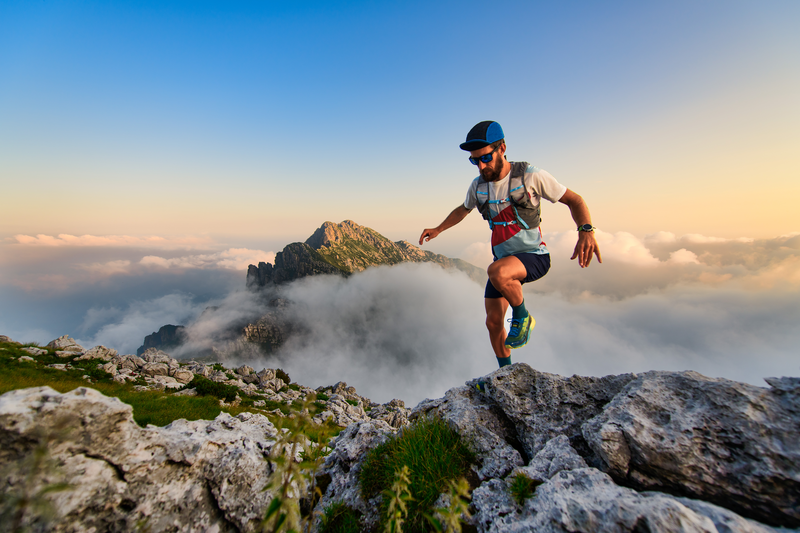
95% of researchers rate our articles as excellent or good
Learn more about the work of our research integrity team to safeguard the quality of each article we publish.
Find out more
MINI REVIEW article
Front. Parasitol. , 10 December 2024
Sec. Antiparasitic Drugs and Drug Resistance
Volume 3 - 2024 | https://doi.org/10.3389/fpara.2024.1508167
Nematode parasitic infections continue to be a major health problem for humans and animals. Drug resistance to currently available treatments only worsen the problem. Drug discovery is expensive and time-consuming, making drug repurposing an enticing option. Emodepside, a broad-spectrum anthelmintic, has shown efficacy in the treatment of nematode parasitic infections in cats and dogs. It is now being considered and trialed for the treatment of onchocerciasis, trichuriasis (whipworm), and hookworm infections in humans. Its unique mechanism of action distinguishes it from traditional anthelmintics, positioning it as a promising candidate for combating resistance to other current drugs. Here, we provide a brief review of the available information on emodepside’s pharmacokinetics, safety, and tolerability. We highlight the potential benefits and risks associated with its use, examining key toxicity effects. By exploring the literature, we aim to provide insights into the risks associated with emodepside that may impact its application in veterinary and human medicine. Although emodepside demonstrates a favorable safety profile, continued monitoring of its toxicity is crucial, particularly in vulnerable populations. This mini-review serves as a concise resource for researchers and clinicians interested in anthelmintic therapy.
Emodepside is a semisynthetic compound belonging to the cyclooctadepsipeptide group, known to show anthelmintic activity against larval and adult stages of filarial nematodes (Hübner et al., 2021). It is a member of the N-methylated cyclooctadepsipeptides, derived from a naturally occurring compound PF1022A, which was isolated in the early 90s from Mycelia sterilia, a fungus that inhabits leaves of Camellia japonica (Krücken et al., 2021; Sasaki et al., 1992). Emodepside is synthesized by adding two morpholine rings in para-position of each of the 2 (R)-phenyl lactic acids of the parent compound PF1022A (Figures 1A, B) (Krücken et al., 2021).
Figure 1. Diagram of structure and protein targets of PF1022A & emodepside. (A) Structure of PF1022A, cyclo[(αR)-α-hydroxybenzenepropanoyl-N-methyl-L-leucyl-(2R)-2-hydroxypropanoyl-N-methyl-L-leucyl-(αR)-α-hydroxybenzenepropanoyl-N-methyl-L-leucyl-(2R)-2-hydroxypropanoyl-N-methyl-L-leucyl]. (B) Structure of emodepside, cyclo[(αR)-α-hydroxy-4-(4-morpholinyl)benzenepropanoyl-N-methyl-L-leucyl-(2R)-2-hydroxypropanoyl-N-methyl-L-leucyl-(αR)-α-hydroxy-4-(4-morpholinyl)benzenepropanoyl-N-methyl-L-leucyl-(2R)-2-hydroxypropanoyl-N-methyl-L-leucyl]; the morpholino group is circled, adapted from Krücken et al., 2021. Emodepside was discovered by the Japanese pharmaceutical company Astellas and then developed and commercialized by Bayer Animal Health. It is prepared by attaching a morpholine ring on each of two D-phenyllactic acids to PF1022A, a fungus, Mycelia sterile of Camellia japonica. (C) Diagram of the 7-transmembrane structure of the latrophilin receptor. (D) Diagram of one of the subunits of the tetrameric SLO-1K receptor; pink open circle, putative emodepside binding site, Raisch et al., 2021; calcium binding sites (red circle) and magnesium binding site (purple circle) (E) The tetrameric structure of the α subunit of the SLO-1K channel; Images created in PowerPoint Microsoft.
Emodepside was developed as a veterinary anthelmintic and has been found to have broad-spectrum activity against nematode infections of humans (Hübner et al., 2021; Bah et al., 2021). Emodepside is being trialed for the treatment of whipworm, hookworm, and onchocerciasis in humans (Mrimi et al., 2023; Gillon et al., 2021). Adverse reactions though mild and reversible, have been reported and seen to increase with dose (Mrimi et al., 2023; Gillon et al., 2021). The veterinary experience of the use of emodepside in dogs and cats has also drawn attention to some of its toxic effects (Gaens et al., 2019; Walther et al., 2016). This mini-review comments on emodepside’s actions, uses as an anthelmintic and toxicity characteristics of emodepside.
Emodepside has broad-spectrum activity against a wide range of animal nematode soil-transmitted parasites, including those of dogs and cats (Figure 2A). In vitro studies have also revealed robust activity against larval and adult stages of several nematode species that serve as models for human soil-transmitted helminths and filarial nematodes (Karpstein et al., 2019; Zahner et al., 2001). Sensitivity to emodepside varies between the worm’s life stage and between the different species of parasite (Karpstein et al., 2019).
Figure 2. Use of emodepside as an anthelmintic in dogs, cats and applications in humans, pharmacokinetics BK channel target locations. (A) Emodepside is effective against a broad spectrum of nematode parasites of cats, dogs, and humans. (B) Proposed pharmacokinetics pathways for emodepside. (C) Emodepside as a substrate for P-glycoprotein (EMA, 2011). A blood-brain barrier with functional p-glycoprotein (left) prevents emodepside from accumulating in the brain. In contrast, a blood-brain barrier defective for the carrier protein (right) lets emodepside into the brain (purple spheres are emodepside, green rectangles are functional p-glycoprotein, and pattern-filled green rectangles are mutants for p-glycoprotein). (D) Wide distribution and functions of BK channels in the human host (Latorre et al., 2017; Peppin et al., 2021; Perry and Sandle, 2009; Delgado-Bermúdez et al., 2024; Henquin et al., 2017; Raisch, 2024; Kunz et al., 2002) NB: BK channels are found in more organs, these are examples; Images created by BioRender.com and in PowerPoint Microsoft.
Emodepside’s spectrum of action extends to nematode parasites of horses, sheep, cattle, chickens, dogs, and cats (Epe and Kaminsky, 2013; Harder et al., 2003). Importantly, emodepside has shown notable activity against nematode parasites resistant to other classes of anthelmintics, such as anthelmintic-resistant populations of Haemonchus contortus and Cooperia oncophora in sheep and cattle, respectively (Harder et al., 2003; Samson-Himmelstjerna et al., 2005).
Emodepside is marketed for the treatment of parasitic nematode infections in dogs and cats. Emodepside is registered for use in combination with praziquantel (as Profender®) for the treatment of hookworm infections, ascarids, and other nematode infections in cats but not dogs in the US, while in Europe, it is approved for use in both cats and dogs (EMA, 2008b; Krücken et al., 2021; Miltsch et al., 2012). This might be due to emodepside’s adulticide activity, which could severely harm heartworm infected dogs in the US, where this disease, in contrast to Europe, is a major threat to dogs. Furthermore, emodepside is recommended by parasitology researchers and the American Animal Hospital Association for off-label use as a last resort for treating multi-anthelmintic drug resistant (MADR) hookworms in dogs. However, its rapid killing for adult heartworms in positive dogs increases the risk of pulmonary embolism and anaphylaxis (Jimenez Castro and Kaplan, 2020; Singler, 2024). Profender is administered orally (as tablets) in dogs and topically (as spot-on) in cats. Emodepside is also part of the spot-on combination with praziquantel and tigolaner (Felpreva®) in cats (Blazejak et al., 2023) for treating: roundworms, lungworms, & hookworm (active emodepside); tapeworms (active praziquantel); and fleas, ticks & mites (active tigolaner). Additionally, emodepside is combined with toltrazuril (Procox®) for the treatment of nematode parasites (active emodepside) and coccidia (active toltrazuril) in dogs and puppies (EMA, 2011). Furthermore, emodepside is used off-label as a last resort for treating multi-anthelmintic drug-resistant (MADR) hookworms in dogs; however, its rapid killing of heartworms in positive dogs increases the risk of pulmonary embolism and anaphylaxis (Singler, 2024).
Emodepside’s broad spectrum of activity and lack of significant toxicity in animals have prompted the proposal to repurpose and trial it for the treatment of onchocerciasis trichuriasis (whipworm), and hookworm in humans.
Emodepside has shown potent activity against both microfilariae and adult Onchocerca worms in preclinical and early clinical studies (Bah et al., 2021; Assmus et al., 2022). In the bovine model of onchocerciasis (O. ochengi), treatment with emodepside yielded rapid and sustained suppression of microfilariae, with paralysis and eventual death of adult female worms (Bah et al., 2021). This finding is significant because current treatments (like ivermectin) primarily target microfilarial stages, allowing the adult worms to survive, albeit somewhat compromised. Phase I clinical trials in healthy volunteers have reported favorable safety and tolerability profiles with no significant adverse effects observed (Gillon et al., 2021). Building on these promising results, Phase II clinical trials have been planned (Assmus et al., 2022) to assess its efficacy in treating onchocerciasis in endemic regions, and results are awaited.
The efficacy of emodepside against Ancylostoma caninum (Cima, 2021), encouraged its trial for the treatment of hookworm infections in humans. The drug underwent Phase II trials and demonstrated dose-dependent efficacy (Mrimi et al., 2023). The most common adverse events reported were headache, blurred vision, and dizziness, which increased with higher doses, although they were mild and self-resolving (Mrimi et al., 2023).
The efficacy of emodepside for treatment against Trichuris vulpis infection in dogs (EMA, 2008b) encouraged its trialing in humans. Emodepside has now been evaluated in Phase II clinical trials for the treatment of Trichuris trichiura (whipworm) infections in humans (Mrimi et al., 2023). The study reported that 5mg of emodepside per participant produced an 85% cure rate compared to the 17% cure rate in the albendazole control. This observed efficacy suggests that emodepside could become a valuable addition to the limited treatment options for human whipworm infections.
Overall, the available evidence underscores emodepside’s potential as a promising treatment option for onchocerciasis, hookworm, and Trichuris infections in humans. However, additional Phase III trials are still required to assess its efficacy and safety under field clinical conditions for the treatment of these neglected tropical diseases.
Early attempts to decipher the mode of action of emodepside (Bay44-4400) using the natural compound (PF1022a) led to the reports that it serves as a ligand for the Haemonchus contortus HC110-R protein, which is structurally similar to the mammalian latrophilin G-coupled protein receptor (Figure 1C) (Saeger et al., 2001; Willson et al., 2004). Latrophilin receptors are expressed in mammals and other organisms, in various tissues, and abundantly in the nervous system, where they play an important role in neurosecretion for neuronal development and function (Moreno-Salinas et al., 2019; Guest, 2008; Harder et al., 2005; Silva and Ushkaryov, 2010). Emodepside was reported to inhibit pharyngeal pumping and locomotion in Caenorhabditis elegans (Amliwala, 2005; Willson et al., 2004). The effects of emodepside on pharyngeal pumping were inhibited by double mutants of lat-1 and lat-2, but the inhibitory effects of emodepside on locomotion were not abolished (Guest et al., 2007). This suggested that emodepside also acted on another receptor, subsequently identified as the SLO-1 K channel (Guest et al., 2007).
Calcium-activated potassium channels referred to as SLO-1K, also known as BK (“big potassium”), channels are large-conductance potassium ion channels composed of four α subunits that are each seven-transmembrane proteins (Figure 1D). These α subunits assemble around the pore to form a tetramer (Figure 1E) They are calcium-activated, voltage-sensitive channels that are selective for potassium ions and exist in various isoforms. SLO-1K channels are widely distributed across various tissues of nematodes, playing critical physiological roles in neurotransmitter release, muscle function, and cell excitability (Gessner et al., 2012; Salkoff et al., 2006; Nowicka-Bauer and Szymczak-Cendlak, 2021). Studies have shown that emodepside acts directly on SLO-1K channels, activating them and causing hyperpolarization resulting in paralysis of the worm (Bull, 2008; Buxton et al., 2011; Holden-Dye et al., 2012). Two binding sites have been suggested: one beneath the channel’s selectivity filter, as shown by Cryo-EM experiments (Raisch et al., 2021), and a putative site on the channel’s RCK1 domain based on molecular docking (Kashyap et al., 2019). The effect of emodepside on SLO-1 channels in Ascaris suum muscle is enhanced by activating the protein kinase C, and NO pathways (Buxton et al., 2011) and diethylcarbamazine-mediated action on TRP channel (Verma et al., 2020; Kashyap et al., 2022) showing that other signaling pathways can modulate the effect of emodepside on these channels.
We point out here that latrophilin receptors and SLO-1K (BK) channels are present in mammalian hosts. This implies that there is a possibility of observing undesirable effects of emodepside on these receptors in the human and animal hosts of the nematode parasites. Nevertheless, it is possible that its anthelmintic specificity is conferred by other mechanisms unique to the target parasite (Raisch and Raunser, 2023).
The pharmacokinetics of emodepside have been investigated in rats where rapid absorption and distribution throughout all organs were observed; the highest concentrations were found in the fat following oral administration (EMA, 2008b). A secondary peak has been seen after four days in cats following oral administration, which was suggested to be due to emodepside having a slow redistribution from the fat back into plasma (Page, 2008). Enterohepatic recycling may contribute to a secondary peak that is seen following administration. Compared to cats, dogs show a shorter elimination time due to both faster metabolism and differences in the route of administration (EMA, 2008b).
In the human Phase I clinical trials with oral administration for onchocerciasis, the reported pharmacokinetic profile of emodepside, was rapid absorption, fast distribution to tissues, and an extended half-life of more than 500 hours in the fasting state: in the fed state emodepside was absorbed more slowly (Gillon et al., 2021, 2020). This contrasts with dogs, where there is more rapid absorption in the fed state (EMA, 2008a). A rapid absorption in humans produces a high peak plasma concentration, Cmax, which is connected to emodepside’s activity against microfilariae, while the long terminal half-life is also important for the elimination of the tissue-dwelling macrofilariae (Gillon et al., 2021).
Emodepside is primarily excreted through bile and then eliminated in the feces, with small amounts in urine, indicating minimal metabolism (CTP, 2014; Vercruysse and Claerebout, 2022; Mencke et al., 2023) and safety for patients with renal impairment. The major excretion products of the drug include unchanged emodepside and hydroxylated derivatives (Mencke et al., 2023; EMA, 2008a), suggesting a level of hepatic metabolism. The liver’s role in metabolism and enterohepatic recycling remains to be characterized. The excretion of a significant proportion of unchanged drug suggests its stability and a reduced risk of forming harmful metabolites and toxicity.
The lipid-soluble nature of emodepside decreases its bioavailability following oral administration (Gillon et al., 2021) and leads to food-drug interactions. The bioavailability of emodepside is reduced following oral administration in the fed versus fasting state (Gillon et al., 2020). The prolonged elimination half-life, which appears connected to enterohepatic recycling, increases the systemic exposure of microfilariae and can increase the macrofilaricidal effect. Optimization of the emodepside dose is important to achieve therapeutic concentrations and minimize toxicity. Figure 2B summarizes the pharmacokinetic pathway for emodepside.
The efficacy and safety of emodepside has been demonstrated against nematode parasites of cats and dogs (Reinemeyer et al., 2005; Schimmel et al., 2011; Altreuther et al., 2011; Böhm et al., 2015; Lee et al., 2019). Emodepside’s potent activity against microfilaria and adult filarial nematodes makes it a potential treatment for neglected tropical infections, including human onchocerciasis (Hübner et al., 2021; Kashyap et al., 2019; Bah et al., 2021). Human Phase IIa trials using dose-ranging, randomized, controlled studies have described the efficacy of emodepside against Trichuris trichiura and hookworm infections (Mrimi et al., 2023).
Repeated dose studies in humans demonstrated emodepside’s safety up to 40 mg per participant with good tolerance up to 20 mg (Gillon et al., 2018). The main adverse effect, visual disturbances, observed in Phase I clinical trials in healthy male subjects were mild and transient (Gillon et al., 2021). Neurotoxicity has occasionally been reported in dogs with a homozygous mutation in the MDR1 gene that codes for a P-glycoprotein (Noack et al., 2021). Symptoms include vomiting, incoordination, seizures, muscle tremors, and dilated pupils, which are more likely to be seen in fed dogs because of the faster absorption from the GI tract (Gaens et al., 2019; EMA, 2008a). The MDR1 mutation is more common in herding breed dogs, including Shetland Sheepdog, Australian Shepherds, Collies, and White Swiss Shepherd (Gramer et al., 2011; Noack et al., 2021). Animals with the P-glycoprotein defect exhibit neurological symptoms when treated with emodepside (Dias, 2014; Elmshäuser et al., 2015; Gaens et al., 2019; Noack et al., 2021) Thus, the presence of MDR1 mutant effects highlights the importance of pharmacogenomic factors that affect emodepside’s safety. Nevertheless, preclinical studies across various animal models have yet to identify significant safety concerns (EMA, 2008b), and current findings support a favorable safety profile for emodepside.
This extensive distributions of SLO-1K channels in host organisms raise questions about potential toxicity of emodepside. Despite the importance of these channels, few studies detail emodepside’s toxicity. The research available generally points to low toxicity in different laboratory animals and pets, varying with the administration routes (EMA, 2008a; Vercruysse and Claerebout, 2014). The major toxicity observed has been in cases of overdose, noncompliance with dosing, and mutations in the multidrug resistance efflux carrier (EMA, 2008a; Gaens et al., 2019). Supplementary Table S1 summarizes the observed toxic effects of emodepside.
Symptoms of depressed neurological and respiratory function have been observed in overdosed cats. Repeated dose toxicity studies revealed various adverse effects, including ataxia and increased motility, in rats (EMA, 2008a). The depressed neurological effects may be associated with an increased opening of ‘BK’ potassium channels (Crisford et al., 2015). The liver, adrenal glands, pancreas, and reproductive system are also target organs of repeated doses of emodepside (EMA, 2008a). The No-Observed Effect Levels (NOEL) vary with the mode of application (EMA, 2008a) and fasting status of the patient. Administration to a fasting human patient increases the possibility of observing toxic effects because of the increased absorption rate from the intestine.
Emodepside is a substrate for a P-glycoprotein, the multidrug resistance protein 1 (MDR1) which plays a crucial role in the uptake, body distribution, and elimination of numerous drugs and is expressed in different organs, including the brain (Figure 2C) (Elmshäuser et al., 2015; EMA, 2008b). Animals with the P-glycoprotein defect exhibit neurological symptoms when treated with emodepside (Gaens et al., 2019; Elmshäuser et al., 2015; Dias, 2014). Ataxia also arises in cats when they lick the spot-on application site immediately after treatment (EMA, 2008a). These observations highlight the importance of considering pharmacogenomic and pharmacokinetic variations in assessing safety.
There are indications that emodepside might have the potential to interfere with embryo-fetal development (Wright and Elsheikha, 2018). Studies in rats and rabbits showed an impact on reproductive performance, only at doses causing parental toxicity, with no primary effect on fertility (CTP, 2014). Embryotoxicity/teratogenicity revealed some adverse effects, encompassing maternal toxicity, fetotoxicity, fetal malformations, and various skeletal/visceral anomalies or deviations; however, no issues were reported with emodepside in pregnant cats (CTP, 2014; Olliaro et al., 2011). Continued surveillance is needed to address any potential reproductive toxicity.
There is limited data on carcinogenicity, mutagenicity, and endocrine toxicity for emodepside. In vitro and in vivo studies show no evidence of genotoxicity, skin or eye irritations (CTP, 2014). However, rare local toxicities like alopecia, pruritis, and inflammation have been reported in cats (EMA, 2008a). Rat studies have revealed hormone deregulation as a cause of observed developmental toxicity (CTP, 2014). More studies are required to confirm these aspects of toxicity.
Studies in humans with emodepside have revealed mild and self-resolving side effects such as headache, visual disorders and dizziness (Gillon et al., 2021; Mrimi et al., 2023). Despite the ubiquitous distribution of BK channels in the body (exemplified in Figure 2D), the recognized target site of emodepside, the adverse effects are predominantly seen in ocular tissues possibly due to the specific need for the eye’s high spatiotemporal precision. The rapid absorption of emodepside revealed by its pharmacokinetic profile suggests that emodepside activates BK channels in retinal cells and the trabecular meshwork (Tanimoto et al., 2012; Cuppoletti et al., 2012), disrupting sharp vision and causing blurring. The response to BK channel activation by emodepside in other tissues may show less sensitivity and hence little side effects due to their unique physiological requirements and activation threshold or even differences in isoforms. While major toxicity reports have not been observed, a 40mg dose of emodepside per participant led to increased reports of eye and central nervous system disorders in some studies (Assmus et al., 2022; Gillon et al., 2020). Also, mutations in the MDR1 gene, which codes for P-glycoprotein, could affect its function and may be linked to symptoms like headaches. Care is required when administering emodepside to individuals with P-glycoprotein impairment or deficiency.
The main risk factors associated with adverse effects of emodepside are noncompliance and MDR1 mutation predisposition. Caution is advised during administration. Again, emodepside’s filaricidal effect on adult parasites could pose a notable adverse reaction in dogs with Dirofilaria immitis because of its location in the pulmonary arteries: rapid paralysis of these parasites in the dog host results in pulmonary embolism and release of Wolbachia antigens. Additionally, there is limited data on emodepside’s use in severely debilitated animals (EMA, 2011; 2008a); thus, its use should be considered carefully. Potential risk factors with emodepside akin to those of diethylcarbamazine warrant further study.
This review examines the safety profile of emodepside, focusing on its toxicological aspects within anthelmintic therapy. Emodepside’s distinct mechanism of action differentiates it from other anthelmintics, making it a promising option for tackling multidrug-resistant infections. The drug’s demonstrated safety, pharmacokinetics, and tolerability enhance its credibility. Additionally, the review provides insights into potential risks associated with emodepside and toxicity concerns.
CN: Writing – original draft, Writing – review & editing. AR: Writing – review & editing. RM: Conceptualization, Funding acquisition, Supervision, Writing – original draft, Writing – review & editing.
The author(s) declare financial support was received for the research, authorship, and/or publication of this article. We acknowledge the NIH National Institute of Allergy and Infectious Diseases grants R01AI047194 and R01AI155413 and the E. A. Benbrook Foundation to RM.
We also acknowledge Brad Keunnen, the Veterinary Medicine and Animal Science Librarian, at Iowa State University for assistance.
The authors declare that the research was conducted in the absence of any commercial or financial relationships that could be construed as a potential conflict of interest.
The author(s) declare that Generative AI was used in the creation of this manuscript. The literature research was supported by some AI-generated insights using: ChatGPT (based on GPT-4); OpenAI, 2024, chat.openai.com; and Perplexity.ai.
All claims expressed in this article are solely those of the authors and do not necessarily represent those of their affiliated organizations, or those of the publisher, the editors and the reviewers. Any product that may be evaluated in this article, or claim that may be made by its manufacturer, is not guaranteed or endorsed by the publisher.
The text, comments, and opinions expressed in this review were composed solely by the authors.
The Supplementary Material for this article can be found online at: https://www.frontiersin.org/articles/10.3389/fpara.2024.1508167/full#supplementary-material
Altreuther G., Gasda N., Schroeder I., Joachim A., Settje T., Schimmel A., et al. (2011). Efficacy of Emodepside plus Toltrazuril Suspension (Procox® Oral Suspension for Dogs) against Prepatent and Patent Infection with Isospora Canis and Isospora Ohioensis-Complex in Dogs. Parasitol. Res. 109, 9–205. doi: 10.1007/s00436-011-2398-0
Amliwala K. (2005). MOLECULAR AND GENETIC DETERMINANTS OF THE INHIBITORY ACTION OF EMODEPSIDE ON C. ELEGANS MUSCLE. Available online at: https://eprints.soton.ac.uk/465608/1/979364.pdf (Accessed November 26, 2024).
Assmus F., Hoglund R. M., Monnot Frédéric, Specht S., Scandale I., Tarning J. (2022). Drug development for the treatment of onchocerciasis: population pharmacokinetic and adverse events modeling of emodepside. PloS Negl. Trop. Dis. 16, e00102195. doi: 10.1371/journal.pntd.0010219
Bah G. S., Schneckener S., Hahnel S. R., Bayang N. H., Fieseler H., Schmuck G. M., et al. (2021). Emodepside targets SLO-1 channels of onchocerca ochengi and induces broad anthelmintic effects in a bovine model of onchocerciasis. PloS Pathog. 17, e1009601. doi: 10.1371/journal.ppat.1009601
Blazejak K., Cvejić D., Hellmann K., Ringeisen H., Hamburg H., Petry G., et al. (2023). Field efficacy and safety of felpreva® (Tigolaner, emodepside and praziquantel) spot-on for the treatment of natural ear mite infestations (Otodectes cynotis) and notoedric mange (Notoedres cati) in cats. Curr. Res. Parasitol. Vector-Borne Dis. 4, 100146. doi: 10.1016/j.crpvbd.2023.100146
Böhm C., Wolken S., Schnyder M., Basso W., Deplazes P., Di Cesare A., et al. (2015). Efficacy of emodepside/praziquantel spot-on (Profender®) against adult aelurostrongylus abstrusus nematodes in experimentally infected cats. Parasitol. Res. 114, 155–645. doi: 10.1007/s00436-015-4521-0
Bull K. (2008). An Investigation into the Mechanism of Action of Emodepside and Other Novel Anthelmintic Compounds (Southampton: Phd, University of Southampton). Available at: https://eprints.soton.ac.uk/466425/.
Buxton S. K., Neveu C., Charvet C., Robertson A. P., Martin R. J. (2011). On the mode of action of emodepside: slow effects on membrane potential and voltage-activated currents in ascaris suum. Br. J. Pharmacol. 164, 453–470. doi: 10.1111/j.1476-5381.2011.01428.x
Cima G. (2021). Drug-resistant hookworms spreading in dogs, parasitologists warn (American Veterinary Medical Association). Available at: https://www.avma.org/javma-news/2021-09-15/drug-resistant-hookworms-spreading-dogs-parasitologists-warn (Accessed Nov 26, 2024).
Crisford A., Ebbinghaus-Kintscher U., Schoenhense E., Harder A., Raming K., O’Kelly I., et al. (2015). The cyclooctadepsipeptide anthelmintic emodepside differentially modulates nematode, insect and human calcium-activated potassium (SLO) channel alpha subunits.” Edited by timothy G. Geary. PloS Negl. Trop. Dis. 9, e00040625. doi: 10.1371/journal.pntd.0004062
CTP (2014). A phase 1, single-blind, randomized, placebo controlled, parallel-group, multiple-dose-escalation study to investigate safety, tolerability, and pharmacokinetics of emodepside (BAY 44-4400) after oral dosing in healthy male subjects. Available online at: https://classic.clinicaltrials.gov/ProvidedDocs/14/NCT03383614/Prot_000.pdf (Accessed November 26, 2024).
Cuppoletti J., Malinowska D. H., Tewari K. P., Chakrabarti J., Ueno R. (2012). Unoprostone isopropyl and metabolite M1 activate BK channels and prevent ET-1–induced [Ca2+]i increases in human trabecular meshwork and smooth muscle. Invest. Ophthalmol. Visual Sci. 53, 5178–5895. doi: 10.1167/iovs.11-9046
Delgado-Bermúdez A., Yeste M., Bonet S., Pinart E. (2024). Physiological role of potassium channels in mammalian germ cell differentiation, maturation, and capacitation. Andrology. doi: 10.1111/andr.13606
Dias C. AndréR. (2014). Possible emodepside toxicosis in a collie with MDR1 gene mutation. (Vasco da Gama, Portugal: Vasco da Gama Higher Education School). Available online at: https://comum.rcaap.pt/bitstream/10400.26/16628/1/Disserta%C3%A7%C3%A3o_Carlos%20Dias.pdf.
Elmshäuser S., Straehle L. C., Kranz J., Krebber R., Geyer J. (2015). Brain penetration of emodepside is increased in P-glycoprotein-deficient mice and leads to neurotoxicosis. J. Veterinary Pharmacol. Ther. 38, 74–79. doi: 10.1111/jvp.12149
EMA (2008a). Profender:EPAR-scientific-discussion. Available online at: https://www.ema.europa.eu/en/documents/scientific-discussion/profender-epar-scientific-discussion_en.pdf (Accessed November 26, 2024).
EMA (2008b). Profender: EPAR-product information. Available online at: https://www.ema.europa.eu/en/documents/product-information/profender-epar-product-information_en.pdfepar-product-information_en.pdf (Accessed November 26, 2024).
EMA (2011). Procox-epar-product-information_en.Pdf. Available online at: https://www.ema.europa.eu/en/documents/product-information/procox-epar-product-information_en.pdf (Accessed November 26, 2024).
Epe C., Kaminsky R. (2013). New advancement in anthelmintic drugs in veterinary medicine. Trends Parasitol. 29, 129–345. doi: 10.1016/j.pt.2013.01.001
Gaens D., Leithäuser C., Hamann M., Geyer J. (2019). Adverse drug reactions after administration of emodepside/praziquantel (Profender®) in an MDR1-mutant Australian shepherd dog: case report. Front. Veterinary Sci. 6. doi: 10.3389/fvets.2019.00296
Gessner G., Cui Y.-M., Otani Y., Ohwada T., Soom M., Hoshi T., et al. (2012). Molecular mechanism of pharmacological activation of BK channels. Proc. Natl. Acad. Sci. United States America 109, 3552–3575. doi: 10.1073/pnas.1114321109
Gillon J. Y., Dennison J., Van Den Berg F., Delhomme S., Cheeseman K. D., Pe A Rossi C., et al. (2020). Safety, pharmacokinetics, relative bioavailability and dose linearity of four formulations of emodepside in healthy male subjects. Preprint. doi: 10.22541/au.159863472.25422049
Gillon J.-Y., Dennison J., van den Berg F., Delhomme S., Cheeseman K. D., Peña Rossi C., et al. (2021). Safety, tolerability and pharmacokinetics of emodepside, a potential novel treatment for onchocerciasis (River blindness), in healthy male subjects. Br. J. Clin. Pharmacol. 87, 3949–3960. doi: 10.1111/bcp.14816
Gillon J.-Y., Rossi C. Peña, Monnot F., Strub-Wourgaft N., Rodriguez M.-L., Don R. (2018). A single-center, first-in-human, randomized, double-blind, placebo-controlled, parallel-group POSTER 534 study to investigate the safety, tolerability and pharmacokinetics of escalating single doses of emodepside (BAY 44-4400) in healthy male subjects.
Gramer I., Leidolf R., Döring B., Klintzsch S., Krämer E.-M., Yalcin E., et al. (2011). Breed distribution of the nt230(Del4) MDR1 mutation in dogs. Veterinary J. 189, 67–715. doi: 10.1016/j.tvjl.2010.06.012
Guest M. (2008). An Investigation into the Mode of Action of the Anthelmintic Emodepside: A Molecular, Pharmacological and Electrophysiological Characterisation (Southampton: Phd, University of Southampton). Available at: https://eprints.soton.ac.uk/66714/.
Guest M., Bull K., Walker R. J., Amliwala K., O’Connor V., Harder A., et al. (2007). The calcium-activated potassium channel, SLO-1, is required for the action of the novel cyclo-octadepsipeptide anthelmintic, emodepside, in caenorhabditis elegans. Int. J. Parasitol. 37, 1577–1588. doi: 10.1016/j.ijpara.2007.05.006
Harder A., Holden-Dye L., Walker R., Wunderlich F. (2005). Mechanisms of action of emodepside. Parasitol. Res. 97 Suppl 1, S1–10. doi: 10.1007/s00436-005-1438-z
Harder A., Schmitt-Wrede H.-P., Krücken Jürgen, Marinovski P., Wunderlich F., Willson J., et al. (2003). Cyclooctadepsipeptides—an anthelmintically active class of compounds exhibiting a novel mode of action. Int. J. Antimicrobial Agents 22, 318–331. doi: 10.1016/S0924-8579(03)00219-X
Henquin J.-C., Dufrane D., Gmyr V., Kerr-Conte J., Nenquin M. (2017). Pharmacological approach to understanding the control of insulin secretion in human islets. Diabetes Obes. Metab. 19, 1061–1705. doi: 10.1111/dom.12887
Holden-Dye L., Crisford A., Welz C., von Samson-Himmelstjerna G., Walker R. J., O’Connor V. (2012). Worms take to the slo lane: A perspective on the mode of action of emodepside. Invertebrate Neurosci. 12, 29–365. doi: 10.1007/s10158-012-0133-x
Hübner M. P., Townson S., Gokool S., Tagboto S., Maclean M. J., Verocai G. G., et al. (2021). Evaluation of the in vitro susceptibility of various filarial nematodes to emodepside. Int. J. Parasitology: Drugs Drug Resistance 17, 27–35. doi: 10.1016/j.ijpddr.2021.07.005
Jimenez Castro P. D., Kaplan R. (2020). Suspected-resistant hookworm infections in dogs and cats. Available online at: https://parasitology.cvm.ncsu.edu/vmp930/supplement/2020_UGA_Resistant_Hookworm_Guidelines.pdf (Accessed November 26, 2024).
Karpstein T., Pasche Valérian, Häberli Cécile, Scandale I., Neodo A., Keiser J. (2019). Evaluation of emodepside in laboratory models of human intestinal nematode and schistosome infections. Parasites Vectors 12, 2265. doi: 10.1186/s13071-019-3476-x
Kashyap S. S., McHugh M. A., Robertson A. P., Martin R. J. (2022). Diethylcarbamazine mediated potentiation of emodepside induced paralysis requires TRP-2 in adult brugia malayi. Int. J. Parasitology. Drugs Drug Resistance 20, 108–112. doi: 10.1016/j.ijpddr.2022.10.002
Kashyap S. S., Verma S., Voronin D., Lustigman S., Kulke D., Robertson A. P., et al. (2019). Emodepside has sex-dependent immobilizing effects on adult brugia malayi due to a differentially spliced binding pocket in the RCK1 region of the SLO-1 K channel. PloS Pathog. 15, e10080415. doi: 10.1371/journal.ppat.1008041
Krücken Jürgen, Holden-Dye L., Keiser J., Prichard R. K., Townson S., Makepeace B. L., et al. (2021). Development of emodepside as a possible adulticidal treatment for human onchocerciasis—The fruit of a successful industrial–academic collaboration. PloS Pathog. 17, e1009682. doi: 10.1371/journal.ppat.1009682
Kunz L., Thalhammer A., Berg F. D., Berg U., Duffy D. M., Stouffer R. L., et al. (2002). Ca 2+ -activated, large conductance K +Channel in the ovary: identification, characterization, and functional involvement in steroidogenesis. J. Clin. Endocrinol. Metab. 87, 5566–5745. doi: 10.1210/jc.2002-020841
Latorre R., Castillo K., Carrasquel-Ursulaez W., Sepulveda R. V., Gonzalez-Nilo F., Gonzalez C., et al. (2017). Molecular determinants of BK channel functional diversity and functioning. Physiol. Rev. 97, 39–875. doi: 10.1152/physrev.00001.2016
Lee S.-H., Ock Y., Choi D., Kwak D. (2019). Gastrointestinal parasite infection in cats in daegu, Republic of Korea, and efficacy of treatment using topical emodepside/praziquantel formulation. Korean J. Parasitol. 57, 2435. doi: 10.3347/kjp.2019.57.3.243
Mencke N., Bäumer W., Fraatz K., Krebber R., Schneider M., Blazejak K. (2023). Plasma pharmacokinetics of tigolaner, emodepside, and praziquantel following topical administration of a combination product (Felpreva®) and of intravenous administration of the individual active ingredients in cats. Curr. Res. Parasitol. Vector-Borne Dis. 4, 100126. doi: 10.1016/j.crpvbd.2023.100126
Miltsch S. M., Krücken Jürgen, Demeler J., Janssen J. I., Krüger N., Harder A., et al. (2012). Decreased emodepside sensitivity in unc-49 γ-aminobutyric acid (GABA)-receptor-deficient caenorhabditis elegans. Int. J. Parasitol. 42, 761–705. doi: 10.1016/j.ijpara.2012.05.009
Moreno-Salinas A. L., Avila-Zozaya M., Ugalde-Silva P., Hernández-Guzmán D. A., Missirlis F., Boucard A. A. (2019). Latrophilins: A neuro-centric view of an evolutionary conserved adhesion G protein-coupled receptor subfamily. Front. Neurosci. 13. doi: 10.3389/fnins.2019.00700
Mrimi E. C., Welsche S., Ali S. M., Hattendorf J., Keiser J. (2023). Emodepside for trichuris trichiura and hookworm infection. New Engl. J. Med. 388, 1863–1755. doi: 10.1056/NEJMoa2212825
Noack S., Harrington J., Carithers D. S., Kaminsky R., Selzer P. M. (2021). Heartworm Disease-Overview, intervention, and industry perspective. Int. J. Parasitology Drug Dev. Resistance. 16, 65–89. doi: 10.1016/j.ijpddr.2021.03.004
Nowicka-Bauer K., Szymczak-Cendlak M. (2021). Structure and function of ion channels regulating sperm motility—An overview. Int. J. Mol. Sci. 22, 3259. doi: 10.3390/ijms22063259
Olliaro P., Seiler Jürg, Kuesel A., Horton J., Clark J. N., Don R., et al. (2011). Potential drug development candidates for human soil-transmitted helminthiases.” Edited by timothy G. Geary. PloS Negl. Trop. Dis. 5, e11385. doi: 10.1371/journal.pntd.0001138
Page S. W. (2008). Antiparasitic drugs. Small Anim. Clin. Pharmacol. 2, 198–260. doi: 10.1016/B978-070202858-8.50012-9
Peppin J. F., Pergolizzi J. V., Kraus A., Raffa R. B. (2021). Short review of ischemia- and hypoxia-protective roles of ‘Big potassium’ (BK) channels. J. Biosci. Medicines 9, 150–605. doi: 10.4236/jbm.2021.96014
Perry M. D., Sandle G. I. (2009). Regulation of colonic apical potassium (BK) channels by cAMP and somatostatin. Am. J. Physiology-Gastrointestinal Liver Physiol. 297, G159–G167. doi: 10.1152/ajpgi.00132.2009
Raisch T. (2024). The complex regulation of slo1 potassium channels from a structural perspective. Biol. Chem. doi: 10.1515/hsz-2024-0037
Raisch T., Brockmann A., Ebbinghaus-Kintscher U., Freigang Jörg, Gutbrod O., Kubicek J., et al. (2021). Small molecule modulation of the drosophila slo channel elucidated by cryo-EM. Nat. Commun. 12, 71645. doi: 10.1038/s41467-021-27435-w
Raisch T., Raunser S. (2023). The modes of action of ion-channel-targeting neurotoxic insecticides: lessons from structural biology. Nat. Struct. Mol. Biol. 30, 1411–1275. doi: 10.1038/s41594-023-01113-5
Reinemeyer C. R., Charles S. D., Buch J., Settje T., Altreuther G., Cruthers L., et al. (2005). Evaluation of the Efficacy of Emodepside plus Praziquantel Topical Solution against Ascarid Infections (Toxocara Cati or Toxascaris Leonina) in Cats. Parasitol. Res. 97, S41–S50. doi: 10.1007/s00436-005-1443-2
Saeger B., Schmitt-Wrede H.-P., Dehnhardt M., Benten P. M., Krücken Jürgen, Harder A., et al. (2001). Latrophilin-like receptor from the parasitic nematode haemonchus contortus as target for the anthelmintic depsipeptide PF1022A. FASEB J. 15, 1332–1345. doi: 10.1096/fj.00-0664fje
Salkoff L., Butler A., Ferreira G., Santi C., Wei A. (2006). High-conductance potassium channels of the SLO family. Nat. Rev. Neurosci. 7, 921–315. doi: 10.1038/nrn1992
Samson-Himmelstjerna G., Harder A., Sangster N. C., Coles G. C. (2005). Efficacy of two cyclooctadepsipeptides, PF1022A and emodepside, against anthelmintic-resistant nematodes in sheep and cattle. Parasitology 130, 343–475. doi: 10.1017/S0031182004006523
Sasaki T., Takagi M., Yaguchi T., Miyadoh S., Okada T., Koyama M. (1992). A new anthelmintic cyclodepsipeptide, PF1022A. J. Antibiotics 45, 692–975. doi: 10.7164/antibiotics.45.692
Schimmel A., Schroeder I., Altreuther G., Settje T., Charles S., Wolken S., et al. (2011). Efficacy of Emodepside plus Toltrazuril (Procox® Oral Suspension for Dogs) against Toxocara Canis, Uncinaria Stenocephala and Ancylostoma Caninum in Dogs. Parasitol. Res. 109, 1–8. doi: 10.1007/s00436-011-2397-1
Silva J.-P., Ushkaryov Y. A. (2010). The latrophilins, ‘Split-personality’ Receptors. Adv. Exp. Med. Biol. 706, 59–75. doi: 10.1007/978-1-4419-7913-1_5
Singler E. (2024). Considerations for extra-label use of emodepside to treat MADR hookworms in dogs (AAHA). Available at: https://www.aaha.org/newstat/publications/considerations-for-extra-label-use-of-emodepside-to-treat-madr-hookworms-in-dogs/.
Tanimoto N., Sothilingam V., Euler T., Ruth P., Seeliger M. W., Schubert T. (2012). BK channels mediate pathway-specific modulation of visual signals in the in vivo mouse retina. J. Neurosci. 32, 4861–4665. doi: 10.1523/JNEUROSCI.4654-11.2012
Vercruysse J., Claerebout E. (2014). “Safety of anthelmintics - pharmacology,” in Merck veterinary manual. Available at: https://www.merckvetmanual.com/pharmacology/anthelmintics/safety-of-anthelmintics (Accessed November 26, 2024).
Vercruysse J., Claerebout E. (2022). “Pharmacokinetics of anthelmintics - pharmacology,” in Merck veterinary manual. Available at: https://www.merckvetmanual.com/pharmacology/anthelmintics/pharmacokinetics-of-anthelmintics (Accessed November 26, 2024).
Verma S., Kashyap S. S., Robertson A. P., Martin R. J. (2020). Diethylcarbamazine activates TRP channels including TRP-2 in filaria, brugia malayi. Commun. Biol. 3, 3985. doi: 10.1038/s42003-020-01128-4
Walther F. M., Allan M. J., Roepke R. K.A. (2016). Safety of concurrent treatment of cats with fluralaner and emodepsid–praziquantel. Parasites Vectors 9, 3225. doi: 10.1186/s13071-016-1618-y
Willson J., Amliwala K., Davis A., Cook A., Cuttle M. F., Kriek N., et al. (2004). Latrotoxin receptor signaling engages the UNC-13-dependent vesicle-priming pathway in C. Elegans. Curr. Biol. 14, 1374–1379. doi: 10.1016/j.cub.2004.07.056
Wright I., Elsheikha H. (2018). Drug interactions amongst companion animal parasiticides. Companion Anim. 23, 314–205. doi: 10.12968/coan.2018.23.6.314
Keywords: emodepside, SLO-1K, toxicity, anthelmintic, pharmacokinetics, river blindness, hookworm, Trichuris
Citation: Njeshi CN, Robertson AP and Martin RJ (2024) Emodepside: the anthelmintic’s mode of action and toxicity. Front. Parasitol. 3:1508167. doi: 10.3389/fpara.2024.1508167
Received: 08 October 2024; Accepted: 18 November 2024;
Published: 10 December 2024.
Edited by:
Paul M. Selzer, Boehringer Ingelheim Vetmedica GmbH, GermanyCopyright © 2024 Njeshi, Robertson and Martin. This is an open-access article distributed under the terms of the Creative Commons Attribution License (CC BY). The use, distribution or reproduction in other forums is permitted, provided the original author(s) and the copyright owner(s) are credited and that the original publication in this journal is cited, in accordance with accepted academic practice. No use, distribution or reproduction is permitted which does not comply with these terms.
*Correspondence: Richard J. Martin, cmptYXJ0aW5AaWFzdGF0ZS5lZHU=
Disclaimer: All claims expressed in this article are solely those of the authors and do not necessarily represent those of their affiliated organizations, or those of the publisher, the editors and the reviewers. Any product that may be evaluated in this article or claim that may be made by its manufacturer is not guaranteed or endorsed by the publisher.
Research integrity at Frontiers
Learn more about the work of our research integrity team to safeguard the quality of each article we publish.