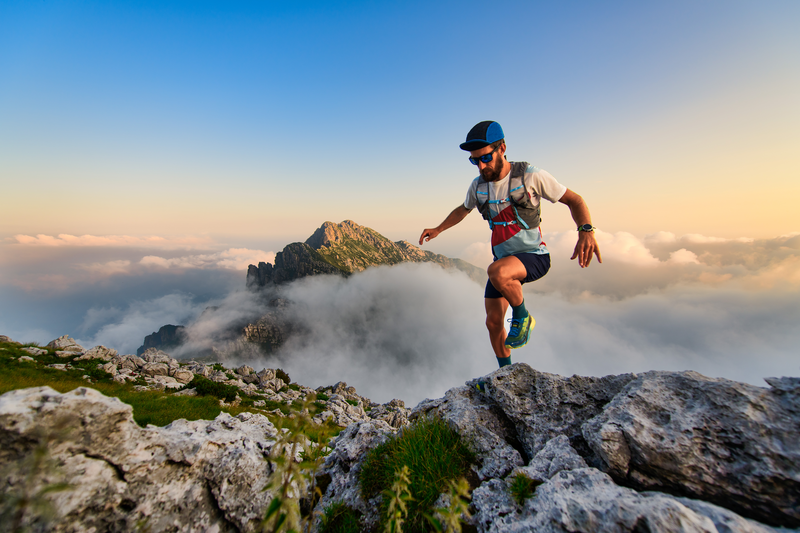
94% of researchers rate our articles as excellent or good
Learn more about the work of our research integrity team to safeguard the quality of each article we publish.
Find out more
ORIGINAL RESEARCH article
Front. Oncol. , 11 September 2024
Sec. Head and Neck Cancer
Volume 14 - 2024 | https://doi.org/10.3389/fonc.2024.1450361
Introduction: The aim of this study was to investigate the genomic changes that occur in the development from dysplasia, cancer and to regional metastases in patients with oral cavity squamous cell carcinoma (OSCC).
Material and methods: We included OSCC patients with lymph node metastases at diagnosis, treated with primary surgery at Rigshospitalet, University of Copenhagen in the period 2007-2014. The resected tumor specimens were evaluated by a pathologist, who marked areas of morphologically normal tissue and dysplasia surrounding the cancer, two areas from the cancer tissue, and one area within the lymph node metastases. From these areas a punch biopsy was taken, and DNA from each sample was extracted and sequenced using Illumina’s TSO500 HT cancer panel.
Results: From 51 OSCC patients, 255 samples were included, comprising a wide variety of genomic alterations. Substantial intratumor heterogeneity was found. The most commonly mutated gene was TP53, mutated in 65% of all samples. Only two patients had no TP53 mutation in any samples. We found that morphologically normal appearing mucosa as well as surrounding dysplasia also contained malignant mutations, supporting the theory of field cancerization. There was a significant lower average tumor mutational burden (TMB) in the lymph node metastases compared to the primary tumors, supporting the theory of clonal selection.
Conclusion: Substantial inter- and intratumor genomic heterogeneity was found. Mutation of TP53 was the most common and was present in all but two patients. Our data strongly supports the theory of clonal selection and the theory of field cancerization.
Every year, nearly 400,000 patients are diagnosed with oral cavity squamous cell carcinoma (OSCC) worldwide (1). In Denmark, OSCC is the second most common head and neck cancer with an increasing incidence over the past decades, currently at 3.5 per 100,000 per year (2). OSCC is often associated with tobacco use and excessive alcohol consumption, which are also known to have a synergistic effect on the malignant development (3–5).
Studies have demonstrated that the genetic alterations in head and neck squamous cell carcinomas are heterogeneous without common mutational signatures (6–8) a finding which might be explained by the carcinogenic effect of tobacco and alcohol introducing random DNA alterations. Substantial intratumor genomic heterogeneity has previously been reported for head and neck cancer as well as squamous cell lung carcinoma, which also is frequently associated with tobacco smoke (6, 7, 9, 10).
OSCC often evolves in a stepwise progression from mucosal dysplasia to invasive carcinoma. However, not all cases of dysplasia undergo malignant transformation—in the cases where this occurs, dysplasia is often present adjacent to the malignant tissue (11). Metastatic spread of OSCC is most frequently to regional lymph nodes of the neck and, if feasible, surgery including cervical lymph node dissection is first-line treatment (12).
In this study, using paired tissue samples from surgically resected primary tumor and lymph node metastases from patients with OSCC, our aim was to investigate the genomic alterations that occur in the development from dysplasia to cancer, and further on to lymph node metastases. In doing so, identifying potential driver mutations which could provide us with a better understanding of the developmental process leading to OSCC.
OSCC patients with lymph node metastases at diagnosis treated with primary surgery were identified from the COrCa database, which is a consecutive, population-based database of 1399 OSCC patients treated at Rigshospitalet, University of Copenhagen in the period from 2007 to 2014 (13). Clinical data on the patients were obtained through the COrCa database. Patients with lichen planus were excluded.
Formalin-fixed paraffin embedded (FFPE) resected tumor specimens and lymph node metastases were identified from the local pathology archive at Rigshospitalet. Tumor specimens were handled according to standard operating procedures and evaluated by a pathologist, who marked areas containing 1) normal tissue in the periphery of the resected specimen, 2) dysplastic tissue surrounding the cancer, 3) two areas from the cancer tissue, and 4) lymph node metastases. From these areas a punch biopsy from the corresponding paraffin block was obtained (Figure 1). In the normal tissue, the punch biopsy was 2 mm in diameter, and from the remaining areas 1 mm in diameter. All biopsies were transferred to Eppendorf tubes.
Figure 1. Surgical specimen containing morphologically normal tissue (1), dysplastic tissue (2), tumor (3), and lymph node metastasis (4)
From each FFPE tumor specimen, DNA was extracted using GeneRead DNA FFPE Kit (Qiagen, Hilden, Germany) according to manufacturer’s instructions, except for twice the amount of proteinase K and deparaffination solution were used, and samples were left overnight for proteinase K digestion at 56°C. The libraries were prepared with Illumina’s TruSight Oncology 500 High-Throughput (TSO500 HT) cancer panel (containing 523 cancer-relevant genes) according to the manufacturer’s instructions, using between 113 - 6675 ng of DNA as input material depending on the available amount of DNA from the biopsies. The libraries were sequenced on an Illumina NovaSeq 6000 (2x150 basepairs) with a minimum average coverage >295x (range 295.3-1781.5). Raw sequencing data (.bcl files) were demultiplexed into individual FastQ read files with Illumina’s bcl2fastq v2.20.0 (Illumina Inc., San Diego, CA) based on their unique index, and each sequencing library quality checked with fastQC v0.11.8.
Sequenced reads were trimmed with BBduk v38.26, mapped to hg38/GRCh38 reference genome using BWA-MEM v0.7.15, and alignment quality control performed with mosdepth v0.2.6. Somatic variants were called for each tumor sample with GATK v4.1.9.0 suite’s Mutect2 using Best Practices guidelines for Tumor-only analyses (i.e. without a paired Normal sample) and an internal Panel-Of-Normals comprising 255 non-cancer samples and a minimum sample count (–min-sample-count) of 11. Furthermore, copy number alterations (CNAs) were called using local TSO500 app v. 2.2.0 as well as TMB estimation. For all relevant analyses, the germline resource used was generated from all gnomAD variant sites with a frequency greater than 3%.
Filtering was performed using Qiagen Clinical Insight(QCI) software (Qiagen Bioinformatics, Aarhus, Denmark/Redwood City, CA, USA) including exclusion of common variants (variants more common than 0.5% in 1000 genomes project, ExAC, gnomAD, or NHLBI ESP exome), and a read depth of ≥10x. Only point mutations and CNAs that were classified as pathogenic or likely pathogenic using QCI software were included in the further data analysis and interpretation. Furthermore, CNAs were only reported if the fold change (fc) were above 2,2 (14).
For creation of the oncoplots indels longer than 10 bps, and variants with a coverage lower than 100x and a VAF lower than 5% were excluded. Further, all variants present in more than 20% of the samples were considered as germline or technical artifacts and therefore, also excluded from downstream analyses.
Oncoplots were generated with maftools R library (15). Signature plots were created using R package sigminer with hg38 reference genome package BSgenome.Hsapiens.UCSC.hg38 and Single base substitutions (SBS) mode (16, 17).
In total, tissue samples from 51 patients with OSCC were included. In all patients, samples of morphologically normal oral mucosa, dysplastic oral mucosa, the primary tumor, and lymph node metastases were available, 255 samples in total. The median age at diagnosis was 63 years (IQR: 56-70), and the majority were men (n=35, 69%). Most patients were smokers or previous smokers at diagnosis (n=43, 84%), and 59% had an excessive alcohol intake or prior excessive alcohol intake (n=30). The tongue and the floor of the mouth (n=22, 43%, and n=21, 41%) were the most common sublocations, and most patients were diagnosed in UICC7 T-stage 2 (n=22, 43%) (Table 1).
The number of point mutations and copy number alterations (CNAs) varied between patients. All patients harbored at least one pathogenic or likely pathogenic point mutation, however not all had relevant CNAs.
The highest number of point mutations in one patient was 16, while the highest number of CNAs in one patient was 13. The patients with highest number of genetic alterations in total had 21 different point mutations and CNAs.
Several of the genomic alterations present in the malignant tissue were also seen in the morphologically normal tissue samples of the same patients, and only six patients (12%) had no genomic alterations in their morphologically normal tissue sample. The remaining had at least one point mutation; no patients had only CNAs in their morphologically normal tissue sample.
Comparing matched tumor samples substantial intratumor heterogeneity was found. Only eight patients (16%) shared all genomic alterations in their matched tumor samples, and even in these cases the VAF and fold changes were different between the two samples.
The overall average tumor mutational burden (TMB) score per sample was 4.5 mut/Mb. For the different tissue categories, the highest average TMB score was 6.1 mut/Mb for tumor samples, while normal tissue samples had the lowest average TMB score at 1.9 mut/Mb. Lymph node metastases had significantly lower average TMB score than tumor samples. There were significant differences in TMB score between all tissue categories, except between dysplasia and lymph node metastasis (Figure 2).
Figure 2. Tumor mutation burden (TMB) in morphologically normal mucosa, dysplasia, carcinoma, and lymph node metastases.
Most variants were missense mutations, followed by frameshift and stop gain mutations. Alteration of TP53 was the most frequent, altered in 65% of all samples. Other commonly mutated genes were FAT1 (32%) and CNAQ (30%). Based on VAF of the most commonly mutated genes in the different tissue categories, CDKN2A appears to be a late event, occurring in the primary tumor, while TP53, FAT1, QNAC, NOTCH1 and BCR are early events, arising in the morphologically normal tissue and dysplasia (Table 2, Figure 3).
Figure 3. Oncoplot including all samples and 40 most frequently mutated genes, including information on type of mutation, and clinical data on patients.
TP53 mutations were very frequent; only two patients (4%) did not have any TP53 mutations in any sample—the remaining patients had between one and seven different mutations in TP53. The most common variant, present in eight patients, was a well-known hotspot missense mutation (c.524G>A p.R175H). The normal tissue samples of 22 patients (41%) contained TP53 mutations, in most cases in very low frequencies around 1-2%, and only in few cases the frequency increased noteworthy going through the pre-cancer, tumor, and metastasis tissue.
The patients (N=28, 55%) with no TP53 mutations in their normal tissue who gained the mutation in the pre-cancer or tumor tissue often had higher frequencies and increasing frequencies throughout the development of the cancer.
Amplifications were seen in 31 different genes, the most common was FGF3, seen in 20 patients (39%), followed by FGF19, and CCND1 seen in 18 (35%) and 26 (51%) patients, respectively. Opposite to the frequent presence of point mutations in normal tissue, amplifications were not seen in the normal tissue samples for any patient and did often not occur before tumor samples.
The highest fold change was seen for FGF3 in a lymph node metastasis sample, at 16.4. FGF3 had the highest fold change in dysplasia and tumor samples as well, 7.4 and 12.3, respectively. Other genes showing high fold change were FGF19 (highest 14.4 in a lymph node metastasis sample), CCND1 (highest 14.3 in a lymph node metastasis samples), and EGFR (highest 11,0 in a tumor sample).
To characterize the biological processes during transition from normal epithelium to dysplasia and cancer in OSCC, we finally performed a gene set enrichment analysis employing gene expression data from Khan et al. (GSE227919), examining a cohort (n=66) of patients with early oral lesions consisting of premalignant lesions (hyperkeratosis and dysplasia) and OSCC as well as normal controls. We examined the gene ontology biological processes C5 collection as described (18). As shown in (Figure 4) there is an upregulation of gene sets involved in epithelium to mesenchymal transition (EMT) during progression to dysplasia, whereas, the progression from dysplasia to carcinoma mainly involves clonal expansion characterized by upregulation of check point and mitotic signaling (Panel B). This tendency is also apparent in the volcano plot where keratins are prominent in dysplasia and in the two-way clusters showing the clear mitotic profile in the carcinoma (C).
Figure 4. Biological processes during transition from normal cell to dysplasia and cancer in oral squamous cell carcinoma. (A) Gene set enrichment analysis (GSEA) of normal oral cells compared with cells undergoing dysplasia (upper panel) and of dysplastic cells compared with squamous cell carcinoma (lower panel). The normalized dataset was generated by Khan et al.21 and was downloaded from the gene expression omnibus (GEO) (GSE227919). The GSEA was performed as described20 in Qlucore Omics Explorer 3.9,0 www.qlucore.com employing the gene ontology biological processes C5 collection of 7647 gene sets available at https://www.gsea-msigdb.org/gsea/msigdb/human/collections.jsp#C5. The table panels show the 10 most enriched gene sets ranked according to their normalized enrichment score (NER). Moreover, the gene set size and matches, as well, the p and q values are indicated. (B) Volcano plot of transcripts exhibiting differential expression between normal cells and dysplasia (left panel) and dysplasia versus cancer (right panel). Upregulated transcripts are show to the right and are marked in red. Outstanding mRNAs are labelled with their gene name. (C) Two-way hierarchical clusters of the two in most enriched gene sets during the transition from normal cell to dysplasia and from dysplasia to cancer, respectively. The class is indicated below and shown on the top of the clusters. Sample codes from the study by Kahn et al. is shown below. (D) Schematic summary of the molecular alterations leading to dysplasia and cancer in oral squamous carcinoma. Mutations occurring during the transition from normal epithelium to dysplasia and cancer are indicated on the top of the schematic representation of the epithelium and the accompanying biological processes are shown below.
Using targeted DNA sequencing, we analyzed 255 paired tissue samples from 51 patients with OSCC. From each patient samples were obtained from morphologically normal mucosa, mucosal dysplasia, two samples from the primary cancer, and lymph node metastases. In line with previous studies, the mutational landscape of OSCC tumors as well as dysplasia and lymph node metastases was heterogeneous, with OSCC tumors also demonstrating substantial intratumor heterogeneity (6, 7, 10, 19). Several of the mutations found in the dysplasia and primary tumor samples were also found in the surrounding morphologically normal tissue, supporting the theory of field cancerization, in which the long-term exposure to carcinogens such as tobacco induces potentially carcinogenic genomic alterations in cells throughout the exposed mucosa (11, 20, 21). - leaving the mucosa which has not undergone malignant transformation in increased risk of doing so over time owing to the accumulation of genomic alterations, which clinically manifests as multifocal carcinoma and/or a tendency to mucosal recurrence outside of the initial T-site (22).
Our data indicate that mutation of TP53, as well as FAT1, GNAQ, NOTCH1, and BCR seems to be early events, arising in the morphologically normal tissue and dysplasia. We found TP53 to be the most frequently mutated gene identified in 49 patients (96%). TP53 is a well-known tumor suppressor gene associated with many different types of cancer, and has been reported as the most frequently mutated gene in OSCC (23–30). TP53 encodes p53 that plays an important role in activating DNA repair and arresting cell growth, as well as inducing apoptosis in cells with DNA damage. Mutation of TP53 has been linked with the multistep process of EMT, including acquiring the ability to break down extracellular matrix, tumor invasion, as well as intra- and extravasation (31, 32). In line with this, mutation of TP53 has been shown to be associated with increased migratory and invasive potential and may increase risk of malignant transformation to OSCC when found in dysplasia (25, 32).
Like TP53, NOTCH1, and FAT1, are involved in EMT through the NOTCH/Jagged pathway and FAT1/HIPPO pathway, respectively (33–35). Knockdown of GNAQ has been shown to induce mesenchymal stem cell like properties in lung cancer cells (36, 37).
Mutation of CDKN2A appears to be a late event, occurring in the primary tumor. CDKN2A affects cell cycle regulation, and encodes both p16(INK4a), which is an inhibitor of cyclin dependent kinase (CDK) and p14(ARF), a p53 stabilizer (38, 39). Germline mutation of CDKN2A has been linked with early onset OSCC (38).
In this way, the variant data implies that EMT in the dysplastic foci precedes clonal expansion of malignant cells in the tumor. The general view is in line with the gene expression data from Khan et al (19), where a gene set enrichment analysis (GSEA) showed that premalignant lesions were enriched in gene signatures associated with cellular plasticity with partial EMT phenotypes, and immune response. Our stepwise analysis of data from Khan et al. indicates that going from normal tissue to dysplasia is characterized by EMT and that tumors exhibit a strong mitotic component compared to the dysplastic foci.
Previous studies show increased risk of malignant transformation over time in benign oral lesions with higher grades of dysplasia, but it has also been suggested that underlying genetic alterations do not necessarily correlate with histomorphology, as some lesions with mild dysplasia have demonstrated genetic alterations similar to those found in lesions with severe dysplasia (40, 41). Patterns of immune infiltration of OSCC may also be linked with prognosis in OSCC (42) – to the best of our knowledge, definitive biomarkers to distinguish between lesions that remain benign and lesions that will undergo malignant transformation has yet to be discovered.
In head and neck squamous cell carcinoma (HNSCC), amplification of 11q13, containing among others CCDN1, FGF3, and FGF19, is frequent and has been linked with a poor prognosis (43, 44). The most frequent amplification in our study was FGF3, seen in 20 patients (39%). This has also been reported as a frequent amplification in OSCC by Nakagaki et al. and Ribeiro et al., along with amplification of CCND1, which was also among the most frequent in our study—co-amplification of FGF3 and CCND1 was often observed in their cohort as well as in our cohort (28, 45). CCND1 is also frequently co-amplified with FGF19 in head and neck squamous cell carcinoma, amplification of which was seen in 18 (35%) of patients in our cohort—FGF19 is involved in HNSCC tumorigenesis and may be useful as a target for therapy (46).
From a clinical perspective, the results of this study, with clear support of the theory of field cancerization, might explain the relatively frequent local recurrences seen in OSCC, even among patients treated radically with surgery. It also underlines the importance of smoking cessation after diagnosis of OSCC. With continued smoking follows a high risk of inducing further malignant mutations in already exposed mucosa.
Unfortunately, our results also indicates that there are no obvious common mutational patterns that can serve as a target of therapy to be used in the broad group of OSCC patients. Targeted therapy probably needs to be individualized to each specific patient, based on their specific mutations.
The strengths of this study lie in the large number of patients, with matched tissue samples from morphologically normal mucosa, dysplasia, tumor, and lymph node metastasis as well as the availability of clinical data. As less than 20% of patients with oral dysplasia will progress to OSCC, future studies should explore differences in dysplasia in patients that later develop OSCC and patients that do not develop OSCC to identify potential biomarkers of increased risk of malignant transformation.
Based on targeted DNA analysis of 255 paired samples from 51 OSCC patients, we found substantial inter- and intratumor genomic heterogeneity. Mutation of the well-known cancer gene TP53 was present in all but two patients. We found that morphologically normal appearing mucosa as well as surrounding dysplasia also contained mutations, supporting the theory of field cancerization and mucosa at risk. Our data as well as data from a previous study by Khan et al. indicates that the development from normal tissue to dysplasia is characterized by EMT, and the development from dysplasia to cancer are characterized by mutations in regulators of proliferation.
According to Danish data protection law, data from this study are not allowed to be made publicly available. Transfer of data requires permission from the Danish data protection authorities for the specific transfer. This can be applied for upon request to the corresponding author.
The studies involving humans were approved by Committee on Health Research Ethics (Case nr.: 2004313). The studies were conducted in accordance with the local legislation and institutional requirements. The participants provided their written informed consent to participate in this study.
JJ: Conceptualization, Formal analysis, Funding acquisition, Investigation, Methodology, Project administration, Writing – original draft, Writing – review & editing. SS: Conceptualization, Data curation, Formal analysis, Funding acquisition, Investigation, Methodology, Visualization, Writing – original draft, Writing – review & editing. JC: Data curation, Formal analysis, Visualization, Writing – original draft, Writing – review & editing. LA: Data curation, Formal analysis, Writing – original draft, Writing – review & editing. FV: Data curation, Formal analysis, Writing – original draft, Writing – review & editing. FN: Conceptualization, Data curation, Formal analysis, Investigation, Methodology, Supervision, Visualization, Writing – original draft, Writing – review & editing. KK: Conceptualization, Resources, Writing – original draft, Writing – review & editing. CG: Conceptualization, Resources, Writing – original draft, Writing – review & editing. CV: Conceptualization, Funding acquisition, Resources, Supervision, Writing – original draft, Writing – review & editing.
The author(s) declare financial support was received for the research, authorship, and/or publication of this article. JJ received grants from the following funds for this project: Tømrermester Jørgen Holm og hustru Elisa F Hansen Mindelegat (Grant number: 20012). Aase og Ejnar Danielsens Fond (Grant number: 20-10-0059). Direktør Michael Hermann Nielsens Fond (Grant number: 2020-0082968). Eva og Henry Fraenkels Mindefond (no grant number). Fabrikant Einar Willumsens mindelegat (no grant number). Kong Christian den Tiendes Fond (no grant number). Kraeftens Bekaempelse (grant number: R214-A12947-18-S7). Rigshospitalets Forskningspuljer (no grant number) CB received a grant for this project from Svend Andersen Fonden (no grant number).
We would like to thank the authors of the study “Total RNA sequencing reveals gene expression and microbial alterations shared by oral pre-malignant lesions and cancer.” By Khan et al., for making their data available for additional analysis.
The authors declare that the research was conducted in the absence of any commercial or financial relationships that could be construed as a potential conflict of interest.
All claims expressed in this article are solely those of the authors and do not necessarily represent those of their affiliated organizations, or those of the publisher, the editors and the reviewers. Any product that may be evaluated in this article, or claim that may be made by its manufacturer, is not guaranteed or endorsed by the publisher.
1. Sung H, Ferlay J, Siegel RL, Laversanne M, Soerjomataram I, Jemal A, et al. Global cancer statistics 2020: GLOBOCAN estimates of incidence and mortality worldwide for 36 cancers in 185 countries. CA Cancer J Clin. (2021) 71:209–49. doi: 10.3322/CAAC.21660
2. Jakobsen KK, Grønhøj C, Jensen DH, Karnov KKS, Agander TK, Specht L, et al. Increasing incidence and survival of head and neck cancers in Denmark: a nation-wide study from 1980 to 2014. Acta Oncol (Madr). (2018) 57:1–9. doi: 10.1080/0284186X.2018.1438657
3. Schmidt BL, Dierks EJ, Homer L, Potter B. Tobacco smoking history and presentation of oral squamous cell carcinoma. J Oral Maxillofac Surg. (2004) 62:1055–8. doi: 10.1016/J.JOMS.2004.03.010
4. Mello FW, Melo G, Pasetto JJ, Silva CAB, Warnakulasuriya S, Rivero ERC. The synergistic effect of tobacco and alcohol consumption on oral squamous cell carcinoma: a systematic review and meta-analysis. Clin Oral Investig. (2019) 23:2849–59. doi: 10.1007/S00784-019-02958-1
5. Andersen AO, Jensen JS, Jakobsen KK, Stampe H, Nielsen KJ, Wessel I, et al. The impact of tobacco smoking on survival of patients with oral squamous cell carcinoma: a population-based retrospective study. Acta Oncol (Madr). (2022) 61:449–58. doi: 10.1080/0284186X.2022.2033830
6. Mroz EA, Rocco JW. Intra-tumor heterogeneity in head and neck cancer and its clinical implications. World J Otorhinolaryngol Head Neck Surg. (2016) 2:60–7. doi: 10.1016/j.wjorl.2016.05.007
7. Gram SB, Alosi D, Bagger FO, Østrup O, von Buchwald C, Friborg J, et al. Clinical implication of genetic intratumor heterogeneity for targeted therapy in head and neck cancer. Acta Oncol (Madr). (2023) 62:1831–9. doi: 10.1080/0284186X.2023.2272293
8. Chai AWY, Lim KP, Cheong SC. Translational genomics and recent advances in oral squamous cell carcinoma. Semin Cancer Biol. (2020) 61:71–83. doi: 10.1016/j.semcancer.2019.09.011
9. Jamal-Hanjani M, Wilson GA, McGranahan N, Birkbak NJ, Watkins TBK, Veeriah S, et al. Tracking the evolution of non-small-cell lung cancer. N Engl J Med. (2017) 376:2109–21. doi: 10.1056/NEJMOA1616288
10. Mroz EA, Tward AM, Hammon RJ, Ren Y, Rocco JW. Intra-tumor genetic heterogeneity and mortality in head and neck cancer: analysis of data from the cancer genome atlas. PloS Med. (2015) 12:1–27. doi: 10.1371/journal.pmed.1001786
11. Mohan M, Jagannathan N. Oral field cancerization: An update on current concepts. Oncol Rev. (2014) 8:13–9. doi: 10.4081/oncol.2014.244
12. Chia C, Key S, Hasan Z, Virk S, Riffat F. Systematic review and meta-analysis of cervical metastases in oral maxillary squamous cell carcinoma. Cancer Rep. (2021) 4:1–13. doi: 10.1002/cnr2.1410
13. Schmidt Jensen J, Jakobsen KK, Mirian C, Christensen JT, Schneider K, Nahavandipour A, et al. The Copenhagen Oral Cavity Squamous Cell Carcinoma database : protocol and report on establishing a comprehensive oral cavity cancer database. Clin Epidemiol. (2019) 11:733–41. doi: 10.2147/CLEP
14. Illumina. TruSight™ Oncology 500 and TruSight Oncology 500 High-Throughput (Accessed February 7, 2024). Pub. no. 1170-2018-010-G.
15. Mayakonda A, Lin DC, Assenov Y, Plass C, Koeffler HP. Maftools: Efficient and comprehensive analysis of somatic variants in cancer. Genome Res. (2018) 28:1747–56. doi: 10.1101/gr.239244.118
16. Wang S, Tao Z, Wu T, Liu XS. Sigflow: an automated and comprehensive pipeline for cancer genome mutational signature analysis. Bioinformatics. (2021) 37:1590–2. doi: 10.1093/bioinformatics/btaa895
17. Wang S, Li H, Song M, Tao Z, Wu T, He Z, et al. Copy number signature analysis tool and its application in prostate cancer reveals distinct mutational processes and clinical outcomes. PloS Genet. (2021) 17. doi: 10.1371/journal.pgen.1009557
18. Subramanian A, Tamayo P, Mootha VK, Mukherjee S, Ebert BL, Gillette MA, et al. Gene set enrichment analysis: A knowledge-based approach for interpreting genome-wide expression profiles. PNAS. (2005) 102:15545–50. doi: 10.3969/j.issn.0372-2112.2018.08.016
19. Khan MM, Frustino J, Villa A, Nguyen BC, Woo SB, Johnson WE, et al. Total RNA sequencing reveals gene expression and microbial alterations shared by oral pre-malignant lesions and cancer. Hum Genomics. (2023) 17:1–14. doi: 10.1186/s40246-023-00519-y
20. Feller G, Khammissa RAG, Ballyram R, Beetge MM, Lemmer J, Feller L. Tumour genetic heterogeneity in relation to oral squamous cell carcinoma and anti-cancer treatment. Int J Environ Res Public Health. (2023) 20. doi: 10.3390/ijerph20032392
21. Califano J, van der Riet P, Westra W, Nawroz H, Clayman G, Piantadosi S, et al. Genetic progression model for head and neck cancer: Implications for field cancerization. Cancer Res. (1996) 56:2488–92. doi: 10.1016/S0194-5998(96)80631-0
22. Peralta-Mamani M, Terrero-Pérez Á, Tucunduva RMA, Rubira CMF, Santos PSDS, Honório HM, et al. Occurrence of field cancerization in clinically normal oral mucosa: A systematic review and meta-analysis. Arch Oral Biol. (2022) 143:143. doi: 10.1016/j.archoralbio.2022.105544
23. Lindemann A, Takahashi H, Patel AA, Osman AA, Myers JN. Targeting the DNA damage response in OSCC with TP53 mutations. J Dent Res. (2018) 97:635–44. doi: 10.1177/0022034518759068
24. Deneka AY, Baca Y, Serebriiskii IG, Nicolas E, Parker MI, Nguyen TT, et al. Association of TP53 and CDKN2A mutation profile with tumor mutation burden in head and neck cancer. Clin Cancer Res. (2022) 28:1925–37. doi: 10.1158/1078-0432.CCR-21-4316
25. Holbrook WP, Ögmundsdottir HM. Oral lichen planus and mutated TP53—A road to cancer? Dent J (Basel). (2022) 10:1–8. doi: 10.3390/dj10090176
26. Stransky N, Egloff AM, Tward AD, Kostic AD, Cibulskis K, Sivachenko A, et al. The mutational landscape of head and neck squamous cell carcinoma. Sci (1979). (2012) 333:1157–60. doi: 10.1126/science.1208130.The
27. Maitra A, Biswas NK, Amin K, Kowtal P, Kumar S, Das S, et al. Mutational landscape of gingivo-buccal oral squamous cell carcinoma reveals new recurrently-mutated genes and molecular subgroups. Nat Commun. (2013) 4. doi: 10.1038/ncomms3873
28. Nakagaki T, Tamura M, Kobashi K, Koyama R, Fukushima H, Ohashi T, et al. Profiling cancer-related gene mutations in oral squamous cell carcinoma from Japanese patients by targeted amplicon sequencing. Oncotarget. (2017) 8:59113–22. doi: 10.18632/oncotarget.19262
29. Jayaprakash C, Varghese VK, Jayaram P, Chakrabarty S, Kudva A, Ray S, et al. Relevance and actionable mutational spectrum in oral squamous cell carcinoma. J Oral Pathol Med. (2020) 49:427–34. doi: 10.1111/jop.12985
30. Levine AJ. P53: 800 million years of evolution and 40 years of discovery. Nat Rev Cancer. (2020) 20:471–80. doi: 10.1038/s41568-020-0262-1
31. Tang Q, Su Z, Gu W, Rustgi A k. Mutant p53 on the path to metastasis. Trends Cancer. (2020) 6:62–73. doi: 10.1016/j.trecan.2019.11.004.Mutant
32. Navarange SS, Bane SM, Mehta D, Shah S, Gupta S, Waghmare SK. Epithelial-to-mesenchymal transition status correlated with ultrastructural features, and TP53 mutation in patient-derived oral cancer cell lines. Mol Biol Rep. (2023) 50:8469–81. doi: 10.1007/s11033-023-08720-x
33. Natsuizaka M, Whelan KA, Kagawa S, Tanaka K, Giroux V, Chandramouleeswaran PM, et al. Interplay between Notch1 and Notch3 promotes EMT and tumor initiation in squamous cell carcinoma. Nat Commun. (2017). doi: 10.1038/s41467-017-01500-9
34. Liao C, Wang Q, An J, Long Q, Wang H, Xiang M, et al. Partial EMT in squamous cell carcinoma: A snapshot. Int J Biol Sci. (2021) 17:3036–47. doi: 10.7150/ijbs.61566
35. Chen ZG, Saba NF, Teng Y. The diverse functions of FAT1 in cancer progression: good, bad, or ugly? J Exp Clin Cancer Res. (2022) 41:1–11. doi: 10.1186/s13046-022-02461-8
36. Nishikawa Y, Miyazaki T, Nakashiro KI, Yamagata H, Isokane M, Goda H, et al. Human FAT1 Cadherin controls cell migration and invasion of oral squamous cell carcinoma through the localization of β-catenin. Oncol Rep. (2011) 26:587–92. doi: 10.3892/or.2011.1324
37. Choi JY, Lee YS, Shim DM, Lee YK, Seo SW. GNAQ knockdown promotes bone metastasis through epithelial-mesenchymal transition in lung cancer cells. Bone Joint Res. (2021) 10:310–20. doi: 10.1302/2046-3758.105.BJR-2020-0262.R3
38. Jeong AR, Forbes K, Orosco RK, Cohen EEW. Hereditary oral squamous cell carcinoma associated with CDKN2A germline mutation: a case report. J Otolaryngol - Head Neck Surge. (2022) 51:1–9. doi: 10.1186/s40463-022-00556-y
39. Usman S, Jamal A, Teh MT, Waseem A. Major molecular signaling pathways in oral cancer associated with therapeutic resistance. Front Oral Health. (2021) 1:603160. doi: 10.3389/froh.2020.603160
40. Warnakulasuriya S, Kovacevic T, Madden P, Coupland VH, Sperandio M, Odell E, et al. Factors predicting Malignant transformation in oral potentially Malignant disorders among patients accrued over a 10-year period in South East England. J Oral Pathol Med. (2011) 40:677–83. doi: 10.1111/j.1600-0714.2011.01054.x
41. Brenes DR, Nipper AJ, Tan MT, Gleber-Netto FO, Schwarz RA, Pickering CR, et al. Mildly dysplastic oral lesions with optically-detectable abnormalities share genetic similarities with severely dysplastic lesions. Oral Oncol. (2022) 135. doi: 10.1016/j.oraloncology.2022.106232
42. Chen Y, Feng Y, Yan F, Zhao Y, Zhao H, Guo Y. A novel immune-related gene signature to identify the tumor microenvironment and prognose disease among patients with oral squamous cell carcinoma patients using ssGSEA: A bioinformatics and biological validation study. Front Immunol. (2022) 13:922195. doi: 10.3389/fimmu.2022.922195
43. Dou S, Zhang L, Wang C, Yao Y, Jiang W, Ye L, et al. EGFR mutation and 11q13 amplification are potential predictive biomarkers for immunotherapy in head and neck squamous cell carcinoma. Front Immunol. (2022) 13:813732. doi: 10.3389/fimmu.2022.813732
44. Ramos-García P, Ruiz-Ávila I, Gil-Montoya JA, Ayén Á, González-Ruiz L, Navarro-Triviño FJ, et al. Relevance of chromosomal band 11q13 in oral carcinogenesis: An update of current knowledge. Oral Oncol. (2017) 72:7–16. doi: 10.1016/j.oraloncology.2017.04.016
45. Ribeiro IP, Marques F, Caramelo F, Pereira J, Patrício M, Prazeres H, et al. Genetic gains and losses in oral squamous cell carcinoma: Impact on clinical management. Cell Oncol. (2014) 37:29–39. doi: 10.1007/s13402-013-0161-5
Keywords: oral squamous cell carcinoma, head and neck cancer, carcinoma, genomics, metastasis
Citation: Jensen JM, Sjöstedt SMS, Carmona JL, Ahlborn LB, Vieira FG, Nielsen FC, Kiss K, Grønhøj C and von Buchwald C (2024) Genomic alterations in the stepwise progression from normal mucosa to metastasizing oral squamous cell carcinoma. Front. Oncol. 14:1450361. doi: 10.3389/fonc.2024.1450361
Received: 17 June 2024; Accepted: 22 August 2024;
Published: 11 September 2024.
Edited by:
Peter Polverini, University of Michigan, United StatesReviewed by:
Eliska Svastova, Slovak Academy of Sciences, SlovakiaCopyright © 2024 Jensen, Sjöstedt, Carmona, Ahlborn, Vieira, Nielsen, Kiss, Grønhøj and von Buchwald. This is an open-access article distributed under the terms of the Creative Commons Attribution License (CC BY). The use, distribution or reproduction in other forums is permitted, provided the original author(s) and the copyright owner(s) are credited and that the original publication in this journal is cited, in accordance with accepted academic practice. No use, distribution or reproduction is permitted which does not comply with these terms.
*Correspondence: Jakob Myllerup Jensen, SmFrb2IubXlsbGVydXAuamVuc2VuQHJlZ2lvbmguZGs=
Disclaimer: All claims expressed in this article are solely those of the authors and do not necessarily represent those of their affiliated organizations, or those of the publisher, the editors and the reviewers. Any product that may be evaluated in this article or claim that may be made by its manufacturer is not guaranteed or endorsed by the publisher.
Research integrity at Frontiers
Learn more about the work of our research integrity team to safeguard the quality of each article we publish.