327–32. doi: 10.1136/archdischild-2018-315412
29. Ramasethu J, Kawakita T. Antibiotic stewardship in perinatal and neonatal care. Semin Fetal Neonatal Med. (2017) 22:278–83. doi: 10.1016/j.siny.2017.07.001
30. Klingenberg C, Kornelisse RF, Buonocore G, Maier RF, Stocker M. Culture-negative early-onset neonatal sepsis - at the crossroad between efficient sepsis care and antimicrobial stewardship. Front Pediatr. (2018) 6:285. doi: 10.3389/fped.2018.00285
31. Ombelet S, Barbé B, Affolabi D, Ronat JB, Lompo P, Lunguya O, et al. Best practices of blood cultures in low- and middle-income countries. Front Med. (2019) 6:131. doi: 10.3389/fmed.2019.00131
32. Ombelet S, Ronat JB, Walsh T, Yansouni CP, Cox J, Vlieghe E, et al. Clinical bacteriology in low-resource settings: today's solutions. Lancet Infect Dis. (2018) 18:e248–58. doi: 10.1016/S1473-3099(18)30093-8
33. Antimicrobial Resistance Collaborators. Global burden of bacterial antimicrobial resistance in 2019: a systematic analysis. Lancet. (2022) 399:629–55. doi: 10.1016/S0140-6736(21)02724-0
34. Dramowski A, Aucamp M, Bekker A, Mehtar S. Infectious disease exposures and outbreaks at a South African neonatal unit with review of neonatal outbreak epidemiology in Africa. Int J Infect Dis. (2017) 57:79–85. doi: 10.1016/j.ijid.2017.01.026
35. Cutland CL, Madhi SA, Zell ER, Kuwanda L, Laque M, Groome M, et al. Chlorhexidine maternal-vaginal and neonate body wipes in sepsis and vertical transmission of pathogenic bacteria in South Africa: a randomised, controlled trial. Lancet. (2009) 374:1909–16. doi: 10.1016/S0140-6736(09)61339-8
36. Pereira L, Chipato T, Mashu A, Mushangwe V, Rusakaniko S, Bangdiwala SI, et al. Randomized study of vaginal and neonatal cleansing with 1% chlorhexidine. Int J Gynaecol Obstet. (2011) 112:234–8. doi: 10.1016/j.ijgo.2010.09.009
37. Strategies to reduce vertical transmission of multi-drug resistant pathogens to neonates (NeoVT-AMR) [Internet]. UK Research and Inovation. Available online at: https://gtr.ukri.org/projects?ref=MR%2FT039035%2F1 (accessed February 3, 2022).
38. Lassi ZS, Fisher Z, Andraweera P, Cummins A, Roberts CT. Effectiveness of birthing kits for clean childbirth: a systematic review. Int Health. (2020) 12:3–10. doi: 10.1093/inthealth/ihz022
39. Shao Y, Forster SC, Tsaliki E, Vervier K, Strang A, Simpson N, et al. Stunted microbiota and opportunistic pathogen colonization in caesarean-section birth. Nature. (2019) 574:117–21. doi: 10.1038/s41586-019-1560-1
40. Seedat F, Stinton C, Patterson J, Geppert J, Tan B, Robinson ER, et al. Adverse events in women and children who have received intrapartum antibiotic prophylaxis treatment: a systematic review. BMC Pregnancy Childbirth. (2017) 17:247. doi: 10.1186/s12884-017-1432-3
41. Akinboyo IC, Zangwill KM, Berg WM, Cantey JB, Huizinga B, Milstone AM, et al. neonatal intensive care unit (NICU) white paper series: practical approaches to Staphylococcus aureus disease prevention. Infect Control Hosp Epidemiol. (2020) 41:1251–7. doi: 10.1017/ice.2020.51
42. Nelson MU, Shaw J, Gross SJ. Randomized placebo-controlled trial of topical mupirocin to reduce Staphylococcus aureus colonization in infants in the neonatal intensive care unit. J Pediatr. (2021) 236:70–7. doi: 10.1016/j.jpeds.2021.05.042
43. Bozzella MJ, Soghier L, Harris T, Zell L, Short BL, Song X. Impact of decolonization on methicillin-resistant Staphylococcus aureus transmission and infection in a neonatal intensive care unit. Infect Control Hosp Epidemiol. (2019) 40:1123–7. doi: 10.1017/ice.2019.217
44. Bharadwaj S, Ho SK, Khong KC, Seet A, Yeo KC, Chan XY, et al. Eliminating MRSA transmission in a tertiary neonatal unit-A quality improvement initiative. Am J Infect Control. (2019) 47:1329–35. doi: 10.1016/j.ajic.2019.06.001
45. Mullany LC, Saha SK, Shah R, Islam MS, Rahman M, Islam M, et al. Impact of 40% chlorhexidine cord cleansing on the bacteriologic profile of the newborn umbilical stump in rural Sylhet District, Bangladesh: a community-based, cluster-randomized trial. Pediatr Infect Dis J. (2012) 31:444–50. doi: 10.1097/INF.0b013e3182468ff0
46. Darmstadt GL, Hossain MM, Choi Y, Shirin M, Mullany LC, Islam M, et al. Safety and effect of chlorhexidine skin cleansing on skin flora of neonates in Bangladesh. Pediatr Infect Dis J. (2007) 26:492–5. doi: 10.1097/01.inf.0000261927.90189.88
47. Dramowski A, Pillay S, Bekker A, Abrahams I, Cotton MF, Coffin SE, et al. Impact of 1% chlorhexidine gluconate bathing and emollient application on bacterial pathogen colonization dynamics in hospitalized preterm neonates - a pilot clinical trial. EClinicalMedicine. (2021) 37:100946. doi: 10.1016/j.eclinm.2021.100946
48. Zhou J, Mei L, Chen S. Effect of chlorhexidine cleansing on healthcare-associated infections in neonates: a systematic review and meta-analysis. Arch Dis Child Fetal Neonatal Ed. (2022) 107:398–407. doi: 10.1136/archdischild-2021-322429
49. Westling T, Cowden C, Mwananyanda L, Kapasa ML, Machona S, Pierre C, et al. Impact of chlorhexidine baths on suspected sepsis and bloodstream infections in hospitalized neonates in Zambia. Int J Infect Dis. (2020) 96:54–60. doi: 10.1016/j.ijid.2020.03.043
50. Silago V, Kovacs D, Msanga DR, Seni J, Matthews L, Oravcová K, et al. Bacteremia in critical care units at Bugando Medical Centre, Mwanza, Tanzania: the role of colonization and contaminated cots and mothers' hands in cross-transmission of multidrug resistant Gram-negative bacteria. Antimicrob Resist Infect Control. (2020) 9:58. doi: 10.1186/s13756-020-00721-w
51. Pires D, de Kraker MEA, Tartari E, Abbas M, Pittet D. ‘Fight antibiotic resistance—it's in your hands': call from the World Health Organization for 5th May 2017. Clin Infect Dis. (2017) 64:1780–3. doi: 10.1093/cid/cix226
52. WHO. WHO Guidelines on Hand Hygiene in Health Care. Geneva: World Health Organisation (2009). Available online at: https://www.who.int/publications-detail-redirect/9789241597906 (accessed February 2, 2022).
53. Allegranzi B, Gayet-Ageron A, Damani N, Bengaly L, McLaws ML, Moro ML, et al. Global implementation of WHO's multimodal strategy for improvement of hand hygiene: a quasi-experimental study. Lancet Infect Dis. (2013) 13:843–51. doi: 10.1016/S1473-3099(13)70163-4
54. Sax H, Allegranzi B, Uçkay I, Larson E, Boyce J, Pittet D. “My five moments for hand hygiene”: a user-centred design approach to understand, train, monitor and report hand hygiene. J Hosp Infect. (2007) 67:9–21. doi: 10.1016/j.jhin.2007.06.004
55. Horng LM, Unicomb L, Alam MU, Halder AK, Shoab AK, Ghosh PK, et al. Healthcare worker and family caregiver hand hygiene in Bangladeshi healthcare facilities: results from the Bangladesh National Hygiene Baseline Survey. J Hosp Infect. (2016) 94:286–94. doi: 10.1016/j.jhin.2016.08.016
56. Sands K, Carvalho MJ, Portal E, Thomson K, Dyer C, Akpulu C, et al. Characterization of antimicrobial-resistant Gram-negative bacteria that cause neonatal sepsis in seven low- and middle-income countries. Nat Microbiol. (2021) 6:512–23. doi: 10.1038/s41564-021-00870-7
57. Agarwal R, Sankar J. Characterisation and antimicrobial resistance of sepsis pathogens in neonates born in tertiary care centres in Delhi, India: a cohort study. Lancet Glob Health. (2016) 4:752–60. doi: 10.1016/S2214-109X(16)30148-6
58. Labi AK, Enweronu-Laryea CC, Nartey ET, Bjerrum S, Ayibor PK, Andersen LP, et al. Bloodstream infections at two neonatal intensive care units in Ghana: multidrug resistant enterobacterales undermine the usefulness of standard antibiotic regimes. Pediatr Infect Dis J. (2021) 40:1115–21. doi: 10.1097/INF.0000000000003284
59. Dramowski A, Madide A, Bekker A. Neonatal nosocomial bloodstream infections at a referral hospital in a middle-income country: burden, pathogens, antimicrobial resistance and mortality. Paediatr Int Child Health. (2015) 35:265–72. doi: 10.1179/2046905515Y.0000000029
60. Kagia N, Kosgei P, Ooko M, Wafula L, Mturi N, Anampiu K, et al. Carriage and acquisition of extended-spectrum β-lactamase-producing enterobacterales among neonates admitted to Hospital in Kilifi, Kenya. Clin Infect Dis. (2019) 69:751–9. doi: 10.1093/cid/ciy976
61. Labi AK, Bjerrum S, Enweronu-Laryea CC, Ayibor PK, Nielsen KL, Marvig RL, et al. High carriage rates of multidrug-resistant gram-negative bacteria in neonatal intensive care units from Ghana. Open Forum Infect Dis. (2020) 7:ofaa109. doi: 10.1093/ofid/ofaa109
62. Tacconelli E, Cataldo MA, Dancer SJ, De Angelis G, Falcone M, Frank U, et al. ESCMID guidelines for the management of the infection control measures to reduce transmission of multidrug-resistant Gram-negative bacteria in hospitalized patients. Clin Microbiol Infect. (2014) 20:1–55. doi: 10.1111/1469-0691.12427
63. Von Dolinger de Brito D, de Almeida Silva H, Jose Oliveira E, Arantes A, Abdallah VOS, Tannus Jorge M, et al. Effect of neonatal intensive care unit environment on the incidence of hospital-acquired infection in neonates. J Hosp Infect. (2007) 65:314–8. doi: 10.1016/j.jhin.2006.01.038
64. López S, Wong Y, Urbina L, Gómez I, Escobar F, Tinoco B, et al. Quality in practice: preventing and managing neonatal sepsis in Nicaragua. Int J Qual Health Care. (2013) 25:599–605. doi: 10.1093/intqhc/mzt060
65. Nakstad B, Kaang T, Gezmu AM, Strysko J. Nosocomial SARS-CoV-2 transmission in a neonatal unit in Botswana: chronic overcrowding meets a novel pathogen. BMJ Case Rep. (2021) 14:e242421. doi: 10.1136/bcr-2021-242421
66. Domanico R, Davis DK, Coleman F, Davis BO. Documenting the NICU design dilemma: comparative patient progress in open-ward and single family room units. J Perinatol. (2011) 31:281–8. doi: 10.1038/jp.2010.120
67. Lester BM, Hawes K, Abar B, Sullivan M, Miller R, Bigsby R, et al. Single-family room care and neurobehavioral and medical outcomes in preterm infants. Pediatrics. (2014) 134:754–60. doi: 10.1542/peds.2013-4252
68. Benenson S, Levin PD, Block C, Adler A, Ergaz Z, Peleg O, et al. Continuous surveillance to reduce extended-spectrum β-lactamase Klebsiella pneumoniae colonization in the neonatal intensive care unit. Neonatology. (2013) 103:155–60. doi: 10.1159/000343150
69. Kaushik A, Kest H, Zauk A, DeBari VA, Lamacchia M. Impact of routine methicillin-resistant Staphylococcus aureus (MRSA) surveillance and cohorting on MRSA-related bloodstream infection in neonatal intensive care unit. Am J Perinatol. (2015) 32:531–6. doi: 10.1055/s-0034-1395481
70. Dramowski A, Aucamp M, Bekker A, Pillay S, Moloto K, Whitelaw AC, et al. NeoCLEAN: a multimodal strategy to enhance environmental cleaning in a resource-limited neonatal unit. Antimicrob Resist Infect Control. (2021) 10:35. doi: 10.1186/s13756-021-00905-y
71. De Geyter D, Blommaert L, Verbraeken N, Sevenois M, Huyghens L, Martini H, et al. The sink as a potential source of transmission of carbapenemase-producing Enterobacteriaceae in the intensive care unit. Antimicrob Resist Infect Control. (2017) 6:24. doi: 10.1186/s13756-017-0182-3
72. Roux D, Aubier B, Cochard H, Quentin R, van der Mee-Marquet N, HAI Prevention Group of the Réseau des Hygiénistes du Centre. Contaminated sinks in intensive care units: an underestimated source of extended-spectrum beta-lactamase-producing Enterobacteriaceae in the patient environment. J Hosp Infect. (2013) 85:106–11. doi: 10.1016/j.jhin.2013.07.006
73. Lowe C, Willey B, O'Shaughnessy A, Lee W, Lum M, Pike K, et al. Outbreak of extended-spectrum β-lactamase–producing Klebsiella oxytoca Infections associated with contaminated handwashing Sinks1. Emerg Infect Dis. (2012) 18:1242–7. doi: 10.3201/eid1808.111268
74. Ram PK, Begum F, Crabtree-Ide C, Uddin MR, Weaver AM, Dostogir Harun MG, et al. Waterless hand cleansing with chlorhexidine during the neonatal period by mothers and other household members: findings from a randomized controlled trial. Am J Trop Med Hyg. (2020) 103:2116–26. doi: 10.4269/ajtmh.19-0773
75. Hopman J, Tostmann A, Wertheim H, Bos M, Kolwijck E, Akkermans R, et al. Reduced rate of intensive care unit acquired gram-negative bacilli after removal of sinks and introduction of ‘water-free' patient care. Antimicrob Resist Infect Control. (2017) 6:59. doi: 10.1186/s13756-017-0213-0
76. Tracy M, Ryan L, Samarasekara H, Leroi M, Polkinghorne A, Branley J. Removal of sinks and bathing changes to control multidrug-resistant gram-negative bacteria in a neonatal intensive care unit: a retrospective investigation. J Hosp Infect. (2020) 104:508–10. doi: 10.1016/j.jhin.2020.01.014
77. Smolders D, Hendriks B, Rogiers P, Mul M, Gordts B. Acetic acid as a decontamination method for ICU sink drains colonized by carbapenemase-producing Enterobacteriaceae and its effect on CPE infections. J Hosp Infect. (2019) 102:82–8. doi: 10.1016/j.jhin.2018.12.009
78. Stjärne Aspelund A, Sjöström K, Olsson Liljequist B, Mörgelin M, Melander E, Påhlman LI. Acetic acid as a decontamination method for sink drains in a nosocomial outbreak of metallo-β-lactamase-producing Pseudomonas aeruginosa. J Hosp Infect. (2016) 94:13–20. doi: 10.1016/j.jhin.2016.05.009
79. El-sayed R, Abu-Salem L, Degla H. Training nurses about applying disinfection technique for safe reuse of disposable suction catheters for neonates on mechanical ventilation. MNJ. (2014) 1:1–10. doi: 10.21608/mnj.2014.148985
80. CDC and ICAN. Best Practices for Environmental Cleaning in Healthcare Facilities in Resource-Limited Settings. Atlanta, GA: US Department of Health and Human Services, CDC. Cape Town: Infection Control Africa Network (2019). Available online at: https://www.cdc.gov/hai/prevent/resource-limited/index.html and http://www.icanetwork.co.za/icanguideline2019/ (accessed February 2, 2022).
81. Gon G, Kabanywanyi AM, Blinkhoff P, Cousens S, Dancer SJ, Graham WJ, et al. The Clean pilot study: evaluation of an environmental hygiene intervention bundle in three Tanzanian hospitals. Antimicrob Resist Infect Control. (2021) 10:8. doi: 10.1186/s13756-020-00866-8
82. Dramowski A, Whitelaw A, Cotton MF. Assessment of terminal cleaning in pediatric isolation rooms: options for low-resource settings. Am J Infect Control. (2016) 44:1558–64. doi: 10.1016/j.ajic.2016.05.026
83. Ku E, Lee G, Jeon M, Choi J, Lee Y. Microbial contamination of reusable suction container and cost analysis of reusable suction container and disposable suction container. J Korean Biol Nurs Sci. (2019) 21:133–40. doi: 10.7586/jkbns.2019.21.2.133
84. Pillay T, Pillay DG, Adhikari M, Pillay A, Sturm AW. An outbreak of neonatal infection with Acinetobacter linked to contaminated suction catheters. J Hosp Infect. (1999) 43:299–304. doi: 10.1016/S0195-6701(99)90426-7
85. Vurayai M, Strysko J, Kgomanyane K, Bayani O, Mokomane M, Machiya T, et al. Characterizing the bioburden of ESBL-producing organisms in a neonatal unit using chromogenic culture media: a feasible and efficient environmental sampling method. Antimicrob Resist Infect Control. (2022) 11:14. doi: 10.1186/s13756-021-01042-2
86. Rahal A, Andreo A, Le Gallou F, Bourigault C, Bouchand C, Ferriot C, et al. Enterobacter cloacae complex outbreak in a neonatal intensive care unit: multifaceted investigations and preventive measures are needed. J Hosp Infect. (2021) 116:87–90. doi: 10.1016/j.jhin.2021.07.012
87. Rettedal S, Löhr IH, Natås O, Giske CG, Sundsfjord A, Øymar K. First outbreak of extended-spectrum β-lactamase-producing Klebsiella pneumoniae in a Norwegian neonatal intensive care unit; associated with contaminated breast milk and resolved by strict cohorting. APMIS. (2012) 120:612–21. doi: 10.1111/j.1600-0463.2012.02879.x
88. Rivero P, Brenner P, Nercelles P. [Impact of copper in the reduction of hospital-acquired infections, mortality and antimicrobial costs in the Adult Intensive Care Unit]. Rev Chilena Infectol. (2014) 31:274–9. doi: 10.4067/S0716-10182014000300004
89. Chapman AK, Aucott SW, Milstone AM. Safety of chlorhexidine gluconate used for skin antisepsis in the preterm infant. J Perinatol. (2012) 32:4–9. doi: 10.1038/jp.2011.148
90. Lashkari HP, Chow P, Godambe S. Aqueous 2% chlorhexidine-induced chemical burns in an extremely premature infant. Arch Dis Child Fetal Neonatal Ed. (2012) 97:F64. doi: 10.1136/adc.2011.215145
91. Sathiyamurthy S, Banerjee J, Godambe SV. Antiseptic use in the neonatal intensive care unit - a dilemma in clinical practice: an evidence based review. World J Clin Pediatr. (2016) 5:159–71. doi: 10.5409/wjcp.v5.i2.159
92. Sethi DK, Felgate H, Diaz M, Faust K, Kiy C, Clarke P, et al. Chlorhexidine gluconate usage is associated with antiseptic tolerance in staphylococci from the neonatal intensive care unit. JAC Antimicrob Resist. (2021) 3:dlab173. doi: 10.1093/jacamr/dlab173
93. Janssen LMA, Tostmann A, Hopman J. Liem KD. 02% chlorhexidine acetate as skin disinfectant prevents skin lesions in extremely preterm infants: a preliminary report. Arch Dis Child Fetal Neonatal Ed. (2018) 103:F97–100. doi: 10.1136/archdischild-2017-312694
94. Tamma PD, Aucott SW, Milstone AM. Chlorhexidine use in the neonatal intensive care unit: results from a national survey. Infect Control Hosp Epidemiol. (2010) 31:846–9. doi: 10.1086/655017
95. Clarke P, Craig JV, Wain J, Tremlett C, Linsell L, Bowler U, et al. Safety and efficacy of 2% chlorhexidine gluconate aqueous versus 2% chlorhexidine gluconate in 70% isopropyl alcohol for skin disinfection prior to percutaneous central venous catheter insertion in preterm neonates: the ARCTIC randomised-controlled feasibility trial protocol. BMJ Open. (2019) 9:e028022. doi: 10.1136/bmjopen-2018-028022
96. Kieran EA, O'Sullivan A, Miletin J, Twomey AR, Knowles SJ, O'Donnell CPF. 2% chlorhexidine-70% isopropyl alcohol versus 10% povidone-iodine for insertion site cleaning before central line insertion in preterm infants: a randomised trial. Arch Dis Child Fetal Neonatal Ed. (2018) 103:F101–6. doi: 10.1136/archdischild-2016-312193
97. Sinha A, Sazawal S, Pradhan A, Ramji S, Opiyo N. Chlorhexidine skin or cord care for prevention of mortality and infections in neonates. Cochrane Database Syst Rev. (2015) CD007835. doi: 10.1002/14651858.CD007835.pub2
98. Mwananyanda L, Pierre C, Mwansa J, Cowden C, Localio AR, Kapasa ML, et al. Preventing bloodstream infections and death in Zambian neonates: impact of a low-cost infection control bundle. Clin Infect Dis. (2019) 69:1360–7. doi: 10.1093/cid/ciy1114
99. Johnson E, Hunt R. Infant skin care: updates and recommendations. Curr Opin Pediatr. (2019) 31:476–81. doi: 10.1097/MOP.0000000000000791
100. Kusari A, Han AM, Virgen CA, Matiz C, Rasmussen M, Friedlander SF, et al. Evidence-based skin care in preterm infants. Pediatr Dermatol. (2019) 36:16–23. doi: 10.1111/pde.13725
101. Burdall O, Willgress L, Goad N. Neonatal skin care: developments in care to maintain neonatal barrier function and prevention of diaper dermatitis. Pediatr Dermatol. (2019) 36:31–5. doi: 10.1111/pde.13714
102. Strunk T, Pupala S, Hibbert J, Doherty D, Patole S. Topical coconut oil in very preterm infants: an open-label randomised controlled trial. Neonatology. (2018) 113:146–51. doi: 10.1159/000480538
103. Visscher M, Odio M, Taylor T, White T, Sargent S, Sluder L, et al. Skin care in the NICU patient: effects of wipes versus cloth and water on stratum corneum integrity. Neonatology. (2009) 96:226–34. doi: 10.1159/000215593
104. Summers A, Visscher MO, Khatry SK, Sherchand JB, LeClerq SC, Katz J, et al. Impact of sunflower seed oil versus mustard seed oil on skin barrier function in newborns: a community-based, cluster-randomized trial. BMC Pediatr. (2019) 19:512. doi: 10.1186/s12887-019-1871-2
105. Danby SG, Alenezi T, Sultan A, Lavender T, Chittock J, Brown K, et al. Effect of olive and sunflower seed oil on the adult skin barrier: implications for neonatal skin care. Pediatr Dermatol. (2013) 30:42–50. doi: 10.1111/j.1525-1470.2012.01865.x
106. Kumar A, Mishra S, Singh S, Ashraf S, Kan P, Ghosh AK, et al. Effect of sunflower seed oil emollient therapy on newborn infant survival in Uttar Pradesh, India: a community-based, cluster randomized, open-label controlled trial. PLoS Med. (2021) 18:e1003680. doi: 10.1371/journal.pmed.1003680
107. Schürer N, Schliep V, Williams ML. Differential utilization of linoleic and arachidonic acid by cultured human keratinocytes. Skin Pharmacol. (1995) 8:30–40. doi: 10.1159/000211328
108. M Man MQ, Feingold KR, Thornfeldt CR, Elias PM. Optimization of physiological lipid mixtures for barrier repair. J Invest Dermatol. (1996) 106:1096–101. doi: 10.1111/1523-1747.ep12340135
109. Darmstadt GL, Badrawi N, Law PA, Ahmed S, Bashir M, Iskander I, et al. Topically applied sunflower seed oil prevents invasive bacterial infections in preterm infants in Egypt: a randomized, controlled clinical trial. Pediatr Infect Dis J. (2004) 23:719–25. doi: 10.1097/01.inf.0000133047.50836.6f
110. Cleminson J, McGuire W. Topical emollient for preventing infection in preterm infants. Cochrane Database Syst Rev. (2021) 5:CD001150. doi: 10.1002/14651858.CD001150.pub4
111. Cotton MF, Sharland M. Antimicrobial stewardship and infection prevention and control in low- and middle-income countries: current status and best practices. Pediatr Infect Dis J. (2022) 41(3S):S1–2. doi: 10.1097/INF.0000000000003355
112. Pittet D. Infection control and quality health care in the new millennium. Am J Infect Control. (2005) 33:258–67. doi: 10.1016/j.ajic.2004.11.004
113. Gray JW. Surveillance of infection in neonatal intensive care units. Early Hum Dev. (2007) 83:157–63. doi: 10.1016/j.earlhumdev.2007.01.006
114. World Health Organization. Report on the burden of endemic health care-associated infection worldwide. Geneva: World Health Organization (2011), p. 40. Available online at: https://apps.who.int/iris/handle/10665/80135 (accessed April 11, 2022).
115. Cailes B, Vergnano S, Kortsalioudaki C, Heath P, Sharland M. The current and future roles of neonatal infection surveillance programmes in combating antimicrobial resistance. Early Hum Dev. (2015) 91:613–8. doi: 10.1016/j.earlhumdev.2015.08.012
116. Dramowski A, Cotton MF, Whitelaw A. Surveillance of healthcare-associated infection in Hospitalised South African children: which method performs best? S Afr Med J. (2016) 107:56–63. doi: 10.7196/SAMJ.2016.v107.i1.11431
117. Thu TA, Hung NV, Quang NN, Archibald LK, Thuy LTT, Harun-Or-Rashid null, et al. A point-prevalence study on healthcare-associated infections in Vietnam: public health implications. Infect Control Hosp Epidemiol. (2011) 32:1039–41. doi: 10.1086/661915
118. Olivier C, Kunneke H, O'Connell N, von Delft E, Wates M, Dramowski A. Healthcare-associated infections in paediatric and neonatal wards: a point prevalence survey at four South African hospitals. SAMJ. (2018) 108:418–22. doi: 10.7196/SAMJ.2018.v108i5.12862
119. Johnson J, Akinboyo IC, Curless MS, Milstone AM, Coffin SE. Saving neonatal lives by improving infection prevention in low-resource units: tools are needed. J Glob Health. (2019) 9:010319. doi: 10.7189/jogh.09.010319
120. World Health Organization. Guide to Implementation: A Guide to the Implementation of the WHO Multimodal Hand Hygiene Improvement Strategy. Geneva: WHO (2009). Available online at: https://www.who.int/gpsc/ 5may/Guide_to_Implementation.pdf (accessed January 2, 2022).
121. World Health Organization. Guidelines on Core Components of Infection Prevention and Control Programmes at the National and Acute Health Care Facility Level. Geneva: WHO (2016). Available online at: https://www.who.int/teams/integrated-health-services/infection-prevention-control/core-components (accessed December 30, 2021).
122. Safdar N, Abad C. Educational interventions for prevention of healthcare-associated infection: a systematic review. Crit Care Med. (2008) 36:933–40. doi: 10.1097/CCM.0B013E318165FAF3
123. Marwick C, Davey P. Care bundles: the holy grail of infectious risk management in hospital? Curr Opin Infect Dis. (2009) 22:364–9. doi: 10.1097/QCO.0b013e32832e0736
124. The national quality measures clearinghouse (NQMCH): measure validity. (2008). Available online at: https://www.qualitymeasures.ahrq.gov/help-and-about/summaries/domain-framework-and-inclusioncriteria#criteria (accessed April 11, 2022).
125. Smulders CA, van Gestel JPJ, Bos AP. Are central line bundles and ventilator bundles effective in critically ill neonates and children? Intensive Care Med. (2013) 39:1352–8. doi: 10.1007/s00134-013-2927-7
126. Legemaat M, Carr PJ, van Rens RM, van Dijk M, Poslawsky IE, van den Hoogen A. Peripheral intravenous cannulation: complication rates in the neonatal population: a multicenter observational study. J Vasc Access. (2016) 17:360–5. doi: 10.5301/jva.5000558
127. Chenoweth KB, Guo JW, Chan B. The extended dwell peripheral intravenous catheter is an alternative method of NICU intravenous access. Adv Neonatal Care. (2018) 18:295–301. doi: 10.1097/ANC.0000000000000515
128. Monasor-Ortolá D, Cortés-Castell E, Martínez-Pascual C, Esteve-Ríos A, Rizo-Baeza MM. Factors influencing the success of peripheral venous access in neonates. J Pediatr Nurs. (2019) 47:e30–5. doi: 10.1016/j.pedn.2019.04.017
129. Gupta P, Rai R, Basu S, Faridi MMA. Life span of peripheral intravenous cannula in a neonatal intensive care unit of a developing country. J Pediatr Nurs. (2003) 18:287–92. doi: 10.1016/S0882-5963(03)00052-6
130. Atay S, Sen S, Cukurlu D. Incidence of infiltration/extravasation in newborns using peripheral venous catheter and affecting factors. Rev Esc Enferm USP. (2018) 52:e03360. doi: 10.1590/s1980-220x2017040103360
131. Perlman SE, Saiman L, Larson EL. Risk factors for late-onset health care-associated bloodstream infections in patients in neonatal intensive care units. Am J Infect Control. (2007) 35:177–82. doi: 10.1016/j.ajic.2006.01.002
132. Fitzgerald FC, Zingg W, Chimhini G, Chimhuya S, Wittmann S, Brotherton H, et al. Interventions to prevent neonatal healthcare-associated infections in low-resource settings: a systematic review. medRxiv. (2020). doi: 10.1101/2020.12.17.20248047
133. Rosenthal VD, Rodríguez-Calderón ME, Rodríguez-Ferrer M, Singhal T, Pawar M, Sobreyra-Oropeza M, et al. Findings of the International Nosocomial Infection Control Consortium (INICC), Part II: impact of a multidimensional strategy to reduce ventilator-associated pneumonia in neonatal intensive care units in 10 developing countries. Infect Control Hosp Epidemiol. (2012) 33:704–10. doi: 10.1086/666342
134. Rosenthal VD, Dueñas L, Sobreyra-Oropeza M, Ammar K, Navoa-Ng JA, de Casares ACB, et al. Findings of the International Nosocomial Infection Control Consortium (INICC), part III: effectiveness of a multidimensional infection control approach to reduce central line-associated bloodstream infections in the neonatal intensive care units of 4 developing countries. Infect Control Hosp Epidemiol. (2013) 34:229–37. doi: 10.1086/669511
135. Darmstadt GL, Saha SK, Ahmed ASMNU, Chowdhury MAKA, Law PA, Ahmed S, et al. Effect of topical treatment with skin barrier-enhancing emollients on nosocomial infections in preterm infants in Bangladesh: a randomised controlled trial. Lancet. (2005) 365:1039–45. doi: 10.1016/S0140-6736(05)71140-5
136. Boo NY, Jamli FM. Short duration of skin-to-skin contact: effects on growth and breastfeeding. J Paediatr Child Health. (2007) 43:831–6. doi: 10.1111/j.1440-1754.2007.01198.x
137. Charpak N, Ruiz-Peláez JG, Z Figueroa de C, Charpak Y. Kangaroo mother versus traditional care for newborn infants < /=2000 grams: a randomized, controlled trial. Pediatrics. (1997) 100:682–8. doi: 10.1542/peds.100.4.682
138. Zhou Q, Lee SK, Jiang Si-yuan, Chen C, Kamaluddeen M, Hu X-jing, et al. Efficacy of an infection control program in reducing ventilator-associated pneumonia in a Chinese neonatal intensive care unit. Am J Infect Control. (2013) 41:1059–64. doi: 10.1016/j.ajic.2013.06.007
139. Azab SFA, Sherbiny HS, Saleh SH, Elsaeed WF, Elshafiey MM, Siam AG, et al. Reducing ventilator-associated pneumonia in neonatal intensive care unit using “VAP prevention Bundle”: a cohort study. BMC Infect Dis. (2015) 15:314. doi: 10.1186/s12879-015-1062-1
140. Resende D. Ó J, Brito D, Abdallah V, Filho P. Reduction of catheter-associated bloodstream infections through procedures in newborn babies admitted in a university hospital intensive care unit in Brazil. Rev Soc Bras Med Trop. (2011) 44:731–4. doi: 10.1590/S0037-86822011000600015
141. Urzúa S, Ferrés M, García P, Sánchez A, Luco M. Strategies to reduce infections and antibiotic use and its effects in a neonatal care unit. Rev Chilena Infectol. (2017) 34:99–107. doi: 10.4067/S0716-10182017000200001
142. Johnson J, Latif A, Randive B, Kadam A, Rajput U, Kinikar A, et al. Implementation of the comprehensive unit-based safety program to improve infection prevention and control practices in four neonatal intensive care units in Pune, India. Front Pediatr. (2022) 9:794637. doi: 10.3389/fped.2021.794637
Keywords: neonate, healthcare-associated infection, antimicrobial resistance, resource-limited, care bundles
Citation: Dramowski A, Aucamp M, Beales E, Bekker A, Cotton MF, Fitzgerald FC, Labi A-K, Russell N, Strysko J, Whitelaw A and Coffin S (2022) Healthcare-Associated Infection Prevention Interventions for Neonates in Resource-Limited Settings. Front. Pediatr. 10:919403. doi: 10.3389/fped.2022.919403
Received: 13 April 2022; Accepted: 02 June 2022;
Published: 07 July 2022.
Edited by:
Sadia Shakoor, Aga Khan University, PakistanReviewed by:
Ioannis Kopsidas, Center for Clinical Epidemiology and Outcomes Research (CLEO), GreeceTisungane Mvalo, University of North Carolina at Chapel Hill, United States
Copyright © 2022 Dramowski, Aucamp, Beales, Bekker, Cotton, Fitzgerald, Labi, Russell, Strysko, Whitelaw and Coffin. This is an open-access article distributed under the terms of the Creative Commons Attribution License (CC BY). The use, distribution or reproduction in other forums is permitted, provided the original author(s) and the copyright owner(s) are credited and that the original publication in this journal is cited, in accordance with accepted academic practice. No use, distribution or reproduction is permitted which does not comply with these terms.
*Correspondence: Angela Dramowski, ZHJhbW93c2tpJiN4MDAwNDA7c3VuLmFjLnph