- 1Department of Neonatology, Ankura Hospital for Women and Children, Hyderabad, India
- 2Department of Neonatology, Motherhood Hospital, Bengaluru, India
- 3Department of Neonatology, National Institute of Medical Sciences, Jaipur, India
Moving from an era of invasive ventilation to that of non-invasive respiratory support, various modalities have emerged resulting in improved neonatal outcomes. Respiratory distress is the commonest problem seen both in preterm and term neonates, and the use of appropriate respiratory support could be lifesaving. This article reviews the currently available non-invasive ventilation (NIV) strategies in neonates including nasal continuous positive airway pressure, nasal intermittent positive pressure ventilation (NIPPV), bi-level CPAP, heated humidified high flow nasal cannula, nasal high-frequency ventilation (NHFV) and non-invasive neutrally adjusted ventilatory assist (NIV-NAVA). Though multiple systematic reviews and meta-analyses have indicated the superiority of synchronized NIPPV over the other forms of non-invasive respiratory support in neonates, there is no single NIV modality that universally suits all. Hence, the choice of NIV for a neonate should be individualized based on its efficacy, the disease pathology, resource settings, the clinician's familiarity and parental values. Future studies should evaluate emerging modalities such as NIV-NAVA and NHFV in the respiratory management of neonates as the evidence pertaining to these is insufficient.
Introduction
The incidence of preterm births has shown a steady increase over the past few decades globally (1). Since prematurity is one of the leading causes of neonatal mortality, this has had a significant impact on childhood mortality rates as it forms the major proportion of under-5 mortality, especially in low- and middle-income countries (LMICs) (2, 3). The occurrence of respiratory distress syndrome (RDS) is postulated to be around 12% among those born preterm. RDS is not only associated with significant short- and long-term morbidities but is also one of the commonest causes of mortality in preterm neonates (3). Moreover, mortality attributed to RDS is 10 times higher in LMICs when compared to high-income countries (4). Administration of antenatal corticosteroids, exogenous surfactant therapy and respiratory support (invasive or non-invasive) form the basis of prevention and treatment of RDS. Over the period of past few decades, invasive mechanical ventilation (IMV) has been supplanted by non-invasive ventilation (NIV) as IMV has been shown to be associated with major morbidities. One of the severe morbidities attributed to IMV is bronchopulmonary dysplasia (BPD) (5, 6). BPD itself has been associated with various adverse outcomes such as pulmonary hypertension, increased susceptibility to respiratory infections during infancy, neurodevelopmental impairment and cerebral palsy (7). Despite advances and improvements in IMV strategies, only volume-targeted ventilation (VTV) has been shown to reliably reduce the occurrence of BPD (8–10).
As early as 1987, Avery et al. had reported a reduced incidence of BPD with the use of nasal continuous positive airway pressure (CPAP) (11). Rapid strides in the field of NIV have happened since then (12). Currently, multiple NIV modalities are being used in treating respiratory distress in neonates. These include heated humidified high flow nasal cannula (HHHFNC), CPAP, bilevel CPAP (BiPAP), non-invasive positive pressure ventilation (NIPPV), nasal high-frequency ventilation (NHFV) and non-invasive ventilation with a neurally adjusted ventilatory assist (NIV-NAVA). These modalities vary with respect to many factors such as their mechanism of action, efficacy, safety cost and healthcare provider's familiarity with their use. Multiple systematic reviews and meta-analyses have been published on NIV and its various aspects in the recent years. The objective of our review was to summarize the findings of these systematic reviews so that it would aid the clinicians to interpret the evidence with ease and hence, guide safe clinical practice.
NIV includes respiratory support modalities that do not require the insertion of an endotracheal tube. The basic layout of the devices utilized for NIV consists of a source of oxygen and airflow, an air-oxygen blender, a servo-controlled humidifier and a nasal interface. Depending on the complexity of the device, a single or double-pressure generator, expiratory flow sensors and sensors for assessing diaphragmatic movement are its various components.
CPAP
The earliest documented use of CPAP in preterm neonates for providing respiratory support was in 1971 by Gregory et al. (13). CPAP administered through nasal prongs or mask was the most prevalent form of non-invasive respiratory support during those times (14, 15). Subsequently, with the introduction and refinement of IMV in neonates, use of CPAP declined in the subsequent decade. This trend changed with the landmark publication by Avery et al. in 1987 (11). They reported the incidence of chronic lung disease (BPD) in 8 tertiary care neonatal intensive care units (NICUs) in North America, specifically to evaluate the likely causes for the difference in the incidence of BPD between these NICUs. The group found that the University of Columbia which used CPAP quite extensively with restricted IMV use had the lowest incidence of BPD. This reinvigorated the interest in CPAP globally, with its use being researched in various disease settings in neonates.
The mechanism of action of CPAP is multi-faceted as listed below:
• It improves the functional residual capacity (FRC) and hence decreases the work of breathing (16).
• It decreases intrapulmonary shunting (17).
• It stabilizes the compliant chest wall of preterm neonates and splints the airway (18, 19).
• It decreases thoracoabdominal asynchrony in the neonate (20).
• It aids in accelerating the growth of the immature lung and improves protein content per gram of the lung tissue (21).
• It reduces alveolar edema, conserves surfactant and decreases the occurrence of apnea, especially in preterm infants (22).
A recent systematic review of pre-clinical studies assessed the short- and long-term effects of CPAP use. This review reported that CPAP use in animal neonatal models resulted in improved ventilation and oxygenation (23). CPAP did not disrupt alveolar architecture or pulmonary microvasculature when compared to other non-ventilated or ventilated animal models. It was also shown that early weaning from IMV to CPAP prevents lung injury. Finally, CPAP not only improved the lung mechanics such as its compliance, it also increased the phosphatidylcholine levels which is essential for surfactant production (23).
Generation and delivery of CPAP
It requires 3 major components: (a) flow generator, (b) positive pressure generator device and (c) airway interface.
Flow generation
A source of warm, humidified, blended air-oxygen mixture with an inline flow generator is present. The flow could be either constant or variable.
Constant flow devices provide a constant flow of gases as set by the clinician. These include the commonly used low-cost bubble CPAP and CPAP delivered through a ventilator. Variable flow devices provide a differential flow of incoming gases which is dependent on the phase of respiratory cycle of the spontaneously breathing neonate. Since this decreases the work of breathing, it has gained popularity since its introduction in 1988 (24). These devices use the Bernoulli principle and gas entrainment mechanisms to create a fluidic flip effect causing the change in direction of incoming gases during the expiratory phase of the neonate, and hence decrease the work of breathing (25, 26). There are several variable flow devices which are presently available for use.
Positive pressure delivery
The positive pressure delivered during CPAP may be generated through one of the following techniques:
• The expiratory valve of the ventilator which adjusts the expiratory pressure.
• Adjustment of the inspiratory flow through flow drivers or ventilators.
• The Bubble CPAP system produces a positive pressure by placing the far end of the expiratory tubing under water. The pressure is adjusted by altering the depth of the tube under the surface of the water.
Underwater bubble CPAP systems are most widely used globally, and more so in low-resource settings. The predominant reasons for their popularity are the low cost and maintenance, simplicity of use coupled with almost equal effectiveness as the other CPAP devices (27–31). Multiple studies have reported that bubble CPAP is as effective as or even better than ventilator-delivered CPAP for delivering effective positive pressure ventilation (27, 28).
A Cochrane review (27) published in 2023 investigated the efficacy and safety of bubble CPAP compared to other forms of CPAP namely, ventilator-driven and variable flow CPAP. The meta-analysis included 15 studies (1437 neonates) and reported that there was a reduced risk of treatment failure with bubble CPAP compared to the other forms [Risk Ration (RR) (95% confidence interval (CI)): 0.76 (0.60–0.95)]. The number needed to treat (NNT) for an additional beneficial outcome was 20. However, bubble CPAP was shown to be associated with an increased risk of nasal injury when compared to the other CPAP devices [RR (95% CI): 2.29 (1.37–3.82)]. There were no significant differences in the risk for mortality [RR (95% CI): 0.93 (0.64–1.36)], pneumothorax [RR (95% CI): 0.73 (0.40–1.34)] or BPD [RR (95% CI): 0.76 (0.53–1.10)].
Bharadwaj et al. (28) performed a meta-analysis (19 studies) to investigate the efficacy of bubble CPAP vs. other forms of CPAP devices in preterm neonates. The authors reported that the primary outcome of treatment failure was significantly lesser in neonates treated with bubble CPAP [RR (95% CI): 0.75 (0.57–0.98)]. There were no differences in the risk of mortality [RR (95% CI): 0.82 (0.47–1.42)], BPD [RR (95% CI): 0.8 (0.53–1.21)] and air leak [RR (95% CI): 0.80 (0.42–1.55)]. However, the risk of nasal injury was higher with bubble CPAP when compared to the other forms [RR (95% CI): 2.04 (1.33–3.140)].
A recent cross-over study evaluated the inspiratory efforts in preterm neonates when 3 different types of variable flow CPAP devices were used. This study did not find any difference between the devices (32).
Airway interface
Following nasal interfaces are used in neonates:
• Long nasal prongs/nasopharyngeal prongs.
• Short binasal prongs.
• Nasal masks.
• Nasal cannula with long and narrow tubing (CLNT) (RAM cannula, Neotech, Valencia) (33).
Traditionally, short binasal prongs used to be the most commonly used interface. This is due to their effectiveness, and hence had replaced the older interfaces namely, single nasal and nasopharyngeal prongs (34). Nasal masks are more contemporary in the evolution of CPAP interfaces, and are much smaller than face masks. Further, nasal masks have been associated with reduced risk of nasal trauma. An adequate seal avoiding leak at the nasal end is paramount for CPAP delivery, which was a cause of concern with the use of nasal masks.
The latest Cochrane review (2022) compared the use of nasal masks vs. nasal prongs for delivery of CPAP (12 studies, 1,604 neonates). The authors reported that the use of nasal masks was associated with lower treatment failure rates and decreased nasal injury (including moderate-severe injury). There was no difference in the outcomes of mortality, BPD or pneumothorax between the two groups (35) (Table 1). Similar results were reported by Razak et al. (36), King et al. (37) and Jasani et al. (38) in their respective meta-analyses. Moreover, King et al. also reported that there was a decreased risk of moderate to severe BPD and need for second dose of surfactant in the nasal mask group when compared to the short binasal prongs group (low to very low certainty of evidence) (37).
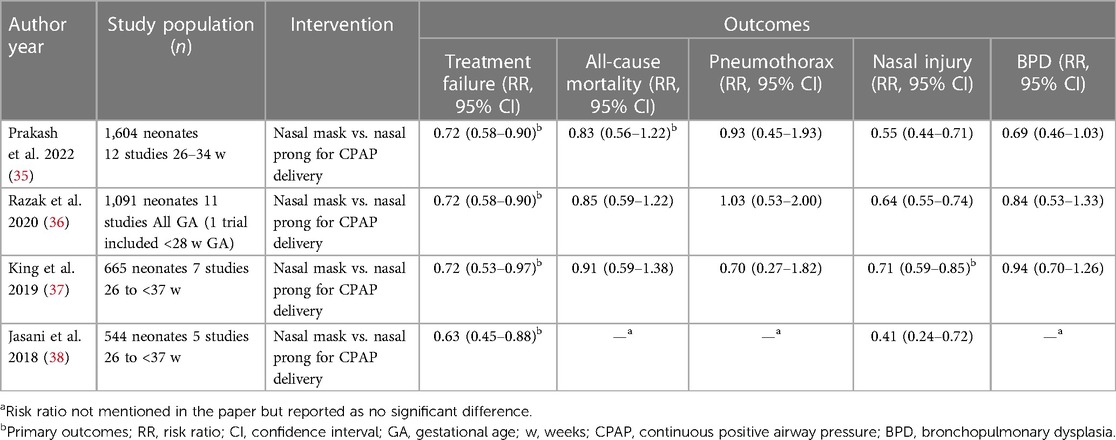
Table 1. Summary of recent meta-analyses comparing nasal mask and short binasal prongs for delivery of NCPAP.
CPAP application in the Delivery room (DR)
Earlier, extremely preterm and very preterm neonates were routinely intubated, and given surfactant in the DR prophylactically. This was followed by a multitude of studies investigating the use of DR CPAP to avoid routine intubation in the DR. The current evidence suggests that early use of CPAP in the DR with selective use of early rescue surfactant using lesser invasive modalities is beneficial and preferable over prophylactic surfactant use followed by IMV in preterm neonates (39–45). CPAP provided in DR has also been found beneficial for late preterm and term neonates (46).
Subramaniam et al. (Cochrane 2021) (44) reported in a meta-analysis of 8 RCTs that prophylactic use of CPAP (application within 15 min of birth in DR) or very early CPAP (application within 60 min of birth) in preterm neonates led to decreased treatment failure (need for IMV) when compared to supportive treatment alone (supplemental oxygen) [RR (95% CI): 0.60 (0.49–0.74)]. However, the risk of BPD was similar between the two groups [RR (95% CI) 0.76 (0.51–1.14)]. The results were also comparable for the outcomes of mortality, BPD or mortality, pulmonary air leaks and intraventricular hemorrhage (IVH) grade >2. Use of prophylactic or very early CPAP was also compared to IMV with or without surfactant therapy. It was reported that the former decreased the risk of the combined outcome of BPD or death, BPD alone and failure of treatment with no effect on the outcomes of mortality, pulmonary air leak or IVH grade >2. The third comparison by the authors was between prophylactic and very early CPAP. The results were derived from a single RCT which reported no difference in mortality or BPD between the two groups. This meta-analysis included neonates of gestational age 24–32 weeks' gestation age (GA).
A network meta-analysis (NMA) published in 2022 compared the use of DR CPAP vs. other interventions. A trend towards decreased risk of IMV was indicated [RR (95% CrI) 0.75 (0.56–1.00)] (45). NMA is a statistical method to compare the efficacy and safety of multiple competing interventions in a single analysis. The final effect estimate is a combination of direct (from RCTs between two interventions) and indirect evidence (where RCTs between two interventions are not available, effect estimate being derived from other RCTs in the network). It provides more robust evidence than pairwise meta-analysis for comparisons where RCTs are available as indirect evidence is also added to the direct evidence from RCTs to derive the final NMA effect estimates. The NMA mentioned here included studies from LMICs only (7 RCTs, 4 observational studies; 4,210 neonates). The authors suggested future studies for evaluating the barriers in improving the effectiveness of DR CPAP in LMICs.
Another systematic review investigated the benefits of DR CPAP in late preterm and term neonates. The authors (2 RCT, 323 neonates) reported a significantly reduced need for admission to the NICU and need for respiratory support in the NICU. However, another systematic review including two observational studies enrolling 8,476 neonates reported that DR CPAP was associated with an increased risk of air leak syndromes when compared with no DR CPAP (46, 47).
CPAP for RDS as primary respiratory support
CPAP is widely used as a primary respiratory support modality in neonates with RDS (48). CPAP for management of neonates with RDS should be initiated in the DR and continued while the neonate is being shifted to the NICU. Initiation of CPAP at birth in neonates who are relatively at higher risk of RDS (like those born prior to 30 weeks' GA) has been recommended by the European consensus guidelines for the management of RDS (49).
In a systematic review assessing the effect of early CPAP (initiated at the beginning of respiratory distress) compared to delayed CPAP (initiated when FiO2 was near 0.60), Ho et al. (48) found that there was no difference in the need for IMV [RR (95% CI): 0.77 (0.43–1.38)], mortality [RR (95% CI): 0.93 (0.43–2.03)], air leak [RR (95% CI): 1.09 (0.39–3.04)] and BPD [RR (95% CI): 1.42 (0.10–20.49)]. Only 4 RCTs (119 neonates, GA: 31–34 weeks) were included in the meta-analysis. These RCTs were performed in the 1970s and early 1980s, an era when both antenatal administration of steroids and respiratory management of preterm neonates were in their nascent stages.
Another Cochrane systematic review compared the initiation of CPAP with low pressure (5 cm H2O or less) vs. moderate to high pressure (>5 cm H2O) in both the settings of primary respiratory support and post-extubation support (50). For primary respiratory support, only 1 RCT with 271 preterm neonates was found to be eligible for inclusion. There was no significant difference in any of the outcomes namely, mortality or BPD [RR (95% CI): 1.02 (0.56–1.85)], mortality [RR (95% CI): 1.04 (0.51–2.12)], BPD [RR (95% CI): 0.80 (0.25–2.57)] or treatment failure [RR (95% CI): 1.00 (0.63–1.57)]. Two RCTs (117 neonates) were eligible for inclusion in the post-extubation setting. The results were quite similar with no significant difference in the composite outcome of mortality or BPD [RR (95% CI): 0.87 (0.51–1.49)], mortality [RR (95% CI): 2.94 (0.12–70.30)], BPD [RR (95% CI): 0.87 (0.51–1.49)] and treatment failure [RR (95% CI): 1.52 (0.92–2.50)] (50).
CPAP for apnea of prematurity
CPAP has been reported to reduce the incidence of mixed and obstructive apnea in preterm neonates (51). It has also been proposed to reduce the frequency and duration of desaturation episodes in central apnea (52). An RCT conducted by Pantalitschka et al. (53) reported that variable flow devices are better than underwater bubble CPAP systems in reducing the risk of apnea of prematurity with median [Interquartile range (IQR)] event rates per hour being 2.8 (1.5–7.7) and 5.4 (3.0–9.8) in variable flow CPAP and constant flow bubble CPAP, respectively. A Cochrane systematic review to assess the efficacy of various CPAP devices in decreasing apnea of prematurity compared to supportive treatment or IMV is currently underway (54).
CPAP for transient tachypnea of newborn (TTN)
CPAP helps in TTN by facilitating the clearance of lung fluid and helps to reduce the duration of NICU stay (55). A retrospective cohort study had evaluated the use of CPAP when compared to nasal cannula in late preterm and term neonates with TTN. The authors reported that after adjusting for birth weight and GA, CPAP decreased the maximal FiO2 requirement [Incidence rate ratio (IRR) (95% CI): 0.85 (0.76–0.96)] (56).
A recent Cochrane review assessed the efficacy of CPAP in TTN compared to other NIV modalities. Only 3 small RCTs were identified: CPAP vs. free flow oxygen, CPAP vs. NIPPV, and CPAP vs. NHFV (57). A meta-analysis was hence not feasible. The authors in their descriptive review concluded that CPAP decreased the duration of tachypnea compared to free flow oxygen [Mean Difference (MD) (95% CI): −21.10 h (−22.92 to −19.28); 1 RCT, 64 neonates)]. There were no differences for the outcomes of need for IMV [RR (95% CI): 0.30 (0.01–6.99)] and pneumothorax (none of the participants developed pneumothorax). NIPPV and CPAP (1 RCT, 40 neonates) were similar in their efficacy for the outcomes of requirement of IMV [RR (95% CI): 4.00 (0.49–32.72), pneumothorax [RR (95% CI): 1.00 (0.07–14.90)] and duration of tachypnea [MD (95% CI): 4.30 h (−19.14 to 27.74)]. The trial comparing NHFV to CPAP (1 study, 46 participants) reported that the duration of tachypnea was reduced in the NHFV group [MD (95% CI): −4.53 h (−5.64 to −3.42)].
Another RCT evaluated the benefit of prophylactic CPAP for 20 minutes in the DR following elective lower segment caesarean section in late preterm and term neonates. The comparator was standard care. The authors concluded that prophylactic CPAP significantly decreased the need for NICU admission (3% vs. 8.8%, p = 0.04) (58).
CPAP for meconium aspiration syndrome (MAS)
CPAP may be of benefit in neonates with MAS. It possibly helps in maintaining FRC as alveoli are known to undergo atelectasis in MAS (59). A multi-centre RCT enrolling 135 neonates compared CPAP to standard care in neonates with moderate to severe respiratory distress due to MAS (60). The study reported that CPAP resulted in a decreased risk of IMV [3% vs. 25%, odds ratio (95% CI): 0.09 (0.02–0.43), p < 0.01].
HHHFNC
HHHFNC works on the principle of providing inhaled gases at a flow higher than the neonate's innate inspiratory flow. This aids in reducing the physiological dead space in the upper airways. HHHFNC consists of 4 basic components: an air oxygen blender for titration of the fraction of inspired oxygen (FiO2) (61), a heating and humidification system which prevents upper airway damage by cold and dry gases, a high flow gas delivery system which delivers gases at flow rates of 2–8 litres/minute and a nasal cannula of varying sizes that occupy less than 50% of the neonates' nares.
The mechanism of action of HHHFNC is multi-factorial. Provision of continuous distending pressure which increases the FRC is one amongst them. It has been reported that distending pressure generated by HHHFNC might be similar to that generated by CPAP (62–67). A linear relationship between the flow rate and the distending pressure generated has been outlined in multiple studies (62, 66, 68). It has also been reported that a similar extent of distending pressure could be generated at relatively lower flow rates in preterm neonates (62, 65). Washout of upper airway dead space is another proposed mechanism of action of HHHFNC. Pre-clinical studies have shown that increased flow rates with HHHFNC result in lower carbon dioxide (CO2) levels (69, 70). Sivieri et al. in their in-vitro study reported better CO2 elimination with HHHFNC when compared to CPAP (70). Preterm neonates have an immature mucocilliary transport system which predisposes them to airway damage. The delivery of heated humidified gases not only protect their immature airway, but also decreases the energy expenditure (71, 72). All these result in decreased work of breathing (73).
HHHFNC for DR stabilization
One in ten newly born infants requires respiratory assistance at birth (74). Various academic societies recommend DR CPAP in preterm neonates with respiratory distress (74, 75). HHHFNC has also been studied for respiratory stabilization in the DR.
A recently published RCT compared HHHFNC vs. CPAP for DR stabilization (76). Spontaneously breathing neonates of 28–36 weeks' GA were enrolled in the study (n = 124). Treatment failure was defined as the need for intubation or upgradation of support within the first 72 h. The authors reported that the non-inferiority of HHHFNC could not be conclusively proven as the 95% CI crossed both the line of no effect and the non-inferiority margin of 10%. There was no statistically significant difference in treatment failure rate in the HHHFNC group (13.1%) compared to the CPAP group (11.1%) [(Risk Difference (RD) (95% CI): 2% (−9.9% to 14.07%), p = 0.73)]. There were also no differences in any of the secondary outcomes of nasal injury, air leaks, time to treatment failure, duration of respiratory support and FiO2 requirement. It is to be noted that the study was underpowered to assess the primary outcome.
Another single-centre retrospective cohort study reported their experience of using HHHFNC for DR stabilisation over a period of 5 years (77). This study evaluated 292 neonates of less than 32 weeks' GA. The authors reported that 45% of these neonates required surfactant therapy. Also, more than three-fourths of these neonates either stayed on HHHFNC or required a lesser form of respiratory support. BPD developed in 36% of the survivors. A pilot study by Reynolds et al. (78) also reported the safe use of HHHFNC for DR stabilisation and transport in newly born preterm infants of less than 30 weeks' gestation. Further, 60% of these neonates did not require any upgradation of respiratory support in the first 72 h of life.
HHHFNC for primary respiratory support
HHHFNC has been compared with CPAP as a primary respiratory support modality for preterm infants diagnosed with respiratory distress soon after birth. Several systematic reviews have been performed within the last 4 years with varying results (Table 1) (79–84). Most of these systematic reviews included preterm neonates of <37 weeks' GA. Only a limited number of studies enrolled extremely low gestational age neonates' (ELGANs) of less than 28 weeks' GA. The primary outcomes evaluated in most of these systematic reviews were quite variable. The Cochrane review reported on the outcomes of risk of mortality or BPD, mortality, BPD, treatment failure within the first 72 h and the need for IMV (80). The authors found that the use of HHHFNC as a primary respiratory support did not result in any significant difference in most of the outcomes except for a higher treatment failure rate in the HHHFNC group when compared to the CPAP group. Also, HHHFNC was associated with a decreased risk of nasal injury when compared to CPAP (Table 2). The review also compared the use of HHHFNC vs. NIPPV for primary respiratory support in preterm neonates. The authors reported there was no statistically significant difference between the two groups for the combined outcome of mortality or BPD, mortality, treatment failure, need for IMV and occurrence of pneumothorax. A similar finding of reduced risk of nasal injury in the HHHFNC group was also reported when compared to NIPPV [RR (95% CI): 0.21(0.09–0.47)].
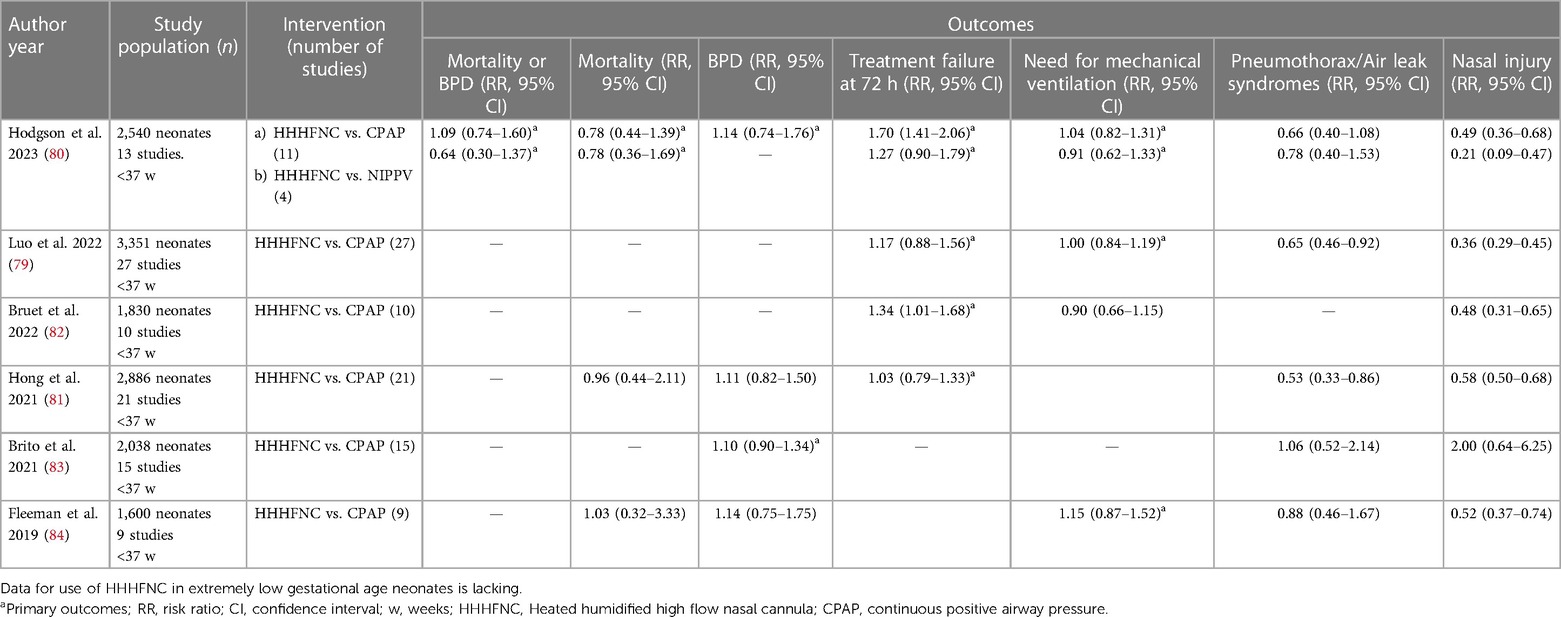
Table 2. Summary of recent meta-analyses comparing HHHFNC and CPAP as primary respiratory support modality.
The systematic review by Luo et al. (79), included an extensive literature search from Chinese databases. This systematic review included a total of 27 studies enrolling 3,351 preterm neonates. The authors reported that HHHFNC use was associated with similar rates of treatment failure and the need for IMV when compared to CPAP (Table 2). HHHFNC also resulted in a lower risk of air leak syndromes, nasal injury, decreased duration of oxygen support and earlier initiation of enteral feeding. A sensitivity analysis was performed after the exclusion of studies with a high risk of bias. The results pertaining to the outcome of decreased duration of oxygen support and earlier initiation of enteral feeding were contradictory. Bruet et al. (82), in their systematic review including 10 studies reported a higher risk of treatment failure with HHHFNC use when compared to CPAP when used as primary support. However, the need for IMV was similar. Further, the authors reported a lower risk of nasal injury with HHHFNC (Table 2). The authors also performed meta-regressions and did not find any significant effects of gestational age, birth weight, flow rates used for HHHFNC, or the type of CPAP devices used on the outcomes.
In conclusion, the use of HHHFNC as primary support in infants with ≥28 weeks GA may not result in any difference in the combined outcome of mortality or BPD when compared to CPAP. However, HHHFNC might be associated with an increased risk of need for upgradation of respiratory support within 72 h of initiation. There is no robust evidence for the use of HHHFNC as primary respiratory support in ELGANs.
HHHFNC for post extubation support
HHHFNC has also been used as a weaning modality after extubation from IMV and its efficacy has been compared with other modes of NIV (81, 83–87). The recently published meta-analysis by Martins et al. (85) (7 studies, 1,044 infants) compared HHHFNC vs. CPAP. The primary outcomes were extubation failure rates at 72 h and 7 days. They reported no significant difference in the primary outcomes. The other outcomes of mortality, BPD, air leak syndrome, pneumothorax and abdominal distension were also similar between the groups (Table 3). As observed in the other systematic reviews on primary respiratory support, HHHFNC was associated with a decreased risk of nasal injury. It is to be noted that the studies including both preterm and term infants were evaluated in this review. An insignificant difference in extubation failure rate has been reported by Hong et al. (81) in their meta-analysis as well (10 studies, 1,378 neonates). It was also reported that HHHFNC was associated with significantly lesser nasal injury. Further, fewer patients developed pneumothorax in the HHHFNC group when compared to CPAP. In conclusion, most of the meta-analysis conducted in the last 5 years suggest no difference in extubation failure rates, mortality or BPD. A decreased risk of nasal injury with HHHFNC as a post-extubation respiratory support modality when compared to CPAP has been reported by most of these meta-analyses (Table 3). Likewise for primary respiratory support, the use of HHHFNC as a post-extubation modality requires further trials in the sub-group of ELGANs.
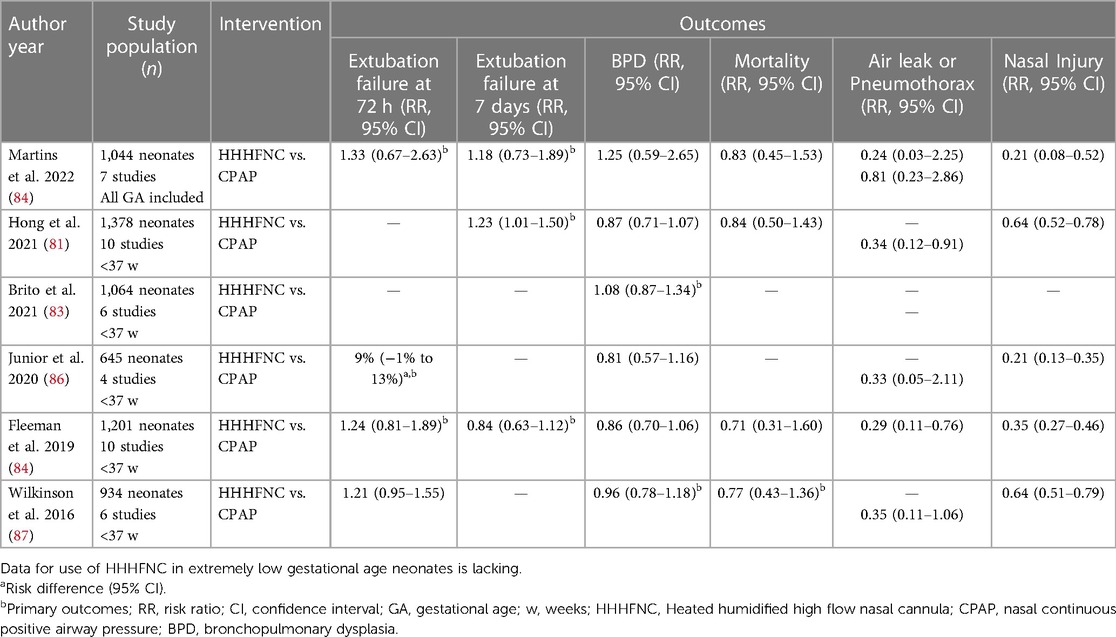
Table 3. Summary of recent meta-analyses comparing HHHFNC vs. CPAP as a respiratory support modality in the post-extubation setting.
HHHFNC as a weaning mode from other NIV modalities
There is no consensus on the timing or method of weaning from various NIV respiratory support modalities (88–90). Various methods like abrupt stoppage of CPAP, gradual lowering of PEEP, intermediate stoppage of CPAP for some time followed by re-application, weaning to HHHFNC, weaning to low flow nasal cannula or head box oxygen have been tried. But none of the strategies have been conclusively shown to be better (41). A recent meta-analysis of 15 trials enrolling 1,547 neonates assessed the various weaning strategies from NIV in preterm neonates (91). The primary outcomes of this systematic review were successful weaning at the first attempt and risk of weaning failure. It was reported that there was no significant difference in successful weaning at the first attempt when a gradual decrease of pressure was used in comparison to sudden discontinuation of CPAP [2 studies, 422 neonates, RR (95% CI): 1.30 (0.93–1.83)]. The postmenstrual age at which successful weaning was achieved was approximately 3 weeks lesser when HHHFNC was used for weaning from CPAP compared to sudden discontinuation of CPAP [MD (95% CI): −2.7 weeks (−3.87 to −1.52)]. However, there was no significant difference in weaning failure rate at first attempt between the step-down strategy and the abrupt stopping of CPAP [4 trials, 327 infants; RR (95% CI): 1.25 (0.79–1.97)]. None of the weaning strategies had any bearing on the incidence of BPD or length of hospital stay (91).
HHHFNC during endotracheal intubation
Intubation of a hypoxemic neonate is often associated with adverse life-threatening events. The use of HHHFNC during endotracheal intubation has been compared to standard care (no nasal high flow or use of supplemental oxygen) in a recently published RCT (92). With the data derived from 251 intubations in 202 infants, the likelihood of successful intubation on the first attempt without physiological instability was significantly higher in the HHHFNC group compared to standard one (50.0% vs. 31.5%, adjusted RD, 17.6 percentage points; 95% CI, 6.0–29.2) with the number needed to treat (NNT) being 6 (95% CI, 4–17) (92).
BiPAP
In this form of non-invasive respiratory support, a low and a high pressure is delivered to the neonatal airway at predefined rates through a nasal interface. The difference between the two pressure levels is usually 4 cm of H2O or less. The rate of delivery of the higher pressure range between 10 and 30 per minute, and the inspiratory time (Ti) is set between 0.5–1 s (93). This device needs an added pressure level as well as cycling of pressures at predefined times. There are specific machines that deliver BiPAP alone. Some ventilators also have the provision for BiPAP. Most machines that provide BiPAP can deliver a maximum positive inspiratory pressure of 15 cm H2O. BiPAP may provide an added benefit over CPAP by delivering a higher mean airway pressure. This might translate to better recruitment of alveoli. However, this is limited by the lack of appropriate measures for synchronisation with the neonate's spontaneous breathing efforts.
Recently, a meta-analysis was performed to compare the efficacy of BiPAP with CPAP. It was found that there was no significant difference in the duration of IMV [MD (95% CI): 0.04 days (−0.31 to 0.39)], incidence of BPD [RR (95% CI): 0.98 (0.60–1.59)], extubation failure or death [RR (95% CI): 1.00 (0.81–1.23)], death [RR (95% CI): 0.62 (0.15–2.48)] (94). This meta-analysis included 4 RCTs which had predominantly included preterm neonates born between 28 and 34 weeks' GA. An RCT comparing BiPAP with CPAP for management of RDS enrolled 85 very low birth weight (VLBW) neonates. This trial reported a reduction in treatment failure rates (4.4% vs. 22.5%) and apnea episodes (13.3% vs. 32.5%) with BiPAP use (95).
Another RCT which enrolled 540 neonates of less than 30 weeks' GA compared BiPAP vs. CPAP for post-extubation support. The authors reported similar outcomes for treatment failure [21% vs. 20%, adjusted odds ratio (95% CI): 1.01 (0.65–1.56), p = 0.97] (96).
NIPPV
This form of non-invasive ventilation involves delivery of varying inspiratory and positive end-expiratory pressures (PEEP) with time cycling at pre-defined rates. The major differences from BiPAP are that the difference between peak inspiratory pressure (PIP) and PEEP can be above 4–5 cm of H2O, it utilises lower inspiratory times, higher respiratory rates, and can be synchronised or non-synchronised with the neonate's spontaneous respiratory effort (97).
NIPPV functions through multiple physiological mechanisms. It provides PEEP similar to CPAP leading to all the benefits ascertained to CPAP. In addition, it delivers PIP which is not provided by CPAP. This results in a higher mean airway pressure than that achieved with CPAP leading to better recruitment of alveoli (96). This also helps in better CO2 removal. The two major forms of NIPPV available are synchronised NIPPV (sNIPPV) and non-synchronised NIPPV (nsNIPPV). A post-extubation study among VLBW neonates had demonstrated decreased inspiratory work of breathing as monitored by esophageal manometry. Further, an improved tidal volume delivery was demonstrated with the use of sNIPPV when compared to CPAP (98). This study also reported improved minute ventilation at lower respiratory rates with sNIPPV use. NIPPV also improves FRC, reduces bradycardia and apnea events (99). Head's paradoxical reflex is considered to be an important physiological mechanism in NIPPV (100). This is an increase in inspiratory reflex in response to inflation of the pharynx by the pressure delivered during NIPPV (99, 100).
Delivery of non-synchronised breaths is bound to cause discomfort to neonates. Few studies have shown that improved ventilation occurs only with the synchronised breaths delivered during nsNIPPV (98, 99, 101, 102). Four types of triggering devices are used for achieving synchronisation in NIPPV. These are the abdominal capsule (Graseby capsule), pressure trigger device, flow trigger device and NAVA trigger device. The abdominal capsule detects the spontaneous effort by sensing abdominal excursion resulting in a delayed response, and hence causing inappropriate synchronisation (especially in neonates with tachypnea) (103, 104). Pressure trigger devices cannot be used for preterm neonates due to their poor inspiratory drive. A pressure trigger device delivers breath In response to decrease in pressure that occurs with a patient's inspiratory effort (101). A flow trigger is ubiquitous in neonatal ventilators for delivery of IMV. But the same flow sensor cannot be used for NIPPV as these circuits have significant leaks at the interface or when the neonate opens the mouth. A pneumotachograph provides a solution to this problem. When it is attached to a software it helps to detect a sudden change in flow with inspiratory effort, and hence circumvents the gradual continuous change in flow caused by leaks in the system (105). This device has been shown to be effective in reducing the need for intubation in neonates (106, 107). The most recent advancement in the field of NIV has been NAVA which synchronises delivered breaths to the electrical signal attained from the neonate's diaphragm making the synchronisation close to the ideal (108). This is made possible by the presence of sensors in a nasogastric tube inserted specifically for this purpose. These devices have also been shown to be effective in decreasing the risk of intubation and extubation failure in preterm neonates with RDS (109).
Interfaces for NIPPV are similar to those used with CPAP (100). A recently conducted non-inferiority trial reported that CLNT (RAM cannula, Neotech, Valencia) was comparable to short binasal prongs for delivering NIPPV in preterm neonates of 24 to 33 weeks' GA (110). 166 preterm neonates were randomized in the study and 14% neonates in CLNT group required intubation within 72 h as compared to 18% in the short binasal prongs group [RD (95% CI): −3.6% (−14.8 to 7.6), within the non-inferiority margin]. The incidence of moderate to severe nasal injury was lower in the CLNT group (5% vs. 17%, p = 0.01), but there was no difference in the other outcomes. On the other hand, another RCT randomized 126 preterm neonates to receive NIPPV by short binasal prongs and CLNT (RAM cannula, Neotech, Valencia), and reported a higher need for IMV (32.8% vs. 9.6%, p = 0.002) and surfactant administration (42.1% vs. 19.3%, p = 0.07) in the CLNT group (111). A meta-analysis (3 RCTs and 3 observational studies) stated that clinical benefit or harm could not be ruled out for the outcome of the need for IMV for CLNT (RAM cannula, Neotech, Valencia) vs. short binasal prongs or nasal mask [RR (95% CI): 1.37 (0.61–3.04)] (112). The final verdict on whether one is better than the other still awaits us as more RCTs are needed to compare the two in various disease settings.
NIPPV as primary respiratory support for RDS
A recent NMA comparing different methods of NIV in preterm neonates for primary respiratory support for RDS assessed 35 studies enrolling 4,078 preterm neonates with a mean GA of 31 weeks (113). NIPPV was found to be more likely to prevent treatment failure and decrease the need for IMV when compared to HHHFNC and CPAP, and was comparable to BiPAP in its efficacy (Table 4). The combined outcome of death or BPD was significantly lower with NIPPV use compared to CPAP. NIPPV was also associated with decreased incidence of air leaks compared to CPAP and BiPAP. The authors also reported that sNIPPV, nsNIPPV and BiPAP individually also decreased the need for IMV [RR (95% CrI): 0.54 (0.32–0.90), 0.61 (0.43–0.83), 0.51 (0.29–0.85)] and treatment failure when compared to CPAP (113). The authors however did not differentiate between the various forms of CPAP delivery systems. Further, a subgroup analysis for ELGANs was not performed.
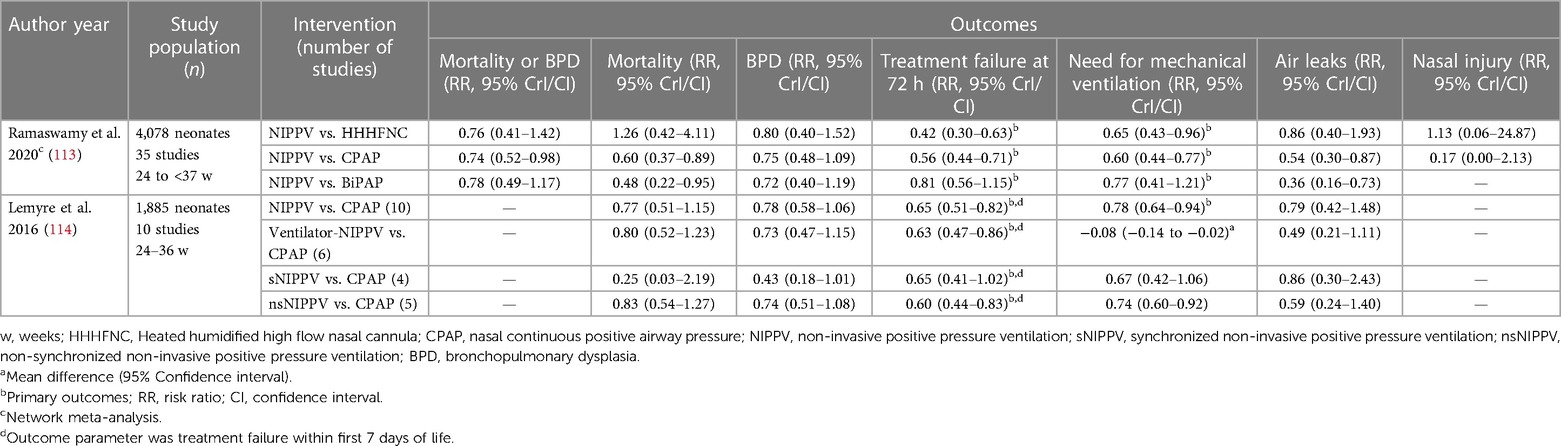
Table 4. Summary of recent meta-analyses comparing NIPPV to other non-invasive ventilation techniques as primary respiratory support modality.
An earlier Cochrane meta-analysis had reported outcomes while comparing synchronised, unsynchronised and ventilator-driven NIPPV with CPAP (114). The review included 10 studies and 1,885 preterm neonates (Table 4). The results of this meta-analysis also showed that NIPPV decreased the treatment failure rates and the need for IMV when compared to CPAP. An unexpected finding in the meta-analysis was the reduced treatment failure and IMV rate with nsNIPPV compared to CPAP, but not with sNIPPV. Other outcomes such as mortality, BPD and air leaks were similar. Benefit of NIPPV over CPAP for the subgroup of ELGANs could not be determined by the authors.
NIPPV for post extubation support
Another NMA by Ramaswamy et al. compared various NIV modalities for post-extubation respiratory support (115). The NMA included 31 studies enrolling 3,899 neonates. The authors reported that the primary outcome of requirement for re-intubation was decreased with the use of nsNIPPV, sNIPPV, NHFV and variable flow CPAP (VFCPAP) when compared to constant flow CPAP (CFCPAP) [RR (95% CrI): 0.44 (0.27–0.67), 0.22 (0.12–0.35), 0.42 (0.18–0.81), 0.73 (0.52–0.99)]. Both sNIPPV and nsNIPPV were better than BiPAP in decreasing the risk of the primary outcome. sNIPPV was ranked as the best intervention as per the surface under the cumulative ranking (SUCRA) values. The authors also reported that nsNIPPV decreased re-intubation rates when compared to VFCPAP and HFNC. sNIPPV also reduced the risk of BPD and air leak compared to nsNIPPV, VFCPAP and CFCPAP. A Cochrane meta-analysis (2017) reported a reduction in the incidence of mortality, respiratory failure and the need for IMV with the use of NIPPV when compared to CPAP (116) (Table 5). Both these reviews did not analyse the ELGAN sub-group separately.
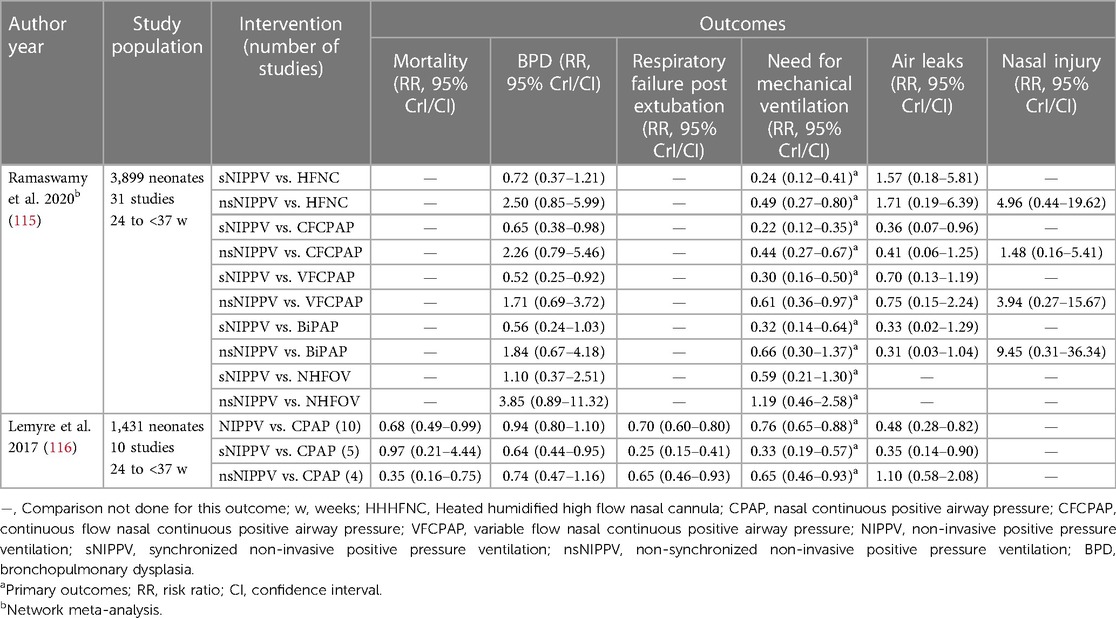
Table 5. Summary of recent meta-analysis comparing NIPPV to other non-invasive ventilation techniques for post extubation support.
Non-invasive ventilation with neurally adjusted ventilatory assist (NIV-NAVA)
As described earlier, NAVA is a technique for achieving synchronisation between the ventilator breaths and neonatal spontaneous effort by assessing the electrical activity of diaphragm (EAdi). This helps in overcoming the major challenges with the synchronisation of NIV in neonates. The synchronisation is expected to be near ideal as both the diaphragm and the respiratory support device are acting upon the same electrical signal reaching the diaphragm (117). NIV-NAVA has been shown to reduce the PIP requirement and improve oxygenation in preterm neonates (118, 119). Firestone et al. reported in their retrospective study that NIV-NAVA reduces the risk of apnea when compared to CPAP (109). Another retrospective study reported a reduction in extubation failure rates with NIV-NAVA when compared to CPAP in preterm neonates (6.3% vs. 37.5%, p = 0.041) (120). The nasal interfaces used with NAVA are similar to those used with other forms of NIV with the addition of a nasogastric tube housing the electrodes for assessing EAdi.
Commonly used initial settings for NIV-NAVA include an appropriate level of PEEP, a PIP which is 5–8 cm H2O above the set PEEP, and a predefined respiratory rate which is set to provide ventilation to the neonate as a backup for apnea (121). The most commonly used EAdi trigger level to initiate a breath is 0.5 microvolt.
Multiple observational studies and RCTs have been conducted comparing NIV-NAVA with other modes of NIV. A retrospective review studying ELGANs showed that NIV-NAVA can be safely used for post-extubation support (74% neonates extubated from IMV after surfactant administration did not require reintubation) (122). A Cochrane meta-analysis (2020) compared the efficacy of NIV-NAVA with other types of non-invasive respiratory support in neonates (123). Only 2 RCTs (23 neonates, >28 weeks' GA) were eligible for inclusion. 1 RCT (16 neonates) reported no significant difference in treatment failure rate between NIV- NAVA and NIPPV [RR (95% CI): 0.33 (0.02–7.14)]. NIV-NAVA was also shown to be associated with significantly increased respiratory rate [MD (95% CI): 7.22 breaths/minute (0.21–14.22)] compared to NIPPV. However, the maximum EAdi signal was similar in both the groups [MD (95% CI): −1.75 microvolt (−3.75 to 0.26)].
Nasal high-frequency ventilation (NHFV)
Incidence of BPD has stayed stable despite rapid advancements in treatment strategies for preterm neonates such as the use of non-invasive support, DR CPAP, early selective surfactant with less invasive surfactant administration technique, avoidance of IMV, use of volume-targeted ventilation and timely administration of medications like corticosteroids and caffeine (124, 125). Most likely cause for this appears to be the increased survival of ELGANs. Hence, there has been a continual search for other provisions that could decrease the incidence of BPD. Amongst the non-invasive respiratory support strategies, NIV-NAVA and NHFV are the most focused ones as of present. NFHV is expected to negate the effect of patient-ventilator asynchrony, and also improve CO2 removal from the lungs (126, 127). There are 2 main types of NHFV: NHFOV and non-invasive high-frequency percussive ventilation (NHFPV). The former is the most researched one in neonatal medicine.
NHFOV encompasses the delivery of constant distending pressure with pressure amplitude oscillations at a very high rate. Hence, a higher mean airway pressure can be delivered along with adequate carbon dioxide elimination with NHFOV (128). Settings, titration of pressure and amplitude in NHFOV is similar to that in invasive HFOV (129). The interfaces used are similar to the ones used with other NIV devices, but with a few cautionary points. Short binasal prongs and nasal masks are the most favoured interfaces due to reliable tidal volume generation and oscillation transmission (130, 131). CLNT is not preferred for NHFOV as it is shown to increase the work of breathing for the neonate (132).
Multiple observational studies have reported the benefits of NHFOV for neonatal respiratory support. It has been reported that NHFOV use results in decreased treatment failure or need for re-intubation, reduces apnea episodes and improves ventilation without any significant adverse effects (133–135). A recent meta-analysis of 10 RCTs (681 neonates) revealed that NHFOV use is associated with significantly lower need for intubation and IMV [Odds Risk (95% CI): 0.29 (0.2–0.4)] when compared to BiPAP and CPAP (132). Three RCTs included ELGANs in this meta-analysis. This meta-analysis also reported that NHFOV is also associated with improved CO2 removal when compared to BiPAP and CPAP [MD (95% CI): −0.46 mm Hg (−0.93 to −0.08)]. These results stayed significant even after adjusting for GA and the use of antenatal corticosteroids (132).
Temperature and humidity during NIV
The upper respiratory passages perform an important function of heating the ambient air to body temperature and humidifying it to prevent injury to the lower respiratory tract (136). Most of this function is performed in the nasal septum and the conchae (136) which are totally bypassed during IMV by an endotracheal tube. In NIV, the heating and humidification functioning of the upper airway is overwhelmed due to the high gas flow rates delivered by it. This predisposes the neonate to barotrauma, volutrauma and hypothermia along with an increased risk of mortality in preterm neonates due to cold and dry respiratory gases (137). Hence, respiratory gases in IMV need to be warmed up to 37 degrees Celsius and humidified to attain a relative humidity of 100% (136). Similar targets are recommended for most NIV respiratory modalities as their interface and the gas flow rates bypass the innate upper respiratory conditioning function system of the neonate (138). A recent review (23 studies, preterm neonates) revealed that most NIV setups fail to attain the desired inspiratory gas temperature and humidity (138). Moreover, many studies have indicated that the environmental air temperature can have a major effect on the inspiratory gas temperature. The use of radiant warmer can also affect respiratory gas conditioning as indicated by studies comparing the inspiratory gas temperature of neonates nursed in radiant warmer to those nursed in incubators. A decreased inspiratory temperature and humidity of the delivered gas in the radiant warmer group was reported by one study (138).
Pain management in neonates on NIV
NIV can cause significant discomfort and pain for a preterm neonate. The plausible reasons could be the asynchrony spontaneously breathing neonates, abutment of the nasal interface, and nasal injury (139). Persistent pain in the early neonatal period has been shown to have long-lasting effects, especially on long-term neurodevelopment (140). The first step in attaining adequate neonatal comfort on NIV is choosing an appropriate modality of NIV which is individualized to the neonate's need. This includes the usage of more comfortable devices like HHHFNC compared to CPAP, or interfaces such as CLNT or nasal mask to prevent nasal injury. Also, appropriate synchronization using sNIPPV, NIV-NAVA or NHFV may also decrease the discomfort. Evidence of the efficacy and safety of these modalities have been discussed earlier. A systematic review assessed 45 studies and reported that nasal injury can be reduced through the use of barrier dressings in neonates on NIV [RD (95% CI): −0.12 (−0.20 to −0.04)], and by preferring HHHFNC or nasal mask over short binasal prongs (139). This should be accompanied by timely identification of stress, discomfort or pain in the neonate by the routine use of clinical pain scores. Neonates with ongoing NIV should be treated with non-pharmacological means when the first signs of pain appear (141). This could be followed by the use of analgesic medications if needed. Routine use of analgesia for neonates on NIV is an evolving and controversial subject as the evidence for the same is insufficient. The EUROPAIN cohort study reported that 18% of neonates on IMV and 9% neonates on NIV received analgesia or sedative medications (142). Paracetamol (11%) was the most commonly used medication followed by opioids (6%). Only 45% of the neonates on NIV had pain assessments documented. Dexmedetomidine use in neonates is currently on the rise (143) and preliminary reports suggest that it is safe and efficacious for neonates (144). Overall, there is a gap in the literature on the use of analgesia or sedation in neonates on NIV.
Conclusions
A holistic approach is needed in the care of preterm neonates to improve their short- and long-term outcomes. NIV is one of the most important components of preterm respiratory care. For most indications, sNIPPV seems to be the most efficacious, and HHHFNC being associated with the least likelihood of nasal injury. Adequately powered RCTs are required to evaluate newer modalities of NIV such as NIV-NAVA and NHFV. Though multiple systematic reviews and meta-analyses have indicated the superiority of one NIV modality over the other in neonates, there is no single NIV modality that universally suits all. Hence, the choice of NIV for a neonate should be individualized based on its efficacy, the disease pathology, resource settings, clinician's familiarity and parental values. Finally, further robust adequately powered trials are needed to compare these modalities in the most vulnerable sub-group of preterm neonates, the ELGAN population.
Author contributions
VR conceptualized the manuscript. RD and GK produced the initial draft. VR revised the initial draft by providing further intellectual inputs. All authors contributed to the article and approved the submitted version.
Acknowledgments
We thank the authors of the original research included in this review.
Conflict of interest
The authors declare that the research was conducted in the absence of any commercial or financial relationships that could be construed as a potential conflict of interest.
Publisher's note
All claims expressed in this article are solely those of the authors and do not necessarily represent those of their affiliated organizations, or those of the publisher, the editors and the reviewers. Any product that may be evaluated in this article, or claim that may be made by its manufacturer, is not guaranteed or endorsed by the publisher.
References
1. Chawanpaiboon S, Vogel JP, Moller AB, Lumbiganon P, Petzold M, Hogan D, et al. Global, regional, and national estimates of levels of preterm birth in 2014: a systematic review and modelling analysis. Lancet Glob Health. (2019) 7(1):e37–46. doi: 10.1016/S2214-109X(18)30451-0
2. Perin J, Mulick A, Yeung D, Villavicencio F, Lopez G, Strong KL, et al. Global, regional, and national causes of under-5 mortality in 2000-19: an updated systematic analysis with implications for the sustainable development goals. Lancet Child Adolesc Health. (2022) 6(2):106–15. doi: 10.1016/S2352-4642(21)00311-4
3. Dyer J. Neonatal respiratory distress syndrome: tackling a worldwide problem. Pharm Ther. (2019) 44(1):12–4. PMID: 30675087
4. Kamath BD, Macguire ER, McClure EM, Goldenberg RL, Jobe AH. Neonatal mortality from respiratory distress syndrome: lessons for low-resource countries. Pediatrics. (2011) 127(6):1139–46. doi: 10.1542/peds.2010-3212
5. Van Marter LJ, Allred EN, Pagano M, Sanocka U, Parad R, Moore M, et al. Do clinical markers of barotrauma and oxygen toxicity explain interhospital variation in rates of chronic lung disease? The neonatology committee for the developmental network. Pediatrics. (2000) 105(6):1194–201. doi: 10.1542/peds.105.6.1194
6. Laughon M, Bose C, Allred EN, O'Shea TM, Ehrenkranz RA, Van Marter LJ, et al. Antecedents of chronic lung disease following three patterns of early respiratory disease in preterm infants. Arch Dis Child Fetal Neonatal Ed. (2011) 96(2):F114–20. doi: 10.1136/adc.2010.182865
7. Homan TD, Nayak RP. Short- and long-term complications of bronchopulmonary dysplasia. Respir Care. (2021) 66(10):1618–29. doi: 10.4187/respcare.08401
8. Courtney SE, Durand DJ, Asselin JM, Hudak ML, Aschner JL, Shoemaker CT, et al. High-frequency oscillatory ventilation versus conventional mechanical ventilation for very-low-birth-weight infants. N Engl J Med. (2002) 347(9):643–52. doi: 10.1056/NEJMoa012750
9. Ramanathan R. Optimal ventilatory strategies and surfactant to protect the preterm lungs. Neonatology. (2008) 93(4):302–8. doi: 10.1159/000121456
10. Klingenberg C, Wheeler KI, McCallion N, Morley CJ, Davis PG. Volume-targeted versus pressure-limited ventilation in neonates. Cochrane Database Syst Rev. (2017) 10(10):CD003666. doi: 10.1002/14651858.CD003666.pub4
11. Avery ME, Tooley WH, Keller JB, Hurd SS, Bryan MH, Cotton RB, et al. Is chronic lung disease in low birth weight infants preventable? A survey of eight centers. Pediatrics. (1987) 79(1):26–30. doi: 10.1542/peds.79.1.26
12. Bhandari V. The potential of non-invasive ventilation to decrease BPD. Semin Perinatol. (2013) 37(2):108–14. doi: 10.1053/j.semperi.2013.01.007
13. Gregory GA, Edmunds LH, Kitterman JA, Phibbs RH, Tooley WH. Continuous positive airway pressure and pulmonary and circulatory function after cardiac surgery in infants less than three months of age. Anesthesiology. (1975) 43(4):426–31. doi: 10.1097/00000542-197510000-00008
14. Kattwinkel J, Nearman HS, Fanaroff AA, Katona PG, Klaus MH. Apnea of prematurity. Comparative therapeutic effects of cutaneous stimulation and nasal continuous positive airway pressure. J Pediatr. (1975) 86(4):588–92. doi: 10.1016/S0022-3476(75)80158-2
15. Caliumi-Pellegrini G, Agostino R, Orzalesi M, Nodari S, Marzetti G, Savignoni PG, et al. Twin nasal cannula for administration of continuous positive airway pressure to newborn infants. Arch Dis Child. (1974) 49(3):228–30. doi: 10.1136/adc.49.3.228
16. Yu VY, Rolfe P. Effect of continuous positive airway pressure breathing on cardiorespiratory function in infants with respiratory distress syndrome. Acta Paediatr Scand. (1977) 66(1):59–64. doi: 10.1111/j.1651-2227.1977.tb07808.x
17. Saunders RA, Milner AD, Hopkin IE. The effects of continuous positive airway pressure on lung mechanics and lung volumes in the neonate. Biol Neonate. (1976) 29(3–4):178–86. doi: 10.1159/000240862
18. Heldt GP, McIlroy MB. Distortion of chest wall and work of diaphragm in preterm infants. J Appl Physiol Bethesda Md. (1987) 62(1):164–9. doi: 10.1152/jappl.1987.62.1.164
19. Courtney SE, Aghai ZH, Saslow JG, Pyon KH, Habib RH. Changes in lung volume and work of breathing: a comparison of two variable-flow nasal continuous positive airway pressure devices in very low birth weight infants. Pediatr Pulmonol. (2003) 36(3):248–52. doi: 10.1002/ppul.10327
20. Locke R, Greenspan JS, Shaffer TH, Rubenstein SD, Wolfson MR. Effect of nasal CPAP on thoracoabdominal motion in neonates with respiratory insufficiency. Pediatr Pulmonol. (1991) 11(3):259–64. doi: 10.1002/ppul.1950110313
21. Zhang S, Garbutt V, McBride JT. Strain-induced growth of the immature lung. J Appl Physiol Bethesda Md 1985. (1996) 81(4):1471–6. doi: 10.1152/jappl.1996.81.4.1471
22. Aly HZ. Nasal prongs continuous positive airway pressure: a simple yet powerful tool. Pediatrics. (2001) 108(3):759–61. doi: 10.1542/peds.108.3.759
23. Gie AG, Hubble TR, Regin Y, Salaets T, Zamora M, Deprest J, et al. A systematic review of the influence of continuous positive airway pressure on fetal and newborn animal models: suggestions to improve neonatal respiratory care. Neonatology. (2021) 118(1):5–14. doi: 10.1159/000511086
24. Moa G, Nilsson K, Zetterström H, Jonsson LO. A new device for administration of nasal continuous positive airway pressure in the newborn: an experimental study. Crit Care Med. (1988) 16(12):1238–42. doi: 10.1097/00003246-198812000-00013
25. Pandit PB, Courtney SE, Pyon KH, Saslow JG, Habib RH. Work of breathing during constant- and variable-flow nasal continuous positive airway pressure in preterm neonates. Pediatrics. (2001) 108(3):682–5. doi: 10.1542/peds.108.3.682
26. Drevhammar T, Berg N, Nilsson K, Jonsson B, Prahl Wittberg L. Flows and function of the infant flow neonatal continuous positive airway pressure device investigated with computational fluid dynamics. Acta Paediatr. (2021) 110(3):811–7. doi: 10.1111/apa.15502
27. Prakash R, De Paoli AG, Davis PG, Oddie SJ, McGuire W. Bubble devices versus other pressure sources for nasal continuous positive airway pressure in preterm infants. Cochrane Database Syst Rev. (2023) 3(3):CD015130. doi: 10.1002/14651858.CD015130
28. Bharadwaj SK, Alonazi A, Banfield L, Dutta S, Mukerji A. Bubble versus other continuous positive airway pressure forms: a systematic review and meta-analysis. Arch Dis Child Fetal Neonatal Ed. (2020) 105(5):526–31. doi: 10.1136/archdischild-2019-318165
29. Kaur C, Sema A, Beri RS, Puliyel JM. A simple circuit to deliver bubbling CPAP. Indian Pediatr. (2008) 45(4):312–4. PMID: 18451452
30. Koyamaibole L, Kado J, Qovu JD, Colquhoun S, Duke T. An evaluation of bubble-CPAP in a neonatal unit in a developing country: effective respiratory support that can be applied by nurses. J Trop Pediatr. (2006) 52(4):249–53. doi: 10.1093/tropej/fmi109
31. McAdams RM, Hedstrom AB, DiBlasi RM, Mant JE, Nyonyintono J, Otai CD, et al. Implementation of bubble CPAP in a rural Ugandan neonatal ICU. Respir Care. (2015) 60(3):437–45. doi: 10.4187/respcare.03438
32. Bordessoule A, Moreira A, Civitillo CF, Combescure C, Polito A, Rimensberger PC. Comparison of inspiratory effort with three variable-flow nasal continuous positive airway pressure devices in preterm infants: a cross-over study. Arch Dis Child Fetal Neonatal Ed. (2021) 106(4):404–7. doi: 10.1136/archdischild-2020-320531
33. Nzegwu NI, Mack T, DellaVentura R, Dunphy L, Koval N, Levit O, et al. Systematic use of the RAM nasal cannula in the Yale-New Haven children’s hospital neonatal intensive care unit: a quality improvement project. J Matern-Fetal Neonatal Med Off J Eur Assoc Perinat Med Fed Asia Ocean Perinat Soc Int Soc Perinat Obstet. (2015) 28(6):718–21. doi: 10.3109/14767058.2014.929659
34. De Paoli AG, Davis PG, Faber B, Morley CJ. Devices and pressure sources for administration of nasal continuous positive airway pressure (NCPAP) in preterm neonates. Cochrane Database Syst Rev. (2008) 2008(1):CD002977. doi: 10.1002/14651858.CD002977
35. Prakash R, De Paoli AG, Oddie SJ, Davis PG, McGuire W. Masks versus prongs as interfaces for nasal continuous positive airway pressure in preterm infants. Cochrane Database Syst Rev. (2022) 11(11):CD015129. doi: 10.1002/14651858.CD015129
36. Razak A, Patel W. Nasal mask vs binasal prongs for nasal continuous positive airway pressure in preterm infants: a systematic review and meta-analysis. Pediatr Pulmonol. (2020) 55(9):2261–71. doi: 10.1002/ppul.24878
37. King BC, Gandhi BB, Jackson A, Katakam L, Pammi M, Suresh G. Mask versus prongs for nasal continuous positive airway pressure in preterm infants: a systematic review and meta-analysis. Neonatology. (2019) 116(2):100–14. doi: 10.1159/000496462
38. Jasani B, Ismail A, Rao S, Patole S. Effectiveness and safety of nasal mask versus binasal prongs for providing continuous positive airway pressure in preterm infants-A systematic review and meta-analysis. Pediatr Pulmonol. (2018) 53(7):987–92. doi: 10.1002/ppul.24014
39. Morley CJ, Davis PG, Doyle LW, Brion LP, Hascoet JM, Carlin JB, et al. Nasal CPAP or intubation at birth for very preterm infants. N Engl J Med. (2008) 358(7):700–8. doi: 10.1056/NEJMoa072788
40. Dunn MS, Kaempf J, de Klerk A, de Klerk R, Reilly M, Howard D, et al. Randomized trial comparing 3 approaches to the initial respiratory management of preterm neonates. Pediatrics. (2011) 128(5):e1069–1076. doi: 10.1542/peds.2010-3848
41. SUPPORT Study Group of the Eunice Kennedy Shriver NICHD Neonatal Research Network, Finer NN, Carlo WA, Walsh MC, Rich W, Gantz MG, et al. Early CPAP versus surfactant in extremely preterm infants. N Engl J Med. (2010) 362(21):1970–9. doi: 10.1056/NEJMoa0911783
42. Sandri F, Ancora G, Lanzoni A, Tagliabue P, Colnaghi M, Ventura ML, et al. Prophylactic nasal continuous positive airways pressure in newborns of 28–31 weeks gestation: multicentre randomised controlled clinical trial. Arch Dis Child Fetal Neonatal Ed. (2004) 89(5):F394–398. doi: 10.1136/adc.2003.037010
43. Abelenda VLB, Valente TCO, Marinho CL, Lopes AJ. Effects of underwater bubble CPAP on very-low-birth-weight preterm newborns in the delivery room and after transport to the neonatal intensive care unit. J Child Health Care Prof Work Child Hosp Community. (2018) 22(2):216–27. doi: 10.1177/1367493517752500
44. Subramaniam P, Ho JJ, Davis PG. Prophylactic or very early initiation of continuous positive airway pressure (CPAP) for preterm infants. Cochrane Database Syst Rev. (2021) 10(10):CD001243. doi: 10.1002/14651858.CD001243.pub4
45. Ramaswamy VV, Abiramalatha T, Bandyopadhyay T, Shaik NB, Pullattayil S AK, Cavallin F, et al. Delivery room CPAP in improving outcomes of preterm neonates in low-and middle-income countries: a systematic review and network meta-analysis. Resuscitation. (2022) 170:250–63. doi: 10.1016/j.resuscitation.2021.10.027
46. Shah BA, Fabres JG, Leone TA, Schmölzer GM, Szyld EG, International Liaison Committee on Resuscitation Neonatal Life Support Task Force. Continuous positive airway pressure for term and ≥34+0 weeks’ gestation newborns at birth: a systematic review. Resusc Plus. (2022) 12:100320. doi: 10.1016/j.resplu.2022.100320
47. Smithhart W, Wyckoff MH, Kapadia V, Jaleel M, Kakkilaya V, Brown LS, et al. Delivery room continuous positive airway pressure and pneumothorax. Pediatrics. (2019) 144(3):e20190756. doi: 10.1542/peds.2019-0756
48. Ho JJ, Subramaniam P, Sivakaanthan A, Davis PG. Early versus delayed continuous positive airway pressure (CPAP) for respiratory distress in preterm infants. Cochrane Database Syst Rev. (2020) 10(10):CD002975. doi: 10.1002/14651858.CD002975.pub2
49. Sweet DG, Carnielli VP, Greisen G, Hallman M, Klebermass-Schrehof K, Ozek E, et al. European consensus guidelines on the management of respiratory distress syndrome: 2022 update. Neonatology. (2023) 120(1):3–23. doi: 10.1159/000528914
50. Bamat N, Fierro J, Mukerji A, Wright CJ, Millar D, Kirpalani H. Nasal continuous positive airway pressure levels for the prevention of morbidity and mortality in preterm infants. Cochrane Database Syst Rev. (2021) 11(11):CD012778. doi: 10.1002/14651858.CD012778.pub2
51. Miller MJ, Carlo WA, Martin RJ. Continuous positive airway pressure selectively reduces obstructive apnea in preterm infants. J Pediatr. (1985) 106(1):91–4. doi: 10.1016/S0022-3476(85)80475-3
52. Eichenwald EC, Committee on Fetus and Newborn, American Academy of Pediatrics. Apnea of prematurity. Pediatrics. (2016) 137(1):e20153757. doi: 10.1542/peds.2015-3757
53. Pantalitschka T, Sievers J, Urschitz MS, Herberts T, Reher C, Poets CF. Randomised crossover trial of four nasal respiratory support systems for apnoea of prematurity in very low birthweight infants. Arch Dis Child Fetal Neonatal Ed. (2009) 94(4):F245–248. doi: 10.1136/adc.2008.148981
54. Ho JJ, Subramaniam P, Zakarija-Grkovic I, Leong JJ, Lim E, Lok JW. Continuous positive airway pressure (CPAP) for apnoea of prematurity. Cochrane Database Syst Rev. (2020) 2020(7):CD013660. doi: 10.1002/14651858.CD013660
55. Gizzi C, Klifa R, Pattumelli MG, Massenzi L, Taveira M, Shankar-Aguilera S, et al. Continuous positive airway pressure and the burden of care for transient tachypnea of the neonate: retrospective cohort study. Am J Perinatol. (2015) 32(10):939–43. doi: 10.1055/s-0034-1543988
56. Chiruvolu A, Claunch KM, Garcia AJ, Petrey B, Hammonds K, Mallett LH. Effect of continuous positive airway pressure versus nasal cannula on late preterm and term infants with transient tachypnea of the newborn. J Perinatol Off J Calif Perinat Assoc. (2021) 41(7):1675–80. doi: 10.1038/s41372-021-01068-9
57. Moresco L, Romantsik O, Calevo MG, Bruschettini M. Non-invasive respiratory support for the management of transient tachypnea of the newborn. Cochrane Database Syst Rev. (2020) 4(4):CD013231. doi: 10.1002/14651858.CD013231.pub2
58. Celebi MY, Alan S, Kahvecioglu D, Cakir U, Yildiz D, Erdeve O, et al. Impact of prophylactic continuous positive airway pressure on transient tachypnea of the newborn and neonatal intensive care admission in newborns delivered by elective cesarean section. Am J Perinatol. (2016) 33(1):99–106. doi: 10.1055/s-0035-1560041
59. Goldsmith JP. Continuous positive airway pressure and conventional mechanical ventilation in the treatment of meconium aspiration syndrome. J Perinatol Off J Calif Perinat Assoc. (2008) 28(Suppl 3):S49–55. doi: 10.1038/jp.2008.156
60. Pandita A, Murki S, Oleti TP, Tandur B, Kiran S, Narkhede S, et al. Effect of nasal continuous positive airway pressure on infants with meconium aspiration syndrome: a randomized clinical trial. JAMA Pediatr. (2018) 172(2):161–5. doi: 10.1001/jamapediatrics.2017.3873
61. Agarwal R, Gupta D. What are high-flow and low-flow oxygen delivery systems? Stroke. (2005) 36(10):2066–7. author reply 2067. doi: 10.1161/01.STR.0000185387.51425.f9
62. Wilkinson DJ, Andersen CC, Smith K, Holberton J. Pharyngeal pressure with high-flow nasal cannulae in premature infants. J Perinatol Off J Calif Perinat Assoc. (2008) 28(1):42–7. doi: 10.1038/sj.jp.7211879
63. Collins CL, Holberton JR, König K. Comparison of the pharyngeal pressure provided by two heated, humidified high-flow nasal cannulae devices in premature infants. J Paediatr Child Health. (2013) 49(7):554–6. doi: 10.1111/jpc.12277
64. Dysart K, Miller TL, Wolfson MR, Shaffer TH. Research in high flow therapy: mechanisms of action. Respir Med. (2009) 103(10):1400–5. doi: 10.1016/j.rmed.2009.04.007
65. Kubicka ZJ, Limauro J, Darnall RA. Heated, humidified high-flow nasal cannula therapy: yet another way to deliver continuous positive airway pressure? Pediatrics. (2008) 121(1):82–8. doi: 10.1542/peds.2007-0957
66. Spence KL, Murphy D, Kilian C, McGonigle R, Kilani RA. High-flow nasal cannula as a device to provide continuous positive airway pressure in infants. J Perinatol Off J Calif Perinat Assoc. (2007) 27(12):772–5. doi: 10.1038/sj.jp.7211828
67. Al-Alaiyan S, Dawoud M, Al-Hazzani F. Positive distending pressure produced by heated, humidified high flow nasal cannula as compared to nasal continuous positive airway pressure in premature infants. J Neonatal-Perinat Med. (2014) 7(2):119–24. doi: 10.3233/NPM-1474113
68. Liew Z, Fenton AC, Harigopal S, Gopalakaje S, Brodlie M, O’Brien CJ. Physiological effects of high-flow nasal cannula therapy in preterm infants. Arch Dis Child Fetal Neonatal Ed. (2020) 105(1):87–93. doi: 10.1136/archdischild-2018-316773
69. Frizzola M, Miller TL, Rodriguez ME, Zhu Y, Rojas J, Hesek A, et al. High-flow nasal cannula: impact on oxygenation and ventilation in an acute lung injury model. Pediatr Pulmonol. (2011) 46(1):67–74. doi: 10.1002/ppul.21326
70. Sivieri EM, Wolfson MR, Abbasi S. Pulmonary mechanics measurements by respiratory inductive plethysmography and esophageal manometry: methodology for infants on non-invasive respiratory support. J Neonatal-Perinat Med. (2019) 12(2):149–59. doi: 10.3233/NPM-1869
71. Wanner A, Salathé M, O’Riordan TG. Mucociliary clearance in the airways. Am J Respir Crit Care Med. (1996) 154(6 Pt 1):1868–902. doi: 10.1164/ajrccm.154.6.8970383
72. Woodhead DD, Lambert DK, Clark JM, Christensen RD. Comparing two methods of delivering high-flow gas therapy by nasal cannula following endotracheal extubation: a prospective, randomized, masked, crossover trial. J Perinatol Off J Calif Perinat Assoc. (2006) 26(8):481–5. doi: 10.1038/sj.jp.7211543
73. Kwon JW. High-flow nasal cannula oxygen therapy in children: a clinical review. Clin Exp Pediatr. (2019) 63(1):3–7. doi: 10.3345/kjp.2019.00626
74. Aziz K, Lee CHC, Escobedo MB, Hoover AV, Kamath-Rayne BD, Kapadia VS, et al. Part 5: neonatal resuscitation 2020 American heart association guidelines for cardiopulmonary resuscitation and emergency cardiovascular care. Pediatrics. (2021) 147(Suppl 1):e2020038505E. doi: 10.1542/peds.2020-038505E
75. Wyllie J, Bruinenberg J, Roehr CC, Rüdiger M, Trevisanuto D, Urlesberger B. European resuscitation council guidelines for resuscitation 2015: section 7. Resuscitation and support of transition of babies at birth. Resuscitation. (2015) 95:249–63. doi: 10.1016/j.resuscitation.2015.07.029
76. Grover R, Singh P, Shubham S, Priyadarshi M, Chaurasia S, Basu S. Delivery room respiratory stabilization of preterm neonates: a randomized, controlled trial. Indian J Pediatr. (2022) 89(8):793–800. doi: 10.1007/s12098-022-04124-0
77. Siva NV, Reynolds PR. Stabilisation of the preterm infant in the delivery room using nasal high flow: a 5—year retrospective analysis. Acta Paediatr. (2021) 110(7):2065–71. doi: 10.1111/apa.15824
78. Reynolds P, Leontiadi S, Lawson T, Otunla T, Ejiwumi O, Holland N. Stabilisation of premature infants in the delivery room with nasal high flow. Arch Dis Child Fetal Neonatal Ed. (2016) 101(4):F284–287. doi: 10.1136/archdischild-2015-309442
79. Luo K, Huang Y, Xiong T, Tang J. High-flow nasal cannula versus continuous positive airway pressure in primary respiratory support for preterm infants: a systematic review and meta-analysis. Front Pediatr. (2022):10:980024. doi: 10.3389/fped.2022.980024
80. Hodgson KA, Wilkinson D, De Paoli AG, Manley BJ. Nasal high flow therapy for primary respiratory support in preterm infants. Cochrane Database Syst Rev. (2023) 5(5):CD006405. doi: 10.1002/14651858.CD006405.pub4
81. Hong H, Li XX, Li J, Zhang ZQ. High-flow nasal cannula versus nasal continuous positive airway pressure for respiratory support in preterm infants: a meta-analysis of randomized controlled trials. J Matern-Fetal Neonatal Med Off J Eur Assoc Perinat Med Fed Asia Ocean Perinat Soc Int Soc Perinat Obstet. (2021) 34(2):259–66. doi: 10.1080/14767058.2019.1606193
82. Bruet S, Butin M, Dutheil F. Systematic review of high-flow nasal cannula versus continuous positive airway pressure for primary support in preterm infants. Arch Dis Child Fetal Neonatal Ed. (2022) 107(1):56–9. doi: 10.1136/archdischild-2020-321094
83. de Jesus Brito S, Tsopanoglou SP, Galvão EL, de Deus FA, de Lima VP. Can high-flow nasal cannula reduce the risk of bronchopulmonary dysplasia compared with CPAP in preterm infants? A systematic review and meta-analysis. BMC Pediatr. (2021) 21(1):407. doi: 10.1186/s12887-021-02881-z
84. Fleeman N, Dundar Y, Shah PS, Shaw BN. Heated humidified high-flow nasal cannula for preterm infants: an updated systematic review and meta-analysis. Int J Technol Assess Health Care. (2019) 35(4):298–306. doi: 10.1017/S0266462319000424
85. Martins C, Pissarra R, Costa S, Soares H, Guimarães H. Comparison between continuous positive airway pressure and high-flow nasal cannula as postextubation respiratory support in neonates: a systematic review and meta-analysis. Turk Arch Pediatr. (2022) 57(6):581–90. doi: 10.5152/TurkArchPediatr.2022.22161
86. Colleti JJ, Azevedo Rd, Araujo O, Carvalho Wd. High-flow nasal cannula as a post-extubation respiratory support strategy in preterm infants: a systematic review and meta-analysis. J Pediatr (Rio J). (2020) 96(4):422–31. doi: 10.1016/j.jped.2019.11.004
87. Wilkinson D, Andersen C, O’Donnell CPF, De Paoli AG, Manley BJ. High flow nasal cannula for respiratory support in preterm infants. Cochrane Database Syst Rev. (2016) 2(2):CD006405. doi: 10.1002/14651858.CD006405.pub3
88. Farley RC, Hough JL, Jardine LA. Strategies for the discontinuation of humidified high flow nasal cannula (HHFNC) in preterm infants. Cochrane Database Syst Rev. (2015) 6(6):CD011079. doi: 10.1002/14651858.CD011079.pub2
89. Abdel-Hady H, Shouman B, Nasef N. Weaning preterm infants from continuous positive airway pressure: evidence for best practice. World J Pediatr WJP. (2015) 11(3):212–8. doi: 10.1007/s12519-015-0022-6
90. Amatya S, Rastogi D, Bhutada A, Rastogi S. Weaning of nasal CPAP in preterm infants: who, when and how? A systematic review of the literature. World J Pediatr WJP. (2015) 11(1):7–13. doi: 10.1007/s12519-014-0535-4
91. Delft Bv, Ginderdeuren FV, Lefevere J, Delft Cv, Cools F. Weaning strategies for the withdrawal of non-invasive respiratory support applying continuous positive airway pressure in preterm infants: a systematic review and meta-analysis. BMJ Paediatr Open. (2020) 4(1):e000858. doi: 10.1136/bmjpo-2020-000858
92. Hodgson KA, Owen LS, Kamlin COF, Roberts CT, Newman SE, Francis KL, et al. Nasal high-flow therapy during neonatal endotracheal intubation. N Engl J Med. (2022) 386(17):1627–37. doi: 10.1056/NEJMoa2116735
93. Permall DL, Pasha AB, Chen XQ. Current insights in non-invasive ventilation for the treatment of neonatal respiratory disease. Ital J Pediatr. (2019) 45(1):105. doi: 10.1186/s13052-019-0707-x
94. Solevåg AL, Cheung PY, Schmölzer GM. Bi-level noninvasive ventilation in neonatal respiratory distress syndrome. A systematic review and meta-analysis. Neonatology. (2021) 118(3):264–73. doi: 10.1159/000514637
95. Zhou B, Zhai JF, Jiang HX, Liu Y, Jin B, Zhang YY, et al. Usefulness of DuoPAP in the treatment of very low birth weight preterm infants with neonatal respiratory distress syndrome. Eur Rev Med Pharmacol Sci. (2015) 19(4):573–7. PMID: 25753873
96. Victor S, Roberts SA, Mitchell S, Aziz H, Lavender T, Extubate Trial Group. Biphasic positive airway pressure or continuous positive airway pressure: a randomized trial. Pediatrics. (2016) 138(2):e20154095. doi: 10.1542/peds.2015-4095
97. Alexiou S, Panitch HB. Physiology of non-invasive respiratory support. Semin Fetal Neonatal Med. (2016) 21(3):174–80. doi: 10.1016/j.siny.2016.02.007
98. Moretti C, Gizzi C, Papoff P, Lampariello S, Capoferri M, Calcagnini G, et al. Comparing the effects of nasal synchronized intermittent positive pressure ventilation (nSIPPV) and nasal continuous positive airway pressure (nCPAP) after extubation in very low birth weight infants. Early Hum Dev. (1999) 56(2–3):167–77. doi: 10.1016/S0378-3782(99)00046-8
99. Owen LS, Manley BJ. Nasal intermittent positive pressure ventilation in preterm infants: equipment, evidence, and synchronization. Semin Fetal Neonatal Med. (2016) 21(3):146–53. doi: 10.1016/j.siny.2016.01.003
100. Cummings JJ, Polin RA, Committee on Fetus and Newborn, American Academy of Pediatrics. Noninvasive respiratory support. Pediatrics. (2016) 137(1):e20153758. doi: 10.1542/peds.2015-3758
101. Moretti C, Gizzi C. Synchronized nasal intermittent positive pressure ventilation. Clin Perinatol. (2021) 48(4):745–59. doi: 10.1016/j.clp.2021.07.005
102. Huang L, Mendler MR, Waitz M, Schmid M, Hassan MA, Hummler HD. Effects of synchronization during noninvasive intermittent mandatory ventilation in preterm infants with respiratory distress syndrome immediately after extubation. Neonatology. (2015) 108(2):108–14. doi: 10.1159/000431074
103. de Waal CG, Kraaijenga JV, Hutten GJ, de Jongh FH, van Kaam AH. Breath detection by transcutaneous electromyography of the diaphragm and the Graseby capsule in preterm infants. Pediatr Pulmonol. (2017) 52(12):1578–82. doi: 10.1002/ppul.23895
104. Owen LS, Morley CJ, Davis PG. Effects of synchronisation during SiPAP-generated nasal intermittent positive pressure ventilation (NIPPV) in preterm infants. Arch Dis Child Fetal Neonatal Ed. (2015) 100(1):F24–30. doi: 10.1136/archdischild-2013-305830
105. Moretti C, Gizzi C, Montecchia F, Barbàra CS, Midulla F, Sanchez-Luna M, et al. Synchronized nasal intermittent positive pressure ventilation of the newborn: technical issues and clinical results. Neonatology. (2016) 109(4):359–65. doi: 10.1159/000444898
106. Ramos-Navarro C, Sanchez-Luna M, Sanz-López E, Maderuelo-Rodriguez E, Zamora-Flores E. Effectiveness of synchronized noninvasive ventilation to prevent intubation in preterm infants. AJP Rep. (2016) 6(3):e264–71. doi: 10.1055/s-0036-1586205
107. Gizzi C, Montecchia F, Panetta V, Castellano C, Mariani C, Campelli M, et al. Is synchronised NIPPV more effective than NIPPV and NCPAP in treating apnoea of prematurity (AOP)? A randomised cross-over trial. Arch Dis Child Fetal Neonatal Ed. (2015) 100(1):F17–23. doi: 10.1136/archdischild-2013-305892
108. Beck J, Reilly M, Grasselli G, Mirabella L, Slutsky AS, Dunn MS, et al. Patient-ventilator interaction during neurally adjusted ventilatory assist in low birth weight infants. Pediatr Res. (2009) 65(6):663–8. doi: 10.1203/PDR.0b013e31819e72ab
109. Firestone KS, Beck J, Stein H. Neurally adjusted ventilatory assist for noninvasive support in neonates. Clin Perinatol. (2016) 43(4):707–24. doi: 10.1016/j.clp.2016.07.007
110. Hochwald O, Riskin A, Borenstein-Levin L, Shoris I, Dinur GP, Said W, et al. Cannula with long and narrow tubing vs short binasal prongs for noninvasive ventilation in preterm infants: noninferiority randomized clinical trial. JAMA Pediatr. (2021) 175(1):36–43. doi: 10.1001/jamapediatrics.2020.3579
111. Gokce IK, Kaya H, Ozdemir R. A randomized trial comparing the short binasal prong to the RAM cannula for noninvasive ventilation support of preterm infants with respiratory distress syndrome. J Matern-Fetal Neonatal Med Off J Eur Assoc Perinat Med Fed Asia Ocean Perinat Soc Int Soc Perinat Obstet. (2021) 34(12):1868–74. doi: 10.1080/14767058.2019.1651268
112. Anand P, Kaushal M, Ramaswamy VV, Pullattayil S AK, Razak A, Trevisanuto D. Nasal cannula with long and narrow tubing for non-invasive respiratory support in preterm neonates: a systematic review and meta-analysis. Children. (2022) 9(10):1461. doi: 10.3390/children9101461
113. Ramaswamy VV, More K, Roehr CC, Bandiya P, Nangia S. Efficacy of noninvasive respiratory support modes for primary respiratory support in preterm neonates with respiratory distress syndrome: systematic review and network meta-analysis. Pediatr Pulmonol. (2020) 55(11):2940–63. doi: 10.1002/ppul.25011
114. Lemyre B, Laughon M, Bose C, Davis PG. Early nasal intermittent positive pressure ventilation (NIPPV) versus early nasal continuous positive airway pressure (NCPAP) for preterm infants. Cochrane Database Syst Rev. (2016) 12(12):CD005384. doi: 10.1002/14651858.CD005384.pub2
115. Ramaswamy VV, Bandyopadhyay T, Nanda D, Bandiya P, More K, Oommen VI, et al. Efficacy of noninvasive respiratory support modes as postextubation respiratory support in preterm neonates: a systematic review and network meta-analysis. Pediatr Pulmonol. (2020) 55(11):2924–39. doi: 10.1002/ppul.25007
116. Lemyre B, Davis PG, De Paoli AG, Kirpalani H. Nasal intermittent positive pressure ventilation (NIPPV) versus nasal continuous positive airway pressure (NCPAP) for preterm neonates after extubation. Cochrane Database Syst Rev. (2017) 2(2):CD003212. doi: 10.1002/14651858.CD003212.pub3
117. Mally PV, Beck J, Sinderby C, Caprio M, Bailey SM. Neural breathing pattern and patient-ventilator interaction during neurally adjusted ventilatory assist and conventional ventilation in newborns. Pediatr Crit Care Med J Soc Crit Care Med World Fed Pediatr Intensive Crit Care Soc. (2018) 19(1):48–55. doi: 10.1097/PCC.0000000000001385
118. Treussart C, Decobert F, Tauzin M, Bourgoin L, Danan C, Dassieu G, et al. Patient-ventilator synchrony in extremely premature neonates during non-invasive neurally adjusted ventilatory assist or synchronized intermittent positive airway pressure: a randomized crossover pilot trial. Neonatology. (2022) 119(3):386–93. doi: 10.1159/000524327
119. Lee J, Parikka V, Oda A, Wallström L, Lehtonen L, Soukka H. NIV-NAVA versus NCPAP immediately after birth in premature infants: a randomized controlled trial. Respir Physiol Neurobiol. (2022) 302:103916. doi: 10.1016/j.resp.2022.103916
120. Lee BK, Shin SH, Jung YH, Kim EK, Kim HS. Comparison of NIV-NAVA and NCPAP in facilitating extubation for very preterm infants. BMC Pediatr. (2019) 19(1):298. doi: 10.1186/s12887-019-1683-4
121. Colaizy TT, Kummet GJ, Kummet CM, Klein JM. Noninvasive neurally adjusted ventilatory assist in premature infants postextubation. Am J Perinatol. (2017) 34(6):593–8. doi: 10.1055/s-0036-1596053
122. Stein H, Beck J, Dunn M. Non-invasive ventilation with neurally adjusted ventilatory assist in newborns. Semin Fetal Neonatal Med. (2016) 21(3):154–61. doi: 10.1016/j.siny.2016.01.006
123. Goel D, Oei JL, Smyth J, Schindler T. Diaphragm-triggered non-invasive respiratory support in preterm infants. Cochrane Database Syst Rev. (2020) 3(3):CD012935. doi: 10.1002/14651858.CD012935.pub2
124. Shah PS, Sankaran K, Aziz K, Allen AC, Seshia M, Ohlsson A, et al. Outcomes of the preterm infants <29 weeks gestation over 10-year period in Canada: a cause for concern? J Perinatol Off J Calif Perinat Assoc. (2012) 32(2):132–8. doi: 10.1038/jp.2011.68
125. Abiramalatha T, Ramaswamy VV, Bandyopadhyay T, Somanath SH, Shaik NB, Pullattayil AK, et al. Interventions to prevent bronchopulmonary dysplasia in preterm neonates: an Umbrella review of systematic reviews and meta-analyses. JAMA Pediatr. (2022) 176(5):502–16. doi: 10.1001/jamapediatrics.2021.6619
126. Pillow JJ. High-frequency oscillatory ventilation: mechanisms of gas exchange and lung mechanics. Crit Care Med. (2005) 33(3 Suppl):S135–141. doi: 10.1097/01.CCM.0000155789.52984.B7
127. Hadj-Ahmed MA, Samson N, Nadeau C, Boudaa N, Praud JP. Laryngeal muscle activity during nasal high-frequency oscillatory ventilation in nonsedated newborn lambs. Neonatology. (2015) 107(3):199–205. doi: 10.1159/000369120
128. Mukerji A, Finelli M, Belik J. Nasal high-frequency oscillation for lung carbon dioxide clearance in the newborn. Neonatology. (2013) 103(3):161–5. doi: 10.1159/000345613
129. De Jaegere A, van Veenendaal MB, Michiels A, van Kaam AH. Lung recruitment using oxygenation during open lung high-frequency ventilation in preterm infants. Am J Respir Crit Care Med. (2006) 174(6):639–45. doi: 10.1164/rccm.200603-351OC
130. De Luca D, Piastra M, Pietrini D, Conti G. Effect of amplitude and inspiratory time in a bench model of non-invasive HFOV through nasal prongs. Pediatr Pulmonol. (2012) 47(10):1012–8. doi: 10.1002/ppul.22511
131. De Luca D, Carnielli VP, Conti G, Piastra M. Noninvasive high frequency oscillatory ventilation through nasal prongs: bench evaluation of efficacy and mechanics. Intensive Care Med. (2010) 36(12):2094–100. doi: 10.1007/s00134-010-2054-7
132. De Luca D, Centorrino R. Nasal high-frequency ventilation. Clin Perinatol. (2021) 48(4):761–82. doi: 10.1016/j.clp.2021.07.006
133. Ali YAH, Seshia MM, Ali E, Alvaro R. Noninvasive high-frequency oscillatory ventilation: a retrospective chart review. Am J Perinatol. (2022) 39(6):666–70. doi: 10.1055/s-0040-1718738
134. Thatrimontrichai A, Sirianansopa K, Janjindamai W, Dissaneevate S, Maneenil G. Comparison of endotracheal reintubation between nasal high-frequency oscillation and continuous positive airway pressure in neonates. Am J Perinatol. (2020) 37(4):409–14. doi: 10.1055/s-0039-1679932
135. Łoniewska B, Tousty J, Michalczyk B, Kordek A, Jankowska A. The use of noninvasive ventilation with high frequency in newborns-A single-center experience. Am J Perinatol. (2019) 36(13):1362–7. doi: 10.1055/s-0038-1677471
136. American Association for Respiratory Care, Restrepo RD, Walsh BK. Humidification during invasive and noninvasive mechanical ventilation. Respir Care. (2012) 57(5):782–8. doi: 10.4187/respcare.01766
137. Laptook AR, Bell EF, Shankaran S, Boghossian NS, Wyckoff MH, Kandefer S, et al. Admission temperature and associated mortality and morbidity among moderately and extremely preterm infants. J Pediatr. (2018) 192:53–59.e2. doi: 10.1016/j.jpeds.2017.09.021
138. Ralphe JL, Dail RB. Temperature and humidity associated with artificial ventilation in the premature infant: an integrative review of the literature. Adv Neonatal Care Off J Natl Assoc Neonatal Nurses. (2018) 18(5):366–77. doi: 10.1097/ANC.0000000000000519
139. Imbulana DI, Manley BJ, Dawson JA, Davis PG, Owen LS. Nasal injury in preterm infants receiving non-invasive respiratory support: a systematic review. Arch Dis Child Fetal Neonatal Ed. (2018) 103(1):F29–35. doi: 10.1136/archdischild-2017-313418
140. Vinall J, Grunau RE. Impact of repeated procedural pain-related stress in infants born very preterm. Pediatr Res. (2014) 75(5):584–7. doi: 10.1038/pr.2014.16
141. Committee on Fetus and Newborn and Section on Anesthesiology and Pain Medicine. Prevention and management of procedural pain in the neonate: an update. Pediatrics. (2016) 137(2):e20154271. doi: 10.1542/peds.2015-4271
142. Carbajal R, Eriksson M, Courtois E, Boyle E, Avila-Alvarez A, Andersen RD, et al. Sedation and analgesia practices in neonatal intensive care units (EUROPAIN): results from a prospective cohort study. Lancet Respir Med. (2015) 3(10):796–812. doi: 10.1016/S2213-2600(15)00331-8
143. O’Mara K, Gal P, Wimmer J, Ransom JL, Carlos RQ, Dimaguila MAVT, et al. Dexmedetomidine versus standard therapy with fentanyl for sedation in mechanically ventilated premature neonates. J Pediatr Pharmacol Ther. (2012) 17(3):252–62. doi: 10.5863/1551-6776-17.3.252
Keywords: neonate, preterm, RDS, non-invasive ventilation, continuous positive airway pressure, high flow oxygen therapy, nasal intermittent positive airway pressure, nasal high frequency ventilation
Citation: Ramaswamy VV, Devi R and Kumar G (2023) Non-invasive ventilation in neonates: a review of current literature. Front. Pediatr. 11:1248836. doi: 10.3389/fped.2023.1248836
Received: 27 June 2023; Accepted: 30 October 2023;
Published: 28 November 2023.
Edited by:
Theodora Boutsikou, National and Kapodistrian University of Athens, GreeceReviewed by:
Angela Paladini, Agostino Gemelli University Polyclinic (IRCCS), ItalyMelinda Matyas, University of Medicine and Pharmacy Iuliu Hatieganu, Romania
Julia Charlton, B.C. Women's Hospital & Health Centre, Canada
© 2023 Ramaswamy, Devi and Kumar. This is an open-access article distributed under the terms of the Creative Commons Attribution License (CC BY). The use, distribution or reproduction in other forums is permitted, provided the original author(s) and the copyright owner(s) are credited and that the original publication in this journal is cited, in accordance with accepted academic practice. No use, distribution or reproduction is permitted which does not comply with these terms.
*Correspondence: Viraraghavan Vadakkencherry Ramaswamy MTkudmlyYUBnbWFpbC5jb20=