- 1Department of Ultrasound Medicine, Nanjing Lishui People’s Hospital, Zhongda Hospital Lishui Branch, Southeast University, Nanjing, China
- 2Department of Neonatology, Nanjing Lishui People’s Hospital, Zhongda Hospital Lishui Branch, Southeast University, Nanjing, China
- 3School of Medical Sciences, University of Sydney, Sydney, New South Wales, Australia
- 4School of Life Sciences, Huaiyin Normal University, Huai’an, China
- 5Department of Dermatology, Nanjing Lishui People’s Hospital, Zhongda Hospital Lishui Branch, Southeast University, Nanjing, China
Lung disease is often life-threatening for both preterm and term newborns. Therefore, an accurate and rapid diagnosis of lung diseases in newborns is crucial, as management strategies differ with different etiologies. To reduce the risk of radiation exposure derived from the conventionally used chest x-ray as well as computed tomography scans, lung ultrasonography (LUS) has been introduced in clinical practice to identify and differentiate neonatal lung diseases because of its radiation-free characteristic, convenience, high accuracy, and low cost. In recent years, it has been proved that LUS exhibits high sensitivity and specificity for identifying various neonatal lung diseases. Here, we offer an updated review of the applications of LUS in neonatal lung diseases based on the reports published in recent years (2017 to present).
Introduction
Caused by various factors, lung disease is a common condition that induces neonatal respiratory distress (1). Neonatal respiratory failure, which results from severe respiratory distress, accounts for a high proportion of neonatal deaths all over the world (2, 3). Therefore, clinicians, especially neonatologists, need to pay more attention to the identification and treatment of neonatal lung disease. Chest x-ray (CXR) and computed tomography (CT) scans are still conventionally used for diagnosing neonatal lung diseases. However, serious concern has been raised about the safety of various radiologic imaging examinations performed in diagnostic procedures, as they may lead to an increased incidence of malignancies (4, 5). In the neonatal intensive care unit (NICU), the most premature neonates are found to receive the highest radiation dose from diagnostic imaging procedures (6). The lack of a standard neonatal x-ray imaging practice at a global level poses a public health risk to neonates (7). Neonates and infants are highly susceptible to radiation toxicity. Therefore, radiation exposure during the neonatal period should be monitored, and alternative approaches without the risk of radiation exposure are needed in the NICU (8, 9). In addition, radiologic examination has various technical limitations and sometimes yields low accuracy (10).
As a powerful diagnostic and monitoring technique without ionizing radiation, ultrasonography has long been applied either for a superficial examination or for a study of deeply seated organs or systems. Traditionally, ultrasonography was not used to examine the lung because of the presence of lung air spaces in which ultrasonic waves are highly dissipated. However, this practice has now been broken, as lung ultrasonography (LUS) has been increasingly introduced in clinical practice since its first application in adult medicine in 1995 (11). As LUS is an accurate, reliable, quick, easy-to-use, real-time, low-cost, and radiation-free imaging modality, reports on its application in neonatology have notably increased during the last decade (12, 13). A 3-year clinical practice report published in 2020 indicated that CXR could be completely replaced by LUS in the NICU (14). Here, we aim to offer an updated review of recent advances and applications of LUS in neonatology and provide a better understanding of neonatal LUS.
Examination technology and method
In order to visualize the neonatal lung more effectively, a high-frequency (≥10 MHz) linear array transducer is suggested. For newborns bearing low gestational age (GA) and/or small birth weight, a higher-frequency probe can be applied. Under a quiet state, LUS examination can be performed in supine, prone, and side position for neonates. Based on the above positions, each hemithorax can be separated into three regions (Figure 1), namely the anterior (A) region, lateral (L) region, and posterior (L) region, by using the anterior and posterior axillary line. With respect to each area, it can be further separated into an upper and a lower region (15). For each region, longitudinal and transverse scans are applied by keeping the probe vertical to the ribs. In respect to the differential diagnosis of neonatal respiratory distress during the first 24 h of life, LUS and CXR exhibit a high concordance (16). Evidence-based guidelines for the use of point-of-care ultrasound (POCUS) in newborns and children provided by the European Society of Paediatric and Neonatal Intensive Care (ESPNIC) have an important role in standardizing the clinical practice of POCUS (17). An updated and detailed specification and guideline for neonatal LUS examination published this year has helped in the standardization of this examination. Therefore, it may be valuable in improving interobserver consistency in diagnostic accuracy, reliability, and patient outcomes (18).
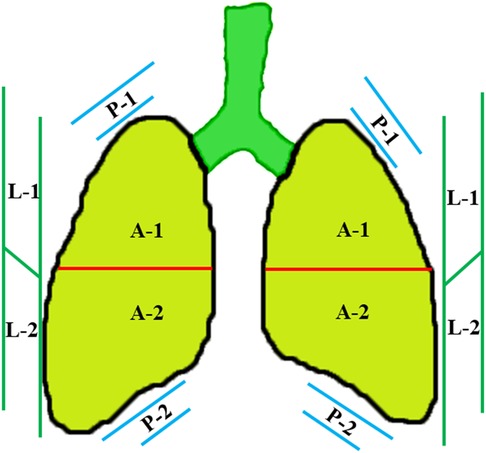
Figure 1. Divisions of the lung field. Each hemithorax can be separated into three regions by the anterior and posterior axillary lines, including the anterior (A) region, lateral (L) region, and posterior (P) region. In respect to each region, it can be further separated into an upper and a lower region.
Normal neonatal LUS appearance
Normally, the ultrasound beam is dissipated by the lung air, which bears high acoustic impedance, and therefore, no acoustic mismatch can be obtained (19). However, as a result of the ultrasound reflected at the pleural–lung interface, the pleura is visualized as a smooth, regular echogenic line, called “pleural line” (Figure 2). In normal conditions, the pleural line thickness in healthy preterm and term newborns should be less than 1 mm (20), and the pleural line shifts to and fro synchronously with breathing. This movement, called “lung sliding” (11), is an indication of a healthy lung and can be easily detected by using a real-time ultrasound system. The repetitive reflection of the ultrasound waves between the pleura and the probe results in the formation of horizontal reverberation artifacts, called “A-lines” (Figure 2A). Parallel to the pleural line, the distal A-lines exhibit an equal interval from one another (19, 21). In addition, B-lines may be detected in normal lungs of neonates within 48 h after birth or longer in premature infants. These B-lines will disappear once the lung fluid is cleared (19, 21, 22). Derived from the ultrasound reflected by the alveolar air–liquid surface, B-lines vertically arise from the pleural line and extend to the screen's edge (Figure 2B). Unlike neonatal lungs, B-lines do not exist in normal children or adult lungs. Therefore, the obtained images containing A-lines as well as lung sliding indicate that there is no lung pathology in the scanned area.
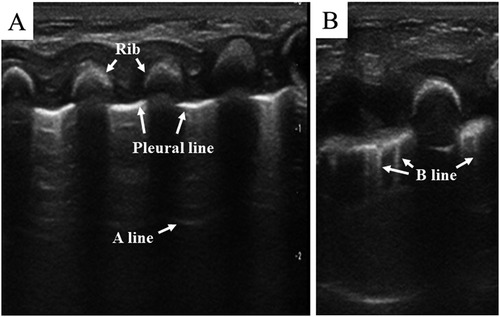
Figure 2. Normal lung pattern on lung ultrasonography. (A) Pleural line: the pleura is visualized as a smooth, regular echogenic line; A-lines: equidistant, horizontal, and echogenic lines distal to the pleural line. (B) B-line: vertical hyperechoic artifacts arising from the pleural line.
Neonatal LUS in diagnosis and description of specific lung diseases
Respiratory distress syndrome
As a common cause of neonatal respiratory failure and early death, neonatal respiratory distress syndrome (RDS) is a manifestation of physiological and structural pulmonary immaturity. Therefore, it mainly occurs in those born prematurely (23). Pulmonary surfactant deficiency of prematurity has been identified as the primary cause of neonatal RDS (24). Pulmonary surfactant administration and mechanical ventilation are usually required to manage severe neonatal RDS (25).
As shown in Figure 3, lung consolidation (hepatization of the lung) with air bronchogram is the most typical LUS appearance of neonatal RDS. The extent of lung consolidation of RDS usually depends on its severity (26). Longitudinal scans show a bilateral white lung (also known as alveolar-interstitial syndrome, AIS), which derives from the emerged compact B-lines observed in all the examined regions. The pleural line is thick, irregular, or blurred. In addition, pleural effusion and lung pulse may be detected in neonates with severe RDS (27, 28). Lung pulse occurs when the normal lung sliding disappears, and the pulmonary tissues beat synchronously with heart pulsation. It is an important sonographic sign of severe lung consolidation, such as atelectasis (29). It should be noted that the LUS appearance of neonatal RDS may be diverse between the bilateral lung and even in the different regions of the same side.
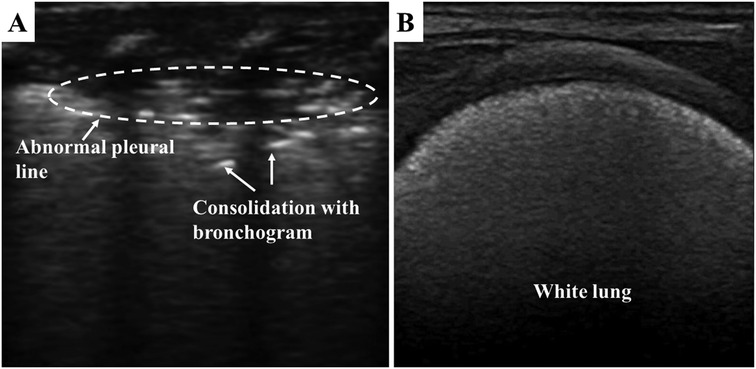
Figure 3. Respiratory distress syndrome on lung ultrasonography. (A) Pleural line is thick, irregular, or blurred. Lung consolidation with the air bronchogram is the most typical appearance of neonatal RDS. (B) white lung is derived from the emerged compact B-lines.
Based on a review of several related studies, LUS produces high sensitivity, specificity, and positive and negative predictive values in neonatal RDS diagnosis, and these values are consistently well above 90% (30–32). It has been confirmed that LUS is a reliable way to evaluate the severity of neonatal RDS (27) and to predict NICU admission in neonates with RDS (33). In addition, LUS has been applied in the follow-up of RDS, and therefore, unnecessary ionizing radiation can be reduced (34–36). As described above, surfactant administration is often needed for preterm babies with RDS. However, timely identification of neonates affected by RDS in need of surfactant therapy remains a challenge. In the current era, several LUS scores have been introduced as an effective predictor of the need for surfactant treatment among neonates with RDS (37–41). Based on the evaluation of treatment effect by LUS scores, poractant alfa produces a more favorable outcome than beractant (42), and the treatment effect of poractant alfa and beractant is superior compared with that of calfactant (43). These positive results indicate that LUS shows promise as a useful tool for decision-making during the treatment of neonatal RDS (44, 45). However, these studies still have various limitations. Thus, large multicenter and well-designed studies are needed prior to using the LUS score as a regular tool for the prediction of surfactant treatment in newborns with RDS (46, 47).
Transient tachypnea of the newborn
As another causative factor for dyspnea in neonates, transient tachypnea of the newborn (TTN) or “wet lung” results from delayed lung fluid clearance. In normal conditions, LUS video recordings show that substantial liquid clearance occurs within the first 20 min, and complete clearance is accomplished within the first 4 h after birth (19, 21, 48). Compared with vaginal delivery, an elective cesarean section may lead to a delayed fluid clearance within the first 3 h of birth (49). In addition, immature GA, influencing the functions of ENac, is also one of the causes of TTN (50). In most cases, TTN presents as a mild or transient respiratory distress, which will improve within 24–48 h. However, symptoms may persist for a long time in some severe cases.
As a result of fluid retention in the lung tissues, pulmonary edema is the common characteristic of TTN. By evaluating the distribution of B-lines, the primary LUS characteristics of TTN, which are related to its severity, include the double lung point (DLP), AIS, or white lung. The DLP means that there is a sharp change in echogenicity as a result of the abundance of B-lines between the upper and the lower lung fields (Figure 4) (48, 51). These appearances are evident bilaterally, but not always symmetrically. In most cases of TTN, the pleural line is regular, and no significant subpleural lung consolidation is present (52). This consistent finding in TTN may be used to rule out other lung disorders such as RDS and pneumonia. The DLP alone is not sufficient reason for performing a diagnosis of TTN. However, LUS can achieve good diagnostic accuracy in detecting TTN when a combination of the DLP and the B-line is applied (53–56). Therefore, exclusive diagnosis is the common method to identify TTN. However, compared with traditional CXR, which exhibits a low sensitivity and specificity for detecting pulmonary edema, LUS performs better in TTN diagnosis (26, 57, 58). Furthermore, LUS can effectively differentiate TTN from RDS (32, 59–61), which is important for providing correct treatment for neonates with respiratory distress. Therefore, LUS is accurate and reliable in diagnosing TTN.
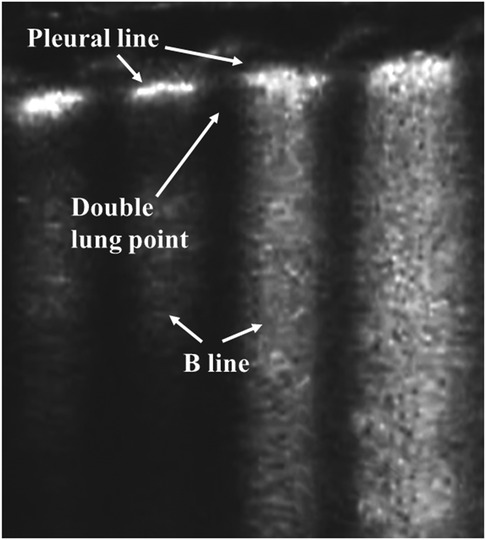
Figure 4. Transient tachypnea of the newborn on lung ultrasonography. Double lung point: there is a sharp change in echogenicity as a result of the abundance of B-lines between the upper and the lower lung fields.
Pneumonia
As a pulmonary inflammatory process, pneumonia leads to significant morbidity and mortality during the neonatal phase, especially in developing countries (62, 63). Epidemiological data indicate that the prevalence of neonatal pneumonia is more severe than presumed (64). Approximately 14% of newborns had experienced pneumonia in China, and potential risk factors contributing to the development of neonatal pneumonia were identified, such as limited or no antenatal care, home delivery, fever at birth, gynecological diseases during pregnancy, and prolonged labor (65). Various bacteria, viruses, or fungi are common pathogens that can induce neonatal pneumonia by intrauterine or postnatal routes (63). Respiratory distress is the common sign of neonatal pneumonia. However, its radiographic findings are often non-specific, which leads to the fact that it may be indistinguishable from RDS and TTN. Therefore, to better determine the type of pneumonia, clinical laboratory findings (such as routine blood tests, C-reactive protein, and blood culture) are needed.
LUS shows promise in diagnosing pneumonia in adults (66) and children (67). These typical LUS appearances of pneumonia mainly include subpleural consolidations with irregular margins and air bronchograms, irregular pleural lines, absent lung sliding, and pleural effusion (68–70). However, reports analyzing the effect of LUS on diagnosing neonatal pneumonia are limited. In a study reported in 2013, LUS exhibited high sensitivity, specificity, and reproducibility in diagnosing neonatal pneumonia (71). A sensitivity of 100% and a specificity of 100% were obtained by applying the presence of large consolidated regions with irregular borders and air bronchograms (Figure 5) as one of the diagnostic criteria for neonatal pneumonia (72). Subsequently, the same research group obtained similar results by introducing LUS into the NICU (26). Based on the detection of ultrasonographic changes in patients before treatment and during discharge (such as the presence or absence of consolidation with air bronchograms, pleural line abnormalities, and pleural effusion), LUS is seen as an effective technique in the diagnosis and monitoring of neonatal viral pneumonia (73). For newborns with infectious pneumonia, the LUS score can be applied to evaluate the severity of the disease, and they are negatively correlated (74). Based on the proposed multiparameter ventilator-associated pneumonia (VAP) score, LUS can be considered an alternative to CXR to diagnose neonatal VAP (75).
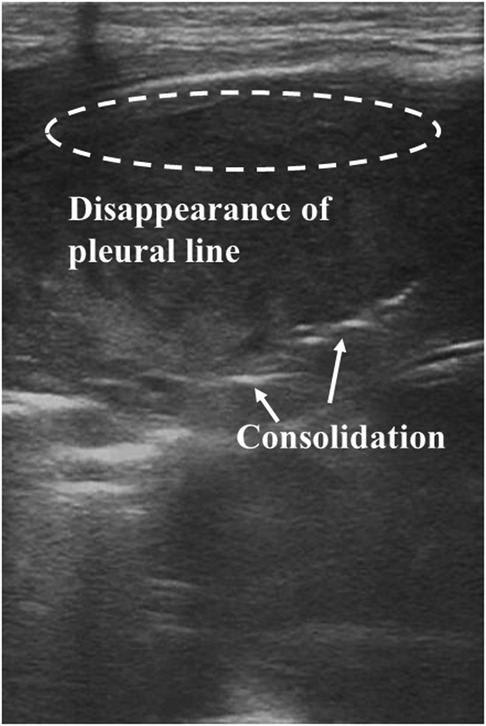
Figure 5. Pneumonia on lung ultrasonography. Ultrasound shows irregular areas of lung consolidation with air bronchograms, disappearance of the pleural line, and A-lines.
Coronavirus Disease 2019 (COVID-19), caused by Severe Acute Respiratory Syndrome Coronavirus-2 (SARS-Cov-2), first occurred in China at the end of 2019 and has since spread to many countries and posed a serious threat to the entire world (76). Apart from being infected by people who have tested positive for SARS-Cov-2, newborn children may catch infection from a vertical transmission in mothers with COVID-19, which, however, has yet to be confirmed (77, 78). LUS has been applied as a method for diagnosing neonatal COVID-19. The related LUS appearances include irregular pleural lines, increased and compact B-lines, and AIS and may coexist with small areas of lung consolidation (77, 79–81). Compared with CXR and CT, LUS exhibits a better sensitivity and safety in diagnosing neonatal COVID-19. Therefore, LUS may be used as an effective method to monitor and evaluate lung lesions in neonates with COVID-19 (82, 83). This acquires more importance in under-resourced areas. Wireless ultrasound probes can be used to reduce the risk of contamination, thus protecting the patient and the physician (84). Thus, LUS is a reliable tool to diagnose and evaluate neonatal pneumonia.
Meconium aspiration syndrome
Caused by antepartum or puerperal inhalation of meconium-stained amniotic fluid, meconium aspiration syndrome (MAS) mainly occurs in term/post-term neonates, leading to respiratory distress of varying severity. The etiology of MAS is complex, and various risk factors such as birth asphyxia and cesarean delivery have been identified for the development of MAS (85, 86). Clinical manifestations, laboratory tests, and radiographic findings can be used to diagnose MAS. Symptomatic and supportive approaches such as mechanical ventilation and surfactant administration are commonly needed to treat MAS (87).
Based on limited reports, LUS appearances of MAS show similarity to that of pneumonia, including irregular subpleural consolidations with air bronchogram, coalescent or sparse B-lines, an abnormal pleural line, and the disappearance of the A-line (88, 89). LUS was performed on six neonates with MAS of variable severity during their first hours of life. Coalescent or sparse B-lines, consolidation, atelectasis, and bronchograms were found, and these results corresponded well with CXR findings (90). In a relatively large-scale study comprising 117 neonates with MAS and 100 controls, similar LUS characteristics were detected, and irregular subpleural consolidations with air bronchograms showed 100% sensitivity and specificity in diagnosing MAS (91). These ultrasonographic findings are not specific to MAS. Particularly, irregular pulmonary consolidations can also be found in cases of pneumonia as described above. However, consolidations are usually found bilaterally in MAS but only on one side in pneumonia. Therefore, it is relatively easy to differentiate between MAS and pneumonia (89).
Air leak syndromes
When air escapes from the alveoli to the extra-alveolar regions, air leak syndromes are formed. Various air leak syndromes (for example, pneumothorax, pneumomediastinum, and pulmonary interstitial emphysema) can be found in neonates. The most common reason for inducing these related syndromes in neonates is inappropriate mechanical ventilation toward the fragile and immature lungs. Thus, these syndromes occur more frequently during the neonatal phase than at any other age (92). Among these air leak syndromes that occur in neonates, pneumothorax is the most common one, and 1%–2% of all term neonates may exhibit spontaneous pneumothorax. However, this incidence may go up to 30% in individuals with various lung pathologies or requiring mechanical ventilation (93). Tube thoracostomy is a safe and effective procedure in the management of pneumothorax (94). Traditionally, CXR or CT has played an important role in diagnosing pneumothorax. In recent years, LUS has been successfully applied in diagnosing neonatal pneumothorax.
On ultrasound, pneumothorax exhibits no lung sliding, no B-lines, clear A-lines, and the presence of the lung point. The air present in the pleural cavity abolishes lung sliding and B-lines. The lung point refers to the interface between a normal pattern with lung sliding and an abnormal pattern without lung sliding (Figure 6). Therefore, the lung point represents the physical border of pneumothorax. As there is too much air in the chest cavity, the highly compressed lung deviates from the chest wall. Therefore, the lung point may not be present in these severe cases. However, the lung point is still a critical and specific characteristic of mild to moderate pneumothorax (95, 96). In adults, a meta-analysis has shown that LUS exhibits a better diagnostic accuracy for pneumothorax than traditional chest radiography (97). A similar advantage of LUS in diagnosing pneumothorax in neonates has also been found in relatively limited studies. A sensitivity of 100% and a similar rate of specificity of LUS for the diagnosis of pneumothorax in neonates were detected. LUS is at least as reliable as CXR in diagnosing neonatal pneumothorax (98–100). In a recent study, it was found that the sensitivities of absent B-lines, absent lung sliding, as well as the presence of the lung point in diagnosing pneumothorax in newborns were 100%, 100%, and 94.28%, respectively, and all the related specificities reached 100%. Compared with CXR, LUS exhibited better sensitivity and specificity in early detection of neonatal pneumothorax (101). Compared with the “mirrored ribs” sign, which lacks specificity for neonatal pneumothorax as can often be observed in other situations or in lungs without pneumothorax, A-lines in the anterior transverse plane show high diagnostic utility in diagnosing pneumothorax (102). Combined with other case reports of using LUS in diagnosing pneumothorax (103, 104) and the proposed procedures for diagnosing pneumothorax by LUS (89), LUS is considered accurate and reliable for diagnosing neonatal pneumothorax. Therefore, these limited data support the opinion that LUS is better than or at least as accurate as CXR in diagnosing neonatal pneumothorax, thus highlighting its treatment efficacy. However, prospective studies are needed to further investigate the practical clinical value of LUS in diagnosing neonatal pneumothorax.
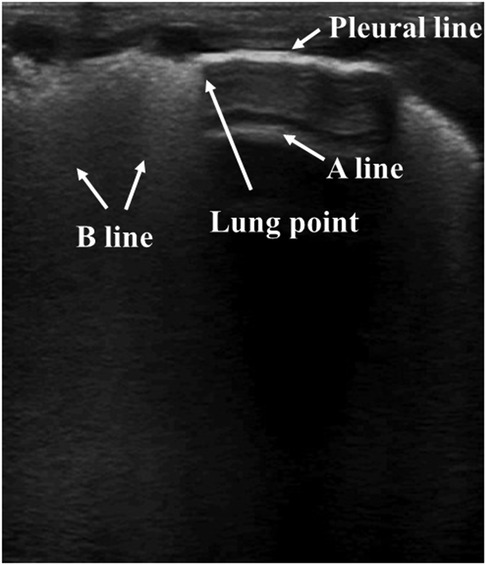
Figure 6. Pneumothorax on lung ultrasonography. Lung point: the transition from the B-lines area (left side) to a hypoechoic area (right side).
For neonatal localized interstitial emphysema, its LUS appearances show bilateral coalescent B-lines and pleural thickness without spared regions. It has been proposed that LUS shows promise as a useful tool for its identification and follow-up (105). LUS examination performed on one neonate with pneumomediastinum (day 2 of life) exhibits multiple A-lines, absence of B-lines, and no pleural shimmering. At the same time, the classic “bar-code” sign can be observed using M-mode ultrasound (106). Thus, it is believed that LUS shows potential to be used as a promising imaging approach to detect pneumomediastinum in newborns.
Bronchopulmonary dysplasia
As a major complication of prematurity, bronchopulmonary dysplasia (BPD) is a common chronic respiratory morbidity seen in one-third of extremely low-birth-weight babies (107). However, it is still challenging to give a standardized definition for BPD, and various definitions have been applied in the literature, leading to broad variations in reporting the incidence of BPD. Among neonates born <28 weeks gestation, this incidence is estimated to be 48%–68%, which significantly enhances along with declining GA (108). The imbalance between lung injury, which can be caused by various factors, and repair in the developing immature lung leads to the development of BPD. Various risk factors occurring during the antenatal, natal, or postnatal period may contribute to BPD (109). As there is no known cure for BPD, infants with BPD often need long-term oxygen treatment, leading to possible lifelong variations in cardiopulmonary activity (110). Various potential cytokines and proteins (111, 112) and chest radiographs (113) have been studied as possible predictors for BPD. However, none of them has been routinely applied in the clinic. Early prediction of BPD is also a potentially interesting application of LUS.
For LUS images of BPD, patterns of unequally distributed B-lines, accompanied by thickened pleural lines and multiple different-sized subpleural consolidations, can be observed (Figure 7) (88, 114, 115). Hyperoxia-exposed preterm rabbits exhibit an LUS appearance similar to human preterm neonates with evolving BPD, and this is valuable in LUS training (116). Persistent retrodiaphragmatic hyperechogenicity detected by LUS with a transabdominal probe has been shown to be capable of predicting the development of BPD (117, 118). Among 50 premature babies clinically identified with BPD, LUS examinations show that more than one-third of them exhibited various other lung pathologies (119). This suggests that a revision of the current diagnostic criteria for BPD, which depend primarily on the length of time of oxygen dependence and may result in an overdiagnosis, is needed. A semiquantitative LUS score has been shown as an effective predictor of BPD. At 1–2 weeks of age, it can predict the diagnosis of BPD, and moderate to severe BPD may be predicted at 4 weeks of age (120). Subsequently, various trials aimed at evaluating the predictive ability of LUS in neonatal BPD have been reported. In preterm neonates with RDS, the LUS pattern is gestational age-dependent and helps to predict BPD in the 28–30-week postmenstrual age (PMA) (121). Among these neonates of <29 weeks of gestation, LUS scores obtained during the first 2 weeks (at days 3, 7, and 14) after birth reveal an excellent prediction of BPD, and the LUS alone is found to be better in predicting BPD than the combined clinical variables related to the prediction of BPD (122). Several reports have shown that LUS scores can predict the occurrence of BPD in preterm neonates, starting from the 7th day of life (DOL) (123–125). The diagnostic accuracy of predicting BPD on the 3rd and 7th DOL can be improved by adding the factors of sex and GA (126). Similarly, when combined with clinical variables, the prediction time of BPD can be brought forward to the 6th DOL compared with the LUS alone (9th to 15th DOLs) (127). A recent meta-analysis on this issue confirms that LUS scores are accurate for the early prediction of BPD in preterm neonates of ≤32 weeks of gestation (128). Based on its convenience and radiation-free feature, LUS is valuable in evaluating the severity of neonatal BPD, therefore providing clues to precision treatment for BPD neonates (115). In summary, LUS, especially when combined with clinical variables related to BPD, is a highly predictive and reproducible diagnostic tool for early prediction of BPD in preterm neonates. Therefore, the application of LUS scores in predicting BPD should be advocated. In addition, larger multicentric prospective studies and more validation studies are needed to determine the optimal LUS scoring procedure.
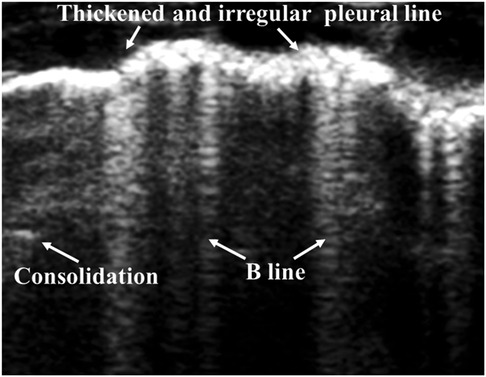
Figure 7. Bronchopulmonary dysplasia on lung ultrasonography. Ultrasound shows unequally distributed B-lines, thickened pleural lines, and multiple different-sized subpleural consolidations.
Thus, LUS shows promise as a predictor of BPD. However, further related research is needed to buttress this finding.
Atelectasis
As a state of collapse and failed aeration of the lung parenchyma, atelectasis presents as a common occurrence in neonates. It can lead to neonatal dyspnea and prolonged illness (129). Atelectasis is a common complication of various pulmonary and chest disorders, including RDS, MAS, and pneumonia, rather than an independent disease. Three different mechanisms accounting for atelectasis have been proposed (129). Airway obstruction is the most common risk factor for atelectasis in infants. Long-term atelectasis may induce other complications such as secondary infection and bronchial damage, and various treatments can be applied according to its cause, duration, and severity (89, 129). The commonly used diagnostic methods for atelectasis include CXR, CT, and bronchoscopy. However, LUS has also been used for diagnosing neonatal lung atelectasis.
On ultrasound, atelectasis exhibits extensive lung consolidation with bronchograms, an abnormal pleural line, no A-lines, no lung sliding, as well as the presence of lung pulse (Figure 8). Compared with the consolidation present in pneumonia, the large areas of consolidation present in atelectasis act as well-defined borders (Figure 8). Combined with CXR and CT, LUS can confirm the presence of neonatal atelectasis, and subsequently, LUS plays a major role in monitoring disease development, without exposing patients to ionizing radiation (130). In a study that enrolled 80 newborns with atelectasis and 50 normal controls, LUS showed 100% sensitivity in diagnosing atelectasis. However, the related sensitivity of CXR was only 75%. Also, large consolidations with clear borders exhibited 100% specificity for neonatal atelectasis (131). In addition, bronchoalveolar lavage under ultrasound monitoring showed high effectiveness in treating neonatal atelectasis without adverse side effects (132). Therefore, LUS is capable of diagnosing neonatal atelectasis accurately and reliably.
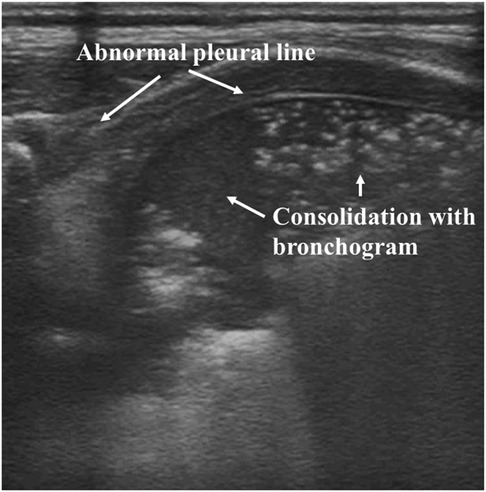
Figure 8. Atelectasis on lung ultrasonography. Ultrasound shows abnormal pleural lines, absent A-lines, and large areas of consolidation.
LUS in other neonatal lung diseases
Apart from these specific neonatal lung diseases described above, LUS has also been reported to diagnose other neonatal lung disorders, which are given in the following paragraphs.
Pulmonary hemorrhage
As a common severe disease in neonates, pulmonary hemorrhage has a high mortality rate, and its rapid and accurate diagnosis is essential for treatment (133). Two reports from the same research group have indicated that LUS exhibits the ability to diagnose neonatal pulmonary hemorrhage (134, 135). Based on the obtained LUS findings, the shred sign, which locates the edge of the consolidation area, possesses a high sensitivity and specificity for diagnosing pulmonary hemorrhage.
Congenital diaphragmatic hernia
Congenital diaphragmatic hernia (CDH), which occurs approximately 2.5 in 10,000 births, induces various levels of pulmonary hypoplasia and pulmonary hypertension (136). It has been reported by two case reports (137, 138) that point-of-care LUS can exclude cardiac disease and pneumothorax rapidly and expedite the diagnosis of CDH. Also, this positive role of LUS in diagnosing CDH has been confirmed by describing LUS patterns in infants with this pathology (139).
Congenital pulmonary airway malformation
Comprising different congenital disorders of the lung and airways, congenital pulmonary airway malformation (CPAM) exhibits an incidence of approximately 1 in 10,000 to 1 in 35,000 births. It causes significant mortality and morbidity in infants (140). The introduction of LUS appearances in newborns with CPAM was first reported in 2018. LUS shows potential as an imaging approach to confirm prenatally identified CPAM, and it can be suspected once cystic lung lesions are observed by LUS in infants with respiratory distress (141). The effectiveness (accuracy and safety) of LUS in the management of neonatal CPAM has been proved by subsequent reports (142, 143). As a result of the high level of consistency with CT, LUS may be used routinely to confirm the diagnosis of neonatal CPAM and for follow-up and management. In addition, a recent report based on a single-center, retrospective cohort study confirms that LUS is valuable in the preliminary qualitative screening of CPAM in infants, and the diagnostic performance of the indirect signs of LUS is better than that of CXR (144).
Bronchiolitis
As a lower respiratory tract disease, bronchiolitis usually occurs in the first life year. A systemic review suggests that LUS aids the clinical management of bronchiolitis in young children by, for example, reducing unnecessary exposure to ionizing radiation and avoiding the needless administration of antibiotics (145). However, its role in neonatal bronchiolitis needs further study.
Congenital chylothorax
Although it is a rare entity, congenital chylothorax is the most common reason for pleural effusion in neonates, and various treatment strategies have been introduced and implemented (146, 147). It has been suggested that LUS should be considered for the diagnosis, monitoring, and follow-up of neonates with congenital chylothorax (147, 148).
Other applications beyond diagnosis
Apart from being used as a diagnostic method for neonatal lung diseases, as described above, LUS can play a more “functional” role in guiding or assisting treatments for neonatal lung diseases through real-time monitoring and assessment. One of the most important applications is the recently proposed use of the LUS score as a semiquantitative assessment of the severity of lung diseases. Derived from LUS scores applied in adults, which have been used to assess pulmonary aeration as well as oxygenation, a modified LUS score for neonates was proposed, and it possesses a good correlation with oxygenation status in newborns. Therefore, it can be used to predict the need for surfactants in preterm infants (149). Its positive role in predicting surfactant need in very- and extremely preterm newborns (39, 150) and also the development of chronic lung disease in preterm neonates (151) has been reported recently. However, the role (for example, accuracy) of the semiquantitative LUS scores in evaluating the severity of neonatal lung diseases is still a matter of debate because, currently, two senior medical experts hold the opposite opinion (152, 153).
LUS scores obtained during the first days of life can provide information about the prognosis of neonatal respiratory failure (154) and predict the need for respiratory support (155–157). In addition, LUS performs a useful role in predicting non-invasive ventilation failure in neonates with respiratory distress, and this is important for the clinician to decide to apply invasive mechanical ventilation to prevent clinical deterioration (154, 158–160). Based on the obtained LUS severity score, point-of-care LUS can predict the weaning readiness off nasal continuous positive airway pressure (NCPAP) in premature infants with evolving BPD (161) and also the success of extubation among ventilated neonates (162–164), thus avoiding their exposure to multiple unsuccessful weaning cycles. As described above, surfactant replacement therapy may be needed in neonates with serious respiratory disease, and early identification of this need is important. The only randomized clinical trial on this issue reported so far indicates that the LUS score improves timeliness in surfactant replacement compared with using FiO2 criteria alone (165). The accuracy of LUS for predicting the need for surfactant replacement increases when combined with the oxygenation index, and this is valuable in clinical decision-making (166, 167). A critical systemic review and meta-analysis has shown that the LUS score could be employed accurately to predict the need for surfactant treatment in early preterm infants with respiratory distress treated with NCPAP (168). In addition, an investigation indicates that LUS-guided surfactant administration in preterm neonates with RDS does not increase pharmaceutical expenditure, including the cost of surfactant therapy and global surfactant utilization (169).
It has been reported that LUS can be used to guide pulmonary recruitment maneuvers during mechanical ventilation in neonates (170, 171). As the developed artifacts resemble sunrays crossing the clouds, the novel S-lines (“sunray lines”) described in the LUS-guided protocol may improve the efficacy and safety of mechanical ventilation strategies in neonates (170). In addition, computer-aided image analyses have been developed to interpret LUS findings. In recent years, various versions of quantitative ultrasound fetal lung maturity analysis software (quantusFLM) have been reported to be used as an automated and non-invasive approach for the prediction of neonatal respiratory morbidity with a performance result similar to that of tests using amniotic fluid (172–174). Point-of-care LUS has also played an important role in guiding or assisting neonatal pulmonary disease management such as guiding bronchoalveolar lavage to treat neonatal atelectasis, MAS, and severe pneumonia, guiding thoracentesis to treat massive pleural effusion and pneumothorax, guiding the application of neonatal mechanical ventilation, and evaluating the effects of surfactant administration (175).
Conclusion
Owing to its radiation-free, convenient, non-invasive, accurate, and less expensive characteristics, LUS has played an important role in the identification and differential diagnosis of various neonatal pulmonary disorders. In addition, because of its easy operation and its commercially automated and non-invasive software, it is worthy and necessary to extend the clinical applications of LUS in neonatal lung diseases further.
Author contributions
JW and HW collected the data and wrote the paper. All authors contributed to the article and approved the submitted version.
Funding
This work was funded by the General Program of Jiangsu Province Hospital Association (No. JSYGY-3-2019-504) and Key Program for the 13th-Five Year Plan from National Health Commission of China (No. YYWS1253).
Conflict of interest
The authors declare that the research was conducted in the absence of any commercial or financial relationships that could be construed as a potential conflict of interest.
Publisher's note
All claims expressed in this article are solely those of the authors and do not necessarily represent those of their affiliated organizations, or those of the publisher, the editors and the reviewers. Any product that may be evaluated in this article, or claim that may be made by its manufacturer, is not guaranteed or endorsed by the publisher.
References
1. Chowdhury N, Giles BL, Dell SD. Full-term neonatal respiratory distress and chronic lung disease. Pediatr Ann. (2019) 48:e175–81. doi: 10.3928/19382359-20190328-01
2. Zhang L, Qiu Y, Yi B, Ni L, Zhang L, Taxi P, et al. Mortality of neonatal respiratory failure from Chinese northwest NICU network. J Matern Fetal Neonatal Med. (2017) 30:2105–11. doi: 10.1080/14767058.2016.1238894
3. Angus DC, Linde-Zwirble WT, Clermont G, Griffin MF, Clark RH. Epidemiology of neonatal respiratory failure in the United States: projections from California and New York. Am J Respir Crit Care Med. (2001) 164:1154–60. doi: 10.1164/ajrccm.164.7.2012126
4. Smith-Bindman R. Is computed tomography safe? N Engl J Med. (2010) 363:1–4. doi: 10.1056/NEJMp1002530
5. Gargani L, Picano E. The risk of cumulative radiation exposure in chest imaging and the advantage of bedside ultrasound. Crit Ultrasound J. (2015) 7:4. doi: 10.1186/s13089-015-0020-x
6. Khattab M, Hagan J, Staib LH, Mustafa A, Goodman TR. Cumulative diagnostic imaging radiation exposure in premature neonates. J Neonatal Perinatal Med. (2022) 15:95–103. doi: 10.3233/npm-210726
7. Gislason-Lee AJ. Patient x-ray exposure and ALARA in the neonatal intensive care unit: Global patterns. Pediatr Neonatol. (2021) 62:3–10. doi: 10.1016/j.pedneo.2020.10.009
8. Kartikeswar GAP, Parikh TB, Pandya D, Pandit A. Ionizing radiation exposure in NICU. Indian J Pediatr. (2020) 87:158–60. doi: 10.1007/s12098-019-03126-9
9. Hall EJ. Lessons we have learned from our children: cancer risks from diagnostic radiology. Pediatr Radiol. (2002) 32:700–6. doi: 10.1007/s00247-002-0774-8
10. Lichtenstein D, Goldstein I, Mourgeon E, Cluzel P, Grenier P, Rouby JJ. Comparative diagnostic performances of auscultation, chest radiography, and lung ultrasonography in acute respiratory distress syndrome. Anesthesiology. (2004) 100:9–15. doi: 10.1097/00000542-200401000-00006
11. Lichtenstein DA, Menu Y. A bedside ultrasound sign ruling out pneumothorax in the critically ill. Lung sliding. Chest. (1995) 108:1345–8. doi: 10.1378/chest.108.5.1345
12. Francesco R, Nadya Y, Fiorella M, Letizia C, Daniele DL. Point-of-care lung ultrasound in neonatology: classification into descriptive and functional applications. Pediatr Res. (2018) 90:524–31. doi: 10.1038/s41390-018-0114-9
13. Escourrou G, De Luca D. Lung ultrasound decreased radiation exposure in preterm infants in a neonatal intensive care unit. Acta Paediatr. (2016) 105:e237–9. doi: 10.1111/apa.13369
14. Gao YQ, Qiu RX, Liu J, Zhang L, Ren XL, Qin SJ. Lung ultrasound completely replaced chest x-ray for diagnosing neonatal lung diseases: a 3-year clinical practice report from a neonatal intensive care unit in China. J Matern Fetal Neonatal Med. (2022) 18:3565–72. doi: 10.1080/14767058.2020.1830369
15. Xirouchaki N, Georgopoulos D. The use of lung ultrasound: a brief review for critical care physicians and pneumonologists review. Pneumon. (2007) 20:134–41.
16. Corsini I, Parri N, Gozzini E, Coviello C, Leonardi V, Poggi C, et al. Lung ultrasound for the differential diagnosis of respiratory distress in neonates. Neonatology. (2019) 115:77–84. doi: 10.1159/000493001
17. Singh Y, Tissot C, Fraga MV, Yousef N, Cortes RG, Lopez J, et al. International evidence-based guidelines on point of care ultrasound (POCUS) for critically ill neonates and children issued by the POCUS working group of the European Society of Paediatric and Neonatal Intensive Care (ESPNIC). Crit Care. (2020) 24:65. doi: 10.1186/s13054-020-2787-9
18. Liu J, Guo G. Specification and guideline for technical aspects and scanning parameter settings of neonatal lung ultrasound examination. J Matern-Fetal Neonatal Med. (2022) 35:1003–16. doi: 10.1080/14767058.2021.1940943
19. Blank DA, Kamlin COF, Rogerson SR, Fox LM, Lorenz L, Kane SC, et al. Lung ultrasound immediately after birth to describe normal neonatal transition: an observational study. Arch Dis Child. (2018) 103:F157–62. doi: 10.1136/archdischild-2017-312818
20. Alonso-Ojembarrena A, Lechuga-Sancho AM, Ruiz-Gonzalez E, Gonzalez-Haba-Martinez B, Lubian-Lopez SP. Pleural line thickness reference values for preterm and term newborns. Pediatr Pulmonol. (2020) 55:2296–301. doi: 10.1002/ppul.24920
21. Blank DA, Rogerson SR, Kamlin COF, Fox LM, Lorenz L, Kane SC, et al. Lung ultrasound during the initiation of breathing in healthy term and late preterm infants immediately after birth, a prospective, observational study. Resuscitation. (2017) 114:59–65. doi: 10.1016/j.resuscitation.2017.02.017
22. Buonsenso D, Soldati G, Curatola A, Morello R, De Rose C, Vacca ME, et al. Lung ultrasound pattern in healthy infants during the first 6 months of life. J Ultrasound Med. (2020) 39:2379–88. doi: 10.1002/jum.15347
23. Wang J, Liu X, Zhu T, Yan C. Analysis of neonatal respiratory distress syndrome among different gestational segments. Int J Clin Exp Med. (2015) 8:16273–9.26629144
24. Thorkelsson T, Sigfusson G. Neonatal lung diseases. In: Wheeler DS, Wong HR, Shanley TP, editors. Pediatric critical care medicine. London: Springer London (2014). p. 249–62.
25. Sweet DG, Carnielli V, Greisen G, Hallman M, Ozek E, Te Pas A, et al. European consensus guidelines on the management of respiratory distress syndrome—2019 update. Neonatology. (2019) 115:432–50. doi: 10.1159/000499361
26. Chen SW, Fu W, Liu J, Wang Y. Routine application of lung ultrasonography in the neonatal intensive care unit. Medicine. (2017) 96:e5826. doi: 10.1097/MD.0000000000005826
27. Pang H, Zhang B, Shi J, Zang J, Qiu L. Diagnostic value of lung ultrasound in evaluating the severity of neonatal respiratory distress syndrome. Eur J Radiol. (2019) 116:186–91. doi: 10.1016/j.ejrad.2019.05.004
28. Liu J, Cao HY, Wang HW, Kong XY. The role of lung ultrasound in diagnosis of respiratory distress syndrome in newborn infants. Iran J Pediatr. (2015) 25:e323. doi: 10.5812/ijp.323
29. Lichtenstein DA, Lascols N, Prin S, Meziere G. The “lung pulse”: an early ultrasound sign of complete atelectasis. Intensive Care Med. (2003) 29:2187–92. doi: 10.1007/s00134-003-1930-9
30. Kurepa D, Zaghloul N, Watkins L, Liu J. Neonatal lung ultrasound exam guidelines. J Perinatol. (2018) 38:11–22. doi: 10.1038/jp.2017.140
31. Jagla M, Grudzien A, Starzec K, Tomasik T, Zasada M, Kwinta P. Lung ultrasound in the diagnosis of neonatal respiratory failure prior to patient transport. J Clin Ultrasound. (2019) 47:518–25. doi: 10.1002/jcu.22766
32. Srinivasan S, Aggarwal N, Makhaik S, Jhobta A, Kapila S, Bhoil R. Role of lung ultrasound in diagnosing and differentiating transient tachypnea of the newborn and respiratory distress syndrome in preterm neonates. J Ultrason. (2022) 22:e1–5. doi: 10.15557/JoU.2022.0001
33. Poerio A, Galletti S, Baldazzi M, Martini S, Rollo A, Spinedi S, et al. Lung ultrasound features predict admission to the neonatal intensive care unit in infants with transient neonatal tachypnoea or respiratory distress syndrome born by caesarean section. Eur J Pediatr. (2021) 180:869–76. doi: 10.1007/s00431-020-03789-z
34. Lovrenski J. Lung ultrasonography of pulmonary complications in preterm infants with respiratory distress syndrome. Ups J Med Sci. (2012) 117:10–7. doi: 10.3109/03009734.2011.643510
35. Sawires HK, Abdel Ghany EA, Hussein NF, Seif HM. Use of lung ultrasound in detection of complications of respiratory distress syndrome. Ultrasound Med Biol. (2015) 41:2319–25. doi: 10.1016/j.ultrasmedbio.2015.04.024
36. Tandircioglu UA, Yigit S, Oguz B, Kayki G, Celik HT, Yurdakok M. Lung ultrasonography decreases radiation exposure in newborns with respiratory distress: a retrospective cohort study. Eur J Pediatr. (2022) 181:1029–35. doi: 10.1007/s00431-021-04296-5
37. Raschetti R, Yousef N, Vigo G, Marseglia G, Centorrino R, Ben-Ammar R, et al. Echography-guided surfactant therapy to improve timeliness of surfactant replacement: a quality improvement project. J Pediatr. (2019) 212:137–143.e1. doi: 10.1016/j.jpeds.2019.04.020
38. Pillers D-A. Lung ultrasound may allow for timelier surfactant administration and reduce radiograph use. J Pediatr. (2019) 204:320–3. doi: 10.1016/j.jpeds.2018.10.072
39. De Martino L, Yousef N, Ben-Ammar R, Raimondi F, Shankar-Aguilera S, De Luca D. Lung ultrasound score predicts surfactant need in extremely preterm neonates. Pediatrics. (2018) 142:1–8. doi: 10.1542/peds.2018-0463
40. Gregorio-Hernandez R, Arriaga-Redondo M, Perez-Perez A, Ramos-Navarro C, Sanchez-Luna M. Lung ultrasound in preterm infants with respiratory distress: experience in a neonatal intensive care unit. Eur J Pediatr. (2020) 179:81–9. doi: 10.1007/s00431-019-03470-0
41. Vardar G, Karadag N, Karatekin G. The role of lung ultrasound as an early diagnostic tool for need of surfactant therapy in preterm infants with respiratory distress syndrome. Am J Perinatol. (2021) 38:1547–56. doi: 10.1055/s-0040-1714207
42. Bozkaya D, Dizdar EA, Korkut S, Ceran B, Alkan M, Oguz SS. Evaluation of different types of natural surfactants by lung ultrasound in respiratory distress syndrome. Am J Perinatol. (2021) 38:590–6. doi: 10.1055/s-0039-1700856
43. Dilli D, Cakmakci E, Akduman H, Oktem A, Aydogan S, Citli R, et al. Comparison of three natural surfactants according to lung ultrasonography scores in newborns with respiratory distress syndrome. J Matern Fetal Neonatal Med. (2021) 34:1634–40. doi: 10.1080/14767058.2019.1643313
44. De Luca D. Respiratory distress syndrome in preterm neonates in the era of precision medicine: a modern critical care-based approach. Pediatr Neonatol. (2021) 62(Suppl 1):S3–9. doi: 10.1016/j.pedneo.2020.11.005
45. Perri A, Tana M, Riccardi R, Iannotta R, Giordano L, Rubortone SA, et al. Neonatal lung ultrasonography score after surfactant in preterm infants: a prospective observational study. Pediatr Pulmonol. (2020) 55:116–21. doi: 10.1002/ppul.24566
46. Lodha A, Bhandari V. Use of lung ultrasound to improve timeliness of surfactant replacement in respiratory distress syndrome: are we ready? J Pediatr. (2019) 212:8–10. doi: 10.1016/j.jpeds.2019.05.057
47. Shafer GJ, Suresh GK. Does lung ultrasound score predict the need for surfactant in extremely preterm neonates? Acta Paediatr. (2019) 5:973. doi: 10.1111/apa.14717
48. Alhassen Z, Vali P, Guglani L, Lakshminrusimha S. Recent advances in pathophysiology and management of transient tachypnea of newborn. J Perinatol. (2021) 41:6–16. doi: 10.1038/s41372-020-0757-3
49. Martelius L, Janer C, Suvari L, Helve O, Lauerma K, Pitkanen O, et al. Delayed lung liquid absorption after cesarean section at term. Neonatology. (2013) 104:133–6. doi: 10.1159/000351290
50. Yurdakok M. Transient tachypnea of the newborn: what is new? J Matern Fetal Neonatal Med. (2010) 23:24–6. doi: 10.3109/14767058.2010.507971
51. Copetti R, Cattarossi L. The ‘double lung point': an ultrasound sign diagnostic of transient tachypnea of the newborn. Neonatology. (2007) 91:203–9. doi: 10.1159/000097454
52. Raimondi F, Yousef N, Rodriguez Fanjul J, De Luca D, Corsini I, Shankar-Aguilera S, et al. A multicenter lung ultrasound study on transient tachypnea of the neonate. Neonatology. (2019) 115:263–8. doi: 10.1159/000495911
53. Wang Y, Li N, Qu Y. Diagnostic accuracy of lung ultrasound for transient tachypnea: a meta-analysis. J Pediatr. (2022) 98:329–37. doi: 10.1016/j.jped.2021.10.003
54. Li CS, Chu SM, Lien R, Mok TY, Hsu KH, Lai SH. Prospective investigation of serial ultrasound for transient tachypnea of the newborn. Pediatr Neonatol. (2021) 62:64–9. doi: 10.1016/j.pedneo.2020.09.002
55. He L, Sun Y, Sheng W, Yao Q. Diagnostic performance of lung ultrasound for transient tachypnea of the newborn: a meta-analysis. PLoS One. (2021) 16:e0248827. doi: 10.1371/journal.pone.0248827
56. Ma HR, Liu J, Yan WK. Accuracy and reliability of lung ultrasound to diagnose transient tachypnoea of the newborn: evidence from a meta-analysis and systematic review. Am J Perinatol. (2022) 39:973–9. doi: 10.1055/s-0040-1721134
57. Liu J, Lovrenski J, Ye Hlaing A, Kurepa D. Neonatal lung diseases: lung ultrasound or chest x-ray. J Matern Fetal Neonatal Med. (2021) 34:1177–82. doi: 10.1080/14767058.2019.1623198
58. Vergine M, Copetti R, Brusa G, Cattarossi L. Lung ultrasound accuracy in respiratory distress syndrome and transient tachypnea of the newborn. Neonatology. (2014) 106:87–93. doi: 10.1159/000358227
59. Ibrahim M, Omran A, AbdAllah NB, Ibrahim M, El-Sharkawy S. Lung ultrasound in early diagnosis of neonatal transient tachypnea and its differentiation from other causes of neonatal respiratory distress. J Neonatal Perinatal Med. (2018) 11:281–7. doi: 10.3233/NPM-181796
60. Liu J, Chen XX, Li XW, Chen SW, Wang Y, Fu W. Lung ultrasonography to diagnose transient tachypnea of the newborn. Chest. (2016) 149:1269–75. doi: 10.1016/j.chest.2015.12.024
61. Liu J, Wang Y, Fu W, Yang CS, Huang JJ. Diagnosis of neonatal transient tachypnea and its differentiation from respiratory distress syndrome using lung ultrasound. Medicine. (2014) 93:e197. doi: 10.1097/MD.0000000000000197
62. Green RJ, Kolberg JM. Neonatal pneumonia in sub-Saharan Africa. Pneumonia. (2016) 8:3. doi: 10.1186/s41479-016-0003-0
63. Mannan MA, Nahar N, Ahmed F, Jahan I, Mosleh T, Khan KA, et al. Neonatal pneumonia in NICU of a tertiary care center. Bangladesh J Child Health. (2018) 42:112–7. doi: 10.3329/bjch.v42i3.39250
64. Liu L, Oza S, Hogan D, Perin J, Rudan I, Lawn JE, et al. Global, regional, and national causes of child mortality in 2000-13, with projections to inform post-2015 priorities: an updated systematic analysis. Lancet. (2015) 385:430–40. doi: 10.1016/S0140-6736(14)61698-6
65. Yang L, Zhang Y, Yu X, Luo M. Prevalence and risk factors of neonatal pneumonia in China: a longitudinal clinical study. Biomed Res. (2018) 29:57–60. doi: 10.4066/biomedicalresearch.29-17-1672
66. Llamas-Alvarez AM, Tenza-Lozano EM, Latour-Perez J. Accuracy of lung ultrasonography in the diagnosis of pneumonia in adults: systematic review and meta-analysis. Chest. (2017) 151:374–82. doi: 10.1016/j.chest.2016.10.039
67. Pereda MA, Chavez MA, Hooper-Miele CC, Gilman RH, Steinhoff MC, Ellington LE, et al. Lung ultrasound for the diagnosis of pneumonia in children: a meta-analysis. Pediatrics. (2015) 135:714–22. doi: 10.1542/peds.2014-2833
68. Chen SW, Zhang MY, Liu J. Application of lung ultrasonography in the diagnosis of childhood lung diseases. Chin Med J. (2015) 128:2672–8. doi: 10.4103/0366-6999.166035
69. Sharma D, Farahbakhsh N. Role of chest ultrasound in neonatal lung disease: a review of current evidences. J Matern Fetal Neonatal Med. (2019) 32:310–6. doi: 10.1080/14767058.2017.1376317
70. Lovrenski J. Pediatric lung ultrasound—pros and potentials. Pediatr Radiol. (2020) 50:306–13. doi: 10.1007/s00247-019-04525-y
71. Seif El Dien HM, Abd ElLatif DAK. The value of bedside lung ultrasonography in diagnosis of neonatal pneumonia. Egypt J Radiol Nuclear Med. (2013) 44:339–47. doi: 10.1016/j.ejrnm.2013.02.005
72. Liu J, Liu F, Liu Y, Wang HW, Feng ZC. Lung ultrasonography for the diagnosis of severe neonatal pneumonia. Chest. (2014) 146:383–8. doi: 10.1378/chest.13-2852
73. Öktem A, Zenciroğlu A, Üner Ç, Aydoğan S, Dilli D, Okumuş N. Efficiency of lung ultrasonography in the diagnosis and follow-up of viral pneumonia in newborn. Am J Perinatol. (2021). doi: 10.1055/s-0041-1729880
74. Meng K, Ying C, Ji J, Yang L. Evaluation of illness severity of neonate infectious pneumonia and neurobehavioral development through ultrasonography under adaption algorithm. Pak J Med Sci. (2021) 37:1682–6. doi: 10.12669/pjms.37.6-WIT.4883
75. Tusor N, De Cunto A, Basma Y, Klein JL, Meau-Petit V. Ventilator-associated pneumonia in neonates: the role of point of care lung ultrasound. Eur J Pediatr. (2021) 180:137–46. doi: 10.1007/s00431-020-03710-8
76. Wu F, Zhao S, Yu B, Chen YM, Wang W, Song ZG, et al. A new coronavirus associated with human respiratory disease in China. Nature. (2020) 579:265–9. doi: 10.1038/s41586-020-2008-3
77. Zhu H, Wang L, Fang C, Peng S, Zhang L, Chang G, et al. Clinical analysis of 10 neonates born to mothers with 2019-nCoV pneumonia. Transl Pediatr. (2020) 9:51–60. doi: 10.21037/tp.2020.02.06
78. Hu X, Gao J, Luo X, Feng L, Liu W, Chen J, et al. Severe acute respiratory syndrome coronavirus 2 (SARS-CoV-2) vertical transmission in neonates born to mothers with coronavirus disease 2019 (COVID-19) pneumonia. Obstet Gynecol. (2020) 136:65–7. doi: 10.1097/AOG.0000000000003926
79. Xiaoyuan F, Xuwei T, Liongkong Z, Weiqi W, Gen L. Application of pulmonary ultrasound in the diagnosis of COVID-19 pneumonia in neonates. Chin J Pediatr. (2020) 58:347–50. doi: 10.3760/cma.j.cn112140-20200228-00154
80. Matsuoka MW, Rocha S, Gibelli M, Nicolau CM, Carvalho W, Suzuki L, et al. Use of lung ultrasound in neonates during the COVID-19 pandemic. Radiol Bras. (2020) 53:401–4. doi: 10.1590/0100-3984.2020.0110
81. Ibarra-Ríos D, Enríquez-Estrada AC, Serpa-Maldonado EV, Miranda-Vega AL, Villanueva-García D, Vázquez-Solano EP, et al. Lung ultrasound characteristics in neonates with positive real time polymerase chain reaction for SARS-CoV-2 on a tertiary level referral hospital in Mexico city. Front Pediatr. (2022) 10:859092. doi: 10.3389/fped.2022.859092
82. Stoicescu ER, Ciuca IM. Is lung ultrasound helpful in COVID-19 neonates? A systematic review. Diagnostics. (2021) 11:2296. doi: 10.3390/diagnostics11122296
83. Caroselli C, Blaivas M, Falzetti S. Diagnostic imaging in newborns, children and adolescents infected with severe acute respiratory syndrome coronavirus 2 (SARS-CoV-2): is there a realistic alternative to lung high-resolution computed tomography (HRCT) and chest x-rays? A systematic review of the literature. Ultrasound Med Biol. (2021) 47:3034–40. doi: 10.1016/j.ultrasmedbio.2021.07.015
84. Prontera G, Perri A, Vento G, D'Andrea V. Use of wireless ultrasound probe in isolated infants: a case report of two SARS-CoV-2-positive mothers’ newborns. Neonatology. (2022) 119:129–32. doi: 10.1159/000519712
85. Chand S, Salman A, Abbassi RM, Siyal AR, Ahmed F, Leghari AL, et al. Factors leading to meconium aspiration syndrome in term- and post-term neonates. Cureus. (2019) 11:e5574. doi: 10.7759/cureus.5574
86. Oliveira CPL, Flor-de-Lima F, Rocha GMD, Machado AP. Guimaraes pereira areias M H F. meconium aspiration syndrome: risk factors and predictors of severity. J Matern Fetal Neonatal Med. (2019) 32:1492–8. doi: 10.1080/14767058.2017.1410700
87. Rashid MA, Pillai T, Azman IB, Hussaini J. Recent advances in meconium aspiration syndrome. Pak J Med Health Sci. (2019) 13:556–8.
88. Cattarossi L. Lung ultrasound: its role in neonatology and pediatrics. Early Hum Dev. (2013) 89(Suppl 1):S17–9. doi: 10.1016/S0378-3782(13)70006-9
89. Liu J, Sorantin E, Cao H-Y. Neonatal lung ultrasonography. Dordrecht, Netherlands: Springer Nature B. V. (2018). 138.
90. Piastra M, Yousef N, Brat R, Manzoni P, Mokhtari M, De Luca D. Lung ultrasound findings in meconium aspiration syndrome. Early Hum Dev. (2014) 90(Suppl 2):S41–3. doi: 10.1016/s0378-3782(14)50011-4
91. Liu J, Cao HY, Fu W. Lung ultrasonography to diagnose meconium aspiration syndrome of the newborn. J Int Med Res. (2016) 44:1534–42. doi: 10.1177/0300060516663954
92. Jeng MJ, Lee YS, Tsao PC, Soong WJ. Neonatal air leak syndrome and the role of high-frequency ventilation in its prevention. J Chin Med Assoc. (2012) 75:551–9. doi: 10.1016/j.jcma.2012.08.001
93. Apiliogullari B, Sunam GS, Ceran S, Koc H. Evaluation of neonatal pneumothorax. J Int Med Res. (2011) 39:2436–40. doi: 10.1177/147323001103900645
94. Okumuş M, Zubarioğlu AU. Neonatal pneumothorax-10 years of experience from a single center. J Pediatr Res. (2020) 7:163–7. doi: 10.4274/jpr.galenos.2019.50133
95. Moreno-Aguilar G, Lichtenstein D. Lung ultrasound in the critically ill (LUCI) and the lung point: a sign specific to pneumothorax which cannot be mimicked. Crit Care. (2015) 19:311. doi: 10.1186/s13054-015-1030-6
96. Lichtenstein D, Meziere G, Biderman P, Gepner A. The “lung point”: an ultrasound sign specific to pneumothorax. Intensive Care Med. (2000) 26:1434–40. doi: 10.1007/s001340000627
97. Alrajab S, Youssef AM, Akkus NI, Caldito G. Pleural ultrasonography versus chest radiography for the diagnosis of pneumothorax: review of the literature and meta-analysis. Crit Care. (2013) 17:R208. doi: 10.1186/cc13016
98. Liu J, Chi JH, Ren XL, Li J, Chen YJ, Lu ZL, et al. Lung ultrasonography to diagnose pneumothorax of the newborn. Am J Emerg Med. (2017) 35:1298–302. doi: 10.1016/j.ajem.2017.04.001
99. Raimondi F, Rodriguez Fanjul J, Aversa S, Chirico G, Yousef N, De Luca D, et al. Lung ultrasound for diagnosing pneumothorax in the critically ill neonate. J Pediatr. (2016) 175:74–78.e1. doi: 10.1016/j.jpeds.2016.04.018
100. Cattarossi L, Copetti R, Brusa G, Pintaldi S. Lung ultrasound diagnostic accuracy in neonatal pneumothorax. Can Respir J. (2016) 2016:2296. doi: 10.1155/2016/6515069
101. Deng BY, Li N, Wu WS, He XG, Li JF, Huang TL, et al. Use of neonatal lung ultrasound for the early detection of pneumothorax. Am J Perinatol. (2019) 37:907–13. doi: 10.1055/s-0039-1688999
102. Montero-Gato J, Rodeño-Fernández L. Ultrasound of pneumothorax in neonates: diagnostic value of the anterior transverse plane and of mirrored ribs. Pediatr Pulm. (2022) 57:1008–14. doi: 10.1002/ppul.25829
103. Thakur A, Kler N, Garg P. Lung ultrasound for detection of pneumothorax in neonates. Indian J Pediatr. (2019) 86:1148. doi: 10.1007/s12098-019-03030-2
104. Migliaro F, Sodano A, Capasso L, Raimondi F. Lung ultrasound-guided emergency pneumothorax needle aspiration in a very preterm infant. BMJ Case Rep. (2014) 2014:bcr2014206803. doi: 10.1136/bcr-2014-206803
105. Balcells C, Del Rio R, Riaza L, Rebollo M, Rodriguez-Fanjul J, Camprubi M. Lung ultrasound: a useful tool for the follow-up of neonatal localized interstitial emphysema. J Pediatr. (2015) 166:1543. doi: 10.1016/j.jpeds.2015.02.016
106. Jariwala PS, Kalaniti K, Wonko N, Daspal S, Mugarab Samedi V. The value of different radiological modalities in assessment of spontaneous pneumomediastinum: case review and diagnostic perspective. AJP Rep. (2019) 9:e72–5. doi: 10.1055/s-0039-1683862
107. Walsh MC, Szefler S, Davis J, Allen M, Van Marter L, Abman S, et al. Summary proceedings from the bronchopulmonary dysplasia group. Pediatrics. (2006) 117:S52–6. doi: 10.1542/peds.2005-0620I
108. Stoll BJ, Hansen NI, Bell EF, Shankaran S, Laptook AR, Walsh MC, et al. Neonatal outcomes of extremely preterm infants from the NICHD neonatal research network. Pediatrics. (2010) 126:443–56. doi: 10.1542/peds.2009-2959
109. Kalikkot Thekkeveedu R, Guaman MC, Shivanna B. Bronchopulmonary dysplasia: a review of pathogenesis and pathophysiology. Respir Med. (2017) 132:170–7. doi: 10.1016/j.rmed.2017.10.014
110. Collins JJP, Tibboel D, de Kleer IM, Reiss IKM, Rottier RJ. The future of bronchopulmonary dysplasia: emerging pathophysiological concepts and potential new avenues of treatment. Front Med. (2017) 4:61. doi: 10.3389/fmed.2017.00061
111. Méndez-Abad P, Zafra-Rodríguez P, Lubián-López S, Benavente-Fernández I. NTproBNP is a useful early biomarker of bronchopulmonary dysplasia in very low birth weight infants. Eur J Pediatr. (2019) 178:755–61. doi: 10.1007/s00431-019-03347-2
112. Ambalavanan N, Carlo WA, D'Angio CT, McDonald SA, Das A, Schendel D, et al. Cytokines associated with bronchopulmonary dysplasia or death in extremely low birth weight infants. Pediatrics. (2009) 123:1132–41. doi: 10.1542/peds.2008-0526
113. Kim HR, Kim JY, Yun B, Lee B, Choi CW, Kim BI. Interstitial pneumonia pattern on day 7 chest radiograph predicts bronchopulmonary dysplasia in preterm infants. BMC Pediatr. (2017) 17:125. doi: 10.1186/s12887-017-0881-1
114. Elsayed YN. Lung ultrasound as a new technique for diagnosis of neonatal respiratory diseases. Neonatal Netw. (2018) 37:224–32. doi: 10.1891/0730-0832.37.4.224
115. Sun YH, Yuan L, Du Y, Zhou JG, Lin SB, Zhang R, et al. Characterization of lung ultrasound imaging in preterm infants with bronchopulmonary dysplasia. Clin Hemorheol Microcirc. (2022) 80:83–95. doi: 10.3233/ch-211132
116. Loi B, Casiraghi C, Catozzi C, Storti M, Lucattelli M, Bartalesi B, et al. Lung ultrasound features and relationships with respiratory mechanics of evolving BPD in preterm rabbits and human neonates. J Appl Physiol. (2021) 131:895–904. doi: 10.1152/japplphysiol.00300.2021
117. Pieper CH, Smith J, Brand EJ. The value of ultrasound examination of the lungs in predicting bronchopulmonary dysplasia. Pediatr Radiol. (2004) 34:227–31. doi: 10.1007/s00247-003-1102-7
118. Avni EF, Cassart M, de Maertelaer V, Rypens F, Vermeylen D, Gevenois PA. Sonographic prediction of chronic lung disease in the premature undergoing mechanical ventilation. Pediatr Radiol. (1996) 26:463–9. doi: 10.1007/BF01377203
119. Liu J, Chen SW, Liu F, Wang Y, Huang JJ. BPD, not BPD, or iatrogenic BPD: findings of lung ultrasound examinations. Medicine. (2014) 93:e133. doi: 10.1097/MD.0000000000000133
120. Alonso-Ojembarrena A, Lubian-Lopez SP. Lung ultrasound score as early predictor of bronchopulmonary dysplasia in very low birth weight infants. Pediatr Pulm. (2019) 54:1404–9. doi: 10.1002/ppul.24410
121. Raimondi F, Migliaro F, Corsini I, Meneghin F, Dolce P, Pierri L, et al. Lung ultrasound score progress in neonatal respiratory distress syndrome. Pediatrics. (2021) 147:1–9. doi: 10.1542/peds.2020-030528
122. Mohamed A, Mohsen N, Diambomba Y, Lashin A, Louis D, Elsayed Y, et al. Lung ultrasound for prediction of bronchopulmonary dysplasia in extreme preterm neonates: a prospective diagnostic cohort study. J Pediatr. (2021) 238:187–92.e2. doi: 10.1016/j.jpeds.2021.06.079
123. Aldecoa-Bilbao V, Velilla M, Teresa-Palacio M, Esponera CB, Barbero AH, Sin-Soler M, et al. Lung ultrasound in bronchopulmonary dysplasia: patterns and predictors in very preterm infants. Neonatology. (2021) 118:537–45. doi: 10.1159/000517585
124. Oulego-Erroz I, Alonso-Quintela P, Terroba-Seara S, Jiménez-González A, Rodríguez-Blanco S. Early assessment of lung aeration using an ultrasound score as a biomarker of developing bronchopulmonary dysplasia: a prospective observational study. J Perinatol. (2021) 41:62–8. doi: 10.1038/s41372-020-0724-z
125. Loi B, Vigo G, Baraldi E, Raimondi F, Carnielli VP, Mosca F, et al. Lung ultrasound to monitor extremely preterm infants and predict bronchopulmonary dysplasia. A multicenter longitudinal cohort study. Am J Respir Crit Care Med. (2021) 203:1398–409. doi: 10.1164/rccm.202008-3131OC
126. Alonso-Ojembarrena A, Serna-Guerediaga I, Aldecoa-Bilbao V, Gregorio-Hernández R, Alonso-Quintela P, Concheiro-Guisán A, et al. The predictive value of lung ultrasound scores in developing bronchopulmonary dysplasia: a prospective multicenter diagnostic accuracy study. Chest. (2021) 160:1006–16. doi: 10.1016/j.chest.2021.02.066
127. Liu X, Lv X, Jin D, Li H, Wu H. Lung ultrasound predicts the development of bronchopulmonary dysplasia: a prospective observational diagnostic accuracy study. Eur J Pediatr. (2021) 180:2781–9. doi: 10.1007/s00431-021-04021-2
128. Pezza L, Alonso-Ojembarrena A, Elsayed Y, Yousef N, Vedovelli L, Raimondi F, et al. Meta-analysis of lung ultrasound scores for early prediction of bronchopulmonary dysplasia. Ann Am Thorac Soc. (2022) 19:659–67. doi: 10.1513/AnnalsATS.202107-822OC
129. Peroni DG, Boner AL. Atelectasis: mechanisms, diagnosis and management. Paediatr Respir Rev. (2000) 1:274–8. doi: 10.1053/prrv.2000.0059
130. Caiulo VA, Gargani L, Caiulo S, Moramarco F, Latini G, Gargasole C, et al. Usefulness of lung ultrasound in a newborn with pulmonary atelectasis. Pediatr Med Chir. (2011) 33:253–5.22428435
131. Liu J, Chen SW, Liu F, Li QP, Kong XY, Feng ZC. The diagnosis of neonatal pulmonary atelectasis using lung ultrasonography. Chest. (2015) 147:1013–9. doi: 10.1378/chest.14-1306
132. Liu J, Ren XL, Fu W, Liu Y, Xia RM. Bronchoalveolar lavage for the treatment of neonatal pulmonary atelectasis under lung ultrasound monitoring. J Matern Fetal Neonatal Med. (2017) 30:2362–6. doi: 10.1080/14767058.2016.1248935
133. Zahr RA, Ashfaq A, Marron-Corwin M. Neonatal pulmonary hemorrhage. NeoReviews. (2012) 13:e302–6. doi: 10.1542/neo.13-5-e302
134. Ren XL, Fu W, Liu J, Liu Y, Xia RM. Lung ultrasonography to diagnose pulmonary hemorrhage of the newborn. J Matern Fetal Neonatal Med. (2017) 30:2601–6. doi: 10.1080/14767058.2016.1256997
135. Liu J, Fu W, Chen SW, Wang Y. Diagnosis of pulmonary hemorrhage of the newborn infants using lung ultrasonography. Chin J Pediatr. (2017) 55:46–9. doi: 10.3760/cma.j.issn.0578-1310.2017.01.009
136. Chatterjee D, Ing RJ, Gien J. Update on congenital diaphragmatic hernia. Anesth Analg. (2020) 131:808–21. doi: 10.1213/ANE.0000000000004324
137. Rankin JH, Elkhunovich M, Seif D, Chilstrom M. Point-of-care ultrasound diagnosis of diaphragmatic hernia in an infant with respiratory distress. Pediatr Emerg Care. (2016) 32:731–3. doi: 10.1097/PEC.0000000000000933
138. Desjardins MP, Weerdenburg KD, Fischer JW. Emergency point-of-care ultrasound diagnosis of diaphragmatic hernia in the pediatric emergency department. Pediatr Emerg Care. (2016) 32:685–7. doi: 10.1097/PEC.0000000000000924
139. Corsini I, Parri N, Coviello C, Leonardi V, Dani C. Lung ultrasound findings in congenital diaphragmatic hernia. Eur J Pediatr. (2019) 178:491–5. doi: 10.1007/s00431-019-03321-y
140. Lakhoo K. Management of congenital cystic adenomatous malformations of the lung. Arch Dis Child. (2009) 94:F73–6. doi: 10.1136/adc.2007.130542
141. Yousef N, Mokhtari M, Durand P, Raimondi F, Migliaro F, Letourneau A, et al. Lung ultrasound findings in congenital pulmonary airway malformation. Am J Perinatol. (2018) 35:1222–7. doi: 10.1055/s-0038-1645861
142. Merli L, Nanni L, Curatola A, Pellegrino M, De Santis M, Silvaroli S, et al. Congenital lung malformations: a novel application for lung ultrasound? J Ultrason. (2019) 24:349–53. doi: 10.1007/s40477-019-00406-0
143. Quercia M, Panza R, Calderoni G, Di Mauro A, Laforgia N. Lung ultrasound: a new tool in the management of congenital lung malformation. Am J Perinatol. (2019) 36:S99–S105. doi: 10.1055/s-0039-1692131
144. Liu D, Zhang G, Liao J, Jiang L, Cai C, Li X, et al. Clinical image feature analysis and diagnostic efficacy evaluation of pulmonary ultrasound in the diagnosis of congenital pulmonary airway malformations in children: based on a single center, retrospective cohort study. Comput Intell Neurosci. (2022) 2022:7490207. doi: 10.1155/2022/7490207
145. Di Mauro A, Ammirabile A, Quercia M, Panza R, Capozza M, Manzionna MM, et al. Acute bronchiolitis: is there a role for lung ultrasound? Diagnostics. (2019) 9:172. doi: 10.3390/diagnostics9040172
146. Resch B, Sever Yildiz G, Reiterer F. Congenital chylothorax of the newborn: a systematic analysis of published cases between 1990 and 2018. Respiration. (2022) 101:84–96. doi: 10.1159/000518217
147. Rocha G, Arnet V, Soares P, Gomes AC, Costa S, Guerra P, et al. Chylothorax in the neonate—a stepwise approach algorithm. Pediatr Pulmonol. (2021) 56:3093–105. doi: 10.1002/ppul.25601
148. Perri A, Tana M, Sbordone A, Patti ML, Sattin G, Vento G. “Open questions” and role of lung ultrasound in the management of congenital chylothorax. Respiration. (2022) 101:16–7. doi: 10.1159/000520313
149. Brat R, Yousef N, Klifa R, Reynaud S, Shankar Aguilera S, De Luca D. Lung ultrasonography score to evaluate oxygenation and surfactant need in neonates treated with continuous positive airway pressure. JAMA Pediatr. (2015) 169:e151797. doi: 10.1001/jamapediatrics.2015.1797
150. Badurdeen S, Kamlin COF, Rogerson SR, Kane SC, Polglase GR, Hooper SB, et al. Lung ultrasound during newborn resuscitation predicts the need for surfactant therapy in very- and extremely preterm infants. Resuscitation. (2021) 162:227–35. doi: 10.1016/j.resuscitation.2021.01.025
151. Abdelmawla M, Louis D, Narvey M, Elsayed Y. A lung ultrasound severity score predicts chronic lung disease in preterm infants. Am J Perinatol. (2019) 36:1357–61. doi: 10.1055/s-0038-1676975
152. De Luca D. Semiquantititative lung ultrasound scores are accurate and useful in critical care, irrespective of patients’ ages: the power of data over opinions. J Ultrasound Med. (2020) 39:1235–9. doi: 10.1002/jum.15195
153. Liu J. The lung ultrasound score cannot accurately evaluate the severity of neonatal lung disease. J Ultrasound Med. (2020) 39:1015–20. doi: 10.1002/jum.15176
154. Gunes AO, Karadag N, Cakir H, Toptan HH, Karatekin G. The associations between lung ultrasonography scores in the first day of life and clinical outcomes. J Ultrasound Med. (2022) 41:417–25. doi: 10.1002/jum.15720
155. Perri A, Sbordone A, Tirone C, Giordano L, Tana M, D'Andrea V, et al. Early lung ultrasound score to predict noninvasive ventilation needing in neonates from 33 weeks of gestational age: a multicentric study. Pediatr Pulmonol. (2022) 57:2227–36. doi: 10.1002/ppul.26031
156. Xi G, Dai J, Wang X, Luo F, Lu C, Yang Y, et al. Ultrasound performed shortly after birth can predict the respiratory support needs of late preterm and term infants: a diagnostic accuracy study. Pediatr Pulmonol. (2021) 56:2155–63. doi: 10.1002/ppul.25389
157. Raimondi F, Migliaro F, Sodano A, Umbaldo A, Romano A, Vallone G, et al. Can neonatal lung ultrasound monitor fluid clearance and predict the need of respiratory support? Crit Care. (2012) 16:R220. doi: 10.1186/cc11865
158. Szymański P, Kruczek P, Hożejowski R, Wais P. Modified lung ultrasound score predicts ventilation requirements in neonatal respiratory distress syndrome. BMC Pediatr. (2021) 21:17. doi: 10.1186/s12887-020-02485-z
159. Rodríguez-Fanjul J, Balcells C, Aldecoa-Bilbao V, Moreno J, Iriondo M. Lung ultrasound as a predictor of mechanical ventilation in neonates older than 32 weeks. Neonatology. (2016) 110:198–203. doi: 10.1159/000445932
160. Raimondi F, Migliaro F, Sodano A, Ferrara T, Lama S, Vallone G, et al. Use of neonatal chest ultrasound to predict noninvasive ventilation failure. Pediatrics. (2014) 134:e1089–94. doi: 10.1542/peds.2013-3924
161. Abdelmawla M, Seleem W, Farooqui M, Eltayeb A, Elsayed Y. Prediction of weaning readiness off nasal CPAP in preterm infants using point-of-care lung ultrasound. Pediatr Pulm. (2022) 57:2128–35. doi: 10.1002/ppul.26014
162. Soliman RM, Elsayed Y, Said RN, Abdulbaqi AM, Hashem RH, Aly H. Prediction of extubation readiness using lung ultrasound in preterm infants. Pediatr Pulmonol. (2021) 56:2073–80. doi: 10.1002/ppul.25383
163. Liang Z, Meng Q, You C, Wu B, Li X, Wu Q. Roles of lung ultrasound score in the extubation failure from mechanical ventilation among premature infants with neonatal respiratory distress syndrome. Front Pediatr. (2021) 9:709160. doi: 10.3389/fped.2021.709160
164. El Amrousy D, Elgendy M, Eltomey M, Elmashad AE. Value of lung ultrasonography to predict weaning success in ventilated neonates. Pediatr Pulmonol. (2020) 55:2452–6. doi: 10.1002/ppul.24934
165. Rodriguez-Fanjul J, Jordan I, Balaguer M, Batista-Muñoz A, Ramon M, Bobillo-Perez S. Early surfactant replacement guided by lung ultrasound in preterm newborns with RDS: the ULTRASURF randomised controlled trial. Eur J Pediatr. (2020) 179:1913–20. doi: 10.1007/s00431-020-03744-y
166. Raimondi F, Migliaro F, Corsini I, Meneghin F, Pierri L, Salomè S, et al. Neonatal lung ultrasound and surfactant administration: a pragmatic, multicenter study. Chest. (2021) 160:2178–86. doi: 10.1016/j.chest.2021.06.076
167. Aldecoa-Bilbao V, Balcells-Esponera C, Herranz Barbero A, Borràs-Novell C, Izquierdo Renau M, Iriondo Sanz M, et al. Lung ultrasound for early surfactant treatment: development and validation of a predictive model. Pediatr Pulmonol. (2021) 56:433–41. doi: 10.1002/ppul.25216
168. Razak A, Faden M. Neonatal lung ultrasonography to evaluate need for surfactant or mechanical ventilation: a systematic review and meta-analysis. Arch Dis Child Fetal Neonatal Ed. (2020) 105:164–71. doi: 10.1136/archdischild-2019-316832
169. De Luca D, Yousef N. Pharmaceutical expenditure is unchanged with ultrasound-guided surfactant administration. Am J Perinatol. (2022) 39:562–6. doi: 10.1055/s-0040-1715821
170. Pierro M, Chioma R, Ciarmoli E, Villani P, Storti E, Copetti R. Lung ultrasound guided pulmonary recruitment during mechanical ventilation in neonates: a case series. J Neonatal Perinatal Med. (2022) 15:357–65. doi: 10.3233/npm-210722
171. Abushady NM, Awad HAS, Kamel DR, Fouda EM, Ahmed NT, Dawoud MO. Role of lung ultrasound in the assessment of recruitment maneuvers in ventilated preterm neonates with respiratory distress syndrome and its correlation with tracheal IL-6 levels: a randomized controlled trial. J Neonatal Perinatal Med. (2021) 14:369–74. doi: 10.3233/npm-200605
172. Burgos-Artizzu XP, Perez-Moreno A, Coronado-Gutierrez D, Gratacos E, Palacio M. Evaluation of an improved tool for non-invasive prediction of neonatal respiratory morbidity based on fully automated fetal lung ultrasound analysis. Sci Rep. (2019) 9:1950. doi: 10.1038/s41598-019-38576-w
173. Palacio M, Bonet-Carne E, Cobo T, Perez-Moreno A, Sabria J, Richter J, et al. Prediction of neonatal respiratory morbidity by quantitative ultrasound lung texture analysis: a multicenter study. Am J Obstet Gynecol. (2017) 217:196e1–e14. doi: 10.1016/j.ajog.2017.03.016
174. Bonet-Carne E, Palacio M, Cobo T, Perez-Moreno A, Lopez M, Piraquive JP, et al. Quantitative ultrasound texture analysis of fetal lungs to predict neonatal respiratory morbidity. Ultrasound Obstet Gynecol. (2015) 45:427–33. doi: 10.1002/uog.13441
Keywords: lung disease, lung ultrasonography, neonate, diagnosis, point of care
Citation: Wang J, Wei H, Chen H, Wan K, Mao R, Xiao P and Chang X (2022) Application of ultrasonography in neonatal lung disease: An updated review. Front. Pediatr. 10:1020437. doi: 10.3389/fped.2022.1020437
Received: 16 August 2022; Accepted: 27 September 2022;
Published: 25 October 2022.
Edited by:
Paul Kingma, Cincinnati Children's Hospital Medical Center, United StatesReviewed by:
Shreyas Arya, Wright State University, United StatesSimón Lubian Lopez, Hospital Universitario Puerta del Mar, Spain
© 2022 Wang, Wei, Chen, Wan, Mao, Xiao and Chang. This is an open-access article distributed under the terms of the Creative Commons Attribution License (CC BY). The use, distribution or reproduction in other forums is permitted, provided the original author(s) and the copyright owner(s) are credited and that the original publication in this journal is cited, in accordance with accepted academic practice. No use, distribution or reproduction is permitted which does not comply with these terms.
*Correspondence: Peng Xiao xp3779@163.com Xin Chang changxinnj@163.com
†These authors have contributed equally to this work and share first authorship
Specialty Section: This article was submitted to Pediatric Pulmonology, a section of the journal Frontiers in Pediatrics