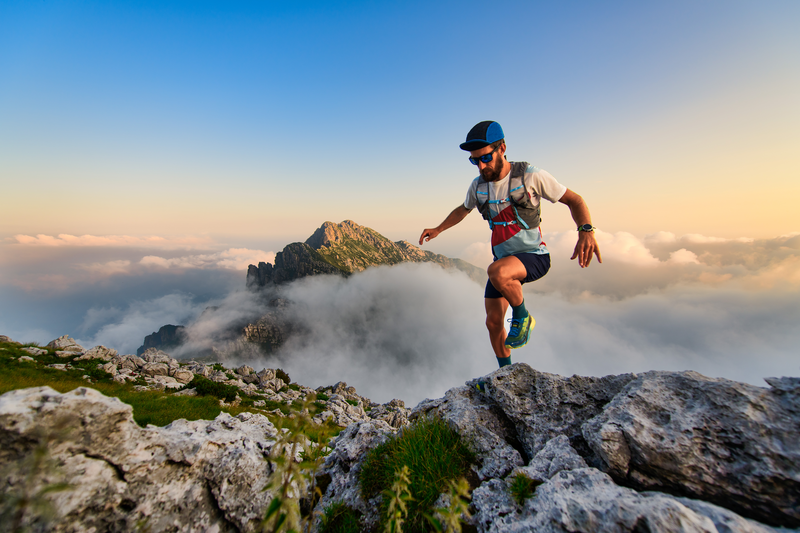
95% of researchers rate our articles as excellent or good
Learn more about the work of our research integrity team to safeguard the quality of each article we publish.
Find out more
SYSTEMATIC REVIEW article
Front. Oncol. , 25 November 2024
Sec. Cancer Molecular Targets and Therapeutics
Volume 14 - 2024 | https://doi.org/10.3389/fonc.2024.1448760
Early detection of cancer typically facilitates improved patient outcomes; however, many cancers are not easily diagnosed at an early stage. One potential route for developing new, non-invasive methods of cancer detection is by testing for cancer-related volatile organic compounds (VOCs) biomarkers in patients’ urine. In this review, 44 studies covering the use and/or identification of cancer-related VOCs were examined, including studies which examined multiple types of cancer simultaneously, as well as diverse study designs. Among these studies the most studied cancers included prostate cancer (29% of papers), lung cancer (22%), breast cancer (20%), and bladder cancer (18%), with a smaller number of studies focused on colorectal cancer, cervical cancer, skin, liver cancer and others. Importantly, most studies which produced a VOC-based model of cancer detection observed a combined sensitivity and specificity above 150%, indicating that urine-based methods of cancer detection show considerable promise as a diagnostic tool. Mass spectrometry (MS) and electronic noses (eNose) were the most employed tools used in the detection of VOCs, while animal-based models were less common. In terms of VOCs of interest, 47 chemical species identified as correlated with various types of cancer in at least two unrelated papers, some of which were consistently up- or down-regulated in cancer patients, and which may represent useful targets for future studies investing urinary VOC biomarkers of cancer. Overall, it was concluded that research in this field has shown promising results, but more work may be needed before the widespread adoption of these techniques takes place.
Early detection of cancer will significantly increase the probability of successful treatment, while late detection often results in advanced diseases at presentation and more limited treatment options (1). Cancer is a leading cause of death in Canada, and worldwide, warranting the development of new tools and techniques to facilitate early cancer detection, which could provide substantive benefits to both patients, as well as to healthcare providers (2, 3). These new detection methods would be particularly valuable for some types of cancer which are either difficult to detect, such as pancreatic ductal adenocarcinoma, hepatocellular carcinoma, colorectal cancer, and lung cancer; or are otherwise significantly deadlier if not detected early, such as breast cancer, prostate cancer, or skin cancer (Table 1) (3, 4).
Table 1. Five-year mortality rates of various cancers at early or late stage – seer cancer statistics.
One potential route for developing these new methods is by creating diagnostic tests for the presence or concentrations of specific compounds, or biomarkers, which can be used as proxies for metabolic processes occurring within the body. Depending on the compounds chosen, this strategy may allow healthcare providers to identify subtle physiological changes in an individual brought on by a particular disease condition, potentially before the onset of any outward symptoms (5). Many different types of compounds have already been extensively used as biomarkers for cancer detection, including proteins (prostate-specific antigen, carcinoembryonic antigen, carbohydrate antigen 19-9, etc.), and nucleic acids/genes (BRCA1, BRCA2, and KRAS genes). However, some of these biomarker tests require relatively complicated and specialized methods to obtain and extract, or require samples be taken from blood or other tissues – which is often highly invasive and requires specialized medical expertise (5).
Smaller volatile organic compounds (VOCs), offer the potential to be more convenient as biomarkers due to their characteristic ability to spontaneously evaporate into a gaseous state, separating themselves from a particular sample without the need for extraction procedures. These compounds are naturally exhaled in breath and excreted in urine and are usually thought to originate as the end products of certain metabolic processes. As a result, monitoring the presence or concentration of these compounds can be used to identify metabolic changes brought on by disease conditions, including cancer (6, 7). Notably, breath and urine are often considered to be most convenient types of samples when developing a diagnostic test, as these fluids are naturally and routinely expelled from the body and can be simply be collected thereafter (8).
While this review focuses on urine-based testing exclusively, it is important to acknowledge that numerous studies have explored breath-cased cancer detection systems, including some included in this review itself which sought to compare the two sample types, and moreover that such methods do offer several advantages (8–11). For instance, breath testing is extremely simple and almost entirely non-invasive – requiring only that patients exhale into a specialized device, something that can be done multiple times per day and on demand. In comparison, collecting urine samples is not necessarily possible at times, as certain health conditions can make urinating on demand difficult, and urine sampling can be perceived as awkward or embarrassing for patients (12).
On the other hand, urine sampling does offer its own advantages. For instance, urine is a much more voluminous and concentrated fluid compared to a breath sample, and therefore may contain more and a higher concentration of VOCs of interest (11). Urine collection also requires very few components as patients must simply urinate into a special sealable cup, and samples can thereafter be frozen for storage. Breath sampling in turn generally requires the use of specialized devices which serve the purpose of preserving and concentrating the sample prior to subsequent analyses (12). Finally, it should be noted that both breath and urine sample composition can be significantly altered by various non-disease factors, and which may need be corrected for when developing a diagnostic tool. In the case of breath sampling, these include factors such as breathing patterns, lung volume, diet, smoking, or environmental exposure (11, 13); while for urination notable factors include diet and level of hydration, time of urination, the presence of medications or supplements, levels of physical activity and others (5, 11, 14–16).
The purpose of this review was to provide a general summary of published literature in the last eleven years, and particularly within the last five years, related to the use of urinary VOCs as potential biomarkers for various types of cancer. To achieve this, published articles were searched using keywords. cancer, volatile’, and ‘urine’. From this initial search, a total of 711 articles were initially identified, and then manually screened for suitability of inclusion. Articles which did not directly relate to the identification, measurement, or at least recognition of volatile compounds, or patterns thereof, produced from the urine of cancer patients were excluded. This left 44 papers to be included in the final review, specific VOCs identified in these papers were included only if they were present exclusively in the cancer patient group, or else were described as statistically significantly up- or down-regulated in cancer patients by the authors. A list of all the referenced papers is included in Table 2.
The studies examined in this review were found to have mainly focused on prostate cancer (covered by 29% of articles), lung cancer (22%), breast cancer (20%), and bladder cancer (18%), while fewer studies were dedicated to pancreatic ductal adenocarcinoma, renal cell adenocarcinoma, and other cancers (4%). Note that in several studies, multiple cancer types were analyzed together. This distribution is justifiable, given prostate cancer, bladder cancer, and breast cancer, all have relatively high 5-year survival rates when detected at an early stage, and as such any new methods which can increase their rate of early detection should be considered highly desirable. Moreover, studies focused on lung cancer, breast cancer, and prostate cancer can further be justified due to these cancers being responsible for the 1st, 4th, and 5th most US cancer-related deaths between 2018 to 2022, and as such represent some of the most lethal cancers overall. On the other hand, colorectal cancer, and pancreatic ductal adenocarcinoma, despite being responsible for the 2nd and 3rd most US cancer deaths between 2018 to 2022, appear to have received considerably less attention from researchers (4). In the former case, this apparent disinterest may be because several new diagnostic technologies are already under development, including methods based on DNA analysis from fecal samples, monitoring changes in the gut microbiome, and using the detection of VOCs present in breath (17). Pancreatic ductal adenocarcinoma, in turn, may be relatively overlooked due to a combination of being relatively rare, and highly lethal even when detected at earlier stages, making it a less attractive option for developing new diagnostic tools (18). Alternatively, it may be difficult to separate the effects of pancreatic ductal adenocarcinoma from pancreatitis, possibly reducing the potential value of a VOC-based diagnostic tool for this specific disease (19).
Unsurprisingly, a diverse set of sampling strategies were employed by the various studies included in this review, the details of which are summarized in Table 3. Despite this, several trends can be noted, for instance the typical study included in this review is relatively small, involving roughly 90 cancer patients, and 64 control subjects, with only a single paper detailing a cancer patient population with more than 200 individuals. It also is common for studies to include larger populations with cancer compared to control populations, and in some cases these numbers appear quite unbalanced. For instance, the most unbalanced study involved in this review utilized 182 cancer patients compared to only 18 control subjects (20), while the second most had 122 cancer patients compared to 18 controls (21). In the more extreme cases, this study design may have negative implications for the statistical strength of these studies, decreasing their generalizability, and increasing the risk of bias influencing the conclusions of these papers. Moreover, most studies utilized hospitalized subjects with well-confirmed cancer diagnoses and at advanced stages, a consequence of which potentially being that their results may not necessarily translate into populations with earlier stage cancers, as the impact of cancer on the metabolome can evolve significantly throughout different stages of the disease (22).
Table 3. Sample sizes and published subject descriptions for urinary cancer voc studies published from 2020 to 2023.
More concerningly however, is the tendency for authors to select cancer and non-cancer test groups with a substantial difference in age, with many studies detailing a cancerous population ten or more years senior compared to their control group. Three studies in particular, detail sample and control populations with more than two decades separating the average ages of the sampling groups (22–24). While these differences should be considered a major confounding factor for these studies, it also does make sense that these studies likely face difficulties in recruiting younger cancer patients, given that the risk of developing cancers generally increases with age (25). Overall, however while some risk factors exist in the sampling strategies of the analyzed studies, many of these issues are simply a consequence of these studies being preliminary in nature, and presumably larger samples sizes of a few thousand individuals will eventually be conducted in the future to validate these initial findings (26).
Finally, in terms of the types of control populations used, roughly one half of studies employed subjects described as either ‘healthy or normal’, 45% specified that they utilized subjects with confirmed negative cancer diagnoses, 26% described their controls as ‘patients’, typically drawn from the non-cancerous patient population of a hospital or clinic, while a mere 10% of studies specified that they utilized non-cancerous subjects experiencing symptoms relevant to a particular type of cancer. This last category should be considered particularly important when comparing results, as a mature VOC-based diagnostic test would presumably be most useful if it could be applied on patients experiencing newly acquired or minor symptoms characteristic of a particular type of cancer, but which had yet to receive more thorough testing. For example, in one study, the researchers choose to include two control sets, one of which was comprised of healthy subjects, while the other were experiencing another, non-cancer disease (27). On the other hand, two groups sought to address this issue by using sets of patients recommended to undergo a conventional cancer test, presumably due to their experience of cancer-related symptoms (28, 29). Those patients which subsequently tested negative for cancer were then selected as the control population.
The reviewed studies explored a range of experimental strategies, including differences in detection platforms and sample handling techniques, for the detection of VOCs related to cancer. Some of these methodological differences could be expected to considerably impact on the accuracy and consistency a developed cancer detection model can achieve. Table 4 outlines the VOC detection platforms, sample handling techniques, and diagnostic accuracies employed by the reviewed studies.
Regarding detection platforms, mass spectrometry (MS), used in 24 of the reviewed studies, was by far the most popular, and was frequently combined with gas chromatography or used in conjunction with other methods. MS functions by ionizing compounds and then separating the resulting fragments according to their mass to charge ratio, as a result such techniques can identify thousands of individual VOCs within sample, and even provide some information regarding their concentration (30). Nuclear magnetic resonance spectroscopy (NMR) is another platform capable of identifying specific VOCs and was utilized in a single paper in this review. This method employs strong magnetic fields to probe the local magnetic fields of samples in order analyze molecular structures. NMR techniques in turn have the advantage of relatively simple sample preparation, allowing for non-destructive analyses of compounds, and enabling the absolute identification of individual VOC concentrations. On the other hand NMR can be less sensitive compared to MS, though the development of new probes for NMR has begun to address this issue (31, 32). However, both MS and particularly NMR systems come with high initial equipment and operating costs. The fact that despite these high costs most of the studies in this review still chose to employ some variety of MS may indicate that researchers are currently more interested in identifying specific VOCs that occur in cancer patients which can be targeted in future studies. Alternatively, MS platforms may also simply be relatively available to researchers, given that they are commonly installed in universities and research institutions, where they can be leased as needed for a particular project.
Ion-mobility spectrometry (IMS), a method related to MS, was employed by six studies and instead separates ion fragments based on their mobility through a carrier gas in an electrical field. These techniques are often characterized by higher speeds than MS but may not provide as high a resolution in exchange (27). Flame ionization detection (FID) in turn is a lower cost system relative to MS and IMS, which similarly seeks to measure generated ions but instead uses a flame to burn initial compounds. Only a single study in this review employed this technique, possibly because such systems are less suitable for identifying specific VOCs when used alone (11).
However, while identifying specific VOCs associated with cancer patients is useful, it is not strictly necessary – only that cancer related VOCs, or patterns thereof can be detected. Animal olfaction operates on this principle, with animals being trained to recognize a particular smell in an initial training period, and then responding when it encounters an identical or similar smell in the future. Animal olfaction was the second major group of VOC detection platforms observed in this review, with 10 studies employing one or more animals to recognize and respond to VOC signatures from a sample, with dogs being the most popular animal followed by nematodes and then rats. Importantly, many animals can detect very low concentrations of certain VOCs – which may in some cases exceed the accuracies of MS platforms. For instance, Tert-butyl mercaptan is detectable around 0.3 parts per billion by the human nose (33), while dogs exhibit even lower levels of detection at concentration of one part per trillion (34), levels of detection comparable to those for MS platforms (35). However, these methods can yield data which may be more difficult to objectively quantify, and which may be less consistent overall due to changes in the animal’s attention, mood and physical health over time or between different animals (20, 34). Furthermore, the animals in question must be trained to recognize and respond to VOCs of interest correctly, a process that takes time, and which is not guaranteed to ultimately succeed (23). Finally, the use of vertebrate and “higher animals” in scientific research necessitates the raising of important questions and considerations related to the ethical treatment of these creatures, potentially complicating their use as diagnostic tools (36).
Sensor-based technologies, often described as ‘electronic noses’ (eNose), constituted the third major group of VOC detection platforms. As their name implies, these technologies seek to mimic animal olfaction using individual, or arrays of sensors, that measure the physical or chemical properties of a gas, such as electrical conductivity or resistance (16, 21). Notably, eNose technologies address some of the potential limitations of animal olfaction, as eNose responses can be expected to be fully consistent between measurements so long as the equipment is not degraded, and responses can be more easily quantified for analytical purposes.
Finally, two studies in this review employed light-based fluorescence sensor arrays which very generally used the detection of specific wavelengths of light to detect and quantify specific VOC compounds in a particular sample (37, 38). These methods are relatively inexpensive compared to MS-based methods and can provide a way to semi-quantify compatible VOCs. However, these methods may also be somewhat more limited in terms of only being suitable for specific VOC’s compatible with the specific method used, as well as potentially requiring the addition of reagents to facilitate measurement, potentially explaining their relative rarity in this review (25, 26).
Aside from detection platforms, sample handling techniques should also be considered important when developing a VOC-based model of cancer detection. For instance, several detection platforms, such as MS, typically require concentrating a sample prior to analysis (39). One technique used by many of the reviewed studies to achieve this concentration is known as headspace sampling, wherein VOCs are collected from the gas phase above a sample in a sealed container. This method in turn is also frequently paired with a technique known as solid phase microextraction (SPME), which uses a small fiber coated with a sorbent material to absorb the VOCs in the headspace of a sample, before being transferred to an analytical system where it can then be heated to release collected compounds. Importantly SPME has the advantage of being low-cost, simple, and not requiring solvents or highly specialized equipment (40). As a result, it was unsurprising that many of the studies employing GC-MS utilized SPME alongside it. However, it should be stated that SPME does impose an upper limit on the concentration of VOCs that can be collected at one time on the fiber, and moreover different compounds may attach to the fiber at different rates – causing there to be no guarantee that SPME will extract a representative set of sample VOCs (21, 22, 40).
Thermal desorption was another method used for concentrating sample VOCs. In these techniques samples are heated in an airtight tube, and a carrier gas transports VOCs to the analytical instrument. This method addresses some of the limitations of SPME, as thermal desorption can allow for the collection of very low concentration VOCs, as well as allowing for the capture of semi-volatile compounds, and providing quantitative or near-quantitative desorption of VOCs (39, 41). However, this method does require specialized equipment and can be somewhat more complex in comparison to SPME, potentially explaining its relative rarity in the reviewed studies. While both SPME and thermal desorption enhance VOC concentration, there were scenarios in the reviewed literature where direct exposure of the samples evidentially was sufficient, as was the case for many of the animal-based detection systems in this review. In these cases, samples are simply placed near a detector for the VOCs to passively diffuse into. This allows for rapid and straightforward collection of VOCs but may suffer from reproducibility issues due to changing environmental factors such as temperature and humidity variations (8).
Many authors did not include a high level of detail on the specific methods used for urine sample collection. This is understandable, as urine testing can be as simple as providing a container to a patient and requesting that they fill part of it. However, there were exceptions to this trend. For instance, some groups specified that only early morning urine specifically was collected (16, 42, 43). This type of sampling is preferable in many instances as overnight the body is typically in a state of low hydration, leading to more concentrated urine and therefore potentially resulting in more and more concentrated VOCs in the sample. Moreover, since diet, hydration and physical activity levels throughout the day can influence urine composition, collecting early morning urine specifically may produce a more consistent baseline between different individual. Some authors further enhanced this approach by also ensuring that the collection of early-morning urine samples was preceded by an overnight fasting period (14, 44).
Other studies specified that mid-stream urine was collected (27, 42). This technique can help to reduce contamination from debris such as cells, or bacteria in the urethra, enhancing sample reliability. In a related approach one group obtained samples from catheterized patients, and then divided their urine samples into different fractions depending on time of excretion (16). While this approach could offer further control regarding contamination, it is likely infeasible for most patients due to its highly invasive nature.
Storage conditions of urine samples was another component of studies that was found to vary between studies. In particular, some researchers advocated for -80°C storage of urine samples as being necessary to preserve VOC signatures (45), while others contended that -20°C was sufficient (46), one group even opted not to freeze their samples at all, storing their samples at 4°C, and another group opted to avoid storage altogether by immediately running their samples following collection (9). Several studies also experimented with modifying their urine samples to enhance VOC release. For instance, many of the reviewed methods heated the urine samples when analyzing them, with temperatures ranging from 37°C to well beyond the boiling point of water. This process increases the kinetic energy of VOCs leading to faster release of VOCs from a sample and can result in a more concentrated headspace above the sample. However certain VOCs may also degrade or undergo chemical transformation, potentially altering their composition or reducing the number of detectable VOCs. Similarly, many studies sought to modify the pH or salinity of their samples, the former of which may stabilize or destabilize certain VOCs, while increasing salinity can alter the solubility of some compounds – driving or pulling them away from the headspace (45, 47, 48). In a similar vein, guanidine hydrochloride was used by several studies in order to denature proteins and facilitate VOC release (47, 49), while O-(2,3,4,5,6-pentafluorobenzyl)hydroxylamine hydrochloride (PFBHA) (43, 50, 51) has been used to derivatize carbonyl compounds and thereby make them more detectable in subsequent analyses.
The final step in developing a VOC-based cancer detection model is evaluating its diagnostic accuracy. To do this, authors usually seek to describe two statistical properties of the test, sensitivity, and specificity. The former property refers to the ability of a test to correctly identify individuals who have the disease in question, and as such can also be described as the “true-positive” rate. Specificity in turn is used to describe the ability of a test to correctly identify individuals who do not have the disease being tested for, or the true negative rate. Both sensitivity and specificity are usually described as a percentage of the surveyed population. As perfect sensitivity and specificity is usually incredibly difficult to achieve, a general rule of thumb is that if the two accuracy scores sum together for more than 150%, then a given test possesses at least some diagnostic value (52).
Interestingly, according to Table 4, most of the studies in this review achieved this benchmark, indicating that the models developed by these studies could plausibly be used to diagnose cancer based on VOC signatures. Moreover, no obvious trends exist between the diagnostic power of all the different platforms analyzed, though eNose-based systems may be seen to have a slightly higher accuracy overall, while animal and GC-MS based systems appear to display larger variances in their effectiveness when comparing study to study. In addition, the significance of storage was also unclear with respect to these values as samples stored at -80°C, generally did not appear to result in increased accuracy of the produced model when compared to -20°C storage, and even the one study which used 4°C still achieved a combined sensitivity and specificity of 150% or higher with their models. Moreover, the value of immediate analyses is also somewhat unclear as one group which opted to avoid long term storage still achieved a relatively unremarkable combined sensitivity and specificity of 169% (9). It may be notable that one study, which required patients to undergo an 8-hour fast followed by early morning urine collection obtained a relatively high combined sensitivity and specificity value of 192% (44), though another study following similar protocols only achieved a combined value of 161.7% (14). Differences in VOC extraction methods similarly did not appear to have a clear effect on model accuracies, though it is potentially noteworthy that two studies which used guanidine hydrochloride both achieved combined sensitivity and specificities approaching 200% (47, 49). However, it is important to note that it may be difficult to draw any concrete conclusions from a direction comparison of the accuracy scores of the various articles, given that different studies had very different sets of goals and operated using diverse sets of samples.
As part of this review, a table listing specific VOCs significantly identified with cancer by the reviewed authors was compiled in Supplementary Table S1, which includes 329 separate entries and 252 unique chemical compounds. Within Supplementary Table S1, the relative amount of VOC entries per cancer type was also determined and further summarized into Figure 1. Notably the most common associations were with urinary bladder cancer (33% of database), breast cancer (22% of database), prostate cancer (18%) and cervical cancer (16%), while entries related to lung cancer, renal cancer or pancreatic cancers together occupied only 11% of the database, possibly indicating that these latter cancers may produce fewer or less useful characteristic urinary volatiles, or else the research for these types is still simply at a less advanced stage. Following this, one or more descriptors were then assigned to each of these compounds included in Supplementary Table S1 based on their molecular structure – a summary of which can be viewed in Figure 2. From this figure it is apparent that the most common chemical structures included in this list are ketones (15%), aromatic compounds (13%), alcohols, aldehydes, cyclic, and polycyclic compounds (~8% each), and phenols and ethers (~5% each). From Supplementary Table S1, every compound identified by two or more distinct studies, 47 chemical species in total, were then refined into Table 5 for further discussion.
Figure 1. Relative proportion of cancer types in Supplementary Table S1.
Unsurprisingly, this secondary list once again included many compounds with one or more ketone groups. For instance, propan-2-one or 2-propanone was identified both as a biomarker of lung cancer (53), as well as a biomarker of newly developed bladder cancer (45), with the former authors attributing its production to altered fatty acid oxidation in cancer cells. Similarly, butan-2-one was found exclusively in the lung cancer population of one study (54) and was also found to be significant marker in breast cancer patients (49). In contrast however, another group observed that this metabolite was downregulated in bladder cancer, seemingly indicating a complex relationship with different types of cancers (51). Other ketones of note include pentan-2-one, which was observed to increase in cancer patients in studies of pancreatic ductal adenocarcinoma (27), bladder cancer (42), and lung cancer (55). However, two of these authors (42, 55) went on to clarify that this metabolite was also associated with several other diseases including Crohn’s disease, inflammatory bowel disease and diabetes which may impair the usefulness of this marker in a hypothetical diagnostic method. The most cited biomarker between all studies however was heptan-(2 or 4)-one, which was identified as significant by a total of seven studies, including two where it was found downregulated (42, 51), as well as two where it was found upregulated (45, 54). Another potential explanation for this relationship was proposed by one group, in which the authors proposed that elevate levels of methyl-ketones the feces of lung cancer patients was caused by a disrupted microbiome metabolism brought on by the disease (56).
Overall, it appears to be typical for these biomarkers to show both up- and down- regulation in different cancers as reported by different authors. Some exceptions to this trend however exist within 5-ethyl-3-methyloxolan-2-one, which was found to increase roughly 2-fold in both lung cancer (53), and bladder cancer (45). Carvone, in turn was found downregulated in studies of bladder cancer (51), and prostate cancer (22), while 1,1,4a-trimethyl-4,5,6,7-tetrahydro-3h-naphthalene-2-one decreased in expression between 0.388- and 2.715-fold in studies of bladder cancer (45), and lung cancer (53). In general, the various authors did not attempt to definitively identify why these biomarkers were capable of diagnosing cancer cases, with most authors simply proposing possibly broad metabolic mechanisms which could have generated a given compound.
Various aldehydes also demonstrated consistent associations with cancer. For example, hexanal was found to be significant in four separate articles for both bladder (37, 38, 51), and prostate cancer (43). One group further went on to attribute their observed decrease in this metabolite to the oxidation of aldehyde dehydrogenase in cancer cells, or increased lipid peroxidation (51). Different conformations of dimethyl benzaldehyde were also found to be significant by several authors and in varying concentrations across cancer types, for bladder cancer (42), prostate cancer (22, 43), and breast cancer patient (14). In one of these studies, a particularly striking increase in concentration for this metabolite was observed, increasing between 30.5- and 251.1-fold for bladder cancer patients, with the authors hypothesizing that these marked differences owed to significantly altered fatty acid metabolism in cancer cells (42). However, another study observed a decrease of this metabolite in their breast cancer population, and attributed this change to modified activity of the cytochrome p450, or an impairment of the oxidation phosphorylation process in the breast cancer cells (14). Another metabolite implicated in multiple cancer studies was nonanal, for pancreatic ductal adenocarcinoma (27), and bladder cancer (45, 57). Interestingly, several different explanations were proposed regarding the nature of this biomarker, with one group speculating that it could have arisen from the reduction of the carboxy group of nonanoic acid and proposing that it may be a non-specific marker of inflammation or general illness and not cancer per se (27). Another theorized that this relationship between nonanal and cancer, which had already been observed in previous studies, could be the result of a dysregulation of an enzyme related to oxidative metabolism which itself is commonly associated with tumor initiation and promotion (57). However, this explanation may not wholly explain things, as one group instead observed a 0.306-fold decrease in nonanal (45). However, while discussing this result, these authors would later conclude that direct comparisons of this result with previous studies was difficult due to differences in sample preparation methodologies.
In terms of alcohols, the most referenced chemical species was 2-ethylhexan-1-ol, however different studies have observed different relationships between this metabolite and cancer. For instance, in two separate studies focused on bladder cancer (45) and prostate cancer (58), the authors observed a significant decrease in this metabolite relative to healthy controls, with one of the groups further speculating that this VOC could play a role in cancer progression and cell apoptosis (58). On the other hand, two more studies both focusing on prostate cancer instead observed that 2-ethylhexan-1-ol was more correlated with their cancer groups and not healthy individuals (22, 57). Previously, two group also proposed that upregulation of this metabolite could be attributed directly to the metabolic activity of lung cancer cells (55, 59). Another alcohol, 2,6-dimethyloct-7-en-2-ol, or dihydromyrcenol, was identified as an important biomarker for bladder cancer, though the authors did not indicate if this was due to being up or down-regulated in patients (45). Two more studies also found this metabolite to also be a significant marker in prostate cancer patients (22, 46), with one of them further observing an upregulation of this metabolite relative to controls and speculating that this may have been caused by cancer cells utilizing this metabolite as an energy source (46).
Interestingly increased expression of phenol and 2-methoxyphenol, appeared to be a consistent feature in bladder cancer (42, 45), and was also identified as significant in prostate cancer patients (22, 57). One final metabolite of note 4-methylpent-3-enoic acid was also found to increase in bladder cancer patients in the two studies which identified it, with one group observing a 1.457-fold increase (45), while the other only observed this metabolite in the cancer group (42).
In this review, the number and distribution of studies related to the identification of urinary VOCs associated with different types of cancer, and their capacity to diagnose incidents of cancer was reviewed. It was noted that in the past five years, approximately six articles per year have been produced on this topic, with prostate, lung, bladder, and breast cancers being among the most extensively studied while pancreatic and colorectal cancer appears to have received less attention. It was further noted that most of the studies analyzed used human patients, with relatively small sample sizes. One major point of diversity among the studies was how control populations were selected, which may have implications for the potential usefulness of a VOC-based cancer test. A relatively large number of studies were noted to have either targeted multiple types, or grades of, cancer simultaneously and attempted to distinguish between them using characteristic VOC signatures. Regarding sample handling methods, neither VOC detection platform nor storage conditions appeared to have a clear influence on the accuracy of the generated models, however comparisons between studies with different objectives is difficult. In addition, many studies did not specify how urine samples were collected, despite there being some advantages associated with specific sampling techniques. Finally, some common VOCs associated with cancer identified in different studies were briefly highlighted. Overall, it was noted that while VOC-based testing for cancer appears to be upheld by the literature as a promising strategy for improving early cancer detection, more work may be needed before widespread adoption of these techniques will occur.
With the discovery of genomic and immunological biomarkers associated with several types of cancer and drugs to target these biomarkers, cancer is transitioning from an incurable disease to a chronic disease. VOCs may provide biomarkers for cancer screening, targets for drug development, and prognostics or pre-clinical indicators of cancer recurrence.
The datasets used for this study have been included in this Review and in the Supplementary Table.
AG: Writing – original draft, Writing – review & editing. BK: Supervision, Writing – review & editing. NA: Conceptualization, Supervision, Writing – review & editing. MA: Conceptualization, Investigation, Methodology, Project administration, Resources, Software, Supervision, Validation, Visualization, Writing – review & editing.
The author(s) declare that no financial support was received for the research, authorship, and/or publication of this article.
The authors declare that the research was conducted in the absence of any commercial or financial relationships that could be construed as a potential conflict of interest.
The author(s) declared that they were an editorial board member of Frontiers, at the time of submission. This had no impact on the peer review process and the final decision.
All claims expressed in this article are solely those of the authors and do not necessarily represent those of their affiliated organizations, or those of the publisher, the editors and the reviewers. Any product that may be evaluated in this article, or claim that may be made by its manufacturer, is not guaranteed or endorsed by the publisher.
The Supplementary Material for this article can be found online at: https://www.frontiersin.org/articles/10.3389/fonc.2024.1448760/full#supplementary-material
Supplementary Table S1 | Complete list of volatile organic compounds significantly linked with cancer.
1. Guerra CE, Sharma PV, Castillo BS. Multi-cancer early detection: the new frontier in cancer early detection. Annu Rev Med. (2024) 75:67–81. doi: 10.1146/annurev-med-050522-033624
2. Ferlay J, Colombet M, Soerjomataram I, Parkin DM, Piñeros M, Znaor A, et al. Cancer statistics for the year 2020: An overview. Int J Cancer. (2021) 149:778–89. doi: 10.1002/ijc.v149.4
3. Ellison LF, Saint-Jacques N. Five-year cancer survival by stage at diagnosis in Canada. Health Rep. (2023) 34:3–15. 10.25318/82-003-x202300100001-eng
4. SEER*Explorer. An Interactive website for SEER cancer statistics (2024). Available online at: https://seer.cancer.gov/statistics-network/explorer/. (Accessed August 01, 2024).
5. Sarhadi VK, Armengol G. Molecular biomarkers in cancer. Biomolecules. (2022) 12:1021. doi: 10.3390/biom12081021
6. Keogh RJ, Riches JC. The use of breath analysis in the management of lung cancer: is it ready for primetime? Curr Oncol Tor Ont. (2022) 29:7355–78. doi: 10.3390/curroncol29100578
7. Einoch Amor R, Nakhleh MK, Barash O, Haick H. Breath analysis of cancer in the present and the future. Eur Respir Rev Off J Eur Respir Soc. (2019) 28:190002. doi: 10.1183/16000617.0002-2019
8. Feil C, Staib F, Berger MR, Stein T, Schmidtmann I, Forster A, et al. Sniffer dogs can identify lung cancer patients from breath and urine samples. BMC Cancer. (2021) 21:917. doi: 10.1186/s12885-021-08651-5
9. Costantini M, Filianoti A, Anceschi U, Bove AM, Brassetti A, Ferriero M, et al. Human urinary volatilome analysis in renal cancer by electronic nose. Biosensors. (2023) 13:427. doi: 10.3390/bios13040427
10. Amundsen T, Sundstrøm S, Buvik T, Gederaas OA, Haaverstad R. Can dogs smell lung cancer? First study using exhaled breath and urine screening in unselected patients with suspected lung cancer. Acta Oncol Stockh Swed. (2014) 53:307–15. doi: 10.3109/0284186X.2013.819996
11. da Costa BRB, da Silva RR, Bigão VLCP, Peria FM, De Martinis BS. Hybrid volatilomics in cancer diagnosis by HS-GC-FID fingerprinting. J Breath Res. (2023) 17:026002. doi: 10.1088/1752-7163/acb284
12. Kim KH, Jahan SA, Kabir E. A review of breath analysis for diagnosis of human health. TrAC Trends Anal Chem. (2012) 33:1–8. doi: 10.1016/j.trac.2011.09.013
13. Kischkel S, Miekisch W, Sawacki A, Straker EM, Trefz P, Amann A, et al. Breath biomarkers for lung cancer detection and assessment of smoking related effects–confounding variables, influence of normalization and statistical algorithms. Clin Chim Acta Int J Clin Chem. (2010) 411:1637–44. doi: 10.1016/j.cca.2010.06.005
14. Li X, Wen X, Luo Z, Tian Y, Qian C, Zhang J, et al. Development of a headspace-solid phase microextraction gas chromatography-high resolution mass spectrometry method for analyzing volatile organic compounds in urine: Application in breast cancer biomarker discovery. Clin Chim Acta Int J Clin Chem. (2023) 540:117236. doi: 10.1016/j.cca.2023.117236
15. Woollam M, Wang L, Grocki P, Liu S, Siegel AP, Kalra M, et al. Tracking the progression of triple negative mammary tumors over time by chemometric analysis of urinary volatile organic compounds. Cancers. (2021) 13:1462. doi: 10.3390/cancers13061462
16. Capelli L, Bax C, Grizzi F, Taverna G. Optimization of training and measurement protocol for eNose analysis of urine headspace aimed at prostate cancer diagnosis. Sci Rep. (2021) 11:20898. doi: 10.1038/s41598-021-00033-y
17. Lawler M, Alsina D, Adams RA, Anderson AS, Brown G, Fearnhead NS, et al. Critical research gaps and recommendations to inform research prioritisation for more effective prevention and improved outcomes in colorectal cancer. Gut. (2018) 67:179–93. doi: 10.1136/gutjnl-2017-315333
18. Nissinen SI, Roine A, Hokkinen L, Karjalainen M, Venäläinen M, Helminen H, et al. Detection of pancreatic cancer by urine volatile organic compound analysis. Anticancer Res. (2019) 39:73–9. doi: 10.21873/anticanres.13081
19. Arasaradnam RP, Wicaksono A, O’Brien H, Kocher HM, Covington JA, Crnogorac-Jurcevic T. Noninvasive diagnosis of pancreatic cancer through detection of volatile organic compounds in urine. Gastroenterol. (2018) 154(3):485–7. doi: 10.1053/j.gastro.2017.09.054
20. Kure S, Iida S, Yamada M, Takei H, Yamashita N, Sato Y, et al. Breast cancer detection from a urine sample by dog sniffing: A preliminary study for the development of a new screening device, and a literature review. Biology. (2021) 10:517. doi: 10.3390/biology10060517
21. Bannaga AS, Kvasnik F, Persaud K, Arasaradnam RP. Differentiating cancer types using a urine test for volatile organic compounds. J Breath Res. (2020) 15:017102. doi: 10.1088/1752-7163/abc36b
22. Riccio G, Berenguer CV, Perestrelo R, Pereira F, Berenguer P, Ornelas CP, et al. Differences in the volatilomic urinary biosignature of prostate cancer patients as a feasibility study for the detection of potential biomarkers. Curr Oncol Tor Ont. (2023) 30:4904–21. doi: 10.3390/curroncol30050370
23. Liu SF, Lu HI, Chi WL, Liu GH, Kuo HC. Sniffer dogs diagnose lung cancer by recognition of exhaled gases: using breathing target samples to train dogs has a higher diagnostic rate than using lung cancer tissue samples or urine samples. Cancers. (2023) 15:1234. doi: 10.3390/cancers15041234
24. Giró Benet J, Seo M, Khine M, Gumà Padró J, Pardo Martnez A, Kurdahi F. Breast cancer detection by analyzing the volatile organic compound (VOC) signature in human urine. Sci Rep. (2022) 12:14873. doi: 10.1038/s41598-022-17795-8
25. White MC, Holman DM, Boehm JE, Peipins LA, Grossman M, Henley SJ. Age and cancer risk: a potentially modifiable relationship. Am J Prev Med. (2014) 46:S7–15. doi: 10.1016/j.amepre.2013.10.029
26. Pourhoseingholi MA, Vahedi M, Rahimzadeh M. Sample size calculation in medical studies. Gastroenterol Hepatol Bed Bench. (2013) 6:14–7.
27. Daulton E, Wicaksono AN, Tiele A, Kocher HM, Debernardi S, Crnogorac-Jurcevic T, et al. Volatile organic compounds (VOCs) for the non-invasive detection of pancreatic cancer from urine. Talanta. (2021) 221:121604. doi: 10.1016/j.talanta.2020.121604
28. Thompson M, Sarabia Feria N, Yoshioka A, Tu E, Civitci F, Estes S, et al. A Caenorhabditis elegans behavioral assay distinguishes early stage prostate cancer patient urine from controls. Biol Open. (2021) 10:bio057398. doi: 10.1242/bio.057398
29. Guest C, Harris R, Sfanos KS, Shrestha E, Partin AW, Trock B, et al. Feasibility of integrating canine olfaction with chemical and microbial profiling of urine to detect lethal prostate cancer. PloS One. (2021) 16:e0245530. doi: 10.1371/journal.pone.0245530
30. Snow NH. Chapter 14 Gas chromatography. In: Ahuja S, Jespersen N, editors. Modern instrumental analysis, vol. 47. (Besloten Vennootschap: Elsevier) (2006). p. 443–83. Available at: https://www.sciencedirect.com/science/article/pii/S0166526X06470148. Comprehensive Analytical Chemistry.
31. Ahmed N, Kidane B, Wang L, Qing G, Tan L, Buduhan G, et al. Non-invasive exploration of metabolic profile of lung cancer with Magnetic Resonance Spectroscopy and Mass Spectrometry. Contemp Clin Trials Commun. (2019) 16:100445. doi: 10.1016/j.conctc.2019.100445
32. Gopinath T, Shin K, Tian Y, Im W, Struppe J, Perrone B, et al. Solid-state NMR MAS CryoProbe enables structural studies of human blood protein vitronectin bound to hydroxyapatite. J Struct Biol. (2024) 216:108061. doi: 10.1016/j.jsb.2024.108061
33. Michanowicz DR, Leventhal OM, Domen JK, Williams SR, Lebel ED, Hill LAL, et al. Natural gas odorants: A scoping review of health effects. Curr Environ Health Rep. (2023) 10:337–52. doi: 10.1007/s40572-023-00403-w
34. Kokocińska-Kusiak A, Woszczyło M, Zybala M, Maciocha J, Barłowska K, Dzięcioł M. Canine olfaction: physiology, behavior, and possibilities for practical applications. Anim Open Access J MDPI. (2021) 11:2463. doi: 10.3390/ani11082463
35. Snow NH. Flying high with sensitivity and selectivity: GC–MS to GC–MS/MS. LC-GC N Am. (2021) 39:61. doi: 10.56530/lcgc.na
36. Kiani AK, Pheby D, Henehan G, Brown R, Sieving P, Sykora P, et al. Ethical considerations regarding animal experimentation. J Prev Med Hyg. (2022) 63:E255–66. doi: 10.15167/2421-4248/jpmh2022.63.2S3.2768
37. Zhu S, Corsetti S, Wang Q, Li C, Huang Z, Nabi G. Optical sensory arrays for the detection of urinary bladder cancer-related volatile organic compounds. J Biophotonics. (2019) 12:e201800165. doi: 10.1002/jbio.201800165
38. Bhattacharyya N, Mukherjee D, Singh S, Ghosh R, Karmakar S, Mallick A, et al. Seeing” invisible volatile organic compound (VOC) marker of urinary bladder cancer: A development from bench to bedside prototype spectroscopic device. Biosens Bioelectron. (2022) 218:114764. doi: 10.1016/j.bios.2022.114764
39. Woolfenden E. Chapter 10 - Thermal desorption gas chromatography. In: Poole CF, editor. Gas chromatography (Second edition), 2nd ed. Elsevier, Amsterdam (2021). p. 267–323. Available at: https://www.sciencedirect.com/science/article/pii/B9780128206751000095. Handbooks in Separation Science.
40. Nolvachai Y, Amaral MSS, Herron R, Marriott PJ. Solid phase microextraction for quantitative analysis – Expectations beyond design? Green Anal Chem. (2023) 4:100048. doi: 10.1016/j.greeac.2022.100048
41. Gao Q, Su X, Annabi MH, Schreiter BR, Prince T, Ackerman A, et al. Application of urinary volatile organic compounds (VOCs) for the diagnosis of prostate cancer. Clin Genitourin Cancer. (2019) 17:183–90. doi: 10.1016/j.clgc.2019.02.003
42. Ligor T, Adamczyk P, Kowalkowski T, Ratiu IA, Wenda-Piesik A, Buszewski B. Analysis of VOCs in urine samples directed towards of bladder cancer detection. Mol Basel Switz. (2022) 27:5023. doi: 10.3390/molecules27155023
43. Lima AR, Pinto J, Carvalho-Maia C, Jerónimo C, Henrique R, Bastos M de L, et al. A panel of urinary volatile biomarkers for differential diagnosis of prostate cancer from other urological cancers. Cancers. (2020) 12:2017. doi: 10.3390/cancers12082017
44. Díaz de León-Martínez L, Flores-Ramírez R, López-Mendoza CM, Rodríguez-Aguilar M, Metha G, Zúñiga-Martínez L, et al. Identification of volatile organic compounds in the urine of patients with cervical cancer. Test concept for timely screening. Clin Chim Acta Int J Clin Chem. (2021) 522:132–40. doi: 10.1016/j.cca.2021.08.014
45. Lett L, George M, Slater R, De Lacy Costello B, Ratcliffe N, García-Fiñana M, et al. Investigation of urinary volatile organic compounds as novel diagnostic and surveillance biomarkers of bladder cancer. Br J Cancer. (2022) 127:329–36. doi: 10.1038/s41416-022-01785-8
46. Khalid T, Aggio R, White P, De Lacy Costello B, Persad R, Al-Kateb H, et al. Urinary volatile organic compounds for the detection of prostate cancer. PloS One. (2015) 10:e0143283. doi: 10.1371/journal.pone.0143283
47. Woollam M, Siegel AP, Munshi A, Liu S, Tholpady S, Gardner T, et al. Canine-inspired chemometric analysis of volatile organic compounds in urine headspace to distinguish prostate cancer in mice and men. Cancers. (2023) 15:1352. doi: 10.3390/cancers15041352
48. Yang M, Jiang J, Hua L, Jiang D, Wang Y, Li D, et al. Rapid detection of volatile organic metabolites in urine by high-pressure photoionization mass spectrometry for breast cancer screening: A pilot study. Metabolites. (2023) 13:870. doi: 10.3390/metabo13070870
49. Grocki P, Woollam M, Wang L, Liu S, Kalra M, Siegel AP, et al. Chemometric analysis of urinary volatile organic compounds to monitor the efficacy of pitavastatin treatments on mammary tumor progression over time. Mol Basel Switz. (2022) 27:4277. doi: 10.3390/molecules27134277
50. Lima AR, Pinto J, Azevedo AI, Barros-Silva D, Jerónimo C, Henrique R, et al. Identification of a biomarker panel for improvement of prostate cancer diagnosis by volatile metabolic profiling of urine. Br J Cancer. (2019) 121:857–68. doi: 10.1038/s41416-019-0585-4
51. Pinto J, Carapito Â, Amaro F, Lima AR, Carvalho-Maia C, Martins MC, et al. Discovery of volatile biomarkers for bladder cancer detection and staging through urine metabolomics. Metabolites. (2021) 11:199. doi: 10.3390/metabo11040199
52. Power M, Fell G, Wright M. Principles for high-quality, high-value testing. Evid Based Med. (2013) 18:5–10. doi: 10.1136/eb-2012-100645
53. Chapman EA, Baker J, Aggarwal P, Hughes DM, Nwosu AC, Boyd MT, et al. GC-MS techniques investigating potential biomarkers of dying in the last weeks with lung cancer. Int J Mol Sci. (2023) 24:1591. doi: 10.3390/ijms24021591
54. Perez-Carrasco V, Soriano-Lerma A, Soriano M, Gutiérrez-Fernández J, Garcia-Salcedo JA. Urinary microbiome: yin and yang of the urinary tract. Front Cell Infect Microbiol. (2021) 11:617002. doi: 10.3389/fcimb.2021.617002
55. Hanai Y, Shimono K, Matsumura K, Vachani A, Albelda S, Yamazaki K, et al. Urinary volatile compounds as biomarkers for lung cancer. Biosci Biotechnol Biochem. (2012) 76:679–84. doi: 10.1271/bbb.110760
56. Botticelli A, Vernocchi P, Marini F, Quagliariello A, Cerbelli B, Reddel S, et al. Gut metabolomics profiling of non-small cell lung cancer (NSCLC) patients under immunotherapy treatment. J Transl Med. (2020) 18:49. doi: 10.1186/s12967-020-02231-0
57. Tyagi H, Daulton E, Bannaga AS, Arasaradnam RP, Covington JA. Urinary volatiles and chemical characterisation for the non-invasive detection of prostate and bladder cancers. Biosensors. (2021) 11:437. doi: 10.3390/bios11110437
58. Liu Q, Fan Y, Zeng S, Zhao Y, Yu L, Zhao L, et al. Volatile organic compounds for early detection of prostate cancer from urine. Heliyon. (2023) 9:e16686. doi: 10.1016/j.heliyon.2023.e16686
59. Pérez Antón A, Ramos ÁG, Del Nogal Sánchez M, Pavón JLP, Cordero BM, Pozas ÁPC. Headspace-programmed temperature vaporization-mass spectrometry for the rapid determination of possible volatile biomarkers of lung cancer in urine. Anal Bioanal Chem. (2016) 408:5239–46. doi: 10.1007/s00216-016-9618-5
60. Einoch Amor R, Levy J, Broza YY, Vangravs R, Rapoport S, Zhang M, et al. Liquid biopsy-based volatile organic compounds from blood and urine and their combined data sets for highly accurate detection of cancer. ACS Sens. (2023) 8:1450–61. doi: 10.1021/acssensors.2c02422
61. Wiesel O, Sung SW, Katz A, Leibowitz R, Bar J, Kamer I, et al. A novel urine test biosensor platform for early lung cancer detection. Biosensors. (2023) 13:627. doi: 10.3390/bios13060627
62. Sato Y, Futamura M, Tanaka Y, Tsuchiya H, Fukada M, Higashi T, et al. Clinical possibility of caenorhabditis elegans as a novel evaluation tool for esophageal cancer patients receiving chemotherapy: A prospective study. Cancers. (2023) 15:3870. doi: 10.3390/cancers15153870
63. Namgong C, Kim JH, Lee MH, Midkiff D. Non-invasive cancer detection in canine urine through Caenorhabditis elegans chemotaxis. Front Vet Sci. (2022) 9:932474. doi: 10.3389/fvets.2022.932474
64. Mozdiak E, Wicaksono AN, Covington JA, Arasaradnam RP. Colorectal cancer and adenoma screening using urinary volatile organic compound (VOC) detection: early results from a single-centre bowel screening population (UK BCSP). Tech Coloproctology. (2019) 23:343–51. doi: 10.1007/s10151-019-01963-6
65. Wang L, Wang Y, Chen A, Teli M, Kondo R, Jalali A, et al. Pitavastatin slows tumor progression and alters urine-derived volatile organic compounds through the mevalonate pathway. FASEB J Off Publ Fed Am Soc Exp Biol. (2019) 33:13710–21. doi: 10.1096/fj.201901388R
66. Ramos ÁG, Antón AP, Sánchez MDN, Pavón JLP, Cordero BM. Urinary volatile fingerprint based on mass spectrometry for the discrimination of patients with lung cancer and controls. Talanta. (2017) 174:158–64. doi: 10.1016/j.talanta.2017.06.003
Keywords: cancer, biomarkers, volatile organic compounds, gas chromatography mass spectrometry, electronic nose, diagnostics, early detection, animal olfaction
Citation: Goertzen A, Kidane B, Ahmed N and Aliani M (2024) Potential urinary volatile organic compounds as screening markers in cancer – a review. Front. Oncol. 14:1448760. doi: 10.3389/fonc.2024.1448760
Received: 13 June 2024; Accepted: 08 November 2024;
Published: 25 November 2024.
Edited by:
Ning Wei, Albert Einstein College of Medicine, United StatesReviewed by:
M. Carmen Martinez-Bisbal, University of Valencia, SpainCopyright © 2024 Goertzen, Kidane, Ahmed and Aliani. This is an open-access article distributed under the terms of the Creative Commons Attribution License (CC BY). The use, distribution or reproduction in other forums is permitted, provided the original author(s) and the copyright owner(s) are credited and that the original publication in this journal is cited, in accordance with accepted academic practice. No use, distribution or reproduction is permitted which does not comply with these terms.
*Correspondence: Michel Aliani, TWljaGVsLkFsaWFuaUBVbWFuaXRvYmEuY2E=
Disclaimer: All claims expressed in this article are solely those of the authors and do not necessarily represent those of their affiliated organizations, or those of the publisher, the editors and the reviewers. Any product that may be evaluated in this article or claim that may be made by its manufacturer is not guaranteed or endorsed by the publisher.
Research integrity at Frontiers
Learn more about the work of our research integrity team to safeguard the quality of each article we publish.